- 1Institute of Industrial Biotechnology (IIIB), GC University Lahore, Lahore, Pakistan
- 2Department of Biology, Faculty of Science, University of Tabuk, Tabuk, Saudi Arabia
- 3School of Applied Sciences, University of Huddersfield, Huddersfield, United Kingdom
The persistent expansion in world energy and synthetic compounds requires the improvement of renewable alternatives in contrast to non-sustainable energy wellsprings. Lignocellulose is an encouraging feedstock to be utilized in biorefineries for its conversion into value-added products, including biomaterials, biofuels and several bio-based synthetic compounds. Aside from all categories, biofuel, particularly bioethanol is the most substantial fuel derived from lignocellulosic biomass and can be obtained through microbial fermentation. Generally, extreme settings are required for lignocellulosic pretreatment which results in the formation of inhibitors during biomassdegradation. Occasionally, lignin polymers also act as inhibitors and are left untreated during the pretreatment, engendering inefficient hydrolysis. The valorization of lignocellulosic biomass by laccases can be viewed as a fundamental trend for improving bioethanol production. However, one of the main obstacles for developing commercially viable biofuel industries is the cost of enzymes, which can be resolved by utilizing laccases derived from microbial sources. Microbial laccases have been considered an exceptionally integral asset for delignification and detoxification of pretreated LCB, which amplify the resultant fermentation and saccharification processes. This review provides a summary of microbial laccases and their role in valorizing LCB to bioethanol, compelling enthralling applications in bio-refining industries all across the globe.
1 Introduction
Renewable fuels are referred as a favorable substitute for global warming besides diminishing dependence on fossil fuels (Fillat et al., 2017). During the end of the 20th century, renewed interests have been developed in the utility of bioethanol and biodiesel (Srivastava et al., 2020). Bioethanol production as a substitute for gasoline is a major concern these days due to its burning speed, elevated antiknock rating, and vaporizing temperature. The traditional (1st generation) bioethanol production is from starch and sugar feedstocks yet, corn, sugarcane juices, and treacle are also being utilized (Mishra and Ghosh, 2016). Conversely, lignocellulosic biomass is perceived as a significant crude material for biofuels, particularly for advanced (2nd generation) bioethanol production (Lin and Lu, 2021) owing to its minimal cost (Rezania et al., 2020) and is also employed at commercial scale production of bioethanol, since back in 2013 (Su et al., 2020). Bioethanol has been proven to release lower greenhouse gas (GHG) emissions as compared to petroleum-based fuels, especially when using sustainable feedstocks and production methods. Studies have shown a reduction of GHG emissions by 12%–19% when using a 10% ethanol blend in gasoline compared to regular gasoline (Graham et al., 2008; Han et al., 2015). Bioethanol derived from certain sources like switchgrass can achieve over 60% reduction in GHG emissions compared to gasoline. In contrast to other fuels, bioethanol is environmentally benign, can be effortlessly stored and is also an alluring choice for the transport sector, consequently delineating its favorability over petroleum and other fuels. Likewise, every year, the bioethanol production rate is escalating globally on account of its vast applications in industrial, pharmaceutical, and cosmetic practices (Choo et al., 2019; Lecksiwilai and Gheewala, 2020).
Lignocellulosic raw materials used for producing bioethanol are usually cheap and cultivable, which ultimately makes the process cost-effective and beneficial for the local economy (Bayrakci Ozdingis and Kocar, 2018). Biochemical changing of lignocellulose addresses the most ideal course among all scientific advancements (Fillat et al., 2017) and involves three significant steps; pretreatment enzymatic hydrolysis, and fermentation (Chen and Liu, 2015; Liu and Chen, 2016). Chemicals (e.g., hydrochloric acid, phosphoric acid, and hydrogen sulfide), biofuels (such as bioethanol, bio-hydrogen, and bio-butanol), and other biopolymers (like lignocellulosic biopolymers) produced as a result of pretreatment participates in the biochemical transformation towards disturbing the firmly related arrangements of lignin, cellulose, and hemicellulose molecules (Kim, 2018). While, during enzymatic hydrolysis, monosaccharides created by the hydrolysis of polysaccharides eventually transformed into ethanol through the process of fermentation by utilizing several microorganisms (Chen and Liu, 2017). Pretreatment is referred as an elementary step for LCB valorization. Yet, despite all its assets, it also exhibits some restrictions such as pretreatment of LCB is not an ecofriendly method as it entails traditional procedures of heating and employment of strong chemicals (Hassan et al., 2018). The process also results in the production of various inhibitors against several microbes along with other enzymes which hinders the fermentation process and ethanol generation, implicating a gridlock for the production of bioethanol (Jönsson and Martín, 2016).
Laccases are frequently utilized for valorizing the LCB and are much vital approach for bioethanol production as the methodology is convenient and also supports the viability at a commercial scale. Laccases are unique oxidative enzymes that produce two water molecules by the reduction of oxygen being their only co-substrate (Hashmi et al., 2016). They are widely utilized in enhancing bioethanol production by efficiently taking off the inhibitory contents of pretreated biomass via delignification and detoxification processes (Moreno et al., 2020), playing a central part in lignin modification and degradation. Moreover, the extended specificity of laccases for substrate makes them appropriate for industrial use as well (Agrawal et al., 2018). According to the international laccase market research report in 2018, overall laccase consumption has been increased to 166 MT from 140.75 MT in just the past 3 years. There are various means by which laccases can be obtained, i.e., plants, animals (Janusz et al., 2020), and microbes. However, microbial laccases are extensively preferred as it is a cheap source, make the process economical, and competently oxidize the toxic and non-toxic substrates. Microbial laccases are usually produced from fungal or bacterial species and are widely employed for the detoxification and delignification of different pretreated and processed un-pretreated feedstocks (Figure 1), either alone or with a laccase mediator system (LMS) (Bayrakci Ozdingis and Kocar, 2018). This review aims to provide an inclusive overview of microbial sources of laccases and also sums up the role of microbial laccases in the valorization of LCB for efficient bioethanol production.
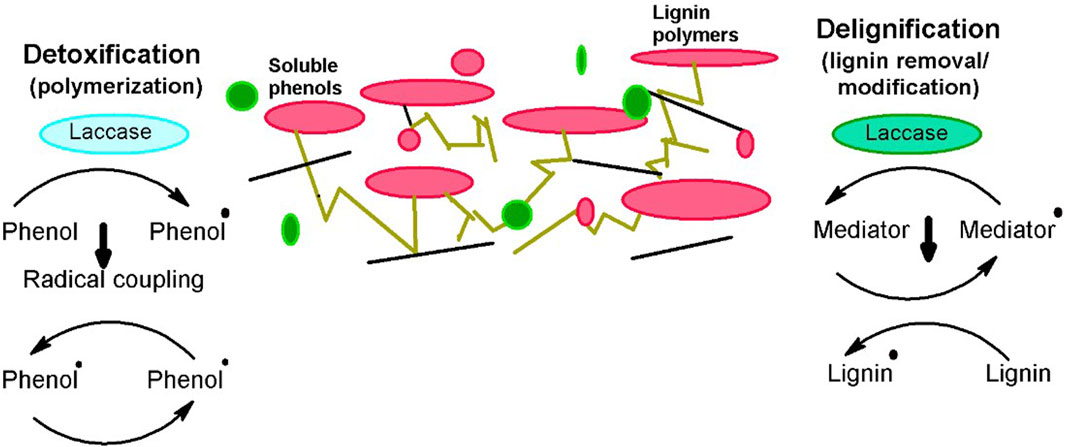
Figure 1. Role of lacasses for efficient conversion of biomass into bioethanol (Fillat et al., 2017).
2 Lignocellulosic biomass conversion
Lignocellulosic biomass can produce maximum sugar amounts through fermentation, ultimately mounting the production of biofuel (E4tech Re-Cord, and Wur, 2015; Fillat et al., 2017). Conversion of LCB involves the processes of pretreatment and hydrolysis however, bioethanol production is chiefly dependent on the hydrolysis step (Binod et al., 2011). Yet, for efficient hydrolysis, pretreatment is regarded as a crucial step to alter lignocellulosic structure (Fillat et al., 2017).
2.1 Pretreatment
Pretreatment of LCB is an imperative procedure to make lignin, cellulose, and hemicellulose more pertinent for enzymatic action by increasing amorphous regions. These products are the core building blocks of lignocellulosic compounds, which makes them defiant to the action of hydrolytic enzymes (Santos et al., 2015). Utilization of LCB for its biochemical conversion into biorefineries necessitates pretreatment to disrupt the inter-component associations between these constituents in the cell wall (Kumari and Singh, 2018). The main objectives of pretreatment comprise; creating a disturbance in the crystalline arrangement of cellulose, attaining efficient hemicellulose elimination and lignin degradation, enhancing the permeability of pretreated constituents, declining the degree of polymerization, and bioethanol production with bare minimum cost (Ji et al., 2017; Espirito Santo et al., 2018; Solarte-Toro et al., 2019). To make the process profitable, pretreatment must help escalate the effectiveness of hydrolysis and fermentation by diminishing the inhibitors besides raising the sugar formation (Chiaramonti et al., 2012). Several advancements have been carried out regarding the pretreatment of LCB, as illustrated in Figure 2.
2.2 Hydrolysis of LCB
Hydrolysis is performed to depolymerize lignocellulosic biomass into simpler sugars, and the degree of hydrolysis relies on the structural arrangements of lignocellulosic compounds. In comparison to hemicellulose, hydrolysis of cellulose is quite tricky due to its crystalline arrangements and the presence of β-D1, 4-glycosidic linkages. This indestructible structural organization makes cellulose exceedingly dense and hard to rupture. Physical (mechanical processes, milling, extrusion, microwave radiation, and extrusion), physicochemical (steam explosion, liquid hot water, ammonium fibre, and CO2 explosion, and wet oxidation), and chemical (alkaline, acid, ionic liquid, organo-solvent, technologies, deep eutectic solvent) pretreatment technologies effectively break down tough biomass for further processing, but they have several limitations. For instance, physical pretreatment methods are energy and cost-intensive as they operate by creating variations in pressure and temperature simultaneously. Thus, requiring high power, energy consumption, and increased production costs. While, chemical pretreatment generates harmful effluents and inhibitors, ultimately causing environmental contamination (Chami Khazraji and Robert, 2013; Abo-State et al., 2014; Shukla et al., 2023). For chemical hydrolysis, dilute, and strong acids are usually utilized. Hydrolysis with dilute acid requires 1%–3% sulfuric acid concentration at a temperature ranging from 180°C to 240°C. During this process, there also appears the formation of several inhibitors, succeeding in the production of hydrolysate and generating little sugar yield. However, the problem can be resolved by setting two temperature ranges such as 140°C–160°C and 160°C–180°C for hemicellulose solubilization and cellulose transformation, respectively, which upsurges the product recovery rate up to 20%. Hydrolysis with strong acid necessitates 20%–40% acid concentration with temperature maintained at 50°C–100°C, leading towards maximum yield, i.e., up to 90% (Hamelinck et al., 2005). Although chemical hydrolysis exhibits several positive points yet, it is unappealing and non-competitive due to the production of inhibitors (Abo et al., 2019).
Enzymatic hydrolysis is a type of hydrolytic reaction by which sugars are set free after the pretreatment of LCB. Ligninases, cellulases, and hemicellulases are the enzymes that usually catalyze this reaction (Rosdee et al., 2020). It is an eco-friendly process and is the foremost step for bioethanol production with minimal energy charge. This process offers a relatively lower generation of acid waste, inhibitory compounds, and other unwanted products. Furthermore, it also eliminates the requirement for corrosion-free equipment for processing (Moreno et al., 2017; Amit et al., 2018). Despite all these positive aspects, some limitations are also associated with enzymatic hydrolysis, such as lignin residues left in pretreated materials limit the process by inhibiting the availability of sugars for the action of hydrolytic enzymes or vice versa, ultimately diminishing the saccharification yield. Various enzymes or substrate-related factors in both positive and negative aspects affect enzymatic hydrolysis. However, enzymatic hydrolysis is still preferred over chemical one because of the non-toxic and biodegradable nature of the enzymes (Pino et al., 2018). To augment the efficiency of enzymatic hydrolysis, the copper-containing oxidases family, i.e., laccases are being used extensively for biofuel (particularly bioethanol) production. Laccases have vast practices in many industrially crucial processes and other biotechnological fields into the bargain. Moreover, laccases can oxidize phenolic, non-phenolic, toxic, and non-toxic substrates, further raising their global value (Hilgers et al., 2018). The potential of microbial lacasses in pretreating LCB residues for bioethanol production has been well studied in the literature. Lacasses degrade the complex polyphenol structure that constitutes lignin, which is the chief recalcitrant component in lignocellulosic structure. Lacasses being extracellular, inducible, and less specific are widely utilized for biomass pretreatment for biofuel production (Plácido and Capareda, 2015).
3 Role of microbial laccases in valorization of lignocellulosic biomass to bioethanol
During pretreatment of LCB, some inhibitors such as phenolic compounds, furan derivatives, levulinic acid, aromatic and inorganic compounds, aliphatic acids, formic acids, and extractives are produced as by-products which create hindrance during the hydrolysis (Maulana Hidayatullah et al., 2020; Qiao et al., 2021). Furthermore, lignin residues in pretreated lignocellulosic materials compress the concentration of hydrolytic enzymes by adsorbing them non-specifically, eventually constraining the saccharification process (Berlin et al., 2006). Laccase-mediated detoxification and delignification processes are generally carried out to decrease the inhibitor concentration and eliminate lignin compounds. These processes require no additional chemicals and occur with minimum energy consumption under milder conditions (Moreno et al., 2015). They also augment the accessible surface area and play a crucial role in reducing the ineffective joining of hydrolytic enzymes, eventually enhancing the worth of both processes (Fillat et al., 2017).
3.1 Why microbial laccases?
As described earlier, laccases are nonspecific and versatile enzymes that are involved in a wide range of processes, including lignin degradation, biofuel synthesis, and biorefinery of biomasses. Besides biofuels production, lacasses are widely utilized for the synthesis of pigments and are also known for their role in the production of antibiotic compounds. They are also extensively studied for their applications in the production of feed, food, and several other consumer products. Furthermore, they are involved in detoxification of toxic compounds and also have potential applications in bioremediation and wastewater treatment (Tiwari et al., 2023). Lacasses also play a vital role in generating bio-based chemicals such as vanillin, catechol, and syringol (Kim, 2021). Moreover, laccase can facilitate the oxidation of lignin compounds, which makes it easier to extract and utilize the sugar molecules and lignocellulosic material components for the production of bio-based plastics (Farmer et al., 2018).
Over the past, fossil fuels were widely utilized as an energy source, both in the industrial and transportation sectors. The increase in energy demand and depletion of fossil fuels augmented the advent of biofuels in the 19th century. In the mid-1970s, biofuel gained much attention in several countries, including Brazil and the United States, due to their fewer toxic effects and more economic gains over fossil fuels. Since then, the utilization of biofuels has been upgraded to 52,219 Ktoe (Kilotonnes of oil equivalent) from 8,082 thousand tonnes (Importance, 2014). Laccases are widely used for bioethanol production (A., 2013) and turned out to be the most efficient tool for valorizing LCB beyond its vast applications in other industrial sectors. However, the cost of these enzymes makes them unbefitting for implementation on a commercial scale. Concisely, laccases have the potential to be an eco-friendly tool for pretreatment of lignocellulosic materials’ delignification and detoxification, enhancing the fermentation and saccharification processes in biorefineries. Literature also depicts that Trichoderma asperellum strain BPLMBT1’s thermostable laccase efficiently delignified LCB, facilitating the synthesis of biohydrogen from delignified biomass (Shanmugam et al., 2018).
For this reason, interest has ascended in the production of laccases by microbial sources to reduce the process expenses. Microbial laccases are competent catalysts and exhibit high stability. They possess broad specificity for substrate and can be easily cloned and expressed in the host. Furthermore, they can oxidize both non-toxic and toxic substrates and also acquire higher redox potential, providing economical services for industrial purposes as presented in Figure 3 (Shraddha et al., 2011; Singh et al., 2011; Prins et al., 2015; Mate and Alcalde, 2017).
3.2 Microbial sources of laccases
Yoshida initially described laccases, identified from a tree named Rhus vernicifera (Wang Z. et al., 2018). Presently, laccases are recognized and studied chiefly in fungi, but these enzymes are also present in some bacterial species as well. White rot fungi are major producers of extracellular laccases throughout their secondary metabolism (Rodríguez-Couto, 2017; Singh and Gupta, 2020). Laccases have been purified from several strains of fungi (Guimarães et al., 2016), but the Trametes genus is typically studied (Wang Q. et al., 2018). Furthermore, laccases produced from basidiomycetes and ascomycetes possess high redox potential, which makes these fungal groups most significant (Wu et al., 2019). Studies have also indicated the active production of laccases from Coprinus comatus, Lentinus edodes, and Lepista luscina sp. Unlike fungal species, laccases purified from bacterial strains have some exceptional characteristics such as bacterial-derived laccases are either extracellular or intracellular and are usually stable even at high pH and temperature (30°C–80°C) ranges, making them a potential candidate for laccases production (Chauhan et al., 2017). Among bacterial species, Streptomyces, Azospirillum, Rhodococcus, Geobacillus, Staphylococcus, Lysinibacillus sphaericus, Bacillus spp., Aquisalibacillus, Pseudomonas, Delftia, Alteromonas, and Enterobacter are some main sources (Axelsson et al., 2012; Muthukumarasamy et al., 2015; Neifar et al., 2016; Rezaei et al., 2017). Moreover, some yeast species naturally produce laccases, including Kluyveromyces lactis, Pichia methalonica, and Pichia pastoris, etc. Some yeast species are also being utilized for recombinant laccases production and basidiomycetes yeast are the most important among all. Some chief microbial sources of laccases are given in Table 1.
Compared to other industrial processes, laccases production through fermentation is considered low to moderate in terms of energy consumption. Fermentation primarily uses energy for maintaining culture growth conditions like temperature and agitation. However, these requirements are generally less demanding than those needed for high-heat chemical processes. Even purification often utilizes chromatography techniques, which may involve low-pressure pumps and refrigeration. The sterile environment required for laccase production disrupts the natural microbial communities present in bioreactors and the specific growth medium and conditions used for laccase-producing organisms favor their growth over other microbes (Malhotra and Suman, 2021).
4 Laccases; structure, functions, and mechanism
Microbial laccases are a group of versatile enzymes with immense potential in various biotechnological applications, particularly those related to biomass conversion. Lacasses are superior to other enzymes such as peroxidases and cellulases because they have broader substrate specificity, oxidizing a wide range of phenolic and non-phenolic compounds using molecular oxygen (O2) as the final electron acceptor. While, peroxidases primarily target specific electron donors in the presence of H2O2 (Rodríguez-Couto, 2021). Laccase pretreatment can improve cellulose accessibility for cellulases, facilitating biofuel production or other applications. It can be better understood by discussing the structure of laccases.
Laccases are usually dimeric or tetrameric glycoproteins, exhibiting 500 amino acids organized into three domains, having beta-barrel topology. They contain four copper atoms per monomer at their active sites, which partake in water production by reducing oxygen molecules. Laccases are classified into three main groups; blue copper (type-1 copper), normal copper (type-2 copper) and binuclear copper cores (type-3 copper) (Figure 4) (Plácido and Capareda, 2015; Yang et al., 2017). Every four atoms of copper have their particular attribute towards electron spin resonance signals. Type-1 copper is blue copper due to its intense absorption close to 600 nm, ensuing blue color. Blue copper has ligand collaboration among one methionine plus two histidine and cysteine molecules and exhibits high redox potential and capacity to oxidize the substrate. Some laccases show lesser absorption, i.e., near 600 nm, and are termed white and yellow laccases depending on the oxidation state of the Zn, Cu, and Fe atoms they contain (Radveikienė et al., 2021; Sondhi et al., 2021). Type-2 or normal copper exhibits zero absorption in the visible spectrum range (Wang S. N. et al., 2018) and uses water and 2 histidine molecules as a ligand (Babiker and Hamadttu, 2019). Type-3 copper uses one hydroxyl bridge and three histidine molecules as ligands, displaying absorption at 330 nm. Laccases have also been categorized into high and low redox potential laccases, notably fungal and bacterial laccases, respectively (Yin et al., 2019; Janusz et al., 2020) on account of the properties and structures of laccases copper cores and ligands along with the distance between the copper atoms (Rencoret et al., 2008). This unique structural arrangement of laccases helps in toxin and lignin degradation of LCB, validating worth functioning of the enzyme (Bagewadi et al., 2017; Janusz et al., 2020).
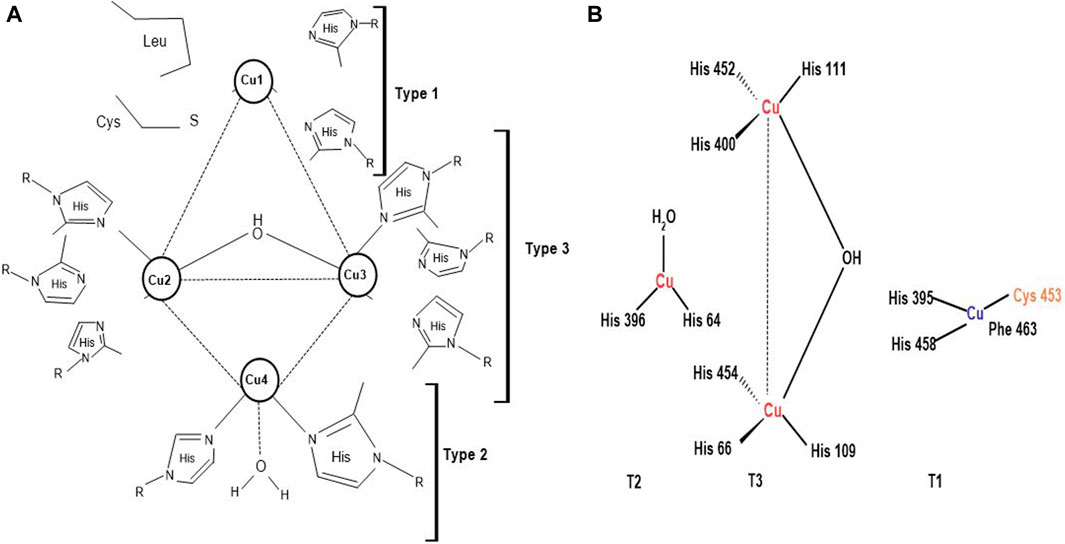
Figure 4. (A) Copper coordination network of laccases; Type 1: Blue copper, Type 2: Normal copper, and Type 3: Binuclear copper core (Agrawal et al., 2018) (B) Active site of laccases (Prajapati, 2018).
4.1 Mechanism of action of laccases
Oxidation catalyzed by laccases can be direct, indirect, or mediator-associated depending upon the substrate size and redox potential. During direct oxidation at Type-1 copper atom, unsettled reactive radicals are generated by one electron substrate oxidation. After four consecutive cycles, the electrons become capable of producing two water molecules by reducing one molecule of oxygen (Kudanga et al., 2011). For mediator-associated or indirect oxidation, the laccase mediator system is used. LMS oxidizes small substrates with little redox potential at first and then uses its generated radicals for oxidizing complex substrates with relatively higher redox potential, which makes it imperative for biofuel manufacturing (Kudanga and Le Roes-Hill, 2014).
As described earlier, laccases produce reactive radicals by substrate oxidation resulting in the generation of water molecules by reducing oxygen with the engagement of copper atoms present at their catalytic center. These copper atoms are also involved in the degradation of lignin. In the very first step, blue copper oxidizes lignin. Later on, Type-1 copper transfers electrons to Type-2, which further transfers these electron to Type-3 (Bishwakarma et al., 2016). Substrates including aromatic diamines, polyphenols, and methoxy-substituted phenols are generally oxidized by laccases via Cα oxidation, Cα-Cβ cleavage and alkyl-aryl cleavage. The cofactor of laccase is oxygen rather than hydrogen per oxidase, necessitating its role during the delignification process. In some cases, laccases require mediators for the aforesaid process due to its vast magnitude and complex lignin structure (Singh Arora and Kumar Sharma, 2010; Sanchez et al., 2011). The mechanism of laccases action is illustrated in Figure 5.
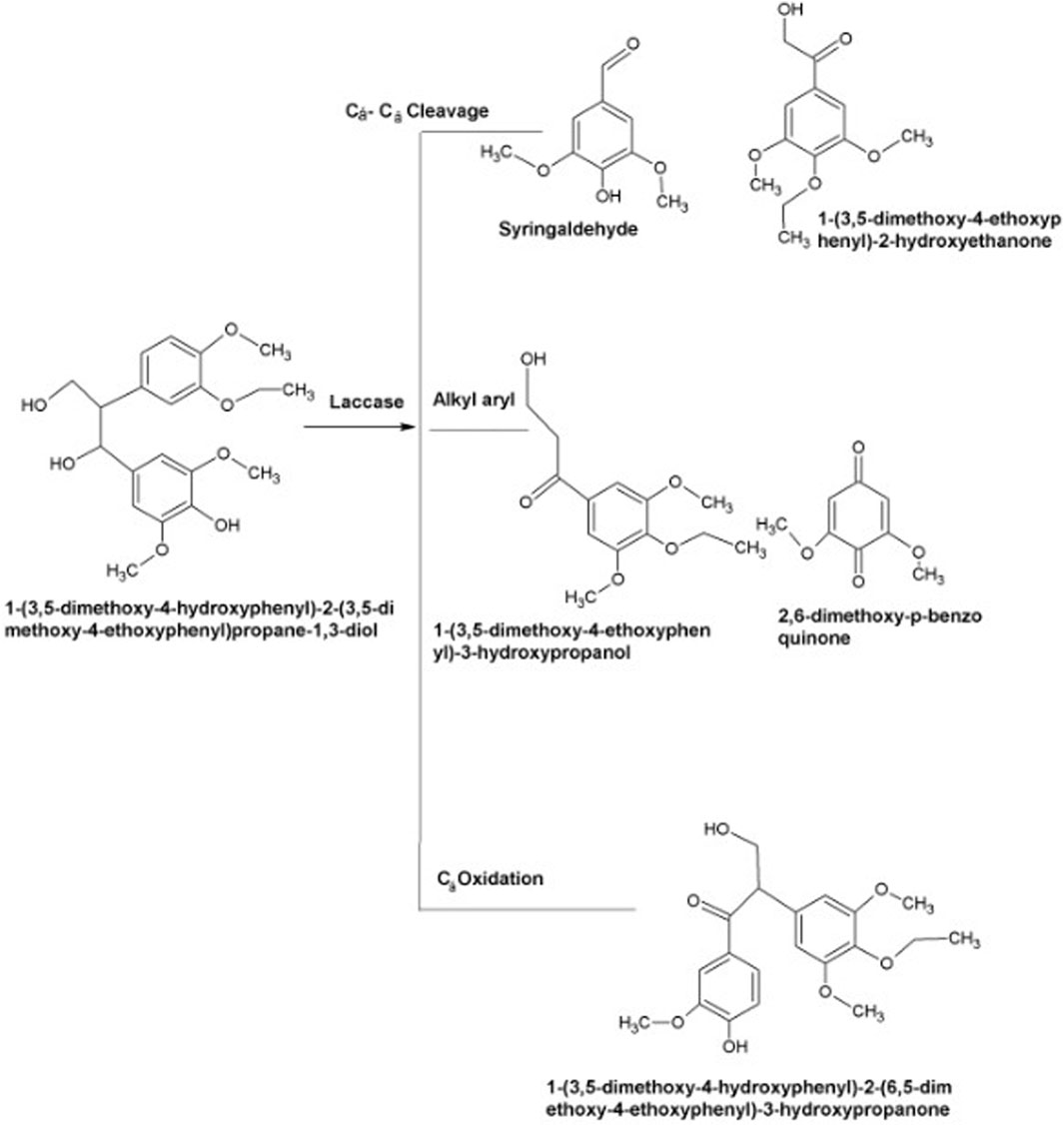
Figure 5. Mechanism of lignin compound (agro-industrial waste) oxidation via laccases as a substitute to acquire fermentable carbohydrates for fuel making (Wei and Xianghua, 2009; Sanchez et al., 2011).
5 Laccase practices for detoxification of pretreated material
Laccases are extensively used to minimize the toxicity of various pretreated compounds and are exceptionally helpful in the detoxification of LCB, eventually resulting in increased efficiency of microbial fermentation (Jurado et al., 2009; Moreno et al., 2012, 2013a; Guo et al., 2013). The pH is a significant factor for the efficient functioning of laccases as slight variations in pH lead to structural alterations in enzymes, consequently making them inactive. These enzymes produce phenoxy radicals by oxidizing phenolic compounds (Sun et al., 2021) and by interacting with pretreated lignocellulosic material via polymerization, yielding oligomers with low inhibitory power (Parawira and Tekere, 2011). Studies have shown that laccases reduce the incidence of pre-hydrolysate phenolic compounds from 88% to 92% (Moreno et al., 2012).
5.1 Microbial laccases mediated detoxification
Laccases being oxidative enzymes, cleave the carbon-carbon and ether bonds in lignin during the formation of phenoxy radicals (De La Torre et al., 2017). Kolb et al. (2012) described the catalytic activities of laccases produced from Trametes versicolor while working on inhibitory compounds. It was proposed that in laccase presence, the reaction time of 1 h for ferulic acid, syringaldehyde, and p-coumaric, 24 h for vanillin, and more than 1 week for 4-hydroxybenzaldehyde is required for their complete removal. Studies have reported 76% and 92%–95% phenol reduction by treating pretreated LCB with laccases extracted from Coltricia perennis (Martínez et al., 2009; Kalyani et al., 2012) and C. rigida or Pycnoporus cinnabarinus, respectively (de Abreu Domingos and da Fonseca, 2018). Two other studies have also demonstrated similar results for the reduction of phenolic compounds in pretreated biomass by treating them with P. cinnabarinus, C. rigida, T. versicolor and Thapsia villosa derived laccases (Kalyani et al., 2012; Moreno et al., 2012).
Laccase activity for phenolic compounds can be enhanced by electron-releasing groups, which lessen the electrochemical potential of relative phenols. Moreover, the presence of methoxy and ethylene groups is also concerned with the escalation of laccases affinity for phenolic compounds (Camarero et al., 2005; 2008). Fungal laccases are more active toward phenols than bacterial laccases due to relatively high redox potential (Glazunova et al., 2018).
5.2 Detoxification through microbial laccases and its effect on enzymatic saccharification
The biofuel production from laccases pretreated lignocellulosic biomass is becoming a great concern internationally (Guo et al., 2018). Thereby, processes of laccase-mediated detoxification have also been assessed concerning enzymatic saccharification owing to their ability to enhance the saccharification yield. Following this concept, Kalyani et al., (2012), showed that the laccases from C. perennis increase the saccharification output by up to 40% by decreasing the phenolic contents in pretreated biomass. Contrastingly, there are some phenolic laccase derivative complexes produced during the saccharification process that decrease the saccharification yield. Several studies highlighted a drop in the sugar concentration when laccases from C. rigida, P. cinnabarinus and T. villosa were used for the pretreatment of LCB, followed by enzymatic hydrolysis (Tabka et al., 2006; Jurado et al., 2009; Moreno et al., 2012, 2013a). De la Torre et al. (2017) also supported this concept by elucidating poor saccharification yield from pretreated LCB. Oliva-Taravilla et al. (2015b, 2016) observed that oxidation of phenolic laccase derivative compounds, i.e., syringaldehyde and vanillin, through laccases of Myceliophthora thermophile, led to the formation of the oligomeric product, which decreases the saccharification yield to 32.6% and 46.6%, respectively. In the same study, more than a 50% decrease in the activity of β-glucosidase and cellulase was observed due to the presence of vanillin. Subsequently, this increased competition between laccases and hydrolytic enzymes eventually lessened glucose recovery (Oliva-Taravilla et al., 2015a). Hence, to overcome this issue, the laccase mediator system has been developed as it is a proficient mean to upturn the saccharification yield (Giacobbe et al., 2018) by abating undefined enzymatic bindings, ultimately improving the efficacy of enzymatic hydrolysis (Heap et al., 2014).
5.3 Detoxification through microbial laccases and its effect on fermentation yield
Yeast, particularly S. cerevisiae is the most common microorganism used for bioethanol production besides estimating laccase detoxification effects. Several studies have demonstrated the enhanced output of bioethanol under laccase activity such as pretreated LCB when detoxified by laccases (produced from T. versicolor or C. rigida) favored more sugar consumption for S. cerevisiae growth which ultimately improved the bioethanol yield (Antošová and Sychrová, 2016). Similarly, laccases produced from P. cinnabarinus, when employed, induced high cell viability, reduced lag phase, and elevated the rates of bioethanol production (Moreno et al., 2013b; 2016a). Fang et al. (2015) also described the use of laccases extracted from Ganoderma lucidum for detoxifying pre-hydrolysate of steam-exploded LCB, which also resulted in improved yeast growth. Laccases from Cyathus stercoreus detoxified 77.5% phenolic compounds, and T. versicolor-derived laccases offered 80% detoxification of LCB with bioethanol yield of 0.374 and 0.43, respectively (Plácido and Capareda, 2015).
Research efforts demonstrated that fungal laccases, mainly derived from Aspergillus oryzae, also raise the fermentation efficiency to 6.8% (Li et al., 2014). Moreover, other fermenting yeasts also confer the same laccase detoxification effects as S. cerevisiae. Chandel et al. (2007) demonstrated that sugarcane bagasse prepared with laccases obtained from C. stercoreus fostered efficient working of Candida shehatae during acid hydrolysate fermentation. Furthermore, the presence of thermo-tolerant yeast elevates the yield and efficiency of fermentation besides saccharification processes, fortifying the concept that temperature is an imperative parameter that has a direct influence on fermentation. Kluyveromyces marxianus is a thermotolerant yeast and can withstand >40°C temperature (Abdel-Banat et al., 2010). Fermentation of wheat straw with Kluyveromyces marxianus, succeeded by steam explosion and laccase (from P. cinnabarinus) assisted detoxification provided ethanol yield similar to the one attained from S. cerevisiae (Moreno et al., 2013b). A higher concentration of bioethanol is also achieved by setting off fermentation and saccharification practices at high-substrate uniformities, in consort with laccase detoxification (Rosgaard et al., 2007). In the case of pretreated wheat straw with 12% (w/v) substrate loading, detoxification by laccases derived from P. cinnabarinus resulted in 16.7 g/L bioethanol concentration by K. marxianus during solid substrate fermentation. Contrastingly, in the same study, 22 g/L of bioethanol concentration was achieved by utilizing laccases obtained from P. cinnabarinus when substrate loading was about 20% (w/v). At this uniformity, the F12 strain of S. cerevisiae, which consumes developed xyloses remained incapable of propagating, and this inhibition was controlled by microbial laccases particularly purified from P. cinnabarinus (Moreno et al., 2013d). P. cinnabarinus-derived laccases were capable of generating 32 g/L bioethanol production from pretreated LCB even at 16% (w/v) substrate loading (Moreno et al., 2013c).
6 Laccase practices for delignification of pretreated material
Laccases mediated delignification is an efficacious process to escalate the valorization of LCB to bioethanol by employing a laccase-mediator system or immobilized laccases (Malhotra and Suman, 2021). During this process, aromatic lignin radicals are created by the oxidation of lignin compounds which successively induce lignin delignification by splitting the aromatic ring, accompanying carbon-carbon or ether bond degradation (Ramalingam et al., 2017). This procedure requires specific cultural conditions, which, in detail, are discussed in Table 2.
6.1 Delignification through laccases and bioethanol productivity
The accumulation and variety of inhibitors’ production hinge on the reaction conditions, biomass composition, and pretreatment methodologies (Bhatia et al., 2020; Agrawal et al., 2021). During biomass pretreatment, several phenolic inhibitors are generated from lignin. Laccases-mediated delignification assists in the removal of these inhibitory compounds for efficient production of bioethanol with increased yield, the mechanism of which is provided in Figure 6. The 1st step performed is the pretreatment which has increased the hydrolysis yield by successive hydrolysis step (2nd step) to form organic compounds that exhibit solubility in their nature (such as amino acids) by crashing down the insoluble organic compounds together with greater molecular weight compounds (i.e., lignin) in the presence of actinomycetes. In the 3rd phase, hydrolysis products are converted into alcohols besides hydrogen which leads the way to the production of biogas and methane in the 4th phase by conversion of carbon compounds, methanol and hydrogen by microbial action, particularly of methanococcus as well as methanobacterium genera (Bagewadi et al., 2017).
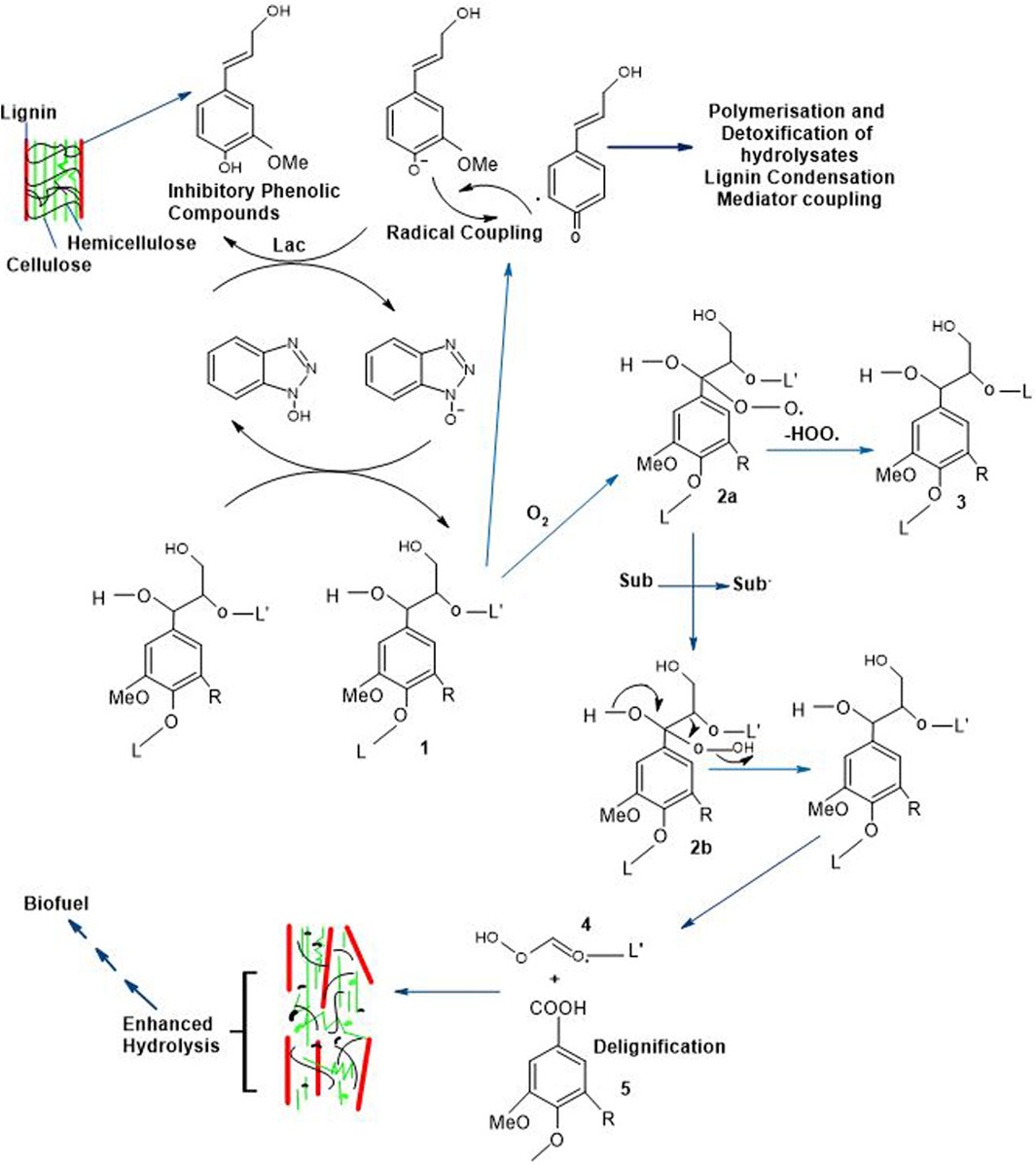
Figure 6. Delignification Strategies of Lignocellulosic Structure and Successive Tread in Bioethanol Production (R = H; Guaiacyl units, R = OMe; Syringyl units, L = Lignin, Sub = Substrate, Sub* = Radical and Lac = Laccases) (Du et al., 2013; Kudanga and Le Roes-Hill, 2014).
Numerous studies screen the delignification of pretreated biomass by laccases alone, prompting a boost in bioethanol production. Two such studies highlighted the attainment of 86% and 84%–89% delignification range by utilization of Pleurotus-derived laccases for Ricinus communis in addition to Lantana camara and Bambusa bambos materials, respectively, increasing the sugar yield up to 2.68 folds (Fillat et al., 2017; Kumar and Banerjee, 2018; Gujjala et al., 2019). It is also evidenced that supplementary sugar yield favors more bioethanol production both commercially and at a laboratory scale. Sitarz et al. (2013), stated that delignification of pretreated LCB from G. lucidum laccases dilated sugar yields by 75%, hence enhancing the bioethanol production. Rencoret et al. (2016) also claimed that laccases from P. cinnabarinus caused 18% delignification of LCB after extraction of alkaline peroxide, sooner or later increasing the sugar yield to 24%–25%. Another research reported that C. perennis-derived laccases upturn the saccharification yield to 48% by eliminating 76% of lignin-derived inhibitors (Bagewadi et al., 2017). (Rico et al. (2014) also demonstrated 50% delignification of pretreated biomass by laccases produced from M. thermophile, consuming methyl syringate as a mediator and conclusively improved the bioethanol productivity (Moreno et al., 2020). Meanwhile, Qiu and Chen, (2012) depicted that laccases attained from Sclerotium are liable to deteriorate the carbohydrate-lignin complex of LCB by amplifying glucose production.
Besides lignin removal, laccases also play an essential role in bioethanol production through the valorization of LCB by changing various properties of hydrolyses. Laccases are accountable for upgrading enzymatic hydrolysis and structural alteration of microfibers (Rocha-Martín et al., 2018). Amendments in porosity, hydrophobicity, and surface area of LCB by laccases aid in reducing the non-specific binding of hydrolytic enzymes; for instance, laccases from Trametes hirsuta raised the surface area and porosity of several alkali-extracted LCB, proliferating the saccharification yield and bioethanol production (Li et al., 2012). Despite all these positive aspects, a few drawbacks are also associated with using laccases alone for delignification of LCB, such as these enzymes can only oxidize phenolic lignin while non-phenolic lignin usually left untreated. However, to get over this problem, the laccase mediator system is widely used for executing appreciable delignification (Abdel-Hamid et al., 2013).
7 Laccase mediator system
The capability of laccases to batter and deteriorate lignin by collaborating with laccase mediators is considered a prodigious prospective for lignin valorization (Wang X. et al., 2018). Delignification with the laccase mediator system (LMS) causes non-phenolic and phenolic lignin degradation by the subsequent mediator and enzyme-catalyzed oxidative processes (Christopher et al., 2014). Delignification concerning LMS is a tranquil process in which small mediator particles get oxidized by laccases and then penetrate the dense structure of lignocelluloses, causing delignification of the sample. The efficiency of delignification through LMS is dependent on the combinations and practices of mediators and laccases; for instance, 1-hydroxybenzotriazole (HBT) with P. cinnabarinus laccases (PcL) and methyl syringate (MeS) with Myceliophthora thermophila laccases (MtL) are the most efficient LMS for delignification (Du et al., 2013).
7.1 Mechanism of laccase mediator system
The mechanism of LMS comprises oxidation of inhibitors particularly non-phenolic in nature by implementing three mechanisms such as electron transfer, ionic mechanism (Du et al., 2020) and radical hydrogen atom transfer (Figure 7). The indication for the existence of all these mechanisms is obtained by measuring the isotopic effects of intra-molecular kinetic and the pattern of degradation of non-phenolic lignin (Barreca et al., 2004).
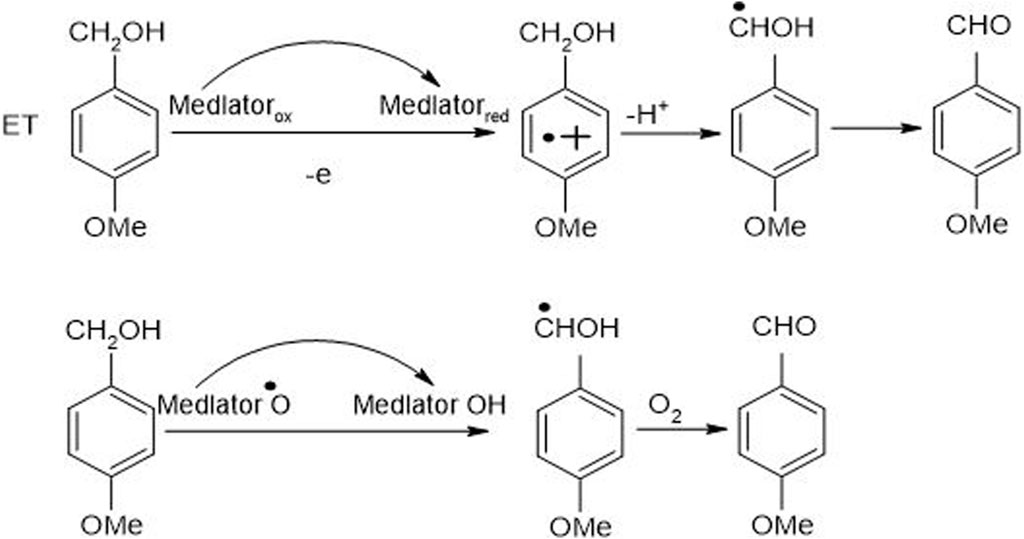
Figure 7. An anticipated mechanism for non-phenolic LMC (4-methoxybenzyl alcohol) oxidation, catalyzed by LMS, to 4-methoxy benzaldehyde along with electron and hydrogen atom transfer (Christopher et al., 2014).
Every mediator used in the laccase mediator system follows its exclusive oxidation mechanism. For instance, the presence of ABTS in LMS causes non-phenolic inhibitor oxidation through the electron transport route. On the contrary, laccase-TEMPO follows an ionic mechanism while laccase-HBT monitors a radical mechanism to oxidize inhibitors (Baiocco et al., 2003). The mechanism adopted by laccase-HBT is quite complex as a coupling intermediated product, i.e., β-aryl radical cation, as well as benzylic Cα radical, are formed during this course. It resulted in the formation of benzotriazole or some stable complexes having the ability to covalently bind some HBT to lignin. Laccase-HBT breaks down the Cα-Cß link during oxidation and results in the formation of carboxylic acid assemblies in treated LCB (Figure 8). Occasionally, oxidation mechanism also affects the molecular weight of lignin model compounds. Few studies highlighted that during the oxidation of non-phenolic and phenolic lignin model compounds, the presence of laccase-HBT, particularly from T. villosa caused a 4 to 5 times decrease in their molecular weight, respectively. Some emblematic products are also being generated from the degradation of lignin by using LMS, including 2,6-dimethoxy-4-((E)-prop-1-enyl)benzaldehyde, 2,6-dimethoxy-4-methylbenzaldehyde, and 4-ethyl-2,6-dimethoxybenzaldehyde (Christopher et al., 2014).
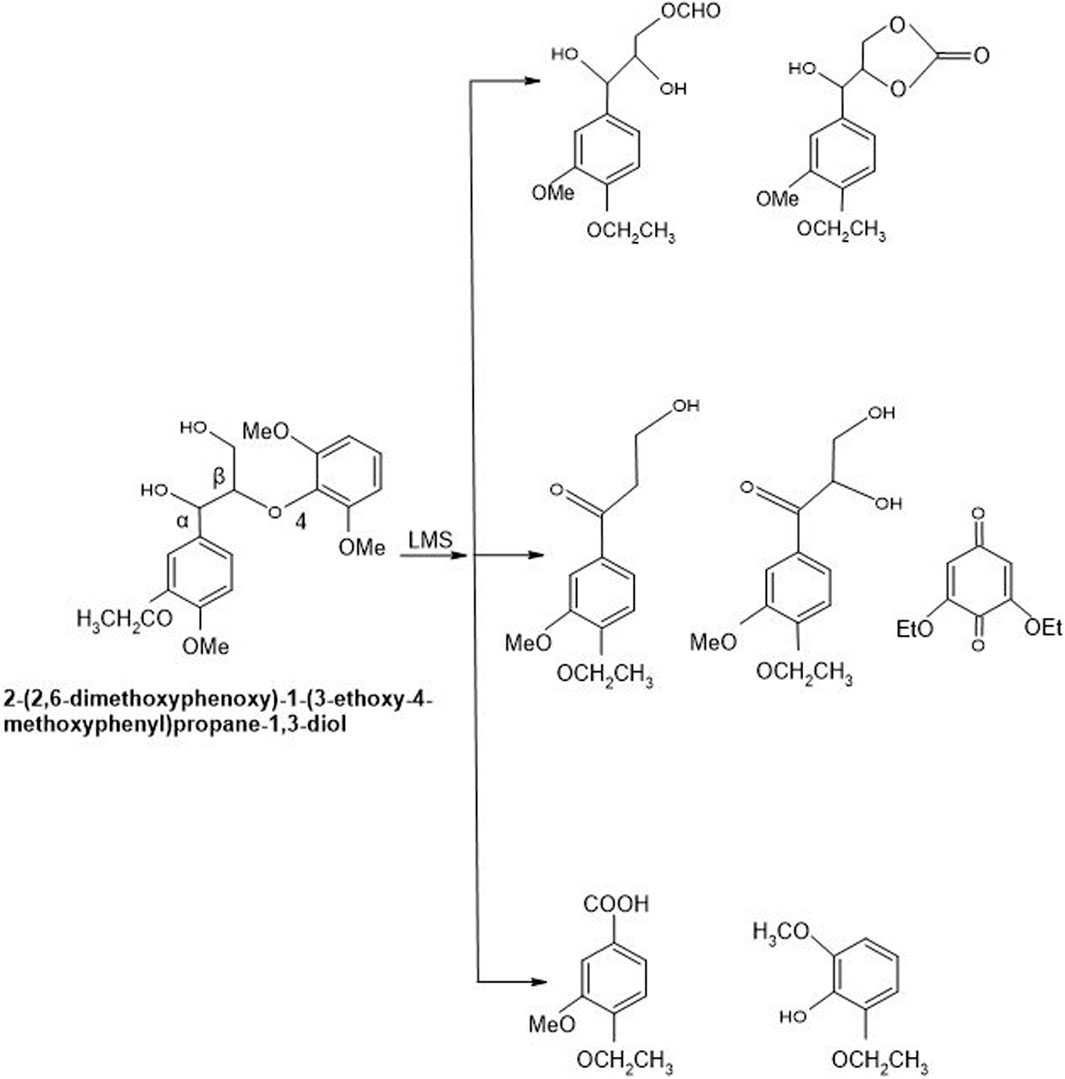
Figure 8. Non-phenolic compound (β-O-4 LMC) cleaved oxidatively by Laccase-HBT system of T. versicolor. By using laccase-HBT, the oxidation of β-O-4-linked non-phenolic lignin model compounds brought about Cα oxidation, aromatic ring-opening, and β-ether cleavage generation of carboxylic acids along with aromatic carbonyl compounds (Kawai et al., 2002).
7.2 LMS mediated delignification and enzymatic hydrolysis
Several research studies have demonstrated the efficiency of LMS in enhancing the processes of delignification (Table 3) and enzymatic hydrolysis such as Rico et al. (2014) studied that pretreatment using LMS led to the 50% delignification of LCB of Eucalyptus globulus wood by subsequent alkaline peroxide abstraction. Similarly, Zanirun et al. (2015) executed 8% delignification of oil palm LCB using HBT mediator in conjunction with Pycnoporus sanguineus laccases. The same study also reported 8.7% delignification of the sample when the ABTS mediator was being exercised, ultimately increasing fermentable sugar yield to 30 g/L.
Moilanen et al. (2014) demonstrated that pretreated spruce LCB when subjected to LMS treatment (laccases from T. hirsute with ABTS, TEMPO, and HBT mediators) brought out increased enzymatic hydrolysis, i.e., 49% in case of TEMPO and 54% with ABTS, while HBT mediator showed no effect. Another study stated 32% and 48% delignification of elephant grass and eucalypt with improved sugar yield of 12% and 61% respectively by treating them with laccases from T. villosa in combination with HBT mediator, followed by alkaline extraction (Gutiérrez et al., 2012). Furthermore, the use of LMS (P. cinnabarinus laccase with HBT mediator) also caused 37% delignification and 60% proliferation in sugar yield after enzymatic hydrolysis (Rencoret et al., 2016) by oxidizing lignin phenolic structures (Flórez Pardo et al., 2015).
Along with delignification, enzymatic hydrolysis can also be enhanced by LMS as one study reported 375.9 mg/g glucose yield as a result of enzymatic hydrolysis by performing LMS of pretreated wheat straw (Deng et al., 2019). Likewise, Moilanen et al. (2014) also reported a 19% increase in enzymatic hydrolysis due to LMS pretreatment of LCB. While, Heap and his colleagues evidenced that LMS of (laccases from T. versicolor with mediator HBT) pretreated LCB of wheat straw boosted 35% glucose yield, followed by the extraction of alkaline peroxide (Heap et al., 2014).
8 Laccases for detoxification and delignification in a lignocellulose-based bio refinery
Delignification and detoxification of lignocellulosic raw materials via laccases are definite, potent, cost-effective, energy-efficient, and eco-friendly processes besides increasing the fermentation and saccharification yields by diminishing the inhibitory compounds (Hashmi et al., 2016). Lesser inhibitory content in pretreated biomass leads to higher product yield in a short period by limiting the side reactions and implementing moderate conditions to proceed with the reaction (Moreno et al., 2015).
There is a huge need to explore new commercial laccases with the potential to detoxify and delignify raw materials at once via biorefinery. Moreno et al. (2016b) assessed MetZyme® to escalate the saccharification and ethanol fermentation of pretreated LCB, bringing about a 5% proliferation in sugar yield. The keystone of biorefinery impression is the maximal usage of bioresources in a tenable way, accompanied by a negligible impact on the environment (Moncada B et al., 2016). Biorefineries are responsible for the production of abundant and inexpensive fuel, along with the production of valuable compounds (Chowdhury et al., 2018). For this purpose, microbial laccases are significant as they support the outcomes and synthesis of new compounds from LCB. They also catalyze the reticulation of phenolic compounds in lignin-holding biomass and also avoid the usage of lethal synthetic adhesives (Shanmugam et al., 2020; Malhotra and Suman, 2021). Lacasses-mediated de-polymerization of lignin into numerous aromatic and phenolic composites is an auspicious methodology, and it has upgraded the status of laccases all across the globe.
9 Genetic engineering of fermenting microbes for laccase production
Remarkable advancements have been made to make delignification and detoxification processes more efficient. Genetic engineering is one of the most encouraging schemes as it genetically modifies fermenting microbes to produce laccases in compensation for traditional ways of manually adding laccases in pretreated biomass. For this purpose, several genes are also being overexpressed resulting in improved tolerance of microbes to inhibitory composites. Among microbes, yeast is the most proficient as it is easily manipulated, requires cost-effective culturing methods, and displays speedy growth along with swift and smooth genetic manipulation (Shukla, 2019; Aza et al., 2021). Therefore, many genetically engineered yeast strains are being developed these days (Antošová and Sychrová, 2016). Random mutagenesis along with heterologous or homologous genes is usually overexpressed to increase yeast forbearance to inhibitory complexes. Dehydrogenase plus reductase genes are widely overexpressed to enhance the detoxification capability of yeast for HMF and furfural inhibitors. In the past few years, different strains have been developed to make this process more efficient. A gene named ADH6p (NADPH-dependent alcohol dehydrogenase enzyme) was developed to improve the HMF as well as furfural reduction in yeast particularly in S. cerevisiae. Research highlighted that the overexpression of the ADH6p gene resulted in increased ethanol production during the fermentation process of spruce hydrolysate. Another study stated that upregulation of the ZWF1 gene from pentose phosphate shunt in yeast caused its shoot-up tolerance for furfuran byproducts. This tolerance is elucidated due to the pervasiveness of the pentose phosphate shunt alongside other pathways which increase the NADPH level intracellularly (Moreno et al., 2015).
The increased bioethanol production from raw materials is the prime purpose of genetic engineering these days. Larsson et al. (2001) genetically modified the strain of S. cerevisiae with laccases extracted from T. versicolor. The PKG1 promotor was used as a control to proliferate its resistance to phenolic inhibitors present in the LCB. The results showed that overexpression of homologous t-SNARE Sso2p can be used to raise the laccase activity twice. Genetic engineering favors detoxification, improved saccharification yield and ethanolic fermentation courses. Then contributes significantly to minimizing the procedure’s cost by eliminating the steps of detoxification and laccases production. Zhang et al. (2012) described the improvement in saccharification yield on account of modified Trichoderma reesei from the laccases of Trametes. Genetically engineered species also raise laccase activity, as Mate and his colleagues reported 34,000 folds increase in laccase activity by genetically engineering S. cerevisiae with laccases from basidiomycete (Mate et al., 2010; Antošová and Sychrová, 2016). These outcomes have corroborated the effectiveness of genetic engineering for proficient bioethanol production by altering the huge array of microorganisms.
10 Conclusion
Worldwide, nations have executed, characterized, or are running after the improvement of bio-economy techniques. The requirements for practical and manageable bio-based procedures support a huge section of the bioeconomy perspective. Laccase practices in biofuel production presently lean on the conception of lignocellulosic biomass degradation by laccases and the laccase mediator system. Both approaches are exceptionally efficient in the oxidative alteration of lignin besides increasing the hydrolysis yield from pretreated biomass. Nonetheless, enzyme production cost and expensive mediators (synthetic) are the contemporary challenges for practicing laccases in valorizing LCB, which can be resolved through microbial culture screening and genetic engineering. Several efforts are being instigated for immobilizing and engineering microbes for active laccases production, yet; the technique obliges more attention to achieve a superior future for biorefinery industries. There is also a huge need to uncover natural, eco-friendly, latest, and cheap mediators, which thrust the applications of these enzymes on the industrial scale, for enhanced biofuel production. Furthermore, a detailed study must be done on intracellular, yellow, white, thermostable, and alkaline laccases besides concentrating on the physiological and thermodynamic properties of laccases. Moreover, efforts should be carried out to devise cost-effective methods for the active production of the enzyme, which will aid in commercializing laccases all across the globe. Future research should focus on optimizing laccase production through genetic engineering for increasing yields and robustness. Additionally, exploring laccase synergy with other enzymes and developing efficient co-expression systems in microorganisms could lead to improved delignification and overall bioethanol conversion efficiency. This line of research has the potential to revolutionize bioethanol production by making it a more commercial and sustainable alternative fuel source.
Author contributions
IH: Conceptualization, Writing–original draft, Writing–review and editing. AS: Data curation, Investigation, Visualization, Writing–original draft. RC: Writing–original draft. AA: Validation, Visualization, Writing–review and editing. AN: Validation, Visualization, Writing–review and editing, Conceptualization, Formal Analysis, Supervision, Writing–original draft. CD: Data curation, Validation, Visualization, Writing–review and editing.
Funding
The author(s) declare that no financial support was received for the research, authorship, and/or publication of this article.
Conflict of interest
The authors declare that the research was conducted in the absence of any commercial or financial relationships that could be construed as a potential conflict of interest.
Publisher’s note
All claims expressed in this article are solely those of the authors and do not necessarily represent those of their affiliated organizations, or those of the publisher, the editors and the reviewers. Any product that may be evaluated in this article, or claim that may be made by its manufacturer, is not guaranteed or endorsed by the publisher.
References
Abdel-Banat, B. M. A., Hoshida, H., Ano, A., Nonklang, S., and Akada, R. (2010). High-temperature fermentation: how can processes for ethanol production at high temperatures become superior to the traditional process using mesophilic yeast?. Appl. Microbiol. Biotechnol. 85, 861–867. doi:10.1007/s00253-009-2248-5
Abdel-Hamid, A. M., Solbiati, J. O., and Cann, I. K. O. (2013). Insights into lignin degradation and its potential industrial applications. Amsterdam, Netherlands: Elsevier. doi:10.1016/B978-0-12-407679-2.00001-6
Abo, B. O., Gao, M., Wang, Y., Wu, C., Ma, H., and Wang, Q. (2019). Lignocellulosic biomass for bioethanol: an overview on pretreatment, hydrolysis and fermentation processes. Rev. Environ. Health 34, 57–68. doi:10.1515/reveh-2018-0054
Abo-State, M. A., Ragab, A. M. E., EL-Gendy, N. S., Farahat, L. A., and Madian, H. R. (2014). Bioethanol production from rice straw enzymatically saccharified by fungal isolates, Trichoderma viride F94 and Aspergillus terreus F98. Soft 3, 19–29. doi:10.4236/soft.2014.32003
Agrawal, K., Chaturvedi, V., and Verma, P. (2018). Fungal laccase discovered but yet undiscovered. Bioresour. Bioprocess. 5, 4. doi:10.1186/s40643-018-0190-z
Agrawal, R., Verma, A., Singhania, R. R., Varjani, S., Di Dong, C., and Kumar Patel, A. (2021). Current understanding of the inhibition factors and their mechanism of action for the lignocellulosic biomass hydrolysis. Bioresour. Technol. 332, 125042. doi:10.1016/j.biortech.2021.125042
Al-Zuhair, S., Ahmed, K., Abdulrazak, A., and El-Naas, M. H. (2013). Synergistic effect of pretreatment and hydrolysis enzymes on the production of fermentable sugars from date palm lignocellulosic waste. J. Ind. Eng. Chem. 19, 413–415. doi:10.1016/j.jiec.2012.09.022
Amit, K., Nakachew, M., Yilkal, B., and Mukesh, Y. (2018). A review of factors affecting enzymatic hydrolysis of pretreated lignocellulosic Biomass. Res. J. Chem. Environ. 22, 62–67.
Antošová, Z., and Sychrová, H. (2016). Yeast hosts for the production of recombinant laccases: a review. Mol. Biotechnol. 58, 93–116. doi:10.1007/s12033-015-9910-1
Arias, M. E., Arenas, M., Rodríguez, J., Soliveri, J., Ball, A. S., and Hernández, M. (2003). Kraft pulp biobleaching and mediated oxidation of a nonphenolic substrate by laccase from Streptomyces cyaneus CECT 3335. Appl. Environ. Microbiol. 69, 1953–1958. doi:10.1128/AEM.69.4.1953-1958.2003
Axelsson, L., Franzén, M., Ostwald, M., Berndes, G., Lakshmi, G., and Ravindranath, N. H. (2012). Jatropha cultivation in southern India: assessing farmers’ experiences. Biofuels, Bioprod. Biorefining 6, 246–256. doi:10.1002/bbb.1324
Aza, P., De Salas, F., Molpeceres, G., Rodríguez-Escribano, D., De La Fuente, I., and Camarero, S. (2021). Protein engineering approaches to enhance fungal laccase production in S. cerevisiae. Int. J. Mol. Sci. 22, 1157–1219. doi:10.3390/ijms22031157
Babiker, M. A. A.-B., and Hamadttu, A. F. E.-S. (2019). Expression profiling, phylogenetic, and structural analyses of a laccase gene from the red palm weevil, Rhynchophorus ferrugineus. Afr. J. Biotechnol. 18, 978–990. doi:10.5897/ajb2018.16860
Bagewadi, Z. K., Mulla, S. I., and Ninnekar, H. Z. (2017). Optimization of laccase production and its application in delignification of biomass. Int. J. Recycl. Org. Waste Agric. 6, 351–365. doi:10.1007/s40093-017-0184-4
Baiocco, P., Barreca, A. M., Fabbrini, M., Galli, C., and Gentili, P. (2003). Promoting laccase activity towards non-phenolic substrates: a mechanistic investigation with some laccase-mediator systems. Org. Biomol. Chem. 1, 191–197. doi:10.1039/b208951c
Barreca, A. M., Sjögren, B., Fabbrini, M., Galli, C., and Gentili, P. (2004). Catalytic efficiency of some mediators in laccase-catalyzed alcohol oxidation. Biocatal. Biotransformation 22, 105–112. doi:10.1080/10242420410001692750
Bayrakci Ozdingis, A. G., and Kocar, G. (2018). Current and future aspects of bioethanol production and utilization in Turkey. Renew. Sustain. Energy Rev. 81, 2196–2203. doi:10.1016/j.rser.2017.06.031
Berlin, A., Balakshin, M., Gilkes, N., Kadla, J., Maximenko, V., Kubo, S., et al. (2006). Inhibition of cellulase, xylanase and β-glucosidase activities by softwood lignin preparations. J. Biotechnol. 125, 198–209. doi:10.1016/j.jbiotec.2006.02.021
Bertrand, B., Martínez-Morales, F., and Trejo-Hernández, M. R. (2013). Fungal laccases: induction and production. Rev. Mex. Ing. Quim 12, 473–488.
Bhatia, S. K., Jagtap, S. S., Bedekar, A. A., Bhatia, R. K., Patel, A. K., Pant, D., et al. (2020). Recent developments in pretreatment technologies on lignocellulosic biomass: effect of key parameters, technological improvements, and challenges. Bioresour. Technol. 300, 122724. doi:10.1016/j.biortech.2019.122724
Binod, P., Janu, K. U., Sindhu, R., and Pandey, A. (2011). “Hydrolysis of lignocellulosic biomass for bioethanol production,” in Biofuels. Amsterdam: Elsevier Inc. doi:10.1016/B978-0-12-385099-7.00010-3
Bishwakarma, S., Maharjan, S., Shrestha, M., and Bhattarai, T. (2018). Metabolic engineering of Saccharomyces cerevisiae for consolidate bioprocessing of lignocellulosic biomass for bio-ethanol production. The Annual Biotech Magazine 2, 66–71.
Camarero, S., Cañas, A. I., Nousiainen, P., Record, E., Lomascolo, A., Martínez, M. J., et al. (2008). P-hydroxycinnamic acids as natural mediators for laccase oxidation of recalcitrant compounds. Environ. Sci. Technol. 42, 6703–6709. doi:10.1021/es8008979
Camarero, S., Ibarra, D., Martínez, M. J., and Martínez, Á. T. (2005). Lignin-derived compounds as efficient laccase mediators for decolorization of different types of recalcitrant dyes. Appl. Environ. Microbiol. 71, 1775–1784. doi:10.1128/AEM.71.4.1775-1784.2005
Chakroun, H., Mechichi, T., Martinez, M. J., Dhouib, A., and Sayadi, S. (2010). Purification and characterization of a novel laccase from the ascomycete Trichoderma atroviride: application on bioremediation of phenolic compounds. Process Biochem. 45, 507–513. doi:10.1016/j.procbio.2009.11.009
Chami Khazraji, A., and Robert, S. (2013). Interaction effects between cellulose and water in nanocrystalline and amorphous regions: a novel approach using molecular modeling. J. Nanomater. 2013, 1–10. doi:10.1155/2013/409676
Chandel, A. K., Kapoor, R. K., Singh, A., and Kuhad, R. C. (2007). Detoxification of sugarcane bagasse hydrolysate improves ethanol production by Candida shehatae NCIM 3501. Bioresour. Technol. 98, 1947–1950. doi:10.1016/j.biortech.2006.07.047
Chantarasiri, A., Boontanom, P., and Nuiplot, N. O. (2017). Isolation and characterization of Lysinibacillus sphaericus BR2308 from coastal wetland in Thailand for the biodegradation of lignin. AACL Bioflux 10, 200–209.
Chauhan, P. S., Goradia, B., and Saxena, A. (2017). Bacterial laccase: recent update on production, properties and industrial applications. 3 Biotech. 7, 323–420. doi:10.1007/s13205-017-0955-7
Chen, H. Z., and Liu, Z. H. (2015). Steam explosion and its combinatorial pretreatment refining technology of plant biomass to bio-based products. Biotechnol. J. 10, 866–885. doi:10.1002/biot.201400705
Chen, H. Z., and Liu, Z. H. (2017). Enzymatic hydrolysis of lignocellulosic biomass from low to high solids loading. Eng. Life Sci. 17, 489–499. doi:10.1002/elsc.201600102
Chiaramonti, D., Prussi, M., Ferrero, S., Oriani, L., Ottonello, P., Torre, P., et al. (2012). Review of pretreatment processes for lignocellulosic ethanol production, and development of an innovative method. Biomass Bioenergy 46, 25–35. doi:10.1016/j.biombioe.2012.04.020
Choo, M. Y., Oi, L. E., Ling, T. C., Ng, E. P., Lee, H. V., and Juan, J. C. (2019). Conversion of microalgae biomass to biofuels. Amsterdam, Netherlands: Elsevier Inc. doi:10.1016/B978-0-12-817536-1.00010-2
Chowdhury, R., Ghosh, S., Debnath, B., and Manna, D. (2018). Indian agro-wastes for 2G biorefineries: strategic decision on conversion processes. Green Energy Technol. 0, 353–373. doi:10.1007/978-981-10-7188-1_16
Christopher, L. P., Yao, B., and Ji, Y. (2014). Lignin biodegradation with laccase-mediator systems. Front. Energy Res. 2, 1–13. doi:10.3389/fenrg.2014.00012
Dana, M., Khaniki, G. B., Mokhtarieh, A. A., and Davarpanah, S. J. (2017). Biotechnological and industrial applications of laccase: a review. J. Appl. Biotechnol. Rep. 4, 675–679.
de Abreu Domingos, R., and da Fonseca, F. V. (2018). Evaluation of adsorbent and ion exchange resins for removal of organic matter from petroleum refinery wastewaters aiming to increase water reuse. J. Environ. Manage. 214, 362–369. doi:10.1016/j.jenvman.2018.03.022
de Freitas, E. N., Bubna, G. A., Brugnari, T., Kato, C. G., Nolli, M., Rauen, T. G., et al. (2017). Removal of bisphenol A by laccases from Pleurotus ostreatus and Pleurotus pulmonarius and evaluation of ecotoxicity of degradation products. Chem. Eng. J. 330, 1361–1369. doi:10.1016/j.cej.2017.08.051
de la Torre, I., Ravelo, M., Segarra, S., Tortajada, M., Santos, V. E., and Ladero, M. (2017). Study on the effects of several operational variables on the enzymatic batch saccharification of orange solid waste. Bioresour. Technol. 245, 906–915. doi:10.1016/j.biortech.2017.08.094
De La Torre, M., Martín-Sampedro, R., Fillat, Ú., Eugenio, M. E., Blánquez, A., Hernández, M., et al. (2017). Comparison of the efficiency of bacterial and fungal laccases in delignification and detoxification of steam-pretreated lignocellulosic biomass for bioethanol production. J. Ind. Microbiol. Biotechnol. 44, 1561–1573. doi:10.1007/s10295-017-1977-1
Deng, Z., Xia, A., Liao, Q., Zhu, X., Huang, Y., and Fu, Q. (2019). Laccase pretreatment of wheat straw: effects of the physicochemical characteristics and the kinetics of enzymatic hydrolysis. Biotechnol. Biofuels 12, 159–212. doi:10.1186/s13068-019-1499-3
Dias, A. A., Freitas, G. S., Marques, G. S. M., Sampaio, A., Fraga, I. S., Rodrigues, M. A. M., et al. (2010). Enzymatic saccharification of biologically pre-treated wheat straw with white-rot fungi. Bioresour. Technol. 101, 6045–6050. doi:10.1016/j.biortech.2010.02.110
Du, X., Li, J., Gellerstedt, G., Rencoret, J., Del Río, J. C., Martínez, A. T., et al. (2013). Understanding pulp delignification by laccase-mediator systems through isolation and characterization of lignin-carbohydrate complexes. Biomacromolecules 14, 3073–3080. doi:10.1021/bm4006936
Du, Y., Ma, H., Huang, L., Pan, Y., Huang, J., and Liu, Y. (2020). Electrochemical characteristics of the decolorization of three dyes by laccase mediator system (LMS) with synthetic and natural mediators. Chemosphere 239, 124779. doi:10.1016/j.chemosphere.2019.124779
E4tech, Re-Cord, and Wur (2015). From the Sugar Platform to biofuels and biochemicals. Final Rep. Eur. Comm. Dir. Energy 183. Available at https://ec.europa.eu/energy/sites/ener/files/documents/ECSugarPlatformfinalreport.pdf.
Emad, A. (2013) “Biofuel: sources, extraction and determination,” in Liquid, gaseous and solid biofuels - conversion techniques. Norderstedt, Germany: bod–books on demand. doi:10.5772/51943
Espirito Santo, M., Rezende, C. A., Bernardinelli, O. D., Pereira, N., Curvelo, A. A. S., deAzevedo, E. R., et al. (2018). Structural and compositional changes in sugarcane bagasse subjected to hydrothermal and organosolv pretreatments and their impacts on enzymatic hydrolysis. Ind. Crops Prod. 113, 64–74. doi:10.1016/j.indcrop.2018.01.014
Fang, Z., Liu, X., Chen, L., Shen, Y., Zhang, X., Fang, W., et al. (2015). Identification of a laccase Glac15 from Ganoderma lucidum 77002 and its application in bioethanol production David Wilson. Biotechnol. Biofuels 8, 2–13. doi:10.1186/s13068-015-0235-x
Farmer, T. J., Comerford, J. W., Pellis, A., and Robert, T. (2018). Post-polymerization modification of bio-based polymers: maximizing the high functionality of polymers derived from biomass. Polym. Int. 67, 775–789. doi:10.1002/pi.5573
Fillat, Ú., Ibarra, D., Eugenio, M. E., Moreno, A. D., Tomás-Pejó, E., and Martín-Sampedro, R. (2017). Laccases as a potential tool for the efficient conversion of lignocellulosic biomass: a review. Fermentation 3, 17. doi:10.3390/fermentation3020017
Financie, R., Moniruzzaman, M., and Uemura, Y. (2016). Enhanced enzymatic delignification of oil palm biomass with ionic liquid pretreatment. Biochem. Eng. J. 110, 1–7. doi:10.1016/j.bej.2016.02.008
Flórez Pardo, L. M., Parra Paz, A. S., López Galán, J. E., and Figueroa Oviedo, J. I. (2015). Using a mediator system to increase the delignification of sugarcane residues with fungal enzymes. CT&F - Cienc. Tecnol. Futur. 6, 81–91. doi:10.29047/01225383.22
Galai, S., Limam, F., and Marzouki, M. N. (2009). A new stenotrophomonas maltophilia strain producing laccase. use in decolorization of synthetics dyes. Appl. Biochem. Biotechnol. 158, 416–431. doi:10.1007/s12010-008-8369-y
Ghobadi Nejad, Z., Borghei, S. M., and Yaghmaei, S. (2019). Biodegradation of synthetic dye using partially purified and characterized laccase and its proposed mechanism. Int. J. Environ. Sci. Technol. 16, 7805–7816. doi:10.1007/s13762-019-02226-5
Giacobbe, S., Pezzella, C., Lettera, V., Sannia, G., and Piscitelli, A. (2018). Laccase pretreatment for agrofood wastes valorization. Bioresour. Technol. 265, 59–65. doi:10.1016/j.biortech.2018.05.108
Glazunova, O. A., Polyakov, K. M., Moiseenko, K. V., Kurzeev, S. A., and Fedorova, T. V. (2018). Structure-function study of two new middle-redox potential laccases from basidiomycetes Antrodiella faginea and Steccherinum murashkinskyi. Int. J. Biol. Macromol. 118, 406–418. doi:10.1016/j.ijbiomac.2018.06.038
Graham, L. A., Belisle, S. L., and Baas, C.-L. (2008). Emissions from light duty gasoline vehicles operating on low blend ethanol gasoline and E85. Atmos. Environ. 42, 4498–4516. doi:10.1016/j.atmosenv.2008.01.061
Guimarães, L. R. C., Woiciechowski, A. L., Karp, S. G., Coral, J. D., Zandoná Filho, A., and Soccol, C. R. (2016). Laccases. Curr. Dev. Biotechnol. Bioeng. Prod. Isol. Purif. Ind. Prod., 199–216. doi:10.1016/B978-0-444-63662-1.00009-9
Gujjala, L. K. S., Bandyopadhyay, T. K., and Banerjee, R. (2019). Production of biodiesel utilizing laccase pretreated lignocellulosic waste liquor: an attempt towards cleaner production process. Energy Convers. Manag. 196, 979–987. doi:10.1016/j.enconman.2019.06.036
Guo, H., Chang, Y., and Lee, D. J. (2018). Enzymatic saccharification of lignocellulosic biorefinery: research focuses. Bioresour. Technol. 252, 198–215. doi:10.1016/j.biortech.2017.12.062
Guo, X., Cavka, A., Jönsson, L. J., and Hong, F. (2013). Comparison of methods for detoxification of spruce hydrolysate for bacterial cellulose production. Microb. Cell Fact. 12, 93–14. doi:10.1186/1475-2859-12-93
Gupta, R., Mehta, G., Khasa, Y. P., and Kuhad, R. C. (2011). Fungal delignification of lignocellulosic biomass improves the saccharification of cellulosics. Biodegradation 22, 797–804. doi:10.1007/s10532-010-9404-6
Gutiérrez, A., Rencoret, J., Cadena, E. M., Rico, A., Barth, D., del Río, J. C., et al. (2012). Demonstration of laccase-based removal of lignin from wood and non-wood plant feedstocks. Bioresour. Technol. 119, 114–122. doi:10.1016/j.biortech.2012.05.112
Hamelinck, C. N., Van Hooijdonk, G., and Faaij, A. P. C. (2005). Ethanol from lignocellulosic biomass: techno-economic performance in short-middle- and long-term. Biomass Bioenergy 28, 384–410. doi:10.1016/j.biombioe.2004.09.002
Han, J., Elgowainy, A., Wang, M., and Divita, V. (2015). Well-to-wheels greenhouse gas emissions analysis of high-octane fuels with various market shares and ethanol blending levels. Argonne, IL, USA: Argonne National Lab.
Hashmi, M., Ragauskas, A. J., and Chandra, R. P. (2016). Lignin valorization via laccase-assisted functionalization BAOJ chemistry. Issue BAOJ Chem. 2.
Hassan, S. S., Williams, G. A., and Jaiswal, A. K. (2018). Emerging technologies for the pretreatment of lignocellulosic biomass. Bioresour. Technol. 262, 310–318. doi:10.1016/j.biortech.2018.04.099
Heap, L. (2014). Investigating the role of laccase and laccase mediator systems to improve the saccharification of biomass for bioethanol production. Manchester, UK: University of Manchester. Ph.D.
Heap, L., Green, A., Brown, D., Van Dongen, B., and Turner, N. (2014). Role of laccase as an enzymatic pretreatment method to improve lignocellulosic saccharification. Catal. Sci. Technol. 4, 2251–2259. doi:10.1039/c4cy00046c
Henske, J. K., Springer, S. D., O’Malley, M. A., and Butler, A. (2018). Substrate-based differential expression analysis reveals control of biomass degrading enzymes in Pycnoporus cinnabarinus. Biochem. Eng. J. 130, 83–89. doi:10.1016/j.bej.2017.11.015
Hilgers, R., Vincken, J. P., Gruppen, H., and Kabel, M. A. (2018). Laccase/mediator systems: their reactivity toward phenolic lignin structures. ACS Sustain. Chem. Eng. 6, 2037–2046. doi:10.1021/acssuschemeng.7b03451
Irfan, M., Mehmood, S., Irshad, M., and Anwar, Z. (2018). Optimized production, purification and molecular characterization of fungal laccase through Alternaria alternata. Turk. J. Biochem. 43, 613–622. doi:10.1515/tjb-2017-0239
Janusz, G., Pawlik, A., Świderska-Burek, U., Polak, J., Sulej, J., Jarosz-Wilkołazka, A., et al. (2020). Laccase properties, physiological functions, and evolution. Int. J. Mol. Sci. 21, 966. doi:10.3390/ijms21030966
Jasim, A. (2017). Medicinal properties of laccase from Basidiomycetes mushroom: a review. Adv. Life Sci. Technol. 54, 99–109.
Ji, H., Song, Y., Zhang, X., and Tan, T. (2017). Using a combined hydrolysis factor to balance enzymatic saccharification and the structural characteristics of lignin during pretreatment of Hybrid poplar with a fully recyclable solid acid. Bioresour. Technol. 238, 575–581. doi:10.1016/j.biortech.2017.04.092
Jimenez-Juarez, N., Roman-Miranda, R., Baeza, A., Sánchez-Amat, A., Vazquez-Duhalt, R., and Valderrama, B. (2005). Alkali and halide-resistant catalysis by the multipotent oxidase from Marinomonas mediterranea. J. Biotechnol. 117, 73–82. doi:10.1016/j.jbiotec.2005.01.002
Jönsson, L. J., and Martín, C. (2016). Pretreatment of lignocellulose: formation of inhibitory by-products and strategies for minimizing their effects. Bioresour. Technol. 199, 103–112. doi:10.1016/j.biortech.2015.10.009
Jurado, M., Prieto, A., Martínez-Alcalá, Á., Martínez, Á. T., and Martínez, M. J. (2009). Laccase detoxification of steam-exploded wheat straw for second generation bioethanol. Bioresour. Technol. 100, 6378–6384. doi:10.1016/j.biortech.2009.07.049
Kalyani, D., Dhiman, S. S., Kim, H., Jeya, M., Kim, I. W., and Lee, J. K. (2012). Characterization of a novel laccase from the isolated Coltricia perennis and its application to detoxification of biomass. Process Biochem. 47, 671–678. doi:10.1016/j.procbio.2012.01.013
Kang, C. W., Kolya, H., Jang, E. S., Zhu, S., and Choi, B. S. (2021). Steam exploded wood cell walls reveals improved gas permeability and sound absorption capability. Appl. Acoust. 179, 108049. doi:10.1016/j.apacoust.2021.108049
Kaparaju, P., and Felby, C. (2010). Characterization of lignin during oxidative and hydrothermal pre-treatment processes of wheat straw and corn stover. Bioresour. Technol. 101, 3175–3181. doi:10.1016/j.biortech.2009.12.008
Karp, S. G., Faraco, V., Amore, A., Letti, L. A. J., Thomaz Soccol, V., and Soccol, C. R. (2015). Statistical optimization of laccase production and delignification of sugarcane bagasse by Pleurotus ostreatus in solid-state fermentation. Biomed. Res. Int. 2015, 1–8. doi:10.1155/2015/181204
Karp, S. G., Woiciechowski, A. L., Soccol, V. T., and Soccol, C. R. (2013). Pretreatment strategies for delignification of sugarcane bagasse: a Review. Braz. Arch. Biol. Technol. 56, 679–689. doi:10.1590/S1516-89132013000400019
Kawai, S., Nakagawa, M., and Ohashi, H. (2002). Degradation mechanisms of a nonphenolic β- O -4 lignin model dimer by Trametes versicolor laccase in the presence of 1-hydroxybenzotriazole. Enzyme Microb. Technol. 30, 482–489. doi:10.1016/S0141-0229(01)00523-3
Kim, D. (2018). Physico-chemical conversion of lignocellulose: inhibitor effects and detoxification strategies: a mini review. Molecules 23, 309. doi:10.3390/molecules23020309
Kim, S. (2021). Mushroom ligninolytic enzymes―features and application of potential enzymes for conversion of lignin into bio-based chemicals and materials. Appl. Sci. 11, 6161. doi:10.3390/app11136161
Kolb, M., Sieber, V., Amann, M., Faulstich, M., and Schieder, D. (2012). Removal of monomer delignification products by laccase from Trametes versicolor. Bioresour. Technol. 104, 298–304. doi:10.1016/j.biortech.2011.11.080
Kudanga, T., and Le Roes-Hill, M. (2014). Laccase applications in biofuels production: current status and future prospects. Appl. Microbiol. Biotechnol. 98, 6525–6542. doi:10.1007/s00253-014-5810-8
Kudanga, T., Nyanhongo, G. S., Guebitz, G. M., and Burton, S. (2011). Potential applications of laccase-mediated coupling and grafting reactions: a review. Enzyme Microb. Technol. 48, 195–208. doi:10.1016/j.enzmictec.2010.11.007
Kumar, S., and Banerjee, R. (2018). Enzymatic delignification and saccharification of Bambusa bambos for biobutanol production. Ind. Crops Prod. 125, 386–394. doi:10.1016/j.indcrop.2018.09.015
Kumari, D., and Singh, R. (2018). Pretreatment of lignocellulosic wastes for biofuel production: a critical review. Renew. Sustain. Energy Rev. 90, 877–891. doi:10.1016/j.rser.2018.03.111
Larsson, S., Cassland, P., and Jönsson, L. J. (2001). Development of a Saccharomyces cerevisiae strain with enhanced resistance to phenolic fermentation inhibitors in lignocellulose hydrolysates by heterologous expression of laccase. Appl. Environ. Microbiol. 67, 1163–1170. doi:10.1128/AEM.67.3.1163-1170.2001
Lecksiwilai, N., and Gheewala, S. H. (2020). Life cycle assessment of biofuels in Thailand: implications of environmental trade-offs for policy decisions. Sustain. Prod. Consum. 22, 177–185. doi:10.1016/j.spc.2020.03.004
Lee, S., Kang, M., Bae, J. H., Sohn, J. H., and Sung, B. H. (2019). Bacterial valorization of lignin: strains, enzymes, conversion pathways, biosensors, and perspectives. Front. Bioeng. Biotechnol. 7, 209–218. doi:10.3389/fbioe.2019.00209
Li, J., Lin, J., Zhou, P., Wu, K., Liu, H., Xiong, C., et al. (2014). One-pot simultaneous saccharification and fermentation: a preliminary study of a novel configuration for cellulosic ethanol production. Bioresour. Technol. 161, 171–178. doi:10.1016/j.biortech.2014.02.130
Li, J., Sun, F., Li, X., Yan, Z., Yuan, Y., and Liu, X. (2012). Enhanced saccharification of corn straw pretreated by alkali combining crude ligninolytic enzymes. J. Chem. Technol. Biotechnol. 87, 1687–1693. doi:10.1002/jctb.3818
Lin, C. Y., and Lu, C. (2021). Development perspectives of promising lignocellulose feedstocks for production of advanced generation biofuels: a review. Renew. Sustain. Energy Rev. 136, 110445. doi:10.1016/j.rser.2020.110445
Linares, N. C., and Loske, A. M. (2018). Enhanced delignification of lignocellulosic biomass by recombinant fungus phanerochaete chrysosporium overexpressing laccases and peroxidases. J Mol Microbiol Biotechnol 28 (1), 1–13. doi:10.1159/000485976
Liu, Z. H., and Chen, H. Z. (2016). Simultaneous saccharification and co-fermentation for improving the xylose utilization of steam exploded corn stover at high solid loading. Bioresour. Technol. 201, 15–26. doi:10.1016/j.biortech.2015.11.023
Lu, C., Wang, H., Luo, Y., and Guo, L. (2010). An efficient system for pre-delignification of gramineous biofuel feedstock in vitro: application of a laccase from Pycnoporus sanguineus H275. Process Biochem. 45, 1141–1147. doi:10.1016/j.procbio.2010.04.010
Maia, L. F., Hoyos, S. L. M., Watanabe, A. K., Moralez, A. T. P., Furlaneto, M. C., and Daniel, J. F. de S. (2021). Extra and intracellular laccase titers of xylophagic bacteria isolated from adult termite. Acta Sci. Technol. 43–e51805. doi:10.4025/actascitechnol.v43i1.51805
Mendonc, S., Jos, A., Dillon, P., and Camassola, M. (2018). PT Graphical abstract SC. Process Biochem. doi:10.1016/j.procbio.2018.03.005
Majolagbe, O., Oloke, J., Deka-Boruah, H., Adetunji, C., Bordoloi, A., and Borah, M. (2020). Extraction and purification of extracellular laccase from wild, mutants and hybrid strains of two white-rot fungus and its applications in decolourization and ligninolysis. J. Microbiol. Biotechnol. Food Sci. 2020, 998–1016.
Malhotra, M., and Suman, S. K. (2021). Laccase-mediated delignification and detoxification of lignocellulosic biomass: removing obstacles in energy generation. Environ. Sci. Pollut. Res. 28, 58929–58944. doi:10.1007/s11356-021-13283-0
Mandic, M., Djokic, L., Nikolaivits, E., Prodanovic, R., O’connor, K., Jeremic, S., et al. (2019). Identification and characterization of new laccase biocatalysts from Pseudomonas species suitable for degradation of synthetic textile dyes. Catalysts 9, 629. doi:10.3390/catal9070629
Martínez, Á. T., Ruiz-Dueñas, F. J., Martínez, M. J., del Río, J. C., and Gutiérrez, A. (2009). Enzymatic delignification of plant cell wall: from nature to mill. Curr. Opin. Biotechnol. 20, 348–357. doi:10.1016/j.copbio.2009.05.002
Martín-Sampedro, R., Eugenio, M. E., García, J. C., Lopez, F., Villar, J. C., and Diaz, M. J. (2012). Steam explosion and enzymatic pre-treatments as an approach to improve the enzymatic hydrolysis of Eucalyptus globulus. Biomass Bioenergy 42, 97–106. doi:10.1016/j.biombioe.2012.03.032
Mate, D., García-Burgos, C., García-Ruiz, E., Ballesteros, A. O., Camarero, S., and Alcalde, M. (2010). Laboratory evolution of high-redox potential laccases. Chem. Biol. 17, 1030–1041. doi:10.1016/j.chembiol.2010.07.010
Mate, D. M., and Alcalde, M. (2017). Laccase: a multi-purpose biocatalyst at the forefront of biotechnology. Microb. Biotechnol. 10, 1457–1467. doi:10.1111/1751-7915.12422
Maulana Hidayatullah, I., Setiadi, T., Tri Ari Penia Kresnowati, M., and Boopathy, R. (2020). Xylanase inhibition by the derivatives of lignocellulosic material. Bioresour. Technol. 300–122740. doi:10.1016/j.biortech.2020.122740
Mishra, A., and Ghosh, S. (2016). “A perspective on current technologies used for bioethanol production from lignocellulosics,” in Advances in Biofeedstocks and Biofuels: Production Technologies for Biofuels, II (Hoboken, New Jersey: John Wiley and Sons). doi:10.1002/9781119117551.ch2
Mishra, S. K., Kumar Srivastava, S., Prakash, V., Lall, A. M., and ma, S. (2017). Production and optimization of laccase from Streptomyces lavendulae. Int. J. Curr. Microbiol. Appl. Sci. 6, 1239–1246. doi:10.20546/ijcmas.2017.605.134
Moilanen, U., Kellock, M., Galkin, S., and Viikari, L. (2011). The laccase-catalyzed modification of lignin for enzymatic hydrolysis. Enzyme Microb. Technol. 49, 492–498. doi:10.1016/j.enzmictec.2011.09.012
Moilanen, U., Kellock, M., Várnai, A., Andberg, M., and Viikari, L. (2014). Mechanisms of laccase-mediator treatments improving the enzymatic hydrolysis of pre-treated spruce. Biotechnol. Biofuels 7, 177–213. doi:10.1186/s13068-014-0177-8
Moncada, B. J., Aristizábal, M. V., and Cardona, A. C. A. (2016). Design strategies for sustainable biorefineries. Biochem. Eng. J. 116, 122–134. doi:10.1016/j.bej.2016.06.009
Moreno, A. D., Alvira, P., Ibarra, D., and Tomás-Pejó, E. (2017). Production of ethanol from lignocellulosic biomass. Production of platform chemicals from sustainable resources, 375–410. doi:10.1007/978-981-10-4172-3_12
Moreno, A. D., Ibarra, D., Alvira, P., Tomás-Pejó, E., and Ballesteros, M. (2015). A review of biological delignification and detoxification methods for lignocellulosic bioethanol production. Crit. Rev. Biotechnol. 35, 342–354. doi:10.3109/07388551.2013.878896
Moreno, A. D., Ibarra, D., Alvira, P., Tomás-Pejó, E., and Ballesteros, M. (2016a). Exploring laccase and mediators behavior during saccharification and fermentation of steam-exploded wheat straw for bioethanol production. J. Chem. Technol. Biotechnol. 91, 1816–1825. doi:10.1002/jctb.4774
Moreno, A. D., Ibarra, D., Ballesteros, I., Fernández, J. L., and Ballesteros, M. (2013a). Ethanol from laccase-detoxified lignocellulose by the thermotolerant yeast Kluyveromyces marxianus—effects of steam pretreatment conditions, process configurations and substrate loadings. Biochem. Eng. J. 79, 94–103. doi:10.1016/j.bej.2013.07.006
Moreno, A. D., Ibarra, D., Ballesteros, I., González, A., and Ballesteros, M. (2013b). Comparing cell viability and ethanol fermentation of the thermotolerant yeast Kluyveromyces marxianus and Saccharomyces cerevisiae on steam-exploded biomass treated with laccase. Bioresour. Technol. 135, 239–245. doi:10.1016/j.biortech.2012.11.095
Moreno, A. D., Ibarra, D., Eugenio, M. E., and Tomás-Pejó, E. (2020). Laccases as versatile enzymes: from industrial uses to novel applications. J. Chem. Technol. Biotechnol. 95, 481–494. doi:10.1002/jctb.6224
Moreno, A. D., Ibarra, D., Fernández, J. L., and Ballesteros, M. (2012). Different laccase detoxification strategies for ethanol production from lignocellulosic biomass by the thermotolerant yeast Kluyveromyces marxianus CECT 10875. Bioresour. Technol. 106, 101–109. doi:10.1016/j.biortech.2011.11.108
Moreno, A. D., Ibarra, D., Mialon, A., and Ballesteros, M. (2016b). A bacterial laccase for enhancing saccharification and ethanol fermentation of steam-pretreated biomass. Fermentation 2, 11–15. doi:10.3390/fermentation2020011
Moreno, A. D., Tomás-Pejó, E., Ibarra, D., Ballesteros, M., and Olsson, L. (2013c). Fed-batch SSCF using steam-exploded wheat straw at high dry matter consistencies and a xylose-fermenting Saccharomyces cerevisiae strain: effect of laccase supplementation. Biotechnol. Biofuels 6, 160–210. doi:10.1186/1754-6834-6-160
Moreno, A. D., Tomás-Pejó, E., Ibarra, D., Ballesteros, M., and Olsson, L. (2013d). In situ laccase treatment enhances the fermentability of steam-exploded wheat straw in SSCF processes at high dry matter consistencies. Bioresour. Technol. 143, 337–343. doi:10.1016/j.biortech.2013.06.011
Muthukumarasamy, N. P., Jackson, B., Joseph Raj, A., and Sevanan, M. (2015). Production of extracellular laccase from Bacillus subtilis MTCC 2414 using Agroresidues as a potential substrate. Biochem. Res. Int. 2015, 1–9. doi:10.1155/2015/765190
Muthupriya, P., Bharathy, N. R. M., and Kumar, K. S. (2019). Production of laccase from fungus Pleurotus Florida cultured in different agro-wastes. International Journal of Pharmacy and Biological Sciences 9 (1), 427–436.
Nagula, K. N., and Pandit, A. B. (2016). Process intensification of delignification and enzymatic hydrolysis of delignified cellulosic biomass using various process intensification techniques including cavitation. Bioresour. Technol. 213, 162–168. doi:10.1016/j.biortech.2016.03.152
Neifar, M., Chouchane, H., Mahjoubi, M., Jaouani, A., and Cherif, A. (2016). Pseudomonas extremorientalis BU118: a new salt-tolerant laccase-secreting bacterium with biotechnological potential in textile azo dye decolourization. 3 Biotech. 6, 107–109. doi:10.1007/s13205-016-0425-7
Nicolcioiu, M. B., Popa, G., and Matei, F. (2018). Biochemical investigations of different mushroom species for their biotechnological potential. Agric. Life, Life Agric. Conf. Proc. 1, 562–567. doi:10.2478/alife-2018-0088
Okuda, N., Soneura, M., Ninomiya, K., Katakura, Y., and Shioya, S. (2008). Biological detoxification of waste house wood hydrolysate using Ureibacillus thermosphaericus for bioethanol production. J. Biosci. Bioeng. 106, 128–133. doi:10.1263/jbb.106.128
Oliva-Taravilla, A., Moreno, A. D., Demuez, M., Ibarra, D., Tomás-Pejó, E., González-Fernández, C., et al. (2015a). Unraveling the effects of laccase treatment on enzymatic hydrolysis of steam-exploded wheat straw. Bioresour. Technol. 175, 209–215. doi:10.1016/j.biortech.2014.10.086
Oliva-Taravilla, A., Tomás-Pejó, E., Demuez, M., González-Fernández, C., and Ballesteros, M. (2015b). Inhibition of cellulose enzymatic hydrolysis by laccase-derived compounds from phenols. Biotechnol. Prog. 31, 700–706. doi:10.1002/btpr.2068
Oliva-Taravilla, A., Tomás-Pejó, E., Demuez, M., González-Fernández, C., and Ballesteros, M. (2016). Phenols and lignin: key players in reducing enzymatic hydrolysis yields of steam-pretreated biomass in presence of laccase. J. Biotechnol. 218, 94–101. doi:10.1016/j.jbiotec.2015.11.004
Parawira, W., and Tekere, M. (2011). Biotechnological strategies to overcome inhibitors in lignocellulose hydrolysates for ethanol production: review. Crit. Rev. Biotechnol. 31, 20–31. doi:10.3109/07388551003757816
Pazarlioǧlu, N. K., Sariişik, M., and Telefoncu, A. (2005). Laccase: production by Trametes versicolor and application to denim washing. Process Biochem. 40, 1673–1678. doi:10.1016/j.procbio.2004.06.052
Pinheiro, V. E., Michelin, M., Vici, A. C., de Almeida, P. Z., and Teixeira de Moraes Polizeli, M. de L. (2020). Trametes versicolor laccase production using agricultural wastes: a comparative study in Erlenmeyer flasks, bioreactor and tray. Bioprocess Biosyst. Eng. 43, 507–514. doi:10.1007/s00449-019-02245-z
Pino, M. S., Rodríguez-Jasso, R. M., Michelin, M., Flores-Gallegos, A. C., Morales-Rodriguez, R., Teixeira, J. A., et al. (2018). Bioreactor design for enzymatic hydrolysis of biomass under the biorefinery concept. Chem. Eng. J. 347, 119–136. doi:10.1016/j.cej.2018.04.057
Placido, J., and Capareda, S. (2015). Ligninolytic enzymes: a biotechnological alternative for bioethanol production. Bioresour. Bioprocess. 2–23. doi:10.1186/s40643-015-0049-5
Placido, J., Imam, T., and Capareda, S. (2013). Evaluation of ligninolytic enzymes, ultrasonication and liquid hot water as pretreatments for bioethanol production from cotton gin trash. Bioresour. Technol. 139C, 203–208. doi:10.1016/j.biortech.2013.04.012
Prajapati, H. V. (2018). Laccase - a wonder molecule: a review of its properties and applications. Int. J. Pure Appl. Biosci. 6, 766–773. doi:10.18782/2320-7051.6233
Preethi, P. S., Gomathi, A., Srinivasan, R., Pavan Kumar, J. G. S., Murugesan, K., and Kodiveri Muthukailannan, G. (2020) “Laccase: recombinant expression, engineering and its promising applications,” in Microbial Enzymes: Roles and Applications in Industries, Microorganisms for Sustainability. Singapore: Springer Nature Singapore, 63–85. doi:10.1007/978-981-15-1710-5_3
Prins, A., Kleinsmidt, L., Khan, N., Kirby, B., Kudanga, T., Vollmer, J., et al. (2015). The effect of mutations near the T1 copper site on the biochemical characteristics of the small laccase from Streptomyces coelicolor A3(2). Enzyme Microb. Technol. 68, 23–32. doi:10.1016/j.enzmictec.2014.10.003
Qiao, H., Ouyang, S., Shi, J., Zheng, Z., and Ouyang, J. (2021). Mild and efficient two-step pretreatment of lignocellulose using formic acid solvent followed by alkaline salt. Cellulose 28, 1283–1293. doi:10.1007/s10570-020-03622-8
Qiu, W., and Chen, H. (2012). Enhanced the enzymatic hydrolysis efficiency of wheat straw after combined steam explosion and laccase pretreatment. Bioresour. Technol. 118, 8–12. doi:10.1016/j.biortech.2012.05.033
Radveikienė, I., Vidžiūnaitė, R., Meškienė, R., Meškys, R., and Časaitė, V. (2021). Characterization of a yellow laccase from botrytis cinerea 241. J. Fungi 7, 143–216. doi:10.3390/jof7020143
Ramalingam, B., Sana, B., Seayad, J., Ghadessy, F. J., and Sullivan, M. B. (2017). Towards understanding of laccase-catalysed oxidative oligomerisation of dimeric lignin model compounds. RSC Adv. 7, 11951–11958. doi:10.1039/c6ra26975c
Ranimol, G., Venugopal, T., Gopalakrishnan, S., and Sunkar, S. (2018). Production of laccase from Trichoderma harzianum and its application in dye decolourisation. Biocatal. Agric. Biotechnol. 16, 400–404. doi:10.1016/j.bcab.2018.09.003
Rencoret, J., Marques, G., Gutiérrez, A., Ibarra, D., Li, J., Gellerstedt, G., et al. (2008). Structural characterization of milled wood lignins from different eucalypt species. Holzforschung 62, 514–526. doi:10.1515/HF.2008.096
Rencoret, J., Pereira, A., del Río, J. C., Martínez, A. T., and Gutiérrez, A. (2016). Laccase-mediator pretreatment of wheat straw degrades lignin and improves saccharification. Bioenergy Res. 9, 917–930. doi:10.1007/s12155-016-9745-z
Rezaei, S., Shahverdi, A. R., and Faramarzi, M. A. (2017). Isolation, one-step affinity purification, and characterization of a polyextremotolerant laccase from the halophilic bacterium Aquisalibacillus elongatus and its application in the delignification of sugar beet pulp. Bioresour. Technol. 230, 67–75. doi:10.1016/j.biortech.2017.01.036
Rezania, S., Oryani, B., Cho, J., Talaiekhozani, A., Sabbagh, F., Hashemi, B., et al. (2020). Different pretreatment technologies of lignocellulosic biomass for bioethanol production: an overview. Energy 199, 117457. doi:10.1016/j.energy.2020.117457
Rico, A., Rencoret, J., Río, J. C., Martínez, A. T., and Gutiérrez, A. (2014). Pretreatment with laccase and a phenolic mediator degrades lignin and enhances saccharification of Eucalyptus feedstock. Biotechnology for Biofuels 7 (1), 6.
Rocha-Martín, J., Martínez-Bernal, C., Zamorano, L. S., Reyes-Sosa, F. M., and Díez García, B. (2018). Inhibition of enzymatic hydrolysis of pretreated corn stover and sugar cane straw by laccases. Process Biochem. 67, 88–91. doi:10.1016/j.procbio.2018.01.021
Rodríguez-Couto, S. (2017). Industrial and environmental applications of white-rot fungi. Mycosphere 8, 456–466. doi:10.5943/mycosphere/8/3/7
Rodríguez-Couto, S. (2021). “Biophotodegradation of pollutants from wastewater,” in Bioremediation for environmental sustainability (Elsevier), 269–281.
Rosdee, N. A. S. M., Masngut, N., Shaarani, S. M., Jamek, S., and Sueb, M. S. M. (2020). Enzymatic hydrolysis of lignocellulosic biomass from pineapple leaves by using endo-1,4-xylanase: effect of pH, temperature, enzyme loading and reaction time. IOP Conf. Ser. Mater. Sci. Eng. 736, 022095. doi:10.1088/1757-899X/736/2/022095
Rosgaard, L., Andric, P., Dam-Johansen, K., Pedersen, S., and Meyer, A. S. (2007). Effects of substrate loading on enzymatic hydrolysis and viscosity of pretreated barley straw. Appl. Biochem. Biotechnol. 143, 27–40. doi:10.1007/s12010-007-0028-1
Salcedo Martnez, S. M., Gutirrez-Soto, G., Rodriguez Garza, C. F., Villarreal Galvan, T. J., Contreras Cordero, J. F., and Hernandez Luna, C. E. (2013). “Purification and partial characterization of a thermostable laccase from pycnoporus sanguineus CS-2 with ability to oxidize high redox potential substrates and recalcitrant dyes,” in Applied Bioremediation - Active and Passive Approaches (INTECH). doi:10.5772/56374
Salvachúa, D., Prieto, A., López-Abelairas, M., Lu-Chau, T., Martínez, Á. T., and Martínez, M. J. (2011). Fungal pretreatment: an alternative in second-generation ethanol from wheat straw. Bioresour. Technol. 102, 7500–7506. doi:10.1016/j.biortech.2011.05.027
Sanchez, O., Sierra, R., and J, C. (2011). Delignification process of agro-industrial wastes an alternative to obtain fermentable carbohydrates for producing fuel. Altern. Fuel. doi:10.5772/22381
Santos, J. I., Fillat, Ú., Martín-Sampedro, R., Ballesteros, I., Manzanares, P., Ballesteros, M., et al. (2015). Lignin-enriched fermentation residues from bioethanol production of fast-growing poplar and forage sorghum. BioResources 10, 5203–5214. doi:10.15376/biores.10.3.5215-5232
Scopel, E., Santos, L. C. dos, Bofinger, M. R., Martínez, J., and Rezende, C. A. (2020). Green extractions to obtain value-added elephant grass co-products in an ethanol biorefinery. J. Clean. Prod. 274, 122769. doi:10.1016/j.jclepro.2020.122769
Shanmugam, S., Hari, A., Ulaganathan, P., Yang, F., Krishnaswamy, S., and Wu, Y.-R. (2018). Potential of biohydrogen generation using the delignified lignocellulosic biomass by a newly identified thermostable laccase from Trichoderma asperellum strain BPLMBT1. Int. J. Hydrogen Energy 43, 3618–3628. doi:10.1016/j.ijhydene.2018.01.016
Shanmugam, S., Krishnaswamy, S., Chandrababu, R., Veerabagu, U., Pugazhendhi, A., and Mathimani, T. (2020). Optimal immobilization of Trichoderma asperellum laccase on polymer coated Fe3O4@SiO2 nanoparticles for enhanced biohydrogen production from delignified lignocellulosic biomass. Fuel 273, 117777. doi:10.1016/j.fuel.2020.117777
Sharma, D., Garlapati, V. K., and Goel, G. (2016). Bioprocessing of wheat bran for the production of lignocellulolytic enzyme cocktail by Cotylidia pannosa under submerged conditions. Bioengineered 7, 88–97. doi:10.1080/21655979.2016.1160190
Shirkavand, E., Baroutian, S., Gapes, D. J., and Young, B. R. (2017). Pretreatment of radiata pine using two white rot fungal strains Stereum hirsutum and Trametes versicolor. Energy Convers. Manag. 142, 13–19. doi:10.1016/j.enconman.2017.03.021
Shraddha, K. N., Shekher, R., Sehgal, S., Kamthania, M., and Kumar, A. (2011). Laccase: microbial sources, production, purification, and potential biotechnological applications. Enzyme Res. 2011, 1–11. doi:10.4061/2011/217861
Shukla, A., Kumar, D., Girdhar, M., Kumar, A., Goyal, A., Malik, T., et al. (2023). Strategies of pretreatment of feedstocks for optimized bioethanol production: distinct and integrated approaches. Biotechnol. Biofuels Bioprod. 16, 44–33. doi:10.1186/s13068-023-02295-2
Shukla, P. (2019). Synthetic biology perspectives of microbial enzymes and their innovative applications. Indian J. Microbiol. 59, 401–409. doi:10.1007/s12088-019-00819-9
Singh, D., and Gupta, N. (2020). Microbial Laccase: a robust enzyme and its industrial applications. Biol. Bratisl. 75, 1183–1193. doi:10.2478/s11756-019-00414-9
Singh, G., Bhalla, A., Kaur, P., Capalash, N., and Sharma, P. (2011). Laccase from prokaryotes: a new source for an old enzyme. Rev. Environ. Sci. Biotechnol. 10, 309–326. doi:10.1007/s11157-011-9257-4
Singh Arora, D., and Kumar Sharma, R. (2010). Ligninolytic fungal laccases and their biotechnological applications. Appl. Biochem. Biotechnol. 160, 1760–1788. doi:10.1007/s12010-009-8676-y
Sitarz, A. K., Mikkelsen, J. D., Højrup, P., and Meyer, A. S. (2013). Identification of a laccase from Ganoderma lucidum CBS 229.93 having potential for enhancing cellulase catalyzed lignocellulose degradation. Enzyme Microb. Technol. 53, 378–385. doi:10.1016/j.enzmictec.2013.08.003
Solarte-Toro, J. C., Romero-García, J. M., Martínez-Patiño, J. C., Ruiz-Ramos, E., Castro-Galiano, E., and Cardona-Alzate, C. A. (2019). Acid pretreatment of lignocellulosic biomass for energy vectors production: a review focused on operational conditions and techno-economic assessment for bioethanol production. Renew. Sustain. Energy Rev. 107, 587–601. doi:10.1016/j.rser.2019.02.024
Sondhi, S., Kaur, R., and Madan, J. (2021). Purification and characterization of a novel white highly thermo stable laccase from a novel Bacillus sp. MSK-01 having potential to be used as anticancer agent. Int. J. Biol. Macromol. 170, 232–238. doi:10.1016/j.ijbiomac.2020.12.082
Song, Q., Deng, X., and Song, R. Q. (2020). Expression of pleurotus ostreatus laccase gene in pichia pastoris and its degradation of corn stover lignin. Microorganisms 8, 601. doi:10.3390/microorganisms8040601
Srivastava, R. K., Shetti, N. P., Reddy, K. R., and Aminabhavi, T. M. (2020). Biofuels, biodiesel and biohydrogen production using bioprocesses. A review. Environ. Chem. Lett. 18, 1049–1072. doi:10.1007/s10311-020-00999-7
Su, T., Zhao, D., Khodadadi, M., and Len, C. (2020). Lignocellulosic biomass for bioethanol: recent advances, technology trends, and barriers to industrial development. Curr. Opin. Green Sustain. Chem. 24, 56–60. doi:10.1016/j.cogsc.2020.04.005
Sun, K., Li, S., Si, Y., and Huang, Q. (2021). Advances in laccase-triggered anabolism for biotechnology applications. Crit. Rev. Biotechnol. 0, 969–993. doi:10.1080/07388551.2021.1895053
Sun, W., Xu, M., Xia, C., Li, A., and Sun, G. (2013). Enhanced production of laccase by Coriolus hirsutus using molasses distillery wastewater. Front. Environ. Sci. Eng. 7, 200–210. doi:10.1007/s11783-012-0457-0
Tabka, M. G., Herpoël-Gimbert, I., Monod, F., Asther, M., and Sigoillot, J. C. (2006). Enzymatic saccharification of wheat straw for bioethanol production by a combined cellulase xylanase and feruloyl esterase treatment. Enzyme Microb. Technol. 39, 897–902. doi:10.1016/j.enzmictec.2006.01.021
Tapia-Tussell, R., Pereira-Patrón, A., Alzate-Gaviria, L., Lizama-Uc, G., Pérez-Brito, D., and Solis-Pereira, S. (2020). Decolorization of textile effluent by Trametes hirsuta bm-2 and lac-T as possible main laccase-contributing gene. Curr. Microbiol. 77, 3953–3961. doi:10.1007/s00284-020-02188-9
Tiwari, A., Chen, C.-W., Haldar, D., Patel, A. K., Dong, C.-D., and Singhania, R. R. (2023). Laccase in biorefinery of lignocellulosic biomass. Appl. Sci. 13, 4673. doi:10.3390/app13084673
Umar, A. (2021). Screening and evaluation of laccase produced by different Trichoderma species along with their phylogenetic relationship. Arch. Microbiol. 203, 4319–4327. doi:10.1007/s00203-021-02420-5
Wang, Q., Ding, L., and Zhu, C. (2018a). Characterization of laccase from a novel isolated white-rot fungi Trametes sp. MA-X01 and its potential application in dye decolorization. Biotechnol. Biotechnol. Equip. 32, 1477–1485. doi:10.1080/13102818.2018.1517028
Wang, S. N., Chen, Q. J., Zhu, M. J., Xue, F. Y., Li, W. C., Zhao, T. J., et al. (2018b). An extracellular yellow laccase from white rot fungus Trametes sp. F1635 and its mediator systems for dye decolorization. Biochimie 148, 46–54. doi:10.1016/j.biochi.2018.02.015
Wang, X., Yao, B., and Su, X. (2018c). Linking enzymatic oxidative degradation of lignin to organics detoxification. Int. J. Mol. Sci. 19, 3373. doi:10.3390/ijms19113373
Wang, Z., Hu, R., Ye, X., Huang, J., Chen, X., and Shi, M. (2018d). Laccase 1 gene from Plutella xylostella (PxLac1) and its functions in humoral immune response. J. Insect Physiol. 107, 197–203. doi:10.1016/j.jinsphys.2018.04.001
Wei, W., and Xianghua, W. E. N. (2009). Expression of lignin peroxidase H2 from Phanerochaete chrysosporium by multi-copy recombinant Pichia strain. J. Environ. Sci. 21, 218–222. doi:10.1016/S1001-0742(08)62254-8
Wu, M. H., Lin, M. C., Lee, C. C., Yu, S. M., Wang, A. H. J., and Ho, T. H. D. (2019). Enhancement of laccase activity by pre-incubation with organic solvents. Sci. Rep. 9, 9754–9811. doi:10.1038/s41598-019-45118-x
Yang, J., Li, W., Bun Ng, T., Deng, X., Lin, J., and Ye, X. (2017). Laccases: production, expression regulation, and applications in pharmaceutical biodegradation. Front. Microbiol. 8, 832. doi:10.3389/fmicb.2017.00832
Yin, Q., Zhou, G., Peng, C., Zhang, Y., Kües, U., Liu, J., et al. (2019). The first fungal laccase with an alkaline pH optimum obtained by directed evolution and its application in indigo dye decolorization. Amb. Express 9, 151–213. doi:10.1186/s13568-019-0878-2
Yu, H., Du, W., Zhang, J., Ma, F., Zhang, X., and Zhong, W. (2010a). Fungal treatment of cornstalks enhances the delignification and xylan loss during mild alkaline pretreatment and enzymatic digestibility of glucan. Bioresour. Technol. 101, 6728–6734. doi:10.1016/j.biortech.2010.03.119
Yu, H., Zhang, X., Song, L., Ke, J., Xu, C., Du, W., et al. (2010b). Evaluation of white-rot fungi-assisted alkaline/oxidative pretreatment of corn straw undergoing enzymatic hydrolysis by cellulase. J. Biosci. Bioeng. 110, 660–664. doi:10.1016/j.jbiosc.2010.08.002
Zanirun, Z., Bahrin, E. K., Lai-Yee, P., Hassan, M. A., and Abd-Aziz, S. (2015). Enhancement of fermentable sugars production from oil palm empty fruit bunch by ligninolytic enzymes mediator system. Int. Biodeterior. Biodegr. 105, 13–20. doi:10.1016/j.ibiod.2015.08.010
Zhang, J., Qu, Y., Xiao, P., Wang, X., Wang, T., and He, F. (2012). Improved biomass saccharification by Trichoderma reesei through heterologous expression of lacA gene from Trametes sp. AH28-2. J. Biosci. Bioeng. 113, 697–703. doi:10.1016/j.jbiosc.2012.01.016
Keywords: biofuels, bioethanol, enzymatic hydrolysis, microbial laccases, detoxification, delignification, laccase mediator system
Citation: Haq Iu, Saleem A, Chaudhary R, Alessa AH, Nawaz A and Du C (2024) Role of microbial laccases in valorization of lignocellulosic biomass to bioethanol. Front. Bioeng. Biotechnol. 12:1441075. doi: 10.3389/fbioe.2024.1441075
Received: 30 May 2024; Accepted: 04 July 2024;
Published: 23 July 2024.
Edited by:
Lei Wang, Ocean University of China, ChinaReviewed by:
Norazah Mohammad Nawawi, Selangor University, MalaysiaAhmed Nawaz, The Open University, United Kingdom
Copyright © 2024 Haq, Saleem, Chaudhary, Alessa, Nawaz and Du. This is an open-access article distributed under the terms of the Creative Commons Attribution License (CC BY). The use, distribution or reproduction in other forums is permitted, provided the original author(s) and the copyright owner(s) are credited and that the original publication in this journal is cited, in accordance with accepted academic practice. No use, distribution or reproduction is permitted which does not comply with these terms.
*Correspondence: Ali Nawaz, YS5uYXdhekBodWQuYWMudWs=
†These authors have contributed equally to this work