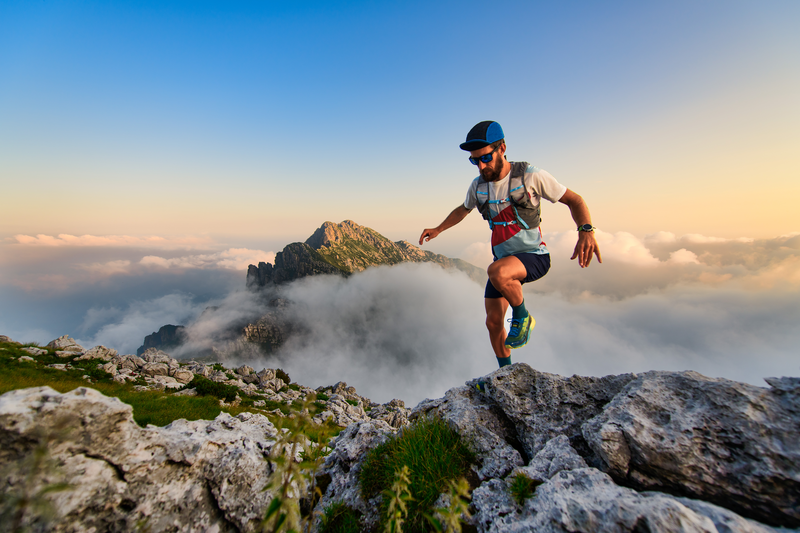
95% of researchers rate our articles as excellent or good
Learn more about the work of our research integrity team to safeguard the quality of each article we publish.
Find out more
MINI REVIEW article
Front. Bioeng. Biotechnol. , 03 July 2024
Sec. Biomaterials
Volume 12 - 2024 | https://doi.org/10.3389/fbioe.2024.1400918
Metal alloys like stainless steel, titanium, and cobalt-chromium alloys are preferable for bio-implants due to their exceptional strength, tribological properties, and biocompatibility. However, long-term implantation of metal alloys can lead to inflammation, swelling, and itching because of ion leaching. To address this issue, polymers are increasingly being utilized in orthopedic applications, replacing metallic components such as bone fixation plates, screws, and scaffolds, as well as minimizing metal-on-metal contact in total hip and knee joint replacements. Ceramics, known for their hardness, thermal barrier, wear, and corrosion resistance, find extensive application in electrochemical, fuel, and biomedical industries. This review delves into a variety of biocompatible materials engineered to seamlessly integrate with the body, reducing adverse reactions like inflammation, toxicity, or immune responses. Additionally, this review examines the potential of various biomaterials including metals, polymers, and ceramics for implant applications. While metallic biomaterials remain indispensable, polymers and ceramics show promise as alternative options. However, surface-modified metallic materials offer a hybrid effect, combining the strengths of different constituents. The future of biomedical implant materials lies in advanced fabrication techniques and personalized designs, facilitating tailored solutions for complex medical needs.
The implant materials are subjected to in vivo and physiological conditions which leads to interaction with the cells, tissue growth, and body solutions (Jin and Chu, 2019). Therefore, the implant materials require bioactive properties that influence the growth of tissue around the implant materials. This bioactive property was influenced by surface engineering and surface modification techniques on the bioinert materials. In addition, the hardness, wear, and corrosion resistance of the implant materials were improved when compared to the bioinert material (Unune et al., 2022). Selecting the implant materials according to the application demands leads to reducing the risk to the patient and avoiding secondary surgeries. The implant materials are differentiated into two main applications, namely, temporary and permanent implants. In temporary implant applications, the devices such as bone fixation plates, screws, nails, and wire are used for an implant. Load-bearing applications such as hip joint, knee joint, ankle, and prosthesis come under permanent application (Jin and Chu, 2019). Biodegradable materials are the best choice for temporary implants because they avoid secondary surgeries to remove the implant materials after the healing period (Peng et al., 2019). On the other hand, permanent application requires non-degradable materials with high mechanical, tribological resistance, and biocompatibility properties to avoid premature failure, and inflammation causes for the patients. The biomaterials such as metals alloys, ceramics, and polymers are used for these temporary and permanent implants based on their application needs. Each material has its unique mechanical, tribological, and biocompatibility properties, all these properties are attained in metallic alloys, ceramic, and polymer-based materials, and these materials can withstand biological function and physiological conditions for orthopaedic application. For instance, metal alloys are most frequently used in load-bearing applications because of their strength (Davidson and Mishra, 1992), whereas polymers are used as a barrier in the metal-on-metal contact area to reduce friction and wear debris (Hussain et al., 2020). In addition, ceramics are used for good corrosion and wear resistance, and bioactive ceramics are employed for enhancing the material performance by coating (Zang and Xun, 2021). This minireview critically analyzed the potential of biocompatible materials, metallic, ceramic and polymeric materials for implant applications.
Nowadays, the need for bio-implant increases for different patients, which includes design, innovative structure, and manufacturing of a bio-implant as a challenging task to develop its complex structure. Materials consist of different properties such as bioinert, biodegradable, bioactive, and bioresorbable to achieve the desired function of the implant in a body. The implant materials should be biocompatible to perform a certain function in a body. The diagrammatic representation of biocompatible material is shown in Figure 1A. The term bio manufacturing refers to the combination of life science and basic engineering to form a product that is biocompatible to enhance the quality of life science. However, the concept is to make composite material, which consists of an integration of synthetic (artificial) and biological materials like protein cells (Bartolo et al., 2012).
Figure 1. (A) Diagrammatic representation of biocompatible behavior, (B) Metallic materials, (C) Ceramic materials, (D) Polymer materials.
The bioinert material can lower the immune reaction and foreign body reaction in the implanted areas. Due to the foreign body interaction, the immune reaction occurs on the implanted materials by forming the fibrous capsule favors, but the implant material has a poor tissue interface (Castner and Ratner, 2002). Hence, the implant fails and undergoes multiple surgeries. Here, researchers have used two approaches such as biological fixation and bioactive fixation to overcome implant failures (Cao and Hench, 1996). In biological fixation, the implant material surface is modified with a porous structure and rough surface to improve tissue ingrowth and angiogenesis. On the other hand, bioactive fixation improves the strong mechanical bond in the bone-implanted area (Salinas et al., 2013). Metallic materials such as titanium, stainless steel, cobalt chromium are employed as bioimplant in specific application like stem, stent, fixation plate and screws (Pandey et al., 2020). Also ceramics like zirconia and alumina are used as load bearing material for hip joint femoral head, joints, and dental cap applications.
Generally, bioactive materials are manufactured to enhance the biological response and cell behavior (Navarro et al., 2008). In the biological environment, the bioactive materials help to avoid fibrous layer formation and prosthesis failure, it also provides consistency to the bone growth and mineralization between the natural bone and artificial bone (implant material). The major factors concerned in implantation are the mechanical properties and the adhesive bond between the tissue and implant material because it helps to enhance the life of the implant material and avoid multiple surgeries (Hench, 1998). Significantly, the pores design factor also plays a major role in cell growth and colonization, but the void pores structure causes impaired vascularization due to endothelial cells (Salem et al., 2002). In addition, large pores affect the integrity of the material (Karande et al., 2004). On the other hand, pores less than 100 nm affect the material factor and nutrients, which causes implant failure and push to multiple surgeries (Zimmermann et al., 2004). Hydroxyapatite, and glass ceramics belongs to bioactive group, which are used as coating on the surface of metallic material to improve the surface property of the implants (Kumar and Singh, 2024; Priyanka et al., 2024).
The material that has the potential to degrade in the physiological environment without causing any toxic effect on the patient is called bioresorbable or bioabsorbable material. Due to the biocompatibility of the bioresorbable material, advanced technologies contributed to the development of the material by using different syntheses, implant designs, and innovative surgical equipment (Freed et al., 1994). Calcium phosphate, sulphate and carbonate are considered as bioresorbable, where these materials are used for the drug delivery application.
Biodegradability is the property of a material to degrade over a period when present in the human body. The biodegradable materials are converted into other elements like CO2, water, and iron by the action of microorganisms. Different biodegradable materials are considered for the temporary bone fixation process. These materials consist of magnesium, Fe, and zinc-based alloys in metallic implants. Polyethylene glycol (PEG) and Polyglycolide (PGA) are included as biodegradable materials in the polymer category.
Metallic biomaterials such as Stainless Steel (SS 316L), Titanium alloys (Ti-6Al-4V), and Cobalt Chromium (Co–Cr) are predominantly employed to replace defective hard tissue. These materials have been extensively studied due to their suitable bulk properties (Al-Amin et al., 2020). The classification of different metallic materials is shown in Figure 1B.
Titanium alloys possess unique properties including exceptional mechanical, corrosion resistance, and biocompatibility with low-density factors. Combining titanium with former metals improves the biocompatible of titanium alloys that are extensively utilized in the manufacturing of bioimplants. Out of these materials, the titanium alloys have different crystal structures like α+β and low young modulus β phase match near to natural bone properties. This material with a specific crystal structure is recognized as a suitable replacement for hard tissue without disclosure of toxic elements (Niinomi, 2002). The elements present in titanium alloys such as (Ta)Tantalum, (Nb) Niobium, and (Zr) Zirconium are considered as harmless which are essential for the strength of bioimplants. It has been proved that a low modulus of titanium alloy can be used to replace natural bone fracture. Ti-6Al-4V alloy is the recommended material for different application in the biomedical field and includes 45% of implant production. As, Ti alloys consist of elastic modulus above that of a natural bone (55–110 GPa) (Majumder et al., 2020) the stress shielding effect can be reduced compared to other materials.
Stainless steel (SS 316L) is a highly recommended metal alloy for biomedical applications because of their mechanical properties and low manufacturing cost (Bekmurzayeva et al., 2018). SS 316L contains 10.5% of chromium elements which helps to alter the surface by forming the metal chromium oxide layer and improving the corrosion resistance. Moreover, SS 316L contains different elements such as iron, nickel, and carbon. The presence of 0.03% carbon in the steel alloy maximizes the yield strength and corrosion resistance for orthopedic applications (Lodhi et al., 2019). Duplex steel is also preferred as an implant by altering the surface of the material using specific surface modification technique. This enhances the longevity of the implant for biomedical application (Pandey et al., 2020; Mahajan et al., 2023a).
Cobalt-Chromium (Co-Cr) alloys are precisely used in load-bearing applications such as hip and knee joints due to their hardness, and tribological properties (Mahajan et al., 2023a). In addition, clinical studies proved that the Co-Cr alloys are good in biocompatibility. In Co-Cr alloys, chromium contains 28 wt%, 6 wt% Mo, and balance wt% of Co and it interacts with cells, and protein solutions in the human body (Bandyopadhyay et al., 2019b; Bandyopadhyay et al., 2019b). The presence of 28 wt% Cr leads to the formation of Cr2O3 oxide layer on the material surface which shields the surface from corrosive environment, also it enhances biocompatibility and improves osseointegration (Alvarez-Vera et al., 2021).
Magnesium-based alloys are recently considered to reduce the difficulties of long-term bioimplants in the human body due to their biodegradable property. After the material degradation, the destroyed elements are further processed to satisfy the basic demands of metabolic pathways. Earlier titanium β-type alloy is used for long-term application due to its strength, low Young’s modulus, and biocompatible. Among different metallic alloys, magnesium is currently focused on replacement joints, knee joints, bone plates, and shoulders (Ibrahim et al., 2017). Moreover, this material is also applicable for cardiovascular stents, trauma, spinal discs, and fixation plates (Rajan Soundararajan et al., 2018). The implementation of low-cost β-type titanium alloy is increased for implant application because of having high biocompatibility by alloying with other elements. This type of material is formed by a cold working process (Yumak and Aslantaş, 2020).
Currently, in metallic materials, magnesium plays an important role in the biomedical field due to its biodegradable properties. This tries to avoid secondary surgery from the clinical perspective. Although the usage of magnesium increases in implant applications, there is an issue with a faster corrosion rate within the human body over some time (Chen et al., 2019). To overcome the limitations of commercially available magnesium, it is recommended to use magnesium alloys such as magnesium zinc, magnesium copper, and magnesium calcium (Uva Narayanan et al., 2023).
Bio-ceramic material is increasingly used in the medical field due to its excellent properties like biocompatible, mechanical strength, aesthetic look, chemical stability, and porous structure (Petit et al., 2018). Here, the challenging part is the interaction of ceramic material with bone tissue, where the ceramic material is biologically stable or reabsorbed over a period. The idea of using bio-ceramic scaffolds, coating ceramic materials, and composite materials changed over a period without altering the biological properties (Pradeep et al., 2022). Nowadays, ceramic materials such as alumina, zirconia, and zirconia-toughened alumina are used to replace fractured bone in orthopedic applications. In addition, additive manufacturing technology is used to make these ceramic materials efficient compared to the conventional subtractive method (Liu et al., 2022). Different materials for ceramic materials are shown in Figure 1C.
The ceramics employed in orthopedic applications are classified into two types such as bioinert and bioactive depending upon the behavior of the material. Therefore, the inert type ceramics are fabricated dense for bearing in the joint replacement to improve the strength of the material against wear and corrosion conditions. The total joint hip replacement includes the metal-on-polyethylene combination, where the long-term reliability of joints in the human body depends upon the wear of its metal component (Todros et al., 2021). In the long term duration, the polyethylene induces macrophages that lead to the bone reabsorbing cytokines resulting in the loss of bone stocks. On the other hand, bioactive ceramics are biologically bonded to the bone, where it is used as a coating material to enhance the fixation of the device because of the osteoconductive property (Uva Narayanan et al., 2023; Bera et al., 2024).
However, the combination of alumina on alumina is also proposed owing to exceptional wear and corrosion resistance of the material but resulted in the loosening of the acetabular product for long-term usage. The major problem with ceramic material is fracture that leads to damage of components when consumed for a long period in the human body. Now the risk of brittle fracture is eliminated by implementing new techniques in the fabrication process. Here the microstructure of the ceramic material depends upon the fabrication process, grain size, distribution of the grains, density, and powder quality (Rony et al., 2018).
The possibility of using ceramics increases in the biomedical field, by improving the surface characteristics of advanced ceramics such as calcium phosphate, silica, and oxide. These ceramics are frequently used in different applications like bio-materials, bio-sensors, implants, drug delivery, and tissue engineering. The wide challenges experienced by the material surface are addressed to overcome the material defects (Treccani et al., 2013). The most essential criterion while developing a biomaterial is to understand the characteristics of the materials towards different bio environments. It also provides detailed information about the behavior of the material such as bio-inert, bio-active, bio-absorbable, biocompatible, and sterilization (Paul, 2019). The nano-structured ceramic materials, coatings, and cement are applied to orthopedic, medical, and dental applications to improve biomedical functions. Recently, ceramic nano-materials such as tri-calcium phosphate, hydroxyl apatite, calcium phosphate, calcium sulfate, bioactive glasses, zirconia ceramics, and alumina are considered for these applications (Balasubramanian et al., 2017).
Earlier, there are many thermoplastic polymers such as polylactic acid, polymethyl methacrylate, polyvinyl chloride, Polyether ether ketone (PEEK), Polyethylene terephthalate (PET), and poly ethylene existed in the biomedical field owing to their outstanding properties (Ranjan, 2023). Different materials for polymers are shown in Figure 1D.
Among different polymers, polyethylene is predominantly consumed by bioimplants because of its tribological properties. However, it has been stated that the wear particles of polyethylene lead to the failure of artificial joints which causes aseptic loosening. Therefore, research area related to the wear of artificial joints develops into an essential field within implant manufacturing. In the future, newly developed materials such as crosslinked with polyethylene, bioceramics, and fiber-reinforced PEEK with enhanced wear resistance and outstanding biocompatibility, are recommended for the fabrication of artificial joints. Lubricant may be considered an important parameter and its purpose is to duplicate the synovial fluids. The chances of high pressure being developed between the joints with a sliding velocity tend to increase the wear of the material. Moreover, the hard particles might scratch the bearing surface, leading to the rise of wear and tear. Artificial joints for total hip and knee are the most popular applications for these polymers (Gang et al., 2018).
At present, there is a chance of adding chitosan as filler to the PVC polymers to improve the biocompatible behavior of the matrix for implant and scaffold application (Ranjan, 2023). In the current situation, the usage of hyper-branched polymers is increasing in biomedical applications such as drug delivery, tissue engineering, and detecting diseases. This is due to the bio-compatible and degradable properties of the polymers that match the requirements of biomedicine. When compared to nano-sized polymers, these hyperbranched polymers are easy to fabricate (Saadati et al., 2021).
The improvement of polymer composites is discussed which is used in biomedical applications in terms of the fabrication process, structure, and physical properties. The necessities of bioactive polymers are essential for bone regenerative, oxygen-releasing, and conductive materials. Most of the applications associated with the biomedical field are listed as polymer stents sutures, porous membranes, artificial heart valves, and barrier films. On the other hand, non-bioactive polymers are developed to make a scaffold of porous structure which is placed as filler for supporting the fractured bone (Guo et al., 2021). Currently, polymer composites are also used as bio-absorbable films in the medical field such as wound healing, skin treatment, and scaffolding (Prasad, 2021). Different materials and their applications are listed in Table 1.
Challenges associated with biocompatible materials include achieving optimal biocompatibility without compromising mechanical strength, durability, or functionality. Additionally, ensuring long-term stability within the biological environment, minimizing immune responses, and addressing issues related to degradation and biocompatibility over time pose significant hurdles. Generally, the metallic alloys are frequently used in the structural application of biomedical such as knee, hip, and dental implants, where high mechanical strength is needed to withstand the loading conditions. Challenges associated with metallic implant materials include corrosion, metal ion release, and potential allergic reactions in patients. Recently, surface coating of metallic alloys with calcium phosphate, hydroxyapatite, and oxide layer has played a major role in overcoming the leaching problem. Another major issue with metal alloy is the stress shielding effect which is due to the increase in the Young’s modulus compared to the natural bone. The problem associated with magnesium alloys are faster degradation rate, where special surface treatment is required to suppress this issue. Ceramic is especially used for specific applications like acetabular cups, dental crowns, scaffolds, and implants. Ceramic implant materials encounter challenges related to their brittleness and susceptibility to fracture, particularly in high-stress environments. On the other hand, polymers are used for bone regenerative, scaffolds, polymer stents, wound healing, and porous membranes in biomedical applications. Polymer implant materials face challenges related to their mechanical properties, such as strength and stiffness, which may not always meet the requirements for load-bearing applications. Moreover, ensuring long-term stability and preventing degradation or wear over time are significant concerns. Finally, the development of cost-effective manufacturing processes and scaling up production while maintaining quality standards remains a challenge in making these materials widely accessible for medical and biological applications.
The future of implant materials lies in the development of advanced biomimetic designs and innovative fabrication techniques, enabling enhanced integration with the body and prolonged durability. Integration of smart materials and nanotechnology holds promise for personalized, regenerative implants capable of real-time monitoring and therapeutic interventions.
AD: Writing–original draft. SP: Writing–original draft. JN: Writing–review and editing. TK: Supervision, Writing–review and editing. SHK: Writing–review and editing, Funding acquisition.
The author(s) declare that financial support was received for the research, authorship, and/or publication of this article.
The authors would like to acknowledge the support of Prince Sultan University, Riyadh for paying the Article Processing Charge (APC) of this publication.
The authors declare that the research was conducted in the absence of any commercial or financial relationships that could be construed as a potential conflict of interest.
All claims expressed in this article are solely those of the authors and do not necessarily represent those of their affiliated organizations, or those of the publisher, the editors and the reviewers. Any product that may be evaluated in this article, or claim that may be made by its manufacturer, is not guaranteed or endorsed by the publisher.
Al-Amin, M., Abdul Rani, A. M., Abdu Aliyu, A. A., Abdul Razak, M. A., Hastuty, S., and Bryant, M. G. (2020). Powder mixed-EDM for potential biomedical applications: a critical review. Mater. Manuf. Process. 35 (16), 1789–1811. doi:10.1080/10426914.2020.1779939
Alvarez-Vera, M., Ortega, J. A., Ortega-Ramos, I. A., Hdz-García, H. M., Muñoz-Arroyo, R., Díaz-Guillén, J. C., et al. (2021). Tribological and microstructural characterization of laser microtextured CoCr alloy tested against UHMWPE for biomedical applications. Wear 477, 203819. doi:10.1016/j.wear.2021.203819
Balasubramanian, S., Gurumurthy, B., and Balasubramanian, A. (2017). Biomedical applications of ceramic nanomaterials: a review. Int. J. Pharm. Sci. Res. 8 (12). doi:10.13040/IJPSR.0975-8232.8(12).4950-59
Bandyopadhyay, A., Shivaram, A., Isik, M., Avila, J. D., Dernell, W. S., and Bose, S. (2019b). Additively manufactured calcium phosphate reinforced CoCrMo alloy: bio-tribological and biocompatibility evaluation for load-bearing implants. Addit. Manuf. 28 (May), 312–324. doi:10.1016/j.addma.2019.04.020
Bartolo, P., Kruth, J.-P., Silva, J., Levy, G., Malshe, A., Rajurkar, K., et al. (2012). Biomedical production of implants by additive electro-chemical and physical processes. CIRP Ann. 61 (2), 635–655. doi:10.1016/j.cirp.2012.05.005
Bekmurzayeva, A., Duncanson, W. J., Azevedo, H. S., and Kanayeva, D. (2018). Surface modification of stainless steel for biomedical applications: revisiting a century-old material. Mater. Sci. Eng. C 93, 1073–1089. doi:10.1016/j.msec.2018.08.049
Bera, T., Manna, I., and Dutta Majumdar, J. (2024). Titanium carbide (TiC) dispersed surface on titanium based alloy (Ti-13Nb-13Zr) by in-situ laser composite surfacing and its wear performance. J. Mater. Process. Technol. 328, 118394. doi:10.1016/j.jmatprotec.2024.118394
Cao, W., and Hench, L. L. (1996). Bioactive materials. Ceram. Int. 22 (6), 493–507. doi:10.1016/0272-8842(95)00126-3
Castner, D. G., and Ratner, B. D. (2002). Biomedical surface science: foundations to frontiers. Surf. Sci. 500 (1–3), 28–60. doi:10.1016/S0039-6028(01)01587-4
Chen, Y., Dou, J., Yu, H., and Chen, C. (2019). Degradable magnesium-based alloys for biomedical applications: the role of critical alloying elements. J. Biomaterials Appl. 33 (10), 1348–1372. doi:10.1177/0885328219834656
Davidson, J. A., and Mishra, A. K. (1992). Surface modification issues for orthopaedic implant bearing surfaces. Mater. Manuf. Process. 7 (3), 405–421. doi:10.1080/10426919208947429
Freed, L. E., Vunjak-Novakovic, G., Biron, R. J., Eagles, D. B., Lesnoy, D. C., Barlow, S. K., et al. (1994). Biodegradable polymer scaffolds for tissue engineering. Nat. Biotechnol. 12 (7), 689–693. doi:10.1038/nbt0794-689
Gang, S., Fengzhou, F., and Chengwei, K. (2018). Tribological performance of bioimplants: a comprehensive review. Nanotechnol. Precis. Eng. 1 (2), 107–122. doi:10.13494/j.npe.20180003
Guo, Z., Poot, A. A., and Grijpma, D. W. (2021). Advanced polymer-based composites and structures for biomedical applications. Eur. Polym. J. 149, 110388. doi:10.1016/j.eurpolymj.2021.110388
Heijnens, L. J., Schotanus, M. G., Verburg, A. D., and van Haaren, E. H. (2021). Disappointing long-term outcome of THA with carbon-fiber-reinforced poly-ether-ether-ketone (CFR-PEEK) as acetabular insert liner: a prospective study with a mean follow-up of 14.3 years. HIP Int. 31 (6), 735–742. doi:10.1177/1120700020918157
Hench, L. L. (1998). Bioceramics. J. Am. Ceram. Soc. 81 (7), 1705–1728. doi:10.1111/j.1151-2916.1998.tb02540.x
Hussain, M., Naqvi, R. A., Abbas, N., Khan, S. M., Nawaz, S., Hussain, A., et al. (2020). Ultra-high-molecular-weight-polyethylene (uhmwpe) as a promising polymer material for biomedical applications: a concise review. Polymers 12 (2), 323. doi:10.3390/polym12020323
Ibrahim, M. Z., Sarhan, A. A. D., Yusuf, F., and Hamdi, M. (2017). Biomedical materials and techniques to improve the tribological, mechanical and biomedical properties of orthopedic implants – a review article. J. Alloys Compd. 714, 636–667. doi:10.1016/j.jallcom.2017.04.231
Jin, W., and Chu, P. K. (2019). “Orthopedic implants,” in Encyclopedia of biomedical engineering (Berlin, Germany: Elsevier), 425–439. doi:10.1016/B978-0-12-801238-3.10999-7
Kamitakahara, M., Ohtsuki, C., and Miyazaki, T. (2008). Review paper: behavior of ceramic biomaterials derived from tricalcium phosphate in physiological condition. J. Biomaterials Appl. 23 (3), 197–212. doi:10.1177/0885328208096798
Kamrani, S., and Fleck, C. (2019). Biodegradable magnesium alloys as temporary orthopaedic implants: a review. BioMetals 32 (2), 185–193. doi:10.1007/s10534-019-00170-y
Karande, T. S., Ong, J. L., and Agrawal, C. M. (2004). Diffusion in musculoskeletal tissue engineering scaffolds: design issues related to porosity, permeability, architecture, and nutrient mixing. Ann. Biomed. Eng. 32 (12), 1728–1743. doi:10.1007/s10439-004-7825-2
Kassapidou, M., Hjalmarsson, L., Johansson, C. B., Hammarström Johansson, P., Morisbak, E., Wennerberg, A., et al. (2020). Cobalt–chromium alloys fabricated with four different techniques: ion release, toxicity of released elements and surface roughness. Dent. Mater. 36 (11), e352–e363. doi:10.1016/j.dental.2020.08.012
Kumar, A., and Singh, G. (2024). Surface modification of Ti6Al4V alloy via advanced coatings: mechanical, tribological, corrosion, wetting, and biocompatibility studies. J. Alloys Compd. 989, 174418. doi:10.1016/j.jallcom.2024.174418
Liu, J., Xiong, S., Mei, H., and Chen, Z. (2022). 3D printing of complex-shaped polymer-derived ceramics with enhanced structural retention. Mater. Manuf. Process. 37 (11), 1267–1279. doi:10.1080/10426914.2022.2030870
Lodhi, M. J. K., Deen, K. M., Greenlee-Wacker, M. C., and Haider, W. (2019). Additively manufactured 316L stainless steel with improved corrosion resistance and biological response for biomedical applications. Addit. Manuf. 27, 8–19. doi:10.1016/j.addma.2019.02.005
Love, B. (2017). “Metallic biomaterials,” in Biomaterials (Berlin, Germany: Elsevier), 159–184. doi:10.1016/B978-0-12-809478-5.00007-9
Mahajan, A., Devgan, S., and Kalyanasundaram, D. (2023a). Surface alteration of Cobalt-Chromium and duplex stainless steel alloys for biomedical applications: a concise review. Mater. Manuf. Process. 38 (3), 260–270. doi:10.1080/10426914.2022.2105873
Majumder, M. K., Kumbhare, V. R., Japa, A., and Kaushik, B. K. (2020). “Nanomaterials and applications,” in Introduction to microelectronics to nanoelectronics (United States: CRC Press), 251–294. doi:10.1201/9781003049203-9
Navarro, M., Michiardi, A., Castaño, O., and Planell, J. A. (2008). Biomaterials in orthopaedics. J. R. Soc. Interface 5 (27), 1137–1158. doi:10.1098/rsif.2008.0151
Niinomi, M. (2002). Recent metallic materials for biomedical applications. Metallurgical Mater. Trans. A 33 (3), 477–486. doi:10.1007/s11661-002-0109-2
Omar, M. A., Baharudin, B.-H., and Sulaiman, S. (2017). Stent manufacturing using cobalt chromium molybdenum (CoCrMo) by selective laser melting technology. AIP Conf. Proc. 2017, 040001. doi:10.1063/1.5010490
Pandey, A., Awasthi, A., and Saxena, K. K. (2020). Metallic implants with properties and latest production techniques: a review. Adv. Mater. Process. Technol. 6 (2), 405–440. doi:10.1080/2374068X.2020.1731236
Paul, S. (2019). Biomedical engineering and its applications in healthcare. Singapore: Springer Singapore. doi:10.1007/978-981-13-3705-5
Peng, H., Wang, K., and Huang, Z. (2019). An injection molding method to prepare chitosan-zinc composite material for novel biodegradable flexible implant devices. Mater. Manuf. Process. 34 (3), 256–261. doi:10.1080/10426914.2018.1532580
Petit, C., Montanaro, L., and Palmero, P. (2018). Functionally graded ceramics for biomedical application: concept, manufacturing, and properties. Int. J. Appl. Ceram. Technol. 15 (4), 820–840. doi:10.1111/ijac.12878
Pradeep, N., Sastry, C. C., Brandão, L., and Meennakshi, B. S. (2022). 3D printing of shrimp derived chitosan with HAp as a bio-composite scaffold. Mater. Manuf. Process. 37 (11), 1257–1266. doi:10.1080/10426914.2021.1973032
Prasad, A. (2021). Bioabsorbable polymeric materials for biofilms and other biomedical applications: recent and future trends. Mater. Today Proc. 44, 2447–2453. doi:10.1016/j.matpr.2020.12.489
Priyanka, C. P., Sudeep, U., Keerthi Krishnan, K., and Ramachandran, K. K. (2024). Surface characterisation and in vitro osteogenic and bacterial adhesion assays of laser treated and hydroxyapatite coated Ti6Al4V bioimplant surfaces. Mater. Today Commun. 39, 108802. doi:10.1016/j.mtcomm.2024.108802
Rajan Soundararajan, S., Vishnu, J., Manivasagam, G., and Rao Muktinutalapati, N. (2018). “Processing of beta titanium alloys for aerospace and biomedical applications,” in Titanium alloys - novel aspects of their processing [working title] (London, UK: IntechOpen). doi:10.5772/intechopen.81899
Ranjan, N. (2023). Chitosan with PVC polymer for biomedical applications: a bibliometric analysis. Mater. Today Proc. 81, 894–898. doi:10.1016/j.matpr.2021.04.274
Rony, L., de Sainte Hermine, P., Steiger, V., Mallet, R., Hubert, L., and Chappard, D. (2018). Characterization of wear debris released from alumina-on-alumina hip prostheses: analysis of retrieved femoral heads and peri-prosthetic tissues. Micron 104, 89–94. doi:10.1016/j.micron.2017.11.002
Saadati, A., Hasanzadeh, M., and Seidi, F. (2021). Biomedical application of hyperbranched polymers: recent Advances and challenges. TrAC Trends Anal. Chem. 142, 116308. doi:10.1016/j.trac.2021.116308
Salem, A. K., Stevens, R., Pearson, R. G., Davies, M. C., Tendler, S. J. B., Roberts, C. J., et al. (2002). Interactions of 3T3 fibroblasts and endothelial cells with defined pore features. J. Biomed. Mater. Res. 61 (2), 212–217. doi:10.1002/jbm.10195
Salinas, A. J., Esbrit, P., and Vallet-Regí, M. (2013). A tissue engineering approach based on the use of bioceramics for bone repair. Biomater. Sci. 1 (1), 40–51. doi:10.1039/C2BM00071G
Sari, M., Hening, P., Ana, I. D., and Yusuf, Y. (2021). Bioceramic hydroxyapatite-based scaffold with a porous structure using honeycomb as a natural polymeric Porogen for bone tissue engineering. Biomaterials Res. 25 (1), 2. doi:10.1186/s40824-021-00203-z
Shahemi, N., Liza, S., Abbas, A. A., and Merican, A. (2018). Long-term wear failure analysis of uhmwpe acetabular cup in total hip replacement. J. Mech. Behav. Biomed. Mater. 87, 1–9. doi:10.1016/j.jmbbm.2018.07.017
Todros, S., Todesco, M., and Bagno, A. (2021). Biomaterials and their biomedical applications: from replacement to regeneration. Processes 9 (11), 1949. doi:10.3390/pr9111949
Treccani, L., Yvonne Klein, T., Meder, F., Pardun, K., and Rezwan, K. (2013). Functionalized ceramics for biomedical, biotechnological and environmental applications. Acta Biomater. 9 (7), 7115–7150. doi:10.1016/j.actbio.2013.03.036
Unune, D. R., Brown, G. R., and Reilly, G. C. (2022). Thermal based surface modification techniques for enhancing the corrosion and wear resistance of metallic implants: a review. Vacuum 203, 111298. doi:10.1016/j.vacuum.2022.111298
Uva Narayanan, C., Suya Prem, A. P., Manivasagam, G., and Ezhilmaran, V. (2023). Investigating the compression and fretting wear behaviour of FDM printed PLA samples for bone fixation. Adv. Mater. Process. Technol., 1–17. doi:10.1080/2374068X.2023.2206174
Wang, W., Zhang, B., Li, M., Li, J., Zhang, C., Han, Y., et al. (2021). 3D printing of PLA/n-HA composite scaffolds with customized mechanical properties and biological functions for bone tissue engineering. Compos. Part B Eng. 224, 109192. doi:10.1016/j.compositesb.2021.109192
Yumak, N., and Aslantaş, K. (2020). A review on heat treatment efficiency in metastable β titanium alloys: the role of treatment process and parameters. J. Mater. Res. Technol. 9 (6), 15360–15380. doi:10.1016/j.jmrt.2020.10.088
Zang, D., and Xun, X. (2021). “Ceramics coated metallic materials: methods, properties and applications,” in Advanced ceramic materials (China: IntechOpen). doi:10.5772/intechopen.93814
Zhu, Y., Liu, K., Deng, J., Ye, J., Ai, F., Ouyang, H., et al. (2019). 3D printed zirconia ceramic hip joint with precise structure and broad-spectrum antibacterial properties. Int. J. Nanomedicine 14, 5977–5987. doi:10.2147/IJN.S202457
Keywords: biomaterials, implants, bio-compatible, metal alloys, ceramics, polymers
Citation: S. AD, P. SPA, Naveen J, Khan T and Khahro SH (2024) Advancement in biomedical implant materials—a mini review. Front. Bioeng. Biotechnol. 12:1400918. doi: 10.3389/fbioe.2024.1400918
Received: 14 March 2024; Accepted: 31 May 2024;
Published: 03 July 2024.
Edited by:
Raghvendra kumar Mishra, Cranfield University, United KingdomReviewed by:
Hansa Mahajan, Ewha Womans University, Republic of KoreaCopyright © 2024 S., P., Naveen, Khan and Khahro. This is an open-access article distributed under the terms of the Creative Commons Attribution License (CC BY). The use, distribution or reproduction in other forums is permitted, provided the original author(s) and the copyright owner(s) are credited and that the original publication in this journal is cited, in accordance with accepted academic practice. No use, distribution or reproduction is permitted which does not comply with these terms.
*Correspondence: Suya Prem Anand P., c3V5YXByZW1hbmFuZC5wQHZpdC5hYy5pbg==; Tabrej Khan, dGtoYW5AcHN1LmVkdS5zYQ==
Disclaimer: All claims expressed in this article are solely those of the authors and do not necessarily represent those of their affiliated organizations, or those of the publisher, the editors and the reviewers. Any product that may be evaluated in this article or claim that may be made by its manufacturer is not guaranteed or endorsed by the publisher.
Research integrity at Frontiers
Learn more about the work of our research integrity team to safeguard the quality of each article we publish.