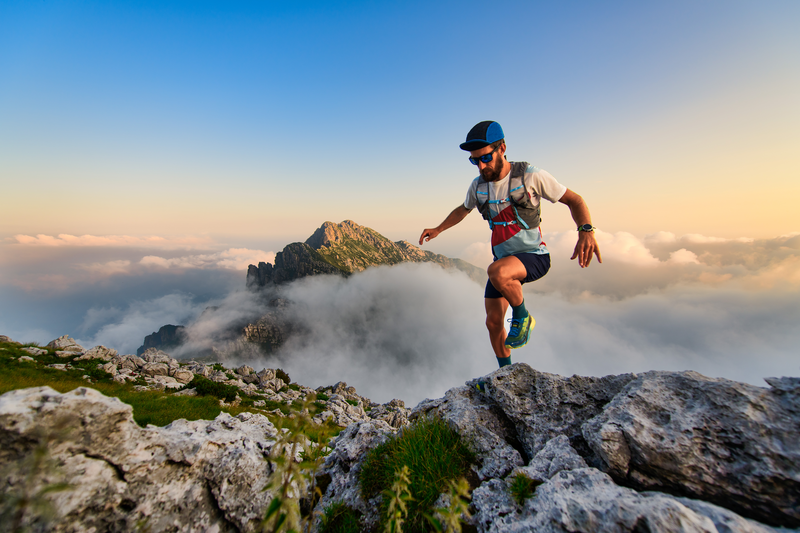
94% of researchers rate our articles as excellent or good
Learn more about the work of our research integrity team to safeguard the quality of each article we publish.
Find out more
ORIGINAL RESEARCH article
Front. Bioeng. Biotechnol. , 26 November 2024
Sec. Biomechanics
Volume 12 - 2024 | https://doi.org/10.3389/fbioe.2024.1453604
Objective: This study was to investigate alterations in contractile properties of the ankle plantar- and dorsiflexors in post-stroke individuals. The correlation between muscle architecture parameters and contractile properties was also evaluated.
Methods: Eight post-stroke individuals and eight age-matched healthy subjects participated in the study. Participants were instructed to perform maximal isometric contraction (MVC) of ankle plantar- and dorsiflexors at four ankle angles, and isokinetic concentric contraction at two angular velocities. B-mode ultrasound images of gastrocnemius medialis (GM) and tibialis anterior (TA) were collected simultaneously during the MVC and isokinetic measurements. Individualized torque-angle and torque-angular velocity relations were established by fitting the experimental data using a second-order polynomial and a rectangular hyperbola function, respectively. Muscle structure parameters, such as fascicle length, muscle thickness and pennation angle of the GM and TA muscles were quantified.
Results: Post-stroke subjects had significantly smaller ankle plantarflexor and dorsiflexor torques. The muscle structure parameters also showed a significant change in the stroke group, but no significant difference was observed in the TA muscle. A narrowed parabolic shape of the ankle PF torque-fiber length profile with a lower width span was also found in the stroke group.
Conclusion: This study showed that the contractile properties and architecture of ankle muscles in post-stroke individuals undergo considerable changes that may directly contribute to muscle weakness, decreased range of motion, and impaired motion function in individuals after stroke.
Skeletal muscles are considered as the biological force generator that is designed to provide the functional requirements for maintaining movement and posture (Dordević et al., 2014). In particular, ankle plantar- and dorsiflexor muscles controlling the ankle joints play an essential role in many daily activities (Hasson et al., 2011). The force developed by contracting muscle in vivo depends on the muscle length and velocity, with active and passive muscle forces contributing to total muscle force (Ward et al., 2009). Force-length and force-velocity relationships thus are commonly used to describe basic properties associated with force production capacity of a muscle. In practice, torque-angle
Stroke is one of the world’s leading causes of death and disability in adults (Aked et al., 2018; Shi et al., 2022). Post-stroke individuals often experience motor impairments dominantly on one side of the body, including muscle weakness, contracture, and spasticity (Hatem et al., 2016; Alamri et al., 2010). Ankle joint is one of the most affected lower-limb joints after stroke that about 60% of stroke individuals discharged from in-patient rehabilitation require an ankle orthosis and 34% of stroke individuals developing ankle contracture (Gao and Zhang, 2008; Chisholm and Perry, 2012; Hara et al., 2017). For many post-stroke individuals, the impaired dorsiflexors could lead to foot drop and increase the risk of stumbling and falling (Gorst et al., 2016). Moreover, weak and/or spastic plantarflexors may also reduce forward propulsion during gait (Vattanasilp et al., 2000; Zhang et al., 2013). Maximal isometric torque was often used in clinical settings as a marker of active muscle functional ability (Ramsay et al., 2014). However, peak torque often occurs at different joint angles for individuals due to variations in muscle-tendon properties, joint mechanics, and neuromuscular control (Kannus, 1991; Timmins et al., 2016). It is essential to take individual joint range of motion into consideration when evaluating muscle functionality in a non-intact group, i.e., post-stroke. It has been previously reported that age-related differences in contractile properties of both dorsi- and plantarflexors (
Neurological impairment after stroke often leads to secondary skeletal muscle structural and functional alterations, which impact contractile properties of the muscle and force production capacity (Zhang et al., 2024). The macroscopic arrangement of muscle fibers is also highly influenced by different factors such as age, gender and diseases (Knarr et al., 2014; Körting et al., 2019). Various noninvasive techniques, including surface electromyography, near-infrared spectroscopy, and ultrasonography, are used to analyze and assess muscle parameters (Guo et al., 2022). Muscle structure parameters, such as fascicle length (FL), muscle thickness (MT) and pennation angle (PA) have been evaluated in post-stroke individuals in vivo using medical imaging techniques, e.g., ultrasonography (US). Schillebeeckx et al. (Schillebeeckx et al., 2021) conducted a systematic review and revealed significant architectural changes, including reduced muscle thickness and fascicle length in individuals after stroke. However, the relationship between these morphological changes and muscle weakness remains unclear and requires further investigation. Gao and Zhang (2008) reported shorter FL and smaller PA in gastrocnemius medialis (GM) at rest compared to a control group. Compared to the less-affected side, decreased FL was also observed in the GM and soleus on the paretic side, respectively (Zhao et al., 2015). For dorsiflexors, muscle volumes, muscle length, FL and PA were nevertheless found similar in tibialis anterior (TA) between paretic and non-paretic sides at neutral position (Ramsay et al., 2014). Although the authors stated that the muscular structure alteration was not the primary inhibitor of the dorsiflexor strength, it is unclear whether there are structural changes through joint range of motion in post-stroke individuals and how these changes are intertwined with force production capacity. Liu et al. (2014) reported that body weight support treadmill training can increase pennation angle and muscle thickness in the tibialis anterior and fascicle length in the medial gastrocnemius, suggesting that early rehabilitation can positively impact muscle structure and improve function in individuals after subacute stroke. To the best of our knowledge, only one study by Gao and Zhang (2008) has investigated the changes in contractile properties of the gastrocnemius muscle using an electrical stimulator. However, there is still a lack of knowledge on the contractile properties of paretic muscle in patients with neurological disorders, particularly under voluntary contraction conditions.
The purpose of the current study was therefore to quantitatively compare muscle architecture parameters, i.e., FL, PA and MT of GM and TA, and contractile properties (
Eight hemiplegic post-stroke individuals (sex: 2F/6M; age: 53.5
In order to measure
Figure 1. Overview of individualized muscle architecture and contractile properties estimation. Ultrasound images were recorded during maximal isometric and isokinetic concentric contractions. Muscle structure parameters such as fascicle length, muscle thickness, and pennation angle of the GM and TA muscles were quantified through data processing.
Participants performed the experiment in a seated semi-upright position with the knee flexed at 20° and the foot was fixated to a footplate of the dynamometer. Initially, the footplate was placed perpendicularly to the subject’s tibia, which was considered as the 0°ankle position, and the ankle joint was carefully aligned with the rotation axis of the dynamometer. Shoulders, hips, and legs were adequately strapped, and the tested foot was especially securely strapped to the footplate. The affected side was tested for the PS group and the right side was tested in the HC group, respectively. Prior to the testing, ankle range of motion (ROM) of each participant was determined and then passively rotated through the test ROM to familiarize the movement. A static gravitational compensation at the neutral position (ankle at 0°) was also applied following the user manual of the dynamometer. To determine the
where
Muscle architecture parameters: US images of GM and TA were analyzed using a previous custom-written MATLAB script (Körting et al., 2019). Firstly, the superficial and deep aponeuroses of each muscle were identified. Three visible muscle fascicles were then selected (the proximal, distal, and intermediate parts of the muscles). MT was calculated as the distance between the superficial and deep aponeuroses, and the average MT from these three regions (proximal, distal, and intermediate) was reported. The superficial and deep PAs were identified as the angles between the fascicle and the superficial aponeurosis and deeper aponeurosis, respectively. The average of the superficial and deep PAs was reported (Son et al., 2020; Körting et al., 2019). The fascicle length FL was calculated as the straight-line distance by dividing MT by the sine of the deep PA. Muscle architecture parameters were identified both at rest, and in MVC at the frame where maximal torque was observed at each angle.
In order to take anthropometrical variance into consideration, TIM and TIV were normalized to the body weight, and FL and MT were normalized to the individual tibia length (the distance between the superior articular surface of the lateral condyle of the tibia to the tip of the medial malleolus). Spearman’s rank order correlation test was used to investigate the correlation between the optimal ankle torque and muscle structure parameters. Wilcoxon Mann-Whitney U test was used to investigate the group differences in normalized joint torque (TIM and TIV) and muscle parameters (FL and MT). Differences were considered statistically significant when
Overall, post-stroke individuals showed a significant reduction in maximal isometric torque for both PF and DF across all tested ankle angles (Table 1), which also resulted in substantially smaller optimal isometric torque values for PF and DF. In the HC group, the optimal isometric torque values were 118.74 (75.70, 172.40) Nm for ankle PF and 32.87 (29.62, 67.94) Nm for ankle DF. The reduction in ankle torque in the PS group was substantial for both ankle PF [11.43 (2.39, 43.65) Nm], resulting in a reduction of 90.35%, and DF [6.23 (0.66, 32.77) Nm] with a reduction of 81.08%. In the HC group, both ankle DF and PF isometric
Table 1. The measured isometric ankle torque (Nm) during isometric maximum voluntary Plantarflexion and Dorsiflexion contraction across subjects at four angle positions (5°dorsiflexion, 0°, 10°, and 20°plantarflexion) in both healthy control (HC) and post-stroke (PS) groups, presented as median (min, max). Values shown in bold indicate a statistically significant difference between the HC and PS groups based on Wilcoxon Mann-Whitney U tests.
Figure 2. The maximal isometric contraction torque-angle relationship of ankle plantarflexors and dorsiflexion in both post-stroke individuals and healthy control groups, fitted using a second-order polynomial with experimental data, shown as mean
The scaled torque of the PF and DF showed no significant difference between groups at all tested velocities (Table 2, PF 60°/s: p = 0.46, 90°/s: p = 0.14; DF 60°/s: p = 0.52, 90°/s: p = 0.71). A significantly reduced torque in post-stroke individuals was found on the extrapolated data at 120°/s in DF (p = 0.02), but not in PF (p = 0.09).
Table 2. The scaled isokinetic peak ankle torque across subjects at three angular velocities (60°/s, 90°/s, and 120°/s (extrapolated)) in both healthy control (HC) and post-stroke (PS) groups, presented as median (min, max). Values shown in bold indicate a statistically significant difference between the HC and PS groups based on Wilcoxon Mann-Whitney U tests.
The shape coefficient A (Table 3), derived from the rectangular hyperbola function, was significantly different between the two groups in DF (p < 0.01) but not in PF (p = 0.20). The shape coefficient A resulted in a steeper decline at lower angular velocities in the PS group’s torque-angular velocity curve, compared to the HC group (Figure 3). No significant difference was found in the shape coefficient B in both PF (p = 0.23) and DF (p = 0.08).
Table 3. The shape coefficient value A and B, derived from fitting a rectangular hyperbola function to model ankle torque-angular relationships during isokinetic Plantarflexion and Dorsiflexion contractions across subjects in both the healthy control (HC) and post-stroke (PS) groups, presented as median (min, max). Values shown in bold indicate a statistically significant difference between the HC and PS groups based on Wilcoxon Mann-Whitney U tests.
Figure 3. The maximal isometric torque-angular relationship of ankle plantarflexors and dorsiflexors in both post-stroke individuals and healthy control groups, fitted using a rectangular hyperbola function with experimental data. Shown as mean
At rest, the normalized FL of GM in the PS group is significantly shorter than in the HC at neutral position and 10°, and 20° of plantarflexion (Figure 4; 0°:
Figure 4. Fascicle length, muscle thickness, and pennation angle of gastrocnemius medialis (GM) in both post-stroke individuals and healthy control groups.
The MT of GM was found significantly smaller in the PS group at all tested angles in both rest and MVC (Figure 4; Rest −5°:
The PA of GM was found significantly smaller in the PS group at all tested angles in MVC (Figure 4; −5°:
For TA, the FL at rest was somewhat shorter in the PS group than in the HC group; however, the differences were not significant. The differences in FL at MVC were almost non-evidently. In addition, no significant differences were found in the MT and PA in any of the tested ankle angles (Figure 5).
Figure 5. Fascicle length, muscle thickness, and pennation angle of tibialis anterior (TA) in both post-stroke individuals and healthy control groups.
In the PS group, the ankle PF
Figure 6. Normalized Ankle plantarflexor (GM) and dorsiflexor (TA) torque–normalized fascicle length profile in both post-stroke individuals and healthy control groups. An individual torque - length curve was fitted for each subject, and the mean of all fitted curves is shown here. The dots illustrated the measured values. OFL: optimal fiber length.
Table 4. The optimal fascicle length (OFL) and width span (%OFL) across subjects in both healthy control (HC) and post-stroke (PS) groups, presented as median (min, max), derived from ankle maximal Isometric Torque–Fascicle Length Profile. Values shown in bold indicate a statistically significant difference between the HC and PS groups based on Wilcoxon Mann-Whitney U tests.
In this work, we investigated contractile properties (
Muscle weakness, defined as the inability to generate normal levels of torque during an MVC is a dominant deficit after hemispheric stroke. The severity of the muscle weakness has been approved to be one of the primary factors associated with physical limitation and may account for 66%–72% of the variation in the temporo-spatial parameters of gait after stroke (Kim and Eng, 2003). Ankle PF were the major contributor to forward progression (Neptune et al., 2001) and the strength of DF was reported to have the strongest association with gait velocity in the paretic side following stroke (Neptune et al., 2001). However, muscle strength of these two muscle groups was less commonly reported in post-stroke group, especially in DF. Compared to controls, significantly reduced maximal dorsiflexion and plantarflexion torque were observed in all tested angles in the current study. Similar observations were reported by Dias et al. (2017) and Kowal et al. (2020) while the ankle joint was only assessed in the neutral position. Son and Rymer (2021) investigated the maximal isometric plantarflexor torque at several joint angles on both sides of post-stroke subjects and also found significantly smaller torque on the paretic side. No previous study has reported maximal isometric dorsiflexion torque across joint angles as in the current study.
Evaluating the MVC
Compared to eccentric contraction, concentric torque production was reported more significantly impaired after stroke. It was shown that PF of the paretic side had a greater reduction in concentric torque than DF at an angular velocity of 30°/s, while eccentric torque had better preservation in both muscle groups (Eng et al., 2009). Kowal et al. (2020) also reported that compared to controls, significantly decreased plantarflexion torque at even higher concentric angular velocities, but no significant differences were found in DF. However, most studies only evaluated at one angular velocity and the overall
Muscle structure adaptation is another factor impacting on the contractile properties of muscle post-stroke. In the rest, significantly shorter fascicle length was observed in the GM in all tested angles except 5 °dorsiflexion, which was aligned with previous findings (Dias et al., 2017). However, Gao and Zhang (2008) also reported the differences were more pronounced in dorsiflexion positions, contrary to our observation. The discrepancy was most probably due to missing data in the post-stroke group that three participants were not able to reach any dorsiflexion position. Compared to controls, no difference in fascicle length was found in TA across all joint angles. Under maximal contraction, muscle fascicle length of both GM and TA did not differ between the paretic side and healthy participants. It indicated that larger fascicle excursion of GM (length differences under the rest and MVC) occurred in healthy muscle and therefore led to a similar length as paretic muscle under the maximal contraction. Similar findings were also reported by Dias et al. (2017). This observation highlighted the importance of restoring fascicle length and range of motion during rehabilitation intervention because the fiber excursion is generally proportional to fiber length (Dias et al., 2017).
The joint torque and fascicle length relationship depicted the association of muscle function at the joint level and muscle’s intrinsic structural property. The ankle range of motion in able-bodied subjects was generally accepted as 50°plantarflexion to 20°dorsiflexion. This range corresponds to the whole ascending limb and a very small portion of the descending limb of the plantarflexion torque and fascicle length relationship in GM. It benefits biomechanically with a rather low passive force and potentially prevents injury from overstretching. This phenomenon may be explained by the fact that muscle passive force is relatively low within this range. Previous studies have evaluated the contractile properties of GM by applying electrical stimulation (Gao and Zhang, 2008). Similar active force-fascicle length and torque-angle behavior were reported. In the present study, the actual measured ROM covered approximately from 30% to the maximal isometric torque on the ascending limb of the torque–fascicle length profile. A similar distribution can be found in the post-stroke group. However, the width span of the torque-fascicle length relationship of GM in the post-stroke group was somewhat narrower and the slopes of the ascending and descending limbs were also steeper. The narrowed width span suggests that post-stroke participants may have a somewhat restricted range in fascicle length excursion during contraction, potentially leading to a limited range of motion at the joint level during daily activities. Further investigation on muscle sarcomere level may provide a further in-depth understanding of fascicle excursion restriction in the post-stroke muscles (Adkins et al., 2022).
The clinical presentation of post-stroke patients is usually a combination of weakness and other symptoms such as spasticity and contractures that are impacted by the muscle architectures and mechanical properties; therefore, comprehensive treatment requires strategies taking multiple factors into consideration as highlighted in the current study. Systematic reviews and studies showed that strength can be improved by progressive resistance training in persons after a stroke (Ouellette et al., 2004; Ada et al., 2006; Flansbjer et al., 2012). These strength improvements are also correlated with increasing muscle function and reducing limb disability. In addition, the effect of a stretching program on spastic PS subjects was investigated by other studies (Saunders et al., 2020). It was found that the reduction of spasticity and muscle stiffness are associated with the increased FL. Therefore, the integration of our research findings into post-stroke rehabilitation interventions holds significant promise for enhancing outcomes related to spasticity and mitigating secondary complications such as weakness and changes in muscle architecture.
We acknowledge that a 38 mm wide transducer might not be able to fully visualize the fascicles in the posterior and distal part of the muscle, therefore linear extrapolation was performed. The fascicles in the middle part of the muscle are commonly intact within the field of view. However, we did not expect a large error due to the extrapolation because gastrocnemius medialis and tibialis anterior muscles exhibit less curvature compared to the biceps femoris (Franchi et al., 2020). We investigated muscle architecture and contractile properties in post-stroke individuals and we acknowledge that factors beyond muscle morphology also contribute to strength loss in the post-stroke. Impaired motor unit recruitment, reduced firing rates, and changes in motor control strategies are known neural factors that significantly affect force generation, independent of muscle size or architecture (Hu et al., 2016).
Due to time constraints and participant safety considerations, concentric contractions were only performed at 60°/s and 90°/s. This restriction reduced our ability to interpret the inter-group difference at a higher extrapolated angular velocity, i.e., 120°/s. However, although it was not directly measured, our observation aligns with findings from Clark et al. (2006), who observed a pronounced velocity-dependent impairment in concentric knee torque production at higher velocities. This trend suggests a consistent response in torque-velocity relationships across studies, likely due to impaired agonist activation and reduced neural drive during faster movements. Authors also acknowledge that future studies including multiple additional high-velocity measures if feasible would provide a complete picture of the velocity-dependent impairments in individuals.
It’s also important to note that we only investigated the muscle architecture of TA from ankle dorsiflexors and GM from ankle plantarflexors. This restriction stems from the limitation that ultrasound can only accurately assess superficial muscles. We analyzed an overall torque/force-length profile for all plantarflexors and dorsiflexors, without distinguishing individual muscles. Future research employing techniques such as simulation could enable the decomposition of torque to individual muscle force, allowing for a direct examination of force/length characteristics in specific muscles. In addition, we only tested on the affected leg and two concentric contraction velocities in the post-stroke individuals to avoid fatigue due to already extensive data acquisition protocol. Future research, with a larger cohort exploring muscle architecture and contractile properties on both paretic and non-paretic sides, can provide a comprehensive understanding of muscle function and mobility after stroke, enhancing the generalizability of findings.
Stroke is a neurological disorder that can lead to muscle weakness and other secondary muscle morphological changes affecting joint function and overall mobility. We investigated the effects of stroke on major ankle joint muscles by examining their active contractile properties (specifically, the
The original contributions presented in the study are included in the article/supplementary material, further inquiries can be directed to the corresponding author.
The studies involving humans were approved by the Swedish Ethical Review Authority. The studies were conducted in accordance with the local legislation and institutional requirements. The participants provided their written informed consent to participate in this study.
RW: Writing–review and editing, Writing–original draft, Validation, Supervision, Resources, Project administration, Methodology, Investigation, Funding acquisition, Formal Analysis, Data curation, Conceptualization. LZ: Writing–review and editing, Writing–original draft, Methodology, Formal Analysis. HJ: Writing–review and editing, Writing–original draft, Methodology, Formal Analysis. OT: Writing–review and editing, Methodology, Data curation, Conceptualization. GP: Writing–review and editing, Methodology, Data curation. AA: Writing–review and editing, Supervision, Methodology, Formal Analysis, Data curation, Conceptualization.
The author(s) declare that financial support was received for the research, authorship, and/or publication of this article. We would like to acknowledge financial support from Promobilia Foundation (A22078, 18014, 21302, and 18200) and Swedish Research Council (2022-03268, 2018–04902 and 2018–00750).
The authors declare that the research was conducted in the absence of any commercial or financial relationships that could be construed as a potential conflict of interest.
All claims expressed in this article are solely those of the authors and do not necessarily represent those of their affiliated organizations, or those of the publisher, the editors and the reviewers. Any product that may be evaluated in this article, or claim that may be made by its manufacturer, is not guaranteed or endorsed by the publisher.
Ada, L., Canning, C. G., and Low, S.-L. (2003). Stroke patients have selective muscle weakness in shortened range. Brain 126, 724–731. doi:10.1093/brain/awg066
Ada, L., Dorsch, S., and Canning, C. G. (2006). Strengthening interventions increase strength and improve activity after stroke: a systematic review. Aust. J. Physiother. 52, 241–248. doi:10.1016/s0004-9514(06)70003-4
Adkins, A. N., Fong, R. M., Dewald, J., and Murray, W. M. (2022). Variability of in vivo sarcomere length measures in the upper limb obtained with second harmonic generation microendoscopy. Front. Physiology 12, 817334–817410. doi:10.3389/fphys.2021.817334
Aked, J., Delavaran, H., Norrving, B., and Lindgren, A. (2018). Temporal trends of stroke epidemiology in southern Sweden: a population-based study on stroke incidence and early case-fatality. Neuroepidemiology 50, 174–182. doi:10.1159/000487948
Alamri, A., Cha, J., and El Saddik, A. (2010). Ar-rehab: an augmented reality framework for poststroke-patient rehabilitation. IEEE Trans. Instrum. Meas. 59, 2554–2563. doi:10.1109/tim.2010.2057750
Alcazar, J., Csapo, R., Ara, I., and Alegre, L. M. (2019). On the shape of the force-velocity relationship in skeletal muscles: the linear, the hyperbolic, and the double-hyperbolic. Front. Physiology 10, 769. doi:10.3389/fphys.2019.00769
Balmaseda, M. T., Fatehi, M. T., Koozekanani, S. H., and Lee, A. L. (1986). Ultrasound therapy: a comparative study of different coupling media. Archives Phys. Med. Rehabilitation 67, 147–150. doi:10.1016/0003-9993(86)90052-3
Caldwell, G. E., Adams III, W. B., and Whetstone, M. R. (1993). Torque/velocity properties of human knee muscles: peak and angle-specific estimates. Can. J. Appl. Physiology 18, 274–290. doi:10.1139/h93-024
Chisholm, A. E., and Perry, S. D. (2012). Ankle-foot orthotic management in neuromuscular disorders: recommendations for future research. Disabil. Rehabilitation Assistive Technol. 7, 437–449. doi:10.3109/17483107.2012.680940
Clark, D. J., Condliffe, E. G., and Patten, C. (2006). Activation impairment alters muscle torque–velocity in the knee extensors of persons with post-stroke hemiparesis. Clin. Neurophysiol. 117, 2328–2337. doi:10.1016/j.clinph.2006.07.131
Dias, C. P., Freire, B., Goulart, N. B., Onzi, E. S., Becker, J., Gomes, I., et al. (2017). Muscle architecture and torque production in stroke survivors: an observational study. Top. Stroke Rehabilitation 24, 206–213. doi:10.1080/10749357.2016.1210873
Dordević, S., Tomažič, S., Narici, M., Pišot, R., and Meglič, A. (2014). In-vivo measurement of muscle tension: dynamic properties of the mc sensor during isometric muscle contraction. Sensors 14, 17848–17863. doi:10.3390/s140917848
Eng, J. J., Lomaglio, M. J., and MacIntyre, D. L. (2009). Muscle torque preservation and physical activity in individuals with stroke. Med. Sci. sports Exerc. 41, 1353–1360. doi:10.1249/mss.0b013e31819aaad1
Flansbjer, U.-B., Lexell, J., and Brogårdh, C. (2012). Long-term benefits of progressive resistance training in chronic stroke: a 4-year follow-up. J. rehabilitation Med. official J. UEMS Eur. Board Phys. Rehabilitation Med. 44, 218–221. doi:10.2340/16501977-0936
Franchi, M. V., Fitze, D. P., Raiteri, B. J., Hahn, D., and Spörri, J. (2020). Ultrasound-derived biceps femoris long-head fascicle length: extrapolation pitfalls. Med. Sci. Sports Exerc. 52, 233–243. doi:10.1249/mss.0000000000002123
Gao, F., and Zhang, L.-Q. (2008). Altered contractile properties of the gastrocnemius muscle poststroke. J. Appl. Physiology 105, 1802–1808. doi:10.1152/japplphysiol.90930.2008
Gorst, T., Lyddon, A., Marsden, J., Paton, J., Morrison, S. C., Cramp, M., et al. (2016). Foot and ankle impairments affect balance and mobility in stroke (faimis): the views and experiences of people with stroke. Disabil. Rehabilitation 38, 589–596. doi:10.3109/09638288.2015.1052888
Guo, W., Sheng, X., and Zhu, X. (2022). Assessment of muscle fatigue based on motor unit firing, muscular vibration and oxygenation via hybrid mini-grid semg, mmg, and nirs sensing. IEEE Trans. Instrum. Meas. 71, 1–10. doi:10.1109/tim.2022.3198472
Hahn, D., Herzog, W., and Schwirtz, A. (2014). Interdependence of torque, joint angle, angular velocity and muscle action during human multi-joint leg extension. Eur. J. Appl. Physiology 114, 1691–1702. doi:10.1007/s00421-014-2899-5
Hara, T., Abo, M., Hara, H., Kobayashi, K., Shimamoto, Y., Samizo, Y., et al. (2017). Effects of botulinum toxin a therapy and multidisciplinary rehabilitation on upper and lower limb spasticity in post-stroke patients. Int. J. Neurosci. 127, 469–478. doi:10.1080/00207454.2016.1196204
Hasson, C. J., Miller, R. H., and Caldwell, G. E. (2011). Contractile and elastic ankle joint muscular properties in young and older adults. PLoS One 6, e15953. doi:10.1371/journal.pone.0015953
Hatem, S. M., Saussez, G., Della Faille, M., Prist, V., Zhang, X., Dispa, D., et al. (2016). Rehabilitation of motor function after stroke: a multiple systematic review focused on techniques to stimulate upper extremity recovery. Front. Hum. Neurosci. 10, 442–522. doi:10.3389/fnhum.2016.00442
Horstman, A., Gerrits, K., Beltman-Kievits, J., Janssen, T., Konijnenbelt, M., and de Haan, A. (2009). Muscle function of knee extensors and flexors after stroke is selectively impaired at shorter muscle lengths. J. Rehabilitation Med. 41, 317–321. doi:10.2340/16501977-0331
Hu, X., Suresh, A. K., Rymer, W. Z., and Suresh, N. L. (2016). Altered motor unit discharge patterns in paretic muscles of stroke survivors assessed using surface electromyography. J. Neural Eng. 13, 046025–46118. doi:10.1088/1741-2560/13/4/046025
Kannus, P. (1991). Relationship between peak torque and angle-specific torques in an isokinetic contraction of normal and laterally unstable knees. J. Orthop. and Sports Phys. Ther. 13, 89–94. doi:10.2519/jospt.1991.13.2.89
Kim, C. M., and Eng, J. J. (2003). The relationship of lower-extremity muscle torque to locomotor performance in people with stroke. Phys. Ther. 83, 49–57. doi:10.1093/ptj/83.1.49
Knarr, B. A., Reisman, D. S., Binder-Macleod, S. A., Higginson, J. S., et al. (2014). Changes in predicted muscle coordination with subject-specific muscle parameters for individuals after stroke. Stroke Res. Treat. 2014, 1–7. doi:10.1155/2014/321747
Körting, C., Schlippe, M., Petersson, S., Pennati, G. V., Tarassova, O., Arndt, A., et al. (2019). In vivo muscle morphology comparison in post-stroke survivors using ultrasonography and diffusion tensor imaging. Sci. Rep. 9, 11836–11911. doi:10.1038/s41598-019-47968-x
Kowal, M., Kołcz, A., Dymarek, R., Paprocka-Borowicz, M., and Gnus, J. (2020). Muscle torque production and kinematic properties in post-stroke patients: a pilot cross-sectional study. Acta Bioeng. Biomechanics 22, 11–20. doi:10.37190/abb-01467-2019-02
Lanza, I. R., Towse, T. F., Caldwell, G. E., Wigmore, D., and Kent-Braun, J. A. (2003). Effects of age on human muscle torque, velocity, and power in two muscle groups. J. Appl. Physiology 95, 2361–2369. doi:10.1152/japplphysiol.00724.2002
Liu, P., Wang, Y., Hu, H., Mao, Y., Huang, D., and Li, L. (2014). Change of muscle architecture following body weight support treadmill training for persons after subacute stroke: evidence from ultrasonography. BioMed Res. Int. 2014, 1–11. doi:10.1155/2014/270676
Neptune, R. R., Kautz, S. A., and Zajac, F. E. (2001). Contributions of the individual ankle plantar flexors to support, forward progression and swing initiation during walking. J. Biomechanics 34, 1387–1398. doi:10.1016/s0021-9290(01)00105-1
Ouellette, M. M., LeBrasseur, N. K., Bean, J. F., Phillips, E., Stein, J., Frontera, W. R., et al. (2004). High-intensity resistance training improves muscle strength, self-reported function, and disability in long-term stroke survivors. Stroke 35, 1404–1409. doi:10.1161/01.str.0000127785.73065.34
Paterson, D. H., Jones, G. R., and Rice, C. L. (2007). Ageing and physical activity: evidence to develop exercise recommendations for older adultsThis article is part of a supplement entitled Advancing physical activity measurement and guidelines in Canada: a scientific review and evidence-based foundation for the future of Canadian physical activity guidelines co-published by Applied Physiology, Nutrition, and Metabolism and the Canadian Journal of Public Health. It may be cited as Appl. Physiol. Nutr. Metab. 32(Suppl. 2E) or as Can. J. Public Health 98(Suppl. 2). Appl. Physiology, Nutr. Metabolism 32, S69–S108. doi:10.1139/h07-111
Ramsay, J. W., Wessel, M. A., Buchanan, T. S., Higginson, J. S., et al. (2014). Poststroke muscle architectural parameters of the tibialis anterior and the potential implications for rehabilitation of foot drop. Stroke Res. Treat. 2014, 1–5. doi:10.1155/2014/948475
Saunders, D. H., Sanderson, M., Hayes, S., Johnson, L., Kramer, S., Carter, D. D., et al. (2020). Physical fitness training for stroke patients. Cochrane Database Syst. Rev. 2020, CD003316. doi:10.1002/14651858.cd003316.pub7
Schillebeeckx, F., De Groef, A., De Beukelaer, N., Desloovere, K., Verheyden, G., and Peers, K. (2021). Muscle and tendon properties of the spastic lower leg after stroke defined by ultrasonography: a systematic review. Eur. J. Phys. Rehabilitation Med. 57, 495–510. doi:10.23736/s1973-9087.20.06462-x
Sharp, S. A., and Brouwer, B. J. (1997). Isokinetic strength training of the hemiparetic knee: effects on function and spasticity. Archives Phys. Med. Rehabilitation 78, 1231–1236. doi:10.1016/s0003-9993(97)90337-3
Shi, Y., Tian, Z., Wang, M., Wu, Y., Yang, B., and Fu, F. (2022). Residual convolutional neural network-based stroke classification with electrical impedance tomography. IEEE Trans. Instrum. Meas. 71, 1–11. doi:10.1109/tim.2022.3165786
Son, J., and Rymer, W. Z. (2021). Longer electromechanical delay in paretic triceps surae muscles during voluntary isometric plantarflexion torque generation in chronic hemispheric stroke survivors. J. Electromyogr. Kinesiol. 56, 102475. doi:10.1016/j.jelekin.2020.102475
Son, J., Rymer, W. Z., and Lee, S. S. (2020). Limited fascicle shortening and fascicle rotation may be associated with impaired voluntary force-generating capacity in pennate muscles of chronic stroke survivors. Clin. Biomech. 75, 105007–105009. doi:10.1016/j.clinbiomech.2020.105007
Tieland, M., Trouwborst, I., and Clark, B. C. (2018). Skeletal muscle performance and ageing. J. Cachexia, Sarcopenia Muscle 9, 3–19. doi:10.1002/jcsm.12238
Timmins, R. G., Shield, A. J., Williams, M. D., and Opar, D. A. (2016). Is there evidence to support the use of the angle of peak torque as a marker of hamstring injury and re-injury risk? Sports Med. 46, 7–13. doi:10.1007/s40279-015-0378-8
Vattanasilp, W., Ada, L., and Crosbie, J. (2000). Contribution of thixotropy, spasticity, and contracture to ankle stiffness after stroke. J. Neurology, Neurosurg. and Psychiatry 69, 34–39. doi:10.1136/jnnp.69.1.34
Ward, S. R., Eng, C. M., Smallwood, L. H., and Lieber, R. L. (2009). Are current measurements of lower extremity muscle architecture accurate? Clin. Orthop. Relat. Res. 467, 1074–1082. doi:10.1007/s11999-008-0594-8
Zhang, L., Van Wouwe, T., Yan, S., and Wang, R. (2024). Emg-constrained and ultrasound-informed muscle-tendon parameter estimation in post-stroke hemiparesis. IEEE Trans. Biomed. Eng. 71, 1798–1809. doi:10.1109/tbme.2024.3352556
Zhang, L.-Q., Chung, S. G., Ren, Y., Liu, L., Roth, E. J., and Rymer, W. Z. (2013). Simultaneous characterizations of reflex and nonreflex dynamic and static changes in spastic hemiparesis. J. Neurophysiology 110, 418–430. doi:10.1152/jn.00573.2012
Keywords: torque-angle relationship, torque-angular velocity relation, fascicle length, muscle thickness, pennation angle, ultrasound
Citation: Wang R, Zhang L, Jalo H, Tarassova O, Pennati GV and Arndt A (2024) Individualized muscle architecture and contractile properties of ankle plantarflexors and dorsiflexors in post-stroke individuals. Front. Bioeng. Biotechnol. 12:1453604. doi: 10.3389/fbioe.2024.1453604
Received: 23 June 2024; Accepted: 12 November 2024;
Published: 26 November 2024.
Edited by:
Huub Maas, VU Amsterdam, NetherlandsReviewed by:
Markus Tilp, University of Graz, AustriaCopyright © 2024 Wang, Zhang, Jalo, Tarassova, Pennati and Arndt. This is an open-access article distributed under the terms of the Creative Commons Attribution License (CC BY). The use, distribution or reproduction in other forums is permitted, provided the original author(s) and the copyright owner(s) are credited and that the original publication in this journal is cited, in accordance with accepted academic practice. No use, distribution or reproduction is permitted which does not comply with these terms.
*Correspondence: Ruoli Wang, cnVvbGlAa3RoLnNl
†These authors share first authorship
Disclaimer: All claims expressed in this article are solely those of the authors and do not necessarily represent those of their affiliated organizations, or those of the publisher, the editors and the reviewers. Any product that may be evaluated in this article or claim that may be made by its manufacturer is not guaranteed or endorsed by the publisher.
Research integrity at Frontiers
Learn more about the work of our research integrity team to safeguard the quality of each article we publish.