- 1Department of Chemistry, N.A.S.College, Meerut, India
- 2Guangxi Key Laboratory of Agricultural Resources Chemistry and Biotechnology, Agricultural College, Yulin Normal University, Yulin, China
- 3Faculty of Agricultural Sciences, GLA University, Mathura, India
- 4Department of Life Sciences and Biological Sciences, IES University, Bhopal, India
- 5College of Biotechnology, Chaudhary Charan Singh Haryana Agricultural University (CCSHAU), Hisar, India
- 6Department of Biotechnology, Graphic Era (Deemed to be University), Dehradun, India
- 7Department of Botany, K.S. Saket P.G. College, Ayodhya, India
- 8Division of Microbiology, Indian Agricultural Research Institute, New Delhi, India
- 9Centre of Advanced Study in Botany, Banaras Hindu University, Varanasi, India
- 10Department of Zoology, Maharaj Singh College, Maa Shakumbhari University, Saharanpur, India
- 11Amity Institute of Biotechnology, Amity University, Noida, India
The natural environment is often contaminated with hydrophobic pollutants such as long-chain hydrocarbons, petrochemicals, oil spills, pesticides, and heavy metals. Hydrophobic pollutants with a toxic nature, slow degradation rates, and low solubility pose serious threats to the environment and human health. Decontamination based on conventional chemical surfactants has been found to be toxic, thereby limiting its application in pharmaceutical and cosmetic industries. In contrast, biosurfactants synthesized by various microbial species have been considered superior to chemical counterparts due to their non-toxic and economical nature. Some biosurfactants can withstand a wide range of fluctuations in temperature and pH. Recently, biosurfactants have emerged as innovative biomolecules not only for solubilization but also for the biodegradation of environmental pollutants such as heavy metals, pesticides, petroleum hydrocarbons, and oil spills. Biosurfactants have been well documented to function as emulsifiers, dispersion stabilizers, and wetting agents. The amphiphilic nature of biosurfactants has the potential to enhance the solubility of hydrophobic pollutants such as petroleum hydrocarbons and oil spills by reducing interfacial surface tension after distribution in two immiscible surfaces. However, the remediation of contaminants using biosurfactants is affected considerably by temperature, pH, media composition, stirring rate, and microorganisms selected for biosurfactant production. The present review has briefly discussed the current advancements in microbially synthesized biosurfactants, factors affecting production, and their application in the remediation of environmental contaminants of a hydrophobic nature. In addition, the latest aspect of the circular bioeconomy is discussed in terms of generating biosurfactants from waste and the global economic aspects of biosurfactant production.
1 Introduction
Rapid industrialization and incessantly increasing anthropogenic activities have raised the concern of multifarious contaminant release as well as accumulation in the natural ecosystems, thereby adversely affecting the ecosystem functioning and human livelihood (Santos B. L. P. et al., 2023; Kabir et al., 2023; Silva et al., 2024). Although conventional methods such as landfilling, incineration, and oxidation–reduction have been widely practiced to mitigate such challenges, especially in developing countries (Hu et al., 2021), the limited land resources, high costs, and the deposition of toxic chemical residues in the environment have raised questions about the traditional mitigation approaches (Bhatt et al., 2022). At the same time, organic contaminants of a hydrophobic nature, such as oil spills, petrochemicals, and long-chain hydrocarbons, have posed significant adverse impacts due to their toxic nature, insolubility, low degradation rate, and high persistence (Kalia et al., 2022; Shaji et al., 2024).
Recently, microbial strains and products thereof have been used for the degradation of environmental contaminants (Kumar et al., 2021). Easy availability, high energy efficiency, lack of toxic residues, and cost effectiveness have made microbes a preferable resource for environmental management. Biosurfactants are one of the emerging microbial biomolecules widely used as lubricants, foaming softeners, dye fixers, emulsion makers, and dispersion stabilizers in various fields, including agriculture, pharmaceuticals, and environmental contaminant cleanup industries (Gharaie et al., 2023; Inès et al., 2023). In general, the term “biosurfactant” refers to biocompatible surface-active molecules with amphiphilic properties and hydrophilic and hydrophobic segments. Usually, the hydrophobic ends are composed of long fatty acids, while the hydrophilic part may be positively or negatively charged or of an amphoteric nature (Farias et al., 2021).
Biosurfactants can enhance interaction between different surfaces by forming micelles (Johnson et al., 2021), recognized for effectively reducing surface tension at air–water or oil–water interfaces (Ribeiro et al., 2020; Luz et al., 2023). When surfactants are introduced into systems comprising oil and water or water and air, surface tension is lowered. Beyond a certain concentration threshold, referred to as the critical micelle concentration (CMC) (Abooali and Soleimani, 2023), surfactants start to self-assemble into micelles (Figure 1). Micelle formation is a key mechanism facilitating a reduction in surface tension by biosurfactants (Patel et al., 2023). These properties enable them to effectively reduce interfacial tension between immiscible phases (Kumar et al., 2021).
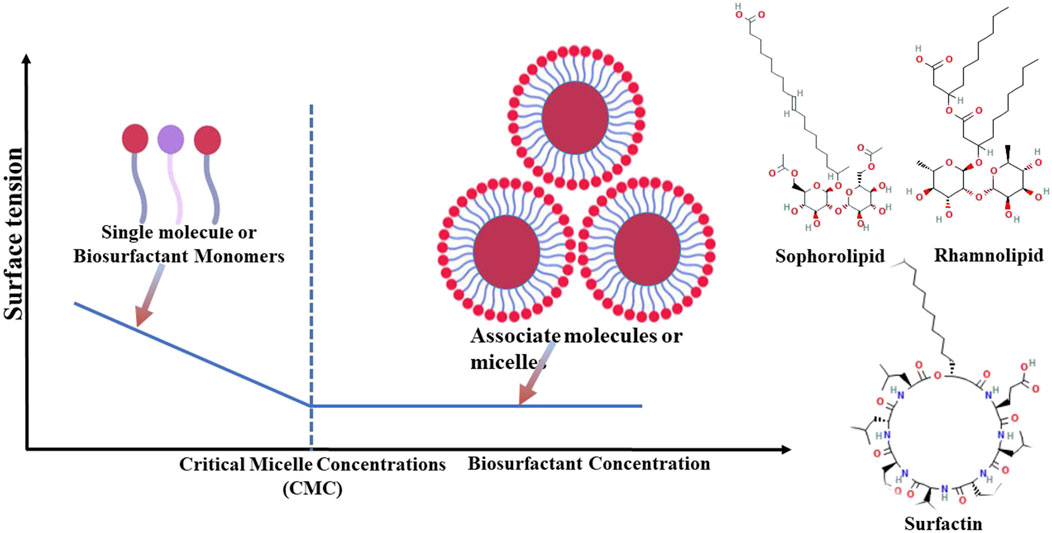
Figure 1. Representation of biosurfactants with their actions for critical micelle concentration and formation of biosurfactant monomers.
Recently, biosurfactants synthesized by microorganisms like bacteria and yeasts have garnered considerable attention for environmental contamination management (Kashif et al., 2022). For instance, Al-Marri et al. (2023) reported a strain of Marinobacter sp., isolated from an oil and gas industrial waste site, that had the ability to produce biosurfactants with a promising role in the biodegradation of hydrocarbons. Similarly, Bellebcir et al. (2023) reported the strain of Streptomyces thinghirensis as having considerable potential for hydrocarbon bioremediation. A list of biosurfactants produced by different microorganisms is presented in Table 1.
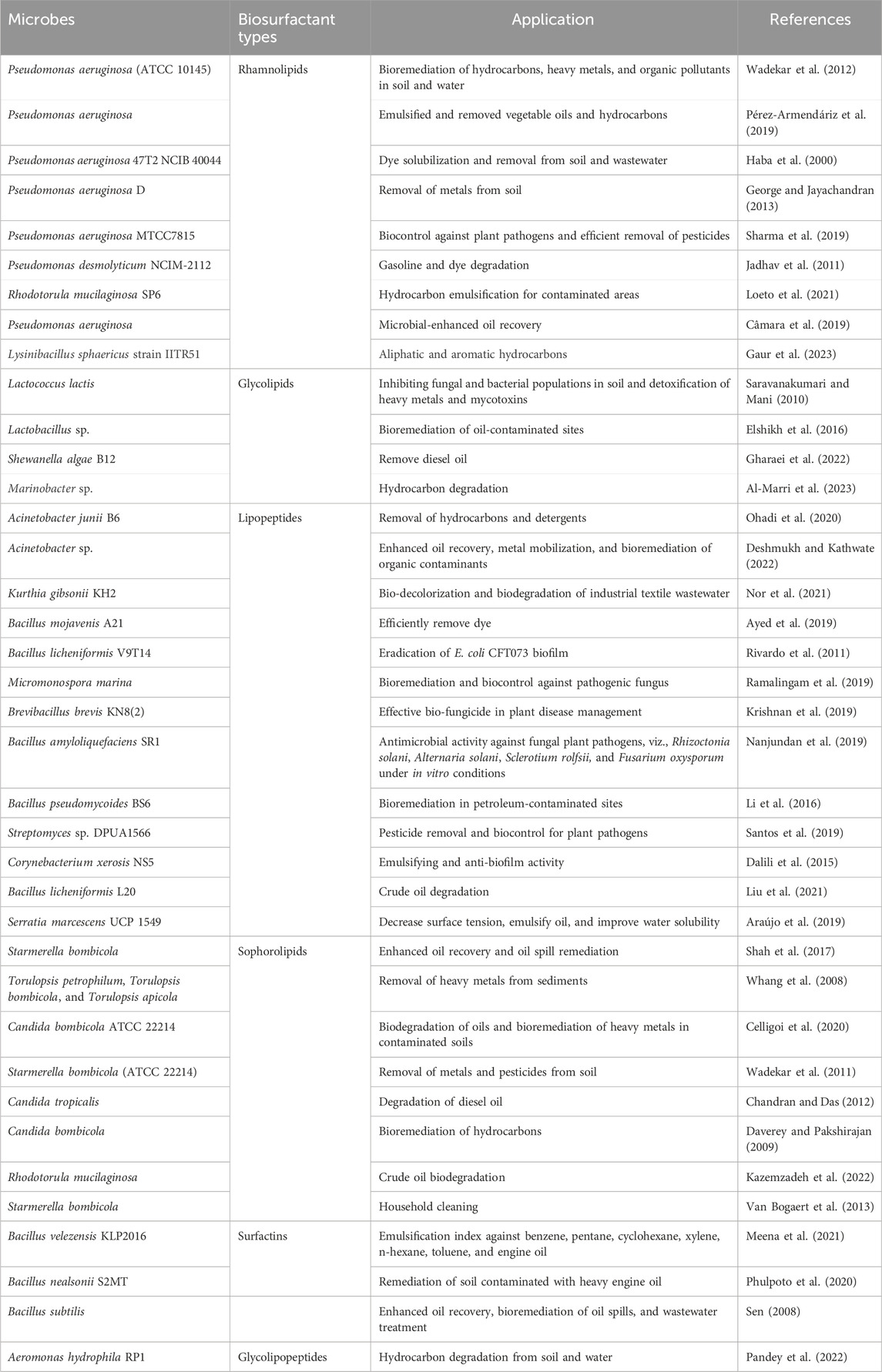
Table 1. Biosurfactant-producing microbes and their various applications in environmental management.
Biosurfactants have been used in different fields, including agriculture, neutraceuticals, pharmacology, cosmetics, and environmental contamination management (Figure 2) due to their ecofriendly and multifunctional properties, thus positioning them as the surfactants of the future (Karnwal, 2023). Microbially produced surfactants offer key advantages like enhanced temperature tolerance, stability at varying pH levels, lower toxicity, and better degradation potential than chemically synthesized surfactants (Karnwal, 2023).
The present review has briefly discussed the current advancements in microbially synthesized biosurfactants, factors affecting production, and the application in remediation of environmental contaminants of hydrophobic nature. In addition, the latest aspects of the circular bio-economy, generating biosurfactants from wastes, and the global economic aspect of biosurfactant production are discussed.
2 Role of microorganisms in biosurfactant production
In the recent past, various microbes, including bacteria, fungi, and yeast strains, have been used to synthesize biosurfactants. To achieve optimal biosurfactant production, the optimization of different parameters is required. Various factors like the choice of microbial strains, the additives used in the growth medium, the substrate, and other intrinsic and external factors like temperature, growth conditions, and pH have been considered for the efficient production of biosurfactants (Kashif et al., 2022). The selection of the microbial strain is an initial and crucial step during the process of biosurfactant production. Optimum production of biosurfactants has been observed during the exponential or stationary growth stages of microbial cultures, especially with limited nutritional resources. At this stage, microorganisms can synthesize biosurfactants either intracellularly (within the cell) or extracellularly (outside the cell) (Kashif et al., 2022). Previously, authors have reported biosynthetic pathways and genetic regulation of biosurfactant-producing microorganisms (Sarubbo et al., 2022). Note that biosurfactants derived from different microorganisms can exhibit variations in their composition and functionality. The properties and classification of biosurfactants can vary on the basis of the origin or methods employed during microorganism isolation. Microorganisms isolated from contaminated environments exhibit a unique attribute to degrade specific chemical pollutants as they can use contaminants as an energy source, thereby allowing them to thrive in harsh environmental conditions (Yesankar et al., 2023). Optimization of growth conditions, isolation methods, and selection of potential microbial strains is crucial for harnessing the potential of biosurfactants for diverse applications ranging from environmental remediation to industrial processes.
The current research focuses on identifying and characterizing microbial strains with superior biosurfactant-producing capabilities (Schultz and Rosado, 2020). Additionally, the choice of substrate, such as the carbon source provided to the microorganisms, can significantly impact biosurfactant production. Some microorganisms have been reported to exhibit enhanced biosurfactant production after exposure to specific substrates, while others may prefer alternative carbon sources (Karnwal, 2023). Therefore, tailoring the growth medium to suit the specific needs of the chosen microbial strain is essential for maximizing production. Moreover, genetic modification and synthetic biology have opened up new opportunities to engineer microorganisms in view of enhancing the quality and quantity of biosurfactants (Yesankar et al., 2023).
Although the exact physiological mechanisms behind biosurfactants are not fully understood, it is believed that biosurfactants enhance cellular motility, promote biofilm formation, and improve nutrient uptake from hydrophobic substrates (Yesankar et al., 2023). However, to gain a comprehensive understanding of biosurfactant molecules, it is crucial to develop efficient and accurate methods for isolation and screening of microbial strains (Karnwal, 2023). Numerous studies have reported biosurfactants produced by microbial strains. For instance, rhamnolipid synthesized by the bacterium Pseudomonas aeruginosa has been extensively studied and is reported to reduce the surface tension and enhance the emulsification of hydrophobic compounds (Conceição et al., 2020). Other classes of biosurfactants, such as glycolipids and lipopeptides, have also been reported for their effectiveness in reducing surface tension and enhancing the emulsification of hydrophobic pollutants (Kashif et al., 2022). A list of potential microbes synthesizing biosurfactants is presented in Table 1.
The functional behavior of biosurfactants to enhance the availability of hydrophobic compounds and improve microbial activities in challenging environments has garnered significant attention in both scientific and industrial communities (Karnwal, 2023). These versatile compounds have found diverse applications in areas such as bioremediation, agriculture, and healthcare, highlighting their importance. Continued research on biosurfactants, including the exploration of new microbial strains and improved production methods, therefore, promises to unlock their full potential for addressing environmental and industrial challenges.
3 Classification of biosurfactants
Biosurfactants are commonly classified according to their molecular weight, CMC, microorganisms responsible for their production, and their mechanism of action. In general, biosurfactants can be classified into two groups based on molecular weight. The first group is high molecular weight (HMW) biosurfactants, which are typically composed of intricate biopolymers like polysaccharides and lipopolysaccharides, with a diverse assortment of biopolymers (Hassanshahian et al., 2020). For instance, some HMW biosurfactants, like rhamnolipids synthesized by the strain Pseudomonas aeruginosa, have shown promise in applications related to enhanced oil recovery due to their ability to form stable emulsions with hydrocarbons (Chafale and Kapley, 2022). The second group is low molecular weight (LMW) biosurfactants like glycolipids and lipopeptides, which are known for their simpler chemical structures and applied in diverse fields. For example, glycolipids like sophorolipids produced by yeasts have been explored for their emulsification and antimicrobial properties, thereby making them a potential candidate for use in various formulations (Liepins et al., 2021). It has been assumed that large molecular-weight biosurfactants excel in producing stable emulsions, while low molecular-weight biosurfactants produced by microorganisms are efficient at lowering surface tension (Jahan et al., 2020). Details of these classifications are presented in Figure 3.
4 Factors influencing the generation of biosurfactants
Developing, identifying, and characterizing microbial strains are the initial steps in biosurfactant production. However, to ensure successful biosurfactant production, consistent growth conditions and energy sources such as carbon and nitrogen are required (Sarubbo et al., 2022). The factors influencing the generation of biosurfactants are presented in Figure 4.
Scaling to industrial-level production of biosurfactants requires careful consideration of methodology, chief energy resources, substrate selection, and recovery processes (Jimoh and Lin, 2019). As microbes require different energy sources for their growth, their optimal concentration must be known for increased biosurfactant production (Karnwal, 2023). Previous studies have reported different energy resources for biosurfactant production (Kumar et al., 2021; Kanaoujiya et al., 2022). Production expenses are heavily dependent on raw material supply; however, conventional and ready-made sources of carbon and nitrogen are too expensive for industrial production (Priyani and Munir, 2021).
Significant progress has been made in biosurfactant production, and researchers have continuously examined and tested different energy sources. Studies have used different chief C and N sources either singly or in conjugation with well-established energy sources like glucose and NH4+ NO3−. (Agarwal and Sharma, 2009; Bajelani et al., 2023). Such resources include residual waste products, such as food industry waste products, oil wastes, and agro waste. Utilization of such energy resources is cost-effective and reduces production costs by a significant percentage (Santos et al., 2023b; dos Remedios Araújo Vieira Neta et al., 2023; Mallik and Banerjee, 2022; Sarubbo et al., 2022).
In this context, various authors have reported different low-cost alternative sources of carbon and nitrogen to efficiently synthesize biosurfactants. For example, Santos B. L. P. et al. (2023) used pineapple peel as a substrate to produce biosurfactants from the Bacillus subtilis strain. dos Remedios Araújo Vieira Neta et al. (2023) evaluated brewer’s spent grain as a carbon source to produce glycolipid biosurfactant from the yeast strain Rhodotorula mucilaginosa LBP5. The strain produced a significant amount of biosurfactants having a critical micelle concentration equivalent to 1.5 g/L. Soyuer and Bilen Ozyurek (2023) used low-cost potato waste water as a substrate to produce the biosurfactants from the Bacillus subtilis strain. Safari et al. (2023) used rice bran oil as a substrate to produce rhamnolipids from P. aeruginosa. Shahabi Rokni et al. (2022) evaluated and optimized sugar beet molasses and other carbon sources for the efficient production of biosurfactants. In a similar fashion, Bouassida et al. (2023) evaluated the different concentration of glucose and their impact on biosurfactant production. Hentati et al. (2019) reported 50 mg L−1 of biosurfactants produced by strain Bacillus stratosphericus using leftover frying oil as an inexpensive supply of carbon.
5 Physical factors involved in biosurfactant production
The synthesis of biosurfactants also depends upon several physical factors like temperature, pH, rate of rotation, and culture growth duration; these factors directly influence microbial growth (Ilori et al., 2005; Al-Bahry et al., 2013). Therefore, physical variables such as the temperature, media, pH, and rate of rotations have been optimized. The temperature largely influences microbial growth, and each of the microbial species showed optimum growth at a particular temperature. For example, the production of glycolipids biosurfactant from candida sp. was reported at the temperature of 27°C–30°C (Santos et al., 2016). However, several studies reported an optimum temperature range of 22°C–30°C for biosurfactant production from the bacterial strains isolated from the open ocean (Twigg et al., 2018; Tripathi et al., 2019).
An optimum pH range is also required for biosurfactant production from the microbial species. According to Jimoh and Lin (2019), the optimum biosurfactant recovery for the pH ranges generally observed in the previous studies was between 5.7 and 7.8. Auhim and Mohamed (2013) investigated Azotobacter chroococcum’s ability to produce BioS under controlled nutritional and environmental conditions and found that maintaining a pH of around 7 resulted in the highest surface tension reduction of 68% and emulsification index (EC24). Souza et al. (2018) reported 10 g L−1 of biosurfactant produced by the strain Wickerhamomyces anomalus using olive oil as an energy source under optimal growth conditions. The surfactant showed stability even at 121°C, a salinity concentration of 300 gL−1, and pH ranging from 6 to 12. Chooklin et al. (2023) reported the bioremediation potential of glycolipid biosurfactant-producing strain Bacillus oceanisediminis. The authors reported an emulsification power of glycolipid equivalent to 65% and a surface tension of 22.67 mN/m after 60 h of cultivation. The proper aeration of the culture is also required for optimum production. The proper aeration at the rotatory shaker directly influences the growth of aerobic microorganisms. The optimum production of biosurfactants was reported at the rotational speed between 50 and 250 rpm (Oliveira et al., 2009; Silvia et al., 2023). However, it has also been reported that faster rotation favors more biosurfactant production (Santos et al., 2016; Twigg et al., 2018).
The incubation time of the biosurfactant-producing microorganisms also plays a crucial role in the synthesis and recovery of biosurfactants. The incubation time varies among the different microbial strains due to different growth rates and growth stages (Santos et al., 2016). A duration of 18–48 h is considered optimum, but in several cases, the incubation time reported is 88–120 h (Felse et al., 2007; Campos et al., 2019). Similarly, the size of the inoculum also plays a significant role in biosurfactant production. In the previous study, the author reported that higher cell density of microbes favors optimum biosurfactant production (Twigg et al., 2018).
6 Role of microbial surfactants in environmental management
Diverse microbial entities are known to play an important role in the successful elimination of both organic and inorganic contaminants affecting environmental homeostasis. Microbial processes and products are of considerable interest in the sequestration of individual and mixtures of contaminants. Microbially synthesized surfactants are described as multifunctional candidate molecules with promising potential in environmental management (Fenibo et al., 2019). To date, varied microbial surfactants of prokaryotic as well as eukaryotic origin and low to high molecular weight have been registered (Lang, 2002). In general, the biologically produced surfactants are of a glycolipid nature, possessing sugars like rhamnose and trehalose. The role of microbially produced surfactants in the remediation of environmental contaminants is presented in the following sections.
6.1 Heavy metal and metalloid remediation
Contamination with hazardous heavy metals and metalloids originating from different natural and anthropogenic sources is an increasing concern to human health and the natural environment worldwide (Singh et al., 2021a; Singh et al., 2021b). Important heavy metals and metalloids that are an increasing risk to terrestrial and aquatic environments include nickel, zinc, copper, cadmium, aluminum, chromium, arsenic, and mercury. As the presence of metal contaminants above the threshold limit has a detrimental impact on exposed life forms (Gupta et al., 2021; Paithankar et al., 2021; Zamora-Ledezma et al., 2021), their elimination from affected sites using sustainable approaches is imperative. In this connection, the contribution of microbially synthesized surfactants in the ecofriendly remediation of heavy metal and metalloid contaminated sites is quite promising (Wang and Mulligan, 2009; Rastogi et al., 2021). The heavy metal sequestration potential of surfactant-producing Pseudomonas sp. CQ2 was recently demonstrated by Sun et al. (2021). The oilfield-isolated Pseudomonas with maximum biosurfactant production determined as 40.7 g/L exhibited 8.7%, 65.7%, and 56.9% greater removal of cadmium, copper, and lead, respectively, than chemically synthesized surfactants, suggesting application in bioremediation of contaminated sites. The study showed the involvement of the carboxyl group of biosurfactants in heavy metal chelation and subsequent elimination. The rhamnolipid surfactant produced by Pseudomonas aeruginosa strain in the sequestration of lead and mercury from sediment samples has recently been reported (Chen et al., 2021). The biosurfactant at 43.73 mg L−1 concentration was 62.5% and 50.2% more effective in removing lead and mercury, respectively, than the common surfactant sodium dodecyl sulfate. The alteration in sediment characteristics after the application of surfactant was noted as an important mechanism responsible for metal removal. Approximately three-fold enhancement in cadmium removal after supplementation of 4 mmol kg−1 biosurfactants, namely, rhamnolipids and saponins, was reported by Mekwichai et al. (2020). The rhamnolipid favored greater uptake of cadmium than saponin. Investigation into the capability of rhamnolipid biosurfactant in combination with a glutamic acid derivative for the elimination of heavy metals from sludge waste using an electrokinetic approach was presented by Tang et al. (2017). The synergistic action of biosurfactant and glutamic acid derivative led to enhancement in the removal of copper, zinc, chromium, lead, nickel, and manganese from 60% to approximately 90% during the treatment process. The glycolipid biosurfactant secreting bacterial strain Burkholderia sp. Z-90 recovered from sewage sludge has demonstrated effectiveness in the removal of zinc, lead, manganese, copper, cadmium, and arsenic (Yang et al., 2016), ranging from 24.1% to 44%, with minimum removal observed for copper. The interaction of soil minerals with bacteria and the complexation of metal with biosurfactant released was ascribed as the plausible mechanism for the elimination of targeted contaminants. The study indicated the influence of metal speciation on biosurfactant-assisted removal. The amount of biosurfactant synthesized was quite low, thereby restricting broad-scale application for remediation of metal and metalloid contaminated sites.
6.2 Pesticide remediation
Increased demand for agricultural products because of the increasing human population, along with the huge postharvest losses of crop produce, has increased the application of diverse hazardous pesticides. The continuous agricultural application of different pesticides has badly affected the aquatic and terrestrial environments (Barbieri et al., 2021; Bijlsma et al., 2021; Egbe et al., 2021; Pérez et al., 2021). The employment of microbially produced surfactants can be viewed as a sustainable tool for the substantial removal of pesticides without compromising the characteristics of the natural environment. Isolation of biosurfactant synthesizing bacteria identified as Pseudomonas and Rhodococcus causing the degradation of chlorpyrifos has been described recently (Lamilla et al., 2021). The glycolipid and glycopeptide nature of produced biosurfactants were suggested by chemical analyses, and one of the surfactants produced by Pseudomonasrhodesiae strain 4 was illustrated to improve the degradation of pesticide by more than 10%. Pesticides with an increased hydrophobic nature could be difficult to treat because of lesser solubility and reduced bioavailability. Increasing the solubility of hydrophobic pesticides using the anionic rhamnolipid biosurfactant, which is stable at a broad range of pH, temperature, and salt and is produced by metal-resistant Lysinibacillus sphaericus strain IITR51, is described by Gaur et al. (2019). The introduction of 90 mg/L microbially produced surfactant was observed to induce the dissolution of endosulfan and hexachlorocyclohexane, ranging from 1.8 to 7.2 fold. Biosurfactants are documented to increase the solubility of hydrophobic pesticides (García-Reyes et al., 2018). The experimental investigation suggested an enhancement in the solubility of pesticides, including endosulfan and methyl parathion, after supplementation of biosurfactant of glycolipid nature originating from Pseudomonas sp. strain B0406. Another study on the application of Pseudomonas sp. (ChlD)-derived rhamnolipid biosurfactant equivalent to 0·1 g L−1 has demonstrated the degradation of pesticide chlorpyrifos by nearly 100% (Singh et al., 2009) after 120-h incubation as confirmed by gas and liquid chromatography. In this connection, the isolation of newer bacterial strains with the simultaneous ability of pesticide degradation and biosurfactant production could be a viable approach for field application to manage organic contaminant-affected sites. The basic mechanism pertaining to improved biological degradation of hydrocarbons lies in 1) accelerated solubility of the contaminant utilized as the substrate 2) and surfactant-assisted increment in microbial cell surface hydrophobicity, facilitating the interaction with the contaminant (Mulligan and Gibbs, 2004). Moreover, the presence of a biosurfactant resulted in an increased surface area of less-soluble contaminants due to the diminution of surface as well as interfacial tension, leading to the increased mobilization and biological availability of targeted contaminants.
6.3 Petroleum, hydrocarbon, and oil contaminant remediation
Oil spills can destroy environmental quality, making terrestrial and aquatic environments unfavorable for different life forms (Samanta et al., 2002; Philibert et al., 2019; Wei et al., 2020). The present-day physico-chemical technology for the remediation of petrochemicals, hydrocarbons, and oil spills is expensive and detrimental to natural environmental characteristics. Therefore, ecofriendly routes, especially microbial ones, should be developed for the successful elimination of contaminants. Surfactant-producing microorganisms are known for the degradation of petrochemicals, hydrocarbons, and oil contaminants. The action mechanism of the biosurfactant molecules in the degradation of petrochemicals or hydrocarbons is a complex process. The use of biosurfactants generally reduces the surface and interfacial tension between the water and hydrocarbon molecules, resulting in increased emulsification and dispersion of hydrocarbons in water. In addition, the biosurfactant molecules form micelles over the hydrophobic petrochemicals or the hydrocarbon molecules, which further increases the solubility of these hydrophobic contaminants in water. These processes increase the bioavailability and solubility of hydrophobic pollutants and are also crucial for microbial degradation (Desai and Banat, 1997).
Some studies have reported that the biosurfactant molecules facilitate the formation of biofilms over the hydrocarbon surfaces. Biofilms are microbial communities embedded in a self-produced extracellular matrix that enhances the stability and efficiency of microbial consortia in degrading pollutants (Zhang and Miller, 1994). It has also been reported that some biosurfactant molecules interact directly with hydrocarbons, initiating chemical changes that make them more susceptible to microbial attack. This can involve processes like oxidation or the breaking of complex hydrocarbon chains into simpler compounds (Rahman et al., 2002).
Previously published reports have reported the role of microbial-synthesized biosurfactants in the bioremediation of petrochemicals, hydrocarbons, or oil spills. Pandey et al. (2024) reported the glycolipid surfactant produced by the Citricoccus zhacaiensis strains, which showed an effective role in the degradation of petrochemical hydrocarbons. The strain showed ≥95% degradation of hydrocarbon pollutants in a 2-week period. Csutak et al. (2024) reported the role of biosurfactant produced by the yeast strain Yarrowia lipolytica CMGB32 in the remediation or degradation of n-hexadecane petrochemical pollutants. Li et al. (2024) also reported the effective role of biosurfactant lipopeptides produced by Raoultella planticola in the degradation of n-hexadecane-contaminated soil.
The contribution of Bacillus velezensis KLP2016 synthesizing a biosurfactant designated as surfactin is noted for the degradation of engine oil (Meena et al., 2021). The presence of biosurfactants at 35°C and 25°C lowered the surface tension of a cell-free medium, pointing toward their application for enhanced biodegradation of engine oil. The media supplementation with different carbon and nitrogen substrates may be tested for further improvement in biosurfactant production and consequent biodegradation. The biosurfactant purified by electrospray ionization coupled with mass spectroscopy revealed a molecular weight equivalent to approximately one kilo Dalton. Recently, the increased remediation of oil sludge based on the composite biosurfactant of lipopeptide (anionic) and sophorolipid (non-ionic) nature employed as a washing agent compared to a single treatment was demonstrated (Bao et al., 2021). Lipopeptide and sophorolipid biosurfactants with a mass ratio of 8:2 exhibited reduced CMC and displayed maximum synergistic action with optimum removal under defined washing conditions of temperature, shaking, treatment time, and sludge to-liquid proportion. In addition, the study also proposed the influence of minerals on oil sludge treatment. The CMC was found to be lower than the standard value. The involvement of P. aeruginosa and Meyerozyma sp. producing a surfactant with a rhamnolipid (critical micelle concentration 40 mg/L) and sophorolipid (critical micelle concentration 50 mg/L) nature, respectively, in crude oil degradation was presented by Rehman et al. (2021). The synthesized biosurfactants were stable at varying pH, temperature, and salt concentrations and led to the 87% to 91% degradation of petroleum hydrocarbons. The biosurfactants were shown to hold promising avenues in the decontamination of oil-contaminated soils. Lai et al. (2009) registered superior oil remediation activity of biosurfactants, namely, rhamnolipids and surfactin, in contrast to the chemically synthesized surfactants Tween 80 and Triton X-100. At 0.2% content of selected biosurfactants and synthetic surfactants, the percent removal of petroleum hydrocarbon ranged from 35% to 63%, with the maximum removal recorded for biosurfactants and the minimum for synthetic surfactants. The analysis suggested an increase in the removal of total petroleum hydrocarbons with increased surfactant and contaminant concentrations.
In contrast to chemical surfactants, biosurfactants are ecofriendly in nature, cost-effective, biodegradable, less toxic, have a large number of functional groups, and do not produce secondary pollutants (Chen et al., 2011; Mao et al., 2015). Moreover, the biosurfactant could have greater chemical diversity and potential in the cleanup of contaminated sites, implying utilization for extensive bioremediation purposes. However, the microbes used for surfactant production followed by contaminant removal may suffer from challenges imposed by environmental variables like pH, temperature, and nutritional requirements. The changing environmental conditions may considerably influence the survivability of microbes as well as the optimum production of biosurfactants. Moreover, the production of biosurfactants by a given microorganism varies considerably from one species to another, implying the isolation of suitable species for efficient remediation. Therefore, process optimization to facilitate the maximum biosurfactant production and subsequent remediation of the contaminant of interest is necessary for harnessing the potential of diverse microbially originated surfactants.
7 Biosurfactant production from waste: a new aspect in the circular bio-economy
In the last two decades, the use of microbial strains for biosurfactant production has been gaining momentum due to its ecofriendly nature, low cost of production, and the easy availability of microorganisms. However, the rate of commercial production of biosurfactants is still very slow due to the high cost of media or the resource materials (Kumar et al., 2021). During biosurfactant production, the optimum growth of microorganisms requires carbon, nitrogen, or energy resources. Although in laboratory conditions, the regular media used for microbial growth does not appear as a hurdle, on a larger or industrial scale, the cost of media or energy resources is a major challenge (Eras-Muñoz et al., 2022; Mgbechidinma et al., 2022).
Currently, research communities are working to find an alternative to the regular media, especially for carbon, nitrogen, or energy resources. Currently, various alternative sources, such as vegetable waste products, agricultural residues, and fruit waste, have been used as alternative media sources for the growth of microorganisms or biosurfactant products (Kumar et al., 2021; Ciurko et al., 2022). Agricultural waste, fruit waste, molasses, sugarcane waste products, sunflower oils, cake bakery products, soybeans, potato peels, etc., have been frequently utilized as an alternative source of the regular media for biosurfactant production. In agricultural waste, rice husk, wheat straw, sugarcane bagasse, and maize cobs are the frequently used alternative sources practiced for biosurfactant production (Kumar et al., 2021; Ciurko et al., 2022; Santos C. V. M. et al., 2023). Optimization of media and the compatibility of microorganisms are priorities for agricultural waste selection (Silva et al., 2024).
The high nutrient contents and rich availability of carbon, nitrogen, protein, and lipid sources in fruit waste offer an alternative or rich media source for biosurfactant production (Kumar et al., 2021; Varjani et al., 2021; Gaur et al., 2022) Generally fruits peels, pulps, and seeds have been considered fruit waste. In recent years, various authors have reported the optimum production of biosurfactants utilizing fruit waste products (Sharma and Pandey, 2020). In current biosurfactant products, various by-products of the sugarcane industries, such as corn, molasses, and sugar beet, have been used due to their high starch and sucrose content, which provide ample supplements for microbial growth (Kumar et al., 2021).
8 The latest global economic aspects of biosurfactant production
Despite the broad and environmentally friendly application of biosurfactants, the limited and expensive nature of raw materials hinders biosurfactant production at the industrial level (Saisa-Ard et al., 2013; Henkel et al., 2017). However, the global trend of biosurfactant production increased significantly in 2022, where the market value of biosurfactants was $1.9 billion, and it is expected to reach $3.2 billion by 2032, with a 5.4% compound annual growth rate (CAGR) growth (https://www.alliedmarketresearch.com/biosurfactant-market).
Europe is currently the largest emerging market for biosurfactants, followed by the United States. The demand for biosurfactants is also growing in Asian countries due to increased infrastructure and awareness about the environmental benefits (Singh et al., 2019). Their rise in the European market is due to the demand in the personal care or cosmetics industries. The major uses of biosurfactants have been reported in the detergent industries, followed by personal care and agricultural chemicals in 2022 (https://www.marketsandmarkets.com/). Evonik Industries AG (Germany), Deugan Biosurfactant supplier (China), and Saraya Co. Ltd. (Japan), etc., are the key market players in the industry (https://www.marketsandmarkets.com/).
Approximately 30%–50% % of total biosurfactant production cost is due to the price of raw materials (Saisa-Ard et al., 2013; Kumar et al., 2021). Successfully transitioning waste products, including agricultural waste, sugarcane waste products, and fruit or vegetable waste, as a carbon or energy source in a circular economy is essential for balancing industrial growth, economic development, and environmental protection. This approach also enhances the strategic use of resources (Varjani et al., 2021; Gaur et al., 2022).
9 Challenges and opportunities for microbial biosurfactant production
The application of biosurfactants as an alternative to fossil-derived surfactants has the potential to diminish carbon dioxide emissions by 8%, amounting to 1.5 × 10 t (Rocha e Silva et al., 2019; Farias et al., 2021). The reduced carbon emissions underscore the importance of biosurfactants in view of sustainable development goal 13. Biosurfactants are receiving considerable attention from scientists worldwide in the pharmaceutical and oil recovery industries because of their inherent properties to increase the solubility and availability of drugs in an aqueous environment (Das and Rao, 2024; Pannu et al., 2024; Purwasena et al., 2024; Rehman et al., 2024). Recently, a biosurfactant released from the yeast Scheffersomyces shehatae has demonstrated the potential for application in cosmetic products (Cedrola et al., 2024). The employment of diverse waste resources of a biological nature for the synthesis of biosurfactants has the potential to restrict the cost of waste disposal (Tang et al., 2023). Thus, the exploitation of waste to support the production of valuable resources strengthens the concept of circular economy (Venkataraman et al., 2024). Furthermore, the successful utilization of waste resources may minimize hazardous impacts on ecosystem integrity.
10 Conclusions
Continuously increasing industrial activities release large numbers of contaminants into the environment and severely affect the soil and water ecosystem through the accumulation of chemicals, industrial effluents, oil spills, etc. The toxic nature and long-term persistence of industrial pollutants pose severe health risks to human beings, and their sustainable mitigation is a serious concern of government and policymakers. A wide range of traditional physical and chemical methods have been followed to mitigate the challenges of industrial pollutants. However, pollutants having a hydrophobic nature, such as hydrocarbons, oil spills, and pesticides, are difficult to manage using traditional methods. In this regard, biosurfactants are being considered for degrading contaminants of a hydrophobic nature. Although biosurfactants produced by microorganisms are intrinsically economical, the need for carbon and nitrogen-based energy resources required for microbial growth is costly. Therefore, there is an urgent need to harness alternative energy sources, appropriate microbial candidates, and growth conditions to optimize the synthesis of biosurfactants by microbes in a sustainable and economical way.
Author contributions
NS: writing–original draft and writing–review and editing. X-HH: funding acquisition, writing–original draft, and writing–review and editing. MS: investigation, supervision, validation, writing–original draft, and writing–review and editing. AK: writing–original draft and writing–review and editing. VS: formal analysis, validation, visualization, writing–original draft, and writing–review and editing. SS: writing–original draft and writing–review and editing. PY: writing–original draft and writing–review and editing. RS: writing–original draft and writing–review and editing. NB: writing–original draft and writing–review and editing. ZW: visualization, writing–original draft, and writing–review and editing. AK: conceptualization, investigation, supervision, visualization, writing–original draft, and writing–review and editing.
Funding
The author(s) declare that financial support was received for the research, authorship, and/or publication of this article. We are grateful for the support provided by the Scientific Startup Foundation for Doctors of Yulin Normal University (grant numbers G2019ZK32 and G2020ZK13).
Acknowledgments
The author (AK) is thankful to the Amity Institute of Biotechnology, Amity University, for providing lab facilities.
Conflict of interest
The authors declare that the research was conducted in the absence of any commercial or financial relationships that could be construed as a potential conflict of interest.
The author(s) declared that they were an editorial board member of Frontiers, at the time of submission. This had no impact on the peer review process and the final decision.
Publisher’s note
All claims expressed in this article are solely those of the authors and do not necessarily represent those of their affiliated organizations, or those of the publisher, the editors, and the reviewers. Any product that may be evaluated in this article, or claim that may be made by its manufacturer, is not guaranteed or endorsed by the publisher.
References
Abooali, D., and Soleimani, R. (2023). Structure-based modeling of critical micelle concentration (CMC) of anionic surfactants in brine using intelligent methods. Sci. Rep. 13, 13361. doi:10.1038/s41598-023-40466-1
Agarwal, P., and Sharma, D. K. (2009). Studies on the production of biosurfactant for the microbial enhanced oil recovery by using bacteriaisolated from oil contaminated wet soil. Pet. Sci. Technol. 27, 1880–1893. doi:10.1080/10916460802686640
Al-Bahry, S. N., Al-Wahaibi, Y. M., Elshafie, A. E., Al-Bemani, A. S., Joshi, S. J., Al-Makhmari, H. S., et al. (2013). Biosurfactantproduction by Bacillus subtilis B20 using date molasses and its possible application in enhanced oil recovery. Int. Biodegr. 81, 141–146. doi:10.1016/j.ibiod.2012.01.006
Al-Marri, S., Eldos, H. I., Ashfaq, M. Y., Saeed, S., Skariah, S., Varghese, L., et al. (2023). Isolation, identification, and screening of biosurfactant-producing and hydrocarbon-degrading bacteria from oil and gas industrial waste. Biotechnol. Rep. 39, e00804. doi:10.1016/j.btre.2023.e00804
Araújo, H. W., Andrade, R. F., Montero-Rodríguez, D., Rubio-Ribeaux, D., Alves da Silva, C. A., and Campos-Takaki, G. M. (2019). Sustainable biosurfactant produced by Serratia marcescens UCP 1549 and its suitability for agricultural and marine bioremediation applications. Microb. Cell Factories. 18, 2–13. doi:10.1186/s12934-018-1046-0
Auhim, H. S., and Mohamed, A. I. (2013). Effect of different environmental and nutritional factors on biosurfactant production from Azotobacter chroococcum. Int. J. Adv. Pharm. Biol. Chem. 2, 477–481.
Ayed, H. B., Azabou, M. C., Hmidet, N., Triki, M. A., and Nasri, M. (2019). Economic production and biocontrol efficiency of lipopeptide biosurfactants from Bacillus mojavenis A21. Biodegradation 30, 273–286. doi:10.1007/s10532-018-9864-7
Bajelani, S., Enayatizamir, N., Agha, A. B. A., and Sharifi, R. (2023). Potential of some microbial isolates on diesel hydrocarbons removal, bio surfactant production and biofilm formation. J. Environ. Health Sci. Eng. 21, 417–428. doi:10.1007/s40201-023-00868-9
Bao, Q., Huang, L., Xiu, J., Yi, L., and Ma, Y. (2021). Study on the treatment of oily sludge in oil fields with lipopeptide/sophorolipid complex bio-surfactant. Ecotoxicol. Environ. Saf. 212, 111964. doi:10.1016/j.ecoenv.2021.111964
Barbieri, M. V., Peris, A., Postigo, C., Moya-Garcés, A., Monllor-Alcaraz, L. S., Rambla-Alegre, M., et al. (2021). Evaluation of the occurrence and fate of pesticides in a typical Mediterranean delta ecosystem (EBRO RIVER delta) and risk assessment for aquatic organisms. Environ. Pollu. 274, 115813. doi:10.1016/j.envpol.2020.115813
Bellebcir, A., Merouane, F., Chekroud, K., Bounabi, H., Vasseghian, Y., Kamyab, H., et al. (2023). Bioprospecting of biosurfactant-producing bacteria for hydrocarbon bioremediation: optimization and characterization. Korean J. Chem. Eng. 40, 2497–2512. doi:10.1007/s11814-023-1418-y
Bhatt, P., Bhandari, G., and Bilal, M. (2022). Occurrence, toxicity impacts and mitigation of emerging micropollutants in the aquatic environments: recent tendencies and perspectives. Environ. Chem. Eng. 10, 107598. doi:10.1016/j.jece.2022.107598
Bijlsma, L., Pitarch, E., Hernández, F., Fonseca, E., Marín, J. M., Ibáñez, M., et al. (2021). Ecological risk assessment of pesticides in the Mijares River (eastern Spain) impacted by citrus production using wide-scope screening and target quantitative analysis. J. Hazard. Mater. 412, 125277. doi:10.1016/j.jhazmat.2021.125277
Bouassida, M., Mnif, I., and Ghribi, D. (2023). Enhanced biosurfactant production by Bacillus subtilis SPB1 using developed fed-batch fermentation: effects of glucose levels and feeding systems. Bioprocess Biosyst. Eng. 46, 555–563. doi:10.1007/s00449-022-02839-0
Câmara, J. M. D. D. A., Sousa, M. A. D. S. B., Barros Neto, E. L. D., and Oliveira, M. C. A. D. (2019). Application of rhamnolipid biosurfactant produced by Pseudomonas aeruginosa in microbial-enhanced oil recovery (MEOR). J. Pet. Explor. Prod. Technol. 9, 2333–2341. doi:10.1007/s13202-019-0633-x
Campos, J. M., Stamford, T. L. M., and Sarubbo, L. A. (2019). Characterization and application of a biosurfactant isolated from Candida utilis in salad dressings. Biodegradation 30, 313–324. doi:10.1007/s10532-019-09877-8
Cedrola, C. C., Barbosa, F. G., Marcelino, P. R. F., da Silva, N. P., Tavares, G. D., Barradas, T. N., et al. (2024). Unlocking the beauty benefits: exploring biosurfactants from Scheffersomyces shehatae for cosmetics. J. Surfactants Deterg. doi:10.1002/jsde.12739
Celligoi, M. A. P., Silveira, V. A., Hipolito, A., Caretta, T. O., and Baldo, C. (2020). Sophorolipids: a review on production and perspectives of application in agriculture. Span. J. Agric. Res. 18, e03R01. doi:10.5424/sjar/2020183-15225
Chafale, A., and Kapley, A. (2022). Biosurfactants as microbial bioactive compounds in microbial enhanced oil recovery. J. Biotechnol. 352, 1–15. doi:10.1016/j.jbiotec.2022.05.003
Chandran, P., and Das, N. (2012). Role of sophorolipid biosurfactant in degradation of diesel oil by Candida tropicalis. Bioremediat J. 16 (1), 19–30. doi:10.1080/10889868.2011.628351
Chen, D., Chen, J., Luan, X., Ji, H., and Xia, Z. (2011). Characterization of anion–cationic surfactants modified montmorillonite and its application for the removal of methyl orange. Chem. Eng. J. 171 (3), 1150–1158. doi:10.1016/j.cej.2011.05.013
Chen, Q., Li, Y., Liu, M., Zhu, B., Mu, J., and Chen, Z. (2021). Removal of Pb and Hg from marine intertidal sediment by using rhamnolipid biosurfactant produced by a Pseudomonas aeruginosa strain. Environ. Technol. Innov. 22, 101456. doi:10.1016/j.eti.2021.101456
Chooklin, S. C., Sakulsawasdipan, K., Sawain, A., Saimmai, A., Rattanapan, N., and Kitcha, W. (2023). Enhancing oil pollution remediation sites with effective emulsifying property of biosurfactant–producing Bacillus oceanisediminis PM 08. ASEAN J. Sci. Technol. Rep. (AJSTR) 26 (3), 52–62. doi:10.55164/ajstr.v26i3.249370
Ciurko, D., Czyżnikowska, Ż., Kancelista, A., Łaba, W., and Janek, T. (2022). Sustainable production of biosurfactant from agro-industrial oil wastes by Bacillus subtilis and its potential application as antioxidant and ACE inhibitor. Int. J. Mol. Sci. 23, 10824. doi:10.3390/ijms231810824
Conceição, K. S., de Alencar Almeida, M., Sawoniuk, I. C., Marques, G. D., de Sousa Faria-Tischer, P. C., Tischer, C. A., et al. (2020). Rhamnolipid production by Pseudomonas aeruginosa grown on membranes of bacterial cellulose supplemented with corn bran water extract. Environ. Sci. Poll. Res. 27, 30222–30231. doi:10.1007/s11356-020-09315-w
Csutak, O. E., Nicula, N. O., Lungulescu, E. M., Marinescu, V., and Corbu, V. M. (2024). Yarrowia lipolytica CMGB32 biosurfactants produced using n-hexadecane: developing strategies for environmental remediation. Appl. Sci. 14, 3048. doi:10.3390/app14073048
Dalili, D., Amini, M., Faramarzi, M. A., Fazeli, M. R., Khoshayand, M. R., and Samadi, N. (2015). Isolation and structural characterization of Coryxin, a novel cyclic lipopeptide from Corynebacterium xerosis NS5 having emulsifying and anti-biofilm activity. Colloids Surf. B Biointerfaces. 135, 425–432. doi:10.1016/j.colsurfb.2015.07.005
Das, S., and Rao, K. B. (2024). A comprehensive review of biosurfactant production and its uses in the pharmaceutical industry. Archives Microbiol. 206 (2), 60. doi:10.1007/s00203-023-03786-4
Daverey, A., and Pakshirajan, K. (2009). Production, characterization, and properties of sophorolipids from the yeast Candida bombicola using a low-cost fermentative medium. Appl. Biochem. Biotechnol. 158, 663–674. doi:10.1007/s12010-008-8449-z
Desai, J. D., and Banat, I. M. (1997). Microbial production of surfactants and their commercial potential. Microbiol. Mol. Biol. 61 (1), 47–64. doi:10.1128/mmbr.61.1.47-64.1997
Deshmukh, N., and Kathwate, G. (2022). Biosurfactant production by Pseudomonas aeruginosa strain LTR1 and its application. Biointerface Res. Appl. Chem. 13, 10. doi:10.33263/BRIAC131.010
dos Remedios Araújo Vieira Neta, M., Azevedo, M. A., Camargo, F. P., Delforno, T. P., Bona, E., Vicente, J. G. P., et al. (2023). Potential of acid-hydrolysated brewer’s spent grain as substrate for biosurfactant production by Rhodotorula mucilaginosa LBP5 oleaginous yeast. Biomass Convers. biorefin., 1–18. doi:10.1007/s13399-023-03837-2
Egbe, C. C., Oyetibo, G. O., and Ilori, M. O. (2021). Ecological impact of organochlorine pesticides consortium on autochthonous microbial community in agricultural soil. Ecotoxicol. Environ. Saf. 207, 111319. doi:10.1016/j.ecoenv.2020.111319
Elshikh, M., Ahmed, S., Funston, S., Dunlop, P., McGaw, M., Marchant, R., et al. (2016). Resazurin-based 96-well plate microdilution method for the determination of minimum inhibitory concentration of biosurfactants. Biotechno. Lett. 38, 1015–1019. doi:10.1007/s10529-016-2079-2
Eras-Muñoz, E., Farré, A., Sánchez, A., Font, X., and Gea, T. (2022). Microbial biosurfactants: a review of recent environmental applications. Bioengineered 13, 12365–12391. doi:10.1080/21655979.2022.2074621
Farias, C. B. B., Almeida, F. C., Silva, I. A., Souza, T. C., Meira, H. M., Rita de Cássia, F., et al. (2021). Production of green surfactants: market prospects. Electron. J. Biotechnol. 51, 28–39. doi:10.1016/j.ejbt.2021.02.002
Felse, P. A., Shah, V., Chan, J., Rao, K. J., and Gross, R. A. (2007). Sophorolipid biosynthesis by Candida bombicola from industrial fatty acid residues. Enzyme Microb. Technol. 40, 316–323. doi:10.1016/j.enzmictec.2006.04.013
Fenibo, E. O., Ijoma, G. N., Selvarajan, R., and Chikere, C. B. (2019). Microbial surfactants: the next generation multifunctional biomolecules for applications in the petroleum industry and its associated environmental remediation. Microorganisms 7, 581. doi:10.3390/microorganisms7110581
García-Reyes, S., Yáñez-Ocampo, G., Wong-Villarreal, A., Rajaretinam, R. K., Thavasimuthu, C., Patiño, R., et al. (2018). Partial characterization of a biosurfactant extracted from Pseudomonas sp. B0406 that enhances the solubility of pesticides. Environ. Technol. 39, 2622–2631. doi:10.1080/21622515.2017.1363295
Gaur, V. K., Bajaj, A., Regar, R. K., Kamthan, M., Jha, R. R., Srivastava, J. K., et al. (2019). Rhamnolipid from a Lysinibacillus sphaericus strain IITR51 and its potential application for dissolution of hydrophobic pesticides. Bioresour. Technol. 272, 19–25. doi:10.1016/j.biortech.2018.09.144
Gaur, V. K., Sharma, P., Sirohi, R., Varjani, S., Taherzadeh, M. J., Chang, J. S., et al. (2022). Production of biosurfactants from agro-industrial waste and waste cooking oil in a circular bioeconomy: an overview. Bioresour. Technol. 343, 126059. doi:10.1016/j.biortech.2021.126059
Gaur, V. K., Tripathi, V., Gupta, P., Thakur, R. S., Kaur, I., Regar, R. K., et al. (2023). Holistic approach to waste mobil oil bioremediation: valorizing waste through biosurfactant production for soil restoration. J. Environ. Manage 348, 119207. doi:10.1016/j.jenvman.2023.119207
George, S., and Jayachandran, K. (2013). Production and characterization of rhamnolipid biosurfactant from waste frying coconut oil using a novel Pseudomonas aeruginosa D. J. Appl. Microbiol. 114, 373–383. doi:10.1111/jam.12069
Gharaei, S., Ohadi, M., Hassanshahian, M., Porsheikhali, S., and Forootanfar, H. (2022). Isolation, optimization, and structural characterization of glycolipid biosurfactant produced by marine isolate Shewanella algae B12 and evaluation of its antimicrobial and anti-biofilm activity. Appl. Biochem. Biotechnol. 194, 1755–1774. doi:10.1007/s12010-021-03782-8
Gharaie, S., Ohadi, M., Hassanshahian, M., Shakibaie, M., Shahriary, P., and Forootanfar, H. (2023). Glycolipopeptide biosurfactant from Bacillus pumilus SG: physicochemical characterization, optimization, antibiofilm and antimicrobial activity evaluation. 3Biotech. 13 13, 321. doi:10.1007/s13205-023-03728-3
Gupta, N., Yadav, K. K., Kumar, V., Krishnan, S., Kumar, S., Nejad, Z. D., et al. (2021). Evaluating heavy metals contamination in soil and vegetables in the region of North India: levels, transfer and potential human health risk analysis. Environ. Toxicol. Pharmacol. 82, 103563. doi:10.1016/j.etap.2020.103563
Haba, E., Espuny, M. J., Busquets, M., and Manresa, A. (2000). Screening and production of rhamnolipids by Pseudomonas aeruginosa 47T2 NCIB 40044 from waste frying oils. J. Appl. Microbiol. 88, 379–387. doi:10.1046/j.1365-2672.2000.00961.x
Hassanshahian, M., Amirinejad, N., and Askarinejad Behzadi, M. (2020). Crude oil pollution and biodegradation at the Persian Gulf: a comprehensive and review study. J. Environ. Health Sci. Eng. 18, 1415–1435. doi:10.1007/s40201-020-00557-x
Henkel, M., Geissler, M., Weggenmann, F., and Hausmann, R. (2017). Production of microbial biosurfactants: status quo of rhamnolipid and surfactin towards large-scale production. Biotechnol. J. 12, 1600561. doi:10.1002/biot.201600561
Hentati, D., Chebbi, A., Hadrich, F., Frikha, I., Rabanal, F., Sayadi, S., et al. (2019). Production, characterization and biotechnological potential of lipopeptide biosurfactants from a novel marine Bacillus stratosphericus strain FLU5. Ecotoxicol. Environ. Saf. 167, 441–449. doi:10.1016/j.ecoenv.2018.10.036
Hu, M., Ye, Z., Zhang, H., Chen, B., Pan, Z., and Wang, J. (2021). Thermochemical conversion of sewage sludge for energy and resource recovery: technical challenges and prospects. Environ. Pollut. Bioavailab. 33, 145–163. doi:10.1080/26395940.2021.1947159
Ilori, M. O., Amobi, C. J., and Odocha, A. C. (2005). Factors affecting biosurfactant production by oil degrading Aeromonas spp. isolated from a tropical environment. Chemosphere 61, 985–992. doi:10.1016/j.chemosphere.2005.03.066
Inès, M., Mouna, B., Marwa, E., and Dhouha, G. (2023). Biosurfactants as emerging substitutes of their synthetic counterpart in detergent formula: efficiency and environmental friendly. J. Polym. Environ. 31, 2779–2791. doi:10.1007/s10924-023-02778-1
Jadhav, M., Kalme, S., Tamboli, D., and Govindwar, S. (2011). Rhamnolipid from Pseudomonas desmolyticum NCIM-2112 and its role in the degradation of Brown 3REL. J. Basic Microbiol. 51, 385–396. doi:10.1002/jobm.201000364
Jahan, R., Bodratti, A. M., Tsianou, M., and Alexandridis, P. (2020). Biosurfactants, natural alternatives to synthetic surfactants: physicochemical properties and applications. Adv. Colloid Interface Sci. 275, 102061. doi:10.1016/j.cis.2019.102061
Jimoh, A. A., and Lin, J. (2019). Biosurfactant: a new frontier for greener technology and environmental sustainability. Ecotoxicol. Environ. Saf. 184, 109607. doi:10.1016/j.ecoenv.2019.109607
Johnson, P., Trybala, A., Starov, V., and Pinfield, V. J. (2021). Effect of synthetic surfactants on the environment and the potential for substitution by biosurfactants. Adv. Colloid Interface Sci. 288, 102340. doi:10.1016/j.cis.2020.102340
Kabir, M., Habiba, U. E., Khan, W., Shah, A., Rahim, S., Farooqi, Z. U. R., et al. (2023). Climate change due to increasing concentration of carbon dioxide and its impacts on environment in 21st century; A mini review. J. King Saud. Univ. Sci. 102693, 102693. doi:10.1016/j.jksus.2023.102693
Kalia, A., Sharma, S., Semor, N., Babele, P. K., Sagar, S., Bhatia, R. K., et al. (2022). Recent advancements in hydrocarbon bioremediation and future challenges: a review. 3 Biotech. 12, 135. doi:10.1007/s13205-022-03199-y
Kanaoujiya, R., Saroj, S. K., Srivastava, S., and Chaudhary, M. K. (2022). Renewable polysaccharide and biomedical application of nanomaterials. J. Nanomater. 2022, 1–16. doi:10.1155/2022/1050211
Karnwal, A. (2023). Prospects of microbial bio-surfactants to endorse prolonged conservation in the pharmaceutical and agriculture industries. Chem. Sel. 8 (26), e202300401. doi:10.1002/slct.202300401
Kashif, A., Rehman, R., Fuwad, A., Shahid, M. K., Dayarathne, H. N. P., Jamal, A., et al. (2022). Current advances in the classification, production, properties and applications of microbial biosurfactants–A critical review. Adv. Colloid Interface Sci. 306, 102718. doi:10.1016/j.cis.2022.102718
Kazemzadeh, S., Emami-Karvani, Z., Naghavi, N. S., and Emtiazi, G. (2022). Production of surface-active sophorolipid biosurfactant and crude oil degradability by novel Rhodotorula mucilaginosa strain SKF2. J. Surfactants Deterg. 25, 439–454. doi:10.1002/jsde.12572
Krishnan, N., Velramar, B., and Velu, R. K. (2019). Investigation of antifungal activity of surfactin against mycotoxigenic phytopathogenic fungus Fusarium moniliforme and its impact in seed germination and mycotoxicosis. Pest. Biochem. Phys. 155, 101–107. doi:10.1016/j.pestbp.2019.01.010
Kumar, M., Yadav, A. N., Saxena, R., Paul, D., and Tomar, R. S. (2021). Biodiversity of pesticides degrading microbial communities and their environmental impact. Biocatal. Agric. Biotechnol. 31, 101883. doi:10.1016/j.bcab.2020.101883
Lai, C. C., Huang, Y. C., Wei, Y. H., and Chang, J. S. (2009). Biosurfactant-enhanced removal of total petroleum hydrocarbons from contaminated soil. J. Hazard. Mater. 167, 609–614. doi:10.1016/j.jhazmat.2009.01.017
Lamilla, C., Schalchli, H., Briceño, G., Leiva, B., Donoso-Piñol, P., Barrientos, L., et al. (2021). A pesticide biopurification system: a source of biosurfactant-producing bacteria with environmental Biotechnology applications. Agronomy 11 (4), 624. doi:10.3390/agronomy11040624
Lang, S. (2002). Biological amphiphiles (microbial biosurfactants). Curr. Opin. Colloid Interface Sci. 7, 12–20. doi:10.1016/S1359-0294(02)00007-9
Li, J., Deng, M., Wang, Y., and Chen, W. (2016). Production and characteristics of biosurfactant produced by Bacillus pseudomycoides BS6 utilizing soybean oil waste. Int. Biodeterior. Biodegr. 112, 72–79. doi:10.1016/j.ibiod.2016.05.002
Li, S., Jiang, X., Zhao, C., Ren, Y., and Luo, L. (2024). Evaluation of lipopeptides biosurfactant from Raoultella planticola for bioremediation in n-hexadecane-contaminated soil. J. Environ. Chem. Eng. 12 (3), 112622. doi:10.1016/j.jece.2024.112622
Liepins, J., Balina, K., Soloha, R., Berzina, I., Lukasa, L. K., and Dace, E. (2021). Glycolipid biosurfactant production from waste cooking oils by yeast: review of substrates, producers and products. Fermentation 7, 136. doi:10.3390/fermentation7030136
Liu, Q., Niu, J., Yu, Y., Wang, C., Lu, S., Zhang, S., et al. (2021). Production, characterization and application of biosurfactant produced by Bacillus licheniformis L20 for microbial enhanced oil recovery. J. Clean. Prod. 307, 127193. doi:10.1016/j.jclepro.2021.127193
Loeto, D., Jongman, M., Lekote, L., Muzila, M., Mokomane, M., Motlhanka, K., et al. (2021). Biosurfactant production by halophilic yeasts isolated from extreme environments in Botswana. FEMS Microbiol. Lett. 368, fnab146. doi:10.1093/femsle/fnab146
Luz, A. M., Barbosa, G., Manske, C., and Tavares, F. W. (2023). Tween-80 on water/oil interface: structure and interfacial tension by molecular dynamics simulations. Langmuir 39, 3255–3265. doi:10.1021/acs.langmuir.2c03001
Mallik, T., and Banerjee, D. (2022). Biosurfactants: the potential green surfactants in the 21st century. J. Adv. Sci. Res. 13, 97–106. doi:10.55218/JASR.202213110
Mao, X., Jiang, R., Xiao, W., and Yu, J. (2015). Use of surfactants for the remediation of contaminated soils: a review. J. Hazard. Mater. 285, 419–435. doi:10.1016/j.jhazmat.2014.12.009
Meena, K. R., Dhiman, R., Singh, K., Kumar, S., Sharma, A., Kanwar, S. S., et al. (2021). Purification and identification of a surfactin biosurfactant and engine oil degradation by Bacillus velezensis KLP2016. Microb. Cell Factories 20, 26–12. doi:10.1186/s12934-021-01519-0
Mekwichai, P., Tongcumpou, C., Kittipongvises, S., and Tuntiwiwattanapun, N. (2020). Simultaneous biosurfactant-assisted remediation and corn cultivation on cadmium-contaminated soil. Ecotoxicol. Environ. Saf. 192, 110298. doi:10.1016/j.ecoenv.2020.110298
Mgbechidinma, C. L., Akan, O. D., Zhang, C., Huang, M., Linus, N., Zhu, H., et al. (2022). Integration of green economy concepts for sustainable biosurfactant production–a review. Bioresour. Technol. 364, 128021. doi:10.1016/j.biortech.2022.128021
Mulligan, C. N., and Gibbs, B. F. (2004). Types, production and applications of biosurfactants. Proc. Indian Natl. Sci. Acad. Part B. 70, 31–56.
Nanjundan, J., Ramasamy, R., Uthandi, S., and Ponnusamy, M. (2019). Antimicrobial activity and spectroscopic characterization of surfactin class of lipopeptides from Bacillus amyloliquefaciens SR1. Microb. Pathog. 128, 374–380. doi:10.1016/j.micpath.2019.01.037
Nor, F. H. M., Abdullah, S., Yuniarto, A., Ibrahim, Z., Nor, M. H. M., and Hadibarata, T. (2021). Production of lipopeptide biosurfactant by Kurthia gibsonii KH2 and their synergistic action in biodecolourisation of textile wastewater. Environ. Technol. Innov. 22, 101533. doi:10.1016/j.eti.2021.101533
Ohadi, M., Forootanfar, H., Dehghannoudeh, G., Eslaminejad, T., Ameri, A., Shakibaie, M., et al. (2020). Antimicrobial, anti-biofilm, and anti-proliferative activities of lipopeptide biosurfactant produced by Acinetobacter junii B6. Microb. Pathog. 138, 103806. doi:10.1016/j.micpath.2019.103806
Oliveira, F. J. S., Vazquez, L., De Campos, N. P., and De Franca, F. P. (2009). Production of rhamnolipids by a Pseudomonas alcaligenes strain. Process Biochem. 44, 383–389. doi:10.1016/j.procbio.2008.11.014
Paithankar, J. G., Saini, S., Dwivedi, S., Sharma, A., and Chowdhuri, D. K. (2021). Heavy metal associated health hazards: an interplay of oxidative stress and signal transduction. Chemosphere 262, 128350. doi:10.1016/j.chemosphere.2020.128350
Pandey, R., Krishnamurthy, B., Al Obaid, S., Ansari, M. J., Batish, D. R., and Singh, H. P. (2024). Glycolipid biosurfactant production and petroleum hydrocarbon degradation by a new strain of Citricoccus zhacaiensis. Biocatal. Agric. Biotechnol. 103248, 103248. doi:10.1016/j.bcab.2024.103248
Pandey, R., Krishnamurthy, B., Singh, H. P., and Batish, D. R. (2022). Evaluation of a glycolipopepetide biosurfactant from Aeromonas hydrophila RP1 for bioremediation and enhanced oil recovery. J. Clean. Prod. 345, 131098. doi:10.1016/j.jclepro.2022.131098
Pannu, S., Pandey, L. M., and Tiwari, P. (2024). “Applications of microbial biosurfactants in oil recovery,” in Microbial approaches for sustainable green technologies (Boca Raton, United States: CRC Press), 68–86.
Patel, P., Patel, R., Mukherjee, A., and Munshi, N. S. (2023). “Microbial biosurfactants for green agricultural technology,” in Sustainable agriculture reviews 60: microbial processes in agriculture (Cham: Springer Nature Switzerland), 389–413. doi:10.1007/978-3-031-24181-9_15
Pérez, D. J., Iturburu, F. G., Calderon, G., Oyesqui, L. A., De Gerónimo, E., Aparicio, V. C., et al. (2021). Ecological risk assessment of current-use pesticides and biocides in soils, sediments and surface water of a mixed land-use basin of the Pampas region, Argentina. Chemosphere 263, 128061. doi:10.1016/j.chemosphere.2020.128061
Pérez-Armendáriz, B., Cal-y-Mayor-Luna, C., El-Kassis, E. G., and Ortega-Martínez, L. D. (2019). Use of waste canola oil as a low-cost substrate for rhamnolipid production using Pseudomonas aeruginosa. Amb. Express 9, 61–69. doi:10.1186/s13568-019-0784-7
Philibert, D. A., Lyons, D., Philibert, C., and Tierney, K. B. (2019). Field-collected crude oil, weathered oil and dispersants differentially affect the early life stages of freshwater and saltwater fishes. Sci. Total Environ. 647, 1148–1157. doi:10.1016/j.scitotenv.2018.08.052
Phulpoto, I. A., Yu, Z., Hu, B., Wang, Y., Ndayisenga, F., Li, J., et al. (2020). Production and characterization of surfactin-like biosurfactant produced by novel strain Bacillus nealsonii S2MT and it's potential for oil contaminated soil remediation. Microb. Cell Factories. 19, 145. doi:10.1186/s12934-020-01402-4
Priyani, N., and Munir, E. (2021)) “Best medium for production and the activity of biosurfactant from local strain of Pseudomonas. J. Phys. Conf. Ser. 1819, 012066. doi:10.1088/1742-6596/1819/1/012066
Purwasena, I. A., Amaniyah, M., Astuti, D. I., Firmansyah, Y., and Sugai, Y. (2024). Production, characterization, and application of Pseudoxanthomonas taiwanensis biosurfactant: a green chemical for microbial enhanced oil recovery (MEOR). Sci. Rep. 14 (1), 10270. doi:10.1038/s41598-024-61096-1
Rahman, K. S. M., Thahira-Rahman, J., McClean, S., Marchant, R., and Banat, I. M. (2002). Rhamnolipid biosurfactant production by strains of Pseudomonas aeruginosa using low-cost raw materials. Biotechnol. Prog. 18 (6), 1277–1281. doi:10.1021/bp020071x
Ramalingam, V., Varunkumar, K., Ravikumar, V., and Rajaram, R. (2019). Production and structure elucidation of anticancer potential surfactin from marine actinomycete Micromonospora marina. Process Biochem. 78, 169–177. doi:10.1016/j.procbio.2019.01.002
Rastogi, S., Tiwari, S., Ratna, S., and Kumar, R. (2021). Utilization of agro-industrial waste for biosurfactant production under submerged fermentation and its synergistic application in biosorption of Pb2+. Bioresour. Technol. 15, 100706. doi:10.1016/j.biteb.2021.100706
Rehman, R., Ali, M. I., Ali, N., Badshah, M., Iqbal, M., Jamal, A., et al. (2021). Crude oil biodegradation potential of biosurfactant-producing Pseudomonas aeruginosa and Meyerozyma sp. J. Hazard. Mater. 418, 126276. doi:10.1016/j.jhazmat.2021.126276
Rehman, R., Jamal, A., Ali, I., Quddus, M., and ur Rehman, A. (2024). “Role of biosurfactants in improving target efficiency of drugs and designing novel drug delivery systems,” in Industrial applications of biosurfactants and microorganisms (Academic Press), 217–247. doi:10.1016/B978-0-443-13288-9.00010-3
Ribeiro, B. G., Guerra, J. M., and Sarubbo, L. A. (2020). Biosurfactants: production and application prospects in the food industry. Biotechnol. Prog. 36, e3030. doi:10.1002/btpr.3030
Rivardo, F., Martinotti, M. G., Turner, R. J., and Ceri, H. (2011). Synergistic effect of lipopeptide biosurfactant with antibiotics against Escherichia coli CFT073 biofilm. Int. J. Antimicrob. Agents. 37, 324–331. doi:10.1016/j.ijantimicag.2010.12.011
Rocha e Silva, N. M. P., Meira, H. M., Almeida, F. C. G., Soares da Silva, R. D. C. F., Almeida, D. G., Luna, J. M., et al. (2019). Natural surfactants and their applications for heavy oil removal in industry. Sep. Purif. Rev. 48, 267–281. doi:10.1080/15422119.2018.1474477
Safari, P., Hosseini, M., Lashkarbolooki, M., Ghorbani, M., and Najafpour Darzi, G. (2023). Evaluation of surface activity of rhamnolipid biosurfactants produced from rice bran oil through dynamic surface tension. J. Pet. Explor. Prod. Technol. 13, 2139–2153. doi:10.1007/s13202-023-01660-z
Saisa-Ard, K., Maneerat, S., and Saimmai, A. (2013). Isolation and characterization of biosurfactants-producing bacteria isolated from palm oil industry and evaluation for biosurfactants production using low-cost substrates. BioTechnologia 94, 275–284.
Samanta, S. K., Singh, O. V., and Jain, R. K. (2002). Polycyclic aromatic hydrocarbons: environmental pollution and bioremediation. Trends Biotechnol. 20, 243–248. doi:10.1016/S0167-7799(02)01943-1
Santos, B. L. P., Vieira, I. M. M., Ruzene, D. S., and Silva, D. P. (2023a). Unlocking the potential of biosurfactants: production, applications, market challenges, and opportunities for agro-industrial waste valorization. Environ. Res. 117879, 117879. doi:10.1016/j.envres.2023.117879
Santos, C. V. M., Vieira, I. M. M., Santos, B. L. P., de Souza, R. R., Ruzene, D. S., Silva, D. P., et al. (2023b). Biosurfactant production from pineapple waste and application of experimental design and statistical analysis. Appl. Biochem. Biotechnol. 195, 386–400. doi:10.1007/s12010-022-04159-1
Santos, D. K. F., Rufino, R. D., Luna, J. M., Santos, V. A., and Sarubbo, L. A. (2016). Biosurfactants: multifunctional biomolecules of the 21st century. Int. J. Mol. Sci. 17, 401. doi:10.3390/ijms17030401
Santos, E., Teixeira, M., Converti, A., Porto, A., and Sarubbo, L. (2019). Production of a new lipoprotein biosurfactant by Streptomyces sp. DPUA1566 isolated from lichens collected in the Brazilian Amazon using agroindustry wastes. Biocatal. Agric. Biotechnol. 17, 142–150. doi:10.1016/j.bcab.2018.10.014
Saravanakumari, P., and Mani, K. (2010). Structural characterization of a novel xylolipid biosurfactant from Lactococcus lactis and analysis of antibacterial activity against multi-drug resistant pathogens. Bioresour. Technol. 101, 8851–8854. doi:10.1016/j.biortech.2010.06.104
Sarubbo, L. A., Maria da Gloria, C. S., Durval, I. J. B., Bezerra, K. G. O., Ribeiro, B. G., Silva, I. A., et al. (2022). Biosurfactants: production, properties, applications, trends, and general perspectives. Biochem. Eng. J. 181, 108377. doi:10.1016/j.bej.2022.108377
Schultz, J., and Rosado, A. S. (2020). Extreme environments: a source of biosurfactants for biotechnological applications. Extremophiles 24, 189–206. doi:10.1007/s00792-019-01151-2
Sen, R. (2008). Biotechnology in petroleum recovery: the microbial EOR. PECSor 34, 714–724. doi:10.1016/j.pecs.2008.05.001
Shah, M. U. H., Sivapragasam, M., Moniruzzaman, M., Talukder, M. M. R., Yusup, S. B., and Goto, M. (2017). Production of sophorolipids by Starmerella bombicola yeast using new hydrophobic substrates. Biochem. Eng. J. 127, 60–67. doi:10.1016/j.bej.2017.08.005
Shahabi Rokni, M., Halajnia, A., Lakzian, A., and Housaindokht, M. R. (2022). Sugar beet molasses bioconversion into biosurfactant: optimization and comparison with other carbon sources. Biomass Convers. biorefin., 1–13. doi:10.1007/s13399-022-03597-5
Shaji, A., Thamarai, P., Deivayanai, V. C., Saravanan, A., and Yaashikaa, P. R. (2024). Progress in sustainable remediation: utilizing biosurfactants for eco-friendly contaminant cleanup. Bioresour. Technol. Rep. 101901, 101901. doi:10.1016/j.biteb.2024.101901
Sharma, S., Datta, P., Kumar, B., Tiwari, P., and Pandey, L. M. (2019). Production of novel rhamnolipids via biodegradation of waste cooking oil using Pseudomonas aeruginosa MTCC7815. Biodegradation 30, 301–312. doi:10.1007/s10532-019-09874-x
Sharma, S., and Pandey, L. M. (2020). Production of biosurfactant by Bacillus subtilis RSL-2 isolated from sludge and biosurfactant mediated degradation of oil. Bioresour. Technol. 307, 123261. doi:10.1016/j.biortech.2020.123261
Silva, M. D. G. C., Medeiros, A. O., Converti, A., Almeida, F. C. G., and Sarubbo, L. A. (2024). Biosurfactants: promising biomolecules for agricultural applications. Sustainability 16 (1), 449. doi:10.3390/su16010449
Silvia, L., Mughayyirah, Y., Amilia, S., Zainuri, M., and Darminto, (2023). Surface modification of SiO2-based methyltrimethoxysilane (MTMS) using cetyltrimethyl ammonium bromide (CTAB) on the wettability effects through hierarchical structure. J. Solgel Sci. Technol. 108 (1), 228–236. doi:10.1007/s10971-023-06202-x
Singh, P., Borthakur, A., Singh, R., Bhadouria, R., Singh, V. K., and Devi, P. (2021a). A critical review on the research trends and emerging technologies for arsenic decontamination from water. Groundw. Sustain. Dev. 14, 100607. doi:10.1016/j.gsd.2021.100607
Singh, P., Patil, Y., and Rale, V. (2019). Biosurfactant production: emerging trends and promising strategies. J. Appl. Microbiol. 126, 2–13. doi:10.1111/jam.14057
Singh, P. B., Sharma, S., Saini, H. S., and Chadha, B. S. (2009). Biosurfactant production by Pseudomonas sp. and its role in aqueous phase partitioning and biodegradation of chlorpyrifos. Lett. Appl. Microbiol. 49, 378–383. doi:10.1111/j.1472-765X.2009.02672.x
Singh, V. K., Singh, R., Kumar, A., Bhadouria, R., and Pandey, S. (2021b). Sewage wastewater and sludge as source of traditional and emerging contaminants in agroecosystems. Sustainable agriculture reviews 50: Emerg. Contam. Agric., 35–59. doi:10.1007/978-3-030-63249-6_2
Souza, K. S. T., Gudiña, E. J., Schwan, R. F., Rodrigues, L. R., Dias, D. R., and Teixeira, J. A. (2018). Improvement of biosurfactant productionby Wickerhamomyces anomalus CCMA 0358 and its potential application in bioremediation. J. Hazard. Mater. 346, 152–158. doi:10.1016/j.jhazmat.2017.12.021
Soyuer, K., and Bilen Ozyurek, S. (2023). An eco-friendly approach to biosurfactant production using low-cost wastes. J. Dispers. Sci. Technol. 45, 1599–1612. doi:10.1080/01932691.2023.2225581
Sun, W., Zhu, B., Yang, F., Dai, M., Sehar, S., Peng, C., et al. (2021). Optimization of biosurfactant production from Pseudomonas sp. CQ2 and its application for remediation of heavy metal contaminated soil. Chemosphere 265, 129090. doi:10.1016/j.chemosphere.2020.129090
Tang, F., Zhang, H., Cheng, H., Wang, Y., Liu, Q., Zhao, C., et al. (2023). New insights of crude oil biodegradation construction by microbial consortium B10: responded substrates, genomics, biodegradation mechanism and pathways. Chem. Eng. J. 478, 147143. doi:10.1016/j.cej.2023.147143
Tang, J., He, J., Liu, T., Xin, X., and Hu, H. (2017). Removal of heavy metal from sludge by the combined application of a biodegradable biosurfactant and complexing agent in enhanced electrokinetic treatment. Chemosphere 189, 599–608. doi:10.1016/j.chemosphere.2017.09.104
Tripathi, L., Twigg, M. S., Zompra, A., Salek, K., Irorere, V. U., Gutierrez, T., et al. (2019). Biosynthesis of rhamnolipid by a Marinobacter species expands the paradigm of biosurfactant synthesis to a new genus of the marine microflora. Microb. Cell Fact. 18, 164. doi:10.1186/s12934-019-1216-8
Twigg, M. S., Tripathi, L., Zompra, A., Salek, K., Irorere, V. U., Gutierrez, T., et al. (2018). Identification and characterisation of short chain rhamnolipid production in a previously uninvestigated, non-pathogenic marine pseudomonad. Appl. Microbiol. Biotechnol. 102, 8537–8549. doi:10.1007/s00253-018-9202-3
Van Bogaert, I. N., Holvoet, K., Roelants, S. L., Li, B., Lin, Y. C., Van de Peer, Y., et al. (2013). The biosynthetic gene cluster for sophorolipids: a biotechnological interesting biosurfactant produced by Starmerella bombicola. Mol. Microbiol. 88, 501–509. doi:10.1111/mmi.12200
Varjani, S., Rakholiya, P., Ng, H. Y., Taherzadeh, M. J., Ngo, H. H., Chang, J. S., et al. (2021). Bio-based rhamnolipids production and recovery from waste streams: status and perspectives. Bioresour. Technol. 319, 124213. doi:10.1016/j.biortech.2020.124213
Venkataraman, S., Rajendran, D. S., and Vaidyanathan, V. K. (2024). An insight into the utilization of microbial biosurfactants pertaining to their industrial applications in the food sector. Food Sci. Biotechnol. 33, 245–273. doi:10.1007/s10068-023-01435-6
Wadekar, S., Kale, S., Lali, A., Bhowmick, D., and Pratap, A. (2011). Sophorolipid production by Starmerella bombicola (ATCC 22214) from virgin and waste frying oils, and the effects of activated earth treatment of the waste oils. J. Am. Oil Chem. Soc. 89, 1029–1039. doi:10.1007/s11746-011-1986-6
Wadekar, S. D., Kale, S. B., Lali, A. M., Bhowmick, D. N., and Pratap, A. P. (2012). Microbial synthesis of rhamnolipids by Pseudomonas aeruginosa (ATCC 10145) on waste frying oil as low cost carbon source. Prep. Biochem. Biotechnol. 42, 249–266. doi:10.1080/10826068.2011.603000
Wang, S., and Mulligan, C. N. (2009). Rhamnolipid biosurfactant-enhanced soil flushing for the removal of arsenic and heavy metals from mine tailings. Process Biochem. 44, 296–301. doi:10.1016/j.procbio.2008.11.006
Wei, Z., Wang, J. J., Gaston, L. A., Li, J., Fultz, L. M., DeLaune, R. D., et al. (2020). Remediation of crude oil-contaminated coastal marsh soil: integrated effect of biochar, rhamnolipid biosurfactant and nitrogen application. J. Hazard. Mater. 396, 122595. doi:10.1016/j.jhazmat.2020.122595
Whang, L. M., Liu, P. W. G., Ma, C. C., and Cheng, S. S. (2008). Application of biosurfactants, rhamnolipid, and surfactin, for enhanced biodegradation of diesel-contaminated water and soil. J. Hazard. Mater. 151, 155–163. doi:10.1016/j.jhazmat.2007.05.063
Yang, Z., Zhang, Z., Chai, L., Wang, Y., Liu, Y., and Xiao, R. (2016). Bioleaching remediation of heavy metal-contaminated soils using Burkholderia sp. Z-90. J. Hazard. Mater. 301, 145–152. doi:10.1016/j.jhazmat.2015.08.047
Yesankar, P. J., Pal, M., Patil, A., and Qureshi, A. (2023). Microbial exopolymeric substances and biosurfactants as ‘bioavailability enhancers’ for polycyclic aromatic hydrocarbons biodegradation. Int. J. Environ. Sci. Technol. 20, 5823–5844. doi:10.1007/s13762-022-04068-0
Zamora-Ledezma, C., Negrete-Bolagay, D., Figueroa, F., Zamora-Ledezma, E., Ni, M., Alexis, F., et al. (2021). Heavy metal water pollution: a fresh look about hazards, novel and conventional remediation methods. Environ. Technol. Innov. 22, 101504. doi:10.1016/j.eti.2021.101504
Keywords: biosurfactants, hydrophobic chemicals, interfacial surface tension, emulsifiers, environmental pollutants, circular economy
Citation: Singh N, Hu X-H, Kumar V, Solanki MK, Kaushik A, Singh VK, Singh SK, Yadav P, Singh RP, Bhardwaj N, Wang Z and Kumar A (2024) Microbially derived surfactants: an ecofriendly, innovative, and effective approach for managing environmental contaminants. Front. Bioeng. Biotechnol. 12:1398210. doi: 10.3389/fbioe.2024.1398210
Received: 15 March 2024; Accepted: 07 August 2024;
Published: 26 August 2024.
Edited by:
Aravind Madhavan, Amrita Vishwa Vidyapeetham University, IndiaReviewed by:
Bishwambhar Mishra, Chaitanya Bharathi Institute of Technology, IndiaSabeela Beevi Ummalyma, Rajagiri College of Social Sciences, India
Copyright © 2024 Singh, Hu, Kumar, Solanki, Kaushik, Singh, Singh, Yadav, Singh, Bhardwaj, Wang and Kumar. This is an open-access article distributed under the terms of the Creative Commons Attribution License (CC BY). The use, distribution or reproduction in other forums is permitted, provided the original author(s) and the copyright owner(s) are credited and that the original publication in this journal is cited, in accordance with accepted academic practice. No use, distribution or reproduction is permitted which does not comply with these terms.
*Correspondence: Ajay Kumar, YWpheWt1bWFyX2JodUB5YWhvby5jb20=; Zhen Wang, d2FuZzc5ODExMDUxMEAxNjMuY29t
†These authors have contributed equally to this work