- Department of Bioscience and Engineering, National Institute of Technology Calicut, Kozhikode, Kerala, India
Diabetes is a metabolic disorder characterized by high blood sugar. Uncontrolled blood glucose affects the circulatory system in an organism by intervening blood circulation. The high blood glucose can lead to macrovascular (large blood vessels) and microvascular (small blood vessels) complications. Due to this, the vital organs (notably brain, eyes, feet, heart, kidneys, lungs and nerves) get worsen in diabetic patients if not treated at the earliest. Therefore, acquiring treatment at an appropriate time is very important for managing diabetes and other complications that are caused due to diabetes. The root cause for the occurrence of various health complications in diabetic patients is the uncontrolled blood glucose levels. This review presents a consolidated account of the applications of various types of three-dimensional (3D) printing and bioprinting technologies in treating diabetes as well as the complications caused due to impaired blood glucose levels. Herein, the development of biosensors (for the diagnosis), oral drug formulations, transdermal drug carriers, orthotic insoles and scaffolds (for the treatment) are discussed. Next to this, the fabrication of 3D bioprinted organs and cell-seeded hydrogels (pancreas engineering for producing insulin and bone engineering for managing bone defects) are explained. As the final application, 3D bioprinting of diabetic disease models for high-throughput screening of ant-diabetic drugs are discussed. Lastly, the challenges and future perspective associated with the use of 3D printing and bioprinting technologies against diabetes and its related chronic complications have been put forward.
1 Introduction
Diabetes, commonly referred to as a “silent-killer disease” or a “rich man’s disease” has been growing as a major chronic disease of concern in recent times (Khan et al., 2019; India Today, 2023). According to International Diabetes Federation (IDF), 10.5% of population at the age group of 20–79 years has diabetes and half of the population among this 10.5% is unaware about the possession of the disease (International Diabetes Federation, 2024). The study by IDF also reported that by the year 2045, it is expected that one in eight adults (equivalent to 783 million) will be diabetic (International Diabetes Federation, 2024). Diabetes is a metabolic disorder characterized by higher concentrations of blood sugar/glucose levels. Pre-diabetes is a kind of metabolically disordered health condition, where the individual possesses high blood sugar level than the normal range (Khan et al., 2019). Although the pre-diabetic condition is characterized by high blood glucose, the detected high blood sugar level might not be appropriate enough to confirm the diabetic condition. Diabetes has been classified into three types, namely, the type 1 diabetes (T1D) where the body is unable to produce enough insulin), type 2 diabetes (T2D) or non-insulin dependent diabetes (where the body produces insulin but is unable to use it effectively) and gestational diabetes (diabetes developed during pregnancy) (Centers for Disease Control and Prevention, 2023).
The high blood glucose initially affects the large blood vessels (leading to macrovascular complications), and if left undiagnosed, also starts affecting the small blood vessels (resulting in microvascular complications). As high blood sugar can bring down the immunity in an individual, it has to be noted that a diabetic patient is susceptible for acquiring infections often than a healthy person (Berbudi et al., 2020). Hence, the treatment measures need to be taken from the beginning stage of diagnosis of diabetes or pre-diabetes. Acquiring treatment at an appropriate time in the early stage is very important for the effective management of diabetes.
In recent years, 3D printing and 3D bioprinting are gaining attention as forefront technologies in diagnosis and treatment of various disorders/diseases. These technologies are extensively used for the production of pharmaceutical drugs, medical products and artificial organs (Cui et al., 2021; Yi et al., 2021; Tripathi et al., 2023). Chakka and Chede (2023) define the term “3D” in “3D Printing and Bioprinting” as “Design,” “Develop” and “Dispense” (Chakka and Chede, 2023). The technology comprises the creation of a three-dimensional (3D) object from a CAD/digital 3D model. 3D printing and 3D bioprinting technologies, despite holding the similar working principle, differ from each other in terms of the composition of the ink used for printing the objects. 3D printing is used for printing solid materials and hence the composition of printing ink is polymers and chemicals. On the other hand, 3D bioprinting is used for the production of substrates, artificial organs and in vivo models that mimics the cellular microenvironment in living organisms. The ink used in 3D bioprinting process consists of biological materials such as cells/living tissues along with (bio) polymers, growth media, regulators, etc. And hence the ink used in a 3D bioprinting process is referred to as “bioink.”
3D printing and bioprinting technologies are used in various ways in the diagnosis and treatment of diabetes. This review highlights the recent applications of 3D printing and bioprinting in treatment of diabetes and some of the common health complications caused due to diabetes. Role of 3D printing and 3D bioprinting technologies in the diagnosis and treatment of diabetes (hyperglycemia) and its associated chronic complications (especially neuropathy, hypertension, dyslipidemia, peripheral diabetic neuropathy, diabetic wounds, diabetic foot ulcers), engineering of pancreas (to overcome graft rejection due to pancreas transplant) and bones (to treat and manage bone fracture and inhibition of osteogenesis) and fabrication of 3D drug testing models for rapid screening of anti-diabetic drugs have been discussed. Lastly, a glimpse of challenges and future perspective associated with 3D printing and 3D bioprinting in the management and treatment of diabetes is discussed.
2 Types of 3D printing
Different types of 3D printing strategies have been used in the production of pharmaceuticals or biomedical devices for the diagnosis and treatment of diabetes. Extrusion-based 3D printing, Fused Deposition Modeling (FDM), Co-axial extrusion-based 3D printing, Stereolithography (SLA) 3D printing, Digital Light Processing (DLP)-based 3D printing, Selective Laser Sintering (SLS) and Ink-Jet Printing (IJP) are the various kinds of 3D printing and bioprinting techniques used in the development of pharmaceuticals and biomedical products. The working principle of some of the 3D printing approaches are described below:
(a) Extrusion-based 3D printing and bioprinting: Extrusion-based bioprinting has been evolving as one of the leading approaches for the manufacture of pharmaceuticals, regenerative medicine and tissue engineering. The principle behind the working of an extrusion-based 3D bioprinter is that ink [drug(s) loaded in the polymeric gel] or bioink (polymer with biological materials) will be extruded through the nozzles to create 3D structures. The so formed 3D bioprinted structures can be further crosslinked for obtaining final 3D structures. Figure 1 presents the schematic of the working principle of an extrusion-based 3D bioprinter.
(b) Fused Deposition Modeling (FDM): FDM, also referred to as “Fused Filament Fabrication” is a process in which the solid/thermoplastic filaments are extruded in a layer-by-layer manner through a nozzle after melting to form a 3D-object (Acierno and Patti, 2023). The main requisite of the printing material for its use in FDM 3D printing is that the molten printing material should be capable of solidifying immediately once the printing is over. FDM is mostly used in the continuous production of pharmaceuticals.
(c) Co-axial 3D bioprinting: Co-axial 3D bioprinting is a strategy that facilitates multimaterial printing that simultaneously dispenses multiple bioinks through a single filament (containing concentric orifices to dispense bioinks concentrically). This kind of 3D printing strategy is mostly used in biofabrication of cell-laden constructs/tissue engineering applications (Hong et al., 2019; Kim and Nam, 2020; Liu et al., 2022) and to a certain extent in the manufacture of pharmaceutical formulations (Talebian et al., 2021). An interested reader can explore for more details on the applications of co-axial 3D bioprinting in tissue engineering by referring to the review by (Kjar et al., 2021).
(d) SLA 3D printing and bioprinting: SLA, also known as “vat polymerization” 3D printing is a process in which the photosensitive resin in the form of liquid is poured into a tank and cured by UV light for solidification (Dassault Systèmes, 2024). SLA 3D printing and bioprinting techniques are mostly used in the fabrication of pharmaceutical formulations and cell scaffolds, especially the bone scaffolds. Figure 2 presents the schematic of principle behind the working of a SLA 3D printer/bioprinter.
(e) DLP-based 3D printing and bioprinting: In DLP-based 3D bioprinting, the digital light source is allowed to project layer-by-layer in the form of surface light on a liquid photosensitive resin surface, followed by solidification of layers. Figure 3 presents the schematic of the working principle of a DLP-based 3D printer/bioprinter.
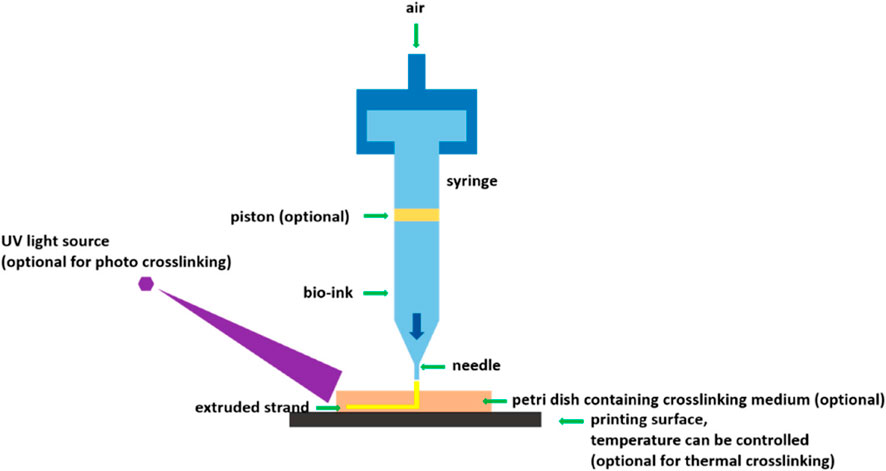
Figure 1. Schematic of an extrusion-based 3D bioprinter [Reproduced from (You et al., 2017] with permission. Copyright 2017 MDPI).
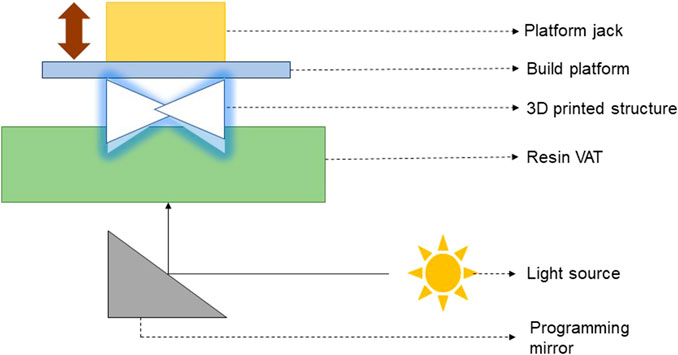
Figure 2. Schematic of the working principle of a SLA 3D printer/bioprinter (Verisqa et al., 2022).
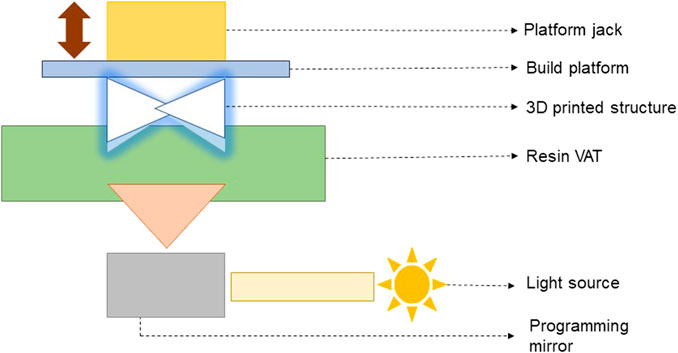
Figure 3. Schematic of the working principle of a DLP-based 3D printer/bioprinter (Verisqa et al., 2022).
3 Applications of 3D printing and 3D bioprinting in diabetic patients
3.1 Diagnostic applications
3.1.1 Biosensors and other analytical tools
Biosensors are analytical devices used in sensing and detection of high glucose level in diabetic patients. Liu et al. (2021) devised and investigated the utility of MN-based biosensor for subcutaneous-level interstitial glucose sensing applications (see Section 3.2.2.) (Liu et al., 2021). Remarkably, Goel and co-workers developed a portable platform using a combination of 3D printing, laser (CO2 laser) and electrode (graphene electrode) technologies that could detect the sugar level in patients with type I and type II diabetes (Kumar et al., 2023). Very recently, Piaggio et al. (2024) proposed a smart-tool for screening diabetic neuropathies based on the integration of 3D printed accessories and a smartphone app. The findings from the experiments conducted with 11 normosubjects established that the smart-tool could serve as a practical solution to improve the SoC of diabetic patients suffering with diabetic neuropathy (Piaggio et al., 2024).
3.2 Therapeutic applications
3.2.1 Oral medications
3D printing has been recognized as one of the promising strategies implemented for the production of oral solid dosage forms (Ventola, 2014; Ahmad et al., 2023). Various printing methods such as 3D extrusion-based printing (Ahmed et al., 2021), Fused Deposition Modeling (FDM) (Ibrahim et al., 2019), Ink-Jet Printing (IJP) (Stranzinger et al., 2021) and Semi-Solid Extrusion (SSE) printing (Cui et al., 2019) have been used by researchers for the production of various types of oral formulations. Khaled et al. (2015) employed 3D extrusion-based printing and produced polypills containing captopril, nifedipine and glipizide with controlled release profiles. These polypills potentially find its use in the treatment of hypertension in diabetic patients (Khaled et al., 2015).
Ahmed et al. (2021) reported Self-Nano Emulsifying Drug Delivery System (SNEDDS)-based 3D-printed tablet formulation containing GLMP and rosuvastatin (RSV) for treating dyslipidemia in diabetic patients (Ahmed et al., 2021). Extrusion-based 3D printing method was employed for the preparation of the formulation. The comparison of SNEDDS tablets with non-SNEDDS tablets indicated that the drug release behaviour of SNEDDS tablets were superior to that of the non-SNEDDS tablets (Ahmed et al., 2021). The researchers surmised that the formulated SNEDDS-based tablets could serve as a promising combined oral medication for diabetes treatment.
Ibrahim et al. (2019) 3D printed Metformin HCL tablets using Poly Vinyl Alcohol (PVA) as polymer by FDM and investigated the drug loading, tablet design and dissolution studies for those 3D printed tablets (Ibrahim et al., 2019). Likewise, Gioumouxouzis et al. (2018) 3D printed bilayer oral solid dosage form combining metformin for prolonged and glimepiride (GLMP) by FDM for immediate drug delivery (Gioumouxouzis et al., 2018). Stranzinger et al. (2021) used Near-Infrared (NIR) hyperspectral imaging as a monitoring tool for on-demand manufacturing of ink-jet printed metformin hydrochloride formulations (Stranzinger et al., 2021).
Cui et al. (2019) utilized SSE 3D printing and fabricated tablets with customized internal structures containing personalized doses in it (Cui et al., 2019). The researchers used glipizide as model drug and investigated the effect of three different concentrations of the polymer HPMC K100LV polymer in the 3D printing of glipizide tablets (Cui et al., 2019). The study revealed that the tailored drug release was achieved with the 3D printed tablets, thereby proving that personalized tablets prepared by SSE 3D printing can be effectively used to modulate the physicochemical properties of drug (Cui et al., 2019).
3.2.2 Transdermal drug delivery systems
Transdermal drug delivery has been gaining attraction as an effective drug delivery system since it offers several advantages such as being patient friendly, non-invasive, and potential to bypass the first-pass metabolism by liver (Alkilani et al., 2015; Wong et al., 2023). It is not astonishing to see that the application of transdermal drug delivery system has also been progressing in the battle against diabetes.
Pere et al. (2018) reported the fabrication of polymeric microneedle (MN) patches containing insulin by means of (SLA) printing (Pere et al., 2018). A biocompatible class I resin, Dental SG was photopolymerized into pyramid and cone designs. Insulin was loaded into three carbohydrate-based drug carriers, namely, the trehalose, mannitol and xylitol, which were further inkjet print-coated onto the pyramid and cone MN designs. Effect of MN design on the insulin release and the integrity of the drug carrier containing insulin were evaluated. The three carriers were reported to maintain good integrity with xylitol exhibiting best performance and insulin release was found to be rapid with both the pyramid and cone designs. The researchers suggest that the proposed biocompatible 3D printed-MNs would be biocompatible as well as scalable (Pere et al., 2018).
Analogous to the discussed work above, Economidou et al. (2019) fabricated 3D printed MN patches with superior skin penetration capacity by photopolymerizing biocompatible resin layer-by-layer in pyramid and spear designs, for intradermal delivery of insulin by means of stereolithography (SLA) (Economidou et al., 2019). Carrier made up of sugar, and insulin were coated onto the surface of resin by Ink-Jet Printing. The researchers concluded that the fabricated MN system was able to exhibit fast insulin action and well-controlled hypoglycemic condition during the in vivo trial experiments with diabetic mice. Following this study, the same research team worked on the optimization of design and manufacturing parameters of 3D printed solid MNs of insulin for improved strength, sharpness, and delivery (Economidou et al., 2021). It was illustrated that the coating morphology, piercing behaviour and fracture of the MNs were influenced by its geometry (Economidou et al., 2021).
Interestingly, very limited number of reports have explored the formulation of MN system by extrusion-based 3D printing. Wu et al. (2020) demonstrated the employability of extrusion-based 3D printing in the fabrication of insulin-loaded MN patch for regulated delivery of insulin and glucose management in diabetic mice (Wu et al., 2020).
A step ahead of transdermal drug delivery, Liu et al. (2021) explored the use of MNs subcutaneous-level interstitial glucose sensing applications (Liu et al., 2021). A biosensor constructed by the integration of four techniques, namely, 3D printing, microfabrication, an electroplating and an enzyme immobilization was investigated for the sensing, continuous and painless monitoring of interstitial glucose level in mice (Liu et al., 2021). The MNs-based biosensing device exhibited a consistent and steady detection of glucose in various biological fluids such as in buffer, plasma, and simulated interstitial fluid (ISF) (Liu et al., 2021). The authors proposed that the electroplating and microfabrication techniques aided the sensor to sense and detect glucose with good sensitivity in a broad detection range (Liu et al., 2021). This finding could possibly pave for the invention of new analytical devices for continuous monitoring of blood glucose levels which will be extremely useful one for diabetic patients.
3.2.3 Orthotic insoles
50% of diabetic patients were believed to develop symptomatic peripheral neuropathy within 25 years of first occurrence of diabetes (Volmer-Thole and Lobmann, 2016). Peripheral diabetic neuropathy is one of the major chronic complications that affects feet, legs, digestive system and heart (Zaino et al., 2023). However, the organ system that often gets affected because of this neuropathy is the nervous system of the legs and feet. The orthotic insoles are special kind of biomedical product that finds its application in the treatment of foot-related complications caused because of peripheral diabetic neuropathy among diabetic patients. Foot insoles are used in relieving pains (especially site-specific pains) in persons hailing diabetic foot complications. Plantar pressures (or distribution of loads on foot) in diabetic patients act as one of the important parameters in evaluating the progression of diabetes (Zuniga et al., 2022). Custom insoles, also referred to as “Standard of Care (SoC) insoles” are well-known for its use in dealing with diabetic foot plantar pressures. Though these SoC insoles are customized, these still do possess a drawback of being dependent on the generic properties of materials. However, recent research has evidenced the 3D Printing strategy could be employed to circumvent this drawback by relying on individualized materials in the fabrication of diabetic-friendly personalized insoles (Mancuso et al., 2023). Mancuso et al. (2023) conducted a pilot study named “DIAPASON” (DIAbetic PAtients Safe ambulatiON) and studied the safety of 3D-printed insoles, when used by an italian patient with foot complications associated with T2D in an ambulatory setting (Mancuso et al., 2023).
In the past few days, integration of 3D printing, scanning, materials chemistry and software application have been highly beneficial in fine-tuning the procedure of insole fabrication process and in enhancing the performance of insoles as well (Zuniga et al., 2022; Mancuso et al., 2023). For instance, Zuniga et al. (2022) developed 3D-printed insoles employing the thermoplastic polyether-polyurethane and thermoplastic polyurethane polyester-based polymer for diabetic patients and evaluated its efficiency in plantar pressure distribution with electronic pressure sensors (Zuniga et al., 2022). The insoles were found to perform well and were reported to possess potential in managing diabetes. The patients also provided feedback that the insoles made up of thermoplastic polyurethane polyester-based were comfortable than that of the standard insole and the insole made up of the thermoplastic polyether-polyurethane. Similarly, Muir et al. (2022) evaluated the plantar distribution potential of 3D printed accommodative insoles (Muir et al., 2022). Patient-specific metamaterials were used in the 3D printed insole design. Likewise, Hudak et al. (2022) developed a novel workflow to fabricate patient-specific 3D printed accommodative foot orthosis with personalized lattice metamaterial (Hudak et al., 2022).
Maharana et al. (2022) proposed a kind of 3D printed, mechanically operated dynamically self-offloading customized therapeutic footwear that involves no use of sensors and actuators (Maharana et al., 2022). The foot dimensions and walking style were customized in the therapeutic footwear made of the polymer, thermoplastic polyurethane (Maharana et al., 2022). The working principle of the therapeutic footwear was based on the mechanism that when a load is applied, the snapping arches in the footwear enters the negative-stiffness regime. In contrast, offloading the load will snap to a different shape, followed by reverting back of the snapping arch to the initial shape when the load disappears (Maharana et al., 2022). The research team hypothesize that the snapping mechanism helps to keep the feet well-balanced, and also assist in quicker healing of the injured sites of the feet (Inidian institute of science, 2022; Maharana et al., 2022).
3.2.4 Diabetic wounds and foot ulcers
Diabetic wounds are another kind of complications that affects the quality of life of most of the diabetic patients with peripheral neuropathy. A typical wound healing process comprises four important phases, namely, hemostasis, inflammation, proliferation and remodeling (Guo and DiPietro, 2010; Huang et al., 2022). Unlike in non-diabetics, the blood circulation gets slowdown in diabetic patients and hence the body’s efficiency to deliver nutrients to the wound regions gets disrupted. This causes the diabetic wounds to heal slowly. Moreover, the diabetic wounds are also associated with high infection risk. Similar to wounds, some diabetic patients also suffer with slow healing foot ulcers, commonly referred to as “Diabetic Foot Ulcers (DFU).” While diabetic wounds are caused by cuts or abrasions, DFU starts as wounds and further progresses as foot sore with poor ability to heal. DFU is a kind of skin lesion caused because of the neuropathic complications in T1D and T2D patients when sugar level is not under control. DFU, if left untreated may lead to amputation (Kushmakov et al., 2018). The impairment of wound healing or DFU in diabetes is mainly attributed to poor immune response (NIH Research Matters, 2020), poor glycemic control (Game, 2008), renal failure (Game, 2008) and visual impairment (Game, 2008). Hence, early detection and treatment for DFU is very much important. For past few years, 3D bioprinting has been explored by several researchers in fabricating scaffolds for wound healing. Our literature survey indicate that good number of review articles have been contributed by researchers in this regard (Tsegay et al., 2022; Freedman et al., 2023; Pita-Vilar et al., 2023; Uchida and Bruschi, 2023). This section presents the recent applications of 3D bioprinting in the production of scaffolds for diabetic wound healing.
Fratini et al. (2023) integrated microfluidics technology with co-axial 3D bioprinting to fabricate a wound dressing that can simultaneously reduce the bacterial load and promoting wound healing (Fratini et al., 2023). Customization of a wound-healing scaffold based on the nature of wound in a patient can help in managing patient’s demand efficiently. Accordingly, Glover et al. (2023) fabricated 3D bioprinted polycaprolactone (PCL) scaffolds of different designs (honeycomb, square, parallel, triangular and double-parallel) loaded with an antibiotic, levoflocixin and investigated the sustained drug release for wound-healing applications (Glover et al., 2023). The impact of scaffold geometry and antibiotic concentration on the mechanical properties were examined. Figure 4 presents the digital images of 3D bioprinted PCL scaffolds of various designs and the fexibility a bioprinted scaffold. Also, the drug release characteristics of scaffolds were investigated in the study. The researchers established that the scaffolds can be modified according to the size of the wound and can serve as a potential strategy for treating DFU when compared with that of the conventional treatment strategies as it could perfectly meet the patient’s demand by being simpler and economical (Glover et al., 2023). Jiang et al. (2023), recently contributed an informative review on the present status and progress on dressing management for DFU (Jiang et al., 2023). Table 1 presents the summary of literature reports available on the 3D bioprinting of scaffolds fabricated for healing of diabetic wounds and DFU.
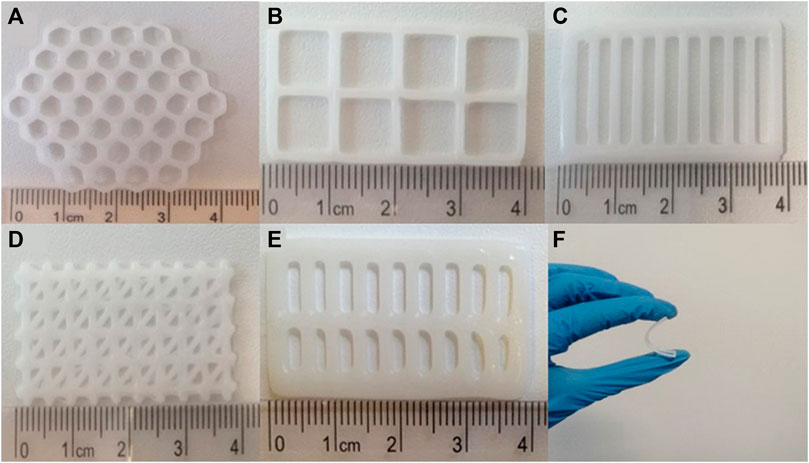
Figure 4. Digital images of 3D bioprinted PCL scaffolds of different designs: (A) honeycomb, (B) square, (C) parallel, (D) triangular, (E) double-parallel, and (F) the fexibility a bioprinted scaffold [Reproduced from (Glover et al., 2023) with permission. Copyright 2023 Springer Nature].
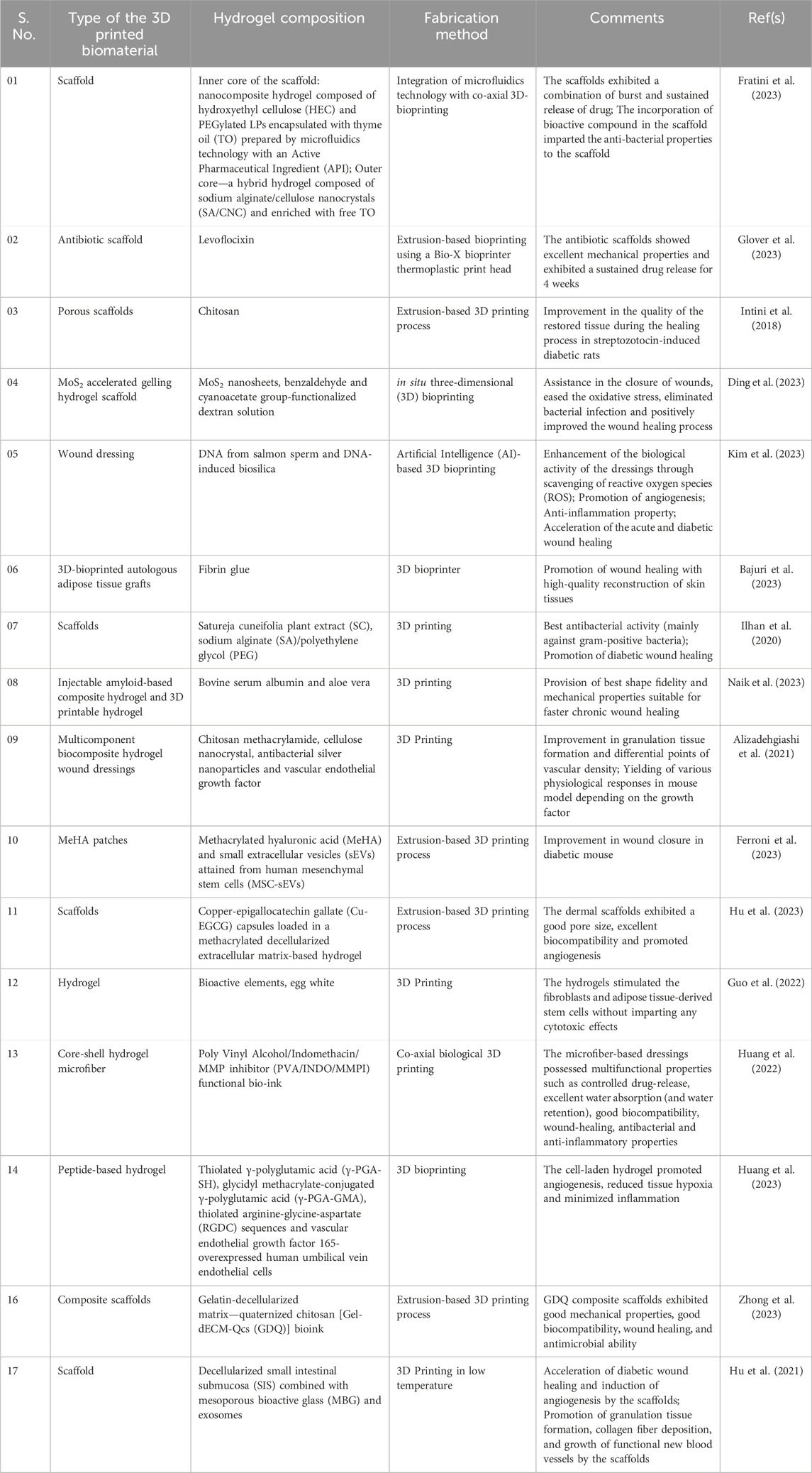
Table 1. Summary of literature reports available on 3D bioprinted hydrogels/scaffolds fabricated for diabetic wound healing and DFU healing applications.
3.3 Engineering of pancreas and bones
3.3.1 3D bioprinted organs, bio scaffolding/encapsulation systems
Among the various organs in the organ system, pancreas play a vital role in maintaining blood glucose level (Sharabi et al., 2015; Roder et al., 2016) by secreting the glucose-maintaining hormones, insulin and glucagon. A pancreas is an elongated organ localized behind the stomach and across the belly. A pancreas consists of an exocrine chamber of secretory cells that synthesizes digestive juice, further releases the juice into pancreatic duct, and an endocrine chamber of islet cells. These islet cells include α cells and β cells that do produce two vital hormones, glucagon and insulin for maintaining and regulating the blood glucose level (Moulle and Parnet, 2019). A schematic demonstrating the structure and constituents of a pancreas is shown in Figure 5.
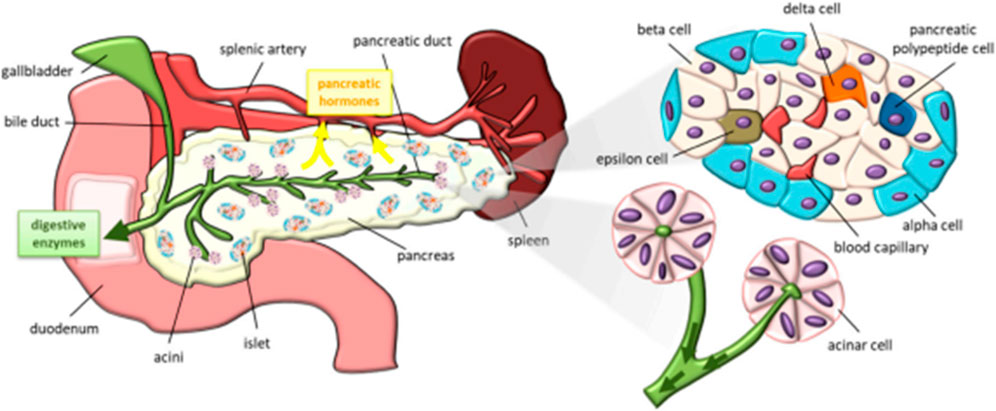
Figure 5. Schematic representation of a pancreas and its constituents [Reproduced from (Moulle and Parnet, 2019) with permission. Copyright 2019 MDPI].
When the function of a pancreas or liver is impaired, the regular glucose metabolism in the body will be disrupted, thereby leading to pre-diabetes or diabetes. Though chemotherapy via intake of drugs such as metformin or insulin therapy can be adopted as a treatment for T2D (the condition where the body is unable to use the produced insulin) or T1D (the body is unable to produce enough insulin) the drug-based or insulin therapy may become inadequate for patients during the course of time. In such a condition, the patients will be in need of pancreatic islets or pancreas transplant (Wszola et al., 2021). Islet transplantation is in forefront among the various strategies available for treating T1D. However, islet transplantation may unfortunately fail due to inevitable reasons such as immune response directed by the host to the transplanted cells, low viability and low functionality of islet cells.
Transplantation of bioartificial organs (bioartificial pancreas) is an attractive strategy that can be employed to circumvent the above-mentioned drawbacks. Fabrication of a bioartificial pancreas is a complicated process and utmost care should be taken with respect to several aspects notably source of islet cells, biocompatibility to the host, possession of vascularization network and enriched nutrient supply. Figure 6 presents the schematic indicating the various factors that influence the fabrication of a bioartificial pancreas (Soetedjo et al., 2021).
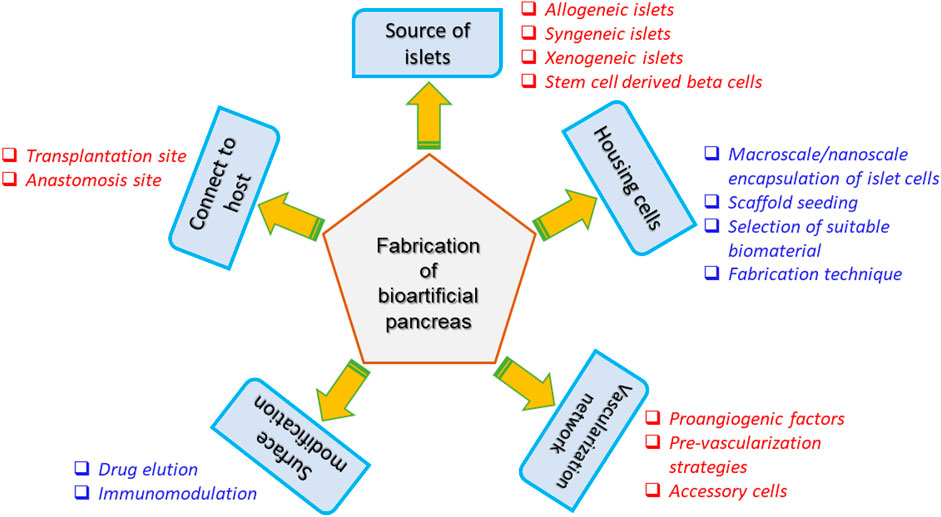
Figure 6. Considerations for the fabrication of bioartificial pancreas (Soetedjo et al., 2021).
3D Bioprinting can be employed to get rid of the drawback of graft rejection by the body through means of facilitating donor-independent T1D treatment strategy. A polymer chosen for bioprinting organs should possess the vital characteristics such biodegradable, biocompatible, 3D printable, crosslinkable and storable. In donor-independent T1D treatment strategy of restoring glucose homeostasis, the human stem cells will be cultured in vitro and differentiated into β cells (insulin-producing cells) and α cells (glucagon-producing cells). The β and α cells will be impregnated into the bioink for the 3D bioprinting of pancreas, which will be transplanted into patients (Wszola et al., 2021). Accordingly, Farina et al. (2017) fabricated a functionalized encapsulation system and examined its utility in the subcutaneous engraftment of islet-like cells (Farina et al., 2017). The encapsulation system was to offer protection against acute hypoxia, enhanced hydrophilicity, good cell attachment and proliferation properties (Farina et al., 2017).
The components in Extracellular matrix (ECM) influence the biological activity, mechanical stability and life span of the in vitro pancreatic culture (Daoud et al., 2011). This demands the fine-tuning of composition of ECM to ensure long-term usage and preservation of in vitro pancreatic cellular/tissue models. Daoud et al. (2011) examined the functions of human islet cells within the three various 3D extracellular matrices, namely, collagen I, collagen I with the ECM components fibronectin/collagen IV, and microfabricated scaffold with ECM-supplementation (Daoud et al., 2011). The researchers demonstrated that the 3D ECM containing the ECM components extended the life span of human islet culture. Surprisingly, the microfabricated scaffold with ECM-supplementation presented an insulin release behavior that is identical to that of the freshly isolated pancreatic islets (Daoud et al., 2011).
Kim et al. (2019) conducted a study and proposed that the pancreatic tissue-derived extracellular matrix can function as a possible candidate to mimic the native microenvironment in transplantable 3D pancreatic tissues through the observations from their research (Kim et al., 2019).
Concurrently, Klak et al. (2023) biofabricated 3D bionic scaffolds of islet cells with dECM (decellularized extracellular matrix)-based bioink using an extrusion-based 3D bioprinter, and investigated its activity on the murine models (Klak et al., 2023). The 3D bioprinted pancreatic petals were found to continue the secretion of insulin and neovascularization after transplantation, thereby dropping the plasma glucose concentration in the murine models (Klak et al., 2023). Also, Kavand et al. (2023) constructed a microstructure that can hold pancreatic islets in a specific location within the anterior chamber of the eye (ACE) and promote tissue engraftment (Kavand et al., 2023). Chen et al. (2022) reported the fabrication of 3D printed mini-capsule device containing islets that can be used for the delivery of islet cells as a treatment of T1D (Chen et al., 2022). DLP-based printing method with an exposure time of 20 s (the exposure time was confined to 20 s in order to ensure long-term survival of the islet cells) was adopted to construct the mini-capsule devices with a groove structure (the groove structure prevents the leakage of islet cells due to gravity settlement) using gelatin methacrylate (GelMA) (Chen et al., 2022). Upon subcutaneous transplantation of the encapsulated islet cells into an immunocompetent mice model, the researchers observed that the islet cells reduced the Foreign Body Reaction (FBR) from host to graft and also reduced the hyperglycemic symptoms without using immunosuppressant for long-term proving to be an effective treatment for T1D (Chen et al., 2022). Similarly, Hwang et al. (2021) designed a 3D bioprinted hybrid encapsulation system containing pancreatic islets as a treatment strategy for T1D (Hwang et al., 2021). The hybrid encapsulation system comprises an outer part polymer capsule (microporous in nature) and an inner part dECM hydrogel with pancreatic islet-like aggregates (nanoporous in nature). The 3D bioprinted hybrid encapsulation system was proven to possess enhanced therapeutic potential by directing structural maturation and functional enhancement of cells (Hwang et al., 2021).
3.3.2 Management of diabetes-associated bone defects
The hyperglycemic condition and altered metabolism in T1D and T2D patients enhance the risk of fracture, impairs fracture healing and interferes with bone forming process (Jiao et al., 2015; Xu et al., 2023). Diabetic micromilieu with chronic inflammation and Diabetic Infectious Micromilieu (DIM) play a major role in the impairment of a bone regeneration process (Li et al., 2022; Xu et al., 2023). Recently, Jiang et al. (2023) 3D bioprinted the scaffolds made up of GelMA and strontium (Sr)-holding bioactive glass nanoparticles (Sr-MBGNs: mesoporous in nature) with a goal of remodeling the pathological diabetic micromilieu into immunomodulating nanocomposite with enhanced angiogenic, osteogenic and anti-inflammatory properties. The researchers observed that the Sr-MBGNs aided as a biomineralization precursor, in turn stimulated the release of calcium, silicon and strontium ions, thereby ensuring bone regeneration efficiency (Xu et al., 2023).
Interestingly, Yang et al. (2021) developed a 3D bioprinted enzyme-functionalized scaffold that performs multiple functions such as angiogenesis, anti-inflammation and osteogenesis (Yang et al., 2021). The so-called “multifunctional scaffold” was composed of alginate polymer, catalase-assisted biomineralized calcium phosphate nanosheets (CaP@CAT NSs) and glucose oxidase (GOx) enzyme. The alginate offered mechanical strength to the scaffold while GOx reduced the hyperglycemic environment by oxidizing glucose into gluconic acid and hydrogen peroxide (H2O2). The CaP@CAT NSs in scaffold was an important constituent of the scaffold for the scavenging of H2O2 molecules. This overall process induced creation of a hypoxic microenvironment, which in turn stimulated neovascularization, thereby promoting bone regeneration (Yang et al., 2021).
Osteogenesis inhibition caused by the stem cell dysfunction is another factor responsible for the impairment of a bone regeneration process in a diabetic patient (Chen et al., 2022). Researchers have observed that activation of Glycogen Synthase Kinase-3β (GSK-3β) by high glucose leads to osteogenesis inhibition by stem cell dysfunction (Zhang et al., 2016; Chen et al., 2021; Chen et al., 2022). As a strategy to promote osteogenesis by inhibiting GSK-3β, Chen et al. (2022) fabricated lithium-based mesoporous bioactive glass (for in vitro study) and lithium-based mesoporous bioactive glass/Poly Lactic-co-Glycolic Acid (PLGA) composite scaffold by 3D printing (for study in diabetic mice), followed by investigation of osteogenesis effect of the scaffolds (Chen et al., 2022). The researchers concluded that the scaffolds were effective enough in reversing the suppression of osteogenic differentiation by augmentation of Itga3 and activation of β-catenin/Tcf7/Ccn4 signaling pathway (Chen et al., 2022).
“Critical-sized bone defect” is a kind of bone defect that fails to heal spontaneously without surgical intervention because of the fact that its size surpasses its intrinsic healing potential (Schemitsch, 2017; Roddy et al., 2018). Sun et al. (2021) fabricated 3D bioprinted scaffolds using bioink composed of polymers gelatin, GelMA, 4-arm poly (ethylene glycol) acrylate (PEG), BMP-4 (Bone Morphogenetic Protein-4)-loaded mesoporous silica nanoparticles (MSNs), RAW264.7 macrophages (a macrophage cell line) and BMSCs (bone marrow stromal cells) (Sun et al., 2021). The researchers observed that the implantation of the scaffold in calvarial critical-size defect models of diabetic rats promoted osteogenic differentiation of BMSCs and this enhanced the bone repair in rat models (Sun et al., 2021).
3.4 3D drug testing models
3D in vitro models are the excellent therapeutic screening tools as they can mimic the exact the microenvironment of biological system. Kim et al. (2021) fabricated 3D bioprinted in vitro models of diseased skin containing the pathophysiological hallmarks such as the adipocyte hypertrophy, inflammatory reactions, insulin resistance and vascular dysfunction found explicitly in patients with T2D (Kim et al., 2021). The developed in vitro model was found to exhibit slow re-epithelization is one of the important hallmarks of diabetic skin. The research team surmised that upon stimulating an intercellular crosstalk between epidermis and dermis, the normal keratinocytes could be differentiated into diabetic epidermis by interacting with diabetic dermis (Kim et al., 2021). The researchers illustrated that this diabetic skin model can act as a perfect disease model for its use in drug development (Kim et al., 2021).
4 Challenges and future perspectives
The 3D printing and bioprinting technologies are expensive. This factor limits their affordability for their use in the manufacture of 3D printed medicines/bioprinted organs for public use. Most of the research related to 3D printing and bioprinting are still limited to lab scale and translational research needs to be given more importance among the researchers. Importance should be given towards understanding the impact of 3D printed and bioprinted products in biological systems by conducting pre-clinical studies and case studies wherever applicable. Secondly, though 3D printing technology can afford fabrication of pharmaceuticals with varying sizes and shapes, the production of anti-diabetic drug formulations with customized doses depending on the age and other factors of patients has not been explored till date. In the current scenario, research and development on personalization or individualization is sparse. Hence, significant importance ought to be provided to the development of patient-centric pharmaceutical/biomedical products rather than the “one-size-fits-all” medication types. Thirdly, efforts have to be improved to obtain Food and Drug Administration (FDA)-approval for the 3D printed and bioprinted products. Till date, Spritam is the only 3D printed medicine that has been approved for use by the Food and Drug Administration (FDA). Therefore, more emphasize need to be given towards 3D printed/bioprinted product development, marketing and its utility for public use. In vitro drug testing models, identification of solutions for the ethical issues against the use of 3D printed or bioprinted products is another area to be focused on.
Author contributions
IS: Conceptualization, Writing–original draft, Writing–review and editing.
Funding
The author(s) declare that no financial support was received for the research, authorship, and/or publication of this article.
Acknowledgments
The author gratefully acknowledges National Institute of Technology Calicut (NITC) for the support.
Conflict of interest
The author declares that the research was conducted in the absence of any commercial or financial relationships that could be construed as a potential conflict of interest.
Publisher’s note
All claims expressed in this article are solely those of the authors and do not necessarily represent those of their affiliated organizations, or those of the publisher, the editors and the reviewers. Any product that may be evaluated in this article, or claim that may be made by its manufacturer, is not guaranteed or endorsed by the publisher.
References
Acierno, D., and Patti, A. (2023). Fused deposition modelling (FDM) of thermoplastic-based filaments: process and rheological properties – an overview. Mater 16, 7664. doi:10.3390/ma16247664
Ahmad, J., Garg, A., Mustafa, G., Mohammed, A. A., and Ahmad, M. Z. (2023). 3D printing technology as a promising tool to design nanomedicine-based solid dosage forms: contemporary research and future scope. Pharm 15, 1448. doi:10.3390/pharmaceutics15051448
Ahmed, T. A., Felimban, R. I., Tayeb, H. H., Rizg, W. Y., Alnadwi, F. H., Alotaibi, H. A., et al. (2021). Development of multi-compartment 3D-printed tablets loaded with self-nanoemulsified formulations of various drugs: a new strategy for personalized medicine. Pharmaceutics 13, 1733. doi:10.3390/pharmaceutics13101733
Alizadehgiashi, M., Nemr, C. R., Chekini, M., Ramos, D. P., Mittal, N., Ahmed, S. U., et al. (2021). Multifunctional 3D-printed wound dressings. ACS Nano 15 (7), 12375–12387. doi:10.1021/acsnano.1c04499
Alkilani, A. Z., McCrudden, M. T. C., and Donnelly, R. F. (2015). Transdermal drug delivery: innovative pharmaceutical developments based on disruption of the barrier properties of the stratum corneum. Pharmaceutics 7 (4), 438–470. doi:10.3390/pharmaceutics7040438
Bajuri, M. Y., Kim, J., Yu, Y., and Hameed, M. S. S. (2023). New paradigm in diabetic foot ulcer grafting techniques using 3D-bioprinted autologous minimally manipulated homologous adipose tissue (3D-AMHAT) with fibrin gel acting as a biodegradable scaffold. Gels 9, 66. doi:10.3390/gels9010066
Berbudi, A., Rahmadika, N., Tjahjadi, A. I., and Ruslami, R. (2020). Type 2 diabetes and its impact on the immune system. Curr. Diabetes Rev. 16 (5), 442–449. doi:10.2174/1573399815666191024085838
Centers for Disease Control and Prevention Diabetes basics. 2023 Available at: https://www.cdc.gov/diabetes/basics/diabetes.html (Accessed 30 December, 2023).
Chakka, L. R. J., and Chede, S. (2023). 3D printing of pharmaceuticals for disease treatment. Front. Med. Technol. 4, 1040052. doi:10.3389/fmedt.2022.1040052
Chen, S., Luo, J., Shen, L., Liu, X., Wang, W., Xu, J., et al. (2022a). 3D printing mini-capsule device for islet delivery to treat type 1 diabetes. ACS Appl. Mat. Interfaces 14, 23139–23151. doi:10.1021/acsami.2c02487
Chen, Y., Chen, L., Huang, R., Yang, W., Chen, S., Lin, K., et al. (2021). Investigation for GSK3β expression in diabetic osteoporosis and negative osteogenic effects of GSK3β on bone marrow mesenchymal stem cells under a high glucose microenvironment. Biochem. Biophys. Res. Commun. 534, 727–733. doi:10.1016/j.bbrc.2020.11.010
Chen, Y., Chen, L., Wang, Y., Lin, K., and Liu, J. (2022b). Lithium-containing bioactive glasses enhanced 3D-printed PLGA scaffolds for bone regeneration in diabetes. Compos. Part B Eng. 230, 109550. doi:10.1016/j.compositesb.2021.109550
Cui, M., Pan, H., Su, Y., Fang, D., Qiao, S., Ding, P., et al. (2021). Opportunities and challenges of three-dimensional printing technology in pharmaceutical development. Acta Pharm. Sin. B 11 (8), 2488–2504. doi:10.1016/j.apsb.2021.03.015
Cui, M., Yang, Y., Jia, D., Li, P., Li, Q., Chen, F., et al. (2019). Effect of novel internal structures on printability and drug release behavior of 3D printed tablets. J. Drug Deliv. Sci. Technol. 49, 14–23. doi:10.1016/j.jddst.2018.10.037
Daoud, J. T., Petropavlovskaia, M. S., Patapas, J. M., Degrandpre, C. E., DiRaddo, R. W., Rosenberg, L., et al. (2011). Long-term in vitro human pancreatic islet culture using three-dimensional microfabricated scaffolds. Biomaterials 32, 1536–1542. doi:10.1016/j.biomaterials.2010.10.036
Dassault Systèmes (2024). SLA - stereolithography. Available at: https://www.3ds.com/make/service/3d-printing-service/sla-stereolithography (Accessed October 04, 2024).
Ding, X., Yu, Y., Li, W., and Zhao, Y. (2023). In situ 3D-bioprinting MoS2 accelerated gelling hydrogel scaffold for promoting chronic diabetic wound healing. Matter 6, 1000–1014. doi:10.1016/j.matt.2023.01.001
Economidou, S. N., Pere, C. P. P., Okereke, M., and Douroumis, D. (2021). Optimisation of design and manufacturing parameters of 3D printed solid microneedles for improved strength, sharpness, and drug delivery. Micromachines 12 (2), 117. doi:10.3390/mi12020117
Economidou, S. N., Pere, C. P. P., Reid, A., Uddin, M. J., Windmill, J. F. C., Lamprou, D. A., et al. (2019). 3D printed microneedle patches using stereolithography (SLA) for intradermal insulin delivery. Mat. Sci. Eng. C 102, 743–755. doi:10.1016/j.msec.2019.04.063
Farina, M., Ballerini, A., Fraga, D. W., Nicolov, E., Hogan, M., Demarchi, D., et al. (2017). 3D printed vascularized device for subcutaneous transplantation of human islets. Biotechnol. J. 12, 1700169. doi:10.1002/biot.201700169
Ferroni, L., D’Amora, U., Gardin, C., Leo, S., Paola, L. D., Tremoli, E., et al. (2023). Stem cell-derived small extracellular vesicles embedded into methacrylated hyaluronic acid wound dressings accelerate wound repair in a pressure model of diabetic ulcer. J. Nanobiotechnology 21, 469. doi:10.1186/s12951-023-02202-9
Fratini, C., Weaver, E., Moroni, S., Irwin, R., Bashi, Y. H. D., Uddin, S., et al. (2023). Combining microfluidics and coaxial 3D-bioprinting for the manufacturing of diabetic wound healing dressings. Biomater. Adv. 153, 213557. doi:10.1016/j.bioadv.2023.213557
Freedman, B. R., Hwang, C., Talbot, S., Hibler, B., Matoori, S., and Mooney, D. J. (2023). Breakthrough treatments for accelerated wound healing. Sci. Adv. 9 (20), eade7007. doi:10.1126/sciadv.ade7007
Game, F. (2008). The advantages and disadvantages of non-surgical management of the diabetic foot. Diabetes/metabolism Res. Rev. 24 (Suppl. 1), S72–S75. doi:10.1002/dmrr.816
Gioumouxouzis, C. I., Baklavaridis, A., Katsamenis, O. L., Markopoulou, C. K., Bouropoulos, N., Tzetzis, D., et al. (2018). A 3D printed bilayer oral solid dosage form combining metformin for prolonged and glimepiride for immediate drug delivery. Eur. J. Pharm. Sci. 120, 40–52. doi:10.1016/j.ejps.2018.04.020
Glover, K., Mathew, E., Pitzanti, G., Magee, E., and Lamprou, D. A. (2023). 3D bioprinted scaffolds for diabetic wound-healing applications. Drug Deliv. 13, 2096–2109. doi:10.1007/s13346-022-01115-8
Guo, L., Niu, X., Chen, X., Lu, F., Gao, J., and Chang, Q. (2022). 3D direct writing egg white hydrogel promotes diabetic chronic wound healing via self-relied bioactive property. Biomater 282, 121406. doi:10.1016/j.biomaterials.2022.121406
Guo, S., and DiPietro, L. A. (2010). Factors affecting wound healing. J. Dent. Res. 89 (3), 219–229. doi:10.1177/0022034509359125
Hong, S., Kim, J. S., Jung, B., Won, C., and Hwang, C. (2019). Coaxial bioprinting of cell-laden vascular constructs using a gelatin-tyramine bioink. Biomater. Sci. 7, 4578–4587. doi:10.1039/C8BM00618K
Hu, Y., Wu, B., Xiong, Y., Tao, R., Panayi, A. C., Chen, L., et al. (2021). Cryogenic 3D printed hydrogel scaffolds loading exosomes accelerate diabetic wound healing. Chem. Eng. J. 426, 130634. doi:10.1016/j.cej.2021.130634
Hu, Y., Xiong, Y., Zhu, Y., Zhou, F., Liu, X., Chen, S., et al. (2023). Copper-epigallocatechin gallate enhances therapeutic effects of 3D-printed dermal scaffolds in mitigating diabetic wound scarring. ACS Appl. Mat. Interfaces 15 (32), 38230–38246. doi:10.1021/acsami.3c04733
Huang, J., Yang, R., Jiao, J., Li, Z., Wang, P., Liu, Y., et al. (2023). A click chemistry-mediated all-peptide cell printing hydrogel platform for diabetic wound healing. Nat. Commun. 14, 7856. doi:10.1038/s41467-023-43364-2
Huang, Q., Wu, T., Wang, L., Zhu, J., Guo, Y., Yu, X., et al. (2022). A multifunctional 3D dressing unit based on the core-shell hydrogel microfiber for diabetic foot wound healing. Biomater. Sci. 10, 2568–2576. doi:10.1039/D2BM00029F
Hudak, Y. F., Li, J., Cullum, S., Strzelecki, B. M., Richburg, C., Kaufman, G. E., et al. (2022). A novel workflow to fabricate patient-specific 3D printed accommodative foot orthosis with personalized latticed metamaterial. Med. Eng. Phys. 104, 103802. doi:10.1016/j.medengphy.2022.103802
Hwang, D. G., Jo, Y., Kim, M., Yong, U., Cho, S., Choi, Y., et al. (2021). A 3D bioprinted hybrid encapsulation system for the delivery of human pluripotent stem cell-derived pancreatic islet-like aggregates. Biofabrication 14, 014101. doi:10.1088/1758-5090/ac23ac
Ibrahim, M., Barnes, M., McMillin, R., Cook, D. W., Smith, S., Halquist, M., et al. (2019). 3D printing of metformin HCl PVA tablets by fused deposition modeling: drug loading, tablet design, and dissolution studies. AAPS PharmSciTech 20, 195. doi:10.1208/s12249-019-1400-5
Ilhan, E., Cesur, S., Guler, E., Topal, F., Albayrak, D., Guncu, M. M., et al. (2020). Development of Satureja cuneifolia-loaded sodium alginate/polyethylene glycol scaffolds produced by 3D-printing technology as a diabetic wound dressing material. Int. J. Biol. Macromol. 161, 1040–1054. doi:10.1016/j.ijbiomac.2020.06.086
India Today (2023) India Today World Diabetes Day 2023: Why is diabetes a ‘silent killer’? Available at: https://www.indiatoday.in/health/story/world-diabetes-day-2023-why-is-diabetes-a-silent-killer-2462684-2023-11-14 (Accessed 30 December, 2023).
Indian Institute of Science (2022). Snapping footwear help prevent diabetic foot complications. Available at: https://www.eurekalert.org/news-releases/955764 (Accessed 29 December, 2023).
International Diabetes Federation Facts and figures.2024 Available at: https://idf.org/about-diabetes/diabetes-facts-figures/ (Accessed 10 April, 2024).
Intini, C., Elviri, L., Cabral, J., Mros, S., Bergonzi, C., Bianchera, A., et al. (2018). 3D-printed chitosan-based scaffolds: an in vitro study of human skin cell growth and an in-vivo wound healing evaluation in experimental diabetes in rats. Carbohydr. Polym. 199, 593–602. doi:10.1016/j.carbpol.2018.07.057
Jiang, P., Li, Q., Luo, Y., Luo, F., Che, Q., Lu, Z., et al. (2023). Current status and progress in research on dressing management for diabetic foot ulcer. Front. Endocrinol. 14, 1–28. doi:10.3389/fendo.2023.1221705
Jiao, H., Xiao, E., and Graves, D. T. (2015). Diabetes and its effect on bone and fracture healing. Curr. Osteoporos. Rep. 13 (5), 327–335. doi:10.1007/s11914-015-0286-8
Kavand, H., Visa, M., Kohler, M., Wijngaart, W., Berggren, P., and Herland, A. (2023). 3D-Printed biohybrid microstructures enable transplantation and vascularization of microtissues in the anterior chamber of the eye. Adv. Mat. doi:10.1002/adma.202306686
Khaled, S. A., Burley, J. C., Alexander, M. R., Yang, J., and Roberts, C. J. (2015). 3D Printing of tablets containing multiple drugs with defined release profiles. Int. J. Pharm. 494 (2), 643–650. doi:10.1016/j.ijpharm.2015.07.067
Khan, R. M. M., Chua, Z. J. Y., Tan, J. C., Yang, Y., Liao, Z., and Zhao, Y. (2019). From pre-diabetes to diabetes: diagnosis, treatments and translational research. Medicina 55 (9), 546. doi:10.3390/medicina55090546
Kim, B. S., Ahn, M., Cho, W., Gao, G., Jang, J., and Cho, D. (2021). Engineering of diseased human skin equivalent using 3D cell printing for representing pathophysiological hallmarks of type 2 diabetes in vitro. Biomaterials 272, 120776. doi:10.1016/j.biomaterials.2021.120776
Kim, J., Shim, I. K., Hwang, D. G., Lee, Y. N., Kim, M., Kim, H., et al. (2019). 3D cell printing of islet-laden pancreatic tissue-derived extracellular matrix bioink constructs for enhancing pancreatic functions. J. Mat. Chem. B 7, 1773–1781. doi:10.1039/C8TB02787K
Kim, M. H., and Nam, S. Y. (2020). Assessment of coaxial printability for extrusion-based bioprinting of alginate-based tubular constructs. Bioprinting 20, e00092. doi:10.1016/j.bprint.2020.e00092
Kim, N., Lee, H., Han, G., Kang, M., Park, S., Kim, D. E., et al. (2023). 3D-Printed functional hydrogel by DNA-induced biomineralization for accelerated diabetic wound healing. Adv. Sci. 10, 2300816. doi:10.1002/advs.202300816
Kjar, A., McFarland, B., Mecham, K., Harward, N., and Huang, Y. (2021). Engineering of tissue constructs using coaxial bioprinting. Bioact. Mat. 6, 460–471. doi:10.1016/j.bioactmat.2020.08.020
Klak, M., Wszola, M., Berman, A., Filip, A., Kosowska, A., Olkowska-Truchanowicz, J., et al. (2023). Bioprinted 3D bionic scaffolds with pancreatic islets as a new therapy for type 1 diabetes-analysis of the results of preclinical studies on a mouse model. J. Funct. Biomater. 14, 371. doi:10.3390/jfb14070371
Kumar, A., Jain, D., Bahuguna, J., Bhaiyya, M., Dubey, S. K., Javed, A., et al. (2023). Machine learning assisted and smartphone integrated homogeneous electrochemiluminescence biosensor platform for sample to answer detection of various human metabolites. Biosens. Bioelectron. 238, 115582. doi:10.1016/j.bios.2023.115582
Kushmakov, R., Gandhi, J., Seyam, O., Jiang, W., Joshi, G., Smith, N. L., et al. (2018). Ozone therapy for diabetic foot. Med. Gas. Res. 8 (3), 111–115. doi:10.4103/2045-9912.241076
Li, B., Shu, R., Dai, W., Yang, F., Xu, H., Shi, X., et al. (2022). Bioheterojunction-engineered polyetheretherketone implants with diabetic infectious micromilieu twin-engine powered disinfection for boosted osteogenicity. Small 18 (45), 2203619. doi:10.1002/smll.202203619
Liu, T., Yang, B., Tian, W., Zhang, X., and Wu, B. (2022). Cryogenic coaxial printing for 3D shell/core tissue engineering scaffold with polymeric shell and drug-loaded core. Polymers 14, 1722. doi:10.3390/polym14091722
Liu, Y., Yu, Q., Luo, X., Yang, L., and Cui, Y. (2021). Continuous monitoring of diabetes with an integrated microneedle biosensing device through 3D printing. Microsyst. Nanoeng. 7, 75. doi:10.1038/s41378-021-00302-w
Maharana, P., Sonawane, J., Belehalli, P., and Ananthasuresh, G. K. (2022). Self-offloading therapeutic footwear using compliant snap-through arches. Wearable Technol. 3, e7. doi:10.1017/wtc.2022.2
Mancuso, M., Bulzomi, R., Mannisi, M., Martelli, F., and Giacomozzi, C. (2023). 3D-Printed insoles for people with type 2 diabetes: an Italian, ambulatory case report on the innovative care model. Diabetol. 4, 339–355. doi:10.3390/diabetology4030029
Moulle, V. S., and Parnet, P. (2019). Effects of nutrient intake during pregnancy and lactation on the endocrine pancreas of the offspring. Nutrients 11 (11), 2708. doi:10.3390/nu11112708
Muir, B. C., Li, J., Hudak, Y. F., Kaufman, G. E., Cullum, S., and Aubin, P. M. (2022). Evaluation of novel plantar pressure-based 3-dimensional printed accommodative insoles – a feasibility study. Clin. Biomech. 98, 105739. doi:10.1016/j.clinbiomech.2022.105739
Naik, K., Singh, P., Yadav, M., Srivastava, S. K., Tripathi, S., Ranjan, R., et al. (2023). 3D printable, injectable amyloid-based composite hydrogel of bovine serum albumin and aloe vera for rapid diabetic wound healing. J. Mat. Chem. B 11, 8142–8158. doi:10.1039/D3TB01151H
NIH Research Matters (2020). Poor immune response impairs diabetic wound healing. Available at: https://www.nih.gov/news-events/nih-research-matters/poor-immune-response-impairs-diabetic-wound-healing (Accessed 23 December, 2023).
Pere, C. P. P., Economidou, S. N., Lall, G., Ziraud, C., Boateng, J. S., Alexander, B. D., et al. (2018). 3D printed microneedles for insulin skin delivery. Int. J. Pharm. 544, 425–432. doi:10.1016/j.ijpharm.2018.03.031
Piaggio, D., Castaldo, R., Garibizzo, G., Iadanza, E., and Pecchia, L. (2024). A smartphone-based tool for screening diabetic neuropathies: a mHealth and 3D printing approach. Biomed. Signal Process. Control. 89, 105807. doi:10.1016/j.bspc.2023.105807
Pita-Vilar, M., Concheiro, A., Alvarez-Lorenzo, C., and Diaz-Gomez, L. (2023). Recent advances in 3D printed cellulose-based wound dressings: a review on in vitro and in vivo achievements. Carbohydr. Polym. 321, 121298. doi:10.1016/j.carbpol.2023.121298
Roddy, E., DeBaun, M. R., Daoud-Gray, A., Yang, Y. P., and Gardner, M. J. (2018). Treatment of critical-sized bone defects: clinical and tissue engineering. Eur. J. Orthop. Surg. Traumatol. 28 (3), 351–362. doi:10.1007/s00590-017-2063-0
Roder, P. V., Wu, B., Liu, Y., and Han, W. (2016). Pancreatic regulation of glucose homeostasis. Exp. Mol. Med. 48, e219. doi:10.1038/emm.2016.6
Schemitsch, E. H. (2017). Size Matters: defining critical in bone defect size. J. Orthop. Trauma 31, S20–S22. doi:10.1097/BOT.0000000000000978
Sharabi, K., Tavares, C. D. J., Rines, A. K., and Puigserver, P. (2015). Molecular pathophysiology of hepatic glucose production. Mol. Asp. Med. 46, 21–33. doi:10.1016/j.mam.2015.09.003
Soetedjo, A. A. P., Lee, J. M., Lau, H. H., Goh, G. L., An, J., Koh, Y., et al. (2021). Tissue engineering and 3D printing of bioartificial pancreas for regenerative medicine in diabetes. Trends Endocrinol. Metabolism 32, 609–622. doi:10.1016/j.tem.2021.05.007
Stranzinger, S., Wolfgang, M., Klotz, E., Scheibelhofer, O., Ghiotti, P., Khinast, J. G., et al. (2021). Near-infrared hyperspectral imaging as a monitoring tool for on-demand manufacturing of inkjet-printed formulations. AAPS PharmSciTech 22, 211. doi:10.1208/s12249-021-02091-x
Sun, X., Ma, Z., Zhao, X., Jin, W., Zhang, C., Ma, J., et al. (2021). Three-dimensional bioprinting of multicell-laden scaffolds containing bone morphogenic protein-4 for promoting M2 macrophage polarization and accelerating bone defect repair in diabetes mellitus. Bioact. Mater 6, 757–769. doi:10.1016/j.bioactmat.2020.08.030
Talebian, S., Shim, I. K., Foroughi, J., Orive, G., Vine, K. L., Kim, S. C., et al. (2021). 3D-Printed coaxial hydrogel patches with mussel-inspired elements for prolonged release of gemcitabine. Polymers. 13, 4367. doi:10.3390/polym13244367
Tripathi, S., Mandal, S. S., Bauri, S., and Maiti, P. (2023). 3D bioprinting and its innovative approach for biomedical applications. MedComm 4, e194. doi:10.1002/mco2.194
Tsegay, F., Elsherif, M., and Butt, H. (2022). Smart 3D printed hydrogel skin wound bandages: a review. Polymers 14 (5), 1012. doi:10.3390/polym14051012
Uchida, D. T., and Bruschi, M. L. (2023). 3D printing as a technological strategy for the personalized treatment of wound healing. AAPS PharmSciTech 24, 41. doi:10.1208/s12249-023-02503-0
Ventola, C. L. (2014). Medical applications for 3D printing: current and projected uses. Pharm. Ther. 39, 704–711.
Verisqa, F., Cha, J., Nguyen, L., Kim, H., and Knowles, J. C. (2022). Digital light processing 3D printing of gyroid scaffold with isosorbide-based photopolymer for bone tissue engineering. Biomol. 12, 1692. doi:10.3390/biom12111692
Volmer-Thole, M., and Lobmann, R. (2016). Neuropathy and diabetic foot syndrome. Int. J. Mol. Sci. 17, 917. doi:10.3390/ijms17060917
Wong, W. F., Ang, K. P., Sethi, G., and Looi, C. Y. (2023). Recent advancement of medical patch for transdermal drug delivery. Medicinia 59 (4), 778. doi:10.3390/medicina59040778
Wszola, M., Nitarska, D., Cywoniuk, P., Gomolka, M., and Klak, M. (2021). Stem cells as a source of pancreatic cells for production of 3D bioprinted bionic pancreas in the treatment of type 1 diabetes. Cells 10 (6), 1544. doi:10.3390/cells10061544
Wu, M., Zhang, Y., Huang, H., Li, J., Liu, H., Guo, Z., et al. (2020). Assisted 3D printing of microneedle patches for minimally invasive glucose control in diabetes. Mat. Sci. Eng. C 117, 111299. doi:10.1016/j.msec.2020.111299
Xu, Z., Qi, X., Bao, M., Zhou, T., Shi, J., Xu, Z., et al. (2023). Biomineralization inspired 3D printed bioactive glass nanocomposite scaffolds orchestrate diabetic bone regeneration by remodeling micromilieu. Bioact. Mater 25, 239–255. doi:10.1016/j.bioactmat.2023.01.024
Yang, C., Zheng, Z., Younis, M. R., Dong, C., Chen, Y., Lei, S., et al. (2021). 3D printed enzyme-functionalized scaffold facilitates diabetic bone regeneration. Adv. Func. Mat. 31, 2101372. doi:10.1002/adfm.202101372
Yi, H., Kim, H., Kwon, J., Choi, Y., Jang, J., and Cho, D. (2021). Application of 3D bioprinting in the prevention and the therapy for human diseases. Signal Transduct. Target Ther. 6, 177. doi:10.1038/s41392-021-00566-8
You, F., Eames, B. F., and Chen, X. (2017). Application of extrusion-based hydrogel bioprinting for cartilage tissue engineering. Int. J. Mol. Sci. 18, 1597. doi:10.3390/ijms18071597
Zaino, B., Goel, R., Devaragudi, S., Prakash, A., Vaghamashi, Y., Sethi, Y., et al. (2023). Diabetic neuropathy: pathogenesis and evolving principles of management. Disease-a-Month 69 (9), 101582. doi:10.1016/j.disamonth.2023.101582
Zhang, B., Liu, N., Shi, H., Wu, H., Gao, Y., He, H., et al. (2016). High glucose microenvironments inhibit the proliferation and migration of bone mesenchymal stem cells by activating GSK3β. J. Bone Min. Metab. 34 (2), 140–150. doi:10.1007/s00774-015-0662-6
Zhong, Y., Ma, H., Lu, Y., Cao, L., Cheng, Y. Y., Tang, X., et al. (2023). Investigation on repairing diabetic foot ulcer based on 3D bio-printing Gel/dECM/Qcs composite scaffolds. Tissue Cell 85, 102213. doi:10.1016/j.tice.2023.102213
Keywords: diabetes, 3D printing, 3D bioprinting, chronic complications, diagnosis, treatment, cell-seeded hydrogels, diabetic disease models
Citation: Sathisaran I (2024) 3D printing and bioprinting in the battle against diabetes and its chronic complications. Front. Bioeng. Biotechnol. 12:1363483. doi: 10.3389/fbioe.2024.1363483
Received: 30 December 2023; Accepted: 22 April 2024;
Published: 28 May 2024.
Edited by:
Sudipto Datta, Indian Institute of Science (IISc), IndiaReviewed by:
Bodhisatwa Das, Indian Institute of Technology Ropar, IndiaDana Akilbekova, Nazarbayev University, Kazakhstan
Copyright © 2024 Sathisaran. This is an open-access article distributed under the terms of the Creative Commons Attribution License (CC BY). The use, distribution or reproduction in other forums is permitted, provided the original author(s) and the copyright owner(s) are credited and that the original publication in this journal is cited, in accordance with accepted academic practice. No use, distribution or reproduction is permitted which does not comply with these terms.
*Correspondence: Indumathi Sathisaran, indumathis@nitc.ac.in