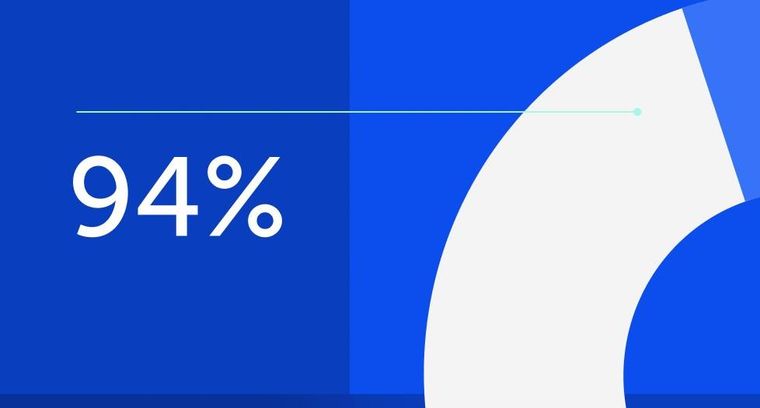
94% of researchers rate our articles as excellent or good
Learn more about the work of our research integrity team to safeguard the quality of each article we publish.
Find out more
MINI REVIEW article
Front. Bioeng. Biotechnol., 06 August 2024
Sec. Biomaterials
Volume 12 - 2024 | https://doi.org/10.3389/fbioe.2024.1454247
This article is part of the Research TopicMicro-Nano-Materials for Drug Delivery, Disease Diagnosis, and Therapeutic TreatmentView all 4 articles
Ionic liquids (ILs) represent an exciting and promising solution for advancing drug delivery platforms. Their unique properties, including broad chemical diversity, adaptable structures, and exceptional thermal stability, make them ideal candidates for overcoming challenges in transdermal drug delivery. Despite encountering obstacles such as side reactions, impurity effects, biocompatibility concerns, and stability issues, ILs offer substantial potential in enhancing drug solubility, navigating physiological barriers, and improving particle stability. To propel the use of IL-based drug delivery in pharmaceutical innovation, it is imperative to devise new strategies and solvents that can amplify drug effectiveness, facilitate drug delivery to cells at the molecular level, and ensure compatibility with the human body. This review introduces innovative methods to effectively address the challenges associated with transdermal drug delivery, presenting progressive approaches to significantly improve the efficacy of this drug delivery system.
ILs have shown significant potential in drug delivery, particularly for enhancing transdermal delivery. Their ionic composition influences their ability to increase skin permeability. ILs are considered environmentally friendly, stable, non-volatile, and non-flammable, making them preferable to conventional solvents. Because of their unique and customizable physical, chemical, and biological properties, ILs are valuable as cosolvents and materials in drug delivery systems and active pharmaceutical ingredient (API) formulations. ILs can be customized in formulations to enhance solubility and stability, thus improving drug bioavailability. Their properties enable the development of innovative delivery methods and can potentially increase treatments’ effectiveness and safety. Furthermore, the, non-toxic, and biodegradable nature of certain ILs underscores their suitability for pharmaceutical use, ensuring minimal environmental impact and better patient compliance. As a result, ILs show great potential for the advancement of drug delivery systems (Lu et al., 2022). ILs can create nanoparticles and micelles, which can deliver therapeutic agents to specific areas of the body. This method increases the effectiveness of drugs and reduces potential side effects. Additionally, ILs can be utilized to produce solid dispersions, significantly improving the solubility and bioavailability of poorly water-soluble drugs (Kapre et al., 2024). This improvement allows better drug absorption and distribution, ultimately leading to better therapeutic results. Consequently, ILs play a vital role in developing new pharmaceutical products, contributing to the creation of treatments that are not only more effective but also safer for patients (Moshikur et al., 2021; Shukla et al., 2023).
ILs have been categorized into three generations based on their discovery timeline and evolving properties. The development of ILs has progressed from the first to the third generation, each characterized by distinct chemical structures and applications. The first generation of ILs, primarily utilized in electroplating, involved the combination of dialkyl imidazolium and alkylpyridinium cations with metal halide anions. These ILs exhibited unique physical properties such as high thermal stability, low melting points, and broad liquid ranges, making them suitable substitutes for certain organic solvents. However, they were hindered by low biodegradability, high aquatic toxicity, and high production costs. The second generation of ILs, which are stable in both water and air, was synthesized using cations such as dialkyl imidazolium, alkylpyridinium, ammonium, and phosphonium, and anions such as tetrafluoroborate and hexafluorophosphate (Brahma et al., 2024). These ILs presented customizable physical and chemical properties, including melting point, viscosity, thermal stability, hydrophilicity, solubility, toxicity, and biodegradability, accomplished by fine-tuning and modifying their anions, cations, and substituents (Magina et al., 2021). Second-generation ILs in drug delivery systems face significant challenges, including toxicity, biocompatibility issues, regulatory barriers, and environmental concerns (Moshikur et al., 2020; Shukla et al., 2023). Formulation and compatibility complexities also limit their use (Amaral et al., 2021). The third generation of ILs introduced natural sources for anions (e.g., amino acids, fatty acids) and cations (e.g., choline). These ILs maintained promising physical and chemical properties and provided reduced toxicity and enhanced biodegradability. The breakthrough of third-generation ILs has prompted increasing research into their applications in biomedicine, including transdermal drug delivery systems, due to their improved safety profiles and environmental compatibility. Figure 1 illustrates the structures and key benefits of third-generation of ionic liquids.
The role of ILs as chemical permeation enhancers is well-documented. ILs have been shown to effectively facilitate transcellular and paracellular transport, bypassing the stratum corneum (SC) by disrupting cell integrity, fluidizing lipids, creating diffusional pathways, and extracting lipid components from the SC. These properties of ILs undoubtedly promise to improve efficiency in transdermal drug delivery, leading to more effective treatments and better patient outcomes (Egorova et al., 2017; Kundu et al., 2017). Room-temperature ionic liquids (RTILs) are highly adaptable for various applications, mainly as chemical permeation enhancers in transdermal drug delivery. They enhance the transport of substances through cells and cellular barriers by disrupting cellular integrity, fluidizing lipids, creating diffusional pathways, and extracting lipids from the outermost layer. This remarkable adaptability significantly improves the performance of transdermal drug delivery systems, resulting in better clinical outcomes (Sidat et al., 2019). Moshikur et al. conducted an in-depth review of strategies for designing and developing ILs, with particular emphasis on biocompatible IL-based formulations and delivery systems. They highlighted the applications and advantages of ILs over traditional organic solvents in drug formulations and delivery mechanisms (Moshikur et al., 2023). Sidat et al. (2019) conducted a detailed review of the potential of biodegradable ILs to advance TDDS. They discussed ILs’ roles as permeation enhancers, drug modification agents, and active pharmaceutical ingredients, suggesting their synergistic use with other chemical permeation enhancers. Additionally, the review addressed the challenges associated with ILs in TDDS, such as the physical properties of ILs, Interactions of ILs with Biomolecules and Membranes, and the impact of permeation enhancement. Shukla et al. (2023) reviewed the expanding role of ILs in drug delivery systems, emphasizing their potential to enhance the solubility of poorly soluble drugs. They highlighted ILs’ customizable properties and recent advancements, showcasing their growing importance in pharmaceutical and biomedical applications. In another literature, Ali et al. (2022) explored ILs for drug delivery and highlighted their tunable properties that address issues with traditional permeation enhancers. They focused on biocompatible IL-based systems using advanced cations and anions to improve permeability and reduce cytotoxicity and skin irritation, showcasing recent advancements and promising applications in drug delivery. This mini-review differs from the aforementioned literature by specifically addressing the challenges related to the use of ILs in TDDS and highlighting innovative approaches to address these challenges. This also focuses on integrating ILs with novel drug delivery carriers to improve their applicability and increase therapeutic potential via the transdermal route. This approach aims to provide a comprehensive understanding of the advances in this field while filling existing gaps and suggesting future directions for research and development in IL-based transdermal drug delivery systems. Figure 2 illustrates the potential application of ILs in transdermal delivery.
The skin permeability of ILs is mainly affected by their physical and chemical properties, which in turn depend on the structures. However, the transdermal route of drug delivery is challenged by the impenetrable nature of SC; hence, achieving optimum permeability remains an issue (Paz Ramos et al., 2020). Significant challenges in using ILs to improve skin permeability include possible skin irritation and toxicity due to their disruptive effects on the skin barrier (Leitch et al., 2020); the cation predominantly influences the skin irritability of ILs; however, the anion also contributes to this effect (Patil and Sasai, 2008). ILs containing alicyclic cations and ammonium cations, such as morpholinium and pyrrolidinium, exhibit lower toxicity and skin irritability than those based on imidazolium and pyridinium cations (Zhang et al., 2006). The variability in efficacy and safety among different ILs requires extensive screening and optimization, which is resource-intensive. Ensuring that ILs are nontoxic, biodegradable, and environmentally friendly while maintaining efficacy is complex. Additionally, the stability of the formulation and compatibility with other pharmaceutical ingredients add to the challenges of developing effective transdermal delivery systems. To overcome the challenge of transdermal delivery of paclitaxel (PTX) caused by its low water solubility, a micelle formulation (MF) was developed using biocompatible surfactants, cholinium oleate ([Cho][Ole]) and sorbitan monolaurate (Span-20). This formulation effectively improved the solubility and stability. Stable micelles formed significantly increased the absorption of PTX. According to Figure 3IA, the transdermal permeation profiles of various formulations showed the superior performance of SAIL-based MF in delivering higher rates of PTX compared to other carriers. Figure 3IB illustrates the total PTX delivery from different formulations over 48 h, with the SAIL-based MF showing a markedly higher delivery efficiency, which confirms its effectiveness in enhancing the transdermal permeation of PTX (Ali et al., 2021). Another study reported ILs as TDD for the treatment of rheumatoid arthritis. At the same time, another study introduced a new transdermal IL patch, SIHDD-PSA, utilizing semi-ionic hydrogen bonding to enhance the delivery of Actarit and Ketoprofen for rheumatoid arthritis treatment. By forming a complex with triethylamine, the ILs improved the solubility and permeability, significantly increasing Actarit loading by approximately 11.34 times and enhancing in vitro permeability by 5.46 times, with similar improvements for Ketoprofen, i.e., 2.39 times increase in permeability. This approach addresses the challenge of skin barrier permeation. It mitigates gastrointestinal side effects associated with oral drug delivery, demonstrating a promising advancement in transdermal therapeutic systems through in vitro and in vivo evaluations (Zhang et al., 2024).
Figure 3. [I] (A) Transdermal permeation profiles of PTX delivered by various MFs with different SAIL/co-surfactant ratios (S/Co); (B) total PTX delivery by various MFs with different S/Co. {adapted with permission from Ali et al. (Ali et al., 2021). Copyright {2021} American Chemical Society.} [II] (A) Cell viability on the human LabCyte EPI-MODEL. (B) Body weight of mouse during the in vivo biocompatibility study and (C) skin histopathological experiment {adapted with permission from Uddin et al. (Uddin et al., 2021). Copyright {2021} American Chemical Society}; [III] Cytotoxicity for ILs with anion and different cations (red left side) and ILs with cation and various anions (blue right side) (adapted from Musial et al. (Musiał et al., 2021), CC-BY 4.0 license).
ILs are widely used in pharmaceuticals and require long-term biocompatibility and safety for patients. First-generation ILs have attracted attention for their unique physical properties; They are sensitive to water and air and have poor biodegradability (Zhuo et al., 2024). ILs can have biocompatibility issues, including toxicity and low biodegradability. These issues can pose challenges to their safety in various applications. However, biocompatible ILs (Bio-ILs) have been developed as a greener alternative to conventional ILs and organic/inorganic solvents (Kanjilal et al., 2023; Zhuo et al., 2024). The biodegradability of ILs is determined by their molecular structure, including anions, cations, and functional groups, and is typically measured concerning these components (Elmobarak et al., 2023). A study investigates lipid-based ionic liquids (LBILs) as carriers for TDDS and demonstrated their biocompatibility. The LBIL-based formulations, especially those using [EDMPC] [linoleate]/O-NDs, showed excellent biocompatibility in both in vitro and in vivo cytotoxicity studies. These formulations did not cause a significant reduction in cell viability or adverse skin reactions Figure 3IIA, B, C, suggesting that they are safe for pharmaceutical use. The results highlight the potential of LBILs as promising biocompatible carriers to improve the transdermal delivery of the peptide drug (Uddin et al., 2021). Another study of biocompatible ILs addresses challenges such as low solubility and poor skin penetration of therapeutic agents. By incorporating surface-active ILs, the study improved the skin permeation and stability of antigenic proteins and adjuvants, demonstrating improved biocompatibility. Preclinical studies showed that the IL-assisted delivery systems (ILDS) were nonirritant and safe for topical applications. ILDS significantly enhanced antigenic protein delivery and immunogenic response, making them promising candidates for transdermal drug delivery and immunotherapy applications (Chowdhury et al., 2021).
Impurities in ILs are crucial, especially their influence on properties such as viscosity. Even minor impurities such as water and halides can significantly alter the properties of the IL. However, less attention has been paid to the third generation of biocompatible ILs to trace contaminants and their biological interactions (Le Donne and Bodo, 2021). Accurate characterization and quantification of contaminants are critical as they affect material properties and biological safety. Techniques such as Karl Fischer titration, HPLC, and Volhard titration (for halides) are used to measure impurities. Improved synthetic methods can reduce impurities by using precursors that yield easily removable by-products, such as water and carbon dioxide, resulting in pure ILs (Stark et al., 2008; Curreri et al., 2021). The impact of impurities on hydrophilic ILs used in gel-based dermal formulations emphasizes that contaminants such as organic materials, halides, and protic residues from incomplete reactions significantly influence the properties and effectiveness. These impurities can drastically alter pH levels, reduce antimicrobial activity, and affect formulation stability by changing viscosity and phase behavior. Such changes are crucial because they can compromise the delivery efficiency and safety of drug substances in dermal applications, emphasizing the need for rigorous control over the purity of IL in pharmaceutical formulations for TDD (Dobler et al., 2016).
Toxicity is a crucial factor in evaluating ILs, as earlier generations had high toxicity, making them unsuitable for medical applications. Furthermore, the structural properties of the cations in the ILs influence their toxicity. ILs containing cations such as ammonium, imidazolium, morpholinium, piperidinium, and pyrrolidinium are often associated with high toxicity and reduced degradability (Mano et al., 2020; Miao et al., 2022). The presence of hydrophilic anions such as chloride, bromide, and organic groups such as carboxylates and sulfonates reduces the overall toxicity of IL, indicating a significant impact on their safety (Flieger and Flieger, 2020; Cho et al., 2021; Chen et al., 2024). Including a polar functional group within the alkyl chain has been shown to reduce both the toxicity and the biodegradation efficiency of ILs. This suggests the possibility of modifying ILs by integrating particular functional groups into their structure, resulting in a more eco-friendly product (Navti et al., 2022). As the lipophilicity or length of the alkyl chain increases, there is an increase in both degradation rate and toxicity, furthermore, from the perspectives of toxicology and microbial degradation (Pham et al., 2010). ILs with polar ether, hydroxyl, and nitrile groups on their side chains show lower cytotoxicity than those with essential alkyl chains (Thuy et al., 2010). The concept of ILs as green chemicals is based on their non-volatile and non-flammable nature, which distinguishes them from conventional organic solvents. Replacing volatile organic solvents with non-volatile ILs in chemical and biological processes can mitigate the risks of inhalation exposure, flammability, and environmental contamination. However, more extensive studies on toxicity and biocompatibility are required to confirm that ILs are intrinsically safe and environmentally friendly. Research shows numerous ILs pose risks to various biological entities, from nucleic acids to multicellular organisms (Agatemor et al., 2018; Shukla et al., 2023). Bioinspired ILs have been formulated to exhibit improved biodegradability and a lower toxicity profile to address this challenge. Among these, choline-based ILs are the most extensively researched (Agatemor et al., 2018; Sidat et al., 2019). A study evaluated the cytotoxicity of 31 ILs in human dermal fibroblasts. The results showed that imidazolium-based ILs with dialkyl phosphate or ethyl sulfate anions had the lowest cytotoxicity. In particular, 1,3-diethyl imidazolium ethyl sulfate is promising as a hydraulic fluid, like the commercial 1-ethyl-3-methylimidazolium ethyl sulfate. Dialkyl phosphate-based ILs proved to be effective as solvents for lignocellulose biomass and eco-friendly bioplastic extractants. Moreover, pyrrolidinium and cyano-based ILs, used in heat transfer and nanofluids, also exhibited lower toxicity compared to other ILs. The NHDF cell line was used to assess IC50 values of ILs, with findings presented in Figure 3III (Musiał et al., 2021). A recent study investigates the safety and suitability for the biological use of newly created double-charged ILs. These ILs, including ibuprofen and piroxicam, are specifically formulated to improve the dissolution of drugs with low solubility. These ILs, which are composed of carboxylic anions and ammonium cations, significantly increased the solubility of drugs in both water and phosphate buffer solutions. This indicates promising potential for use in drug delivery systems. The evaluation of cytotoxicity using fibroblast L929 cells showed that most of these ILs had low toxicity, with IC50 values higher than 100 mM, demonstrating good biocompatibility (Agostinho et al., 2021).
Stability is a prerequisite for the use of ILs. ILs can be used at elevated temperatures, as electrolytes, or under irradiation. Therefore, the thermal, electrochemical, and radiolytic stabilities of ILs are essential and need to be known before their use. The decomposition temperature of ILs, which ranges from 200°C to 400°C, is strongly influenced by the type of anion, highlighting its crucial role in thermal stability. In addition to structural factors such as the type and modification, this stability is also related to the coordination ability of the anion, its nucleophilicity, and hydrophilicity, alongside structural factors like cation type and alterations of the cation (Cao and Mu, 2014). Although ILs are generally non-flammable, they are susceptible to thermal decomposition. When exposed to high temperatures during processes, these changes can cause ILs to degrade structurally, potentially producing undesirable by-products and leading to accidents (He et al., 2021).
In our discussion, we have explored the various complexities associated with IL-based transdermal delivery. However, a range of emerging advancements are specifically designed to address these challenges. These cutting-edge approaches are summarized in detail in Table 1.
ILs represent an exciting area of research in targeted drug delivery because of their remarkable structural versatility and accessible coupling properties. The ability to modify IL properties for various biological applications underscores their potential to enhance drug permeability across physiological barriers, increase the solubility of poorly soluble drugs, and develop innovative IL-based drug delivery formulations. The effectiveness of ILs as solvent media, solubility or permeation enhancers, and potential drug delivery carriers has sparked significant interest in drug delivery. Moreover, the integration of ILs with nanostructures has marked a significant advancement. The combination of ILs with nanoparticles not only enhances stability, activity, and performance and enables precise control over size and morphology. This synergy is valuable in drug delivery applications and has the potential to lead to superior therapeutic outcomes. The development of combined IL-nanostructures drug-delivery systems holds the promise of expanding pharmaceutical applications and improving therapeutic efficiency. Future research must prioritize critical areas to unleash the potential of ILs in drug delivery. Additional efforts are crucial in fine-tuning the properties of ILs to maximize their efficacy as drug delivery systems. This involves exploring new IL compositions and combinations to enhance their biocompatibility and therapeutic effectiveness. Additionally, comprehensive investigations of the safety and toxicity of ILs to address potential side effects. Understanding the long-term impact of IL exposure on human health will be pivotal to their clinical implementation. The transition of IL-based drug delivery systems from research to clinical use will be facilitated by formulating precise regulatory guidelines for their approval. Thorough clinical trials will be indispensable to confirm IL-based formulations’ safety and therapeutic benefits for extensive use in medical practice. Additionally, further research is vital to combine ILs with nanotechnology to leverage the synergistic benefits fully. This includes developing innovative IL-nanotechnology structures tailored to specific therapeutic applications. By addressing these areas, the field of IL-based drug delivery can make strides in developing highly efficient, safe, and therapeutic options that have the potential to revolutionize drug delivery and improve patient outcomes.
AJ: Conceptualization, Formal Analysis, Writing–review and editing, Data curation, Writing–original draft. AS: Funding acquisition, Resources, Supervision, Writing–original draft, Writing–review and editing. SP: Data curation, Investigation, Writing–original draft, Writing–review and editing. ME: Formal Analysis, Methodology, Writing–review and editing, Funding acquisition. NM: Formal Analysis, Writing–review and editing, Data curation, Methodology, Writing–original draft. SJ: Formal Analysis, Investigation, Supervision, Writing–review and editing. RN: Formal Analysis, Writing–review and editing, Data curation, Investigation. UP: Formal Analysis, Investigation, Resources, Writing–review and editing.
The author(s) declare that financial support was received for the research, authorship, and/or publication of this article. APC (in part) is supported by the Deanship of University Scientific Research, Al-Ahliyya Amman University, Amman, Jordan, and Researchers Supporting Project, AlMaarefa University, Riyadh, Saudi Arabia.
The authors would like to express their gratitude to the Department of Pharmacy, Birla Institute of Science and Technology-Pilani; Institute of Pharmaceutical Research, GLA University, Mathura; Department of Basic Medical Sciences, College of Medicine, AlMaarefa University, Riyadh, Saudi Arabia, Department of Pharmaceutical Sciences, Dr. Harisingh Gour University, Sagar, India; Dean, Faculty of Pharmacy, Allied Medical Sciences and Scientific Research at Al-Ahliyya Amman University, Amman, Jordan, for their support and required facilities.
The authors declare that the research was conducted in the absence of any commercial or financial relationships that could be construed as a potential conflict of interest.
All claims expressed in this article are solely those of the authors and do not necessarily represent those of their affiliated organizations, or those of the publisher, the editors and the reviewers. Any product that may be evaluated in this article, or claim that may be made by its manufacturer, is not guaranteed or endorsed by the publisher.
Agatemor, C., Ibsen, K. N., Tanner, E. E. L., and Mitragotri, S. (2018). Ionic liquids for addressing unmet needs in healthcare. Bioeng. Transl. Med. 3 (1), 7–25. doi:10.1002/btm2.10083
Agostinho, D. A., Santos, F., Esperança, J. M., Duarte, A. R., and Reis, P. M. (2021). New non-toxic biocompatible dianionic ionic liquids that enhance the solubility of oral drugs from BCS class II. J. Ionic Liq. 1 (1), 100003. doi:10.1016/j.jil.2021.100003
Ali, M. K., Moshikur, R. M., Goto, M., and Moniruzzaman, M. (2022). Recent developments in ionic liquid-assisted topical and transdermal drug delivery. Pharm. Res. 39 (10), 2335–2351. doi:10.1007/s11095-022-03322-x
Ali, M. K., Moshikur, R. M., Wakabayashi, R., Moniruzzaman, M., and Goto, M. (2021). Biocompatible ionic liquid-mediated micelles for enhanced transdermal delivery of paclitaxel. ACS Appl. Mater. Interfaces 13 (17), 19745–19755. doi:10.1021/acsami.1c03111
Amaral, M., Pereiro, A. B., Gaspar, M. M., and Reis, C. P. (2021). Recent advances in ionic liquids and nanotechnology for drug delivery. Nanomedicine 16 (1), 63–80. doi:10.2217/nnm-2020-0340
Brahma, S., and Gardas, R. L. (2024). “History and development of ionic liquids,” in Handbook of ionic liquids: fundamentals, applications, and sustainability. Editors S. Rajkhowa, P. Singh, A. Sen, and J. Sarma (John Wiley and Sons), 1–28.
Cao, Y., and Mu, T. (2014). Comprehensive investigation on the thermal stability of 66 ionic liquids by thermogravimetric analysis. Industrial Eng. Chem. Res. 53 (20), 8651–8664. doi:10.1021/ie5009597
Chen, X., Li, Z., Yang, C., and Yang, D. (2024). Ionic liquids as the effective technology for enhancing transdermal drug delivery: design principles, roles, mechanisms, and future challenges. Asian J. Pharm. Sci. 19, 100900. doi:10.1016/j.ajps.2024.100900
Cho, C.-W., Pham, T. P. T., Zhao, Y., Stolte, S., and Yun, Y.-S. (2021). Review of the toxic effects of ionic liquids. Sci. Total Environ. 786, 147309. doi:10.1016/j.scitotenv.2021.147309
Chowdhury, M. R., Moshikur, R. M., Wakabayashi, R., Moniruzzaman, M., and Goto, M. (2021). Biocompatible ionic liquids assisted transdermal co-delivery of antigenic protein and adjuvant for cancer immunotherapy. Int. J. Pharm. 601, 120582. doi:10.1016/j.ijpharm.2021.120582
Curreri, A. M., Mitragotri, S., and Tanner, E. E. (2021). Recent advances in ionic liquids in biomedicine. Adv. Sci. 8 (17), 2004819. doi:10.1002/advs.202004819
Dinh, L., Lee, S., Abuzar, S. M., Park, H., and Hwang, S.-J. (2022). Formulation, preparation, characterization, and evaluation of dicarboxylic ionic liquid donepezil transdermal patches. Pharmaceutics 14 (1), 205. doi:10.3390/pharmaceutics14010205
Dobler, D., Schmidts, T., Zinecker, C., Schlupp, P., Schäfer, J., and Runkel, F. (2016). Hydrophilic ionic liquids as ingredients of gel-based dermal formulations. Aaps Pharmscitech 17, 923–931. doi:10.1208/s12249-015-0421-y
Egorova, K. S., Gordeev, E. G., and Ananikov, V. P. (2017). Biological activity of ionic liquids and their application in pharmaceutics and medicine. Chem. Rev. 117 (10), 7132–7189. doi:10.1021/acs.chemrev.6b00562
Elmobarak, W. F., Almomani, F., Tawalbeh, M., Al-Othman, A., Martis, R., and Rasool, K. (2023). Current status of CO2 capture with ionic liquids: development and progress. Fuel 344, 128102. doi:10.1016/j.fuel.2023.128102
Flieger, J., and Flieger, M. (2020). Ionic liquids toxicity—benefits and threats. Int. J. Mol. Sci. 21 (17), 6267. doi:10.3390/ijms21176267
He, H., Pan, Y., Meng, J., Li, Y., Zhong, J., Duan, W., et al. (2021). Predicting thermal decomposition temperature of binary imidazolium ionic liquid mixtures from molecular structures. ACS omega 6 (20), 13116–13123. doi:10.1021/acsomega.1c00846
Islam, M. R., Chowdhury, M. R., Wakabayashi, R., Kamiya, N., Moniruzzaman, M., and Goto, M. (2020a). Ionic liquid-in-oil microemulsions prepared with biocompatible choline carboxylic acids for improving the transdermal delivery of a sparingly soluble drug. Pharmaceutics 12 (4), 392. doi:10.3390/pharmaceutics12040392
Islam, M. R., Chowdhury, M. R., Wakabayashi, R., Tahara, Y., Kamiya, N., Moniruzzaman, M., et al. (2020b). Choline and amino acid based biocompatible ionic liquid mediated transdermal delivery of the sparingly soluble drug acyclovir. Int. J. Pharm. 582, 119335. doi:10.1016/j.ijpharm.2020.119335
Islam, M. R., Uddin, S., Chowdhury, M. R., Wakabayashi, R., Moniruzzaman, M., and Goto, M. (2021). Insulin transdermal delivery system for diabetes treatment using a biocompatible ionic liquid-based microemulsion. ACS Appl. Mater. Interfaces 13 (36), 42461–42472. doi:10.1021/acsami.1c11533
Islam, R., Nabila, F. H., Wakabayashi, R., Kamiya, N., Moniruzzaman, M., and Goto, M. (2024). Ionic Liquid-Based patch formulation for enhanced transdermal delivery of sparingly soluble drug. J. Mol. Liq. 397, 124184. doi:10.1016/j.molliq.2024.124184
Kandasamy, S., Moniruzzaman, M., Sivapragasam, M., Shamsuddin, M. R., and Mutalib, M. I. A. (2018). Formulation and characterization of acetate based ionic liquid in oil microemulsion as a carrier for acyclovir and methotrexate. Sep. Purif. Technol. 196, 149–156. doi:10.1016/j.seppur.2017.08.044
Kanjilal, B., Zhu, Y., Krishnadoss, V., Unagolla, J. M., Saemian, P., Caci, A., et al. (2023). Bioionic liquids: enabling a paradigm shift toward advanced and smart biomedical applications. Adv. Intell. Syst. 5 (5), 2200306. doi:10.1002/aisy.202200306
Kapre, S., Palakurthi, S. S., Jain, A., and Palakurthi, S. (2024). DES-igning the future of drug delivery: a journey from fundamentals to drug delivery applications. J. Mol. Liq. 400, 124517. doi:10.1016/j.molliq.2024.124517
Kundu, N., Roy, S., Mukherjee, D., Maiti, T. K., and Sarkar, N. (2017). Unveiling the interaction between fatty-acid-modified membrane and hydrophilic imidazolium-based ionic liquid: understanding the mechanism of ionic liquid cytotoxicity. J. Phys. Chem. B 121 (34), 8162–8170. doi:10.1021/acs.jpcb.7b06231
Le Donne, A., and Bodo, E. (2021). Cholinium amino acid-based ionic liquids. Biophys. Rev. 13 (1), 147–160. doi:10.1007/s12551-021-00782-0
Leitch, A. C., Abdelghany, T. M., Probert, P. M., Dunn, M. P., Meyer, S. K., Palmer, J. M., et al. (2020). The toxicity of the methylimidazolium ionic liquids, with a focus on M8OI and hepatic effects. Food Chem. Toxicol. 136, 111069. doi:10.1016/j.fct.2019.111069
Lu, B., Liu, T., Wang, H., Wu, C., Chen, H., Liu, Z., et al. (2022). Ionic liquid transdermal delivery system: progress, prospects, and challenges. J. Mol. Liq. 351, 118643. doi:10.1016/j.molliq.2022.118643
Magina, S., Barros-Timmons, A., Ventura, S. P., and Evtuguin, D. V. (2021). Evaluating the hazardous impact of ionic liquids–challenges and opportunities. J. Hazard. Mater. 412, 125215. doi:10.1016/j.jhazmat.2021.125215
Mahamat Nor, S. B., Woi, P. M., and Ng, S. H. (2017). Characterisation of ionic liquids nanoemulsion loaded with piroxicam for drug delivery system. J. Mol. Liq. 234, 30–39. doi:10.1016/j.molliq.2017.03.042
Mano, B., Jesus, F., Gonçalves, F. J., Ventura, S. P., and Pereira, J. L. (2020). Applicability of heuristic rules defining structure–ecotoxicity relationships of ionic liquids: an integrative assessment using species sensitivity distributions (SSD). Green Chem. 22 (18), 6176–6186. doi:10.1039/d0gc02486d
Miao, S., Atkin, R., and Warr, G. (2022). Design and applications of biocompatible choline amino acid ionic liquids. Green Chem. 24 (19), 7281–7304. doi:10.1039/d2gc02282f
Moshikur, R. M., Carrier, R. L., Moniruzzaman, M., and Goto, M. (2023). Recent advances in biocompatible ionic liquids in drug formulation and delivery. Pharmaceutics 15 (4), 1179. doi:10.3390/pharmaceutics15041179
Moshikur, R. M., Chowdhury, M. R., Moniruzzaman, M., and Goto, M. (2020). Biocompatible ionic liquids and their applications in pharmaceutics. Green Chem. 22 (23), 8116–8139. doi:10.1039/d0gc02387f
Moshikur, R. M., and Goto, M. (2021). “Ionic liquids as active pharmaceutical ingredients (APIs),” in Application of ionic liquids in drug delivery. Editors M. Goto, and M. Moniruzzaman (Singapore: Springer Singapore), 13–33.
Musiał, M., Zorębski, E., Malarz, K., Kuczak, M., Mrozek-Wilczkiewicz, A., Jacquemin, J., et al. (2021). Cytotoxicity of ionic liquids on normal human dermal fibroblasts in the context of their present and future applications. ACS Sustain. Chem. Eng. 9 (22), 7649–7657. doi:10.1021/acssuschemeng.1c02277
Nabila, F. H., Islam, R., Shimul, I. M., Moniruzzaman, M., Wakabayashi, R., Kamiya, N., et al. (2024). Ionic liquid-mediated ethosome for transdermal delivery of insulin. Chem. Commun. 60 (30), 4036–4039. doi:10.1039/d3cc06130b
Navti, P. D., Pandey, A., Nikam, A. N., Padya, B. S., Kalthur, G., Koteshwara, K. B., et al. (2022). Ionic liquids assisted topical drug delivery for permeation enhancement: formulation strategies, biomedical applications, and toxicological perspective. AAPS PharmSciTech 23 (5), 161. doi:10.1208/s12249-022-02313-w
Niesyto, K., Keihankhadiv, S., Mazur, A., Mielańczyk, A., and Neugebauer, D. (2024a). Ionic liquid-based polymer matrices for single and dual drug delivery: impact of structural topology on characteristics and in vitro delivery efficiency. Int. J. Mol. Sci. 25 (2), 1292. doi:10.3390/ijms25021292
Niesyto, K., Mazur, A., and Neugebauer, D. (2024b). Piperacillin/tazobactam Co-delivery by micellar ionic conjugate systems carrying pharmaceutical anions and encapsulated drug. Pharmaceutics 16 (2), 198. doi:10.3390/pharmaceutics16020198
Pansuriya, R., Patel, T., Kumar, S., Aswal, V. K., Raje, N., Hoskins, C., et al. (2024). Multifunctional ionic hydrogel-based transdermal delivery of 5-fluorouracil for the breast cancer treatment. ACS Appl. Bio Mater. 7 (5), 3110–3123. doi:10.1021/acsabm.4c00152
Patil, M. L., and Sasai, H. (2008). Recent developments on chiral ionic liquids: design, synthesis, and applications. Chem. Rec. 8 (2), 98–108. doi:10.1002/tcr.20143
Paz Ramos, A., Gooris, G., Bouwstra, J., Molinari, M., and Lafleur, M. (2020). Raman and AFM-IR chemical imaging of stratum corneum model membranes. Can. J. Chem. 98 (9), 495–501. doi:10.1139/cjc-2019-0471
Pham, T. P. T., Cho, C.-W., and Yun, Y.-S. (2010). Environmental fate and toxicity of ionic liquids: a review. Water Res. 44 (2), 352–372. doi:10.1016/j.watres.2009.09.030
Shukla, M. K., Tiwari, H., Verma, R., Dong, W. L., Azizov, S., Kumar, B., et al. (2023). Role and recent advancements of ionic liquids in drug delivery systems. Pharmaceutics 15 (2), 702. doi:10.3390/pharmaceutics15020702
Sidat, Z., Marimuthu, T., Kumar, P., du Toit, L. C., Kondiah, P. P. D., Choonara, Y. E., et al. (2019). Ionic liquids as potential and synergistic permeation enhancers for transdermal drug delivery. Pharmaceutics 11 (2), 96. doi:10.3390/pharmaceutics11020096
Stark, A., Behrend, P., Braun, O., Müller, A., Ranke, J., Ondruschka, B., et al. (2008). Purity specification methods for ionic liquids. Green Chem. 10 (11), 1152–1161. doi:10.1039/b808532c
Tanner, E. E., Ibsen, K. N., and Mitragotri, S. (2018). Transdermal insulin delivery using choline-based ionic liquids (CAGE). J. Control. release 286, 137–144. doi:10.1016/j.jconrel.2018.07.029
Thuy, P. T. P., Cho, C.-W., and Yun, Y.-S. (2010). Environmental fate and toxicity of ionic liquids: a review. Water Res. 44 (2), 352–372. doi:10.1016/j.watres.2009.09.030
Uddin, S., Islam, M. R., Chowdhury, M. R., Wakabayashi, R., Kamiya, N., Moniruzzaman, M., et al. (2021). Lipid-based ionic-liquid-mediated nanodispersions as biocompatible carriers for the enhanced transdermal delivery of a peptide drug. ACS Appl. bio Mater. 4 (8), 6256–6267. doi:10.1021/acsabm.1c00563
Wang, C., Zhu, J., Zhang, D., Yang, Y., Zheng, L., Qu, Y., et al. (2018). Ionic liquid – microemulsions assisting in the transdermal delivery of Dencichine: preparation, in-vitro and in-vivo evaluations, and investigation of the permeation mechanism. Int. J. Pharm. 535 (1), 120–131. doi:10.1016/j.ijpharm.2017.10.024
Zhang, F., Li, L., Zhang, X., Yang, H., Fan, Y., Zhang, J., et al. (2024). Ionic liquid transdermal patches of two active ingredients based on semi-ionic hydrogen bonding for rheumatoid arthritis treatment. Pharmaceutics 16 (4), 480. doi:10.3390/pharmaceutics16040480
Zhang, S., Sun, N., He, X., Lu, X., and Zhang, X. (2006). Physical properties of ionic liquids: database and evaluation. J. Phys. Chem. reference data 35 (4), 1475–1517. doi:10.1063/1.2204959
Keywords: ionic liquid, biocompatibility, transdermal drug delivery, toxicity, challenges, sustainability, green cosolvents, ionic liquids drug delivery
Citation: Jain A, Shakya AK, Prajapati SK, Eldesoqui M, Mody N, Jain SK, Naik RR and Patil UK (2024) An insight into pharmaceutical challenges with ionic liquids: where do we stand in transdermal delivery?. Front. Bioeng. Biotechnol. 12:1454247. doi: 10.3389/fbioe.2024.1454247
Received: 24 June 2024; Accepted: 25 July 2024;
Published: 06 August 2024.
Edited by:
Tingting Peng, Jinan University, ChinaReviewed by:
Vitor Francisco, University of Coimbra, PortugalCopyright © 2024 Jain, Shakya, Prajapati, Eldesoqui, Mody, Jain, Naik and Patil. This is an open-access article distributed under the terms of the Creative Commons Attribution License (CC BY). The use, distribution or reproduction in other forums is permitted, provided the original author(s) and the copyright owner(s) are credited and that the original publication in this journal is cited, in accordance with accepted academic practice. No use, distribution or reproduction is permitted which does not comply with these terms.
*Correspondence: Ankit Jain, YW5raXQuakBwaWxhbmkuYml0cy1waWxhbmkuYWMuaW4=; Umesh K. Patil, dW1lc2hwYXRpbDI5QGdtYWlsLmNvbQ==
Disclaimer: All claims expressed in this article are solely those of the authors and do not necessarily represent those of their affiliated organizations, or those of the publisher, the editors and the reviewers. Any product that may be evaluated in this article or claim that may be made by its manufacturer is not guaranteed or endorsed by the publisher.
Research integrity at Frontiers
Learn more about the work of our research integrity team to safeguard the quality of each article we publish.