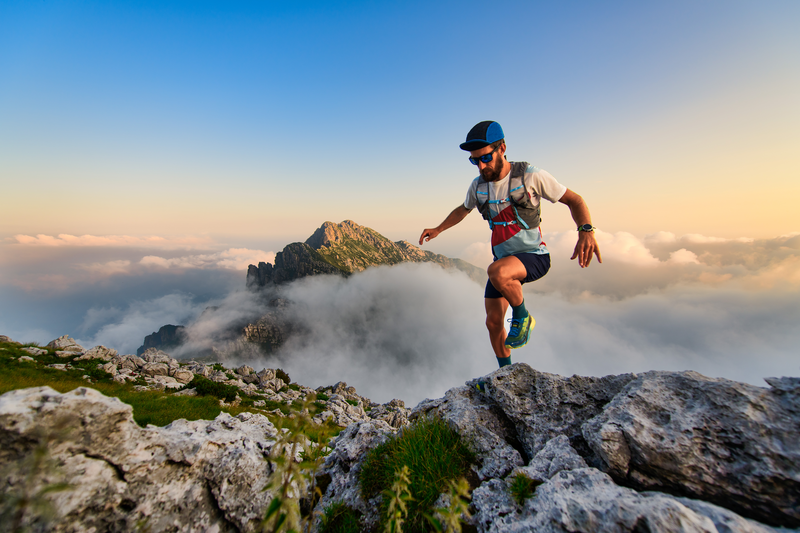
94% of researchers rate our articles as excellent or good
Learn more about the work of our research integrity team to safeguard the quality of each article we publish.
Find out more
REVIEW article
Front. Bioeng. Biotechnol. , 28 November 2024
Sec. Biomaterials
Volume 12 - 2024 | https://doi.org/10.3389/fbioe.2024.1488748
This article is part of the Research Topic Advances in Glycopeptide Hydrogel for Tissue Engineering View all articles
Wound healing is a physiological process occurring after the onset of a skin lesion aiming to reconstruct the dermal barrier between the external environment and the body. Depending on the nature and duration of the healing process, wounds are classified as acute (e.g., trauma, surgical wounds) and chronic (e.g., diabetic ulcers) wounds. The latter, often affect millions of people globally, take months to heal or not heal non-healing chronic wounds, are typically susceptible to microbial infection, and are a major cause of morbidity. Wounds can be treated with a variety of non-surgical (topical formulations, wound dressings) and surgical (debridement, skin grafts/flaps) methods. Three-dimensional (3D)-(bio) printing and traditional wound dressings are two examples of modern experimental techniques. This review focuses on several types of anti-inflammatory wound dressings, especially focusing on hydrogels and traditional macro-fungi like L. rhinocerotis as agents that promote wound healing. In this study, we introduced novel anti-inflammatory hydrogel dressings and offered innovative methods for application and preparation to aid in the healing. Additionally, we summarize the key elements required for wound healing and discuss our analysis of potential future issues. These findings suggest that L. rhinocerotis and various anti-inflammatory hydrogels can be considered as conventional and alternative macro-fungi for the treatment of non-communicable diseases. We summarized the development of functional hydrogel dressings and traditional Lignosus rhinoceros used for wound healing agents in recent years, as well as the current situation and future trends, in light of their preparation mechanisms and functional effects.
The treatment of wounds and the therapeutic challenges they provide have become major concerns in healthcare with a significant financial impact on worldwide (Grainger, 2015; Rani and Ritter, 2016). The skin, one of the most fundamental organs in the body that shields us from the harsh external environment, is frequently injured by traumas, severe burns, ulcers, and other wounds that compromise its ability to act as a protective barrier and play an important role in sensory perception. Moreover, these injuries have a significant financial impact on society and have an adverse effect on the emotional wellbeing of the patient (Dabrowska et al., 2018; Hu et al., 2019). As a consequence, it is imperative to find efficient therapeutic approaches to encourage wound healing.
Wound healing is a dynamic and complex process of tissue regeneration and growth that includes four stages (Maksimova et al., 2014). The first one is the coagulation and hemostasis that begins from the micro vascular bed injury and includes fibrin clot formation, degranulation and aggregation (Solomennikov et al., 2019). As a consequence, this leads to involvement of fibroblasts, macrophages and endothelial cells in wound repair (Solomennikov et al., 2019). The second, inflammatory stage, is accompanied by infiltration of the woundand its surrounding tissue with inflammatory cells—granulocytes (specifically, fragmented nuclei neutrophils) during the 24–48 h after injury and monocytes 48–72 h after injury. Next, lymphocytes migrate to the wound area recruited by IgG and interleukin 1 IL-1). The third stage, proliferation, continues from 3 days to 2 weeks after injury, followed by fibrin scaffold replacement by a newly formed granulation tissue as a result of extracellular matrix components (collagens types I and III, laminin 1, nidogen) and glycosaminoglycan synthesis by fibroblasts as well as auto- and paracrine actions of fibroblasts. The final stage is a remodeling phase, involving the maturation and reconstruction of nascent tissues, is the final stage of wound healing (Su et al., 2021; Naskar and Kim, 2020). The maturation process mainly includes the degradation of excess collagen fibers by collagenase, rearrangement of collagen and regression of overgrown capillaries, which may last for months to years. Ultimately, the granulation tissue formed in wound healing evolves into normal connective tissue (Naskar and Kim, 2020; Okonkwo and DiPietro, 2017). Numerous endogenous and exogenous adverse factors can disrupt the physiological healing processes, among which the inflammatory phase is the most susceptible to interference. Wound tissue produces various proinflammatory cytokines and chemokine’s at the initial step in the inflammatory phase, which results in the infiltration of neutrophils and macrophages at injured sites. Neutrophils are required to remove debris and digest invading bacteria through phagocytosis, releasing caustic proteolytic enzymes and producing free radicals in the process of their cleansing activities. Additional cells present in wound sites include macrophages, which mediate angiogenesis, fibroplasia and extracellular matrix (ECM) production, thereby bridging the inflammatory and proliferative phases importantly; moderate inflammation facilitates the removal of necrotic tissue, kills local bacteria and promotes wound healing (Kasuya and Tokura, 2014).
The use of traditional medicine or natural compounds has been of great interest in the pharmaceutical field for many years including the development of wound healing agents (Chin et al., 2018; Lai et al., 2016). For example, herbal extracts have been investigated for their healing properties and developed as advanced wound dressings (Monirul Islam et al., 2022). Lignosus rhinocerotis is a medicinal mushroom and traditionally used for wound treatment (Lai et al., 2014), by indigenous communities. Its sclerotium holds various medicinal values including anti-microbial and antiviral activities (Eik et al., 2012), making it a good candidate as a natural wound healing agent. Traditionally, people cut the sclerotium of the mushroom into pieces and boil in water prior to its application for medicinal purposes (Chang and Lee, 2004). Consequently, hot water extraction is used to mimic the conventional L. rhinocerotis preparation used for wound healing. Many studies have been carried out to improve the hemostatic effect and quicken the rate at which wounds heal. However, in order to meet additional requirements like infection prevention and easy administration to the affected areas, a new type of wound dressing is still required to improve results (Agarwal et al., 2022).
Hydrogels are generally a series of relatively hydrophilic polymers with the ability to form a three-dimensional cross linked network and preserve a large amount of water. Due to their high water content, hydrogels show similar properties with human body tissue and higher biocompatibility, which makes them suitable for biomedical applications (Zhang et al., 2020). Hydrogels have unique advantages in wound dressings due to their excellent biological, physicochemical, and mechanical properties (Alven and Aderibigbe, 2020), including excellent biocompatibility (Graca et al., 2020), a biological barrier effect blocking harmful factors, such as bacteria, from entering the wound (Norahan et al., 2023), and the ability to deliver cargos that promote wound healing in response to environmental changes (Dimatteo et al., 2018). It is a great concern that antibiotic overuse has led to the development of drug-resistant bacterial strains. Therefore, a variety of antimicrobial hydrogels have recently been synthesized to effectively avoid development of drug resistant bacteria (Farazin et al., 2023). Thus, hydrogels have widespread applications in the field of wound healing, and various functional hydrogels have been developed with adhesive, antioxidant, antibacterial, hemostatic, and tissue regenerative properties (Liang et al., 2021). The great potential of super molecular hydrogels has attracted tremendous interest from researchers and they have been used for numerous biomedical applications in the past decade.
Thermo gels, or thermo-responsive hydrogels, are a subclass of the super molecular hydrogels that gelate via hydrophobic interactions. Thermo gels can undergo a sol–gel phase transition because they are constituted of amphiphilic polymers with both hydrophilic parts and hydrophobic parts (Kim and Matsunaga, 2017). Thermo gels typically refer to thermo-sensitive hydrogels which can form a gel at a higher temperature and return back to a liquid at a lower temperature within a certain temperature range, which is contrary to the conventional melt transition behavior (Dou et al., 2014). This gelation procedure does not need any other assistance or other triggers such as enzymes; thus, it is considered as a benign phase conversion procedure. The lack of toxic crosslinking agents renders it more likely that thermo gels would show intrinsic biocompatibility as an injectable in situ hydrogel (Liow et al., 2016). Considering thermo gel polymers used in biomedical application, two main categories can be identified on the basis of biodegradability: Firstly, non-biodegradable thermo gels, which include (1) polyacrylates and (2) Pluronic. Secondly, biodegradable thermo gels, which include (1) polyesters, (2) polypeptides, and (3) polysaccharides (Lanzalaco and Armelin, 2017). To undergo phase transition from room temperature to body temperature, thermo gels that can undergo gelation within the range of 25°C–37 °C are especially valuable in the biomedical field. Due to phase transition at physiological temperatures, thermo gels have been used in diverse biomedical applications (Patel et al., 2018).
The aim of the present work is to comprehensively review the various types of 3D- (bio) printed hydrogel constructs (e.g., bioactive/antibacterial hydrogels, composite hydrogels, cell-laden hydrogels) that have been used in wound healing applications. The constructs are methodically presented in a tabulated form giving detailed information concerning the selected materials (e.g., sodium alginate, gelatin, peptides, etc.), the crosslinking (e.g., ionic, chemical) and (bio) printing methods (e.g., extrusion, digital light processing, etc.), the type of encapsulated bioactive (e.g., growth factors, antibacterial agents).
This review highlights the parameters for wound healing enhancement Anti-inflammatory hydrogel wound dressings and details the scientific findings of macrofungi traditionally utilized for wound rehabilitation. It subsequently focuses on Lignosus rhinoceros and reporting on its phytochemicals and pharmacological properties which may be beneficial for wound rehabilitation. The prospects on the potential of L. rhinocerus as a wound healing agent are also deliberated as the conclusion to this review.
A hydrogel’s network structure of cross-linked polymer chains is its solid state (Ullah et al., 2015). The hydrogel’s molecular weight is infinite due to its 3- D dimensional network structure. The most important molecular properties that determine the hydrogel structure are the mesh size and the molecular weight of the polymer chain between the crosslinks (Ganji et al., 2010). Crosslinking of hydrogels can be obtained by chemical (covalent) and physical (hydrogen bonding/entanglement) crosslinks. The swelling of a hydrogel is mainly defined by the diffusion of water into the hydrogel (Holback and Yeo, 2011). There are three stages to the hydrogel swelling: 1. Primary bound water: the point at which water molecules connect to the hydrophilic group; 2. Secondary exceed water: the water molecules’ interaction with the hydrophobic groups already in place; and 3. Free water: When the body swells to equilibrium, water fills the empty spaces (Gibas and Janik, 2010). The rate of swelling depends on the concentration of polymer and the crosslinking density (Okay, 2010). The high degree of crosslinking density causes a decrease in swelling ratio and it increases the brittleness of hydrogel.
Hydrogels are classified by source, preparation, ionic charge, responsiveness, crosslinking, and physical properties because of their customizable and repeatable mechanical or physicochemical features. For biomedical applications, a visual representation of this additional classification is given. Its main disadvantage is the lack of biocompatibility with some of the polymers (Ullah et al., 2015).
Poly (ethylene glycol), poly (acrylic acid), poly (ethylene oxide), poly (vinyl alcohol), etc., are some of the synthetic polymers used as hydrogels (Lee and Mooney, 2001). Physically cross-linked injectable PNIPA Am-polyethylene glycol hydrogel improves cellular response, axonal growth and helps in recovering sensory motor function. These fabricated hydrogels could be a suitable candidate for the regeneration of axons (Bonnet et al., 2020). Polyethylene glycol is a biodegradable, biocompatible, and suitable chemical for the healing of spinal cord injuries because it inhibits the degeneration of nerve fibers (Kong et al., 2017). Additionally, a hydrogel composed of polyvinyl alcohol and poly acrylic acid may be an effective biomaterial for vascular access with decreased platelet adherence (Mannarino et al., 2020). Gelatin methacrylate and cellulose derivatives are examples of semi-synthetic polymers (Utech and Boccaccini, 2016). Most hydrogels that combine the benefits of natural and synthetic polymers are semi-synthetic or hybrid hydrogels. The hydrogel exhibits both mechanical and biological activity when it combines natural protein with synthetic polymer material. According to Berkovitch and Seliktar (2017), the hydrogel utilized to encapsulate the anterior root ganglion cells was made with polyethylene glycol and proteins.
Hydrogels have been the material of choice for biomedical applications because of their advantageous physical properties, flexible production methods, mutable structures, and desired biocompatibility. Hydrogels are materials that are permeable and can absorb water up to hundreds of times their dry weight due to their three-dimensional (3D) cross-linked networks. Hydrogels swell in fluids due to thermodynamic force, yet they do not break down (Campbell et al., 2018). The molecular chains that comprise hydrogels are bound together by cross linkers, which can be chemical or physical. There are various categories for hydrogels. For example, the different binding methods of hydrogels allow them to be classified into physical and chemical categories. Physical hydrogels are produced by a combination of physical and ionic interactions. (Dodero et al., 2019), hydrogen bonds (Zhang et al., 2016), entanglement of chains (Yang et al., 2016). In general, physical hydrogels are characterized as reversible when being heated or treated with other stimulations (Stern and Cui, 2019).
In contrast, chemical hydrogels are usually stable due to covalently cross linked networks (Sun et al., 2012). Hydrogels can be classified as natural or synthetic based on where the components came from. Based on their chemical composition, synthetic hydrogels can be broadly divided into five groups: poly (acrylamide), poly (hydroxyl alkyl methacrylates), poly (N-vinyl pyrrolidone), poly (acrylic acid), and poly (vinyl alcohol). Natural hydrogels based on peptides and polysaccharides have been investigated most extensively (Xing et al., 2019). Polysaccharides have abundant and easily obtained from renewable sources, such as (plants and marine organisms) (Kumar et al., 2019). For large-scale production, polysaccharide synthesis is a viable, inexpensive, and simple approach (Gupta et al., 2019). Additionally, polysaccharides’ unique chemical and physical characteristics such as their biocompatibility, biodegradability, and lack of immunological responses—are precisely support their extensive use in biological materials (Yang et al., 2017).
Polysaccharide-based hydrogels have special qualities such a high water retention capacity in addition to the specific qualities of polysaccharides themselves. Polysaccharide-based hydrogels have a flexible structure and network configuration that make them useful for biosensors, wound healing, and diffusion control.
The wound healing mechanism is divided into four phases: (i) hemostasis, (ii) inflammation, (iii) proliferation, and (iv) remodeling. A series of complicated intercellular interactions involving a plethora of soluble factors, intercellular matrix components, immune cells, and signaling molecules occurs during these phases (Komi et al., 2020).
The hemostatic process involves several highly complex biological mechanisms. The small blood vessels and capillaries surrounding the incision first contract an attempt to reduce local blood flow. Subsequently, a variety of physiologically active substances are produced by platelets, including proteases, growth factors, and cytokines, such as TGFβ (transforming growth factor), PDGF (platelet-derived growth factor), IGF, and EGF (epidermal growth factor) (Scopelliti et al., 2022). The development of biofilms at the site of a chronic lesion caused by bacterial invasion may obstruct the proliferation of epidermal and endothelial cells. Life can be in threat in serious cases (Chen et al., 2021).
Wound healing is slowed affected by prolonged inflammation caused by bacterial infection. Therefore, antibiotics are utilized to fight bacterial infections in the wound site and are commonly used as one of the standard treatments for wound care management (Drucker, 2012). Finding an effective treatment is necessary to reduce bacterial colonization and infection, which will subsequently reduce the duration of inflammation and improve the wound healing process (Negut et al., 2018).
Neutrophils and macrophages recruitment into the wound area is an essential event in the inflammatory phase for homeostasis maintenance, fighting pathogen invasion, and dead tissue removal. Previous studies have shown that the number of macrophages that migrate and infiltrate into the wound area is at maximum on day 3 post-wounding and remains until day 7. The number of neutrophils that migrated to the wound site was maximum at 12 h post-wounding and continuously declined until day 3. The inflammatory phase progression can be accelerated by early infiltration and elimination of neutrophils and macrophages, which prevent excessive inflammation that may lead to chronic wound (s) and scar formation (Maeda et al., 2011).
The second phase of wound healing is re epithelialization, a process that involves keratinocyte proliferation and migration to ensure an adequate supply of cells to encase the wound. The process of re-epithelialization which eventually leads to wound closure is one of the parameters for consideration of a healed wound. This re-epithelialization process is flawed in chronic wounds. Growth factors, cytokines, integrin’s, keratins, matrix metalloproteinase, chemokine’s, and extracellular matrix (ECM) play key roles in the regulation of keratinocyte proliferation and modulating wound closure (Pastar et al., 2014). Furthermore, studies have shown that several growth factors participate in angiogenesis, including vascular endothelial growth factor (VEGF), angiopoietin, FGF, and TGFβ, and that the production of a temporary matrix in the granulation tissue is facilitated by the involvement of fibroblasts (Darby et al., 2014). Furthermore, fibroblasts differentiate into α-smooth muscle actin-expressing myo-fibroblasts, which initiate the wound closure process (Zhang et al., 2020). Nevertheless, hyper activation of fibroblasts and myo-fibroblasts is associated with abnormal scar formation (Zhang et al., 2020). Moreover, macrophages are also involved in granulation tissue formation, secreting a variety of growth factors (FGF, TGFβ, EGF and PDGF) (Barrientos et al., 2008). There are significant differences in tissue structure and physiological characteristics between oral mucosa and skin that lead to variations in the healing enhancement (Table 1).
Table 1. The difference in structure, surroundings and healing processes between oral mucosa and skin.
The final stage of healing is remodeling, which is intended to remove unnecessary vessels, fibroblasts, and inflammatory cells (Nourian Dehkordi et al., 2019). Granulation and re-epithelialization are the primary processes involved in wound closure. Granulation tissue is a temporary matrix, nevertheless. Indeed, the shift in the ECM’s composition is a significant aspect of wound remodeling. Consequently, the ECM febrile network’s structure is organized and aligned (Li et al., 2019). Fibroblasts secrete enzymes that degrade the extracellular matrix (ECM), and active protease particularly MMPs control the production of new collagen and the destruction of existing collagen, which plays a role in wound healing (Visse and Nagase, 2003). Subsequently, the prior, transient extracellular matrix (ECM) transforms from a loose fibronectin tissue network to a denser, larger collagen bundle. Type I collagen eventually changes from type III collagen (Finnerty et al., 2016). In addition, collagen alignment tends to remain constant, which strengthens newly formed tissue. Skin appendages that may renew include sweat glands, sebaceous glands, and hair (Feng et al., 2019).
Typical wound treatments comprise surgical (e.g., debridement, skin grafts/flaps), and non-surgical (e.g., topical formulations, skin replacement, wound dressings incorporating or not growth factors, bioactive agents, nanoparticles) methods. Modern approaches include growth factors/cytokine therapy, stem cells (SCs) therapy, vacuum-aided wound closure, and three-dimensional (bio) printed wound dressings. Another approach involves the bioengineering of skin substitutes based on combinations of biomaterials, growth factors and cells (Saifullah and Sharma, 2023).
In wound dressing applications, anti-bacterial and anti-inflammatory hydrogels have a good impact. A multifunctional and pH-responsive composite hydrogel made of carboxylates agarose and tannic acid, which is ironically cross-linked with zinc salts for wound healing (Ninan et al., 2016). The pH-responsive property of carboxylate cellulose combined with anti-microbial, anti-inflammatory, anti-oxidant property of tannic acid showed increased compressive strength and anti-bacterial activity. Dextran is another polysaccharide used for synthesizing hydrogel. It helps in situ gelation and controlled release of immobilized growth factor with the help of chitosan micro particles and showed better wound healing in vivo (Ribeiro et al., 2013). Chitosan is an excellent wound healing material because of its homeostatic nature (Ahmed and Ikram, 2016). A physically cross-linked chitosan hydrogel has the ability to reconstruct the skin of third-degree burn, where they tested in pig dorsal area (Boucard et al., 2007). An injectable hydrogel constructed via disulphide bond cross-linking of thiolated polyethylene glycol and silver nitrate. This hydrogel is loaded with desferrioxamine, an angiogenic drug and this could repair the diabetic wound with its angiogenic activity.
Wound dressings are typically compresses or sterile pads that are applied directly onto the surface of wounds in order to protect them from further injury and promote their healing process.
Required characteristics of wound dressings (Farahani et al., 2021; Yang et al., 2018).
• Absorption of wound exudate;
• Permeability of water vapors;
• Maintenance of a moist wound environment (at the dressing/wound interface), thus
• promoting autolytic debridement and removal of dead tissue;
• No adherence to the wound surface and easy removal without trauma;
• Prevention of microbial (e.g., bacteria, fungi, viruses) transport;
• Enhancement of epidermal migration and promotion of angiogenesis and synthesis of
• connective tissue;
• Exchange of gas between wounded skin tissue and the environment;
• Mechanical strength to protect the body from further injury;
• Biocompatibility/biodegradability and nontoxicity;
• Thermal insulation
Traditional WDs belong to passive dressings and are usually applied to dry and well-cleaned wounds and include gauzes and bandages. Gauze dressings are presented by woven and non-woven cotton fibers, viscose, and polyesters. The main function of these dressings is exudate and fluid absorption from an open wound due to their fibrous structure. For example, Xeroform ™ (non-occlusive dressing) is a petrolatum gauze impregnated with 3% bismuth tribromo phenate and is used to cover dry or exudative mild wounds (Barillo et al., 2017). Bandages are applied as secondary WDs made from natural cotton, wool, cellulose, rayon, polyester, or polyamide. It should be highlighted that cotton bandages could be used for wound cleaning but they shed fibers and stick to the wound surface; they are generally used for dry venous and arterial ulcers. Rayon, polyester and polyamide bandages are non-adherent absorbent secondary dressings that are permeable for liquid and water vapors that do not stick to the wound surface and hence are suitable for granulated wounds with a mild to moderate exudate.
Since traditional WDs cannot provide enough drainage of the wound, they have been replaced by modern dressings, which are characterized by semi-permeability and the presence of a highly absorbent layer. In addition, modern WDs accelerate the formation of granulation tissue and facilitate the migration of epithelial cells from the edges of the wound to its center (Silva et al., 2019). The applicable summaries of wound dressings are presented in Table 2.
Modern WDs are usually semi-occlusive or occlusive and presented mainly by synthetic polymers and divided into interactive, advanced interactive, and bioactive categories (Przekora, 2020). Interactive dressings include semi-permeable films and foams, advanced interactive dressings are presented by hydrocolloids and hydrogels, while tissue-engineered skin equivalents belong to bioactive WDs (Panisi et al., 2021; Assis et al., 2021). Dressings such as Granuflex ™, Comfeel ™, and Tegasorb ™ are available as sheets or thin films (Westby et al., 2017). Hydrocolloids are prescribed for full-size and partial wounds with low to medium exudation, wounds with scab formation and that can remain on the wound surface for up to 7 days (Kamiska et al., 2020).
Biocompatibility is a vital requirement for a hydrogel to maintain tissue homeostasis by presenting a suitable matrix without damaging the local tissue during chronic wound healing. Biodegradability and the biodegradation rate of the hydrogels are other important aspects because they provide temporary template during the proliferation of fibroblasts, re-epithelialization and neovascularization, and remodeling of chronic wounds. Bio-adhesively also plays an important role for the long-term stability of the hydrogel dressings around the wound area, improving the homeostatic effect, keeping the wound moist, and absorbing the tissue exudates during healing. Since the prolonged healing of chronic wounds can increase the risk of infection which adversely affect the healing process, antimicrobial hydrogels can be useful to prevent infections. As indicated in Section (Schultz et al., 2011), the inflammatory phase is the main reason for the delayed healing of chronic wounds. Anti-inflammatory hydrogels can shorten the wound healing period by facilitating the transition from the inflammatory to the proliferation stage. Limited oxygen and nutrient delivery to the wound area is another reason for the delayed chronic wound healing. Pro-angiogenic hydrogels can stimulate angiogenesis to deliver the required nutrients and oxygen to the wound bed to accelerate chronic wound healing. The functionality of hydrogels can be further improved by incorporating various types of drugs or therapeutic agents into the hydrogels. Drug or therapeutic agent releasing hydrogels can provide controlled and sustained delivery in response to the environmental stimuli. Some commercial hydrogel dressings in wound healing are summarized applications of these dressings differ depending on their material components.
Modern dressings are considered the top choice for curing various wound types due to their exceptional biocompatibility/biodegradability, ability to maintain a moist environment and temperature, pain relief, and improvement of a hypoxic environment. Those most commonly used in clinical practice are films, foams, hydrocolloids, alginates and hydrogels (Nguyen et al., 2023). Figure 1 and Table 3 shows examples of commercially available modern dressings.
Figure 1. Different forms hydrogel wound dressing available in market. (A) Neoheal® hydrogel sheet used for wound dressing, (B) Amorphous gel that can be sued for necrotic wounds and burns, (C) - Hydrogel film and (D) Hydrogel impregnated gauze.
Film dressings are very thin, transparent polyurethane sheets of increased flexibility, which adhere to the per wound skin and achieve maintenance of moisture in the wound environment. They are impermeable to water and microorganisms but permeable to oxygen, water vapors and carbon dioxide. Due to their strong adherence, they can be applied to moving surfaces like joints but they cause pain and damage to the per-wound upon removal. On the other hand, excessive accumulation of wound fluid beneath the dressing can result in loosening of the adhesive and leakage of the fluid, leading to maceration and facilitating bacterial invasion. Film dressings are typically applied to superficial and newly healed wounds, including graft sites of split skin and peripheral venous catheter sites (Nguyen et al., 2023).
Foam dressings most commonly consist of polyurethane foam covered by a silicone film which acts as a microbial and water barrier. They are highly absorbing, depending on the foam thickness, texture and pore size, and can maintain a moist wound environment. They also provide thermal insulation. Their increased absorption capacity qualifies them for the treatment of exuding wounds. They can be attached to the wound for up to 7 days, depending on the volume of the wound exudate. They are usually applied to minimal and moderately exuding wounds, burns, chronic wounds and ulcers. Then again, they are not recommended for the treatment of dry epithelial wounds, necrotic wounds and those needing frequent changes [6].
Unlike traditional dressings, such as bandages and gauzes, hydrogel dressings are widely acknowledged for their excellent properties, including mechanical properties that are compatible with biological tissues and exceptional water retention capacity which can keep the wound moist and continuously absorb exudate. In addition, their opportune biodegradation avoids secondary damage during dressing replacement, making them ideal wound dressing materials [74]. Furthermore, compared to other emerging dressings, such as foam and films, hydrogels possess a three-dimensional porous network structure similar to that of a natural ECM, providing a framework for cells to proliferate and migrate. More importantly, hydrogel dressings can be structurally and biochemically designed and functionally integrated to acquire various advantageous properties (Giliomee et al., 2022). Anti-inflammatory hydrogel dressings are foremost representatives. Wound dressing classifications used in this manuscript are presented below in Figure 1 and Table 3; for each type of dressing suitable wounds are briefly named.
Hydrocolloid dressings usually comprise self-adhesive hydrophilic colloid granules (e.g., carboxymethyl cellulose, gelatin, pectin) of various sizes, coated with a water-resistant polyurethane (PU) film protecting the wound from external agents such as bacteria, environmental agents, etc. They are capable of absorbing large amounts of wound exudate while being impermeable to vapors and oxygen, maintaining this way a moist wound environment, stimulating epithelialization and synthesis of collagen, and decreasing the pH of wound fluid resulting in bacteria reduction. In addition, they prevent infection and promote the removal of damaged/infected tissue via autolysis. Moreover, they do not need secondary dressings. They are frequently impregnated with active agents in order to treat lower-extremity and/or pressure ulcers. They are typically applied to low and or moderate exuding wounds, granular and necrotic wounds, as well as acute wounds such as partial and/or full-thickness burns and (post) surgical wounds (Nguyen et al., 2023).
Alginates have been extensively used in wound healing because of their valuable properties, like enhanced absorption capability, biocompatibility, non-toxicity and permeability to gases (e.g., oxygen, etc.) and liquids. They have been manufactured in various wound dressing forms, such as films, porous sheets, Nano fibers, hydrogels (Nguyen et al., 2023). In comparison with traditional dressings such as gauze, alginate dressings absorb excess wound exudate while retaining their structural integrity, thus providing a moist wound environment, diminishing bacterial infection and stimulating wound healing (Nguyen et al., 2023). Furthermore, they can diminish wound odor and inflammation, and exhibit hemostatic properties (Nguyen et al., 2023). Upon application onto the wound, alginate forms a gel and easily sloughs when removing the dressing or rinsing with sterile saline. A secondary dressing is usually required in order to stabilize the non-adhesive alginate dressing. Alginate is appropriate for the treatment of both acute and chronic exuding wounds like pressure ulcers, diabetic foot ulcers, (infected) surgical wounds and burns. In the absence of adequate liquid necessary to form a gel, alginate could leave fiber’s at the wound site which could cause inflammation (Nguyen et al., 2023). Alginate dressings are usually combined with various synthetic polymers to increase their mechanical properties. The therapeutic efficiency of the composite dressing is dependent on the ratio of synthetic polymers to alginate, the degree of crosslinking as well as the encapsulation of antimicrobial agents and/or nanoparticles (Zhou et al., 2020). Table 3 shows commercially available modern dressings.
The development of hydrogels as potential wound dressings for pressure ulcers, dry chronic wounds, necrotic wounds, burns, etc., has received a lot of attention because of their three-dimensional (3D) porous structure mimicking extracellular matrix (ECM), their high water absorption and their mechanical properties (e.g., elasticity, softness) providing a cooling/soothing effect and facilitating the dressing application and removal, their oxygen permeability and their ability to encapsulate various active ingredients (e.g., pharmaceutics, growth factors (Chun et al., 2018; Yuan et al., 2023). In particular, injectable hydrogels have triggered research interest due to their ability to fill irregular wounds, thus avoiding gel fragmentation, and their inherent self-healing properties (Wang et al., 2022).
Both natural (e.g., collagen, chitosan, hyaluronic acid, alginate, gelatin, etc.) and synthetic (e.g., poly (ethylene glycol methacrylate), poly (ethylene oxide), poly (hydroxyethyl methacrylate), poly (acrylic acid), etc.) polymers have been used for the formation of hydrogels (Yuan et al., 2023), with a preference towards natural polymers exhibiting biocompatibility, nontoxicity, enhanced cell attachment and strong activity against bacteria (Wang et al., 2022). Nevertheless, the performance of hydrogels formed using a single natural or synthetic polymer is often limited, so the research interest has focused on the design/development of multifunctional hydrogels with superior properties to be applied as wound dressings (Yuan et al., 2023). including hydrogels efficiently encapsulating conductive agents, which further promote wound healing via regulation of cell activities like adhesion, proliferation and migration (Wang et al., 2022), as well as Gels pharmaceutics, bioactive agents and/or nanoparticles (Yang et al., 2018). It should be pointed out that all hydrogel properties (e.g., physicochemical, rheological/mechanical, biological) can be readily affected by their chemical composition (i.e., selection of polymer backbones, functional groups, crosslinking mechanism and secondary crosslinking interactions, as well as integration of Nano composites) resulting in the formation of hydrogels exhibiting critical characteristics like inject ability, stimuli responsiveness, self-healing (Wang et al., 2022).
Hydrogel synthesis by 3D printing is the most advanced technique that involves sophisticated instruments and multistep processes. 3D printing is an expensive technique with controlled quality and pore structure. Limitations of this method are filament resolution (Wang et al., 2022). The 3D-printed porous constructs promote oxygen exchange and ease the removal of metabolites, improving this way of cell proliferation (Tsegay et al., 2022). The introduction of 3D printing to wound dressings has revealed promising results as a consequence of the method’s capability for controlled design and fabrication of dressings exhibiting tuned microstructure (Tsegay et al., 2022). Furthermore, it allows the temporally and spatially controlled release of various bioactive agents (e.g., drugs, growth factors, antimicrobial agents, nanoparticles) (Alizadehgiashi et al., 2021).
Hydrogels are a popular class of biomaterial inks owing to their biomimetic properties and their benign processing conditions entitling them as suitable candidates for TE applications. They are usually printed in the form of their precursor materials and their final structure is obtained via crosslinking during or post 3D printing (Giliomee et al., 2022). Shape fidelity and collapsing are typical challenges in 3D hydrogel printing related to the viscoelastic properties of the ink and its solid content, respectively. Ideally, the ink should be able to flow through the nozzle throughout the printing process and preserve its shape post printing (Leppiniemi et al., 2017). Hydrogel precursors need to have a suitable viscosity to preserve their structural integrity until crosslinking. This can be facilitated by the increase in the polymer concentration, the addition of composites and the use of (near) gel-phase inks such as gelatin solutions which can be printed at a temperature close to their sol–gel transition (near gel-phase inks) and partially crosslinked hydrogels like alginate solutions containing low concentrations of calcium chloride (gel-phase inks) (Tsegay et al., 2022), as well as via the use of rheology modifers such as cellulose nanofbrils, which could improve ink printability and achieve shape fdelity post printing (Leppiniemi et al., 2017).
Recent advances in 3D printing technologies (e.g., extrusion-based 3D printing) permit hydrogel customization according to wound size and depth (Guo and Longaker, 2023), and enable the formation of multi-component hydrogels exhibiting various microstructures and networks of interconnected pores which facilitate the transport of oxygen, nutrients, metabolic wastes (Guo and Longaker, 2023), as well as the temporal and spatial control of bioactive’s release thus promoting bacteria reduction, favoring tissue proliferation and decreasing healing time (Guo and Longaker, 2023).
Various types of polymers, both natural (e.g., sodium alginate (SA), chitosan, gelatin, carboxymethyl cellulose (CMC-Na) (Zhang et al., 2021), and synthetic (e.g., 4-arm PEG (Giliomee et al., 2022), 2-hydroxyethyl methacrylate (HEMA), polyethylene glycol dimethacrylate (PEGDA), poly (acrylic acid) (PAA) (Tsegay et al., 2022), as well as combinations there have been used for Gels 2024, 10, 147 19 of 61 the formation of hydrogel inks. From a chemical point of view, the selected materials should be easily modifed with various chemical groups in order to be cross-linked via different mechanisms (e.g., free radical, ionic, etc.) and functionalized with appropriate molecules (e.g., functional polymers, adhesion peptides, peptides cleavable by proteases). They should also undergo hydrolysis and/or enzymatic degradation, potentially exhibiting inherent antibacterial properties, stimuli responsiveness, etc. Finally, they could permit the formation of a reversible 3D network via dynamic chemical bonding to enable self-healing (Yuan et al., 2023).
Sodium alginate (SA), a cost-effective marine-derived polysaccharide, characterized by excellent biocompatibility, enhanced aqueous solubility and minimal toxicity, has been widely utilized in 3D printing of wound dressings since it can rapidly crosslink with divalent captions (e.g., Ca2+, Mn2+, Ba2+, Cu2+) and absorbs excess wound fluid while it maintains a physiological moist wound environment (Xu et al., 2022). On the other hand, shape infidelity, excessive swelling, rapid degradation rate, low mechanical properties, etc., could hinder its use in the 3D printing of wound dressings (Liao et al., 2023). Nevertheless, modifcation and/or enhancement of SA by combination with different organic/inorganic materials (e.g., various polymers such as gellan gum (Xu et al., 2022), collagen, gelatin, cellulose, etc., and/or nanoparticles) can improve its performance (Kim et al., 2023). In this respect, Wang and coworkers designed and fabricated a bilayer membrane (BLM) comprising a layer of alginate hydrogel printed on a poly (lactic-co-glycolic acid) (PLGA) fbrous membrane mimicking the dermis and epidermis, respectively. The PLGA membrane was found toprevent bacterial invasion and maintain the hydrogel moisture content, while the hydrogel layer stimulated cell adhesion and proliferation.
Medicinal mushrooms have been valued and used since ancient times by the Chinese, Korean, Japanese, Egyptians, and European communities. They are valued not only for the culinary purposes but also for their nutritional and medicinal values (Tan et al., 2009). The greatest attribute of mushrooms, besides their taste, is their peculiar healing properties. Recently, ethnomycological knowledge of medicinal mushrooms for their curative properties is being tapped. Lignosus rhinocerotis, however, was only collected from the wild. In the wild, the tiger milk mushroom grows solitary and makes the collection process time and energy consuming. Since the 2000s, large-scale cultivation of L. rhinocerotis in a controlled environment was made successful in Malaysia, overcoming the cost and supply problem (Lau et al., 2015a). Commercialization of this mushroom was then made possible and this opened the opportunity to investigate the potential pharmacological and nutraceutical properties of this mushroom.
This mushroom consists of the pileus (cap), stipe (stem), and sclerotium (tuber). Its morphology is unusual for a polypore as the fruiting body (cap and stem) raises from the tuber under the ground, rather than from woody substrate. The cap and stem are woody while the sclerotium is a compacted mass of fungal mycelium containing food reserves. The sclerotium is white and gives a milk-like solution; and it even tastes like milk (Tan et al., 2009). As the irregular shaped sclerotium remains underground, the collection of the mushroom is challenging. Thus, due to lack of samples, limited study is done on this national treasure.
This mushroom is known as “cendawan susu rimau” which literally means “tiger milk mushroom (Lau et al., 2015b). Early documentation of this mushroom were given by Corner (1989). In the early 20th century, the taxonomy of L. rhinocerotis depended primarily on morphological observations. However, in recent years, specimens of “susu rimau” collected in these regions were confirmed as L. rhinocerotis on the basis of both micro- and macro morphological characteristics, as well as molecular approaches. To date, L. rhinocerotis is noted to be the “most commonly occurring member of Lignosus in Malaysia” (Tan et al., 2015; Lai et al., 2011). Figure 2 shows the taxonomic classification of L. rhinocerotis.
According to folklore, it is believed that the mushroom emerges on the spot where the milk of a tigress had accidently dribbled during lactation. The sclerotium of the mushroom resembles the “congealed white mass of milk” (Chang, 2015). Different tribal communities in Malaysia have different referral names, such as “betes kismas” by the Semai (Chang, 2010), “tish am ong” by the Kensiu (Mohammad et al., 2012). Lignosus rhinocerotis found in China were called “how gui kou or hurulingzhi” (in Chinese), which means “tiger milk Ganoderma” (Yokota, 2011). In Japan, it is known as “hijiritake” (Lee et al., 2009).
Besides the traditional beliefs that L. rhinocerotis was derived from the tiger’s milk, there are many other folklore beliefs about this mushroom. The Semai (indigenous people of Malaysia) believes that L. rhinocerotis could reinstate the spirit of a crop and guarantee a lavish harvest. The sclerotia are habitually used during paddy farming and prayer ritual for a bountiful crop yields (Haji Taha, 2006). Alternatively, some crops, for instance, paddy is positioned in a flower-filled container, and suspended over the mushroom (Nallathamby et al., 2018).
The L. rhinocerotis are occasionally sold in Traditional Chinese Medicine (TCM). They are used by the TCM practitioner to revitalize the body of the patients. According to Sabaratnam et al. (2013), infusions of L. rhinocerotis are said to improve the overall wellness of the individual by enhancing the vitality, energy, and alertness. There are many ways this mushroom is prepared and consumed to treat illness. The mushrooms are grounded or sliced, then boiled with water for drinking or soaked into Chinese wine for external applications (Chang and Lee, 2004). The sclerotium is also eaten raw and with betel leaves to relieve a cough and sore throat. The preparation methods of decoction and/or topical medicine vary among tribes.
The anti-inflammatory activity of the sclerotium of L. rhinocerotis was previously reported with its hot aqueous, cold aqueous, and methanol extracts (Lee et al., 2012; Lee et al., 2013). Lee et al. (2014), reported that the three extracts of L. rhinocerotis exhibited anti-inflammatory properties as shown by the carrageenan-induced paw edema test using Sprague-Dawley rats. The cold aqueous extract, the most potent extract, was subjected to separation by Sephadex G50 gel filtration chromatography. The resulting high-molecular-weight protein fraction was further assessed for anti-inflammatory activity in lipopolysaccharide (LPS), induced RAW 264.7 macrophage cells. The protein fraction was shown to inhibit tumor necrosis factor alpha (TNF-α) production. The anti-inflammatory effect of L. rhinocerotis hot aqueous and ethanol extracts on RAW 264.7 cells was further tested (Baskaran, 2015). The ethanol extract showed significant decrease (48.3%–88.5%) of nitric oxide (NO) production from 0.01 to 100 μg/mL dose-dependently but the aqueous extract did not show a significant reduction. The ethanol extract was able to activate signal transducer and activator of transcription 3 (STAT3) pathways by reducing inducible nitric oxide synthase (iNOS) and cyclooxygenase-2 (COX-2) expressions while increasing the interleukin 10 (IL-10) expression. Nallathamby et al. (2016) analyzed the ethyl acetate fraction from the ethanol extract of L. rhinocerotis. The fraction significantly reduced the NO production in microglial (BV2) cells by 12%–70% at 10 and 100 μg/mL; respectively. The major compounds of the ethyl acetate fraction were revealed as linoleic acid, oleic acid, and ethyl linoleate. The identified compounds were further tested individually for their anti-inflammatory activities. Treatment with linoleic acid significantly suppressed iNOS and COX2 expression by 1.2-fold as compared to the control. In another study, LPS-induced BV2 cells pretreated with hot aqueous extract (500 μg/mL), n-butanol fraction of hot aqueous extract (250 μg/mL), and ethyl acetate fraction of hot aqueous extract (250 μg/mL), showed maximum inhibition of NO production by 88.95, 86.50, and 85.93%, respectively (Seow et al., 2017). These studies represent the first evidence of anti-inflammatory properties of L. rhinocerotis using brain microglial BV2 cells.
Four extracts of the wild L. rhinocerotis sclerotium, i.e., petroleum ether, chloroform, methanol, and aqueous extracts, were screened for their anti-microbial properties (Mohanarji et al., 2012). The four extracts were tested against 15 pathogenic bacteria, including Staphylococcus, Corynebacterium, Bacillus, Streptococcus, Klebsiella, Salmonella, Pseudomonas, Escherichia, and Micrococcus spp.; as well as four fungi species including Candida spp. and Mucor sp. Antifungal and antibacterial activities of the extracts were evaluated by measuring the inhibition zone using disc diffusion assay. The methanol and aqueous extracts (30 mg/mL) showed significant inhibition against the tested microbes except for Streptococcus pyogenes and Serratia marcescens. A qualitative phytochemical analysis showed the presence of alkaloids, protein, gums and mucilage, and flavonoids in the extracts of L. rhinocerotis (Mohanarji et al., 2012).
Fungi are members of a large, diverse group of heterotrophic organisms which are frequently found living on dead, decaying wood, and other organic matter. They are eukaryotic, with a scope of internal membrane systems, and membrane-bound organelles, and possess a distinct cell wall that is made largely from polysaccharides and chitin (Richardson and Warnock, 2012). The consumption of medicinal mushrooms has long been practiced and there is growing interest in the discovery of bioactive compounds with medicinal values from industries and the scientific communities alike (Pereira et al., 2005). This section provides an overview of the capacities of fungi for wound healing enhancement, both in modern science and traditional knowledge. Figure 3 shows the effect of macro fungi L. rhinocerotis for wound healing.
The use of traditional medicine or natural compounds has been of great interest in the pharmaceutical field for many years including the development of wound healing agents (Chin et al., 2018; Lai et al., 2016). For example, herbal extracts have been investigated for their healing properties and developed as advanced wound dressings (Monirul Islam et al., 2022). Lignosus rhinocerotis is a medicinal mushroom and traditionally used for wound treatment (Lai et al., 2014) by indigenous communities in Malaysia. Its sclerotium holds various medicinal values including anti-microbial and antiviral activities (Eik et al., 2012; Yap et al., 2014), making it a good candidate as a natural wound healing agent. Traditionally, people cut the sclerotium of the mushroom into pieces and boil in water prior to its application for medicinal purposes (Chang and Lee, 2004). Therefore, hot water extraction was applied in this study to resemble the traditional preparation of L. rhinocerotis.
In addition to that, the wound dressing should provide a hemostatic effect to avoid excessive bleeding. In addition to high antioxidant and anti-inflammatory (Elzayat et al., 2018), the peel extract of traditional medicine or natural compounds exhibits hemostatic activity owing to its high polyphenols content such as ellagitannins (Soltani et al., 2014). Therefore, the combination of both extracts in a wound dressing is anticipated to provide synergistic effects to allow faster healing of acute wounds. Moreover, none utilize the combination both extracts that offers hemostatic and healing properties that may be useful for sport-related wounds that are urgently needed in facilitating the speedy recovery of injured athletes (Scott et al., 2020). Several studies have been carried out for increasing the rate of wound healing and hemostatic effects (Chang et al., 2022; Hu et al., 2022). However, a new class of wound dressing is still required for achieving better outcomes by fulfilling other needs such as the prevention of infections and easy application on the affected areas (Agarwal et al., 2022). Other than offering multiple actions for speedy wound healing, the combination of thermo responsive gels and both extracts is expected to allow self-medication. Table 1 shows the summary of the macro fungi studied for their wound healing activity. The Table 4 showed the Summary of macro-fungi utilized as traditional wound dressing treatment.
In the current studies, extracts of L. rhinocerotis were incorporated into topical thermo responsive gel formulations. Phytochemical screening of extracts was first carried out using Gas Chromatography-Mass Spectrophotometry (GC-MS) to identify potential compounds that might contribute to the wound healing effects. Later, all the gels were evaluated for various properties including T gel, texture, rheology, and morphology as well as biological effects (antibacterial and thrombin activities). Wound healing effects of the gels were also evaluated via the in vitro cell migration assay for wound (Faris Taufeq et al., 2023).
In addition to scientific research on the wound healing activity of fungi, there are a few mushrooms that are traditionally utilized for wound treatment. These macro fungi were reported in the literature for traditional wound treatment practices. Table 5 summarizes the macro-fungi utilized for traditional wound treatment.
The sclerotium of wild L. rhinocerus (Common name: Tiger Milk Mushroom) has been used by Aborigines as a traditional medicine to treat wounds, asthma, fever, breast cancer, stomach cancer, and food poisoning (Tan et al., 2009). The L. rhinoceros powder also was mixed with Chinese rice wine and applied topically for treating lumps, sores, and boils (Wong, 2011).
H. utriformis (Common name: Puffball) has been used in traditional practices for the treatment of wounds but lacks scientific reports. The fruiting bodies of H. utriformis are used in traditional medicine for surgical and burn wound dressings (Petrovi et al., 2019). When the mature fruit body of H. utriformis bursts or is impacted, clouds of brown dust-like spores are emitted and the spore powder is useful to stop bleeding. The practice can be found in the rural state of Europe, North America, and India. A review on “Puffball Usage among North American Indians” has reported that the Indian group from the Missouri River region utilized the puffball as a hemostat. The dried puffball was pulverized and applied to the wound to stop bleeding (Burk, 1983). An extensive survey among the Baiga and Bharia tribes in Madhya Pradesh, central India state, have been showing that the giant puffball was utilized to stop bleeding for healing wounds (Rai et al., 1993). An early report in 1860, reported on the usage of puffball as an anesthetic, like chloroform, for burnt treatment (Wei et al., 2011).
M. esculenta (common name: Yellow morel) has been used in Chinese medicine for thousands of years (Wei et al., 2011). It is also highly utilized by the various tribal group from Kupwara district (Kashmir, India) and Neelum Valley (Azad Jammu and Kashmir, Pakistan). The fruiting body of M. esculenta was pulverized to powder for wound application to speed up healing and acts as an antiseptic (Mahmood et al., 2011; Lone et al., 2012).
F. fomentarius (common name: Hoof fungus) was classified by The Greek physician Hippocrates circa 450 BCE as a potent anti-inflammatory agent for cauterizing wounds (Stamets and Zwickey, 2014). In European, West Siberian, and Indian folk medicine, F. fomentarius fruit body was made as part of the bandage material. It was pounded with water until it soften and externally applied to wounds to stop the bleeding (Vaidya and Rabba, 1993). In German and Austria, F. fomentarius was called a wound sponge or surgical sponge. It is widely used as a styptic by farmers, surgeons, and dentists up to the 19th century (Grienke et al., 2014a).
In the last 20 years, a wide variety of biomaterials have been synthesized and used for skin wound healing. However, this is the first time that a native ECM molecule (collagen type I), a biocompatible natural polymer (chitosan), and an inflammation-controlling molecule (dexamethasone) have been combined into a hydrogel that proved to be capable of sustaining mesenchymal stem cells culture, showing that cells remained viable and expressed the anti-inflammatory factor IL-10 upon culture in the hydrogel. Therefore, the next step in our research would be to evaluate the in vivo response of hADMSC seeded into the COL1/CHS/Dex hydrogels using a burn animal model.
Due to the declining numbers of wild type L. rhinocerus, some institutes or companies were making the initiative on developing techniques for the cultivation of L. rhinocerus, such as LiGNOTM Biotech Sdn. Bhd. (cultivar TM02VR), Hong Kong Polytechnic University, and Sanming Mycological Institute (Thuraisingam et al., 2010; Heo et al., 2011) to avoid this valuable mushroom from being extinct. Various in-depth scientific studies were then called for to elucidate the bioactivities of these cultivars.
L. rhinocerus was reported to immunomodulation by increasing cytokines (IL-5, IL-6, and MIP-2) expression in RAW 264.7 cells conceivably through the NF-jB/MAPK signaling pathways (Sum et al., 2020). Both signaling pathways have been implicated in corneal epithelial wound healing, scratch injury, and cutaneous wound healing (Thuraisingam et al., 2010; Heo et al., 2011). MIP-2 dominates the early part of the inflammation phase by regulating the migration of granulocytes including neutrophils and stem cells (Reinke and Sorg, 2012). Meanwhile, pro-inflammatory IL-5 and IL-6 cytokines are released to promote inflammation by inducing immune cells to the wound site and concurrently activating growth factors that contribute to angiogenesis and collagen synthesis (Hayta et al., 2018). Increased release of IL-5, IL-6, and MIP-2 caused by L. rhinocerus will accelerate the inflammatory phase by early infiltration and elimination of neutrophils and macrophages.
Wound inflammation is complexly regulated. The process of healing can be disrupted by both excessive inflammation infiltration later on and insufficient inflammation levels in the early stages, with the latter occurring more frequently in wound healing. As a result, in recent years, a variety of sophisticated anti-inflammatory biomaterials have been used in wound healing, particularly in the treatment of chronic wounds. One of the most popular of them is the anti-inflammatory hydrogel dressing, which mimics skin functions chemically, physically, and electrically. This review summarizes the scope of emerging anti-inflammatory hydrogel dressings and traditional Lignosus rhinoceros used for wound healing agents focusing on wound healing. However, the development of anti-inflammatory hydrogel/Lignosus rhinoceros dressings for enhanced wound healing is still in the early stages. Whether previous treatments can be turned into practical applications, these techniques also face specific barriers regarding their biocompatibility and technological outcomes. Unfortunately, the complexity of chronic wound healing in humans cannot be fully replicated in animal models for which therapies have been explored, and human physiology varies significantly from that of mouse Moreover, hydrogel safety needs to be thoroughly evaluated because it can lead to unwanted immunological responses such infections, allergies, and autoimmune diseases. Therefore, extensive study is needed for the development of hydrogel dressings with potential anti-inflammatory properties for effective use in clinical applications.
Future developments in anti-inflammatory hydrogel dressings and the medicinal mushroom Lignosus rhinoceros could eventually make it possible to precisely regulate the processes involved in wound healing. Wearable sensors and imaging devices may be used to monitor the level of wound inflammation in real-time and other technologies that include automated stimulus responsiveness may be utilized to adjust therapeutic approaches. Such advances in the development of precise treatment of wounds improve patient curing rates, alleviate pain and reduce costs. Meanwhile, anti-inflammatory hydrogels may also be loaded with other functional components, such as hemostatic, conductive and adhesive materials, making anti-inflammatory hydrogel dressings more powerful and predictable for clinical applications but medicinal mushrooms are low cost as compare to hydrogels, which is a vitally important subject in translational research, particularly in the use of advanced anti-inflammatory hydrogel dressings. Translational research may generate clinically meaningful outcomes in wound healing that improve human health and allow fundamental scientific findings to be translated more efficiently into practical applications. This review has the potential to enhance not just the meaning of wound healing but also the reach of theoretical studies. When developing and innovating new functional hydrogel wound dressings and traditional mushrooms micro-fungi used for wound healing agents, this review can offer new ideas and mechanisms for researchers in the future, advancing the field of wound dressing therapy and expanding the market for functional hydrogel dressings.
SA: Writing–original draft. HU: Writing–review and editing. NM: Writing–review and editing. WW: Writing–review and editing. JZ: Funding acquisition, Supervision, Writing–review and editing.
The author(s) declare that financial support was received for the research, authorship, and/or publication of this article. This review was funded by Ministry of Science and Technology of the People’s Republic of China (No: 31872520); China Agriculture Research System and National Natural Science Foundation of China (CARS-37).
The authors would like to thanks Lanzhou Institute of Husbandry and Pharmaceutical Sciences, Chinese Academy of Agriculture Sciences, providing feedback on this manuscript.
The authors declare that the research was conducted in the absence of any commercial or financial relationships that could be construed as a potential conflict of interest.
All claims expressed in this article are solely those of the authors and do not necessarily represent those of their affiliated organizations, or those of the publisher, the editors and the reviewers. Any product that may be evaluated in this article, or claim that may be made by its manufacturer, is not guaranteed or endorsed by the publisher.
Agarwal, R., Niezgoda, J., Niezgoda, J., Madetipati, N., and Gopalakrishnan, S. (2022). Advances in hemostatic wound dressings: clinical implications and insight. Adv. Skin and Wound Care 35 (2), 113–121. doi:10.1097/01.asw.0000790488.72494.57
Ahmed, S., and Ikram, S. (2016). Chitosan based scaffolds and their applications in wound healing. Achiev. life Sci. 10 (1), 27–37. doi:10.1016/j.als.2016.04.001
Ahmed, T. S., Magaji, M. G., Yaro, A. H., Musa, A. M., and Adamu, A. K. (2011). Aqueous methanol extracts of Cochlospermum tinctorium (A. Rich) possess analgesic and anti-inflammatory activities. J. Young Pharm. 3 (3), 237–242. doi:10.4103/0975-1483.83774
Alizadehgiashi, M., Nemr, C. R., Chekini, M., Pinto Ramos, D., Mittal, N., Ahmed, S. U., et al. (2021). Multifunctional 3D-printed wound dressings. ACS nano 15 (7), 12375–12387. doi:10.1021/acsnano.1c04499
Al-Tabbaa, O., and Ankrah, S. (2016). Social capital to facilitate engineered’™university industry collaboration for technology transfer: a dynamic perspective. Technol. Forecast. Soc. Change 104, 1–15. doi:10.1016/j.techfore.2015.11.027
Alven, S., and Aderibigbe, B. A. (2020). Chitosan and cellulose-based hydrogels for wound management. Int. J. Mol. Sci. 21 (24), 9656. doi:10.3390/ijms21249656
Anusiya, G., Gowthama Prabu, U., Yamini, N. V., Sivarajasekar, N., Rambabu, K., Bharath, G., et al. (2021). A review of the therapeutic and biological effects of edible and wild mushrooms. Bioengineered 12 (2), 11239–11268. doi:10.1080/21655979.2021.2001183
Assis, A. C. L. d., Moreira, L. M. C. d. C., Rocha, B. P., Pereira, M. R. B., de Melo, D. F., Moura, R. O. d., et al. (2021). N-Acylhydrazone derivative-loaded cellulose acetate films: thermoanalytical, spectroscopic, mechanical and morphological characterization. Polymers 13 (14), 2345. doi:10.3390/polym13142345
Aswathy, S. H., Narendrakumar, U., and Manjubala, I. (2020). Commercial hydrogels for biomedical applications. Heliyon 6 (4), e03719. doi:10.1016/j.heliyon.2020.e03719
Atkin, L., Stephenson, J., and Bateman, S. (2015). Foam dressings: a review of the literature and evaluation of fluid-handling capacity of four leading foam dressings. Wounds U. K. 11 (1), 75–81.
Barillo, D. J., Barillo, A. R., Korn, S., Lam, K., and Attar, P. S. (2017). The antimicrobial spectrum of Xeroform ®. Burns 43 (6), 1189–1194. doi:10.1016/j.burns.2016.10.023
Barrientos, S., Stojadinovic, O., Golinko, M. S., Brem, H., and Tomic-Canic, M. (2008). PERSPECTIVE ARTICLE: growth factors and cytokines in wound healing. Wound repair Regen. 16 (5), 585–601. doi:10.1111/j.1524-475x.2008.00410.x
Baskaran, A. (2015). Suppression of lipopolysaccharide and hydrogen peroxide-induced inflammatory responses in raw 264.7 macrophage by pleurotus giganteus and lignosus rhinocerotis/Asweni a/p Baskaran. University of Malaya.
Berkovitch, Y., and Seliktar, D. (2017). Semi-synthetic hydrogel composition and stiffness regulate neuronal morphogenesis. Int. J. Pharm. 523 (2), 545–555. doi:10.1016/j.ijpharm.2016.11.032
Bonnet, M., Trimaille, T., Brezun, J.-M., Feron, F., Gigmes, D., Marqueste, T., et al. (2020). Motor and sensitive recovery after injection of a physically cross-linked PNIPAAm-g-PEG hydrogel in rat hemisectioned spinal cord. Mater. Sci. Eng. C 107, 110354. doi:10.1016/j.msec.2019.110354
Boucard, N. g., Viton, C., Agay, D., Mari, E., Roger, T., Chancerelle, Y., et al. (2007). The use of physical hydrogels of chitosan for skin regeneration following third-degree burns. Biomaterials 28 (24), 3478–3488. doi:10.1016/j.biomaterials.2007.04.021
Brand, M., Laier, C., and Young, K. S. (2014). Internet addiction: coping styles, expectancies, and treatment implications. Front. Psychol. 5, 1256. doi:10.3389/fpsyg.2014.01256
Brumberg, V., Astrelina, T., Malivanova, T., and Samoilov, A. (2021). Modern wound dressings: hydrogel dressings. Biomedicines 9 (9), 1235. doi:10.3390/biomedicines9091235
Campbell, K. T., Stilhano, R. S., and Silva, E. A. (2018). Enzymatically degradable alginate hydrogel systems to deliver endothelial progenitor cells for potential revasculature applications. Biomaterials 179, 109–121. doi:10.1016/j.biomaterials.2018.06.038
Chang, L., Chang, R., Liu, X., Ma, X., Chen, D., Wang, Y., et al. (2022). Self-healing hydrogel based on polyphosphate-conjugated pectin with hemostatic property for wound healing applications. Biomater. Adv. 139, 212974. doi:10.1016/j.bioadv.2022.212974
Chang, Y. S. (2015). Tiger's milk is a fungus with medicinal value. Kuala Lumpur: New Straits Times Press. [Google Scholar].
Chang, Y. S., and Lee, S. S. (2004). Utilisation of macrofungi species in Malaysia. Fungal Divers. 15 (2), 15–22.
Chen, T.-Y., Wen, T.-K., Dai, N.-T., and Hsu, S.-h. (2021). Cryogel/hydrogel biomaterials and acupuncture combined to promote diabetic skin wound healing through immunomodulation. Biomaterials 269, 120608. doi:10.1016/j.biomaterials.2020.120608
Chin, C.-Y., Jalil, J., Ng, P. Y., and Ng, S.-F. (2018). Development and formulation of Moringa oleifera standardised leaf extract film dressing for wound healing application. J. Ethnopharmacol. 212, 188–199. doi:10.1016/j.jep.2017.10.016
Chun, H. J., Park, K., Kim, C.-H., and Khang, G. (2018). Novel biomaterials for regenerative medicine. Springer.
Dabrowska, A. K., Spano, F., Derler, S., Adlhart, C., Spencer, N. D., and Rossi, R. M. (2018). The relationship between skin function, barrier properties, and body-dependent factors. Skin Res. Technol. 24 (2), 165–174. doi:10.1111/srt.12424
Darby, I. A., Laverdet, B., Bonta, F., and Desmoulire, A. (2014). Fibroblasts and myofibroblasts in wound healing. Clin. Cosmet. investigational dermatology 7, 301–311. doi:10.2147/ccid.s50046
Dimatteo, R., Darling, N. J., and Segura, T. (2018). In situ forming injectable hydrogels for drug delivery and wound repair. Adv. drug Deliv. Rev. 127, 167–184. doi:10.1016/j.addr.2018.03.007
Dodero, A., Pianella, L., Vicini, S., Alloisio, M., Ottonelli, M., and Castellano, M. (2019). Alginate-based hydrogels prepared via ionic gelation: an experimental design approach to predict the crosslinking degree. Eur. Polym. J. 118, 586–594. doi:10.1016/j.eurpolymj.2019.06.028
Dou, Q. Q., Liow, S. S., Ye, E., Lakshminarayanan, R., and Loh, X. J. (2014). Biodegradable thermogelling polymers: working towards clinical applications. Adv. Healthc. Mater. 3 (7), 977–988. doi:10.1002/adhm.201300627
Drucker, C. R. (2012). Update on topical antibiotics in dermatology. Dermatol. Ther. 25 (1), 6–11. doi:10.1111/j.1529-8019.2012.01493.x
Dutzan, N., Abusleme, L., Bridgeman, H., Greenwell-Wild, T., Zangerle-Murray, T., Fife, M. E., et al. (2017). On-going mechanical damage from mastication drives homeostatic Th17 cell responses at the oral barrier. Immunity 46 (1), 133–147. doi:10.1016/j.immuni.2016.12.010
Eftekharizadeh, F., Dehnavieh, R., Hekmat, S. N., and Mehrolhassani, M. H. (2016). Health technology assessment on super oxidized water for treatment of chronic wounds. Med. J. Islamic Repub. Iran 30, 384.
Eik, L.-F., Naidu, M., David, P., Wong, K.-H., Tan, Y.-S., and Sabaratnam, V. (2012). Lignosus rhinocerus (Cooke) Ryvarden: a medicinal mushroom that stimulates neurite outgrowth in PC-12 cells. Evidence-Based Complementary Altern. Med. 2012, 1–7. doi:10.1155/2012/320308
Elzayat, E. M., Auda, S. H., Alanazi, F. K., and Al-Agamy, M. H. (2018). Evaluation of wound healing activity of henna, pomegranate and myrrh herbal ointment blend. Saudi Pharm. J. 26 (5), 733–738. doi:10.1016/j.jsps.2018.02.016
Farahani, M., Gharachorloo, M., Farahani, M., and Manthouri, M. (2021). Parsbert: transformer-based model for Persian language understanding. Neural Process. Lett. 53, 3831–3847. doi:10.1007/s11063-021-10528-4
Farazin, A., Shirazi, F. A., and Shafiei, M. (2023). Natural biomarocmolecule-based antimicrobial hydrogel for rapid wound healing: a review. Int. J. Biol. Macromol. 125454.
Faris Taufeq, F. Y., Habideen, N. H., Rao, L. N., Podder, P. K., and Katas, H. (2023). Potential hemostatic and wound healing effects of thermoresponsive wound dressing gel loaded with Lignosus rhinocerotis and punica granatum extracts. Gels 9 (1), 48. doi:10.3390/gels9010048
Feng, C.-J., Lin, C.-H., Tsai, C.-H., Yang, I. C., and Ma, H. (2019). Adipose-derived stem cells-induced burn wound healing and regeneration of skin appendages in a novel skin island rat model. J. Chin. Med. Assoc. 82 (8), 635–642. doi:10.1097/jcma.0000000000000134
Finnerty, C. C., Jeschke, M. G., Branski, L. K., Barret, J. P., Dziewulski, P., and Herndon, D. N. (2016). Hypertrophic scarring: the greatest unmet challenge after burn injury. Lancet 388 (10052), 1427–1436. doi:10.1016/s0140-6736(16)31406-4
Firlar, I., Altunbek, M., McCarthy, C., Ramalingam, M., and Camci-Unal, G. (2022). Functional hydrogels for treatment of chronic wounds. Gels 8 (2), 127. doi:10.3390/gels8020127
Ganji, F., Vasheghani, F. S., and Vasheghani, F. E. 2010. Theoretical description of hydrogel swelling: a review.
Gibbs, S., Silva Pinto, A. N., Murli, S., Huber, M., Hohl, D., and Ponec, M. (2000). Epidermal growth factor and keratinocyte growth factor differentially regulate epidermal migration, growth, and differentiation. Wound repair Regen. 8 (3), 192–203. doi:10.1046/j.1524-475x.2000.00192.x
Giliomee, J., du Toit, L. C., Klumperman, B., and Choonara, Y. E. (2022). Investigation of the 3D Printability of covalently cross-linked polypeptide-based hydrogels. ACS omega 7 (9), 7556–7571. doi:10.1021/acsomega.1c05873
Glim, J. E., van Egmond, M., Niessen, F. B., Everts, V., and Beelen, R. H. J. (2013). Detrimental dermal wound healing: what can we learn from the oral mucosa? Wound repair Regen. 21 (5), 648–660. doi:10.1111/wrr.12072
Graca, M. F. P., Miguel, S. P., Cabral, C. t. S. D., and Correia, I. J. (2020). Hyaluronic acid Based wound dressings: a review. Carbohydr. Polym. 241, 116364. doi:10.1016/j.carbpol.2020.116364
Grainger, D. W. (2015). Enzymatically crosslinked scaffolds. Nat. Mater. 14 (7), 662–663. doi:10.1038/nmat4337
Grienke, U., Zall, M., Peintner, U., and Rollinger, J. M. (2014a). European medicinal polypores A modern view on traditional uses. J. Ethnopharmacol. 154 (3), 564–583. doi:10.1016/j.jep.2014.04.030
Grienke, U., Zll, M., Peintner, U., and Rollinger, J. M. (2014b). European medicinal polyporesâ A modern view on traditional uses. J. Ethnopharmacol. 154 (3), 564–583.
Grndemann, C., Reinhardt, J. K., and Lindequist, U. (2020). European medicinal mushrooms: do they have potential for modern medicine an update. Phytomedicine 66, 153131. doi:10.1016/j.phymed.2019.153131
Guo, J. L., and Longaker, M. T. (2023). Bioprinted hydrogels for fibrosis and wound healing: treatment and modeling. Gels 9 (1), 19. doi:10.3390/gels9010019
Gupta, A., Kowalczuk, M., Heaselgrave, W., Britland, S. T., Martin, C., and Radecka, I. (2019). The production and application of hydrogels for wound management: a review. Eur. Polym. J. 111, 134–151. doi:10.1016/j.eurpolymj.2018.12.019
Hayta, S. B., Guner, R., Cam, S., and Akyol, M. (2018). Rosacea is associated with thyroid autoimmunity: a case control study. Acta Endocrinol. Buchar. 14 (2), 248–251. doi:10.4183/aeb.2018.248
Heo, S. C., Jeon, E. S., Lee, I. H., Kim, H. S., Kim, M. B., and Kim, J. H. (2011). Tumor necrosis factor-α-activated human adipose tissue–derived mesenchymal stem cells accelerate cutaneous wound healing through paracrine mechanisms. J. investigative dermatology 131 (7), 1559–1567. doi:10.1038/jid.2011.64
Hixon, K. R., Klein, R. C., Eberlin, C. T., Linder, H. R., Ona, W. J., Gonzalez, H., et al. (2019). A critical review and perspective of honey in tissue engineering and clinical wound healing. Adv. wound care 8 (8), 403–415. doi:10.1089/wound.2018.0848
Holback, H., and Yeo, Y. (2011). Intratumoral drug delivery with nanoparticulate carriers. Pharm. Res. 28, 1819–1830. doi:10.1007/s11095-010-0360-y
Hu, H., Luo, F., Zhang, Q., Xu, M., Chen, X., Liu, Z., et al. (2022). Berberine coated biocomposite hemostatic film based alginate as absorbable biomaterial for wound healing. Int. J. Biol. Macromol. 209, 1731–1744. doi:10.1016/j.ijbiomac.2022.04.132
Hu, P., Yang, Q., Wang, Q., Shi, C., Wang, D., Armato, U., et al. (2019). Mesenchymal stromal cells-exosomes: a promising cell-free therapeutic tool for wound healing and cutaneous regeneration. Burns and trauma 7, 38. doi:10.1186/s41038-019-0178-8
In, S. M., An, H. G., Kim, J.-Y., and Lee, K.-I. (2021). Columellar wound immediately after open rhinoseptoplasty treated with application of DuoDERM Extra Thin. J. Craniofacial Surg. 32 (1), e98–e99.
Kamika, M. S., Cybulska, A. M., Skonieczna-Aydecka, K., Augustyniuk, K., Grochans, E., and Karakiewicz, B. (2020). Effectiveness of hydrocolloid dressings for treating pressure ulcers in adult patients: a systematic review and meta-analysis. Int. J. Environ. Res. Public Health 17 (21), 7881. doi:10.3390/ijerph17217881
Kamiska, M. S., Cybulska, A. M., Skonieczna-ydecka, K., Augustyniuk, K., Grochans, E., and Karakiewicz, B. (2020). Effectiveness of hydrocolloid dressings for treating pressure ulcers in adult patients: a systematic review and meta-analysis. Int. J. Environ. Res. Public Health 17 (21), 7881. doi:10.3390/ijerph17217881
Kammona, O., Tsanaktsidou, E., and Kiparissides, C. (2024). Recent developments in 3D-(bio) printed hydrogels as wound dressings. Gels 10 (2), 147. doi:10.3390/gels10020147
Kasuya, A., and Tokura, Y. (2014). Attempts to accelerate wound healing. J. dermatological Sci. 76 (3), 169–172. doi:10.1016/j.jdermsci.2014.11.001
Kim, N., Lee, H., Han, G., Kang, M., Park, S., Kim, D. E., et al. (2023). 3D-Printed functional hydrogel by DNA-induced biomineralization for accelerated diabetic wound healing. Adv. Sci. 10 (17), 2300816. doi:10.1002/advs.202300816
Kim, Y.-J., and Matsunaga, Y. T. (2017). Thermo-responsive polymers and their application as smart biomaterials. J. Mater. Chem. B 5 (23), 4307–4321. doi:10.1039/c7tb00157f
Komi, D. E. A., Khomtchouk, K., and Santa Maria, P. L. (2020). A review of the contribution of mast cells in wound healing: involved molecular and cellular mechanisms. Clin. Rev. allergy and Immunol. 58, 298–312. doi:10.1007/s12016-019-08729-w
Kong, X.-b., Tang, Q.-y., Chen, X.-y., Tu, Y., Sun, S.-z., and Sun, Z.-l. (2017). Polyethylene glycol as a promising synthetic material for repair of spinal cord injury. Neural Regen. Res. 12 (6), 1003–1008. doi:10.4103/1673-5374.208597
Kordestani, S. S. (2019). Atlas of wound healing: a tissue engineering approach. Elsevier Health Sciences.
Kumar, S., Marrero-Berrios, I., Kabat, M., and Berthiaume, F. (2019). Recent advances in the use of algal polysaccharides for skin wound healing. Curr. Pharm. Des. 25 (11), 1236–1248. doi:10.2174/1381612825666190521120051
Lai, J. C.-Y., Lai, H.-Y., Rao, N. K., and Ng, S.-F. (2016). Treatment for diabetic ulcer wounds using a fern tannin optimized hydrogel formulation with antibacterial and antioxidative properties. J. Ethnopharmacol. 189, 277–289. doi:10.1016/j.jep.2016.05.032
Lai, W.-H. (2011). Willingness-to-engage in technology transfer in industry university collaborations. J. Bus. Res. 64 (11), 1218–1223. doi:10.1016/j.jbusres.2011.06.026
Lai, W. H., Siti Murni, M. J., Fauzi, D., Abas Mazni, O., and Saleh, N. M. (2011). Optimal culture conditions for mycelial growth of Lignosus rhinocerus. Mycobiology 39 (2), 92–95. doi:10.4489/myco.2011.39.2.092
Lai, W. H., Zainal, Z., and Daud, F. (2014). Preliminary study on the potential of polysaccharide from indigenous Tiger's Milk mushroom (Lignosus rhinocerus) as anti-lung cancer agent. Pap. Read. A. T. AIP Conf. Proc.
Lanzalaco, S., and Armelin, E. (2017). Poly (N-isopropylacrylamide) and copolymers: a review on recent progresses in biomedical applications. Gels 3 (4), 36. doi:10.3390/gels3040036
Lau, B. F., Abdullah, N., Aminudin, N., Lee, H. B., and Tan, P. J. (2015a). Ethnomedicinal uses, pharmacological activities, and cultivation of Lignosus spp. (tiger׳s milk mushrooms) in Malaysia – a review. J. Ethnopharmacol. 169, 441–458. doi:10.1016/j.jep.2015.04.042
Lee, A. R., Roh, S.-S., and Kim, H.-K. (2018). Anti-microbial activity and anti-inflammatory effects of fucoidan extracts. Asian J. Beauty Cosmetol. 16 (2), 191–200. doi:10.20402/ajbc.2017.0171
Lee, K. Y., and Mooney, D. J. (2001). Hydrogels for tissue engineering. Chem. Rev. 101 (7), 1869–1880. doi:10.1021/cr000108x
Lee, S. S., Chang, Y. S., and Noraswati, M. N. R. (2009). Utilization of macrofungi by some indigenous communities for food and medicine in Peninsular Malaysia. For. Ecol. Manag. 257 (10), 2062–2065. doi:10.1016/j.foreco.2008.09.044
Lee, S. S., Fung, S. Y., Sim, S. M., and Tan, N. H. (2013). “Anti-inflammatory effect of the cultivated sclerotium of Lignosus rhinocerus (Cooke), the tiger milk mushroom,” in Paper read at proceedings of the 38th annual conference of the Malaysian society for biochemistry and molecular biology (kuala lumpur).
Lee, S. S., Tan, N. H., Fung, S. Y., Sim, S. M., Tan, C. S., and Ng, S. T. (2014). Anti-inflammatory effect of the sclerotium of Lignosus rhinocerotis (cooke) ryvarden, the tiger milk mushroom. BMC complementary Altern. Med. 14, 1–8. doi:10.1186/1472-6882-14-359
Lee, S. S., Tan, N. H., Fung, S. Y., Tan, C. S., Ng, S. T., and Sim, S. M. (2012). “Anti-inflammatory activity of Lignosus rhinocerus (tiger milk mushroom) sclerotia,” in Paper read at 18th congress of the international society for mushroom science. Beijing.
Leppiniemi, J., Lahtinen, P., Paajanen, A., Mahlberg, R., Metsa-Kortelainen, S., Pinomaa, T., et al. (2017). 3D-printable bioactivated nanocellulose alginate hydrogels. ACS Appl. Mater. and interfaces 9 (26), 21959–21970. doi:10.1021/acsami.7b02756
Li, Y., Xiao, Z., Zhou, Y., Zheng, S., An, Y., Huang, W., et al. (2019). Controlling the multiscale network structure of fibers to stimulate wound matrix rebuilding by fibroblast differentiation. ACS Appl. Mater. and interfaces 11 (31), 28377–28386. doi:10.1021/acsami.9b06439
Liang, Y., He, J., and Guo, B. (2021). Functional hydrogels as wound dressing to enhance wound healing. ACS nano 15 (8), 12687–12722. doi:10.1021/acsnano.1c04206
Liao, W., Duan, X., Xie, F., Zheng, D., Yang, P., Wang, X., et al. (2023). 3D-bioprinted double-crosslinked angiogenic alginate/chondroitin sulfate patch for diabetic wound healing. Int. J. Biol. Macromol. 236, 123952. doi:10.1016/j.ijbiomac.2023.123952
Liow, S. S., Dou, Q., Kai, D., Karim, A. A., Zhang, K., Xu, F., et al. (2016). Thermogels: in situ gelling biomaterial. ACS Biomater. Sci. Eng. 2, 295–316. doi:10.1021/acsbiomaterials.5b00515
Lone, F. A., Lone, S., Aziz, M. A., and Malla, F. A. (2012). Ethnobotanical studies in the tribal areas of district Kupwara, Kashmir, India. Intl J. Pharma BioScie 3 (4), 399–411.
Maeda, S., Fujimoto, M., Matsushita, T., Hamaguchi, Y., Takehara, K., and Hasegawa, M. (2011). Inducible costimulator (ICOS) and ICOS ligand signaling has pivotal roles in skin wound healing via cytokine production. Am. J. pathology 179 (5), 2360–2369. doi:10.1016/j.ajpath.2011.07.048
Mahmood, A., Malik, R. N., Shinwari, Z. K., and Mahmood, A. (2011). Ethnobotanical survey of plants from Neelum, azad Jammu and Kashmir, Pakistan. Pak. J. Bot. 43 (1), 105–110.
Mak, I. W. C., Chu, C. M., Pan, P. C., Yiu, M. G. C., and Chan, V. L. (2009). Long-term psychiatric morbidities among SARS survivors. General Hosp. psychiatry 31 (4), 318–326. doi:10.1016/j.genhosppsych.2009.03.001
Maksimova, N. V., Lyundup, A. V., Lyubimov, R. O., Mel'nichenko, G. A., and Nikolenko, V. N. (2014). Pathophysiological aspects of wound healing in normal and diabetic foot. Ann. Russ. Acad. Med. Sci. 69 (11-12), 110–117. doi:10.15690/vramn.v69i11-12.1192
Mannarino, C. N., Michelson, K., Jackson, L., Paquette, E., and McBride, M. E. (2020). Post-operative discharge education for parent caregivers of children with congenital heart disease: a needs assessment. Cardiol. Young 30 (12), 1788–1796. doi:10.1017/s1047951120002759
Mohammad, N. S., Milow, P., and Ong, H. C. (2012). Traditional medicinal plants used by the Kensiu tribe of lubuk ulu legong, kedah, Malaysia. Stud. Ethno-medicine 6 (3), 149–153. doi:10.1080/09735070.2012.11886432
Mohanarji, S., Dharmalingam, S., and Kalusalingam, A. (2012). Screening of Lignosus rhinocerus extracts as antimicrobial agents against selected human pathogens. J. Pharm. Biomed. Sci. (JPBMS) 18 (18).
Monirul Islam, M., Hr, V., Durga Bhavani, P., Goudanavar, P. S., Naveen, N. R., Ramesh, B., et al. (2022). Optimization of process parameters for fabrication of electrospun nanofibers containing neomycin sulfate and Malva sylvestris extract for a better diabetic wound healing. Drug Deliv. 29 (1), 3370–3383. doi:10.1080/10717544.2022.2144963
Nallathamby, N., Phan, C.-W., Seow, S. L.-S., Baskaran, A., Lakshmanan, H., Abd Malek, S. N., et al. (2018). A status review of the bioactive activities of tiger milk mushroom Lignosus rhinocerotis (Cooke) Ryvarden. Front. Pharmacol. 8, 998. doi:10.3389/fphar.2017.00998
Naskar, A., and Kim, K.-s. (2020). Recent advances in nanomaterial-based wound-healing therapeutics. Pharmaceutics 12 (6), 499. doi:10.3390/pharmaceutics12060499
Negut, I., Grumezescu, V., and Grumezescu, A. M. (2018). Treatment strategies for infected wounds. Molecules 23 (9), 2392. doi:10.3390/molecules23092392
Nguyen, H. M., Le, T. T. N., Nguyen, A. T., Le, H. N. T., and Pham, T. T. (2023). Biomedical materials for wound dressing: recent advances and applications. RSC Adv. 13 (8), 5509–5528. doi:10.1039/d2ra07673j
Ninan, N., Forget, A., Shastri, V. P., Voelcker, N. H., and Blencowe, A. (2016). Antibacterial and anti-inflammatory pH-responsive tannic acid-carboxylated agarose composite hydrogels for wound healing. ACS Appl. Mater. and interfaces 8 (42), 28511–28521. doi:10.1021/acsami.6b10491
Norahan, M. H., Pedroza-González, S. C., Sánchez-Salazar, M. G., Álvarez, M. M., and de Santiago, G. T. (2023). Structural and biological engineering of 3D hydrogels for wound healing. Bioact. Mater. 24, 197–235. doi:10.1016/j.bioactmat.2022.11.019
Nourian Dehkordi, A., Mirahmadi Babaheydari, F., Chehelgerdi, M., and Raeisi Dehkordi, S. (2019). Skin tissue engineering: wound healing based on stem-cell-based therapeutic strategies. Stem cell Res. and Ther. 10 (1), 111–120. doi:10.1186/s13287-019-1212-2
Okay, O. (2010). General properties of hydrogels. Hydrogel sensors actuators Eng. Technol., 1–14. doi:10.1007/978-3-540-75645-3_1
Okonkwo, U. A., and DiPietro, L. A. (2017). Diabetes and wound angiogenesis. Int. J. Mol. Sci. 18 (7), 1419. doi:10.3390/ijms18071419
Panisi, C., Guerini, F. R., Abruzzo, P. M., Balzola, F., Biava, P. M., Bolotta, A., et al. (2021). Autism spectrum disorder from the womb to adulthood: suggestions for a paradigm shift. J. personalized Med. 11 (2), 70. doi:10.3390/jpm11020070
Pastar, I., Stojadinovic, O., Yin, N. C., Ramirez, H., Nusbaum, A. G., Sawaya, A., et al. (2014). Epithelialization in wound healing: a comprehensive review. Adv. wound care 3 (7), 445–464. doi:10.1089/wound.2013.0473
Patel, M., Lee, H. J., Park, S., Kim, Y., and Jeong, B. (2018). Injectable thermogel for 3D culture of stem cells. Biomaterials 159, 91–107. doi:10.1016/j.biomaterials.2018.01.001
Patil, G. D., Patil, A. H., Jadhav, S. A., Patil, C. R., and Patil, P. S. (2019). A new method to prepare superhydrophobic cotton fabrics by post-coating surface modification of ZnO nanoparticles. Mater. Lett. 255, 126562. doi:10.1016/j.matlet.2019.126562
Pereira, M. M., Silva, J. o. J. P. d., Pinto, M. A., Silva, M. F. a. d., Machado, M. P., Lenzi, H. L., et al. (2005). Experimental leptospirosis in marmoset monkeys (Callithrix jacchus): a new model for studies of severe pulmonary leptospirosis. Am. J. Trop. Med. Hyg. 72, 13–20. doi:10.4269/ajtmh.2005.72.13
Petrovi, P., Vunduk, J., Klaus, A., Carevi, M., Petkovi, M., VukoviÄ, N., et al. (2019). From mycelium to spores: a whole circle of biological potency of mosaic puffball. South Afr. J. Bot. 123, 152–160. doi:10.1016/j.sajb.2019.03.016
Przekora, A. (2020). A concise review on tissue engineered artificial skin grafts for chronic wound treatment: can we reconstruct functional skin tissue in vitro? Cells 9 (7), 1622. doi:10.3390/cells9071622
Qin, Y., Song, D., Chen, H., Cheng, W., Jiang, G., and Cottrell, G. (2017). A dual-stage attention-based recurrent neural network for time series prediction. arXiv Prepr. arXiv:1704.02971, 2627–2633. doi:10.24963/ijcai.2017/366
Rai, B. K., Ayachi, S. S., and Arvinder Rai, A. R. (1993). A note on ethno-myco-medicines from central India. Mycologist 7, 192–193. doi:10.1016/s0269-915x(09)80397-2
Rani, S., and Ritter, T. (2016). The exosome - A naturally secreted nanoparticle and its application to wound healing. Adv. Mater. 28 (27), 5542–5552. doi:10.1002/adma.201504009
Reinke, J. M., and Sorg, H. (2012). Wound repair and regeneration. Eur. Surg. Res. 49 (1), 35–43. doi:10.1159/000339613
Ribeiro, M. P., Morgado, P. I., Miguel, S. P., Coutinho, P., and Correia, I. J. (2013). Dextran-based hydrogel containing chitosan microparticles loaded with growth factors to be used in wound healing. Mater. Sci. Eng. C 33 (5), 2958–2966. doi:10.1016/j.msec.2013.03.025
Richardson, M. D., and Warnock, D. W. (2012). Fungal infection: diagnosis and management. John Wiley and Sons.
Rippon, M., Davies, P., and White, R. (2012). Taking the trauma out of wound care: the importance of undisturbed healing. J. wound care 21 (8), 359–368. doi:10.12968/jowc.2012.21.8.359
Sabaratnam, V., Kah-Hui, W., Naidu, M., and David, P. R. (2013). Neuronal health can culinary and medicinal mushrooms help? J. traditional complementary Med. 3 (1), 62–68. doi:10.4103/2225-4110.106549
Saifullah, Q., and Sharma, A. (2024). Current trends on innovative technologies in topical wound care for advanced healing and management. Current Drug Research Reviews 16 (3), 319–332.
Schrementi, M. E., Ferreira, A. M., Zender, C., and DiPietro, L. A. (2008). Site-specific production of TGF-β in oral mucosal and cutaneous wounds. Wound repair Regen. 16 (1), 80–86. doi:10.1111/j.1524-475x.2007.00320.x
Schultz, G. S., Davidson, J. M., Kirsner, R. S., Bornstein, P., and Herman, I. M. (2011). Dynamic reciprocity in the wound microenvironment. Wound repair Regen. 19 (2), 134–148. doi:10.1111/j.1524-475x.2011.00673.x
Scopelliti, F., Cattani, C., Dimartino, V., Mirisola, C., and Cavani, A. (2022). Platelet derivatives and the immunomodulation of wound healing. Int. J. Mol. Sci. 23 (15), 8370. doi:10.3390/ijms23158370
Scott, N., Hughes, J., Forbes-Haley, C., East, C., Holmes, S., Wilson, E., et al. (2020). Elite and Professional sports facial injuries management a consensus report. Br. J. Oral Maxillofac. Surg. 58 (10), e254–e259. doi:10.1016/j.bjoms.2020.08.046
Seow, A. N., Choong, Y. O., Moorthy, K., and Chan, L. M. (2017). Intention to visit Malaysia for medical tourism using the antecedents of Theory of Planned Behaviour: a predictive model. Int. J. Tour. Res. 19 (3), 383–393. doi:10.1002/jtr.2120
Silva, J. M., Pereira, C. V., Mano, F., Silva, E., Castro, V. n. I. B., Sá-Nogueira, I., et al. (2019). Therapeutic role of deep eutectic solvents based on menthol and saturated fatty acids on wound healing. ACS Appl. Bio Mater. 2 (10), 4346–4355. doi:10.1021/acsabm.9b00598
Solomennikov, A. V., Tyukavin, A. I., and Arseniev, N. A. (2019). The effect of platelet degranulation on the formation of local inflammatory process. J. Med. Biol. Stud. 7, 280–289. doi:10.17238/issn2542-1298.2019.7.3.280
Soltani, R., Haghighat, A., Fanaei, M., and Asghari, G. (2014). Evaluation of the effect of green tea extract on the prevention of gingival bleeding after posterior mandibular teeth extraction: a randomized controlled trial. Evidence-Based Complementary Altern. Med. 2014 (1), 857651. doi:10.1155/2014/857651
Stamets, P., and Zwickey, H. (2014). Medicinal mushrooms: ancient remedies meet modern science. Integr. Med. A Clinician's J. 13 (1), 46–47.
Stern, D., and Cui, H. (2019). Crafting polymeric and peptidic hydrogels for improved wound healing. Adv. Healthc. Mater. 8 (9), 1900104. doi:10.1002/adhm.201900104
Su, J., Li, J., Liang, J., Zhang, K., and Li, J. (2021). Hydrogel preparation methods and biomaterials for wound dressing. Life 11 (10), 1016. doi:10.3390/life11101016
Sum, A. Y. C., Li, X., Yeng, Y. Y. H., Razif, M. F. M., Abd Jamil, A. H., Ting, N. S., et al. (2020). The immunomodulating properties of tiger milk medicinal mushroom, Lignosus rhinocerus TM02® cultivar (agaricomycetes) and its associated carbohydrate composition. Int. J. Med. Mushrooms 22 (8), 803–814. doi:10.1615/intjmedmushrooms.2020035658
Sun, J.-Y., Zhao, X., Illeperuma, W. R. K., Chaudhuri, O., Oh, K. H., Mooney, D. J., et al. (2012). Highly stretchable and tough hydrogels. Nature 489 (7414), 133–136. doi:10.1038/nature11409
Szpaderska, A. M., Walsh, C. G., Steinberg, M. J., and DiPietro, L. A. (2005). Distinct patterns of angiogenesis in oral and skin wounds. J. Dent. Res. 84 (4), 309–314. doi:10.1177/154405910508400403
Szpaderska, A. M., Zuckerman, J. D., and DiPietro, L. A. (2003). Differential injury responses in oral mucosal and cutaneous wounds. J. Dent. Res. 82 (8), 621–626. doi:10.1177/154405910308200810
Tan, C. S., Ng, S. T., Vikineswary, S., Lo, F. P., and Tee, C. S. (2009). “Genetic markers for identification of a Malaysian medicinal mushroom, Lignosus rhinocerus (Cendawan Susu Rimau),” in Paper read at international symposium on molecular markers in horticulture 859.
Tan, W.-C., Kuppusamy, U. R., Phan, C.-W., Tan, Y.-S., Raman, J., Anuar, A. M., et al. (2015). Ganoderma neo-japonicum Imazeki revisited: domestication study and antioxidant properties of its basidiocarps and mycelia. Sci. Rep. 5 (1), 12515. doi:10.1038/srep12515
Thuraisingam, T., Xu, Y. Z., Eadie, K., Heravi, M., Guiot, M.-C., Greemberg, R., et al. (2010). MAPKAPK-2 signaling is critical for cutaneous wound healing. J. investigative dermatology 130 (1), 278–286. doi:10.1038/jid.2009.209
Tsegay, F., Elsherif, M., and Butt, H. (2022). Smart 3D printed hydrogel skin wound bandages: a review. Polymers 14 (5), 1012. doi:10.3390/polym14051012
Ullah, F., Othman, M. B. H., Javed, F., Ahmad, Z., and Akil, H. M. (2015). Classification, processing and application of hydrogels: a review. Mater. Sci. Eng. C 57, 414–433. doi:10.1016/j.msec.2015.07.053
Utech, S., and Boccaccini, A. R. (2016). A review of hydrogel-based composites for biomedical applications: enhancement of hydrogel properties by addition of rigid inorganic fillers. J. Mater. Sci. 51, 271–310. doi:10.1007/s10853-015-9382-5
Vaidya, J. G., and Rabba, A. S. (1993). Fungi in folk medicine. Mycologist 7 (3), 131–133. doi:10.1016/s0269-915x(09)80073-6
Visse, R., and Nagase, H. (2003). Matrix metalloproteinases and tissue inhibitors of metalloproteinases: structure, function, and biochemistry. Circulation Res. 92 (8), 827–839. doi:10.1161/01.res.0000070112.80711.3d
Wang, S., Ong, P. J., Liu, S., Thitsartarn, W., Tan, M. J. B. H., Suwardi, A., et al. (2022). Recent advances in host-guest supramolecular hydrogels for biomedical applications. Chemistry–An Asian J. 17 (18), e202200608. doi:10.1002/asia.202200608
Wei, W., Luo, X., Zheng, L., Yu, M., Jiang, N., Xu, X.-y., et al. (2011). Isolation of a wild Morchella spp. strain and the effects of its extract on ethanol-induced gastric mucosal lesions in rats. Z. für Naturforsch. C 66 (1-2), 0055–0062. doi:10.5560/znc.2011.66c0055
Westby, M. J., Dumville, J. C., Soares, M. O., Stubbs, N., and Norman, G. (2017). Dressings and topical agents for treating pressure ulcers. Cochrane Database Syst. Rev. 6 (6). doi:10.1002/14651858.cd011947.pub2
Xing, R., Liu, Y., Zou, Q., and Yan, X. (2019). Self-assembled injectable biomolecular hydrogels towards phototherapy. Nanoscale 11 (46), 22182–22195. doi:10.1039/c9nr06266a
Xu, L., Chen, Y., Zhang, P., Tang, J., Xue, Y., Luo, H., et al. (2022). 3D printed heterogeneous hybrid hydrogel scaffolds for sequential tumor photothermal-chemotherapy and wound healing. Biomaterials Sci. 10 (19), 5648–5661. doi:10.1039/d2bm00903j
Yang, J. M., Olanrele, O. S., Zhang, X., and Hsu, C. C. (2018). Fabrication of hydrogel materials for biomedical applications. Novel Biomaterials for Regenerative Medicine, 197–224.
Yang, X., Liu, W., Li, N., Wang, M., Liang, B., Ullah, I., et al. (2017). Design and development of polysaccharide hemostatic materials and their hemostatic mechanism. Biomaterials Sci. 5 (12), 2357–2368. doi:10.1039/c7bm00554g
Yang, Y., Wang, X., Yang, F., Shen, H., and Wu, D. (2016). A universal soaking strategy to convert composite hydrogels into extremely tough and rapidly recoverable double-network hydrogels. Adv. Mater. 28 (33), 7178–7184. doi:10.1002/adma.201601742
Yap, H.-Y. Y., Chooi, Y.-H., Firdaus-Raih, M., Fung, S.-Y., Ng, S.-T., Tan, C.-S., et al. (2014). The genome of the Tiger Milk mushroom, Lignosus rhinocerotis, provides insights into the genetic basis of its medicinal properties. BMC genomics 15, 1–12. doi:10.1186/1471-2164-15-635
Yokota, A. (2011). Tropical forests and people's likehood: stalls of traditional medicine vendors in Kota Kinabalu. JIRCAS Newsl. 62, 9–10.
Yuan, N., Shao, K., Huang, S., and Chen, C. (2023). Chitosan, alginate, hyaluronic acid and other novel multifunctional hydrogel dressings for wound healing: a review. Int. J. Biol. Macromol. 240, 124321. doi:10.1016/j.ijbiomac.2023.124321
Zhang, H. J., Sun, T. L., Zhang, A. K., Ikura, Y., Nakajima, T., Nonoyama, T., et al. (2016). Tough physical double network hydrogels based on amphiphilic triblock copolymers. Adv. Mater. 28 (24), 4884–4890. doi:10.1002/adma.201600466
Zhang, K., Wang, Y., Wei, Q., Li, X., Guo, Y., and Zhang, S. (2021). Design and fabrication of sodium alginate/carboxymethyl cellulose sodium blend hydrogel for artificial skin. Gels 7 (3), 115. doi:10.3390/gels7030115
Zhang, T., Wang, X.-F., Wang, Z.-C., Lou, D., Fang, Q.-Q., Hu, Y.-Y., et al. (2020). Current potential therapeutic strategies targeting the TGF-Î2/Smad signaling pathway to attenuate keloid and hypertrophic scar formation. Biomed. and Pharmacother. 129, 110287. doi:10.1016/j.biopha.2020.110287
Keywords: anti-inflammatory hydrogel, wound dressings, Lignosus rhinoceros, wound healing, wound
Citation: Arbab S, Ullah H, Muhammad N, Wang W and Zhang J (2024) Latest advance anti-inflammatory hydrogel wound dressings and traditional Lignosus rhinoceros used for wound healing agents. Front. Bioeng. Biotechnol. 12:1488748. doi: 10.3389/fbioe.2024.1488748
Received: 30 August 2024; Accepted: 15 November 2024;
Published: 28 November 2024.
Edited by:
Jinjian Huang, Nanjing University, ChinaReviewed by:
Yanhan Ren, University of Massachusetts Medical School, United StatesCopyright © 2024 Arbab, Ullah, Muhammad, Wang and Zhang. This is an open-access article distributed under the terms of the Creative Commons Attribution License (CC BY). The use, distribution or reproduction in other forums is permitted, provided the original author(s) and the copyright owner(s) are credited and that the original publication in this journal is cited, in accordance with accepted academic practice. No use, distribution or reproduction is permitted which does not comply with these terms.
*Correspondence: Jiyu Zhang, aW5memp5QHNpbmEuY29t
Disclaimer: All claims expressed in this article are solely those of the authors and do not necessarily represent those of their affiliated organizations, or those of the publisher, the editors and the reviewers. Any product that may be evaluated in this article or claim that may be made by its manufacturer is not guaranteed or endorsed by the publisher.
Research integrity at Frontiers
Learn more about the work of our research integrity team to safeguard the quality of each article we publish.