- 1Department of Rehabilitation, Seventh People’s Hospital of Shanghai University of Traditional Chinese Medicine, Shanghai, China
- 2Department of Traditional Chinese Medicine, Shanghai Puxing Community Healthcare Center, Shanghai, China
- 3Department of Orthopedics and Traumatology, Seventh People’s Hospital of Shanghai University of Traditional Chinese Medicine, Shanghai, China
Background: Abnormal brain activation patterns in patients with knee osteoarthritis (KOA) at rest have been revealed, but it is unclear how brain activation patterns change during movement. This study aimed to investigate the alterations in brain activation patterns in KOA patients during knee isokinetic movement, and the correlation between cortical activity changes and pain severity and dysfunction.
Methods: Eighteen patients with KOA and 18 healthy controls (HC) were recruited, and to performed the knee isokinetic test with three speeds. Functional near-infrared spectroscopy (fNIRS) was used to detect the cerebral cortex hemodynamics changes of primary somatosensory (S1), primary motor (M1) and somatosensory association cortex (SAC) in the region of interest (ROI) during movement. Then, we evaluated potential correlations between M1, S1 and SAC values and Western Ontario and McMaster Universities Osteoarthritis Index (WOMAC) and visual analog scale (VAS) scores.
Results: The results showed that peak torque of knee extension in KOA patients was significantly smaller than that in HC. For HC, unilateral knee movement activated bilateral ROIs. The contralateral activation was dominant, showing the phenomenon of high contralateral activation. For KOA patients, there were no statistical difference in the activation level between the left and right of the cerebral cortex, with both sides showing lower activation levels compared to HC. Further analysis found that the contralateral M1, S1, and SAC of the affected knee in KOA patients were significantly lower than those in HC, while no difference was found on the ipsilateral side. Moreover, during isokinetic movement at 180°/s, VAS score in KOA patients was negatively correlated with the activation level of the contralateral S1 and M1 values, and WOMAC was negatively correlated with the activation level of the contralateral M1 value.
Conclusion: Contralateral activation of the sensorimotor cortex exists during unilateral knee movement, but in KOA patients, this contralateral cortical activation is suppressed. Furthermore, the clinical pain and dysfunction in KOA patients are associated with activation levels of specific brain regions. These findings can provide a better understanding of KOA brain science and are expected to contribute to the development of central intervention for the disease.
1 Introduction
Knee osteoarthritis (KOA) is a chronic degenerative bone and joint disease common in middle-aged and older adults (Glyn-Jones et al., 2015). It often leads to persistent pain and functional changes, which severely restricts daily activities and movements (Mantana et al., 2020). According to the World Health Organization (WHO), KOA is recognized as one of the most prevalent disabling conditions worldwide (Cross et al., 2014). It has been reported that, more than 1/3 of the elderly suffer from KOA, and accounts for 2.2% of overall years impacted by disability (Cross et al., 2014; Leigh F et al., 2021). Due to the aging of the global population, the incidence of KOA is expected to continue to increase, with adverse social and economic consequences (Ian J et al., 2017).
Previous studies showed that although KOA is typically considered a peripheral joint disease, it has been demonstrated that the occurrence and development of KOA are related to abnormalities in the central nervous system (CNS) (Enrique et al., 2017; Ye et al., 2023). However, less is known about the effects of KOA on CNS changes (Mansfield et al., 2022). It has been found that as even after KOA patients receive traditional rehabilitation management, abnormal activation patterns and changes of CNS still persist (Özgül et al., 2021). Several studies have used different methods to observe the abnormal CNS changes caused by KOA (Shanahan et al., 2015; Cheng et al., 2022; Kang et al., 2022). Jerin discovered that abnormalities in electroencephalography (EEG) patterns at sensory discriminative, and descending inhibitory cortical areas were exhibited in KOA patients (Jerin et al., 2023). Cheng and Kang found by magnetic resonance imaging (MRI) that compared with healthy controls, KOA patients had decreased motor cortex (gray matter and white matter) volume and altered resting-state brain networks, and the degree of change was correlated with disease severity (Cheng et al., 2022; Kang et al., 2022). Shanahan et al. found by MRI that KOA patients experienced significant changes in the motor cortex activation sites when performing visually guided force-matched isometric contraction task, and these changes were associated with modified motor function and behavior (Shanahan et al., 2015). The authors believe that this may be an important reason for the poor motor performance of KOA patients (Shanahan et al., 2015). This partly confirms that KOA patients have motor deficits associated with decreased neural activation. However, these studies were conducted in a resting state and cannot detect the brain activation changes during real movement tasks, which is important for understanding of KOA. The patterns of brain activation observed during movement can reflect the dysfunction of patients in daily life activities, and reveal the potential obstacles of patients in motor control and coordination (Andres M et al., 2017). Furthermore, it is unclear brain activation patterns relate to function and pain during movement. Especially the function, which is more meaningful reflected in movement tasks. Consequently, an in-depth understanding of brain activation patterns during movement is crucial for advancing the fields of neuroscience and neurorehabilitation related to KOA.
In recent years, advances in functional near-infrared spectroscopy (fNIRS) provides convenience for the study of brain activation patterns during movement (Stephane and Pierre, 2018; Cristina et al., 2020; Alka et al., 2021). The brain activation pattern is defined as the movement task-related activation representations in the specific area of the brain cerebral cortex (Eliassen et al., 2001). The rationale for fNIRS measured brain activation pattern is based on the concept that neural activation in response to external stimuli or movement tasks results in increased hemodynamic in the activated area. Borot L used fNIRS to observe the brain activation patterns during limb movement in healthy participants and found that fNIRS measurements were highly reliable and valid compared with fMRI measurements (Borot et al., 2018). For the application of fNIRS in KOA, Özgül et al. (2021) found that movement improved clinical assessments of pain severity after 6 weeks of exercise training in KOA patients, accompanied by changes in prefrontal cortex activation monitored by fNIRS. Pollonini et al. (2020) used fNIRS research to find that after the treatment of transcranial direct current stimulation (tDCS), the excitability of related functional brain regions in patients with KOA significantly increased. The above studies observed changes of cortical hemodynamic in brain regions of KOA patients through peripheral and central interventions respectively, but these were only monitored by fNIRS in a resting state and lacked healthy controls.
It is unclear how the brain activation patterns in KOA change during real movement tasks, especially including the hemodynamic changes of primary somatosensory (S1), primary motor (M1) and somatosensory association cortex (SAC). In addition, there is a lack of research comparing cerebral hemodynamic changes in KOA patients and healthy controls during movement, and whether these changes can serve as reliable objective measurements of pain and functional changes in patients with KOA. Therefore, this study used fNIRS to explore the changes of brain activation patterns in KOA patients and healthy subjects during movement, and the correlation between these changes and pain severity and dysfunction in patients with KOA, which will provide evidence and potential targets for brain activation changes for subsequent interventions, and open a new perspective for the rehabilitation of musculoskeletal chronic diseases. We hypothesized that KOA patients had different brain activation patterns during knee isokinetic movement compared to the healthy controls, and the clinical pain and dysfunction in KOA patients were associated with activation levels in specific brain regions.
2 Materials and methods
2.1 Study design
A biomechanical-fNIRS cross-section study was conducted to examine the brain activation patterns and knee torque in the KOA patients during knee isokinetic movement at three speeds (60, 120, and 180°/s) as compared to the healthy controls (HC), and to identify the changes of brain activation patterns during movement, and the correlation between these changes and pain severity and dysfunction in KOA patients.
2.2 Sample size calculation
The determination of the sample size was accomplished by conducting a priori power analysis using the G*Power software (version 3.1.9.7). A previous study reported that the patients with KOA showed reduced maximal knee extensor isometric torque compared with the healthy controls (mean value: 1.35 vs. 1.87 Nm/kg) (Carlos et al., 2021). The present study calculated the effect size was 1.14 based on this result, an α error of 0.05 and β of 0.95 (power level of 0.95), allocation ratio 1, and determined that a minimum of 36 participants were required to ensure an appropriate group size for the study, resulting in a sample size of 18 individuals per group. This sample size was deemed sufficient to yield reliable and valid results for the present study.
2.3 Participants
Eighteen patients with KOA (63.88 ± 6.50 years) and eighteen demographically similar HC (61.22 ± 5.86 years) were included in this study. KOA patients were recruited from Shanghai Seventh People’s Hospital and nearby community centers through flyers, posters, and referrals from orthopedists and physiotherapists between February and March 2023. The inclusion criteria were as follows: 1) diagnosis of KOA according to the clinical classification criteria of American College of Rheumatology; 2) age range 50–75 years old; 3) having Kellgren-Lawrence grade Ⅱ and Ⅲ; 4) knee pain score (At least in the past 3 months) ≥ 3 on a 10-point scale; 5) knee symptoms and imaging manifestations are only on the right side; 6) signing informed consent form. The exclusion criteria included the following: 1) patients with other joint diseases such as rheumatoid arthritis or other inflammatory disease; 2) patients with a history of knee injury (e.g., meniscus, ligament injury, surgery); 3) patients with cardiovascular and cerebrovascular diseases, neurological or psychiatric disorder; 4) consuming medications that alter the excitability of the cerebral cortex (e.g., sedatives, stimulants). Healthy controls were recruited from communities around the hospital through flyers and posters. All participants were required to have the right dominant lower limb, which was identified by preference for kicking. Furthermore, they did not include participants who may affect exercise and brain activity (history of neurological, physical, or psychiatric illness).
2.4 Experimental procedure
The experiment was conducted in the rehabilitation evaluation room of the hospital, and the test environment was kept quiet throughout the procedure. All participants’ height, weight and other demographic characteristics were collected before the test. KOA patients were also evaluated with clinical measures by an experienced physiotherapist. Clinical pain was evaluated using the Visual Analog Scale (VAS) for pain, a measure of pain perception rated on a scale of 0–10 (Myles et al., 2017). KOA functional symptoms were assessed using the Western Ontario and McMaster Universities Osteoarthritis Index (WOMAC), an index specifically designed for OA ranging from 0 to 96 (Seror et al., 2007).
Before the formal test, the participants underwent two familiarization sessions to ensure they were proficient in using the technology. This practice was essential to guarantee that they were comfortable and able to perform the tasks correctly. Participants were instructed to sit on a biodex isokinetic dynamometer (System 4, Biodex Inc., Shirley, NY, United States) after wearing fNIRS device. Fasten fixation straps around the shoulders, waist and distal thigh of the test leg to increase safety and prevent any unnecessary movement during the test. The test side of all participants was on the right side. The axis of the dynamometer is positioned in alignment with the centre of the lateral femoral condyle. The lever arm was then adjusted to match the length of the leg being tested, with resistance applied to the front of the ankle. Before each trial, each participant was asked to remain in a resting state, sitting quietly for 5 min and avoiding random movements and speech to minimize the brain hemodynamic response generated by their activity. During the test, the participants completed three sets of angular velocity tests in random order: low (60°/s), medium (120°/s) and high (180°/s). The pattern was CONCENTRIC/CONCENTRIC, and each set was repeated 3 times. During each testing session, participants actively completed the flexion and extension of the knee at a constant angular speed, and exerted the maximum muscle strength as possible. Took a 5 min break between each set of tests, which allowed the fNIRS spectral signal for each test to be returned to the baseline. Each test was synchronized with a marker to keep the duration of each test consistent with the duration recorded by fNIRS. As shown in Figure 1. The isokinetic tester records the participants’ peak torque data at different angular speeds in real time.
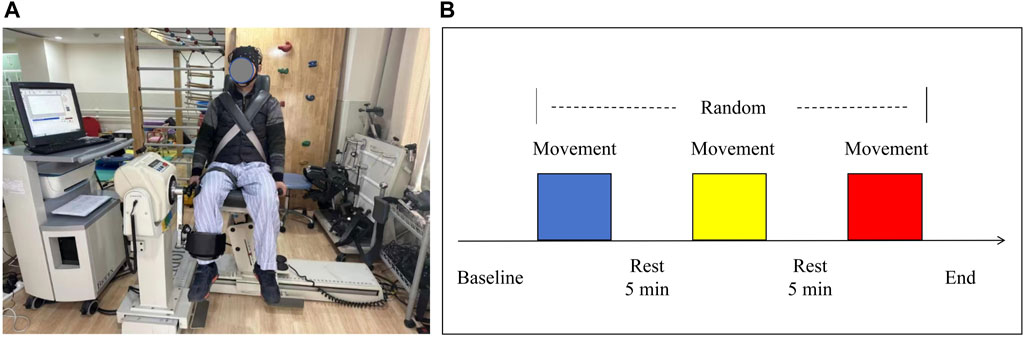
Figure 1. (A) Experimental setup and (B) schematic diagram of the flow for each testing session. Three colors randomly represent the isokinetic movement at 60°/s, 120°/s, and 180°/s speeds.
2.5 fNIRS data acquisition and preprocessing
The fNIRS data was collected while the participants performed the above three sets of isokinetic movements. In order to evaluate the changes of brain activity during movement, a multi-channel wireless portable fNIRS system Brite24 system (Artinis Medical Systems, Netherlands) was used to acquire fNIRS signals from cerebral regions, making the measurement of oxyhemoglobin (HbO) and deoxyhemoglobin (HbR) possible. HbO and HbR are indirect indicators of neural activity. The changes in their concentrations reflect the local hemodynamic responses, which are closely coupled with the underlying neuronal activity. The light source of the system generates near-infrared light with two wavelengths of 670 and 850 nm, with a sampling rate of 10 Hz. Each sensor of the instrument consists of a light emitting diode and a detector photodiode, with an inter-probe distance of 30 mm. There were 10 light sources and 8 detectors in total, which constitute 24 channels. According to the location of the fNIRS channel recorded digitally in 3D, the regions covered by the probe include six regions of interest (ROI) in the bilateral brain: primary somatosensory (S1), primary motor (M1) and somatosensory association cortex (SAC). Figure 2; Table 1 shows the channel assignment information for ROI.
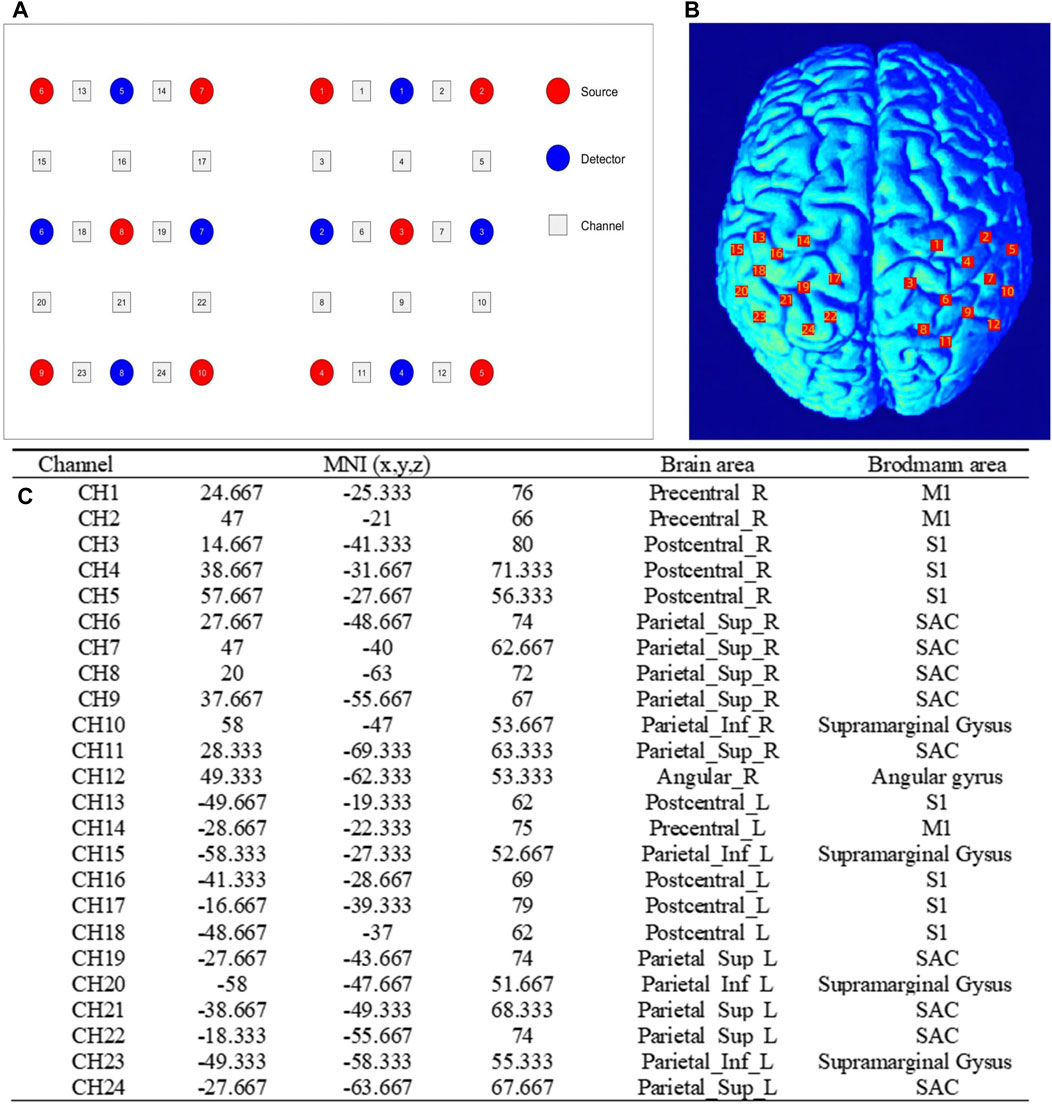
Figure 2. Channel assignment information for ROI. (A) the positions of optical emitters (red markers) and detectors (blue markers) in the fNIRS channel; (B) and schematic representation of fNIRS channel alignment with ROI. ROI: regions of interest; (C) the table related to the channel MNI coordinate, brain and Brodmann area correspondences. MNI, Montreal Neurological Institute; M1, primary motor; S1, primary somatosensory; SAC, somatosensory association cortex.
It was important to ensure that the position of the electrode Cz on the scalp surface was accurately located for research purposes. This was done by measuring the intersection from the nasal root to the anterior occipital protuberance line and from the left ear to the right ear anterior fossa line. This helps to ensure consistency in the placement of the electrode cap on the subjects when they are wearing it. Additionally, visually checking the alignment along the midsagittal plane further confirmed the accuracy of the electrode Cz position.
Raw data were extracted from Oxysoft software (v3.0.95, Artinis Medical Systems). After manual inspection and deletion of poor-quality channels, Matlab scripts from the Homer3 toolbox was used for data preprocessing (Theodore et al., 2009). The initial step involved converting the original light intensity data into optical density data by employing the hmrIntensity2OD function. To ensure data accuracy, a combination method of moving standard deviation, spline interpolation, and wavelet artefact correction function (SDThresh = 20, AMPThresh = 0.5, tMotion = 0.5 s, tMask = 2 s, p = 0.99, and iqr = 0.1) were employed to eliminate potential head motion artefacts. The corrected signal was filtered by 0.01–0.2 Hz bandpass filter (zero phase, fifth-order Butterworth filter) to eliminate physiological noise like respiration, heartbeat, and low-frequency signal drift. Finally, the corrected optical density data were converted into relative hemoglobin concentration changes in HbO and HbR by using the modified Beer-Lambert law (Kocsis et al., 2006). Since studies have shown that HbO concentration has superior signal-to-noise ratio, greater repeatability and stability over time, and a higher correlation with blood oxygen level dependent signals observed by fMRI, we used HbO concentration for follow-up analysis (Gary et al., 2002; Li. et al., 2023).
2.6 Statistical analysis
SPSS 21.0 software (IBM Corporation, Armonk, NY) was used for statistical analysis of results. The frequencies of count data were calculated in statistical descriptions. Data of measured data were described as mean ± standard deviation. Normality of the data was assessed with Shapiro-Wilk test. Demographic characteristics between groups were compared using independent sample t-test or Chi-square t-test. The differences in activation between left and right brain regions were analyzed by paired t-test. To assess whether brain activation differed between groups (KOA and HC) at different speeds (60°/s, 120°/s, and 180°/s), two-way repeated-measures ANOVA or non-parametric test (when normality was not met) was performed for each brain region. In addition, Pearson’s correlations were used to determine the association between changes in brain activity and VAS and WOMAC scores. Pearson’s correlations were graded as low (r < 0.30), moderate (0.30 < r < 0.60), and high (r > 0.60) (Monticone et al., 2018). The significance level was set at P < 0.05 for all analyses.
3 Results
3.1 Demographics and characteristics of the population
All 36 participants completed the study. The demographics and characteristics of the KOA patients and HC are presented in Table 2. There were no significant differences between the two groups in terms of age, gender, height, weight and BMI (P > 0.05). The mean VAS and WOMAC scores of patients with KOA were 4.50 ± 0.98 and 46.44 ± 5.75, respectively.
3.2 Isokinetic peak torque of knee extension between KOA patients and HC
Table 3 shows peak torque of knee extension of KOA patients and HC at the different speeds. There was no significant interaction effect between group and speed for peak torque (P = 0.186). A significant main effect of group was found for peak torque (P = 0.005). Compared to the HC, KOA patients had significantly smaller peak torque at all speeds. However, there were no significant speed effects for peak torque (P = 0.157).
3.3 Bilateral differences in cerebral cortex activation
Figure 3 cerebral cortex activation maps show mean HbO levels in HC and KOA patients during unilateral knee isokinetic movements at three speeds. For HC, bilateral activation of M1, S1, and SAC was observed across all speeds. There was no statistically significant difference in activation levels between the left and right of cerebral cortex, except for S1 under 180°/s speed, but the contralateral activation was dominant, showing the phenomenon of high contralateral activation. For KOA patients, there were no statistical difference in the activation level between the left and right of the cerebral cortex, with both sides showing lower activation levels compared to HC. As shown in Table 4.
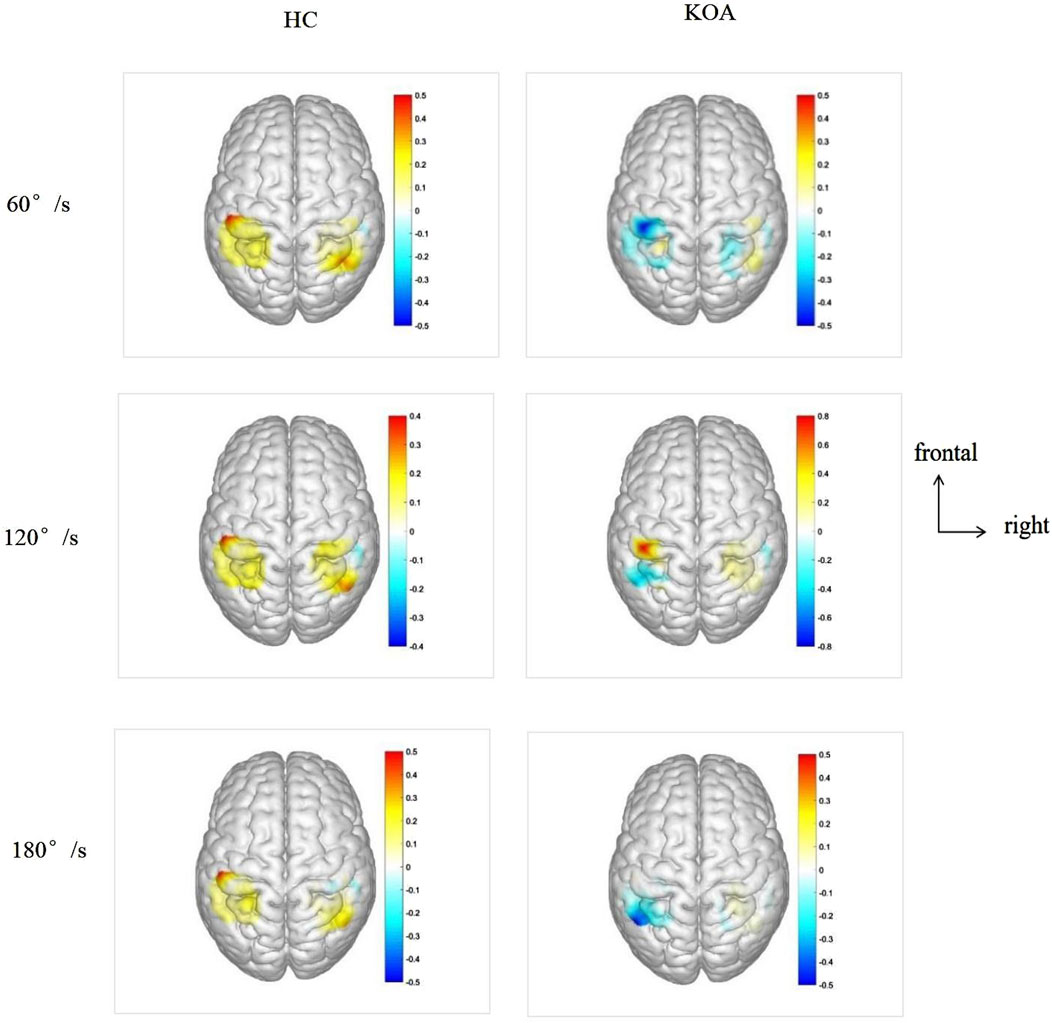
Figure 3. Cerebral cortex activation maps show mean HbO levels in HC and KOA patients during unilateral knee isokinetic movements at three speeds. The transition from blue to red signifies increasing activation intensity from low to high. HbO, Oxyhemoglobin; KOA, knee osteoarthritis; HC, healthy controls.
3.4 Differences in cerebral cortical activation between KOA patients and HC
Figure 4 shown the differences in cerebral cortical activation between KOA patients and HC. For the left of M1, S1, and SAC, there was no significant interaction effect between group and speed. Similarly, there was no significant interaction effect on the right. A significant main effect of group was found for the left of M1, S1, and SAC (PM1 = 0.017; PS1 = 0.005; PSAC = 0.002), while the right side had no difference. The left of M1, S1, and SAC in KOA patients were significantly lower than those in HC, indicating that the left side of cerebral cortex were inhibited in KOA patients compared with that in HC. Additionally, speed had no significant main effect on either left or right side.
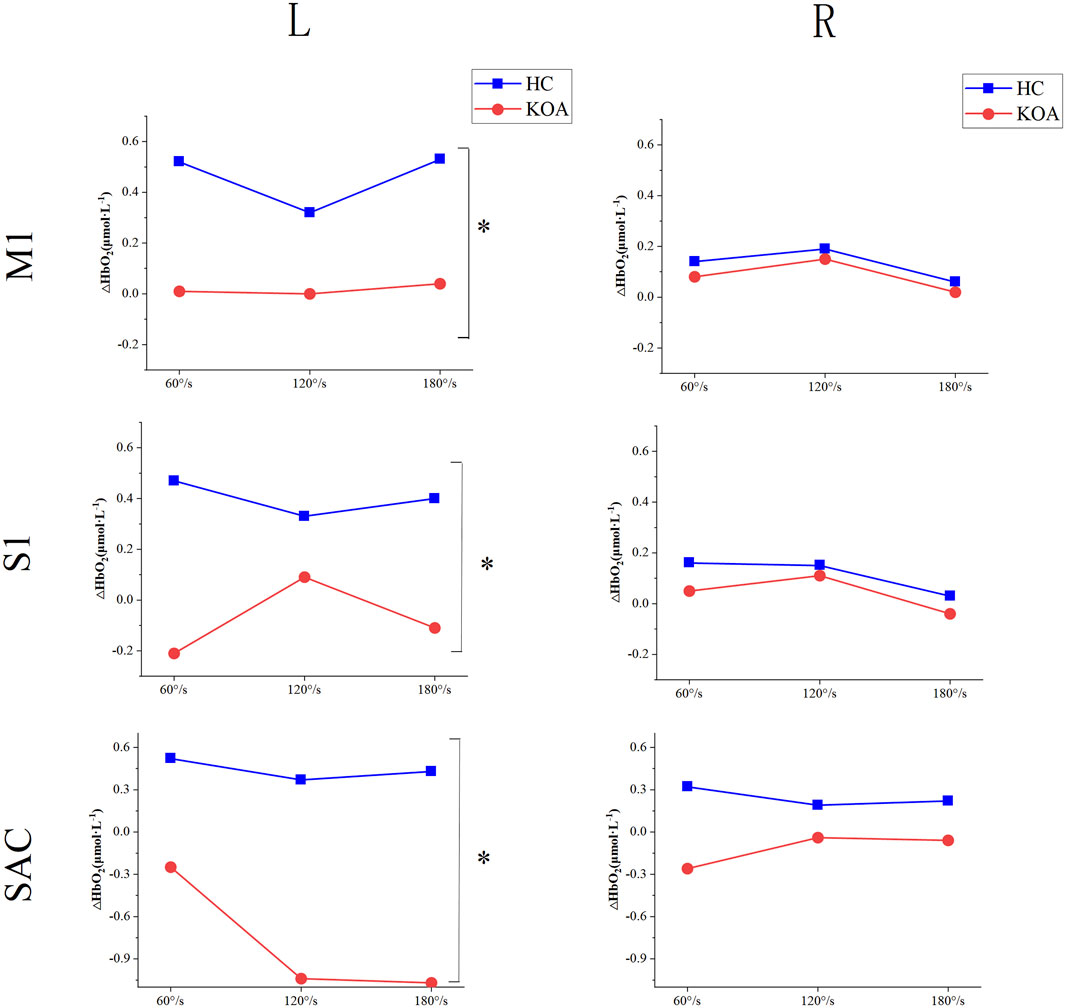
Figure 4. Differences in cerebral cortical activation between KOA patients and HC. KOA, knee osteoarthritis; HC, healthy controls; S1, primary somatosensory; M1, primary motor; SAC, somatosensory association cortex.
3.5 Correlations between cerebral cortical activation and clinical pain (VAS) and KOA functional symptoms (WOMAC) measures
For the clinical pain, correlation analysis revealed that the VAS scores in KOA patients were significantly negatively correlated with the left M1 (moderate correlation: r = −0.512, P = 0.030) and S1 (high correlation: r = −0.684, P = 0.002) values under isokinetic movement at 180°/s speed (Figure 5A). For the KOA functional symptoms, correlation analysis revealed that the WOMAC scores in KOA patients were significantly negatively correlated with the left M1 (moderate correlation: r = −0.583, P = 0.011) values under isokinetic movement at 180°/s speed (Figure 5B). In addition, there was no correlation between VAS and WOMAC and SAC.
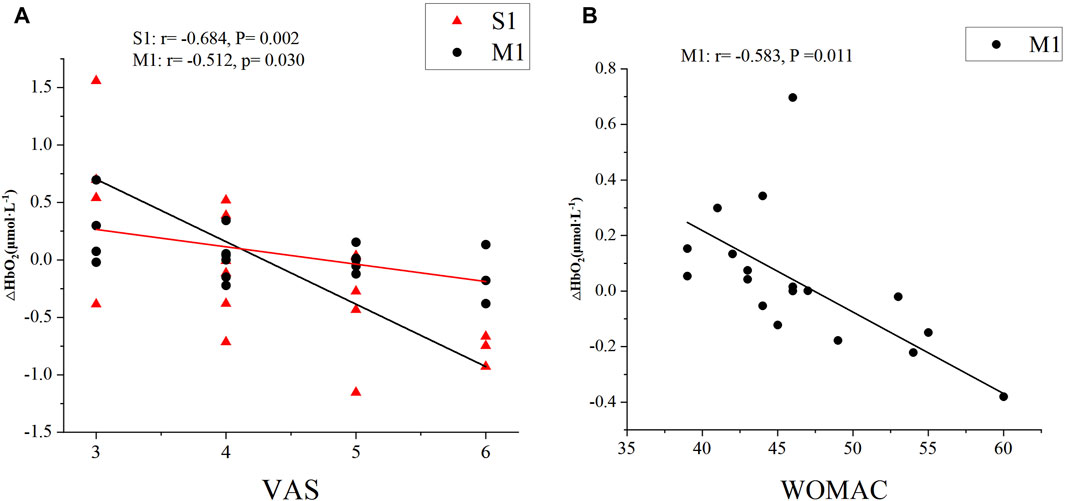
Figure 5. Correlations between cerebral cortical activation and (A) VAS, (B) WOMAC scores. VAS, Visual Analog Scale; WOMAC, Western Ontario and McMaster Universities Osteoarthritis Index; S1, primary somatosensory; M1, primary motor.
4 Discussion
The main objective of this study was to investigate the alterations in brain activation patterns in KOA patients during movement. Specifically, we compared the changes of cerebral cortical blood flow monitored by fNIRS between KOA patients and healthy subjects during isokinetic movement at different velocities. The correlation between changes in brain activation and pain severity and dysfunction in KOA patients were also evaluated to provide evidence and potential targets for brain activation changes for subsequent interventions, and open a new perspective for the rehabilitation of musculoskeletal diseases.
The results of peak torque showed that KOA patients exhibited significantly decreased muscle strength during isokinetic movements. This is consistent with previous findings that osteoarthritis patients exhibit decreased muscle strength and muscle imbalance (Neil et al., 2010; Pua et al., 2011). This may be due to the pathological changes of limited joint mobility and muscle function deterioration in osteoarthritis patients (Hideki et al., 2011). The limited joint mobility can reduce the range of motion of the muscles around the joint, thereby limiting the muscle’s ability to contract and unable to exert maximum force. Meanwhile, the chronic inflammatory response caused by osteoarthritis can also lead to muscle atrophy and dysfunction, further reducing the patient’s muscle strength level (Zhang et al., 2024). In addition, symptoms such as joint pain and muscle spasms may also inhibit the patient from exerting maximum muscle strength during the test. Therefore, the combined effects of limited joint mobility, muscle dysfunction, and pain lead to lower peak torque values in osteoarthritis patients compared to healthy individuals during isokinetic muscle strength testing. This provides a basis for developing targeted muscle strength training in clinical practice.
In this study, we used the fNIRS method as a neuroimaging tool for brain activation changes during movement. fNIRS has been recognized as a reliable and effective alternative to fMRI and EEG measures in previous research (Xu et al., 2010; Antonio et al., 2017). This method is a novel noninvasive technique that boasts attributes such as safety, portability, and the ability to tolerate motion artifacts, which enhance the methodological robustness and strength of studies investigating real-time brain networks during movement (Cristina et al., 2020; Alka et al., 2021).
Throughout the experimental activities, we observed that healthy people showed a large increase in cortical blood flow in the contralateral brain region during unilateral knee movement, including M1, S1and SAC. Many research had provided evidence for the lateralization of motor and somatosensory functions in the brain (Kwong et al., 1992; Jonathan P et al., 2010; Alahmadi et al., 2015). For example, Kwong and Jonathan et al. used fMRI technique to find that the contralateral motor cortex was significantly activated when subjects performed unilateral limb movements (Kwong et al., 1992; Jonathan P et al., 2010). Alahmadi et al. (2015) reported differences in activation changes of brain regions when using dominant versus non-dominant hands during a motor task, reflecting the lateralized characteristics of motor functions. Additionally, several studies have investigated the cortical activation patterns in response to unilateral limb stimulation, and found that single-sided limb stimulation led to significant activation in the contralateral cortical regions, supporting the lateralized nature of somatosensory processing (Bong Soo et al., 2003; Susan et al., 2008; Simone et al., 2010). Similarly, Baumgärtner et al. (2010) employed high-resolution fMRI and detected obvious somatotopic representations of heat and mechanical pain in the operculo-insular cortex, further demonstrating the lateralized organization of pain-related functions. Taken together, the results of this study are consistent with the above research, which proves that unilateral limb movement or sensory stimulation can cause the activation of the contralateral cortex, reflecting the anatomical and functional lateralization of motor and somatosensory functions.
However, in some disease states, such as osteoarthritis and stroke, activation of the cerebral cortex may be inhibited. In this study, it was found that compared with healthy subjects, the activation of the contralateral brain region in KOA patients was remarkable inhibited during limb movement. This phenomenon is also manifested in other diseases. For instance, Li et al. found that compared to healthy controls, patients with chronic ankle instability showed significantly decreased activation intensity of the motor cortex during unilateral movements (Li et al., 2024). Similarly, Hu et al. (2017) reported that stroke patients exhibited lower cortical activation levels than healthy individuals during movements. Furthermore, Karin et al. (2012) demonstrated that chronic pain patients also displayed reduced cortical activation during limb movements relative to healthy controls. These findings suggest that disease states may alter the patterns of cortical activation during movement, which may be related to impaired sensorimotor function in patients.
In addition, we observed that the activation of the cerebral cortex was not affected by the speed environment during limb movement at all speeds. This may be because under isokinetic muscle strength movement, we find that although the motion speed was different under different conditions, the torque force of the patient remains unchanged. Numerous studies have utilized fMRI and fNIRS to elucidate the relationship between brain activity in the motor regions and the levels of force exerted (Kenichi et al., 2014; Teo et al., 2023). It has been reported that the activation of contralateral M1 increases logarithmically with the increase of force output, which is inconsistent with the observed motion behavior (Karin B et al., 2012). Additionally, considering the presence of cortico-cortical connections from the sensory cortex to the motor cortex, it is reasonable to infer that information from S1 was involved in the control of movement, either through direct or indirect means. It is worth noting that the S1 region showed activation during movement in this study.
At the same time, we found that the clinical pain and dysfunction in KOA patients were significantly negatively correlated with activation levels of specific brain regions under isokinetic movement at 180°/s speed. Existing studies have shown that chronic pain can cause changes in the functional activity of the cerebral cortex, such as decreased activation in the sensory cortex, motor cortex, and prefrontal cortex, which may reflect the brain’s adaptive regulatory mechanism to persistent pain stimuli (Apkarian et al., 2011; Baliki et al., 2012). The results of this study further confirm this phenomenon, suggesting that the functional changes in the cerebral cortex of knee osteoarthritis patients may be the neurophysiological basis of their pain and functional impairment. This negative correlation may be due to the neuroplastic changes in the brain in response to chronic pain, including inhibition of sensory pathways, impairment of motor control, and imbalance of emotional regulation (Seminowicz et al., 2013). In addition, some studies have also found that through neuromodulation techniques such as tDCS, the activity of the cerebral cortex can be regulated, thereby improving the symptoms of patients with chronic pain (Khedr et al., 2005; Pollonini et al., 2020; Montero-Hernandez et al., 2023). These studies support the findings of this study, that is, the activation level of the cerebral cortex is closely related to pain perception. On the other hand, these showed correlations at speed of 180°/s, which may be mainly due to the low external interference at high speed and higher consistency of brain activation among patients. In summary, these research results provide important evidence for further exploring the neural mechanisms of pain and developing new treatment methods.
There are several limitations in this study that need to be considered. First, we only recruited right-sided KOA patients, so it is difficult to directly extrapolate results to left-sided or bilateral KOA patients. Second, we only performed simple unilateral limb movements, so we cannot know the effects of complex multi-limb movements on brain activation. Finally, the current study did not perform traditional neuroimaging (such as fMRI) assessments, limiting further understanding of the potential mechanisms that might explain the under-activation observed in patients with KOA. Further studies may address these shortcomings in the future by expanding the inclusion criteria of patients, conducting complex movement studies of multiple limbs, and using structural neuroimaging.
5 Conclusion
In conclusion, this study further clarified that there was high contralateral activation of the sensorimotor cortex during unilateral knee movement in healthy individuals, whereas contralateral cortical activation was suppressed in KOA patients. Furthermore, the clinical pain of KOA patients was negatively correlated with the activation level of the contralateral S1 region, and the dysfunction was negatively correlated with the activation level of the contralateral S1 and M1 regions. These findings can provide a better understanding of KOA brain science and are expected to contribute to the development of central intervention for the disease.
Data availability statement
The raw data supporting the conclusions of this article will be made available by the authors, without undue reservation.
Ethics statement
The studies involving humans were approved by the Ethics Committee of Shanghai Seventh People’s Hospital. The studies were conducted in accordance with the local legislation and institutional requirements. The participants provided their written informed consent to participate in this study. Written informed consent was obtained from the individual(s) for the publication of any potentially identifiable images or data included in this article.
Author contributions
KY: Data curation, Formal Analysis, Methodology, Software, Writing–original draft, Writing–review and editing. YD: Data curation, Formal Analysis, Methodology, Software, Writing–original draft, Writing–review and editing. LC: Conceptualization, Data curation, Formal Analysis, Writing–review and editing. CC: Data curation, Formal Analysis, Writing–review and editing. XY: Formal Analysis, Writing–review and editing. HX: Data curation, Writing–review and editing. YT: Formal Analysis, Writing–review and editing. TL: Formal Analysis, Writing–review and editing. LY: Formal Analysis, Writing–review and editing. XW: Formal Analysis, Validation, Writing–review and editing. BL: Conceptualization, Funding acquisition, Resources, Supervision, Validation, Writing–review and editing. LJ: Conceptualization, Funding acquisition, Resources, Supervision, Writing–original draft, Writing–review and editing.
Funding
The author(s) declare that financial support was received for the research, authorship, and/or publication of this article. This study was supported by grants through Shanghai Pudong New Area Foundation for Excellent Young Medical Talents (PWRq2022-19) and Shanghai Pudong New Area Foundation for Chinese Medicine Peak Discipline (YC-2023-0601) and Shanghai Pudong New Area Foundation for Shiyinyu National Famous Traditional Chinese Medicine Inheritance Studio (YC-2023-0120).
Acknowledgments
We thank all our participants for their participation in our experimental study, and also thank all our field experimentalists who contributed to all aspects of this study.
Conflict of interest
The authors declare that the research was conducted in the absence of any commercial or financial relationships that could be construed as a potential conflict of interest.
Publisher’s note
All claims expressed in this article are solely those of the authors and do not necessarily represent those of their affiliated organizations, or those of the publisher, the editors and the reviewers. Any product that may be evaluated in this article, or claim that may be made by its manufacturer, is not guaranteed or endorsed by the publisher.
References
Alahmadi, A., Pardini, M., Samson, R., D'Angelo, E., Friston, K., Toosy, A., et al. (2015). Differential involvement of cortical and cerebellar areas using dominant and nondominant hands: an FMRI study. Hum. brain Mapp. 36 (12), 5079–5100. doi:10.1002/hbm.22997
Alka, B., Roee, H., and Manuel E, H. (2021). Brain activation changes while walking in adults with and without neurological disease: systematic review and meta-analysis of functional near-infrared spectroscopy studies. Brain Sci. 11 (3), 291. doi:10.3390/brainsci11030291
Andres, M. L., William, D. H., and Suneil, K. K. (2017). What have we learned about movement disorders from functional neurosurgery? Annu. Rev. Neurosci. 40 (0), 453–477. doi:10.1146/annurev-neuro-070815-013906
Antonio, M. C., Filippo, Z., Francesco, D. P., and Arcangelo, M. (2017). Simultaneous functional near-infrared spectroscopy and electroencephalography for monitoring of human brain activity and oxygenation: a review. Neurophotonics 4 (4), 1. doi:10.1117/1.NPh.4.4.041411
Apkarian, V. A., Hashmi, J. A., and Baliki, M. N. (2011). Pain and the brain: specificity and plasticity of the brain in clinical chronic pain. Pain 152 (3 Suppl. l), S49–s64. doi:10.1016/j.pain.2010.11.010
Baliki, M. N., Petre, B., Torbey, S., Herrmann, K. M., Huang, L., Schnitzer, T. J., et al. (2012). Corticostriatal functional connectivity predicts transition to chronic back pain. Nat. Neurosci. 15 (8), 1117–1119. doi:10.1038/nn.3153
Baumgärtner, U., Iannetti, G., Zambreanu, L., Stoeter, P., Treede, R., and Tracey, I. (2010). Multiple somatotopic representations of heat and mechanical pain in the operculo-insular cortex: a high-resolution fMRI study. J. neurophysiology 104 (5), 2863–2872. doi:10.1152/jn.00253.2010
Bong Soo, H., Sung Ho, J., Yongmin, C., Woo Mok, B., Seung Kwan, L., and Duk Sik, K. (2003). Functional magnetic resonance image finding of cortical activation by neuromuscular electrical stimulation on wrist extensor muscles. Am. J. Phys. Med. Rehabil. 82 (1), 17–20. doi:10.1097/00002060-200301000-00003
Borot, L., Vergotte, G., and Perrey, S. (2018). Different hemodynamic responses of the primary motor cortex accompanying eccentric and concentric movements: a functional nirs study. Brain Sci. 8 (5), 75. doi:10.3390/brainsci8050075
Carlos, R.-L., David, B., Frank, P. L., Dieter, V. A., and Evelien, V. R. (2021). Reduced knee extensor torque production at low to moderate velocities in postmenopausal women with knee osteoarthritis. Scand. J. Med. Sci. Sports 31 (11), 2144–2155. doi:10.1111/sms.14035
Cheng, S., Dong, X., Zhou, J., Tang, C., He, W., Chen, Y., et al. (2022). Alterations of the white matter in patients with knee osteoarthritis: a diffusion tensor imaging study with tract-based spatial statistics. Front. Neurol. 13, 835050. doi:10.3389/fneur.2022.835050
Cristina, U., Stella, A., Turgut, D., Roee, H., Andrea L, R., Carmina, C.-T., et al. (2020). Functional near-infrared spectroscopy to study cerebral hemodynamics in older adults during cognitive and motor tasks: a review. Front. Aging Neurosci. 11 (0). doi:10.3389/fnagi.2019.00367
Cross, M., Smith, E., Hoy, D., Nolte, S., Ackerman, I., Fransen, M., et al. (2014). The global burden of hip and knee osteoarthritis: estimates from the global burden of disease 2010 study. Ann. Rheum. Dis. 73 (7), 1323–1330. doi:10.1136/annrheumdis-2013-204763
Eliassen, J. C., Souza, T., and Sanes, J. N. (2001). Human brain activation accompanying explicitly directed movement sequence learning. Exp. Brain Res. 141 (3), 269–280. doi:10.1007/s002210100822
Enrique, L., Jo, N., Carol A, C., Trudy, R., Vikki, W., Isabel, B., et al. (2017). Clinical descriptors for the recognition of central sensitization pain in patients with knee osteoarthritis. Disabil. Rehabil. 40 (23), 2836–2845. doi:10.1080/09638288.2017.1358770
Gary, S., Joseph, P. C., John, H. T., and David, A. B. (2002). A quantitative comparison of simultaneous BOLD fMRI and NIRS recordings during functional brain activation. Neuroimage 17 (2), 719–731. doi:10.1016/s1053-8119(02)91227-9
Glyn-Jones, S., Palmer, A. J., Agricola, R., Price, A. J., Vincent, T. L., Weinans, H., et al. (2015). Osteoarthr. lancet 386 (9991), 376–387. doi:10.1016/s0140-6736(14)60802-3
Hideki, T., Takumi, N., Kozo, N., and Lars, E. (2011). Prevention and management of knee osteoarthritis and knee cartilage injury in sports. Br. J. Sports Med. 45 (4), 304–309. doi:10.1136/bjsm.2010.082321
Hu, J., Du, J., Xu, Q., Yang, F., Zeng, F., Dai, X., et al. (2017). Altered coupling between motion-related activation and resting-state brain activity in the ipsilesional sensorimotor cortex after cerebral stroke. Front. Neurol. 8 (0), 339. doi:10.3389/fneur.2017.00339
Ian, J. W., Steven, W., David T, F., Robert D, J., Kimberly, T. W., Heli, M., et al. (2017). Knee osteoarthritis has doubled in prevalence since the mid-20th century. Proc. Natl. Acad. Sci. U. S. A. 114 (35), 9332–9336. doi:10.1073/pnas.1703856114
Jerin, M., Divya B, A., Matthew, H., Dirk, D. R., and Ramakrishnan, M. (2023). EEG-based cortical alterations in individuals with chronic knee pain secondary to osteoarthritis: a cross-sectional investigation. J. Pain 25 (5). doi:10.1016/j.jpain.2023.11.012
Jonathan, P. T., Steven, A. K., Keith, M., Chris, G., Mark, B., Michelle, B., et al. (2010). An fMRI study of the differences in brain activity during active ankle dorsiflexion and plantarflexion. Brain Imaging Behav. 4 (2), 121–131. doi:10.1007/s11682-010-9091-2
Kang, B. X., Ma, J., Shen, J., Xu, H., Wang, H. Q., Zhao, C., et al. (2022). Altered brain activity in end-stage knee osteoarthritis revealed by resting-state functional magnetic resonance imaging. Brain Behav. 12 (1), e2479. doi:10.1002/brb3.2479
Karin, B. J., Rita, L., Eva, K., Frank, P., Serena, C., Peter, F., et al. (2012). Patients with fibromyalgia display less functional connectivity in the brain's pain inhibitory network. Mol. Pain 8 (0), 1744-8069–1748-32. doi:10.1186/1744-8069-8-32
Kenichi, S., Naomi, K., and Junya, T. (2014). Changes in ipsilateral motor cortex activity during a unilateral isometric finger task are dependent on the muscle contraction force. Physiol. Meas. 35 (3), 417–428. doi:10.1088/0967-3334/35/3/417
Khedr, E. M., Kotb, H., Kamel, N. F., Ahmed, M. A., Sadek, R., and Rothwell, J. C. (2005). Longlasting antalgic effects of daily sessions of repetitive transcranial magnetic stimulation in central and peripheral neuropathic pain. J. Neurol. Neurosurg. Psychiatry 76 (6), 833–838. doi:10.1136/jnnp.2004.055806
Kocsis, L., Herman, P., and Eke, A. (2006). The modified Beer-Lambert law revisited. Phys. Med. Biol. 51 (5), N91–N98. doi:10.1088/0031-9155/51/5/n02
Kwong, K., Belliveau, J., Chesler, D., Goldberg, I., Weisskoff, R., Poncelet, B., et al. (1992). Dynamic magnetic resonance imaging of human brain activity during primary sensory stimulation. Proc. Natl. Acad. Sci. U. S. A. 89 (12), 5675–5679. doi:10.1073/pnas.89.12.5675
Leigh, F. C., Rebecca, J. C., Kelli, D. A., and Yvonne, G. (2021). Racial/ethnic, socioeconomic, and geographic disparities in the epidemiology of knee and hip osteoarthritis. Rheum. Dis. Clin. North Am. 47 (1), 1–20. doi:10.1016/j.rdc.2020.09.001
Li, H., Liu, J., Tian, S., Fan, S., Wang, T., Qian, H., et al. (2023). Language reorganization patterns in global aphasia-evidence from fNIRS. Front. Neurol. 13 (0), 1025384. doi:10.3389/fneur.2022.1025384
Li, Y., Wang, Z., Shen, Y., Yang, Y., Wang, X., Liu, H., et al. (2024). Differences in cortical activation during dorsiflexion and plantarflexion in chronic ankle instability: a task-fMRI study. Clin. Orthop. Relat. Res. 482 (5), 814–826. doi:10.1097/corr.0000000000002903
Mansfield, C., Culiver, A., Briggs, M., Schmitt, L., Grooms, D., and Oñate, J. (2022). The effects of knee osteoarthritis on neural activity during a motor task: a scoping systematic review. Gait and posture 96, 221–235. doi:10.1016/j.gaitpost.2022.05.035
Mantana, V., Patcharin, N., Sirikarn, S., and Benjawan, A. (2020). Identification of knee osteoarthritis disability phenotypes regarding activity limitation: a cluster analysis. BMC Musculoskelet. Disord. 21 (1), 237. doi:10.1186/s12891-020-03260-y
Montero-Hernandez, S., Pollonini, L., Park, L., Martorella, G., Miao, H., Mathis, K., et al. (2023). Self-administered transcranial direct current stimulation treatment of knee osteoarthritis alters pain-related fNIRS connectivity networks. Neurophotonics 10 (1), 015011. doi:10.1117/1.NPh.10.1.015011
Monticone, M., Capone, A., Frigau, L., Marongiu, G., Abelli, P., Mola, F., et al. (2018). Development of the Italian version of the High-Activity Arthroplasty Score (HAAS-I) following hip and knee total arthroplasty: cross-cultural adaptation, reliability, validity and sensitivity to change. J. Orthop. Surg. Res. 13 (1), 81. doi:10.1186/s13018-018-0782-5
Myles, P. S., Myles, D. B., Galagher, W., Boyd, D., Chew, C., MacDonald, N., et al. (2017). Measuring acute postoperative pain using the visual analog scale: the minimal clinically important difference and patient acceptable symptom state. Br. J. Anaesth. 118 (3), 424–429. doi:10.1093/bja/aew466
Neil, A. S., Natalie, A. G., David, T. F., Michael, H., Mei, Y., Michael, N., et al. (2010). Effect of quadriceps strength and proprioception on risk for knee osteoarthritis. Med. Sci. Sports Exerc 42 (11), 2081–2088. doi:10.1249/MSS.0b013e3181dd902e
Özgül, Ö., Zeliha Candan, A., Hasan, B., and Sinem Burcu, E. (2021). Changes in prefrontal cortex activation with exercise in knee osteoarthritis patients with chronic pain: an fNIRS study. J. Clin. Neurosci. 90 (0), 144–151. doi:10.1016/j.jocn.2021.05.055
Pollonini, L., Miao, H., and Ahn, H. (2020). Longitudinal effect of transcranial direct current stimulation on knee osteoarthritis patients measured by functional infrared spectroscopy: a pilot study. Neurophotonics 7 (2), 1. doi:10.1117/1.NPh.7.2.025004
Pua, Y., Liang, Z., Peck-Hoon, O., Adam, L. B., Ngai-Nung, L., and Ross, A. C. (2011). Associations of knee extensor strength and standing balance with physical function in knee osteoarthritis. Arthritis Care Res. Hob. 63 (12), 1706–1714. doi:10.1002/acr.20615
Seminowicz, D. A., Shpaner, M., Keaser, M. L., Krauthamer, G. M., Mantegna, J., Dumas, J. A., et al. (2013). Cognitive-behavioral therapy increases prefrontal cortex gray matter in patients with chronic pain. J. Pain 14 (12), 1573–1584. doi:10.1016/j.jpain.2013.07.020
Seror, R., Tubach, F., Baron, G., Falissard, B., Logeart, I., Dougados, M., et al. (2007). Individualising the Western Ontario and McMaster Universities osteoarthritis index (WOMAC) function subscale: incorporating patient priorities for improvement to measure functional impairment in hip or knee osteoarthritis. Ann. Rheum. Dis. 67 (4), 494–499. doi:10.1136/ard.2007.074591
Shanahan, C. J., Hodges, P. W., Wrigley, T. V., Bennell, K. L., and Farrell, M. J. (2015). Organisation of the motor cortex differs between people with and without knee osteoarthritis. Arthritis Res. Ther. 17 (1), 164. doi:10.1186/s13075-015-0676-4
Simone, P., Filippo, Z., Stefano, V., Francesca, M., Matilde, E., Francesco, P., et al. (2010). Primary sensory and motor cortex activities during voluntary and passive ankle mobilization by the SHADE orthosis. Hum. Brain Mapp. 32 (1), 60–70. doi:10.1002/hbm.20998
Stephane, P., and Pierre, B. (2018). Studying brain activity in sports performance: contributions and issues. Prog. Brain Res. 240 (0), 247–267. doi:10.1016/bs.pbr.2018.07.004
Susan, F., Xia, L., Samia, A., Thomas, P. W., Margaret, P., Richard, B., et al. (2008). fMRI analysis of active, passive and electrically stimulated ankle dorsiflexion. Neuroimage 44 (2). doi:10.1016/j.neuroimage.2008.09.017
Teo, W., Clara Xinru, T., Alicia M, G., Saqif, M., Ang, Y., and Christopher, L. (2023). Brain activation associated with low- and high-intensity concentric versus eccentric isokinetic contractions of the biceps brachii: an fNIRS study. Scand. J. Med. Sci. Sports 34 (1). doi:10.1111/sms.14499
Theodore, J. H., Solomon, G. D., Maria, A. F., and David, A. B. (2009). HomER: a review of time-series analysis methods for near-infrared spectroscopy of the brain. Appl. Opt. 48 (10), D280. doi:10.1364/ao.48.00d280
Xu, C., Signe, B., Daniel, M. B., Gary, H. G., and Allan, L. R. (2010). A quantitative comparison of NIRS and fMRI across multiple cognitive tasks. Neuroimage 54 (4). doi:10.1016/j.neuroimage.2010.10.069
Ye, Y., Yan, X., Wang, L., Xu, J., and Li, T. (2023). Transcranial direct current stimulation attenuates chronic pain in knee osteoarthritis by modulating BDNF/TrkB signaling in the descending pain modulation system. Neurosci. Lett. 810, 137320. doi:10.1016/j.neulet.2023.137320
Keywords: knee osteoarthritis, cortex activation, functional near-infrared spectroscopy, isokinetic, pain
Citation: Yang K, Ding Y, Chu L, Cheng C, Yu X, Xu H, Tao Y, Liu T, Yin L, Wu X, Liu B and Jiang L (2024) Altered activation patterns of the sensory-motor cortex in patients with knee osteoarthritis during knee isokinetic movement at different speeds. Front. Bioeng. Biotechnol. 12:1444731. doi: 10.3389/fbioe.2024.1444731
Received: 06 June 2024; Accepted: 09 August 2024;
Published: 21 August 2024.
Edited by:
Divya Bharatkumar Adhia, University of Otago, New ZealandReviewed by:
Jerin Mathew, University of Otago, New ZealandSentong Wang, The University of Electro-Communications, Japan
Copyright © 2024 Yang, Ding, Chu, Cheng, Yu, Xu, Tao, Liu, Yin, Wu, Liu and Jiang. This is an open-access article distributed under the terms of the Creative Commons Attribution License (CC BY). The use, distribution or reproduction in other forums is permitted, provided the original author(s) and the copyright owner(s) are credited and that the original publication in this journal is cited, in accordance with accepted academic practice. No use, distribution or reproduction is permitted which does not comply with these terms.
*Correspondence: Liming Jiang, qyjlm1487@163.com; Bingli Liu, gukelaoliu@126.com
†These authors share first authorship