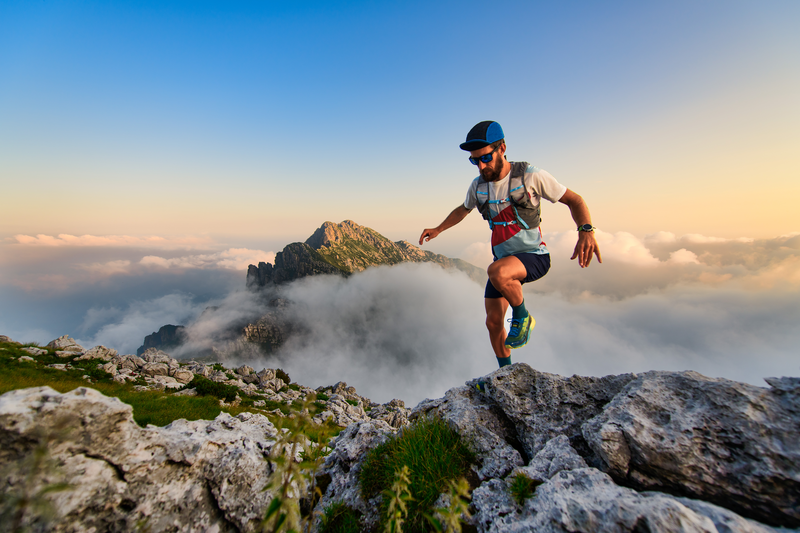
94% of researchers rate our articles as excellent or good
Learn more about the work of our research integrity team to safeguard the quality of each article we publish.
Find out more
REVIEW article
Front. Bioeng. Biotechnol. , 05 August 2024
Sec. Tissue Engineering and Regenerative Medicine
Volume 12 - 2024 | https://doi.org/10.3389/fbioe.2024.1428793
This article is part of the Research Topic Translation of Mesenchymal Stem Cells in vivo: Evidence from preclinical and clinical testing. View all articles
Wound healing is a complicated obstacle, especially for chronic wounds. Mesenchymal stem cell-derived exosomes may be a promising cell-free approach for treating skin wound healing. Exosomes can accelerate wound healing by attenuating inflammation, promoting angiogenesis, cell proliferation, extracellular matrix production and remodeling. However, many issues, such as off-target effects and high degradation of exosomes in wound sites need to be addressed before applying into clinical therapy. Therefore, the bioengineering technology has been introduced to modify exosomes with greater stability and specific therapeutic property. To prolong the function time and the local concentration of exosomes in the wound bed, the use of biomaterials to load exosomes emerges as a promising strategy. In this review, we summarize the biogenesis and characteristics of exosomes, the role of exosomes in wound healing, and the therapeutic applications of modified-exosomes in wound healing. The challenges and prospects of exosomes in wound healing are also discussed.
Wound healing is a complicated process, especially for chronic wounds, such as diabetic ulcers. Prolonged wound healing is an intractable problem for clinicians and a heavy burden for patients and society. Therefore, many different therapies have been introduced in wound care, including skin flap transplantation, skin substitutes, which methods are limited by high cost, infection, scarring during cutaneous wound healing. As translational and regenerative medicine progress, stem cell-based therapy have gained wide popularity due to their regenerative capacity (Joseph et al., 2020). Corroborating evidence shows that stem cell administration promotes regenerative healing of wounded skin primarily mainly through paracrine mechanisms by releasing growth factors and extracellular vesicles (EVs), including exosomes (Tkach and Théry, 2016; Zhang et al., 2020). The contents of EVs from stem cells were transferred to surrounding microenvironment and exhibit their therapeutic effects even when parental cells were cleared by the host. Moreover, EVs are easily and stably stored, not rejected by the immune system, and have a homing effect. Therefore, the use of extracellular vesicles presents a potential cell-free translational therapy (Ho et al., 2023).
Exosomes are a subset of small lipid bilayer EVs, with 40–160 nm in diameter. They are secreted from most cells into extracellular fluids by multivesicular bodies (MVBs), including stem cells and immune cells involved in wound healing (An et al., 2021). Exosomes are important participants in intercellular communication by transferring their cargo contents (proteins, RNA, lipids and other biologically active components) to the cytoplasm of target cells, thereby changing the biological properties of the recipient cells. Accumulated evidences verifies that exosomes can inhibit inflammation, enhance neovascular formation, promote cell proliferation and stimulate collage deposition to accelerate wound healing (Ha et al., 2020; Zhou et al., 2020; An et al., 2021). There are increasing studies describing a relationship between exosomes and cutaneous wound healing and summarized their underlying molecular mechanisms.
Although exosomes have great potential for accelerating skin wound healing, how to maximize the therapeutic effect of exosomes depends on other factors, like exosomes engineering and the delivery methods of exosomes (Dong et al., 2023; Zhao et al., 2023). In this review, we first described the characteristic of exosomes, the role of exosomes in wound healing process. More importantly, we summarized and discussed the application of exosomes to the wound healing, including how to modify exosomes by bioengineering and the delivery methods of exosomes in detail.
The EVs are generally classified into two major categories: ectosomes and exosomes. Ectosomes are generated by directly budding outwards from the plasma membrane, producing large vesicles with the size range of 50 nm to 1 um in diameter. By contrast, exosomes are formed by a endosomal way, with 40nm–160 nm in diameter (Meldolesi, 2018). The generation of exosomes is a tightly regulated process, mainly including three main processes (Figure 1): endocytic vesicles, multivesicular bodies (MVBs) formation, and secretion of exosomes (Arya et al., 2024).
Figure 1. The generation and release of exosomes. Early endosomes are formed by an endosomal way, matured to form MVBs by ESCRT-depend and non-depend pathways, and then secreted into intercellular microenvironment. Exosomes can transmit donor cell information to recipient cells through three different pathways, including 1) exosomes directly fuse with cell membranes through interacting with trans-membrane proteins on the surface of recipient cells; 2) exosomes recruit into the cell body through filopodia and endocytosed by recipient cells; 3) ligands on exosomes interact with receptors on the membrane of recipient cells, and then trigger intracellular signaling cascades.
The cell membrane buds inward to form early endosomes, in which “intraluminal vesicles” (ILVs) develop, and then matured to form MVBs. Generally, the mechanism that described the generation of ILVs and MVBs is “Endosome Sorting Complex Required for Transport” (ESCRT) -dependent and non-dependent pathways (Arya et al., 2024). The Rab family of small GTPases plays a vital regulatory mediator in intracellular transport and secretion. Besides, Rab27a and Rab27b are demonstrated to promote the formation and stability of MVBs (Ruivo et al., 2022). KIBRA was shown to regulate exosome secretion by blocking proteasome degradation of Rab27a, and KIBRA depletion may result in an increase in the quantity and size of MVBs (Song et al., 2019). Generally, the MVBs transfer to the plasma membrane and released into the extracellular space, but some are degraded by fusing with the lysosome (Arya et al., 2024).
Exosomes, often known as “cargo,” usually encapsulate variety of bioactive molecules depending on the source and state of cell and its microenvironment. Exosomes can transfer the effector molecules to the recipient cells via following three pathways (Figure 1): I) Exosomes directly deliver inclusions to recipient cells by combining with recipient cells by fusing with the cell membrane through interacting with trans-membrane proteins on the surface; II) Exosomes are recruited to cell body by filopodia and then endocytosed by recipient cells that resemble virus entry to cells; III) The ligands on exosomes interact with the receptor on recipient cell membrane, and then trigger the intracellular signaling cascade (Liang et al., 2021).
Exosomes involved in skin wound healing are mainly derived from adipose-derived stem cells (ADSCs) (Jiang et al., 2022a; Liang et al., 2022; Shi et al., 2022), bone marrow mesenchymal stem cells (BMSCs) (Pomatto et al., 2021; Born et al., 2022; Wang et al., 2022), umbilical cord-derived mesenchymal stem cells (UCMSCs) (Yang et al., 2020; Yan et al., 2022b), macrophages (Li et al., 2019), human umbilical vein endothelial cells (HUVECs), epidermal stem cells (ESCs) (Xu et al., 2020) and dermal fibroblasts (Han et al., 2021). Based on the abundant sources and assured efficacy, ADSCs and BMSCs were the most dependable source of exosomes, which are studied by most of researches. Besides, exosomes are also isolated from non-cellular sources, such as serum (Xu et al., 2018; Chen L. et al., 2021), platelet-rich plasma (PRP) (Guo et al., 2017) and milk (Yan et al., 2022a). Although they have abundant sources, their stability and effectiveness still need to be further confirmed.
Different approaches have been developed for the isolation and purification of exosomes, including ultracentrifugation, ultrafiltration, precipitation, size exclusion chromatography, immunoaffinity capture and microfluidics (Coumans et al., 2017; Li et al., 2017). To date, the widely accepted method is the differential ultracentrifugation. No uniform strategy has been established for exosome isolation with the highest quality and properties, and a combination of different methods is usually required to achieve optimal results. The isolation of exosomes can be detected by transmission electron microscope (TEM) and nanoparticle tracking analysis (NTA), and the exosomal markers, including tetraspanins (CD9, CD81, and CD63) (Fan et al., 2023; Ai et al., 2024), tumor susceptibility gene 101 proteins (TSG101) (Liu et al., 2021), heat shock proteins (HSP60 and HSP90) (Liu et al., 2021; Ghafourian et al., 2022) can be analyzed by Western blotting method.
Wound healing proceeds through four distinct but overlapping phases (Figure 2): Hemostasis phase, inflammation response, cellular proliferation and tissue remodeling phase (Wilkinson and Hardman, 2020; Bian et al., 2022). i) Hemostasis phase: the primary step of healing to end bleeding. The platelets are activated and support a stable clot by thrombin, contributing to the generation of a fibrin clot entrapping blood cells in the injured area (Hoffman, 2018; Zhu et al., 2018). ii) Inflammatory phase: deteriorating bacteria and pathogens, local and system immune response. Above events mainly depend on the recruitment and activation of neutrophils and macrophages which clear invading bacteria and cellular debris via direct functions or the release of multiple mediators (Shah and Amini-Nik, 2017; Raziyeva et al., 2021). iii) Proliferative phase: granulation tissue is formed, which depends on epithelialization and myofibroblasts functions. Epithelial cells proliferate and migrate from the margins to the injured area to form epithelialization (Ben Amar and Wu, 2014). Fibroblasts proliferate and synthesize matrix and collagen to provide an external bed for cell attachment scaffolding and repair (Shpichka et al., 2019). Meanwhile, abundant blood vessels is urgently required to provide sufficient oxygen and nutrients for granulation tissue (Wilkinson and Hardman, 2020). (iiii) Remodeling phase: collagen deposition and the entirely closed wound. Collagen is cross-linked between collagen fibers and remodeled gradually from type III to type I collagen. New tissue completely covers the injured area, and slowly attains strength and flexibility, and finally scar tissue is formed (Shpichka et al., 2019; Wilkinson and Hardman, 2020).
Figure 2. The stages of wound healing. In hemostasis phase, the platelet aggregates to the wound to block blood loss and an initial fibrin clot is formed; In inflammatory phase, local and system immune respond to attenuate invading bacteria and pathogens, with the recruitment and activation of neutrophils and macrophages; In proliferative phase, granulation tissue is formed, along with abundant blood vessels, re-epithelialization, fibroblasts proliferation and migration, and extracellular matrix synthesis; In remodeling phase, with matrix deposition and remodeling, the wound entirely closed, with possible scar formation.
In various phases of wound healing process, exosomes regulate the function of keratinocytes, fibroblasts, macrophages and endothelial cells, laying a better foundation for reducing inflammation, forming subsequent angiogenesis, accelerating the proliferation phase, and aiding in tissue remodeling (Figure 3). In the subsequent section, we will elaborate on the impact of exosomes on wound healing (Table 1).
Figure 3. The mechanisms of exosomes in wound healing process. (A) Exosomes increase the M2/M1 polarization rate by up-regulating the driver anti-inflammatory cytokines (IL-10) and down-regulating the levels of inflammatory factors (IL-1β, TNF-α), and induce T cells differentiated into Treg cells through TGF-β pathway, upregulation IDO or Foxp3. (B) Exosomes stimulate endothelial cells proliferation and migration through activating the PKA pathway or PTEN/PI3K/AKT pathway or MAPK pathway, and transferring miRNAs (miR-126-3p, miR-21-3p, miR-146a-3p) to promote angiogenesis. (C) Exosomes promote the proliferation and migration of keratinocytes via Wnt/β-catenin pathway to enhance re-epithelization, and fibroblasts via AKT pathway to promote ECM synthesis and remodel. Lately, exosomes regulate the differentiation of fibroblasts to myofibroblasts, and the ratio of collagen I to collagen III to reduce scar formation.
Injury leads to immediate activation of clotting cascade to initiated the recruitment of inflammatory cells. It has been verified that the increased pro-inflammatory cellular infiltrates, largely composed of polymorphonuclear neutrophils (PMNs), macrophages and lymphocytes, contribute to delayed wound healing. More evidences demonstrated that MSC-exos exerted an immunosuppression effect by interacting with immune cells or regulating the ratio of pro-inflammatory and anti-inflammatory factors (Whiteside, 2018; Qian et al., 2021). For instance, MSC-exos can increase the M2/M1 polarization rate by modulating the levels of inflammatory factors, such as up-regulating the driver anti-inflammatory cytokine (IL-10) and down-regulating the levels of inflammatory factors (IL-1β, TNF-α) (Curtale et al., 2013; Yang et al., 2015; Li et al., 2016; Lo Sicco et al., 2017; Tao et al., 2021). Exosomes can induce differentiation of T cells and increase the transformation to anti-inflammatory phenotypes, such as regulatory T (Treg) cells, through up-regulating IDO, Foxp3 (Chen et al., 2016; Harrell et al., 2019; Heo et al., 2019; Zhou et al., 2021). These studies indicate that exosomes can suppress the occurrence of excessive inflammation and the adverse effects of inflammation during wound healing process.
Angiogenesis plays a key role in wound healing. This process mainly includes the proliferation, migration and tube formation of vascular endothelial cells (Demidova-Rice et al., 2012). New blood vessels carry oxygen, nutrients, and various growth factors to maintain the survival, proliferation, and differentiation ability of new tissues (Baru et al., 2021). Exosomes contain a variety of growth factors (VEGF, BFGF, and HGF), cytokines and chemokines, which promote endothelial cell proliferation and migration indirectly promote angiogenesis (Han et al., 2020). HUVECs-exos upregulated the expression of endogenous VEGF upon activation of PKA signaling, as well as the pro-angiogenic genes Angpt1 and Flk1 (Xue et al., 2018). Delivery of HGF by exosomes can maintain vascular stability and promote neovascularization through activating the PTEN/PI3K/Akt and MAPK pathways (Oliveira et al., 2018; Zhang et al., 2018; Yan et al., 2021; Yang et al., 2021; Zhao et al., 2022). In addition, a variety of miRNAs contained in exosomes can also promote endothelial cell proliferation and migration. For instance, MSCs-exos include miR-126-3p, miR-21-3p, miR-146a-3p have the ability to increase the proliferation and migration of endothelial cells via PI3K/AKT and ERK signaling pathways, respectively (Tao et al., 2017; Hu et al., 2018; Wu et al., 2020b; Chen G. Q. et al., 2021). Taken together, these findings indicate that exosomes have great potential on angiogenesis to promote wound healing.
The wound proliferative phase mainly focuses on cell proliferation, re-epithelialization and synthesis of matrix proteins to form the granulation tissue, providing sufficient oxygen and nutrients for wound healing and better regeneration of damaged tissue (Eming et al., 2014; Bian et al., 2022). Epithelial cells at the wound edge proliferate and migrate towards the wound center, promoting re-epithelialization and closure of the wound edge. UMSCs-exos were revealed to inhibit HaCaT cell apoptosis by activating the Wnt/β-catenin signaling pathway and promote cell proliferation and re-epithelialization in a rat model of deep second-degree burns (Zhang et al., 2015; Ma et al., 2019). Exosomes from different stem cells have been shown to promote migration and proliferation of fibroblasts. ADSCs-exos can be internalized by fibroblasts and stimulate fibroblast proliferation, migration, and collagen synthesis in a dose-dependent manner (An et al., 2021). The granulation tissues, mainly composed of fibronectin, replaces the provisional extracellular matrix (ECM) during the proliferative phase and creates a scaffold for early deposition of type III collagen and later type I collagen synthesis (Diegelmann and Evans, 2004). Han et al. (2021) revealed that exosomes derived from autologous dermal fibroblasts promote collagen deposition to stimulate diabetic cutaneous wound healing by the Akt/β-catenin pathway. Zhao et al. (2021) showed that ADSCs-exos also accelerate wound healing by promoting collagen synthesis and deposition. In summary, exosomes from different stem cell sources can have a promoting effect on granulation tissue expansion, re-epithelialization, and matrix protein synthesis.
Wound remodeling phase mainly includes secretion of extracellular matrix (ECM), collagen remodeling, and the proliferation of neoplastic granulation tissue to cover the damaged area. During the reorganization and remodeling of collagen fibers in damaged skin, exosomes derived from ADSCs contain lncRNA MALAT1 and other factors that stimulate the migration and angiogenesis of fibroblasts, which promote the remodeling of collagen fibers (He et al., 2020). Exosomes also modulate TGF-β levels, causing myofibroblasts to appear transiently, promoting wound contraction and collagen fiber reorganization (Fang et al., 2016). But overproliferated fibroblasts and over-deposited collagen may result in scar formation. In contrast, ADSC-exos reduced collagen deposition after injection into injured tissues in vivo, increased the ratio of collagen III to collagen I, and inhibited ECM over-deposition by secreting Matrix metalloproteinase-1 (MMP-1), attenuating scar formation (Hu et al., 2016; Wang et al., 2017; Li et al., 2021). These studies suggest that exosomes from different stem cell sources play a crucial role in promoting collagen remodeling and ameliorating scarring, and are expected to provide new therapeutic strategies for wound treatment.
Through the above aspects, exosomes were a promising approach for treating wound healing. However, its clinical efficacy still depends on many other factors, such as specific target capability, delivery methods and high concentration stability. In this part, we will summarize and discuss how to engineer exosomes, optimize delivery methods and modify dosage and frequency (Figure 4).
Figure 4. The local application of bio-engineered exosomes into wound healing. Exosomes can be modified by the following ways: 1) Direct exosomes engineering and 2) parental cell-based exosomes engineering. The methods of direct modification are divided into chemical methods including co-incubation, direct fusion with liposomes packaging targets molecules and conjugate ligands to the exosomal surface, and physical methods including electroporation, sonication and extrusion. The parental cell-based exosomes engineering can be achieved by genetic engineered cells or changing the cultural environment of cells or incubating with desired molecules. Biomaterials-based exosomal delivery systems for local application depends on different forms, including hydrogels, nanofiber materials or the combination of both via injection, smearing or wound dressings.
To improve the effective application of exosomes for accelerating wound healing, bioengineering techniques have been incorporated by researchers into exosome-based therapy to enhance their targeting efficacy, loading efficiency and stability. Currently engineering strategies mainly fall into two categories: direct exosome engineering and parental cell-based exosome engineering (Lu et al., 2022). These methods result in different loading efficiency and stability of agents with exosomes.
The exosomes usually work as a carrier, target substrates can be directly delivered into exosomes through biochemical or physical methods.
The biochemical methods to modify exosomes is an easy, fast but high effective method. Some small-molecule drugs and RNAs were incorporated into exosomes via directly co-incubating, which interact with the lipid layers of membrane (Zhuang et al., 2022). This method is limited to the low loading efficiency, depending on the gradient concentration and the hydrophobicity of drugs (Sadeghi et al., 2023). A surfactant molecular saponin could increase membrane permeabilization, improving the loading efficiency (Fuhrmann et al., 2015; Johnsen et al., 2016). However, there are concerns regarding the concentration of saponin, especially when the exosomes are used in vivo (Johnsen et al., 2016).
Another method is direct modification through membrane fusion with liposomes embedded with target molecules. Matsuoka T et al. created HER2-containing exosomes with liposomes via freeze-thaw method (Matsuoka and Yashiro, 2015). This strategy not only improves the characteristics and stability of exosomes, but also reduce the immunogenicity.
Another strategy is to conjugate functional ligands to the surface of exosomes using chemical methods, such as click chemistry or azide-alkyne cycloaddition (Villata et al., 2020). For instance, the ligand cyclo (Arg-Gly-Asp-D-Tyr-Lys) peptide [c (RGDyK)], targeting at integrin αvβ3, were conjugated to modify exosomes (Arosio and Casagrande, 2016; Khan et al., 2016). This conjugation reactions were mildly on exosomes structure or function, maintaining the size of exosomes (Smyth et al., 2014). Based on this strategy, metabolic glycoengineering technique is created to track the localization of the therapeutic cells through exosomes. In above, biochemical modification to the exosome surface can determine cell-type targeting specificity, overcoming off-target effects. However, this method may cause exosome aggregation or surface protein inactivation (Mondal et al., 2023). And it needs attention that temperature, salt concentration or pressure used may result in surface protein denaturation, membrane rupture or excessive osmotic pressure.
Electroporation is a promising physical strategy for large compounds which is difficult to encapsulate in exosomes. Electroporation can create small temporary pores on the exosome membrane by disturbing the phospholipid bilayer through an electrical field (Luan et al., 2017). For instance, loading miR-21-5p into ADSCs-derived exosomes by electroporation methods demonstrated excellent effects on skin wound healing through accelerating re-epithelialization, angiogenesis and collagen remodeling (Lv et al., 2020). Yan et al. successfully encapsulated miR-31-5p mimic into milk-derived exosomes, and then showed that it could enhance wound healing by promoting angiogenesis in vivo (Yan et al., 2022a).
Sonication is also widely used method for exosome engineering. For this method, drugs are diffused into the exosomes through compromising the exosome membrane integrity by the mechanical shear stress from the sonicator probe. Kim et al. (2016) observed that the membrane integrity of the exosomes is recovered within an hour. In certain instances, drugs not only are encapsulated inside the exosomes but also adhered to the membrane’s outer layers, causing a bulk of drugs firstly burst and release and the following slow release. Generally, the sonication method has more higher loading efficiency than co-incubation and electroporation (Luan et al., 2017).
Extrusion is an another method to load exogenous cargos to exosomes by harsh mechanical force. In contrast to electroporation, the exosome membrane is disrupted and then followed by drugs passing through (Khongkow et al., 2019; Van Deun et al., 2020; Hajipour et al., 2021). According to previous studies, extrusion has some important shortcomings, including low throughout, low loading efficiency and the disruption of the membrane stability resulting in other undesirable side effects (Fuhrmann et al., 2015; Zhao et al., 2023).
The parental cell-based exosome engineering aims to produce specifically labeled exosomes or improve the yield of exosomes without any change in exosome structures. The genetic engineering of parental cells was a convenient and stable method. The donor cells are modified via lentivirus or specific mRNAs by inserting the desired target-coding sequence, and then the exosomes carrying target cargoes were isolated and applied for wound healing (Golchin et al., 2022). Exosomes produced from Nrf2-overexpressed ADSCs could lower the inflammation level, enhance the formation of granulation tissue formation and promote the growth factor expression (Li et al., 2018). Exosomes derived from circular RNAs (circRNAs) mmu_circ_0000250 modified ADSCs promoted wound healing by absorption of miR-138-3p and upregulation of SIRT1.
Another method to change the cargoes in exosomes is realized by changing the cultural environment of donor cells or incubating with desired molecules. This method focuses on the preconditioning of parental cells, mainly include hypoxic, cytokine and chemical preconditioning (Lu et al., 2023). For example, atorvastatin-pretreated MSCs promote diabetic wound healing by enhancing angiogenesis through AKT/eNOS pathway (Yu et al., 2020). Exosomes derived from ADSCs cultured under hypoxia condition accelerate wound healing via activating PI3K/Akt pathway (Wang et al., 2021). Compared with genetic engineering of parental cells, this method is relatively simple, but the loading efficiency is low and cytotoxic (Antimisiaris et al., 2018).
In summary, no matter direct bioengineering modified exosomes or parental cell-based exosomes engineering, they in a certain extent showed benefits to the wound healing. However, the contents of exosomes were diverse and complex. The alteration of a single components may cause other unexpected changes, resulting in some safety concerns.
From above aspects, engineered exosomes might be a promising approach for accelerating wound healing. But exosomes not only are rapidly degraded from the systemic circulation, but also are easier to aggregate over time, which limits the clinical application. Therefore, to improve the therapeutic effect of exosomes, the combination of exosomes and biomaterials were applied to deliver exosomes, achieving a controlled way to release the exosomes for long time, which can be organized into local application combined with scaffold materials and systemic application by injection.
Materials loaded with exosomes delivery methods for skin wounds includes local injection and wound dressing. Most studies applied hydrogels by subcutaneous injection onto the wound bed, or around the wounds, or combination of both methods. Some studies delivered hydrogels by intradermal injection around the wound beds, directly interacting with cells in the dermis. To perforate through subcutaneous tissue microneedles (MNs) have progressively attracted more attention. However, the injection method may bring pain to the patients, and the dosage or frequency are unavoidable and intractable issues. In addition, free exosomes injected are usually lapsed rapidly. Another delivery method, biomedical dressings have been discussed extensively in the application of exosomes in wound healing therapy. To maintain a desirable moist environment and exosomes biological activity, hydrogel was widely used and studied. However, the small pore diameter, low porosity and friability limited its application. Therefore, the combination of fiber and hydrogel will become the major trend of wound dressing in the future.
Nowadays, biomaterials-based exosome delivery systems for local application depends on different forms, including hydrogels, nanofiber materials or the combination of both.
Hydrogel are three-dimensional hydrophilic polymer networks by cross-linking to form matrices with water. Because of their inherent similarities to extracellular matrix (ECM), good biocompatibility, favorable oxygen transport and high water content, hydrogels are employed in drug delivery systems as desired therapeutic carriers. The following polymers are commonly used to make hydrogels: Natural (alginate, chitosan, gelatin, collagen and so on), or synthetic (polyethylene glycol, PEG), polylactic-co-glycolic acid, PLGA), poly (hydroxyethylmethacrylate, PHEMA), or the combination of both.
The hydrogel encapsulated exosomes through the following three methods: i) Mix the polymer with the exosomes, and then add a crosslinker to form 3D hydrogel in vitro, which benefits from optional properties such as mechanical strength and size when applied into the wound healing. Jiang et al. fabricated a matrix metalloproteinase (MMP)-response hydrogel by mixing PEG, maleimide, sulfhydryl and MMP to deliver ADSC-exos into wound sites (Jiang et al., 2022b). ii) The polymers and exosomes are firstly incorporated to the target sites, and followed by the addition of crosslinkers to form gels in vivo, which can be delivered by direct injection. Wang et al. (2019) constructed a hydrogel by Pluronic F127, poly-Ɛ-L-lysine and oxidative hyaluronic acid in vivo to deliver ADSC-derived exosomes intended for wound healing. For above two methods, the presence of crosslinker may cause cytotoxicity in vivo. iii) Immerse the dehydrated porous hydrogels in an exosome-containing solution, called as “breathing” technique. Dehydration of hydrogels can be achieved by lyophilization or solvents. By using this method, Xu et al. (2018) developed a hydrogel containing chitosan and silk fibroin to deliver platelet-rich-plasma-derived exosomes. This method presents less cytotoxicity and slowly releases exosomes.
To prolong the function time and the local concentration of exosomes in the wound bed, the scaffolds could slowly release exosomes depending on specific chemical or physical environmental stimuli, named “smart” hydrogels. A matrix metalloproteinase degradable polyethylene glycol (MMP-PEG) hydrogel was fabricated by Jiang et al. (2022b) to regulate the release of exosomes through reacting with MMP proteins. Zhao et al. (2020) developed a ROS-scavenging hydrogel, composed of polyvinyl alcohol (PVA) of a ROS-responsive linker, N1-(4-boronobenzyl)-N3-(4-boronophenyl)-N1, N1, N3, N3-tetramethylpropane-1, 3-diaminium (TPA) to promote wound closure by decreasing the ROS accumulation in the infective wound environment. The goal of both control and gradual release is to maintain the local exosomes or drugs at suitable concentration depending on the specialized micro-environment.
To assist the therapeutic effect of exosomes, the hydrogels are designed to benefit the wound healing, including increasing oxygen enrichment, accelerating cell proliferation, migration and angiogenesis formation, and improving the antibacterial properties. For example, Liu et al. fabricated a AMSC-exos-loaded β-chitin nanofiber (β-ChNF) hydrogel, which accelerated re-epithelialization and increased collagen expression in the rat full-thickness skin injury model (Liu et al., 2022). Parvaiz et al. loaded exosomes into an oxygen-releasing antioxidant scaffold, polyurethane-calcium peroxide cryogels, which controlled the continuous release of oxygen and exosomes for more than 10 days (Shiekh et al., 2018). Geng et al. (2022) reported a carboxyethyl chitosan-dialdehyde carboxymethyl cellulose (CEC-DCMC) hydrogel, which exhibited remarkable antibacterial characteristics against the further infection of diabetic wounds. Although the loading scaffolds indeed exhibit various excellent characteristics, adding some non-medical components into materials is inevitable, which delay their clinical transformation. So how to balance the efficiency and safety may be further evaluated.
The nanomaterials also are an ideal wound dressing considering good biocompatibility, low toxicity, colloidal stability and acceptable biodegradability, which can carry exosomes through the nanoscale properties.
Khalatbari et al. (2022) entrapped the alginate loaded with BMSCs-exos into silk fibroin to make a naturally-based polymers which had reasonable water vapor transfer rates and good swelling properties and excellent biocompatibility, making them suitable for wound dressing. A functional phosphoethanolamine phospholipid-grafted poly-L-lactic acid micro/nanofibers (DSPE-PLLA) was fabricated to carry and retain the slow release of exosomes from AMSCs, releasing the inflammatory response, promoting cell proliferation, angiogenesis and collagen deposition (Li et al., 2022). Compared to hydrogels, the polymers have more mechanical properties, an increase in diameter of scaffolds, which benefits to cell adhesion, proliferation and differentiation. In addition, proper vapor and oxygen permeability more easily balanced to the excretion of wound exudates, reducing the accumulation of bacteria and other infection factors. However, the polymers are generally combined with hydrogels, which are more conductive to the biological viability of exosomes. In addition, nanocomposites with nanomaterial-modified microfluidic channels are expected to achieve high capture rates and high throughput screening of exosomes in the future (Zou et al., 2023).
For systemic application of exosomes to enhance wound healing, we found Han et al. (2022) delivered BMSC-derived exosomal IncRNA KLF3-AS1 via tail vein injection. In addition, Zhou et al. systematically study the effect of different local ADSC-Exos smearing, injection and systemic intravenous administration for non-diabetic wound healing, and results showed that the combination of local smearing and intravenous administration could promote re-epithelialization, improve angiogenesis and collagen synthesis, achieving a fast wound healing process. Wounds associated with metabolic diseases, such as diabetes, may face more other systemic problems. The systemic application of exosomes may play a significant therapeutic role in systemic disease.
Skin wound healing is a complex multi-phase biological process. Stem cells have been verified a promising strategy in regenerative medicine. Exosomes, known as a main bioactive factor of MSC via paracrine activity are shown to have the same therapeutic effects as MSCs. And compared to MSCs, exosomes exhibit lower immune rejection and tumorigenesis risk in vivo (Zhang et al., 2014). However, many issues should be improved before applying exosomes into clinic.
Firstly, how to achieve a large-scale acquisition of exosomes from parental cells needs to be improved. At present, the more common separation and purification methods, such as ultracentrifugation, size exclusion chromatography, take a long time and complicated steps, resulting in low yield (Zou et al., 2023). Another challenge during the application is the absence of a standard manufacturing process to ensure the purification and clinical safety, limiting the clinical applications. In addition, storage and transport conditions of exosomes need to be addressed, which is studied by researchers to explore the ideal conditions (Levy et al., 2023).
Secondly, research on exosomes is mainly applied on animal models. Few clinical trials have been conducted to validate the effectiveness of exosomes on wound healing. A randomised clinical trial showed that human adipose stem cell-derived exosomes combined with carbon monoxide and laser treatment of acne significantly reduced scarring and erythema, initially confirming the efficacy and safety of exosomes in the clinic, so more clinical trials are needed in the future (Kwon et al., 2020). Moreover, the heterogeneity of exosomes from various sources and states necessitates further characterization to distinguish between subpopulations with distinct functions before utilized in clinical therapy (van Niel et al., 2018).
To improve the targeted therapeutic effects, exosomes can potentially be modified by directly engineering exosomes or modifying parental cells. However, engineered exosomes may face some of the shortcomings, such as low loading efficiency, the biosecurity of application. So it is necessary to rationally regulate the distribution, clearance and therapeutic efficacy of the cargo within exosomes at the wound bed. And how to maximize the therapeutic effect of engineered exosomes combined with materials and more efficient delivery methods needs to be further explore in future.
In conclusion, this review provides new insights into the role of exosomes in skin wound healing and the modification and delivery of exosomes. However, further research is still needed to explore the relevant mechanisms of exosomes in skin wound progression. And more researches are required to make engineered exosomes more accessible and feasible for clinical applications into wound healing.
YnS: Writing–original draft. SZ: Writing–original draft. YkS: Writing–original draft. HL: Writing–original draft. XZ: Writing–original draft. XW: Writing–review and editing. YW: Writing–review and editing. TW: Writing–review and editing. BL: Writing–review and editing, Supervision. LY: Writing–review and editing, Supervision. JW: Writing–original draft, Writing–review and editing, Supervision.
The author(s) declare that financial support was received for the research, authorship, and/or publication of this article. This study is supported by “Shandong Science and Technology Innovation Project on Medical and Health (No. 202304030716)” and “Shandong Science and Technology Innovation Project on traditional Chinese medicine (No. Q-2022146)” and The National College Students Innovation and Entrepreneurship Training Program of China (Grant Nos. 202310439100)” and “Tai’an city science and technology innovation development project (No.2023NS232).”
The authors declare that the research was conducted in the absence of any commercial or financial relationships that could be construed as a potential conflict of interest.
All claims expressed in this article are solely those of the authors and do not necessarily represent those of their affiliated organizations, or those of the publisher, the editors and the reviewers. Any product that may be evaluated in this article, or claim that may be made by its manufacturer, is not guaranteed or endorsed by the publisher.
EVs, extracellular vesicles; MVBs, multivesicular bodies; ILVs, intraluminal vesicles; ESCs, epidermal stem cells; MSCs, mesenchymal stem cells; MSC-exos, mesenchymal stem cell-derived exosomes; ADSCs, adipose-derived stem cells; BMSCs, bone marrow mesenchymal stem cells; HUVECs, human umbilical vein endothelial cells; UCMSCs, umbilical cord blood mesenchymal stem; SMSCs, Synovium - derived mesenchymal stem cells; UCBs, Umbilical cord blood mesenchymal stem cells; UMSCs, Human umbilical cord-derived mesenchymal stem cells.
Ai, Y., Guo, C., Garcia-Contreras, M., Sánchez, B. L., Saftics, A., Shodubi, O., et al. (2024). Endocytosis blocks the vesicular secretion of exosome marker proteins. Sci. Adv. 10 (19), eadi9156. doi:10.1126/sciadv.adi9156
An, Y., Lin, S., Tan, X., Zhu, S., Nie, F., Zhen, Y., et al. (2021). Exosomes from adipose-derived stem cells and application to skin wound healing. Cell Prolif. 54 (3), e12993. doi:10.1111/cpr.12993
Antimisiaris, S. G., Mourtas, S., and Marazioti, A. (2018). Exosomes and exosome-inspired vesicles for targeted drug delivery. Pharmaceutics 10 (4), 218. doi:10.3390/pharmaceutics10040218
Arosio, D., and Casagrande, C. (2016). Advancement in integrin facilitated drug delivery. Adv. Drug Deliv. Rev. 97, 111–143. doi:10.1016/j.addr.2015.12.001
Arya, S. B., Collie, S. P., and Parent, C. A. (2024). The ins-and-outs of exosome biogenesis, secretion, and internalization. Trends Cell Biol. 34 (2), 90–108. doi:10.1016/j.tcb.2023.06.006
Baru, O., Nutu, A., Braicu, C., Cismaru, C. A., Berindan-Neagoe, I., Buduru, S., et al. (2021). Angiogenesis in regenerative dentistry: are we far enough for therapy? Int. J. Mol. Sci. 22 (2), 929. doi:10.3390/ijms22020929
Ben Amar, M., and Wu, M. (2014). Re-epithelialization: advancing epithelium frontier during wound healing. J. R. Soc. Interface 11 (93), 20131038. doi:10.1098/rsif.2013.1038
Bian, D., Wu, Y., Song, G., Azizi, R., and Zamani, A. (2022). The application of mesenchymal stromal cells (MSCs) and their derivative exosome in skin wound healing: a comprehensive review. Stem Cell Res. Ther. 13 (1), 24. doi:10.1186/s13287-021-02697-9
Born, L. J., Chang, K. H., Shoureshi, P., Lay, F., Bengali, S., Hsu, A. T. W., et al. (2022). HOTAIR-loaded mesenchymal stem/stromal cell extracellular vesicles enhance angiogenesis and wound healing. Adv. Healthc. Mater 11 (5), e2002070. doi:10.1002/adhm.202002070
Chen, B., Sun, Y., Zhang, J., Zhu, Q., Yang, Y., Niu, X., et al. (2019). Human embryonic stem cell-derived exosomes promote pressure ulcer healing in aged mice by rejuvenating senescent endothelial cells. Stem Cell Res. Ther. 10 (1), 142. doi:10.1186/s13287-019-1253-6
Chen, G., Wu, Y., Zou, L., and Zeng, Y. (2023). Effect of MicroRNA-146a modified adipose-derived stem cell exosomes on rat back wound healing. Int. J. Low. Extrem Wounds 22 (4), 704–712. doi:10.1177/15347346211038092
Chen, G. Q., Wu, Y. L., Zou, L. J., and Zeng, Y. L. (2021a). Effect of MicroRNA-146a modified adipose-derived stem cell exosomes on rat back wound healing. Int. J. Low. Extrem. WOUNDS 22, 704–712. doi:10.1177/15347346211038092
Chen, L., Qin, L., Chen, C., Hu, Q., Wang, J., and Shen, J. (2021b). Serum exosomes accelerate diabetic wound healing by promoting angiogenesis and ECM formation. Cell Biol. Int. 45 (9), 1976–1985. doi:10.1002/cbin.11627
Chen, W., Huang, Y., Han, J., Yu, L., Li, Y., Lu, Z., et al. (2016). Immunomodulatory effects of mesenchymal stromal cells-derived exosome. Immunol. Res. 64 (4), 831–840. doi:10.1007/s12026-016-8798-6
Coumans, F. A. W., Brisson, A. R., Buzas, E. I., Dignat-George, F., Drees, E. E. E., El-Andaloussi, S., et al. (2017). Methodological guidelines to study extracellular vesicles. Circ. Res. 120 (10), 1632–1648. doi:10.1161/circresaha.117.309417
Curtale, G., Mirolo, M., Renzi, T. A., Rossato, M., Bazzoni, F., and Locati, M. (2013). Negative regulation of Toll-like receptor 4 signaling by IL-10-dependent microRNA-146b. Proc. Natl. Acad. Sci. U. S. A. 110 (28), 11499–11504. doi:10.1073/pnas.1219852110
Demidova-Rice, T. N., Durham, J. T., and Herman, I. M. (2012). Wound healing angiogenesis: innovations and challenges in acute and chronic wound healing. Adv. Wound Care 1 (1), 17–22. doi:10.1089/wound.2011.0308
Diegelmann, R. F., and Evans, M. C. (2004). Wound healing: an overview of acute, fibrotic and delayed healing. Front. Biosci. 9, 283–289. doi:10.2741/1184
Dong, J., Wu, B., and Tian, W. (2023). How to maximize the therapeutic effect of exosomes on skin wounds in diabetes mellitus: review and discussion. Front. Endocrinol. 14, 1146991. doi:10.3389/fendo.2023.1146991
Eming, S. A., Martin, P., and Tomic-Canic, M. (2014). Wound repair and regeneration: mechanisms, signaling, and translation. Sci. Transl. Med. 6 (265), 265sr6. doi:10.1126/scitranslmed.3009337
Fan, Y., Pionneau, C., Cocozza, F., Boëlle, P. Y., Chardonnet, S., Charrin, S., et al. (2023). Differential proteomics argues against a general role for CD9, CD81 or CD63 in the sorting of proteins into extracellular vesicles. J. Extracell. Vesicles 12 (8), e12352. doi:10.1002/jev2.12352
Fang, S., Xu, C., Zhang, Y., Xue, C., Yang, C., Bi, H., et al. (2016). Umbilical cord-derived mesenchymal stem cell-derived exosomal MicroRNAs suppress myofibroblast differentiation by inhibiting the transforming growth factor-β/SMAD2 pathway during wound healing. Stem Cells Transl. Med. 5 (10), 1425–1439. doi:10.5966/sctm.2015-0367
Fuhrmann, G., Serio, A., Mazo, M., Nair, R., and Stevens, M. M. (2015). Active loading into extracellular vesicles significantly improves the cellular uptake and photodynamic effect of porphyrins. J. Control Release 205, 35–44. doi:10.1016/j.jconrel.2014.11.029
Geng, X., Qi, Y., Liu, X., Shi, Y., Li, H., and Zhao, L. (2022). A multifunctional antibacterial and self-healing hydrogel laden with bone marrow mesenchymal stem cell-derived exosomes for accelerating diabetic wound healing. Biomater. Adv. 133, 112613. doi:10.1016/j.msec.2021.112613
Ghafourian, M., Mahdavi, R., Akbari Jonoush, Z., Sadeghi, M., Ghadiri, N., Farzaneh, M., et al. (2022). The implications of exosomes in pregnancy: emerging as new diagnostic markers and therapeutics targets. Cell Commun. Signal 20 (1), 51. doi:10.1186/s12964-022-00853-z
Golchin, A., Shams, F., Basiri, A., Ranjbarvan, P., Kiani, S., Sarkhosh-Inanlou, R., et al. (2022). Combination therapy of stem cell-derived exosomes and biomaterials in the wound healing. Stem Cell Rev. Rep. 18 (6), 1892–1911. doi:10.1007/s12015-021-10309-5
Guo, S. C., Tao, S. C., Yin, W. J., Qi, X., Yuan, T., and Zhang, C. Q. (2017). Exosomes derived from platelet-rich plasma promote the re-epithelization of chronic cutaneous wounds via activation of YAP in a diabetic rat model. Theranostics 7 (1), 81–96. doi:10.7150/thno.16803
Ha, D. H., Kim, H. K., Lee, J., Kwon, H. H., Park, G. H., Yang, S. H., et al. (2020). Mesenchymal stem/stromal cell-derived exosomes for immunomodulatory therapeutics and skin regeneration. Cells 9 (5), 1157. doi:10.3390/cells9051157
Hajipour, H., Farzadi, L., Roshangar, L., Latifi, Z., Kahroba, H., Shahnazi, V., et al. (2021). A human chorionic gonadotropin (hCG) delivery platform using engineered uterine exosomes to improve endometrial receptivity. Life Sci. 275, 119351. doi:10.1016/j.lfs.2021.119351
Han, X., Wu, P., Li, L., Sahal, H. M., Ji, C., Zhang, J., et al. (2021). Exosomes derived from autologous dermal fibroblasts promote diabetic cutaneous wound healing through the Akt/β-catenin pathway. Cell Cycle 20 (5-6), 616–629. doi:10.1080/15384101.2021.1894813
Han, Y., Ren, J., Bai, Y., Pei, X., and Han, Y. (2020). Corrigendum to “Exosomes from hypoxia-treated human adipose-derived mesenchymal stem cells enhance angiogenesis through VEGF/VEGF-R” [Int. J. Biochem. Cell Biol. 109 April (2019) 59-68]. Int. J. Biochem. Cell Biol. 126, 105805. doi:10.1016/j.biocel.2020.105805
Han, Z.-F., Cao, J.-H., Liu, Z.-Y., Yang, Z., Qi, R.-X., and Xu, H.-L. (2022). Exosomal lncRNA KLF3-AS1 derived from bone marrow mesenchymal stem cells stimulates angiogenesis to promote diabetic cutaneous wound healing. Diabetes Res. Clin. Pract. 183, 109126. doi:10.1016/j.diabres.2021.109126
Harrell, C. R., Fellabaum, C., Jovicic, N., Djonov, V., Arsenijevic, N., and Volarevic, V. (2019). Molecular mechanisms responsible for therapeutic potential of mesenchymal stem cell-derived secretome. Cells 8 (5), 467. doi:10.3390/cells8050467
He, L., Zhu, C., Jia, J., Hao, X. Y., Yu, X. Y., Liu, X. Y., et al. (2020). ADSC-Exos containing MALAT1 promotes wound healing by targeting miR-124 through activating Wnt/β-catenin pathway. Biosci. Rep. 40 (5). doi:10.1042/bsr20192549
Heo, J. S., Choi, Y., and Kim, H. O. (2019). Adipose-derived mesenchymal stem cells promote M2 macrophage phenotype through exosomes. Stem Cells Int. 2019, 1–10. doi:10.1155/2019/7921760
Ho, J., Yue, D., Cheema, U., Hsia, H. C., and Dardik, A. (2023). Innovations in stem cell therapy for diabetic wound healing. Adv. Wound Care (New Rochelle) 12 (11), 626–643. doi:10.1089/wound.2021.0104
Hoffman, M. (2018). The tissue factor pathway and wound healing. Semin. Thromb. Hemost. 44 (2), 142–150. doi:10.1055/s-0037-1606181
Hu, L., Wang, J., Zhou, X., Xiong, Z., Zhao, J., Yu, R., et al. (2016). Exosomes derived from human adipose mensenchymal stem cells accelerates cutaneous wound healing via optimizing the characteristics of fibroblasts. Sci. Rep. 6, 32993. doi:10.1038/srep32993
Hu, Y., Rao, S. S., Wang, Z. X., Cao, J., Tan, Y. J., Luo, J., et al. (2018). Exosomes from human umbilical cord blood accelerate cutaneous wound healing through miR-21-3p-mediated promotion of angiogenesis and fibroblast function. Theranostics 8 (1), 169–184. doi:10.7150/thno.21234
Jiang, T., Liu, S., Wu, Z., Li, Q., Ren, S., Chen, J., et al. (2022a). ADSC-exo@MMP-PEG smart hydrogel promotes diabetic wound healing by optimizing cellular functions and relieving oxidative stress. Mater Today Bio 16, 100365. doi:10.1016/j.mtbio.2022.100365
Jiang, T., Liu, S., Wu, Z., Li, Q., Ren, S., Chen, J., et al. (2022b). ADSC-exo@MMP-PEG smart hydrogel promotes diabetic wound healing by optimizing cellular functions and relieving oxidative stress. Mater. Today Bio 16, 100365. doi:10.1016/j.mtbio.2022.100365
Johnsen, K. B., Gudbergsson, J. M., Skov, M. N., Christiansen, G., Gurevich, L., Moos, T., et al. (2016). Evaluation of electroporation-induced adverse effects on adipose-derived stem cell exosomes. Cytotechnology 68 (5), 2125–2138. doi:10.1007/s10616-016-9952-7
Joseph, A., Baiju, I., Bhat, I. A., Pandey, S., Bharti, M., Verma, M., et al. (2020). Mesenchymal stem cell-conditioned media: a novel alternative of stem cell therapy for quality wound healing. J. Cell. Physiology 235 (7-8), 5555–5569. doi:10.1002/jcp.29486
Khalatbari, E., Tajabadi, M., and Khavandi, A. (2022). Multifunctional exosome-loaded silk fibroin/alginate structure for potential wound dressing application. Mater. Today Commun. 31, 103549. doi:10.1016/j.mtcomm.2022.103549
Khan, F. A., Pandupuspitasari, N. S., Chun-Jie, H., Ao, Z., Jamal, M., Zohaib, A., et al. (2016). CRISPR/Cas9 therapeutics: a cure for cancer and other genetic diseases. Oncotarget 7 (32), 52541–52552. doi:10.18632/oncotarget.9646
Khongkow, M., Yata, T., Boonrungsiman, S., Ruktanonchai, U. R., Graham, D., and Namdee, K. (2019). Surface modification of gold nanoparticles with neuron-targeted exosome for enhanced blood-brain barrier penetration. Sci. Rep. 9 (1), 8278. doi:10.1038/s41598-019-44569-6
Kim, M. S., Haney, M. J., Zhao, Y., Mahajan, V., Deygen, I., Klyachko, N. L., et al. (2016). Development of exosome-encapsulated paclitaxel to overcome MDR in cancer cells. Nanomedicine 12 (3), 655–664. doi:10.1016/j.nano.2015.10.012
Kwon, H. H., Yang, S. H., Lee, J., Park, B. C., Park, K. Y., Jung, J. Y., et al. (2020). Combination treatment with human adipose tissue stem cell-derived exosomes and fractional CO2 laser for acne scars: a 12-week prospective, double-blind, randomized, split-face study. Acta Derm. Venereol. 100 (18), adv00310. doi:10.2340/00015555-3666
Levy, D., Jeyaram, A., Born, L. J., Chang, K. H., Abadchi, S. N., Hsu, A. T. W., et al. (2023). Impact of storage conditions and duration on function of native and cargo-loaded mesenchymal stromal cell extracellular vesicles. Cytotherapy 25 (5), 502–509. doi:10.1016/j.jcyt.2022.11.006
Li, J., Yan, S., Han, W., Dong, Z., Li, J., Wu, Q., et al. (2022). Phospholipid-grafted PLLA electrospun micro/nanofibers immobilized with small extracellular vesicles from rat adipose mesenchymal stem cells promote wound healing in diabetic rats. Regen. Biomater. 9, rbac071. doi:10.1093/rb/rbac071
Li, M., Wang, T., Tian, H., Wei, G., Zhao, L., and Shi, Y. (2019). Macrophage-derived exosomes accelerate wound healing through their anti-inflammation effects in a diabetic rat model. Artif. Cells Nanomed Biotechnol. 47 (1), 3793–3803. doi:10.1080/21691401.2019.1669617
Li, P., Kaslan, M., Lee, S. H., Yao, J., and Gao, Z. (2017). Progress in exosome isolation techniques. Theranostics 7 (3), 789–804. doi:10.7150/thno.18133
Li, X., Liu, L., Yang, J., Yu, Y., Chai, J., Wang, L., et al. (2016). Exosome derived from human umbilical cord mesenchymal stem cell mediates MiR-181c attenuating burn-induced excessive inflammation. EBioMedicine 8, 72–82. doi:10.1016/j.ebiom.2016.04.030
Li, X., Xie, X., Lian, W., Shi, R., Han, S., Zhang, H., et al. (2018). Exosomes from adipose-derived stem cells overexpressing Nrf2 accelerate cutaneous wound healing by promoting vascularization in a diabetic foot ulcer rat model. Exp. Mol. Med. 50 (4), 1–14. doi:10.1038/s12276-018-0058-5
Li, Y., Zhang, J., Shi, J., Liu, K., Wang, X., Jia, Y., et al. (2021). Exosomes derived from human adipose mesenchymal stem cells attenuate hypertrophic scar fibrosis by miR-192-5p/IL-17RA/Smad axis. Stem Cell Res. Ther. 12 (1), 221. doi:10.1186/s13287-021-02290-0
Liang, Y., Duan, L., Lu, J., and Xia, J. (2021). Engineering exosomes for targeted drug delivery. Theranostics 11 (7), 3183–3195. doi:10.7150/thno.52570
Liang, Z. H., Pan, N. F., Lin, S. S., Qiu, Z. Y., Liang, P., Wang, J., et al. (2022). Exosomes from mmu_circ_0001052-modified adipose-derived stem cells promote angiogenesis of DFU via miR-106a-5p and FGF4/p38MAPK pathway. Stem Cell Res. Ther. 13 (1), 336. doi:10.1186/s13287-022-03015-7
Liu, J., Ren, L., Li, S., Li, W., Zheng, X., Yang, Y., et al. (2021). The biology, function, and applications of exosomes in cancer. Acta Pharm. Sin. B 11 (9), 2783–2797. doi:10.1016/j.apsb.2021.01.001
Liu, Y., Liu, Y., Zhao, Y., Wu, M., Mao, S., Cong, P., et al. (2022). Application of adipose mesenchymal stem cell-derived exosomes-loaded β-chitin nanofiber hydrogel for wound healing. Folia Histochem Cytobiol. 60 (2), 167–178. doi:10.5603/FHC.a2022.0015
Lo Sicco, C., Reverberi, D., Balbi, C., Ulivi, V., Principi, E., Pascucci, L., et al. (2017). Mesenchymal stem cell-derived extracellular vesicles as mediators of anti-inflammatory effects: endorsement of macrophage polarization. Stem Cells Transl. Med. 6 (3), 1018–1028. doi:10.1002/sctm.16-0363
Lu, S., Lu, L., Liu, Y., Li, Z., Fang, Y., Chen, Z., et al. (2022). Native and engineered extracellular vesicles for wound healing. Front. Bioeng. Biotechnol. 10, 1053217. doi:10.3389/fbioe.2022.1053217
Lu, Y., Mai, Z., Cui, L., and Zhao, X. (2023). Engineering exosomes and biomaterial-assisted exosomes as therapeutic carriers for bone regeneration. Stem Cell Res. Ther. 14 (1), 55. doi:10.1186/s13287-023-03275-x
Luan, X., Sansanaphongpricha, K., Myers, I., Chen, H., Yuan, H., and Sun, D. (2017). Engineering exosomes as refined biological nanoplatforms for drug delivery. Acta Pharmacol. Sin. 38 (6), 754–763. doi:10.1038/aps.2017.12
Lv, Q., Deng, J., Chen, Y., Wang, Y., Liu, B., and Liu, J. (2020). Engineered human adipose stem-cell-derived exosomes loaded with miR-21-5p to promote diabetic cutaneous wound healing. Mol. Pharm. 17 (5), 1723–1733. doi:10.1021/acs.molpharmaceut.0c00177
Ma, T., Fu, B., Yang, X., Xiao, Y., and Pan, M. (2019). Adipose mesenchymal stem cell-derived exosomes promote cell proliferation, migration, and inhibit cell apoptosis via Wnt/β-catenin signaling in cutaneous wound healing. J. Cell Biochem. 120 (6), 10847–10854. doi:10.1002/jcb.28376
Matsuoka, T., and Yashiro, M. (2015). Recent advances in the HER2 targeted therapy of gastric cancer. World J. Clin. Cases 3 (1), 42–51. doi:10.12998/wjcc.v3.i1.42
Meldolesi, J. (2018). Exosomes and ectosomes in intercellular communication. Curr. Biol. 28 (8), R435–r444. doi:10.1016/j.cub.2018.01.059
Mondal, J., Pillarisetti, S., Junnuthula, V., Saha, M., Hwang, S. R., Park, I. K., et al. (2023). Hybrid exosomes, exosome-like nanovesicles and engineered exosomes for therapeutic applications. J. Control Release 353, 1127–1149. doi:10.1016/j.jconrel.2022.12.027
Oliveira, A. G., Araújo, T. G., Carvalho, B. D., Rocha, G. Z., Santos, A., and Saad, M. J. A. (2018). The role of hepatocyte growth factor (HGF) in insulin resistance and diabetes. Front. Endocrinol. 9, 503. doi:10.3389/fendo.2018.00503
Pomatto, M., Gai, C., Negro, F., Cedrino, M., Grange, C., Ceccotti, E., et al. (2021). Differential therapeutic effect of extracellular vesicles derived by bone marrow and adipose mesenchymal stem cells on wound healing of diabetic ulcers and correlation to their cargoes. Int. J. Mol. Sci. 22 (8), 3851. doi:10.3390/ijms22083851
Qian, X., An, N., Ren, Y., Yang, C., Zhang, X., and Li, L. (2021). Immunosuppressive effects of mesenchymal stem cells-derived exosomes. Stem Cell Rev. Rep. 17 (2), 411–427. doi:10.1007/s12015-020-10040-7
Raziyeva, K., Kim, Y., Zharkinbekov, Z., Kassymbek, K., Jimi, S., and Saparov, A. (2021). Immunology of acute and chronic wound healing. Biomolecules 11 (5), 700. doi:10.3390/biom11050700
Ruivo, C. F., Bastos, N., Adem, B., Batista, I., Duraes, C., Melo, C. A., et al. (2022). Extracellular vesicles from pancreatic cancer stem cells lead an intratumor communication network (EVNet) to fuel tumour progression. Gut 71 (10), 2043–2068. doi:10.1136/gutjnl-2021-324994
Sadeghi, S., Tehrani, F. R., Tahmasebi, S., Shafiee, A., and Hashemi, S. M. (2023). Exosome engineering in cell therapy and drug delivery. Inflammopharmacology 31 (1), 145–169. doi:10.1007/s10787-022-01115-7
Shah, A., and Amini-Nik, S. (2017). The role of phytochemicals in the inflammatory phase of wound healing. Int. J. Mol. Sci. 18 (5), 1068. doi:10.3390/ijms18051068
Shi, R., Jin, Y., Zhao, S., Yuan, H., Shi, J., and Zhao, H. (2022). Hypoxic ADSC-derived exosomes enhance wound healing in diabetic mice via delivery of circ-Snhg11 and induction of M2-like macrophage polarization. Biomed. Pharmacother. 153, 113463. doi:10.1016/j.biopha.2022.113463
Shiekh, P. A., Singh, A., and Kumar, A. (2018). Oxygen-releasing antioxidant cryogel scaffolds with sustained oxygen delivery for tissue engineering applications. ACS Appl. Mater Interfaces 10 (22), 18458–18469. doi:10.1021/acsami.8b01736
Shpichka, A., Butnaru, D., Bezrukov, E. A., Sukhanov, R. B., Atala, A., Burdukovskii, V., et al. (2019). Skin tissue regeneration for burn injury. Stem Cell Res. Ther. 10 (1), 94. doi:10.1186/s13287-019-1203-3
Smyth, T., Petrova, K., Payton, N. M., Persaud, I., Redzic, J. S., Graner, M. W., et al. (2014). Surface functionalization of exosomes using click chemistry. Bioconjug Chem. 25 (10), 1777–1784. doi:10.1021/bc500291r
Song, L., Tang, S., Han, X., Jiang, Z., Dong, L., Liu, C., et al. (2019). KIBRA controls exosome secretion via inhibiting the proteasomal degradation of Rab27a. Nat. Commun. 10 (1), 1639. doi:10.1038/s41467-019-09720-x
Tao, S. C., Guo, S. C., Li, M., Ke, Q. F., Guo, Y. P., and Zhang, C. Q. (2017). Chitosan wound dressings incorporating exosomes derived from MicroRNA-126-overexpressing synovium mesenchymal stem cells provide sustained release of exosomes and heal full-thickness skin defects in a diabetic rat model. STEM CELLS Transl. Med. 6 (3), 736–747. doi:10.5966/sctm.2016-0275
Tao, Y., Zhou, J., Wang, Z., Tao, H., Bai, J., Ge, G., et al. (2021). Human bone mesenchymal stem cells-derived exosomal miRNA-361-5p alleviates osteoarthritis by downregulating DDX20 and inactivating the NF-κB signaling pathway. Bioorg Chem. 113, 104978. doi:10.1016/j.bioorg.2021.104978
Tkach, M., and Théry, C. (2016). Communication by extracellular vesicles: where we are and where we need to go. Cell 164 (6), 1226–1232. doi:10.1016/j.cell.2016.01.043
Van Deun, J., Roux, Q., Deville, S., Van Acker, T., Rappu, P., Miinalainen, I., et al. (2020). Feasibility of mechanical extrusion to coat nanoparticles with extracellular vesicle membranes. Cells 9 (8), 1797. doi:10.3390/cells9081797
van Niel, G., D’Angelo, G., and Raposo, G. (2018). Shedding light on the cell biology of extracellular vesicles. Nat. Rev. Mol. Cell Biol. 19 (4), 213–228. doi:10.1038/nrm.2017.125
Villata, S., Canta, M., and Cauda, V. (2020). EVs and bioengineering: from cellular products to engineered nanomachines. Int. J. Mol. Sci. 21 (17), 6048. doi:10.3390/ijms21176048
Wang, C., Wang, M., Xu, T., Zhang, X., Lin, C., Gao, W., et al. (2019). Engineering bioactive self-healing antibacterial exosomes hydrogel for promoting chronic diabetic wound healing and complete skin regeneration. Theranostics 9 (1), 65–76. doi:10.7150/thno.29766
Wang, J., Wu, H., Peng, Y., Zhao, Y., Qin, Y., Zhang, Y., et al. (2021). Hypoxia adipose stem cell-derived exosomes promote high-quality healing of diabetic wound involves activation of PI3K/Akt pathways. J. Nanobiotechnology 19 (1), 202. doi:10.1186/s12951-021-00942-0
Wang, L., Cai, Y., Zhang, Q., and Zhang, Y. (2022). Pharmaceutical activation of Nrf2 accelerates diabetic wound healing by exosomes from bone marrow mesenchymal stem cells. Int. J. Stem Cells 15 (2), 164–172. doi:10.15283/ijsc21067
Wang, L., Hu, L., Zhou, X., Xiong, Z., Zhang, C., Shehada, H. M. A., et al. (2017). Exosomes secreted by human adipose mesenchymal stem cells promote scarless cutaneous repair by regulating extracellular matrix remodelling. Sci. Rep. 7 (1), 13321. doi:10.1038/s41598-017-12919-x
Whiteside, T. L. (2018). Exosome and mesenchymal stem cell cross-talk in the tumor microenvironment. Semin. Immunol. 35, 69–79. doi:10.1016/j.smim.2017.12.003
Wilkinson, H. N., and Hardman, M. J. (2020). Wound healing: cellular mechanisms and pathological outcomes. Open Biol. 10 (9), 200223. doi:10.1098/rsob.200223
Wu, D., Kang, L., Tian, J., Wu, Y., Liu, J., Li, Z., et al. (2020a). <p>Exosomes Derived from Bone Mesenchymal Stem Cells with the Stimulation of Fe<sub>3</sub>O<sub>4</sub> Nanoparticles and Static Magnetic Field Enhance Wound Healing through Upregulated miR-21-5p</p>. Int. J. Nanomedicine 15, 7979–7993. doi:10.2147/ijn.S275650
Wu, D., Kang, L., Tian, J. J., Wu, Y. H., Liu, J. Y., Li, Z. Y., et al. (2020b). Exosomes derived from bone mesenchymal stem cells with the stimulation of Fe3O4 nanoparticles and static magnetic field enhance wound healing through upregulated miR-21-5p. Int. J. NANOMEDICINE 15, 7979–7993. doi:10.2147/IJN.S275650
Xu, J., Bai, S., Cao, Y., Liu, L., Fang, Y., Du, J., et al. (2020). miRNA-221-3p in endothelial progenitor cell-derived exosomes accelerates skin wound healing in diabetic mice. Diabetes Metab. Syndr. Obes. 13, 1259–1270. doi:10.2147/dmso.S243549
Xu, N., Wang, L., Guan, J., Tang, C., He, N., Zhang, W., et al. (2018). Wound healing effects of a Curcuma zedoaria polysaccharide with platelet-rich plasma exosomes assembled on chitosan/silk hydrogel sponge in a diabetic rat model. Int. J. Biol. Macromol. 117, 102–107. doi:10.1016/j.ijbiomac.2018.05.066
Xue, C., Shen, Y., Li, X., Li, B., Zhao, S., Gu, J., et al. (2018). Exosomes derived from hypoxia-treated human adipose mesenchymal stem cells enhance angiogenesis through the PKA signaling pathway. Stem Cells Dev. 27 (7), 456–465. doi:10.1089/scd.2017.0296
Yan, C., Chen, J., Wang, C., Yuan, M., Kang, Y., Wu, Z., et al. (2022a). Milk exosomes-mediated miR-31-5p delivery accelerates diabetic wound healing through promoting angiogenesis. Drug Deliv. 29 (1), 214–228. doi:10.1080/10717544.2021.2023699
Yan, C., Xv, Y., Lin, Z., Endo, Y., Xue, H., Hu, Y., et al. (2022b). Human umbilical cord mesenchymal stem cell-derived exosomes accelerate diabetic wound healing via ameliorating oxidative stress and promoting angiogenesis. Front. Bioeng. Biotechnol. 10, 829868. doi:10.3389/fbioe.2022.829868
Yan, L. T., He, X. X., Tang, Y. H., Zhao, X. M., Luo, G., and Wang, X. H. (2021). HGF can reduce accumulation of inflammation and regulate glucose homeostasis in T2D mice. J. PHYSIOLOGY Biochem. 77 (4), 613–624. doi:10.1007/s13105-021-00828-7
Yang, J., Chen, Z., Pan, D., Li, H., and Shen, J. (2020). Umbilical cord-derived mesenchymal stem cell-derived exosomes combined pluronic F127 hydrogel promote chronic diabetic wound healing and complete skin regeneration. Int. J. Nanomedicine 15, 5911–5926. doi:10.2147/ijn.S249129
Yang, J., Liu, X. X., Fan, H., Tang, Q., Shou, Z. X., Zuo, D. M., et al. (2015). Extracellular vesicles derived from bone marrow mesenchymal stem cells protect against experimental colitis via attenuating colon inflammation, oxidative stress and apoptosis. PLoS One 10 (10), e0140551. doi:10.1371/journal.pone.0140551
Yang, Y., Zhao, Y. L., Zhang, L. J., Zhang, F., and Li, L. J. (2021). The application of mesenchymal stem cells in the treatment of liver diseases: mechanism, efficacy, and safety issues. Front. Med. 8, 655268. doi:10.3389/fmed.2021.655268
Yu, M., Liu, W., Li, J., Lu, J., Lu, H., Jia, W., et al. (2020). Exosomes derived from atorvastatin-pretreated MSC accelerate diabetic wound repair by enhancing angiogenesis via AKT/eNOS pathway. Stem Cell Res. Ther. 11 (1), 350. doi:10.1186/s13287-020-01824-2
Zhang, B., Wang, M., Gong, A., Zhang, X., Wu, X., Zhu, Y., et al. (2015). HucMSC-exosome mediated-wnt4 signaling is required for cutaneous wound healing. Stem Cells 33 (7), 2158–2168. doi:10.1002/stem.1771
Zhang, B., Yin, Y., Lai, R. C., Tan, S. S., Choo, A. B., and Lim, S. K. (2014). Mesenchymal stem cells secrete immunologically active exosomes. Stem Cells Dev. 23 (11), 1233–1244. doi:10.1089/scd.2013.0479
Zhang, H. Y., Wang, Y., Bai, M., Wang, J. Y., Zhu, K. G., Liu, R., et al. (2018). Exosomes serve as nanoparticles to suppress tumor growth and angiogenesis in gastric cancer by delivering hepatocyte growth factor siRNA. CANCER Sci. 109 (3), 629–641. doi:10.1111/cas.13488
Zhang, X., Wang, W., Wang, Y., Zhao, H., Han, X., Zhao, T., et al. (2020). Extracellular vesicle-encapsulated miR-29b-3p released from bone marrow-derived mesenchymal stem cells underpins osteogenic differentiation. Front. Cell Dev. Biol. 8, 581545. doi:10.3389/fcell.2020.581545
Zhao, B., Zhang, X., Zhang, Y., Lu, Y., Zhang, W., Lu, S., et al. (2021). Human exosomes accelerate cutaneous wound healing by promoting collagen synthesis in a diabetic mouse model. Stem Cells Dev. 30 (18), 922–933. doi:10.1089/scd.2021.0100
Zhao, H., Huang, J., Li, Y., Lv, X., Zhou, H., Wang, H., et al. (2020). ROS-scavenging hydrogel to promote healing of bacteria infected diabetic wounds. Biomaterials 258, 120286. doi:10.1016/j.biomaterials.2020.120286
Zhao, H., Li, Z., Wang, Y., Zhou, K., Li, H., Bi, S., et al. (2023). Bioengineered MSC-derived exosomes in skin wound repair and regeneration. Front. Cell Dev. Biol. 11, 1029671. doi:10.3389/fcell.2023.1029671
Zhao, Y., Ye, W. L., Wang, Y. D., and Chen, W. D. (2022). HGF/c-met: a key promoter in liver regeneration. Front. Pharmacol. 13, 808855. doi:10.3389/fphar.2022.808855
Zhou, C., Zhang, Y., Yan, R., Huang, L., Mellor, A. L., Yang, Y., et al. (2021). Exosome-derived miR-142-5p remodels lymphatic vessels and induces Ido to promote immune privilege in the tumour microenvironment. Cell Death Differ. 28 (2), 715–729. doi:10.1038/s41418-020-00618-6
Zhou, X., Brown, B. A., Siegel, A. P., El Masry, M. S., Zeng, X., Song, W., et al. (2020). Exosome-Mediated crosstalk between keratinocytes and macrophages in cutaneous wound healing. ACS Nano 14 (10), 12732–12748. doi:10.1021/acsnano.0c03064
Zhu, J., Li, F., Wang, X., Yu, J., and Wu, D. (2018). Hyaluronic acid and polyethylene glycol hybrid hydrogel encapsulating nanogel with hemostasis and sustainable antibacterial property for wound healing. ACS Appl. Mater. Interfaces 10 (16), 13304–13316. doi:10.1021/acsami.7b18927
Zhuang, Z., Liu, M., Luo, J., Zhang, X., Dai, Z., Zhang, B., et al. (2022). Exosomes derived from bone marrow mesenchymal stem cells attenuate neurological damage in traumatic brain injury by alleviating glutamate-mediated excitotoxicity. Exp. Neurol. 357, 114182. doi:10.1016/j.expneurol.2022.114182
Zou, J., Yang, W., Cui, W., Li, C., Ma, C., Ji, X., et al. (2023a). Therapeutic potential and mechanisms of mesenchymal stem cell-derived exosomes as bioactive materials in tendon-bone healing. J. Nanobiotechnology 21 (1), 14. doi:10.1186/s12951-023-01778-6
Keywords: skin wound healing, exosoems, bioengeneering, hydrogel, nanofiber
Citation: Sun Y, Zhang S, Shen Y, Lu H, Zhao X, Wang X, Wang Y, Wang T, Liu B, Yao L and Wen J (2024) Therapeutic application of mesenchymal stem cell-derived exosomes in skin wound healing. Front. Bioeng. Biotechnol. 12:1428793. doi: 10.3389/fbioe.2024.1428793
Received: 08 May 2024; Accepted: 25 July 2024;
Published: 05 August 2024.
Edited by:
Chiara Gentili, University of Genoa, ItalyReviewed by:
Xin Xing, Johns Hopkins University, United StatesCopyright © 2024 Sun, Zhang, Shen, Lu, Zhao, Wang, Wang, Wang, Liu, Yao and Wen. This is an open-access article distributed under the terms of the Creative Commons Attribution License (CC BY). The use, distribution or reproduction in other forums is permitted, provided the original author(s) and the copyright owner(s) are credited and that the original publication in this journal is cited, in accordance with accepted academic practice. No use, distribution or reproduction is permitted which does not comply with these terms.
*Correspondence: Bing Liu, bGl1YmluZ0BzZGZtdS5lZHUuY24=; Lan Yao, NDAyMDcwOTY1QHFxLmNvbQ==; Jie Wen, d2VuamllMTk4OTExQDE2My5jb20=
†These authors have contributed equally to this work
Disclaimer: All claims expressed in this article are solely those of the authors and do not necessarily represent those of their affiliated organizations, or those of the publisher, the editors and the reviewers. Any product that may be evaluated in this article or claim that may be made by its manufacturer is not guaranteed or endorsed by the publisher.
Research integrity at Frontiers
Learn more about the work of our research integrity team to safeguard the quality of each article we publish.