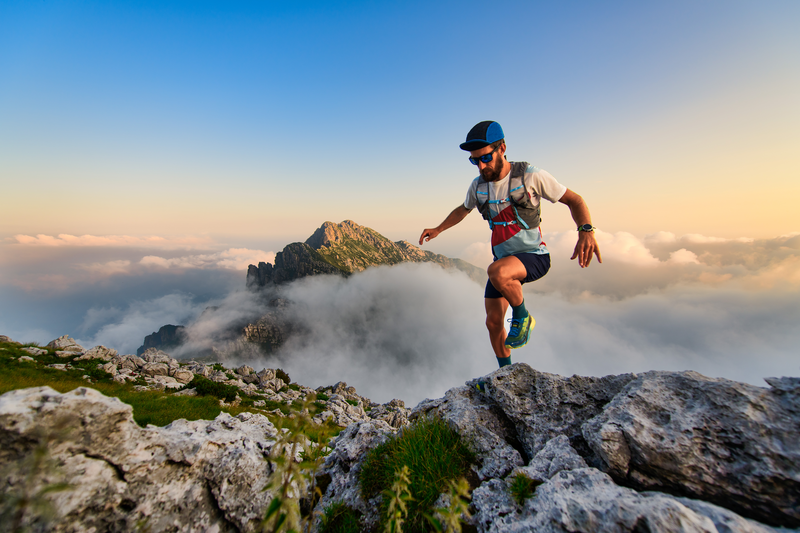
94% of researchers rate our articles as excellent or good
Learn more about the work of our research integrity team to safeguard the quality of each article we publish.
Find out more
REVIEW article
Front. Bioeng. Biotechnol. , 26 August 2024
Sec. Tissue Engineering and Regenerative Medicine
Volume 12 - 2024 | https://doi.org/10.3389/fbioe.2024.1417600
This review aimed to summarize the recent advances and challenges in the field of regenerative therapies for lumbar disc degeneration. The current first-line treatment options for symptomatic lumbar disc degeneration cannot modify the disease process or restore the normal structure, composition, and biomechanical function of the degenerated discs. Cell-based therapies tailored to facilitate intervertebral disc (IVD) regeneration have been developed to restore the IVD extracellular matrix or mitigate inflammatory conditions. Human clinical trials on Mesenchymal Stem Cells (MSCs) have reported promising outcomes exhibited by MSCs in reducing pain and improving function. Nucleus pulposus (NP) cells possess unique regenerative capacities. Biomaterials aimed at NP replacement in IVD regeneration, comprising synthetic and biological materials, aim to restore disc height and segmental stability without compromising the annulus fibrosus. Similarly, composite IVD replacements that combine various biomaterial strategies to mimic the native disc structure, including organized annulus fibrosus and NP components, have shown promise. Furthermore, preclinical studies on regenerative medicine therapies that utilize cells, biomaterials, growth factors, platelet-rich plasma (PRP), and biological agents have demonstrated their promise in repairing degenerated lumbar discs. However, these therapies are associated with significant limitations and challenges that hinder their clinical translation. Thus, further studies must be conducted to address these challenges.
Lumbar degenerative disc disease (LDDD), an age-associated disease characterized by severe back pain and disability (Ohtori et al., 2015), is highly prevalent across the world. The pathogenesis of LDDD involves age-related (Ohnishi et al., 2018) or injury-induced (Wang et al., 2023) degeneration of the intervertebral discs (IVDs). Decreased hydration, reduced proteoglycan content, loss of disc height, annular fissuring, and ingrowth of nerve and blood vessels (Fujii et al., 2019) are observed in degenerating discs. These changes can lead to structural breakdown, biomechanical dysfunction, instability, herniation, and nerve compression. Current treatment options, such as medications (Chaparro et al., 2014), physical therapy (Hayden et al., 2021), cognitive functional therapy (Kent et al., 2023), epidural injections (Deyo and Mirza, 2016), and spinal fusion procedures (Otsuki et al., 2023; Shimizu et al., 2021), only provide temporary symptomatic relief. A treatment strategy that can restore normal disc structure, composition, and function remains to be established. Tissue engineering aims to repair and regenerate discs using cells, biomaterials, growth factors, and platelet-rich plasma (PRP). This literature review summarized the recent advances and challenges in the field of regenerative therapies for lumbar disc degeneration.
IVDs comprise a central gelatinous nucleus pulposus surrounded by the annulus fibrosus and cartilaginous endplates. The proteoglycan-rich nucleus is capable of absorbing water and resisting compressive load. The collagen fibers constituting the highly organized lamellar annulus fibrosus provide tensile strength (Sakai and Andersson, 2015). The proteoglycan and water content of the discs reduces with aging and injury. In addition, further changes, such as disorganized matrix; loss of collagen organization; ingrowth of nerves and vessels; decreased cell viability; and increased inflammatory cytokine (IL-1β, IL-6, and TNF-α), C-reactive protein levels, and type II collagen levels, have also been reported (Khan et al., 2017; de Queiroz et al., 2016; Stürmer et al., 2005; Wang et al., 2010; Goode et al., 2012) (Figure 1). These molecular changes result in structural breakdown, reduction in disc height, annular fissuring, radial bulging, altered biomechanics, nerve compression, instability, herniation, and lower back pain.
Figure 1. The scheme summarizing the modalities of the regenerative therapies and the pathological conditions of the intervertebral disc degeneration.
The first-line treatment options for symptomatic LDDD include the administration of medications, such as non-steroidal anti-inflammatory drugs, muscle relaxants, and opioids, to alleviate pain (Chaparro et al., 2014; Deyo and Mirza, 2016). Physical therapy aims to strengthen core muscles, thereby improving ergonomics (Hayden et al., 2021). Short-term pain relief can be achieved with epidural steroid injections and intradiscal electrothermal therapy (Deyo and Mirza, 2016). Spinal fusion procedures using cages and screws have been performed to stabilize the affected segments in severe cases; however, this can result in increased stress on adjacent segments (Otsuki et al., 2023; Shimizu et al., 2021). Discectomy is performed to remove herniated nucleus material compressing the nerves (Özer and Demirtaş, 2023; Goparaju et al., 2023). Disc arthroplasty involves the replacement of degenerated discs with artificial implants (van den Eerenbeemt et al., 2010; Siepe et al., 2014; Gornet et al., 2017; Park et al., 2018). Nevertheless, the inability of these approaches to modify the disease process or restore the normal structure, composition, and biomechanical function of the degenerated discs underscores the requirement for developing biological therapies that facilitate disc regeneration.
Cell therapy is an innovative treatment approach that involves infusing living cells into a patient to repair or replace damaged tissue or modify the behavior of local cells (Sakai and Andersson, 2015). Cell-based therapies tailored to facilitate IVD regeneration in patients with IVD degeneration have been developed to restore the IVD extracellular matrix (ECM) or mitigate the inflammatory conditions that are characteristic of disc deterioration (Fujii et al., 2019; Sakai et al., 2022). Measures should be taken to ensure that the transplanted cells thrive within the hostile environment of a degenerating IVD by directly contributing to ECM synthesis and inducing a reparative shift in native cell activity via paracrine signaling or by facilitating the arrival of regenerative cells and preventing the infiltration of harmful cells. This would help achieve successful treatment outcomes. Animal studies have demonstrated the potential of cell transplantation to decelerate or halt degenerative processes in some cases (Hiraishi et al., 2018; Nukaga et al., 2019). Minimally invasive procedures, such as a needle injection under image guidance, are used to introduce the cells into the IVD. The cells can be encapsulated in supportive matrices to enhance retention and functionality.
Mesenchymal stem cells (MSCs) have attracted considerable interest in recent years. MSCs derived from the bone marrow (Orozco et al., 2011; Elabd et al., 2016; Henriksson et al., 2019; Noriega et al., 2017; Amirdelfan et al., 2021), adipose tissue (Kumar et al., 2017), peripheral blood (Haufe and Mork, 2006), or umbilical cords (Pang et al., 2014; Lewandrowski et al., 2023) exhibit potential for chondrogenic differentiation and high proliferative ability. Furthermore, they can be harvested easily from autologous and allogeneic sources. MSCs exhibit remarkable versatility, including the capacity for immunomodulation. This property could be beneficial in tempering the inflammatory environment of the IVD (Weiss and Dahlke, 2019). Environmental stressors within the IVD can affect the proliferation and differentiation capabilities of MSCs; thus, the adaptability and survival of MSCs within IVDs require further study (Gay et al., 2019).
Human clinical trials have predominantly focused on MSCs owing to the promising outcomes exhibited by MSCs in reducing pain and improving function in patients with IVD degeneration (Table 1). Trials using adipose- and bone marrow-derived MSCs have reported improvement in pain and disability scores (Orozco et al., 2011; Elabd et al., 2016; Henriksson et al., 2019; Noriega et al., 2017; Amirdelfan et al., 2021; Kumar et al., 2017; Pang et al., 2014; Lewandrowski et al., 2023). Moreover, the safety profiles of MSCs are generally favorable, and the incidence of serious adverse events is rare (Amirdelfan et al., 2021), underscoring the potential viability of MSC therapies.
Studies on other types of cells, such as the nucleus pulposus (NP) cells (Mochida et al., 2015; Beall et al., 2021) and chondrocytes from articular or hyaline cartilage (Coric et al., 2013; Tschugg et al., 2017), have elucidated the unique regenerative capacities inherent to cells native to avascular tissues, such as the IVD. However, the limitations associated with accessibility and phenotypic stability limit the widespread application of these treatment strategies. Clinical application of these cells, although limited, suggests their safety and potential efficacy in improving the status of the IVD and patient outcomes.
The treatment outcomes of emerging therapies utilizing less-defined cell products, such as stromal vascular fraction (SVF) (Comella et al., 2017) and bone marrow concentrate (BMC) (Pettine et al., 2015), vary. These complex mixtures, which comprise various types of cells and bioactive factors, offer a multifaceted approach to tissue regeneration. The precise mechanisms of action and individual contributions of these components are unclear; however, early results suggest a potential for facilitating significant clinical improvement in certain cases (Comella et al., 2017; Pettine et al., 2015). Nevertheless, the heterogeneity of these products hinders standardization and quality control.
Animal experiments have used iPS cells as a cell source and induced differentiation into notochordal cells (Sheyn et al., 2019), nucleus pulposus-like cells (Zhang et al., 2020), or cartilaginous tissue (Kamatani et al., 2022). These cells were transplanted into IVDs subsequently. Their application in human beings is anticipated in the future.
Biomaterials aimed at NP replacement in IVD regeneration, comprising synthetic and biological materials designed to restore disc height and segmental stability without compromising the annulus fibrosus, are predominantly injectable materials (Iatridis et al., 2013; Mehrkens et al., 2012). The properties of synthetic materials must match the mechanical properties of the native disc to achieve successful outcomes. This ensures the integration of the material with the surrounding structures and the restoration of the motion characteristics without inducing adverse immune responses. Furthermore, durability and minimal wear-debris generation are critical factors affecting clinical viability (Bowles and Setton, 2017; Li et al., 2022).
Biologically based materials can be remodeled by the body. Consequently, a different set of criteria, primarily focusing on the ability to support cell-mediated tissue regeneration, must be satisfied by these materials to achieve successful outcomes. This category comprises materials that serve as cell-delivery vehicles to promote NP tissue regeneration (Bowles and Setton, 2017; Li et al., 2022).
Initial strategies for NP replacement involved the administration of synthetic polymers that hydrate in situ to mimic the natural hydration of NP. This approach aimed to restore disc pressure and height. Copolymeric hydrogel (polyacrylonitrile [PAN] and polyacrylamide) encased in a polyethylene jacket (PDNTM) is one such combination (Ray, 2002). Other materials, such as polyvinyl alcohol (PVA) and polyvinyl pyrrolidone (PVP) reinforced with a Dacron mesh (NeuDisc™) (Bertagnoli et al., 2005),hydrating PAN (NucleoFix™/Gelstix™) (Bowles and Setton, 2017; Ceylan et al., 2019; Koetsier et al., 2022), in situ curing polymer using inflatable polyurethane balloon (DASCOR™) (Tsantrizos et al., 2008; Ahrens et al., 2009), in situ curing polymerized water-in-oil emulsion composite (DiscCell™) (Pelletier et al., 2016), glutaraldehyde cross-linked elastin and silk polypeptide (BioDisc™) (Yuksel et al., 2002), and hydrogel of a chemically cross-linked elastin and silk polypeptide (NuCore™) (Berlemann and Schwarzenbach, 2009) have also been used. However, these materials are associated with significant limitations such as uncontrolled swelling, mechanical complications (e.g., stiffness), and device migration. Clinical trials have explored various materials, such as hydrogels and polymers (Ceylan et al., 2019; Koetsier et al., 2022; Ahrens et al., 2009; Berlemann and Schwarzenbach, 2009), that can transition into a gel or solid state in situ, to minimize the damage to the annulus fibrosus during implantation. Ceylan et al. (2019) reported GelStixTM demonstrated mean VAS score and ODI score improvement after implantation. Moreover, RCT is being conducted (NCT 02763956) (Koetsier et al., 2022). However, progress in the domain of market approval has been limited (Bowles and Setton, 2017) (Table 2) owing to the focus on mechanical restoration rather than biological integration or interaction with local cells.
Engineering complete IVD replacements using materials that facilitate cellular survival and matrix remodeling has garnered interest in recent years, indicating a trend toward the use of more biologically integrated solutions. Composite IVD replacements that combine various biomaterial strategies to mimic the native disc structure, including organized annulus fibrosus and NP components, have shown promise (Mizuno et al., 2006; Sakai et al., 2006; Nesti et al., 2008; Nerurkar et al., 2010; Zhuang et al., 2011; Bowles et al., 2011; Park et al., 2012; Martin et al., 2014; Choy and Chan, 2015; Chik et al., 2015; Ukeba et al., 2021) (Table 3). This strategy aims to replicate the appearance and mechanical functions of the native disc; however, it has exhibited varying degrees of success in mimicking the mechanical properties and achieving integration with the native tissue.
Challenges related to the management of the disc space, integration with the native tissue, and the mechanical environment of the spine have been reported by in vivo animal studies on composite discs. These findings emphasize the requirement for conducting further research to address these limitations that hinder successful clinical translation (Mizuno et al., 2006; Sakai et al., 2006; Zhuang et al., 2011; Bowles et al., 2011; Martin et al., 2014; Ukeba et al., 2021). Techniques to engineer motion segments, including the disc and adjacent bony structures, aim to overcome the challenges associated with integration and improve patient outcomes.
Although promising, the field of IVD regeneration through NP replacement is associated with several limitations associated with material design, biological integration, and mechanical performance. Thus, further studies must be conducted in the future to better understand and overcome these obstacles for successful clinical application.
Growth factors, such as TGF-β (Sun et al., 2023; Risbud et al., 2006); GDF-5,6 (Gantenbein-Ritter et al., 2011; Clarke et al., 2014); FGF-2 (Tsai et al., 2007); IGF-1 (Osada et al., 1996); BMP-2, 4, and 7 (Tim et al., 2003; Du et al., 2022; Ellman et al., 2013); and CTGF (Matta et al., 2018), have been evaluated in previous studies (Table 4) These factors can stimulate matrix synthesis, cell proliferation, and differentiation. However, concerns regarding spatiotemporal delivery and uncontrolled differentiation remain.
Injecting GDF-6 into the IVD attenuated inflammatory gene expression and improved disc degeneration in a rabbit puncture model (Miyazaki et al., 2018). Phase 1/2 clinical trials on the intradiscal injection of recombinant human growth and differentiation factor-5 (rhGDF-5) have reported promising results (NCT00813813 and NCT01124006).
PRP, which comprises concentrated autologous platelets and growth factors (Akeda et al., 2019), has shown potential in increasing cell proliferation, matrix production, and disc height in preclinical models. PRP can be classified into four categories, comprising four types of preparation, based on the number of leukocytes and fibrin content (Akeda et al., 2019). Two randomized controlled trials have investigated the effects of PRP. Tuakli-Wosornu et al. reported that injecting PRP into the IVD resulted in significant improvement in lower back pain and function over 8 weeks; moreover, the improvement was maintained at the 1-year follow-up visit (Tuakli-Wosornu et al., 2016). Akeda et al. reported that injecting PRP into the IVD resulted in a significant improvement in the disability score at 26 weeks and walking ability scores at 4 and 8 weeks compared with those achieved with corticosteroid injection (Akeda et al., 2022) (Table 1). Optimal formulations and delivery methods continue to be explored. Small molecules and drugs aim to inhibit inflammatory cytokines and MMPs, while also regulating the expression of catabolic/anabolic genes.
Nuclear factor-κB (NF-κB) decoy and TNF-α inhibitors are biologics that exert anti-inflammatory effects. NF-κB, a transcription factor, regulates the inflammatory cytokine levels. NF-κB decoy, an oligodeoxynucleotide containing the NF-κB binding site that entraps NF-κB subunits, can suppress NF-κB activity. Intradiscal injection of NF-κB can suppress inflammatory gene expression in degenerated discs and restore disc height loss (Kato et al., 2021). Notably, intradiscal injection of a TNF-α inhibitor (etanercept) improved discogenic pain in humans within a 2-month follow-up period in a previous study (Sainoh et al., 2016).
In recent years, studies on the multi-strategy synergistic therapies that incorporate cells, biomaterials, and growth factors for intervertebral disc degeneration have also been conducted. Wei et al. reported that TGF-1 was embedded with MSCs in decellularized annulus fibrosus matrix (DAFM) hydrogels reinforced with polyethylene glycol diacrylate (PEGDA) to facilitate the controlled release of TGF-1 while preserving the hydrogel’s porous structure. Following infusion into a rat model with AF injury, there was a notable increase in the migration of AF cells to the injury site, which promoted anabolic upregulation (Wei et al., 2022). Sun et al. (2020) utilized 3D printing and electrospinning technology to load TGF-β3, CTGF and bone marrow-derived MSCs onto polydopamine nanoparticles and polycaprolactone scaffolds, respectively, mimicking the structure of AF and achieving mechanical properties similar to those of natural AF in the rodents. Although these studies have demonstrated that multi-strategy combination has great potential for IVD repair, further studies in large animal models are necessary.
In vitro and in vivo studies have been conducted using small and large animal models of rodents (such as mice and rats), rabbits; dogs; sheep; goats; pigs; and monkeys; to evaluate regenerative techniques (Poletto et al., 2023). The use of small animal models is a cost-effective approach for screening. Rodents, such as mice and rats, are the most commonly used animal species (54%) in IVD studies (Poletto et al., 2023). However, the physiological characteristics of rodents vary from those of humans. In contrast, the disc size and disc degeneration observed in large animals, such as dogs, sheep, and pigs, is similar to that in humans; nevertheless, replicating the slow, progressive human disc pathology in these animals is difficult. IVD degeneration can be induced via bacterial, chemical, genetic, noninvasive, spontaneous, and surgical methods (Poletto et al., 2023; Oichi et al., 2020). The experimental time points vary depending on the species. The commonly used time points for different species are as follows: rodents, 2 or 4 weeks; rabbits, 4 weeks; sheep, 24 weeks; goats, 12 weeks; pigs, 12 weeks; and monkeys, >104 weeks (Poletto et al., 2023). A single animal model that can recapitulate the entirety of human IVD degeneration remains to be established. However, the results can be interpreted reliably if the limitations of a selected animal species are recognized.
Preclinical studies have demonstrated the promising results of regenerative disc therapies. Nevertheless, these therapies are associated with significant limitations that hinder their translation into clinical practice. These limitations include insufficient graft retention and integration into the disc space, limited survival and proliferation of the cells within the harsh disc microenvironment, uncontrolled differentiation of stem cells, mechanical instability of the implanted scaffolds, and concerns regarding long-term safety. Furthermore, the regulatory requirements for devices and biologics set forth by the U.S. Food and Drug Administration also pose hurdles. Clinical implementation of regenerative disc therapies requires optimization of cell sources, biomaterials, growth factors, PRP, gene therapy, mechanical stimulation, and delivery methods. Evaluation of clinically relevant models and personalized regenerative therapies tailored to individual patients can be achieved using biomarkers, advanced imaging modalities, and bioreactors.
Preclinical studies on regenerative medicine therapies that utilize cells, biomaterials, growth factors, PRP, and biological agents have demonstrated their promise in repairing degenerated lumbar discs. However, these therapies are associated with significant limitations and challenges that hinder their clinical translation. Thus, further studies must be conducted in the future to address these challenges. Advances in the fields of tissue engineering, biomaterials, stem cells, and biological factors will facilitate regenerative therapies to halt or reverse progressive disc degeneration and improve the clinical outcomes and quality of life of patients with LDDD.
TSo: Conceptualization, Methodology, Project administration, Writing–original draft, Writing–review and editing. KS: Writing–original draft, Writing–review and editing, Conceptualization, Methodology, Project administration. TSh: Writing–review and editing, Writing–original draft, Supervision. KM: Writing–review and editing, Writing–original draft, Supervision. SM: Supervision, Writing–review and editing, Writing–original draft. BO: Supervision, Writing–review and editing, Writing–original draft.
The author(s) declare that financial support was received for the research, authorship, and/or publication of this article. This study was supported by JSPS KAKENHI Grant number JP24K19575.
The authors declare that the research was conducted in the absence of any commercial or financial relationships that could be construed as a potential conflict of interest.
All claims expressed in this article are solely those of the authors and do not necessarily represent those of their affiliated organizations, or those of the publisher, the editors and the reviewers. Any product that may be evaluated in this article, or claim that may be made by its manufacturer, is not guaranteed or endorsed by the publisher.
Ahrens, M., Tsantrizos, A., Donkersloot, P., Martens, F., Lauweryns, P., Le Huec, J. C., et al. (2009). Nucleus replacement with the DASCOR disc arthroplasty device: interim two-year efficacy and safety results from two prospective, non-randomized multicenter European studies. Spine (Phila Pa 1976) 34 (13), 1376–1384. doi:10.1097/brs.0b013e3181a3967f
Akeda, K., Ohishi, K., Takegami, N., Sudo, T., Yamada, J., Fujiwara, T., et al. (2022). Platelet-rich plasma releasate versus corticosteroid for the treatment of discogenic low back pain: a double-blind randomized controlled trial. J. Clin. Med. 11 (2), 304. doi:10.3390/jcm11020304
Akeda, K., Yamada, J., Linn, E. T., Sudo, A., and Masuda, K. (2019). Platelet-rich plasma in the management of chronic low back pain: a critical review. J. Pain Res. 12, 753–767. doi:10.2147/JPR.S153085
Amirdelfan, K., Bae, H., McJunkin, T., DePalma, M., Kim, K., Beckworth, W. J., et al. (2021). Allogeneic mesenchymal precursor cells treatment for chronic low back pain associated with degenerative disc disease: a prospective randomized, placebo-controlled 36-month study of safety and efficacy. Spine J. 21 (2), 212–230. doi:10.1016/j.spinee.2020.10.004
Beall, D. P., Davis, T., DePalma, M. J., Amirdelfan, K., Yoon, E. S., Wilson, G. L., et al. (2021). Viable disc tissue allograft supplementation; one- and two-level treatment of degenerated intervertebral discs in patients with chronic discogenic low back pain: one year results of the VAST randomized controlled trial. Pain Physician 24 (6), 465–477.
Berlemann, U., and Schwarzenbach, O. (2009). An injectable nucleus replacement as an adjunct to microdiscectomy: 2 year follow-up in a pilot clinical study. Eur. Spine J. 18 (11), 1706–1712. doi:10.1007/s00586-009-1136-0
Bertagnoli, R., Sabatino, C. T., Edwards, J. T., Gontarz, G. A., Prewett, A., and Parsons, J. R. (2005). Mechanical testing of a novel hydrogel nucleus replacement implant. Spine J. 5 (6), 672–681. doi:10.1016/j.spinee.2004.12.004
Bowles, R. D., Gebhard, H. H., Hartl, R., and Bonassar, L. J. (2011). Tissue-engineered intervertebral discs produce new matrix, maintain disc height, and restore biomechanical function to the rodent spine. Proc. Natl. Acad. Sci. U S A. 108 (32), 13106–13111. doi:10.1073/pnas.1107094108
Bowles, R. D., and Setton, L. A. (2017). Biomaterials for intervertebral disc regeneration and repair. Biomaterials 129, 54–67. doi:10.1016/j.biomaterials.2017.03.013
Ceylan, A., Asik, I., Ozgencil, G. E., and Erken, B. (2019). Clinical results of intradiscal hydrogel administration (GelStix) in lumbar degenerative disc disease. Turk J. Med. Sci. 49 (6), 1634–1639. doi:10.3906/sag-1901-1
Chaparro, L. E., Furlan, A. D., Deshpande, A., Mailis-Gagnon, A., Atlas, S., and Turk, D. C. (2014). Opioids compared with placebo or other treatments for chronic low back pain: an update of the Cochrane Review. Spine (Phila Pa 1976) 39 (7), 556–563. doi:10.1097/BRS.0000000000000249
Chik, T. K., Chooi, W. H., Li, Y. Y., Ho, F. C., Cheng, H. W., Choy, T. H., et al. (2015). Bioengineering a multicomponent spinal motion segment construct--a 3D model for complex tissue engineering. Adv. Healthc. Mater 4 (1), 99–112. doi:10.1002/adhm.201400192
Choy, A. T., and Chan, B. P. (2015). A structurally and functionally biomimetic biphasic scaffold for intervertebral disc tissue engineering. PLoS One 10 (6), e0131827. doi:10.1371/journal.pone.0131827
Clarke, L. E., McConnell, J. C., Sherratt, M. J., Derby, B., Richardson, S. M., and Hoyland, J. A. (2014). Growth differentiation factor 6 and transforming growth factor-beta differentially mediate mesenchymal stem cell differentiation, composition, and micromechanical properties of nucleus pulposus constructs. Arthritis Res. Ther. 16 (2), R67. doi:10.1186/ar4505
Comella, K., Silbert, R., and Parlo, M. (2017). Effects of the intradiscal implantation of stromal vascular fraction plus platelet rich plasma in patients with degenerative disc disease. J. Transl. Med. 15 (1), 12. doi:10.1186/s12967-016-1109-0
Coric, D., Pettine, K., Sumich, A., and Boltes, M. O. (2013). Prospective study of disc repair with allogeneic chondrocytes presented at the 2012 Joint Spine Section Meeting. J. Neurosurg. Spine 18 (1), 85–95. doi:10.3171/2012.10.SPINE12512
de Queiroz, B. Z., Pereira, D. S., Lopes, R. A., Felicio, D. C., Silva, J. P., Rosa, N. M., et al. (2016). Association between the plasma levels of mediators of inflammation with pain and disability in the elderly with acute low back pain: data from the back complaints in the elders (BACE)-Brazil study. Spine (Phila Pa 1976) 41 (3), 197–203. doi:10.1097/BRS.0000000000001214
Deyo, R. A., and Mirza, S. K. (2016). Herniated lumbar intervertebral disk. N. Engl. J. Med. 374 (18), 1763–1772. doi:10.1056/NEJMcp1512658
Du, J., Garcia, J. P., Bach, F. C., Tellegen, A. R., Grad, S., Li, Z., et al. (2022). Intradiscal injection of human recombinant BMP-4 does not reverse intervertebral disc degeneration induced by nuclectomy in sheep. J. Orthop. Transl. 37, 23–36. doi:10.1016/j.jot.2022.08.006
Elabd, C., Centeno, C. J., Schultz, J. R., Lutz, G., Ichim, T., and Silva, F. J. (2016). Intra-discal injection of autologous, hypoxic cultured bone marrow-derived mesenchymal stem cells in five patients with chronic lower back pain: a long-term safety and feasibility study. J. Transl. Med. 14 (1), 253. doi:10.1186/s12967-016-1015-5
Ellman, M. B., Kim, J., An, H. S., Chen, D., Kc, R., Li, X., et al. (2013). Lactoferricin enhances BMP7-stimulated anabolic pathways in intervertebral disc cells. Gene 524 (2), 282–291. doi:10.1016/j.gene.2013.04.003
Fujii, K., Yamazaki, M., Kang, J. D., Risbud, M. V., Cho, S. K., Qureshi, S. A., et al. (2019). Discogenic back pain: literature review of definition, diagnosis, and treatment. JBMR Plus 3 (5), e10180. doi:10.1002/jbm4.10180
Gantenbein-Ritter, B., Benneker, L. M., Alini, M., and Grad, S. (2011). Differential response of human bone marrow stromal cells to either TGF-β1 or rhGDF-5. Eur. Spine J. 20 (6), 962–971. doi:10.1007/s00586-010-1619-z
Gay, M. H., Mehrkens, A., Rittmann, M., Haug, M., Barbero, A., Martin, I., et al. (2019). Nose to back: compatibility of nasal chondrocytes with environmental conditions mimicking a degenerated intervertebral disc. Eur. Cell Mater 37, 214–232. doi:10.22203/eCM.v037a13
Goode, A. P., Marshall, S. W., Kraus, V. B., Renner, J. B., Sturmer, T., Carey, T. S., et al. (2012). Association between serum and urine biomarkers and lumbar spine individual radiographic features: the Johnston County Osteoarthritis Project. Osteoarthr. Cartil. 20 (11), 1286–1293. doi:10.1016/j.joca.2012.08.003
Goparaju, P., Rajamani, P. A., Kulkarni, A. G., Kumar, P., Adbalwad, Y. M., Bhojraj, S., et al. (2023). A 2-year outcomes and complications of various techniques of lumbar discectomy: a multicentric prospective study. Glob. Spine J., 21925682231220042. doi:10.1177/21925682231220042
Gornet, M., Buttermann, G., Guyer, R., Yue, J., Ferko, N., and Hollmann, S. (2017). Defining the ideal lumbar total disc replacement patient and standard of care. Spine (Phila Pa 1976) 42 (Suppl 24), S103–S107. doi:10.1097/BRS.0000000000002453
Haufe, S. M., and Mork, A. R. (2006). Intradiscal injection of hematopoietic stem cells in an attempt to rejuvenate the intervertebral discs. Stem Cells Dev. 15 (1), 136–137. doi:10.1089/scd.2006.15.136
Hayden, J. A., Ellis, J., Ogilvie, R., Malmivaara, A., and van Tulder, M. W. (2021). Exercise therapy for chronic low back pain. Cochrane Database Syst. Rev. 9 (9), CD009790. doi:10.1002/14651858.CD009790.pub2
Henriksson, H. B., Papadimitriou, N., Hingert, D., Baranto, A., Lindahl, A., and Brisby, H. (2019). The traceability of mesenchymal stromal cells after injection into degenerated discs in patients with low back pain. Stem Cells Dev. 28 (17), 1203–1211. doi:10.1089/scd.2019.0074
Hiraishi, S., Schol, J., Sakai, D., Nukaga, T., Erickson, I., Silverman, L., et al. (2018). Discogenic cell transplantation directly from a cryopreserved state in an induced intervertebral disc degeneration canine model. JOR Spine 1 (2), e1013. doi:10.1002/jsp2.1013
Iatridis, J. C., Nicoll, S. B., Michalek, A. J., Walter, B. A., and Gupta, M. S. (2013). Role of biomechanics in intervertebral disc degeneration and regenerative therapies: what needs repairing in the disc and what are promising biomaterials for its repair? Spine J. 13 (3), 243–262. doi:10.1016/j.spinee.2012.12.002
Kamatani, T., Hagizawa, H., Yarimitsu, S., Morioka, M., Koyamatsu, S., Sugimoto, M., et al. (2022). Human iPS cell-derived cartilaginous tissue spatially and functionally replaces nucleus pulposus. Biomaterials 284, 121491. doi:10.1016/j.biomaterials.2022.121491
Kato, K., Akeda, K., Miyazaki, S., Yamada, J., Muehleman, C., Miyamoto, K., et al. (2021). NF-kB decoy oligodeoxynucleotide preserves disc height in a rabbit anular-puncture model and reduces pain induction in a rat xenograft-radiculopathy model. Eur. Cell Mater 42, 90–109. doi:10.22203/eCM.v042a07
Kent, P., Haines, T., O'Sullivan, P., Smith, A., Campbell, A., Schutze, R., et al. (2023). Cognitive functional therapy with or without movement sensor biofeedback versus usual care for chronic, disabling low back pain (RESTORE): a randomised, controlled, three-arm, parallel group, phase 3, clinical trial. Lancet 401 (10391), 1866–1877. doi:10.1016/S0140-6736(23)00441-5
Khan, A. N., Jacobsen, H. E., Khan, J., Filippi, C. G., Levine, M., Lehman, R. A., et al. (2017). Inflammatory biomarkers of low back pain and disc degeneration: a review. Ann. N Y Acad. Sci. 1410 (1), 68–84. doi:10.1111/nyas.13551
Koetsier, E., van Kuijk, S. M. J., Maino, P., Dukanac, J., Scascighini, L., Cianfoni, A., et al. (2022). Efficacy of the Gelstix nucleus augmentation device for the treatment of chronic discogenic low back pain: protocol for a randomised, sham-controlled, double-blind, multicentre trial. BMJ Open 12 (3), e053772. doi:10.1136/bmjopen-2021-053772
Kumar, H., Ha, D. H., Lee, E. J., Park, J. H., Shim, J. H., Ahn, T. K., et al. (2017). Safety and tolerability of intradiscal implantation of combined autologous adipose-derived mesenchymal stem cells and hyaluronic acid in patients with chronic discogenic low back pain: 1-year follow-up of a phase I study. Stem Cell Res. Ther. 8 (1), 262. doi:10.1186/s13287-017-0710-3
Lewandrowski, K. U., Dowling, A., Vera, J. C., Leon, J. F. R., Telfeian, A. E., and Lorio, M. P. (2023). Pain relief after allogenic stem cell disc therapy. Pain Physician 26 (2), 197–206.
Li, Y., Chen, L., Gao, Y., Zou, X., and Wei, F. (2022). Oxidative stress and intervertebral disc degeneration: pathophysiology, signaling pathway, and therapy. Oxid. Med. Cell Longev. 2022, 1–14. doi:10.1155/2022/1984742
Martin, J. T., Milby, A. H., Chiaro, J. A., Kim, D. H., Hebela, N. M., Smith, L. J., et al. (2014). Translation of an engineered nanofibrous disc-like angle-ply structure for intervertebral disc replacement in a small animal model. Acta Biomater. 10 (6), 2473–2481. doi:10.1016/j.actbio.2014.02.024
Matta, A., Karim, M. Z., Gerami, H., Jun, P., Funabashi, M., Kawchuk, G., et al. (2018). NTG-101: a novel molecular therapy that halts the progression of degenerative disc disease. Sci. Rep. 8 (1), 16809. doi:10.1038/s41598-018-35011-4
Mehrkens, A., Muller, A. M., Valderrabano, V., Scharen, S., and Vavken, P. (2012). Tissue engineering approaches to degenerative disc disease--a meta-analysis of controlled animal trials. Osteoarthr. Cartil. 20 (11), 1316–1325. doi:10.1016/j.joca.2012.06.001
Miyazaki, S., Diwan, A. D., Kato, K., Cheng, K., Bae, W. C., Sun, Y., et al. (2018). ISSLS PRIZE IN BASIC SCIENCE 2018: growth differentiation factor-6 attenuated pro-inflammatory molecular changes in the rabbit anular-puncture model and degenerated disc-induced pain generation in the rat xenograft radiculopathy model. Eur. Spine J. 27 (4), 739–751. doi:10.1007/s00586-018-5488-1
Mizuno, H., Roy, A. K., Zaporojan, V., Vacanti, C. A., Ueda, M., and Bonassar, L. J. (2006). Biomechanical and biochemical characterization of composite tissue-engineered intervertebral discs. Biomaterials 27 (3), 362–370. doi:10.1016/j.biomaterials.2005.06.042
Mochida, J., Sakai, D., Nakamura, Y., Watanabe, T., Yamamoto, Y., and Kato, S. (2015). Intervertebral disc repair with activated nucleus pulposus cell transplantation: a three-year, prospective clinical study of its safety. Eur. Cell Mater 29, 202–212. ; discussion 12. doi:10.22203/ecm.v029a15
Nerurkar, N. L., Sen, S., Huang, A. H., Elliott, D. M., and Mauck, R. L. (2010). Engineered disc-like angle-ply structures for intervertebral disc replacement. Spine (Phila Pa 1976) 35 (8), 867–873. doi:10.1097/BRS.0b013e3181d74414
Nesti, L. J., Li, W. J., Shanti, R. M., Jiang, Y. J., Jackson, W., Freedman, B. A., et al. (2008). Intervertebral disc tissue engineering using a novel hyaluronic acid-nanofibrous scaffold (HANFS) amalgam. Tissue Eng. Part A 14 (9), 1527–1537. doi:10.1089/ten.tea.2008.0215
Noriega, D. C., Ardura, F., Hernandez-Ramajo, R., Martin-Ferrero, M. A., Sanchez-Lite, I., Toribio, B., et al. (2017). Intervertebral disc repair by allogeneic mesenchymal bone marrow cells: a randomized controlled trial. Transplantation 101 (8), 1945–1951. doi:10.1097/TP.0000000000001484
Nukaga, T., Sakai, D., Schol, J., Sato, M., and Watanabe, M. (2019). Annulus fibrosus cell sheets limit disc degeneration in a rat annulus fibrosus injury model. JOR Spine 2 (2), e1050. doi:10.1002/jsp2.1050
Ohnishi, T., Sudo, H., Tsujimoto, T., and Iwasaki, N. (2018). Age-related spontaneous lumbar intervertebral disc degeneration in a mouse model. J. Orthop. Res. 36 (1), 224–232. doi:10.1002/jor.23634
Ohtori, S., Inoue, G., Miyagi, M., and Takahashi, K. (2015). Pathomechanisms of discogenic low back pain in humans and animal models. Spine J. 15 (6), 1347–1355. doi:10.1016/j.spinee.2013.07.490
Oichi, T., Taniguchi, Y., Oshima, Y., Tanaka, S., and Saito, T. (2020). Pathomechanism of intervertebral disc degeneration. JOR Spine 3 (1), e1076. doi:10.1002/jsp2.1076
Orozco, L., Soler, R., Morera, C., Alberca, M., Sanchez, A., and Garcia-Sancho, J. (2011). Intervertebral disc repair by autologous mesenchymal bone marrow cells: a pilot study. Transplantation 92 (7), 822–828. doi:10.1097/TP.0b013e3182298a15
Osada, R., Ohshima, H., Ishihara, H., Yudoh, K., Sakai, K., Matsui, H., et al. (1996). Autocrine/paracrine mechanism of insulin-like growth factor-1 secretion, and the effect of insulin-like growth factor-1 on proteoglycan synthesis in bovine intervertebral discs. J. Orthop. Res. 14 (5), 690–699. doi:10.1002/jor.1100140503
Otsuki, B., Fujibayashi, S., Shimizu, T., Murata, K., Masuda, S., and Matsuda, S. (2023). Minimally invasive LLIF surgery to decrease the occurrence of adjacent-segment disease compared to conventional open TLIF. Eur. Spine J. 32 (9), 3200–3209. doi:10.1007/s00586-023-07806-1
Özer, M. İ., and Demirtaş, O. K. (2023). Comparison of lumbar microdiscectomy and unilateral biportal endoscopic discectomy outcomes: a single-center experience. J. Neurosurg. Spine 40 (3), 351–358. doi:10.3171/2023.10.SPINE23718
Pang, X., Yang, H., and Peng, B. (2014). Human umbilical cord mesenchymal stem cell transplantation for the treatment of chronic discogenic low back pain. Pain Physician 17 (4), E525–E530.
Park, H. J., Lee, C. S., Chung, S. S., Park, S. J., Kim, W. S., Park, J. S., et al. (2018). Radiological and clinical long-term results of heterotopic ossification following lumbar total disc replacement. Spine J. 18 (5), 762–768. doi:10.1016/j.spinee.2017.09.003
Park, S. H., Gil, E. S., Cho, H., Mandal, B. B., Tien, L. W., Min, B. H., et al. (2012). Intervertebral disk tissue engineering using biphasic silk composite scaffolds. Tissue Eng. Part A 18 (5-6), 447–458. doi:10.1089/ten.TEA.2011.0195
Pelletier, M. H., Cohen, C. S., Ducheyne, P., and Walsh, W. R. (2016). Restoring segmental biomechanics through nucleus augmentation: an in vitro study. Clin. Spine Surg. 29 (10), 461–467. doi:10.1097/bsd.0b013e3182aa6841
Pettine, K. A., Murphy, M. B., Suzuki, R. K., and Sand, T. T. (2015). Percutaneous injection of autologous bone marrow concentrate cells significantly reduces lumbar discogenic pain through 12 months. Stem Cells 33 (1), 146–156. doi:10.1002/stem.1845
Poletto, D. L., Crowley, J. D., Tanglay, O., Walsh, W. R., and Pelletier, M. H. (2023). Preclinical in vivo animal models of intervertebral disc degeneration. Part 1: a systematic review. JOR Spine 6 (1), e1234. doi:10.1002/jsp2.1234
Ray, C. D. (2002). The PDN® prosthetic disc-nucleus device. Eur. Spine J. 11 (2), S137–S142. doi:10.1007/s00586-002-0425-7
Risbud, M. V., Di Martino, A., Guttapalli, A., Seghatoleslami, R., Denaro, V., Vaccaro, A. R., et al. (2006). Toward an optimum system for intervertebral disc organ culture: TGF-beta 3 enhances nucleus pulposus and anulus fibrosus survival and function through modulation of TGF-beta-R expression and ERK signaling. Spine (Phila Pa 1976) 31 (8), 884–890. doi:10.1097/01.brs.0000209335.57767.b5
Sainoh, T., Orita, S., Miyagi, M., Inoue, G., Kamoda, H., Ishikawa, T., et al. (2016). Single intradiscal administration of the tumor necrosis factor-alpha inhibitor, etanercept, for patients with discogenic low back pain. Pain Med. 17 (1), 40–45. doi:10.1111/pme.12892
Sakai, D., and Andersson, G. B. (2015). Stem cell therapy for intervertebral disc regeneration: obstacles and solutions. Nat. Rev. Rheumatol. 11 (4), 243–256. doi:10.1038/nrrheum.2015.13
Sakai, D., Mochida, J., Iwashina, T., Hiyama, A., Omi, H., Imai, M., et al. (2006). Regenerative effects of transplanting mesenchymal stem cells embedded in atelocollagen to the degenerated intervertebral disc. Biomaterials 27 (3), 335–345. doi:10.1016/j.biomaterials.2005.06.038
Sakai, D., Schol, J., and Watanabe, M. (2022). Clinical development of regenerative medicine targeted for intervertebral disc disease. Med. Kaunas. 58 (2), 267. doi:10.3390/medicina58020267
Sheyn, D., Ben-David, S., Tawackoli, W., Zhou, Z., Salehi, K., Bez, M., et al. (2019). Human iPSCs can be differentiated into notochordal cells that reduce intervertebral disc degeneration in a porcine model. Theranostics 9 (25), 7506–7524. doi:10.7150/thno.34898
Shimizu, T., Fujibayashi, S., Otsuki, B., Murata, K., and Matsuda, S. (2021). Indirect decompression via oblique lateral interbody fusion for severe degenerative lumbar spinal stenosis: a comparative study with direct decompression transforaminal/posterior lumbar interbody fusion. Spine J. 21 (6), 963–971. doi:10.1016/j.spinee.2021.01.025
Siepe, C. J., Heider, F., Wiechert, K., Hitzl, W., Ishak, B., and Mayer, M. H. (2014). Mid-to long-term results of total lumbar disc replacement: a prospective analysis with 5- to 10-year follow-up. Spine J. 14 (8), 1417–1431. doi:10.1016/j.spinee.2013.08.028
Stürmer, T., Raum, E., Buchner, M., Gebhardt, K., Schiltenwolf, M., Richter, W., et al. (2005). Pain and high sensitivity C reactive protein in patients with chronic low back pain and acute sciatic pain. Ann. Rheum. Dis. 64 (6), 921–925. doi:10.1136/ard.2004.027045
Sun, B., Lian, M., Han, Y., Mo, X., Jiang, W., Qiao, Z., et al. (2020)). A 3D-Bioprinted dual growth factor-releasing intervertebral disc scaffold induces nucleus pulposus and annulus fibrosus reconstruction. Bioact. Mater 6 (1), 179–190. doi:10.1016/j.bioactmat.2020.06.022
Sun, R., Zhu, J., Sun, K., Gao, L., Zheng, B., and Shi, J. (2023). Strontium ranelate ameliorates intervertebral disc degeneration via regulating TGF-β1/NF-κB Axis. Int. J. Med. Sci. 20 (13), 1679–1697. doi:10.7150/ijms.86665
Tim, Y. S., Su Kim, K., Li, J., Soo Park, J., Akamaru, T., Elmer, W. A., et al. (2003). The effect of bone morphogenetic protein-2 on rat intervertebral disc cells in vitro. Spine (Phila Pa 1976) 28 (16), 1773–1780. doi:10.1097/01.BRS.0000083204.44190.34
Tsai, T. T., Guttapalli, A., Oguz, E., Chen, L. H., Vaccaro, A. R., Albert, T. J., et al. (2007). Fibroblast growth factor-2 maintains the differentiation potential of nucleus pulposus cells in vitro: implications for cell-based transplantation therapy. Spine (Phila Pa 1976) 32 (5), 495–502. doi:10.1097/01.brs.0000257341.88880.f1
Tsantrizos, A., Ordway, N. R., Myint, K., Martz, E., and Yuan, H. A. (2008). Mechanical and biomechanical characterization of a polyurethane nucleus replacement device injected and cured in situ within a balloon. SAS J. 2 (1), 28–39. doi:10.1016/sasj-2007-0113-rr
Tschugg, A., Diepers, M., Simone, S., Michnacs, F., Quirbach, S., Strowitzki, M., et al. (2017). A prospective randomized multicenter phase I/II clinical trial to evaluate safety and efficacy of NOVOCART disk plus autologous disk chondrocyte transplantation in the treatment of nucleotomized and degenerative lumbar disks to avoid secondary disease: safety results of Phase I-a short report. Neurosurg. Rev. 40 (1), 155–162. doi:10.1007/s10143-016-0781-0
Tuakli-Wosornu, Y. A., Terry, A., Boachie-Adjei, K., Harrison, J. R., Gribbin, C. K., LaSalle, E. E., et al. (2016). Lumbar intradiskal platelet-rich plasma (PRP) injections: a prospective, double-blind, randomized controlled study. PM R. 8 (1), 1–10. doi:10.1016/j.pmrj.2015.08.010
Ukeba, D., Yamada, K., Tsujimoto, T., Ura, K., Nonoyama, T., Iwasaki, N., et al. (2021). Bone marrow aspirate concentrate combined with in situ forming bioresorbable gel enhances intervertebral disc regeneration in rabbits. J. Bone Jt. Surg. Am. 103 (8), e31. doi:10.2106/JBJS.20.00606
van den Eerenbeemt, K. D., Ostelo, R. W., van Royen, B. J., Peul, W. C., and van Tulder, M. W. (2010). Total disc replacement surgery for symptomatic degenerative lumbar disc disease: a systematic review of the literature. Eur. Spine J. 19 (8), 1262–1280. doi:10.1007/s00586-010-1445-3
Wang, D., Lai, A., Gansau, J., Seifert, A. C., Munitz, J., Zaheer, K., et al. (2023). Lumbar endplate microfracture injury induces Modic-like changes, intervertebral disc degeneration and spinal cord sensitization - an in vivo rat model. Spine J. 23 (9), 1375–1388. doi:10.1016/j.spinee.2023.04.012
Wang, H., Ahrens, C., Rief, W., Gantz, S., Schiltenwolf, M., and Richter, W. (2010). Influence of depression symptoms on serum tumour necrosis factor-alpha of patients with chronic low back pain. Arthritis Res. Ther. 12 (5), R186. doi:10.1186/ar3156
Wei, Q., Liu, D., Chu, G., Yu, Q., Liu, Z., Li, J., et al. (2022). TGF-β1-supplemented decellularized annulus fibrosus matrix hydrogels promote annulus fibrosus repair. Bioact. Mater 19 (19), 581–593. doi:10.1016/j.bioactmat.2022.04.025
Weiss, A. R. R., and Dahlke, M. H. (2019). Immunomodulation by mesenchymal stem cells (MSCs): mechanisms of action of living, apoptotic, and dead MSCs. Front. Immunol. 10, 1191. doi:10.3389/fimmu.2019.01191
Yuksel, U., Walsh, S., Curd, D., and Black, K. (2002). Fatigue durabiity of a novel disc nucleus repair system. Spine J. 2 (5), 103–104. doi:10.1016/S1529-9430(02)00233-4
Zhang, Y., Zhang, Z., Chen, P., Ma, C. Y., Li, C., Au, T. Y. K., et al. (2020). Directed differentiation of notochord-like and nucleus pulposus-like cells using human pluripotent stem cells. Cell Rep. 30 (8), 2791–2806 e5. doi:10.1016/j.celrep.2020.01.100
Keywords: lumbar degenerative disc disease, regenerative therapy, cell therapy, biomaterials, growth factors, animal models
Citation: Sono T, Shima K, Shimizu T, Murata K, Matsuda S and Otsuki B (2024) Regenerative therapies for lumbar degenerative disc diseases: a literature review. Front. Bioeng. Biotechnol. 12:1417600. doi: 10.3389/fbioe.2024.1417600
Received: 12 May 2024; Accepted: 19 August 2024;
Published: 26 August 2024.
Edited by:
Pablo Taboada, University of Santiago de Compostela, SpainReviewed by:
Peikai Chen, The University of Hong Kong, ChinaCopyright © 2024 Sono, Shima, Shimizu, Murata, Matsuda and Otsuki. This is an open-access article distributed under the terms of the Creative Commons Attribution License (CC BY). The use, distribution or reproduction in other forums is permitted, provided the original author(s) and the copyright owner(s) are credited and that the original publication in this journal is cited, in accordance with accepted academic practice. No use, distribution or reproduction is permitted which does not comply with these terms.
*Correspondence: Takashi Sono, dHM0NjYyNDFAa3VocC5reW90by11LmFjLmpw
Disclaimer: All claims expressed in this article are solely those of the authors and do not necessarily represent those of their affiliated organizations, or those of the publisher, the editors and the reviewers. Any product that may be evaluated in this article or claim that may be made by its manufacturer is not guaranteed or endorsed by the publisher.
Research integrity at Frontiers
Learn more about the work of our research integrity team to safeguard the quality of each article we publish.