- 1Elisabeth Bruyère Hospital, Ottawa, ON, Canada
- 2Bone and Joint Research Laboratory, Ottawa Hospital Research Institute, Ottawa, ON, Canada
- 3Department of Cellular and Molecular Medicine, Faculty of Medicine, University of Ottawa, Ottawa, ON, Canada
- 4The Ottawa Hospital, Department of Medicine, Division of Physical Medicine and Rehabilitation, Ottawa, ON, Canada
Focal cartilage defects are common in youth and older adults, cause significant morbidity and constitute a major risk factor for developing osteoarthritis (OA). OA is the most common musculoskeletal (MSK) disease worldwide, resulting in pain, stiffness, loss of function, and is currently irreversible. Research into the optimal regenerative approach and methods in the setting of either focal cartilage defects and/or OA holds to the ideal of resolving both diseases. The two fundamentals required for cartilage regenerative treatment are 1) the biological element contributing to the regeneration (e.g., direct application of stem cells, or of an exogenous secretome), and 2) the vehicle by which the biological element is suspended and delivered. The vehicle provides support to the regenerative process by providing a protective environment, a structure that allows cell adherence and migration, and a source of growth and regenerative factors that can activate and sustain regeneration. Models of cartilage diseases include osteochondral defect (OCD) (which usually involve one focal lesion), or OA (which involves a more diffuse articular cartilage loss). Given the differing nature of these models, the optimal regenerative strategy to treat different cartilage diseases may not be universal. This could potentially impact the translatability of a successful approach in one condition to that of the other. An analogy would be the repair of a pothole (OCD) versus repaving the entire road (OA). In this narrative review, we explore the existing literature evaluating cartilage regeneration approaches for OCD and OA in animal then in human studies and the vehicles used for each of these two conditions. We then highlight strengths and challenges faced by the different approaches presented and discuss what might constitute the optimal cartilage regenerative delivery vehicle for clinical cartilage regeneration.
1 Introduction
Focal cartilage defects are common and cause significant morbidity, including pain, swelling, mechanical symptoms (such as a feeling of joint instability), and functional disability (Mandelbaum et al., 1998; Liu et al., 2022). Over a quarter of asymptomatic middle-aged individuals with no family history of cartilage loss were found to have some degree of focal cartilage defect; those with a family history of knee osteoarthritis (OA) demonstrated a cartilage defect prevalence of >50% (Ding et al., 2007; Everhart et al., 2019). In those with symptomatic knee OA, cartilage defects have a prevalence of >80% (Ding et al., 2007; Everhart et al., 2019). Correspondingly, cartilage defects represent an underrecognized, yet major risk factor for the development of OA (Mandelbaum et al., 1998; Arøen et al., 2004; Cicuttini et al., 2005; Ding et al., 2007; Guermazi et al., 2017; Everhart et al., 2019; Liu et al., 2022). While loss of the articular cartilage is often described as the hallmark of OA, OA is a total joint disease also affecting bone, synovium, meniscus and other intra- and extra-articular tissues such as ligament and muscle (Barr et al., 2015; Martel-Pelletier et al., 2016; Cunha et al., 2019; Hunter and Bierma-Zeinstra, 2019). Pain, loss of function, joint stiffness and reduced quality of life are well-described sequelae of OA (Barr et al., 2015; Martel-Pelletier et al., 2016; Hunter and Bierma-Zeinstra, 2019; Campbell and McGonagle, 2020). Additionally, while cartilage defects are a risk factor for OA (Martel-Pelletier et al., 2016; Hunter and Bierma-Zeinstra, 2019), OA can occur independently of a pre-established focal cartilage defect (Ding et al., 2007; Everhart et al., 2019). In addition, detection of focal cartilage defects requires advanced imaging (e.g., MRI), and may not be appreciated until more advanced stages. As such, patients will often present for medical treatment at more advanced stages of cartilage degeneration and joint dysfunction, with OA already established (Finley et al., 2018). The optimal method of regenerating cartilage for both focal cartilage defects and OA is actively researched (Clinicaltrials.gov, 2023) with the hope of discovering a treatment that could resolve both pathologies.
Stem cells have been delivered to cartilage lesions (both focal cartilage defects and OA) in the clinical setting before basic or translational evidence supported the regenerative efficacy of the various approaches (Marks et al., 2017; Canada, 2019; ISfSC, 2021). Stem cell delivery was rapidly extended to clinical practice, often at high cost to the patients (Marks et al., 2017; Canada, 2019; ISfSC, 2021). These two factors led national healthcare governing bodies to step in and limit this practice (Marks et al., 2017; Canada, 2019; ISfSC, 2021). The upside was an expansion of the basic science and translational research in this area to build a more solid foundation upon which to eventually base clinical regenerative treatment. Two major components of this regenerative treatment include 1) the biological element contributing to the regeneration (e.g., stem cells, extravesicular vesicles), and 2) the vehicle by which these biological elements are delivered (e.g., organic fluids, engineered scaffolds) (Campbell et al., 2022). Proposed advantages of using stem cells or EVs as the biological element have been reviewed previously (Kim et al., 2020; Taghiyar et al., 2021; Campbell et al., 2022) and include a direct ability to repair damaged cartilage (for stem cells), immunomodulation capacity to reduce inflammation within the OA joint, as well as paracrine effects that recruit other endogenous regenerative cells to the site of injury. While these potential advantages have been described for stem cells and EVs, there is currently no consensus regarding which method is more advantageous. The vehicle should be considered equally important to the biological element as it supports the regenerative process. A protective 3-dimensional (3D) environment may preserve stem cells’ ability to differentiate; a structure may allow cell adherence and migration; a source of growth and regenerative factors may activate and sustain regeneration (Kim et al., 2019).
Various animal models of cartilage injury have been described, such as: focal osteochondral defects (OCD; a localized osteochondral defect surgically imposed through the articular surface) and various OA models such as the destabilization of the medial meniscus (DMM) model, the anterior cruciate ligament transection (ACLT) model, and chemical models for generalized OA-like cartilage loss; these models are described in detail by Cully (2015) (Culley et al., 2015) and Cope (2019) (Cope et al., 2019). OCD versus OA models cause different sizes and distributions of cartilage injuries, and potentially differing endogenous intra-articular responses. Consequently, the model may impact the optimal regenerative delivery vehicle selected to the specific injury or condition in need of repair when moving from translational to clinical therapy (Liu et al., 2022).
In this narrative review, we evaluated the existing literature on cartilage regeneration both focal OCDs and OA in both animal models and clinical trials. Since the optimal stem cell population (Campbell et al., 2022), or use of their secretome (Kim et al., 2020; Taghiyar et al., 2021), for cartilage regeneration has been reviewed elsewhere, we focus here on the vehicles used in these studies and consider both the context in which they were used, as well as their general applicability to focal OCDs and OA. We conclude by looking towards the application of scaffold technology in clinical trials.
2 Animal models of focal osteochondral defects
The last decade saw advancements in vehicle engineering to treat focal OCDs in animal models (Lam et al., 2014; Shimomura et al., 2014; Li et al., 2015a; Dashtdar et al., 2015; Ha et al., 2015; Goodrich et al., 2016; Park et al., 2017; Ruan et al., 2017; Li et al., 2018; Lu et al., 2018; Muttigi et al., 2018; Ye et al., 2018; Jia et al., 2019; Jin et al., 2019; Kondo et al., 2019; Park et al., 2019; Pascual-Garrido et al., 2019; Wang et al., 2019; Xu et al., 2019; Cho et al., 2020; Wu et al., 2020; You et al., 2020; Deng et al., 2021; Heirani-Tabasi et al., 2021; Murata et al., 2022; Zheng et al., 2022) (Table 1). In the rat, intra-articular needle injection delivered the vehicle, such as hyaluronic acid (HA) hydrogels (Li et al., 2018; Xu et al., 2019). While direct placement of the vehicle into the OCD, a more precise delivery of the vehicle is limited by the technical challenges of placing a pre-formed scaffold in a small lesion (∼1.5–2 mm in width and <1 mm in depth in rats, smaller in mice). Two studies reported improved histologic-based cartilage scoring outcomes after implanting a gel or cell pellet scaffold into the OCD of the rat knee (Xu et al., 2019; Zheng et al., 2022). The most common model however remains the rabbit knee OCD (Table 1); providing a relatively large joint for surgical scaffold implantation handled with the naked eye and relatively lower cost (versus dog or horse, for example,). In the mid-2010s, vehicles used in rabbit OCD models included bilayered hydrogels that cross-linked at body temperature (Lam et al., 2014), synthetic hydroxyapatite (Shimomura et al., 2014) and Polyvinyl alcohol-chitosan composite hydrogel (Dashtdar et al., 2015), implanted during open knee surgery. Nanofiber scaffolds, composed of ultra-fine biodegradable polymeric fibers that simulate the natural extracellular matrix (ECM), have also emerged in animal OCD studies (Kazemnejad et al., 2017; Ahmadian et al., 2023). Nanofiber scaffolds may be constructed using different techniques, including electrospinning, self-assembly, phase separation, and drawing (Ahmadian et al., 2023). Natural nanofiber hydrogel scaffolds (scaffolds with high water content and elastic properties with cross-linked, multiporous networks (Li et al., 2019)) include alginate, gelatin, agarose, HA, fibrin, and collagen, while synthetic scaffolds often contain polycaprolactone, polyethylene glycol, polyurethane, poly (pdioxanone), and/or poly (lactic acid) (Kazemnejad et al., 2017; Ahmadian et al., 2023). For the interested reader, reviews describing the advantages of nanofiber scaffold technology are available (Coburn et al., 2012; Kazemnejad et al., 2017; Ahmadian et al., 2023).
Since the evolution of nanofiber technology, more sophisticated vehicles have been bioprinted that incorporated distinct osteogenic and chondrogenic regions or zones matching the anatomy of the predefined OCD (Yilmaz et al., 2020). For example, 2D-nanopattern chondroitin sulfate-coated scaffolds featured an inverse pattern of nanograting (250-nm line, 250-nm space, and 150-nm height) or nanohole (225-nm diameter, 400-nm pitch, and 300-nm height) patterning with cells delivered as a bilayered construct (Wu et al., 2020). In doing so, it was hypothesized that stem cells differentiated on specific nanotopographic patterns based on a zonally stratified cartilage construct would possess topographically induced mechanical memory, and could thus retain an induced chondrogenic phenotype (Wu et al., 2020). Correspondingly, these constructs included layer-defined pre-differentiation of incorporated mesenchymal stem cells (MSCs) to enhance their osteogenic and/or chondrogenic abilities, depending on where they were implanted (subchondral bone or articular surface) (Wu et al., 2020). In addition to stem cells, various growth factors have also been incorporated into these scaffolds to enhance anabolic cellular activity. For example, a biphasic scaffold utilizing gelatin methacryloyl (GM) in one phase and parathyroid hormone (PTH) covalently immobilized on silk fibroin (SF) with methacrylic anhydride (MA) in a second resulted in a corresponding biomechanical gradient due to the differing physical properties of the constituents (Deng et al., 2021). Benefits of potential growth factor use, such as insulin-like growth factor, fibroblast growth factors, bone morphogenic proteins and transforming growth factor beta, for cartilage regeneration have recently been reviewed (Hernigou et al., 2022; Trippel, 2023); however a growth factor that robustly augments articular cartilage healing remains elusive (Trippel, 2023).
Another interesting approach to OCD repair was injecting a mimetic hydrogel composed of polyethylene glycol, chondroitin sulfate, a matrix metalloprotein-2 degradable peptide crosslinker, and arginyl-glycyl-aspartic acid (Pascual-Garrido et al., 2019). This scaffold allowed cells encapsulation during the hydrogel formation process, with the final combination being formed in situ within the articular chondral defect, then cross-linked to maintain shape (Pascual-Garrido et al., 2019). In this study, the authors included the matrix metalloprotein-2 degradable peptide cross-linking photoinitiator (CVPLSLYSGC) that polymerized under blue light. After the defect was generated, a red light in the operating room allowed defect visualization and polymer delivery. Polymer solution was then injected onto the chondral defect, then photopolymerization was performed in situ by use of a 405-nm blue light in order to establish the final placing of the customized regenerative material (Pascual-Garrido et al., 2019). The majority of small animal OCD studies reported positive structural outcomes following stem cells delivery.
Larger animals studies of OCD have included the use of HA hydrogel (Ha et al., 2015) and hanging drop MSC aggregates (Kondo et al., 2019) in minipigs, as well as platelet-enhanced fibrin (Goodrich et al., 2016) and 3D-based microspheroids (Murata et al., 2022) in horses. These proof-of-concept studies (Table 1) often reported positive structural outcomes following stem cell application.
Overall, scaffolds used over the last decade evaluating the treatment of OCDs in animal models have been roughly evenly divided between engineered hydrogels (Lam et al., 2014; Ha et al., 2015; Park et al., 2017; Li et al., 2018; Lu et al., 2018; Jia et al., 2019; Pascual-Garrido et al., 2019; Wang et al., 2019; Xu et al., 2019; Cho et al., 2020), engineered nanofiber products (Shimomura et al., 2014; Li et al., 2015a; Dashtdar et al., 2015; Ruan et al., 2017; Jin et al., 2019; Kondo et al., 2019; Wu et al., 2020; Deng et al., 2021; Murata et al., 2022), and biological products (Goodrich et al., 2016; Muttigi et al., 2018; Ye et al., 2018; Park et al., 2019; You et al., 2020; Heirani-Tabasi et al., 2021; Zheng et al., 2022) (Figure 1). For details regarding structural outcomes following treatment delivery in both small and large animal OCD models, readers are directed to recent reviews by Jiang (Jiang et al., 2021) and Liu (Liu et al., 2022). In a narrative review, Jiang outlined that the current biomaterials and scaffolds used for the delivery of stem cells still have barriers to overcome, including the biocompatibility and degradation effects of polymer materials, as well as a need for better understanding regarding the effect of the degrading scaffold on stem cell activity and functionality in the body (Jiang et al., 2021). Though the majority of studies included in the review demonstrated improvement in structural outcomes, screening for the most regenerative subgroups of stem cells and preventing premature stem cell differentiation were also recommended (Jiang et al., 2021). Liu performed a narrative review summarizing MSC therapies for articular cartilage regeneration in large animals (Liu et al., 2022). Liu concluded that, given the biomechanical/anatomical differences and potent healing capacity of small animals, preclinical studies should utilize large animal models to adequately develop translatable therapeutics to humans, and that there was no clear consensus or standard regarding critical aspects of MSC therapy (e.g., cell concentration at implantation, need for pre-differentiation) for large animal cartilage regeneration (Liu et al., 2022).
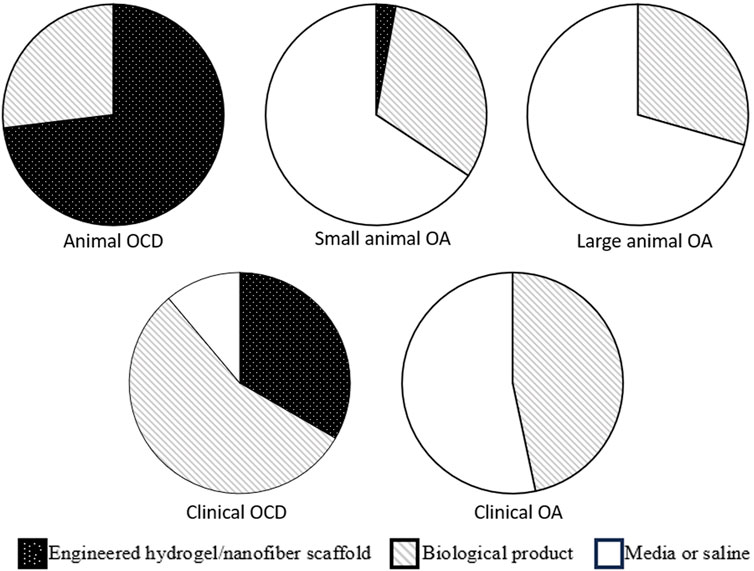
FIGURE 1. Graphical summary of scaffold types used in animal and clinical models of osteochondral defect and osteoarthritis. It can be seen that the proportion of engineered scaffolds is much larger in OCD models (animal models in particular), as compared to OA. OCD, osteochondral defect; OA, osteochondral defect.
3 Animal models of osteoarthritis
Small animal models of OA have the advantage of allowing larger sample size, less animal housing space requirement, and reduced cost versus larger animal models (Mukherjee et al., 2022). Small animal models of OA include mouse, rat, guinea pig and rabbit (Sato et al., 2012; Toghraie et al., 2012; ter Huurne et al., 2012; van Buul et al., 2014; Kim et al., 2014; Singh et al., 2014; Deng et al., 2015; Kuroda et al., 2015; Saulnier et al., 2015; Wang et al., 2015; Yang et al., 2015; Chiang et al., 2016; Hermeto et al., 2016; Latief et al., 2016; Li et al., 2016; Morille et al., 2016; Ozeki et al., 2016; Mei et al., 2017a; Mei et al., 2017b; Parrilli et al., 2017; Chang et al., 2018; Choi et al., 2018; Desando et al., 2018; Fan et al., 2018; Neybecker et al., 2018; Cheng et al., 2019; Desando et al., 2019; Mahmoud et al., 2019; McKinney et al., 2019; Vinod et al., 2019; Zhou et al., 2019; Jeon et al., 2020; Tong et al., 2020; Wang et al., 2020; Xing et al., 2020; Zhi et al., 2020; Tang et al., 2021; Xing et al., 2021; Juskovic et al., 2022; Kwapisz et al., 2022; Wang et al., 2023) (Table 2). Techniques to initiate OA include anterior cruciate transection (ACLT), ACLT with destabilization of the medial meniscus (DMM), chemically- (e.g., Mono-iodoacetate [MIA] or papain intraarticular injection) or collagenase-induced, as well as spontaneous OA (Culley et al., 2015). The end-result of these OA-initiating techniques is to recapitulate the total joint involvement of human OA (Culley et al., 2015; Cope et al., 2019). Contrary to OCD, the entire articular surface may be involved, and must be considered when initiating a treatment. In order to treat the entire articular surface, investigators have largely relied on fluid-based vehicles to deliver their regenerative biological elements, including saline/PBS, serum, and media (Toghraie et al., 2012; ter Huurne et al., 2012; van Buul et al., 2014; Singh et al., 2014; Saulnier et al., 2015; Yang et al., 2015; Latief et al., 2016; Li et al., 2016; Ozeki et al., 2016; Mei et al., 2017b; Chang et al., 2018; Choi et al., 2018; Fan et al., 2018; Neybecker et al., 2018; Cheng et al., 2019; Desando et al., 2019; Mahmoud et al., 2019; Zhou et al., 2019; Jeon et al., 2020; Tong et al., 2020; Zhi et al., 2020; Tang et al., 2021; Xing et al., 2021; Juskovic et al., 2022; Wang et al., 2023) (Table 2; Figure 1). More recently, HA has become commonly used (Sato et al., 2012; Deng et al., 2015; Kuroda et al., 2015; Wang et al., 2015; Chiang et al., 2016; Vinod et al., 2019; Wang et al., 2020; Xing et al., 2020; Kwapisz et al., 2022). As well, semi-permeable microspheres, such as Poly (D,L-lactide-co-glycolide)-poloxamer P188-poly (D,L-lactideco-glycolide) polymer microspheres (Morille et al., 2016) and sodium alginate microspheres (McKinney et al., 2019), have been used to encapsulate bone marrow-derived MSCs. The delivery of these encapsulated MSCs seeks to capitalize on their anabolic paracrine activity such as facilitation of tissue remodeling, recruitment of stem and progenitor cells and modulation of the immune response through the secretion of factors (e.g., interleukin-6 and stromal-cell-derived factor-1) (Morille et al., 2016; McKinney et al., 2019). Studies evaluating vehicles for both direct engraftment of stem cells, as well as paracrine-emphasizing, reported positive structural chondrogenic outcomes (Morille et al., 2016; McKinney et al., 2019; Vinod et al., 2019; Wang et al., 2020; Xing et al., 2020).
Large animal OA models have the advantage of more closely approximating human joints (e.g., with respect to cartilage thickness, articular biomechanics) and can more closely recapitulate OA to the human condition (Mukherjee et al., 2022). Large animal model OA studies (Al Faqeh et al., 2012; Guercio et al., 2012; Jiang et al., 2014; Song et al., 2014; Tsai et al., 2014; Ude et al., 2014; Delling et al., 2015; Desando et al., 2016; Yun et al., 2016; Barrachina et al., 2018; Feng et al., 2018; Lv et al., 2018; Srzentić Dražilov et al., 2018; Ude et al., 2018; Cabon et al., 2019; Jiang et al., 2019; Oh et al., 2021; Sanghani-Kerai et al., 2021; Armitage et al., 2022) include the use of dog, sheep, monkey and horse (Table 3). Like the small animal OA models, large animal models include surgically- (DMM, ACLT) and chemically-induced (MIA or papain intra-articular injection) methods. Similar to small animal, stem cell delivery for large animal OA studies over the last decade have largely relied on fluid-based vehicles including saline or culture media (Al Faqeh et al., 2012; Jiang et al., 2014; Song et al., 2014; Tsai et al., 2014; Ude et al., 2014; Delling et al., 2015; Barrachina et al., 2018; Srzentić Dražilov et al., 2018; Ude et al., 2018; Cabon et al., 2019; Jiang et al., 2019; Oh et al., 2021), and biological products such as HA or platelet-rich plasma (Guercio et al., 2012; Desando et al., 2016; Yun et al., 2016; Feng et al., 2018; Lv et al., 2018; Sanghani-Kerai et al., 2021; Armitage et al., 2022) (PRP, Table 3 and Figure 1). Outcomes across the majority of studies included inhibited cartilage degeneration (Morille et al., 2016; Chang et al., 2018), increased collagen II expression (Chang et al., 2018), reduced inflammatory markers (Cheng et al., 2019; Zhou et al., 2019; Wang et al., 2020) and reduced catabolic markers (Desando et al., 2016; Cheng et al., 2019). A detailed description of the outcomes reported in many of these small and large animal studies is well-summarized in by Wang (Wang et al., 2022) and Liu (Liu et al., 2022). Wang performed a systematic review in knee osteoarthritis (OA) that included 72 preclinical studies, focusing on the characteristics of animal models and cell doses, using MSCs for the treatment of knee OA (Wang et al., 2022). Wang found a correlation between the weight of the animals used and the dose of MSCs applied in the experimental treatment; however, while cartilage recovery was reported in the majority of studies, there did not seem to be a dose-response relationship across the preclinical studies included in the review (Wang et al., 2022).
It is critical to note that neither small nor large OA animal models used the more advanced tissue engineering stem cell delivery methods (Figure 1). This is likely owing to the diffuse nature of articular cartilage loss in the OA joint, making it difficult to engineer or “print” a scaffold that could be applied the non-uniform and diffusely compromised articular surface.
4 Clinical studies of focal cartilage injury and osteoarthritis
Clinical studies on focal cartilage defects (Buda et al., 2013; Saw et al., 2013; Akgun et al., 2015; Koh et al., 2016; de Windt et al., 2017; Kamei et al., 2018; Shimomura et al., 2018; de Girolamo et al., 2019; Gobbi and Whyte, 2019; Hashimoto et al., 2019; Monckeberg et al., 2019; Pipino et al., 2019; Kyriakidis et al., 2020; Neckar et al., 2022; Shetty et al., 2022; Venosa et al., 2022; Abdelhamid et al., 2023; Song et al., 2023) (Table 4) often included individuals with isolated cartilage lesions of International Cartilage Repair Society (ICRS) grades 3–4 or equivalent (severely abnormal cartilage with loss of ≥50% cartilage depth). Vehicles included liquid delivery systems such as saline (Kamei et al., 2018; Shimomura et al., 2018), HA (Buda et al., 2013; Saw et al., 2013; Hashimoto et al., 2019; Shetty et al., 2022; Song et al., 2023), and PRP (Monckeberg et al., 2019; Venosa et al., 2022). Scaffolds included HA- or collagen-based membranes (Buda et al., 2013; Akgun et al., 2015), fibrin glue (Koh et al., 2016; de Windt et al., 2017; de Girolamo et al., 2019), or tissue-engineered materials (Gobbi and Whyte, 2019; Pipino et al., 2019; Kyriakidis et al., 2020; Neckar et al., 2022; Abdelhamid et al., 2023) (Figure 1). Fluid-based vehicles were often injected under arthroscopic guidance, while scaffolds shaped to match the focal cartilage defect were often placed using mini arthrotomy (Table 4). As with the OA clinical trials, the vast majority of the studies reported that the interventions led to positive clinical outcomes. Many of these studies reported improved cartilage structural outcomes assessed by MRI. Summary of these outcomes for clinical OCD studies are presented by Jiang (Jiang et al., 2021) and Lv (Lv et al., 2023). In a narrative review, Lv concluded that while MSCs have therapeutic value, a stronger evidence base is needed until they can be clinically applied (Lv et al., 2023). It was also noted that injecting MSCs has significant limitations, including cell death at injection and significant leakage at the injection site and that overcoming these limitations via biomaterial-assisted cell cartilage repair may be the optimal direction for OA treatment (Lv et al., 2023).
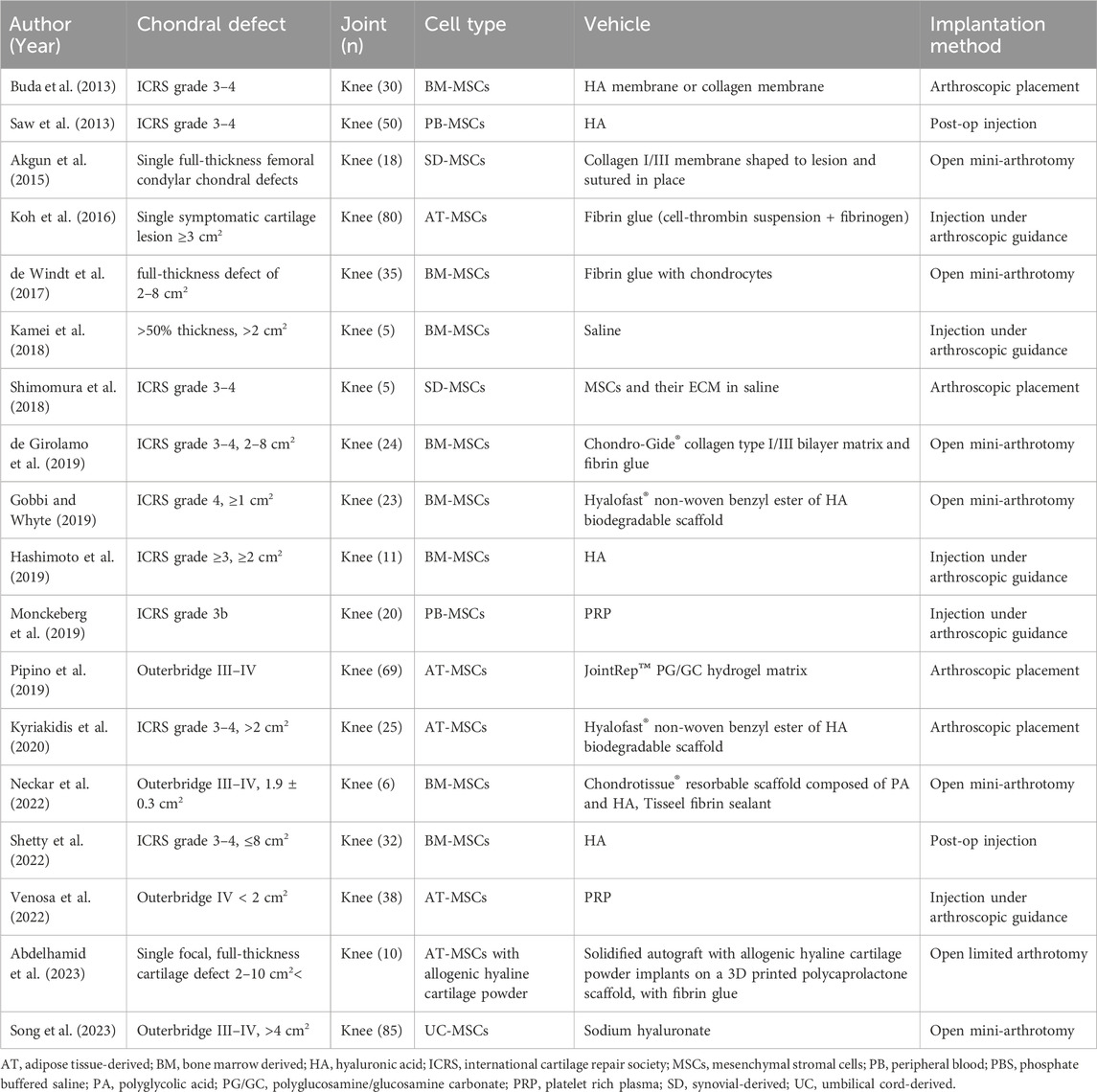
TABLE 4. Clinical studies using stem cells for isolated chondral lesions and associated injection vehicle.
Like small and large animal models of OA, randomized controlled trials evaluating stem use for the treatment of OA in humans (Vega et al., 2015; Gupta et al., 2016; Lamo-Espinosa et al., 2016; Goncars et al., 2017; Shapiro et al., 2017; Emadedin et al., 2018; Freitag et al., 2019; Lee et al., 2019; Lu et al., 2019; Matas et al., 2019; Anz et al., 2020; Bastos et al., 2020; Garza et al., 2020; Lamo-Espinosa et al., 2020; Chen et al., 2021) included a broad spectrum of OA severity (KL grades 1–4 included) and have largely made use of fluid-based vehicles such as saline (Goncars et al., 2017; Emadedin et al., 2018; Freitag et al., 2019; Lee et al., 2019; Matas et al., 2019), Ringer’s lactate (Vega et al., 2015; Garza et al., 2020), proprietary electrolyte solutions (Gupta et al., 2016; Lu et al., 2019; Chen et al., 2021), HA (Lamo-Espinosa et al., 2016), or PRP (Anz et al., 2020; Bastos et al., 2020; Lamo-Espinosa et al., 2020) (Table 5). Stem cell delivery was often performed by intra-articular injection, with or without imaging guidance. These studies generally reported no major adverse events with the treatment provided. A majority of trials reported improved clinical outcomes, though no study reported improved structural outcomes. Clinical outcomes reported by these trials were summarized by Jiang (Jiang et al., 2021) and Lv (Lv et al., 2023) in the reviews noted above.
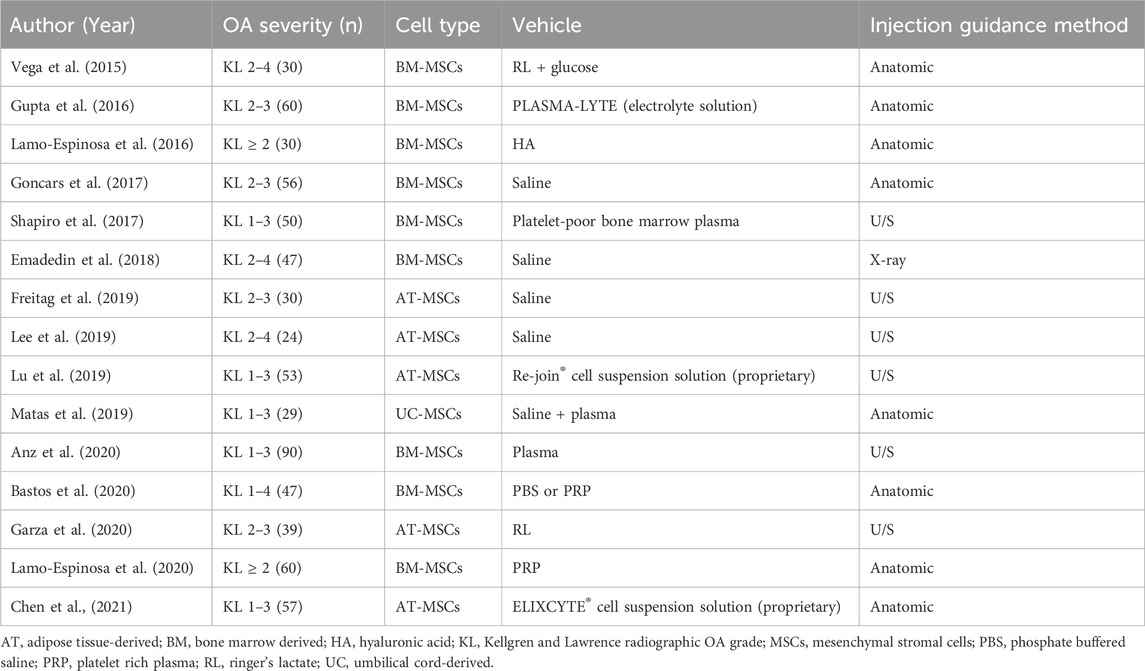
TABLE 5. Human randomized controlled trials using stem cells for knee OA and associated injection vehicle.
5 Lessons learned from the current literature
Emerging technologies, including 3D printing of biological scaffolds, allow the standardized computer-assisted creation of delivery vehicles with precise control over composition, pore size, stiffness and geometry, reviewed in detail by Kim (2019) (Kim et al., 2019) and Lafuente-Merchan (2022) (Lafuente-Merchan et al., 2022). These differing 3D environments influence migration, cell-cell integrin interactions, signaling and phenotype of the cells encapsulated within the scaffold (Kim et al., 2019; Lafuente-Merchan et al., 2022). These regenerative cells, as well as growth factors important for regenerative cell viability, proliferation and differentiation can be embedded in the scaffold, adapted specifically to the tissue requiring repair (Kim et al., 2019; Campbell, 2020). Solubility of the scaffold can be adjusted to emphasize anabolic paracrine functionality of incorporated cells as well as growth factor release rate (Murata et al., 2022). Overall, it is anticipated that these combined advantages will provide superior cartilage regeneration capacity for injured cartilage (Mandrycky et al., 2016; Kim et al., 2019; Lafuente-Merchan et al., 2022).
Ultimately, the ideal regenerative vehicle could treat all forms of cartilage injury, including OCDs and OA. The development of such vehicles was unfortunately delayed by the clinical use of stem cells for cartilage regeneration prior to establishing a strong evidence base (Marks et al., 2017; Canada, 2019; ISfSC, 2021). Formal regulation of stem cell application in the clinical setting created considerable movement forward towards this goal of applying tissue engineering technology to cartilage regeneration. Based on the available literature, advanced multi-layered scaffolds with molecular and cellular composition gradients optimized for the tissues to be repaired are applied to animal models of OCD (Wu et al., 2020; Deng et al., 2021; Murata et al., 2022). Implantation of nanopatterned differentiated MSCs as stratified bilayered hydrogel constructs improved the repair quality of cartilage defects, as indicated by histological scoring, mechanical properties, and polarized microscopy analysis in rabbit (Wu et al., 2020). A GM + SF-PTH/GM + SF-MA osteochondral biphasic scaffold promoted the regeneration of OCDs in rabbit and maintained hyaline cartilage phenotype, as evaluated by histologic staining and immunohistochemistry (Deng et al., 2021). Synovial fluid-derived MSCs contained within 3D spheroids reduced the sizes of OCDs in an equine model as seen on MRI and the histology-based ICRS scale (Murata et al., 2022). This technology has been piloted in clinical studies of OCD (Abdelhamid et al., 2023), with positive structural results (Jiang et al., 2021; Lv et al., 2023). Given the large number of individuals affected by OCD, the research advances represent an important step forward (Mandelbaum et al., 1998; Ding et al., 2007; Everhart et al., 2019; Liu et al., 2022). Presently, however applying these technologies to OA, the most common musculoskeletal disease worldwide, faces more challenges (Barr et al., 2015; Martel-Pelletier et al., 2016; Hunter and Bierma-Zeinstra, 2019; Campbell and McGonagle, 2020). One of the challenges presented by the OA joint is the heterogeneous quality of diffuse articular cartilage damage. Studies treating OCD have the advantage of “filling” a lesion of pre-defined dimensions (in the case of animal models), or that is limited in its dimensions (in the case of clinical OCDs). In OA, the articular damage is often less delineated, making it difficult to pre-fabricate a scaffold that will treat the entire articular surface. An analogy would be the repair of a pothole (OCD) versus repaving the entire road (OA). Other challenges in OA regenerative treatment includes the non-chondrogenic contributors to the OA joint (e.g., subchondral bone changes, synovial hypertrophy, altered forces across the joint due to abnormal joint alignment, etc.) that must also be considered (McGonagle et al., 2017).
6 Future directions
Scaffold engineering advances for OCD are progressing rapidly with ever-more sophisticated methods of delivery. For those with OA, current strategies to optimize the delivery vehicle have included the use of existing biological fluids such as PRP, or synthesized biological material such as HA. PRP has been cited to reduce the occurrence of inflammation, improve angiogenesis, and promote the proliferation and differentiation of chondrocytes by secreting cytokines, chemokines, and growth factors, as well as exosomes with similar effects, so as to promote the healing of bone and cartilage injuries (Liang et al., 2022). In clinical studies, patients with knee OA treated with PRP showed improvement in physical function and pain, suggesting that intra-articular injection of PRP may be a potential therapeutic strategy for relieving knee pain (Liang et al., 2022; Riewruja et al., 2022). HA is a naturally-occurring, non-toxic, biodegradable biopolymer essential for bone growth and chondrocyte differentiation, and has been reported to stimulate stem cell chondrogenesis as well as cartilage-specific ECM production by stem cells (Matsumoto et al., 2009; Yoon et al., 2011; Toh et al., 2012; Davoudi et al., 2018; Park et al., 2020). HA may also provide a protective milieu for the cells in the damaged joint surface environment and ensure cells remain localized to cartilage injury (Li et al., 2015b; Campbell, 2020). HA is used clinically to treat OA via intra-articular application (Chavda et al., 2022). For OA, vehicles such as PRP and HA may represent a compromise between form and function, with custom and commercial versions of each being developed towards regenerative optimization (Gato-Calvo et al., 2019; Chavda et al., 2022).
Ideally, tissue engineering advances for cartilage repair will 1 day be applicable to the OA joint. Possible solutions for matching OA cartilage loss may include joint surface modeling using CT or MRI that would allow the printing of a cartilage scaffold to be overlain on the damaged articular surface. Such an approach is currently being evaluated for the custom manufacturing of total knee arthroplasty components (Beckmann et al., 2020; Moret et al., 2021), but might also be applicable to cartilage regeneration. Applying a fluidic vehicle that will polymerize over the articular surface at body temperature or with another catalyzing stimulus, such as specific wavelengths of light, could secure a regenerating agent in place while permitting the supportive benefits of the scaffold. Concurrent treatment, such as joint distraction or alignment correction (McGonagle et al., 2017; Jansen and Mastbergen, 2022) may be of benefit when applying cartilage regeneration treatments to the OA joint in order to reduce the potential for damage to the treatment components during the regeneration process.
7 Conclusion
Regenerative stem cell delivery for cartilage repair has advanced considerably over the last decade with the most impressive advances incorporating both structural and biological features designed to enhance cartilage repair. At present these advances are being directed towards focal OCDs in animal models, foreshadowing competition with popular biological products such as HA and PRP. While the application of such technologies to OCD in the clinical setting is likely just around the corner, whether their application can be adapted to the more diffusely damaged articular surface of the common and debilitating OA joint is yet to be seen, and whether they prove superior to biological agents such as HA or PRP in the OA setting is yet to be demonstrated.
Author contributions
TC: Writing–original draft, Writing–review and editing. GT: Writing–review and editing.
Funding
The author(s) declare financial support was received for the research, authorship, and/or publication of this article. This work is supported by funding from the Bruyère Academic Medical Organization Incentive Fund, The Ottawa Hospital Department of Medicine Translational Research Grant and the University of Ottawa Translational Research Grant.
Acknowledgments
The authors thank Dee Cao for her assistance in summarizing a number of the studies presented in this review.
Conflict of interest
The authors declare that the research was conducted in the absence of any commercial or financial relationships that could be construed as a potential conflict of interest.
Publisher’s note
All claims expressed in this article are solely those of the authors and do not necessarily represent those of their affiliated organizations, or those of the publisher, the editors and the reviewers. Any product that may be evaluated in this article, or claim that may be made by its manufacturer, is not guaranteed or endorsed by the publisher.
References
Abdelhamid, M. M., Eid, G., Othman, M. H. M., Ibrahim, H., Elsers, D., Elyounsy, M., et al. (2023). The evaluation of cartilage regeneration efficacy of three-dimensionally biofabricated human-derived biomaterials on knee osteoarthritis: a single-arm, open label study in Egypt. J. Pers. Med. 13, 748. doi:10.3390/jpm13050748
Ahmadian, E., Eftekhari, A., Janas, D., and Vahedi, P. (2023). Nanofiber scaffolds based on extracellular matrix for articular cartilage engineering: a perspective. Nanotheranostics 7, 61–69. doi:10.7150/ntno.78611
Akgun, I., Unlu, M. C., Erdal, O. A., Ogut, T., Erturk, M., Ovali, E., et al. (2015). Matrix-induced autologous mesenchymal stem cell implantation versus matrix-induced autologous chondrocyte implantation in the treatment of chondral defects of the knee: a 2-year randomized study. Arch. Orthop. Trauma Surg. 135, 251–263. doi:10.1007/s00402-014-2136-z
Al Faqeh, H., Nor Hamdan, B. M., Chen, H. C., Aminuddin, B. S., and Ruszymah, B. H. (2012). The potential of intra-articular injection of chondrogenic-induced bone marrow stem cells to retard the progression of osteoarthritis in a sheep model. Exp. Gerontol. 47, 458–464. doi:10.1016/j.exger.2012.03.018
Anz, A. W., Hubbard, R., Rendos, N. K., Everts, P. A., Andrews, J. R., and Hackel, J. G. (2020). Bone marrow aspirate concentrate is equivalent to platelet-rich plasma for the treatment of knee osteoarthritis at 1 Year: a prospective, randomized trial. Orthop. J. Sports Med. 8, 232596711990095. doi:10.1177/2325967119900958
Armitage, A. J., Miller, J. M., Sparks, T. H., Georgiou, A. E., and Reid, J. (2022). Efficacy of autologous mesenchymal stromal cell treatment for chronic degenerative musculoskeletal conditions in dogs: a retrospective study. Front. Vet. Sci. 9, 1014687. doi:10.3389/fvets.2022.1014687
Arøen, A., Løken, S., Heir, S., Alvik, E., Ekeland, A., Granlund, O. G., et al. (2004). Articular cartilage lesions in 993 consecutive knee arthroscopies. Am. J. sports Med. 32, 211–215. doi:10.1177/0363546503259345
Barr, A. J., Campbell, T. M., Hopkinson, D., Kingsbury, S. R., Bowes, M. A., and Conaghan, P. G. (2015). A systematic review of the relationship between subchondral bone features, pain and structural pathology in peripheral joint osteoarthritis. Arthritis Res. Ther. 25, 228. doi:10.1186/s13075-015-0735-x
Barrachina, L., Remacha, A. R., Romero, A., Vitoria, A., Albareda, J., Prades, M., et al. (2018). Assessment of effectiveness and safety of repeat administration of proinflammatory primed allogeneic mesenchymal stem cells in an equine model of chemically induced osteoarthritis. BMC Vet. Res. 14, 241. doi:10.1186/s12917-018-1556-3
Bastos, R., Mathias, M., Andrade, R., Amaral, R., Schott, V., Balduino, A., et al. (2020). Intra-articular injection of culture-expanded mesenchymal stem cells with or without addition of platelet-rich plasma is effective in decreasing pain and symptoms in knee osteoarthritis: a controlled, double-blind clinical trial. Knee Surg. Sports Traumatol. Arthrosc. 28, 1989–1999. doi:10.1007/s00167-019-05732-8
Beckmann, J., Steinert, A. F., Huber, B., Rudert, M., Köck, F. X., Buhs, M., et al. (2020). Customised bi-compartmental knee arthroplasty shows encouraging 3-year results: findings of a prospective, multicenter study. Knee Surg. Sports Traumatol. Arthrosc. 28, 1742–1749. doi:10.1007/s00167-019-05595-z
Buda, R., Vannini, F., Cavallo, M., Baldassarri, M., Luciani, D., Mazzotti, A., et al. (2013). One-step arthroscopic technique for the treatment of osteochondral lesions of the knee with bone-marrow-derived cells: three years results. Musculoskelet. Surg. 97, 145–151. doi:10.1007/s12306-013-0242-7
Cabon, Q., Febre, M., Gomez, N., Cachon, T., Pillard, P., Carozzo, C., et al. (2019). Long-Term safety and efficacy of single or repeated intra-articular injection of allogeneic neonatal mesenchymal stromal cells for managing pain and lameness in moderate to severe canine osteoarthritis without anti-inflammatory pharmacological support: pilot clinical study. Front. Vet. Sci. 6, 10. doi:10.3389/fvets.2019.00010
Campbell, T. M. (2020). CORR Insights®: fibrin clots maintain the viability and proliferative capacity of human mesenchymal stem cells: an in vitro study. Clin. Orthop. Relat. Res. 478, 665–667. doi:10.1097/corr.0000000000001138
Campbell, T. M., Dilworth, F. J., Allan, D. S., and Trudel, G. (2022). The hunt is on! In pursuit of the ideal stem cell population for cartilage regeneration. Front. Bioeng. Biotechnol. 10, 866148. doi:10.3389/fbioe.2022.866148
Campbell, T. M., and McGonagle, D. (2020). Flexion contracture is a risk factor for knee osteoarthritis incidence, progression and earlier arthroplasty: data from the Osteoarthritis Initiative. Ann. Phys. Rehabil. Med. 64, 101439. doi:10.1016/j.rehab.2020.09.005
Chang, Y. H., Wu, K. C., Liu, H. W., Chu, T. Y., and Ding, D. C. (2018). Human umbilical cord-derived mesenchymal stem cells reduce monosodium iodoacetate-induced apoptosis in cartilage. Ci Ji Yi Xue Za Zhi 30, 71–80. doi:10.4103/tcmj.tcmj_23_18
Chavda, S., Rabbani, S. A., and Wadhwa, T. (2022). Role and effectiveness of intra-articular injection of hyaluronic acid in the treatment of knee osteoarthritis: a systematic review. Cureus 14, e24503. doi:10.7759/cureus.24503
Chen, C. F., Hu, C. C., Wu, C. T., Wu, H. H., Chang, C. S., Hung, Y. P., et al. (2021). Treatment of knee osteoarthritis with intra-articular injection of allogeneic adipose-derived stem cells (ADSCs) ELIXCYTE®: a phase I/II, randomized, active-control, single-blind, multiple-center clinical trial. Stem Cell Res. Ther. 12, 562. doi:10.1186/s13287-021-02631-z
Cheng, J. H., Wang, C. J., Chou, W. Y., Hsu, S. L., Chen, J. H., and Hsu, T. C. (2019). Comparison efficacy of ESWT and Wharton’s jelly mesenchymal stem cell in early osteoarthritis of rat knee. Am. J. Transl. Res. 11, 586–598.
Chiang, E. R., Ma, H. L., Wang, J. P., Liu, C. L., Chen, T. H., and Hung, S. C. (2016). Allogeneic mesenchymal stem cells in combination with hyaluronic acid for the treatment of osteoarthritis in rabbits. PLoS One 11, e0149835. doi:10.1371/journal.pone.0149835
Cho, H., Kim, J., Kim, S., Jung, Y. C., Wang, Y., Kang, B. J., et al. (2020). Dual delivery of stem cells and insulin-like growth factor-1 in coacervate-embedded composite hydrogels for enhanced cartilage regeneration in osteochondral defects. J. Control Release 327, 284–295. doi:10.1016/j.jconrel.2020.08.002
Choi, S., Kim, J. H., Ha, J., Jeong, B. I., Jung, Y. C., Lee, G. S., et al. (2018). Intra-articular injection of alginate-microencapsulated adipose tissue-derived mesenchymal stem cells for the treatment of osteoarthritis in rabbits. Stem Cells Int. 2018, 1–10. doi:10.1155/2018/2791632
Cicuttini, F., Ding, C., Wluka, A., Davis, S., Ebeling, P. R., and Jones, G. (2005). Association of cartilage defects with loss of knee cartilage in healthy, middle-age adults: a prospective study. Arthritis Rheum. 52, 2033–2039. doi:10.1002/art.21148
Coburn, J. M., Gibson, M., Monagle, S., Patterson, Z., and Elisseeff, J. H. (2012). Bioinspired nanofibers support chondrogenesis for articular cartilage repair. Proc. Natl. Acad. Sci. U. S. A. 109, 10012–10017. doi:10.1073/pnas.1121605109
Cope, P. J., Ourradi, K., Li, Y., and Sharif, M. (2019). Models of osteoarthritis: the good, the bad and the promising. Osteoarthr. Cartil. 27, 230–239. doi:10.1016/j.joca.2018.09.016
Culley, K. L., Dragomir, C. L., Chang, J., Wondimu, E. B., Coico, A., Plumb, D. A., et al. (2015). “Mouse models of osteoarthritis: surgical model of posttraumatic osteoarthritis induced by destabilization of the medial meniscus,” in Osteoporosis and osteoarthritis, methods in molecular biology. Editors J. J. Westendorf, and A. J. van Wijnen (New York: Springer Science+Business Media).
Cunha, J. E., Barbosa, G. M., Castro, P., Luiz, B. L. F., Silva, A. C. A., Russo, T. L., et al. (2019). Knee osteoarthritis induces atrophy and neuromuscular junction remodeling in the quadriceps and tibialis anterior muscles of rats. Sci. Rep. 9, 6366. doi:10.1038/s41598-019-42546-7
Dashtdar, H., Murali, M. R., Abbas, A. A., Suhaeb, A. M., Selvaratnam, L., Tay, L. X., et al. (2015). PVA-chitosan composite hydrogel versus alginate beads as a potential mesenchymal stem cell carrier for the treatment of focal cartilage defects. Knee Surg. Sports Traumatol. Arthrosc. 23, 1368–1377. doi:10.1007/s00167-013-2723-5
Davoudi, S., Chin, C. Y., Cooke, M. J., Tam, R. Y., Shoichet, M. S., and Gilbert, P. M. (2018). Muscle stem cell intramuscular delivery within hyaluronan methylcellulose improves engraftment efficiency and dispersion. Biomaterials 173, 34–46. doi:10.1016/j.biomaterials.2018.04.048
de Girolamo, L., Schönhuber, H., Viganò, M., Bait, C., Quaglia, A., Thiebat, G., et al. (2019). Autologous matrix-induced chondrogenesis (AMIC) and AMIC enhanced by autologous concentrated bone marrow aspirate (BMAC) allow for stable clinical and functional improvements at up to 9 Years follow-up: results from a randomized controlled study. J. Clin. Med. 8, 392. doi:10.3390/jcm8030392
Delling, U., Brehm, W., Metzger, M., Ludewig, E., Winter, K., and Jülke, H. (2015). In vivo tracking and fate of intra-articularly injected superparamagnetic iron oxide particle-labeled multipotent stromal cells in an ovine model of osteoarthritis. Cell Transpl. 24, 2379–2390. doi:10.3727/096368914x685654
Deng, C., Yang, J., He, H., Ma, Z., Wang, W., Zhang, Y., et al. (2021). 3D bio-printed biphasic scaffolds with dual modification of silk fibroin for the integrated repair of osteochondral defects. Biomater. Sci. 9, 4891–4903. doi:10.1039/d1bm00535a
Deng, M. W., Wei, S. J., Yew, T. L., Lee, P. H., Yang, T. Y., Chu, H. Y., et al. (2015). Cell therapy with G-CSF-mobilized stem cells in a rat osteoarthritis model. Cell Transpl. 24, 1085–1096. doi:10.3727/096368914x680091
Desando, G., Bartolotti, I., Cavallo, C., Schiavinato, A., Secchieri, C., Kon, E., et al. (2018). Short-Term homing of hyaluronan-primed cells: therapeutic implications for osteoarthritis treatment. Tissue Eng. Part C Methods 24, 121–133. doi:10.1089/ten.tec.2017.0336
Desando, G., Bartolotti, I., Martini, L., Giavaresi, G., Nicoli Aldini, N., Fini, M., et al. (2019). Regenerative features of adipose tissue for osteoarthritis treatment in a rabbit model: enzymatic digestion versus mechanical disruption. Int. J. Mol. Sci. 20, 2636. doi:10.3390/ijms20112636
Desando, G., Giavaresi, G., Cavallo, C., Bartolotti, I., Sartoni, F., Nicoli Aldini, N., et al. (2016). Autologous bone marrow concentrate in a sheep model of osteoarthritis: new perspectives for cartilage and meniscus repair. Tissue Eng. Part C Methods 22, 608–619. doi:10.1089/ten.tec.2016.0033
de Windt, T. S., Vonk, L. A., Slaper-Cortenbach, I. C. M., Nizak, R., van Rijen, M. H. P., and Saris, D. B. F. (2017). Allogeneic MSCs and recycled autologous chondrons mixed in a one-stage cartilage cell transplantion: a first-in-man trial in 35 patients. Stem Cells 35, 1984–1993. doi:10.1002/stem.2657
Ding, C., Cicuttini, F., and Jones, G. (2007). Tibial subchondral bone size and knee cartilage defects: relevance to knee osteoarthritis. Osteoarthr. Cartil. 15, 479–486. doi:10.1016/j.joca.2007.01.003
Emadedin, M., Labibzadeh, N., Liastani, M. G., Karimi, A., Jaroughi, N., Bolurieh, T., et al. (2018). Intra-articular implantation of autologous bone marrow-derived mesenchymal stromal cells to treat knee osteoarthritis: a randomized, triple-blind, placebo-controlled phase 1/2 clinical trial. Cytotherapy 20, 1238–1246. doi:10.1016/j.jcyt.2018.08.005
Everhart, J. S., Abouljoud, M. M., Kirven, J. C., and Flanigan, D. C. (2019). Full-thickness cartilage defects are important independent predictive factors for progression to total knee arthroplasty in older adults with minimal to moderate osteoarthritis: data from the osteoarthritis initiative. J. Bone Jt. Surg. Am. 101, 56–63. doi:10.2106/jbjs.17.01657
Fan, M. P., Si, M., Li, B. J., Hu, G. H., Hou, Y., Yang, W., et al. (2018). Cell therapy of a knee osteoarthritis rat model using precartilaginous stem cells. Eur. Rev. Med. Pharmacol. Sci. 22, 2119–2125. doi:10.26355/eurrev_201804_14745
Feng, C., Luo, X., He, N., Xia, H., Lv, X., Zhang, X., et al. (2018). Efficacy and persistence of allogeneic adipose-derived mesenchymal stem cells combined with hyaluronic acid in osteoarthritis after intra-articular injection in a sheep model. Tissue Eng. Part A 24, 219–233. doi:10.1089/ten.tea.2017.0039
Finley, C. R., Chan, D. S., Garrison, S., Korownyk, C., Kolber, M. R., Campbell, S., et al. (2018). What are the most common conditions in primary care? Systematic review. Can. Fam. Physician 64, 832–840.
Freitag, J., Bates, D., Wickham, J., Shah, K., Huguenin, L., Tenen, A., et al. (2019). Adipose-derived mesenchymal stem cell therapy in the treatment of knee osteoarthritis: a randomized controlled trial. Regen. Med. 14, 213–230. doi:10.2217/rme-2018-0161
Garza, J. R., Campbell, R. E., Tjoumakaris, F. P., Freedman, K. B., Miller, L. S., Santa Maria, D., et al. (2020). Clinical efficacy of intra-articular mesenchymal stromal cells for the treatment of knee osteoarthritis: a double-blinded prospective randomized controlled clinical trial. Am. J. Sports Med. 48, 588–598. doi:10.1177/0363546519899923
Gato-Calvo, L., Magalhaes, J., Ruiz-Romero, C., Blanco, F. J., and Burguera, E. F. (2019). Platelet-rich plasma in osteoarthritis treatment: review of current evidence. Ther. Adv. Chronic Dis. 10, 204062231982556. doi:10.1177/2040622319825567
Gobbi, A., and Whyte, G. P. (2019). Long-term clinical outcomes of one-stage cartilage repair in the knee with hyaluronic acid-based scaffold embedded with mesenchymal stem cells sourced from bone marrow aspirate concentrate. Am. J. Sports Med. 47, 1621–1628. doi:10.1177/0363546519845362
Goncars, V., Jakobsons, E., Blums, K., Briede, I., Patetko, L., Erglis, K., et al. (2017). The comparison of knee osteoarthritis treatment with single-dose bone marrow-derived mononuclear cells vs. hyaluronic acid injections. Med. Kaunas. 53, 101–108. doi:10.1016/j.medici.2017.02.002
Goodrich, L. R., Chen, A. C., Werpy, N. M., Williams, A. A., Kisiday, J. D., Su, A. W., et al. (2016). Addition of mesenchymal stem cells to autologous platelet-enhanced fibrin scaffolds in chondral defects: does it enhance repair? J. Bone Jt. Surg. Am. 98, 23–34. doi:10.2106/jbjs.o.00407
Guercio, A., Di Marco, P., Casella, S., Cannella, V., Russotto, L., Purpari, G., et al. (2012). Production of canine mesenchymal stem cells from adipose tissue and their application in dogs with chronic osteoarthritis of the humeroradial joints. Cell Biol. Int. 36, 189–194. doi:10.1042/cbi20110304
Guermazi, A., Hayashi, D., Roemer, F. W., Niu, J., Quinn, E. K., Crema, M. D., et al. (2017). Brief report: partial- and full-thickness focal cartilage defects contribute equally to development of new cartilage damage in knee osteoarthritis: the multicenter osteoarthritis study. Arthritis Rheumatol. 69, 560–564. doi:10.1002/art.39970
Gupta, P. K., Chullikana, A., Rengasamy, M., Shetty, N., Pandey, V., Agarwal, V., et al. (2016). Efficacy and safety of adult human bone marrow-derived, cultured, pooled, allogeneic mesenchymal stromal cells (Stempeucel®): preclinical and clinical trial in osteoarthritis of the knee joint. Arthritis Res. Ther. 18, 301. doi:10.1186/s13075-016-1195-7
Ha, C. W., Park, Y. B., Chung, J. Y., and Park, Y. G. (2015). Cartilage repair using composites of human umbilical cord blood-derived mesenchymal stem cells and hyaluronic acid hydrogel in a minipig model. Stem Cells Transl. Med. 4, 1044–1051. doi:10.5966/sctm.2014-0264
Hashimoto, Y., Nishida, Y., Takahashi, S., Nakamura, H., Mera, H., Kashiwa, K., et al. (2019). Transplantation of autologous bone marrow-derived mesenchymal stem cells under arthroscopic surgery with microfracture versus microfracture alone for articular cartilage lesions in the knee: a multicenter prospective randomized control clinical trial. Regen. Ther. 11, 106–113. doi:10.1016/j.reth.2019.06.002
Heirani-Tabasi, A., Hosseinzadeh, S., Rabbani, S., Ahmadi Tafti, S. H., Jamshidi, K., Soufizomorrod, M., et al. (2021). Cartilage tissue engineering by co-transplantation of chondrocyte extracellular vesicles and mesenchymal stem cells, entrapped in chitosan-hyaluronic acid hydrogel. Biomed. Mater 16, 055003. doi:10.1088/1748-605x/ac0cbf
Hermeto, L. C., DeRossi, R., Oliveira, R. J., Pesarini, J. R., Antoniolli-Silva, A. C., Jardim, P. H., et al. (2016). Effects of intra-articular injection of mesenchymal stem cells associated with platelet-rich plasma in a rabbit model of osteoarthritis. Genet. Mol. Res. 15. doi:10.4238/gmr.15038569
Hernigou, J., Verdonk, P., Homma, Y., Verdonk, R., Goodman, S. B., and Hernigou, P. (2022). Nonoperative and operative bone and cartilage regeneration and orthopaedic biologics of the hip: an orthoregeneration network (ON) foundation hip review. Arthrosc. J. Arthrosc. Relat. Surg. official Publ. Arthrosc. Assoc. N. Am. Int. Arthrosc. Assoc. 38, 643–656. doi:10.1016/j.arthro.2021.08.032
Hunter, D. J., and Bierma-Zeinstra, S. (2019). Osteoarthritis. Lancet (London, Engl. 393, 1745–1759. doi:10.1016/s0140-6736(19)30417-9
Jansen, M. P., and Mastbergen, S. C. (2022). Joint distraction for osteoarthritis: clinical evidence and molecular mechanisms. Nat. Rev. Rheumatol. 18, 35–46. doi:10.1038/s41584-021-00695-y
Jeon, H. J., Yoon, K. A., An, E. S., Kang, T. W., Sim, Y. B., Ahn, J., et al. (2020). Therapeutic effects of human umbilical cord blood-derived mesenchymal stem cells combined with cartilage acellular matrix mediated via bone morphogenic protein 6 in a rabbit model of articular cruciate ligament transection. Stem Cell Rev. Rep. 16, 596–611. doi:10.1007/s12015-020-09958-9
Jia, Z., Zhu, F., Li, X., Liang, Q., Zhuo, Z., Huang, J., et al. (2019). Repair of osteochondral defects using injectable chitosan-based hydrogel encapsulated synovial fluid-derived mesenchymal stem cells in a rabbit model. Mater Sci. Eng. C Mater Biol. Appl. 99, 541–551. doi:10.1016/j.msec.2019.01.115
Jiang, B., Fu, X., Yan, L., Li, S., Zhao, D., Wang, X., et al. (2019). Transplantation of human ESC-derived mesenchymal stem cell spheroids ameliorates spontaneous osteoarthritis in rhesus macaques. Theranostics 9, 6587–6600. doi:10.7150/thno.35391
Jiang, L., Ma, A., Song, L., Hu, Y., Dun, H., Daloze, P., et al. (2014). Cartilage regeneration by selected chondrogenic clonal mesenchymal stem cells in the collagenase-induced monkey osteoarthritis model. J. Tissue Eng. Regen. Med. 8, 896–905. doi:10.1002/term.1676
Jiang, S., Tian, G., Li, X., Yang, Z., Wang, F., Tian, Z., et al. (2021). Research progress on stem cell therapies for articular cartilage regeneration. Stem Cells Int. 2021, 1–25. doi:10.1155/2021/8882505
Jin, L., Zhao, W., Ren, B., Li, L., Hu, X., Zhang, X., et al. (2019). Osteochondral tissue regenerated via a strategy by stacking pre-differentiated BMSC sheet on fibrous mesh in a gradient. Biomed. Mater 14, 065017. doi:10.1088/1748-605x/ab49e2
Juskovic, A., Nikolic, M., Ljujic, B., Matic, A., Zivkovic, V., Vucicevic, K., et al. (2022). Effects of combined allogenic adipose stem cells and hyperbaric oxygenation treatment on pathogenesis of osteoarthritis in knee joint induced by monoiodoacetate. Int. J. Mol. Sci. 23, 7695. doi:10.3390/ijms23147695
Kamei, N., Ochi, M., Adachi, N., Ishikawa, M., Yanada, S., Levin, L. S., et al. (2018). The safety and efficacy of magnetic targeting using autologous mesenchymal stem cells for cartilage repair. Knee Surg. Sports Traumatol. Arthrosc. 26, 3626–3635. doi:10.1007/s00167-018-4898-2
Kazemnejad, S., Khanmohammadi, M., Baheiraei, N., and Arasteh, S. (2017). Current state of cartilage tissue engineering using nanofibrous scaffolds and stem cells. Avicenna J. Med. Biotechnol. 9, 50–65.
Kim, H., Bae, C., Kook, Y. M., Koh, W. G., Lee, K., and Park, M. H. (2019). Mesenchymal stem cell 3D encapsulation technologies for biomimetic microenvironment in tissue regeneration. Stem Cell Res. Ther. 10, 51. doi:10.1186/s13287-018-1130-8
Kim, J. E., Lee, S. M., Kim, S. H., Tatman, P., Gee, A. O., Kim, D. H., et al. (2014). Effect of self-assembled peptide-mesenchymal stem cell complex on the progression of osteoarthritis in a rat model. Int. J. Nanomedicine 9 141–157. Suppl. 1. doi:10.2147/ijn.s54114
Kim, Y. G., Choi, J., and Kim, K. (2020). Mesenchymal stem cell-derived exosomes for effective cartilage tissue repair and treatment of osteoarthritis. Biotechnol. J. 15, e2000082. doi:10.1002/biot.202000082
Koh, Y. G., Kwon, O. R., Kim, Y. S., Choi, Y. J., and Tak, D. H. (2016). Adipose-derived mesenchymal stem cells with microfracture versus microfracture alone: 2-year follow-up of a prospective randomized trial. Arthrosc. J. Arthrosc. Relat. Surg. official Publ. Arthrosc. Assoc. N. Am. Int. Arthrosc. Assoc. 32, 97–109. doi:10.1016/j.arthro.2015.09.010
Kondo, S., Nakagawa, Y., Mizuno, M., Katagiri, K., Tsuji, K., Kiuchi, S., et al. (2019). Transplantation of aggregates of autologous synovial mesenchymal stem cells for treatment of cartilage defects in the femoral condyle and the femoral groove in microminipigs. Am. J. Sports Med. 47, 2338–2347. doi:10.1177/0363546519859855
Kuroda, K., Kabata, T., Hayashi, K., Maeda, T., Kajino, Y., Iwai, S., et al. (2015). The paracrine effect of adipose-derived stem cells inhibits osteoarthritis progression. BMC Musculoskelet. Disord. 16, 236. doi:10.1186/s12891-015-0701-4
Kwapisz, A., Bowman, M., Walters, J., Cosh, H., Burnikel, B., Tokish, J., et al. (2022). Human adipose- and amnion-derived mesenchymal stromal cells similarly mitigate osteoarthritis progression in the dunkin hartley Guinea pig. Am. J. Sports Med. 50, 3963–3973. doi:10.1177/03635465221126683
Kyriakidis, T., Iosifidis, M., Michalopoulos, E., Melas, I., Stavropoulos-Giokas, C., and Verdonk, R. (2020). Good mid-term outcomes after adipose-derived culture-expanded mesenchymal stem cells implantation in knee focal cartilage defects. Knee Surg. Sports Traumatol. Arthrosc. 28, 502–508. doi:10.1007/s00167-019-05688-9
Lafuente-Merchan, M., Ruiz-Alonso, S., García-Villén, F., Gallego, I., Gálvez-Martín, P., Saenz-Del-Burgo, L., et al. (2022). Progress in 3D bioprinting technology for osteochondral regeneration. Pharmaceutics 14, 1578. doi:10.3390/pharmaceutics14081578
Lam, J., Lu, S., Lee, E. J., Trachtenberg, J. E., Meretoja, V. V., Dahlin, R. L., et al. (2014). Osteochondral defect repair using bilayered hydrogels encapsulating both chondrogenically and osteogenically pre-differentiated mesenchymal stem cells in a rabbit model. Osteoarthr. Cartil. 22, 1291–1300. doi:10.1016/j.joca.2014.06.035
Lamo-Espinosa, J. M., Blanco, J. F., Sánchez, M., Moreno, V., Granero-Moltó, F., Sánchez-Guijo, F., et al. (2020). Phase II multicenter randomized controlled clinical trial on the efficacy of intra-articular injection of autologous bone marrow mesenchymal stem cells with platelet rich plasma for the treatment of knee osteoarthritis. J. Transl. Med. 18, 356. doi:10.1186/s12967-020-02530-6
Lamo-Espinosa, J. M., Mora, G., Blanco, J. F., Granero-Molto, F., Nunez-Cordoba, J. M., Sanchez-Echenique, C., et al. (2016). Intra-articular injection of two different doses of autologous bone marrow mesenchymal stem cells versus hyaluronic acid in the treatment of knee osteoarthritis: multicenter randomized controlled clinical trial (phase I/II). J. Transl. Med. 14, 246. doi:10.1186/s12967-016-0998-2
Latief, N., Raza, F. A., Bhatti, F. U., Tarar, M. N., Khan, S. N., and Riazuddin, S. (2016). Adipose stem cells differentiated chondrocytes regenerate damaged cartilage in rat model of osteoarthritis. Cell Biol. Int. 40, 579–588. doi:10.1002/cbin.10596
Lee, W. S., Kim, H. J., Kim, K. I., Kim, G. B., and Jin, W. (2019). Intra-articular injection of autologous adipose tissue-derived mesenchymal stem cells for the treatment of knee osteoarthritis: a phase IIb, randomized, placebo-controlled clinical trial. Stem Cells Transl. Med. 8, 504–511. doi:10.1002/sctm.18-0122
Li, J., Chen, G., Xu, X., Abdou, P., Jiang, Q., Shi, D., et al. (2019). Advances of injectable hydrogel-based scaffolds for cartilage regeneration. Regen. Biomater. 6, 129–140. doi:10.1093/rb/rbz022
Li, J., Huang, Y., Song, J., Li, X., Zhang, X., Zhou, Z., et al. (2018). Cartilage regeneration using arthroscopic flushing fluid-derived mesenchymal stem cells encapsulated in a one-step rapid cross-linked hydrogel. Acta Biomater. 79, 202–215. doi:10.1016/j.actbio.2018.08.029
Li, M., Luo, X., Lv, X., Liu, V., Zhao, G., Zhang, X., et al. (2016). In vivo human adipose-derived mesenchymal stem cell tracking after intra-articular delivery in a rat osteoarthritis model. Stem Cell Res. Ther. 7, 160. doi:10.1186/s13287-016-0420-2
Li, X., Li, Y., Zuo, Y., Qu, D., Liu, Y., Chen, T., et al. (2015a). Osteogenesis and chondrogenesis of biomimetic integrated porous PVA/gel/V-n-HA/pa6 scaffolds and BMSCs construct in repair of articular osteochondral defect. J. Biomed. Mater Res. A 103, 3226–3236. doi:10.1002/jbm.a.35452
Li, Y., Meng, H., Liu, Y., and Lee, B. P. (2015b). Fibrin gel as an injectable biodegradable scaffold and cell carrier for tissue engineering. ScientificWorldJournal 2015, 1–10. doi:10.1155/2015/685690
Liang, Y., Li, J., Wang, Y., He, J., Chen, L., Chu, J., et al. (2022). Platelet rich plasma in the repair of articular cartilage injury: a narrative review. Cartilage 13, 194760352211184. doi:10.1177/19476035221118419
Liu, T. P., Ha, P., Xiao, C. Y., Kim, S. Y., Jensen, A. R., Easley, J., et al. (2022). Updates on mesenchymal stem cell therapies for articular cartilage regeneration in large animal models. Front. Cell Dev. Biol. 10, 982199. doi:10.3389/fcell.2022.982199
Lu, J., Shen, X., Sun, X., Yin, H., Yang, S., Lu, C., et al. (2018). Increased recruitment of endogenous stem cells and chondrogenic differentiation by a composite scaffold containing bone marrow homing peptide for cartilage regeneration. Theranostics 8, 5039–5058. doi:10.7150/thno.26981
Lu, L., Dai, C., Zhang, Z., Du, H., Li, S., Ye, P., et al. (2019). Treatment of knee osteoarthritis with intra-articular injection of autologous adipose-derived mesenchymal progenitor cells: a prospective, randomized, double-blind, active-controlled, phase IIb clinical trial. Stem Cell Res. Ther. 10, 143. doi:10.1186/s13287-019-1248-3
Lv, X., He, J., Zhang, X., Luo, X., He, N., Sun, Z., et al. (2018). Comparative efficacy of autologous stromal vascular fraction and autologous adipose-derived mesenchymal stem cells combined with hyaluronic acid for the treatment of sheep osteoarthritis. Cell Transpl. 27, 1111–1125. doi:10.1177/0963689718773333
Lv, Z., Cai, X., Bian, Y., Wei, Z., Zhu, W., Zhao, X., et al. (2023). Advances in mesenchymal stem cell therapy for osteoarthritis: from preclinical and clinical perspectives. Bioeng. (Basel) 10, 195. doi:10.3390/bioengineering10020195
Mahmoud, E. E., Adachi, N., Mawas, A. S., Deie, M., and Ochi, M. (2019). Multiple intra-articular injections of allogeneic bone marrow-derived stem cells potentially improve knee lesions resulting from surgically induced osteoarthritis: an animal study. Bone Jt. J. 101-B, 824–831. doi:10.1302/0301-620x.101b7.bjj-2018-1532.r1
Mandelbaum, B. R., Browne, J. E., Fu, F., Micheli, L., Mosely, J. B., Erggelet, C., et al. (1998). Articular cartilage lesions of the knee. Am. J. sports Med. 26, 853–861. doi:10.1177/03635465980260062201
Mandrycky, C., Wang, Z., Kim, K., and Kim, D. H. (2016). 3D bioprinting for engineering complex tissues. Biotechnol. Adv. 34, 422–434. doi:10.1016/j.biotechadv.2015.12.011
Marks, P. W., Witten, C. M., and Califf, R. M. (2017). Clarifying stem-cell therapy’s benefits and risks. N. Engl. J. Med. 376, 1007–1009. doi:10.1056/nejmp1613723
Martel-Pelletier, J., Barr, A. J., Cicuttini, F. M., Conaghan, P. G., Cooper, C., Goldring, M. B., et al. (2016). Osteoarthritis. Nat. Rev. Dis. Prim. 2, 16072. doi:10.1038/nrdp.2016.72
Matas, J., Orrego, M., Amenabar, D., Infante, C., Tapia-Limonchi, R., Cadiz, M. I., et al. (2019). Umbilical cord-derived mesenchymal stromal cells (MSCs) for knee osteoarthritis: repeated MSC dosing is superior to a single MSC dose and to hyaluronic acid in a controlled randomized phase I/II trial. Stem Cells Transl. Med. 8, 215–224. doi:10.1002/sctm.18-0053
Matsumoto, K., Li, Y., Jakuba, C., Sugiyama, Y., Sayo, T., Okuno, M., et al. (2009). Conditional inactivation of Has2 reveals a crucial role for hyaluronan in skeletal growth, patterning, chondrocyte maturation and joint formation in the developing limb. Development 136, 2825–2835. doi:10.1242/dev.038505
McGonagle, D., Baboolal, T. G., and Jones, E. (2017). Native joint-resident mesenchymal stem cells for cartilage repair in osteoarthritis. Nat. Rev. Rheumatol. 13, 719–730. doi:10.1038/nrrheum.2017.182
McKinney, J. M., Doan, T. N., Wang, L., Deppen, J., Reece, D. S., Pucha, K. A., et al. (2019). Therapeutic efficacy of intra-articular delivery of encapsulated human mesenchymal stem cells on early stage osteoarthritis. Eur. Cell Mater 37, 42–59. doi:10.22203/ecm.v037a04
Mei, L., Shen, B., Ling, P., Liu, S., Xue, J., Liu, F., et al. (2017b). Culture-expanded allogenic adipose tissue-derived stem cells attenuate cartilage degeneration in an experimental rat osteoarthritis model. PLoS One 12, e0176107. doi:10.1371/journal.pone.0176107
Mei, L., Shen, B., Xue, J., Liu, S., Ma, A., Liu, F., et al. (2017a). Adipose tissue-derived stem cells in combination with xanthan gum attenuate osteoarthritis progression in an experimental rat model. Biochem. Biophys. Res. Commun. 494, 285–291. doi:10.1016/j.bbrc.2017.10.039
Monckeberg, J. E., Rafols, C., Apablaza, F., Gerhard, P., and Rosales, J. (2019). Intra-articular administration of peripheral blood stem cells with platelet-rich plasma regenerated articular cartilage and improved clinical outcomes for knee chondral lesions. Knee 26, 824–831. doi:10.1016/j.knee.2019.05.008
Moret, C. S., Hirschmann, M. T., Vogel, N., and Arnold, M. P. (2021). Customised, individually made total knee arthroplasty shows promising 1-year clinical and patient reported outcomes. Arch. Orthop. Trauma Surg. 141, 2217–2225. doi:10.1007/s00402-021-04045-1
Morille, M., Toupet, K., Montero-Menei, C. N., Jorgensen, C., and Noël, D. (2016). PLGA-based microcarriers induce mesenchymal stem cell chondrogenesis and stimulate cartilage repair in osteoarthritis. Biomaterials 88, 60–69. doi:10.1016/j.biomaterials.2016.02.022
Mukherjee, P., Roy, S., Ghosh, D., and Nandi, S. K. (2022). Role of animal models in biomedical research: a review. Lab. Anim. Res. 38, 18. doi:10.1186/s42826-022-00128-1
Murata, D., Ishikawa, S., Sunaga, T., Saito, Y., Sogawa, T., Nakayama, K., et al. (2022). Osteochondral regeneration of the femoral medial condyle by using a scaffold-free 3D construct of synovial membrane-derived mesenchymal stem cells in horses. BMC Vet. Res. 18, 53. doi:10.1186/s12917-021-03126-y
Muttigi, M. S., Kim, B. J., Choi, B., Yoshie, A., Kumar, H., Han, I., et al. (2018). Matrilin-3 codelivery with adipose-derived mesenchymal stem cells promotes articular cartilage regeneration in a rat osteochondral defect model. J. Tissue Eng. Regen. Med. 12, 667–675. doi:10.1002/term.2485
Neckar, P., Potockova, H., Branis, J., Havlas, V., Novotny, T., Lykova, D., et al. (2022). Treatment of knee cartilage by cultured stem cells and three dimensional scaffold: a phase I/IIa clinical trial. Int. Orthop. 47, 2375–2382. doi:10.1007/s00264-022-05505-y
Neybecker, P., Henrionnet, C., Pape, E., Mainard, D., Galois, L., Loeuille, D., et al. (2018). In vitro and in vivo potentialities for cartilage repair from human advanced knee osteoarthritis synovial fluid-derived mesenchymal stem cells. Stem Cell Res. Ther. 9, 329. doi:10.1186/s13287-018-1071-2
Oh, J., Son, Y. S., Kim, W. H., Kwon, O. K., and Kang, B. J. (2021). Mesenchymal stem cells genetically engineered to express platelet-derived growth factor and heme oxygenase-1 ameliorate osteoarthritis in a canine model. J. Orthop. Surg. Res. 16, 43. doi:10.1186/s13018-020-02178-4
Ozeki, N., Muneta, T., Koga, H., Nakagawa, Y., Mizuno, M., Tsuji, K., et al. (2016). Not single but periodic injections of synovial mesenchymal stem cells maintain viable cells in knees and inhibit osteoarthritis progression in rats. Osteoarthr. Cartil. 24, 1061–1070. doi:10.1016/j.joca.2015.12.018
Park, S. H., Park, J. Y., Ji, Y. B., Ju, H. J., Min, B. H., and Kim, M. S. (2020). An injectable click-crosslinked hyaluronic acid hydrogel modified with a BMP-2 mimetic peptide as a bone tissue engineering scaffold. Acta Biomater. 117, 108–120. doi:10.1016/j.actbio.2020.09.013
Park, Y. B., Ha, C. W., Kim, J. A., Han, W. J., Rhim, J. H., Lee, H. J., et al. (2017). Single-stage cell-based cartilage repair in a rabbit model: cell tracking and in vivo chondrogenesis of human umbilical cord blood-derived mesenchymal stem cells and hyaluronic acid hydrogel composite. Osteoarthr. Cartil. 25, 570–580. doi:10.1016/j.joca.2016.10.012
Park, Y. B., Ha, C. W., Kim, J. A., Kim, S., and Park, Y. G. (2019). Comparison of undifferentiated versus chondrogenic predifferentiated mesenchymal stem cells derived from human umbilical cord blood for cartilage repair in a rat model. Am. J. Sports Med. 47, 451–461. doi:10.1177/0363546518815151
Parrilli, A., Giavaresi, G., Ferrari, A., Salamanna, F., Desando, G., Grigolo, B., et al. (2017). Subchondral bone response to injected adipose-derived stromal cells for treating osteoarthritis using an experimental rabbit model. Biotech. Histochem 92, 201–211. doi:10.1080/10520295.2017.1292366
Pascual-Garrido, C., Aisenbrey, E. A., Rodriguez-Fontan, F., Payne, K. A., Bryant, S. J., and Goodrich, L. R. (2019). Photopolymerizable injectable cartilage mimetic hydrogel for the treatment of focal chondral lesions: a proof of concept study in a rabbit animal model. Am. J. Sports Med. 47, 212–221. doi:10.1177/0363546518808012
Pipino, G., Risitano, S., Alviano, F., Wu, E. J., Bonsi, L., Vaccarisi, D. C., et al. (2019). Microfractures and hydrogel scaffolds in the treatment of osteochondral knee defects: a clinical and histological evaluation. J. Clin. Orthop. Trauma 10, 67–75. doi:10.1016/j.jcot.2018.03.001
Riewruja, K., Phakham, S., Sompolpong, P., Reantragoon, R., Tanavalee, A., Ngarmukos, S., et al. (2022). Cytokine profiling and intra-articular injection of autologous platelet-rich plasma in knee osteoarthritis. Int. J. Mol. Sci. 23, 890. doi:10.3390/ijms23020890
Ruan, S. Q., Yan, L., Deng, J., Huang, W. L., and Jiang, D. M. (2017). Preparation of a biphase composite scaffold and its application in tissue engineering for femoral osteochondral defects in rabbits. Int. Orthop. 41, 1899–1908. doi:10.1007/s00264-017-3522-2
Sanghani-Kerai, A., Black, C., Cheng, S. O., Collins, L., Schneider, N., Blunn, G., et al. (2021). Clinical outcomes following intra-articular injection of autologous adipose-derived mesenchymal stem cells for the treatment of osteoarthritis in dogs characterized by weight-bearing asymmetry. Bone Jt. Res. 10, 650–658. doi:10.1302/2046-3758.1010.bjr-2020-0540.r1
Sato, M., Uchida, K., Nakajima, H., Miyazaki, T., Guerrero, A. R., Watanabe, S., et al. (2012). Direct transplantation of mesenchymal stem cells into the knee joints of Hartley strain Guinea pigs with spontaneous osteoarthritis. Arthritis Res. Ther. 14, R31. doi:10.1186/ar3735
Saulnier, N., Viguier, E., Perrier-Groult, E., Chenu, C., Pillet, E., Roger, T., et al. (2015). Intra-articular administration of xenogeneic neonatal Mesenchymal Stromal Cells early after meniscal injury down-regulates metalloproteinase gene expression in synovium and prevents cartilage degradation in a rabbit model of osteoarthritis. Osteoarthr. Cartil. 23, 122–133. doi:10.1016/j.joca.2014.09.007
Saw, K. Y., Anz, A., Siew-Yoke Jee, C., Merican, S., Ching-Soong Ng, R., Roohi, S. A., et al. (2013). Articular cartilage regeneration with autologous peripheral blood stem cells versus hyaluronic acid: a randomized controlled trial. Arthrosc. J. Arthrosc. Relat. Surg. official Publ. Arthrosc. Assoc. N. Am. Int. Arthrosc. Assoc. 29, 684–694. doi:10.1016/j.arthro.2012.12.008
Shapiro, S. A., Kazmerchak, S. E., Heckman, M. G., Zubair, A. C., and O’Connor, M. I. (2017). A prospective, single-blind, placebo-controlled trial of bone marrow aspirate concentrate for knee osteoarthritis. Am. J. Sports Med. 45, 82–90. doi:10.1177/0363546516662455
Shetty, A. A., Kim, S. J., Shanmugasundaram, S., Shetty, N., Stelzeneder, D., and Kim, C. S. (2022). Injectable cultured bone marrow derived mesenchymal cells vs chondrocytes in the treatment of chondral defects of the knee - RCT with 6 years follow-up. J. Clin. Orthop. Trauma 28, 101845. doi:10.1016/j.jcot.2022.101845
Shimomura, K., Moriguchi, Y., Ando, W., Nansai, R., Fujie, H., Hart, D. A., et al. (2014). Osteochondral repair using a scaffold-free tissue-engineered construct derived from synovial mesenchymal stem cells and a hydroxyapatite-based artificial bone. Tissue Eng. Part A 20, 2291–2304. doi:10.1089/ten.tea.2013.0414
Shimomura, K., Yasui, Y., Koizumi, K., Chijimatsu, R., Hart, D. A., Yonetani, Y., et al. (2018). First-in-Human pilot study of implantation of a scaffold-free tissue-engineered construct generated from autologous synovial mesenchymal stem cells for repair of knee chondral lesions. Am. J. Sports Med. 46, 2384–2393. doi:10.1177/0363546518781825
Singh, A., Goel, S. C., Gupta, K. K., Kumar, M., Arun, G. R., Patil, H., et al. (2014). The role of stem cells in osteoarthritis: an experimental study in rabbits. Bone Jt. Res. 3, 32–37. doi:10.1302/2046-3758.32.2000187
Song, F., Tang, J., Geng, R., Hu, H., Zhu, C., Cui, W., et al. (2014). Comparison of the efficacy of bone marrow mononuclear cells and bone mesenchymal stem cells in the treatment of osteoarthritis in a sheep model. Int. J. Clin. Exp. Pathol. 7, 1415–1426.
Song, J. S., Hong, K. T., Kim, N. M., Hwangbo, B. H., Yang, B. S., Victoroff, B. N., et al. (2023). Clinical and magnetic resonance imaging outcomes after human cord blood-derived mesenchymal stem cell implantation for chondral defects of the knee. Orthop. J. Sports Med. 11, 232596712311583. doi:10.1177/23259671231158391
Srzentić Dražilov, S., Mrkovački, J., Spasovski, V., Fazlagić, A., Pavlović, S., and Nikčević, G. (2018). The use of canine mesenchymal stem cells for the autologous treatment of osteoarthritis. Acta Vet. Hung 66, 376–389. doi:10.1556/004.2018.034
Taghiyar, L., Jahangir, S., Khozaei Ravari, M., Shamekhi, M. A., and Eslaminejad, M. B. (2021). Cartilage repair by mesenchymal stem cell-derived exosomes: preclinical and clinical trial update and perspectives. Adv. Exp. Med. Biol. 1326, 73–93. doi:10.1007/5584_2021_625
Tang, S., Chen, P., Zhang, H., Weng, H., Fang, Z., Chen, C., et al. (2021). Comparison of curative effect of human umbilical cord-derived mesenchymal stem cells and their small extracellular vesicles in treating osteoarthritis. Int. J. Nanomedicine 16, 8185–8202. doi:10.2147/ijn.s336062
ter Huurne, M., Schelbergen, R., Blattes, R., Blom, A., de Munter, W., Grevers, L. C., et al. (2012). Antiinflammatory and chondroprotective effects of intraarticular injection of adipose-derived stem cells in experimental osteoarthritis. Arthritis Rheum. 64, 3604–3613. doi:10.1002/art.34626
Toghraie, F., Razmkhah, M., Gholipour, M. A., Faghih, Z., Chenari, N., Torabi Nezhad, S., et al. (2012). Scaffold-free adipose-derived stem cells (ASCs) improve experimentally induced osteoarthritis in rabbits. Arch. Iran. Med. 15, 495–499. 012158/AIM.0011.
Toh, W. S., Lim, T. C., Kurisawa, M., and Spector, M. (2012). Modulation of mesenchymal stem cell chondrogenesis in a tunable hyaluronic acid hydrogel microenvironment. Biomaterials 33, 3835–3845. doi:10.1016/j.biomaterials.2012.01.065
Tong, W., Zhang, X., Zhang, Q., Fang, J., Liu, Y., Shao, Z., et al. (2020). Multiple umbilical cord-derived MSCs administrations attenuate rat osteoarthritis progression via preserving articular cartilage superficial layer cells and inhibiting synovitis. J. Orthop. Transl. 23, 21–28. doi:10.1016/j.jot.2020.03.007
Trippel, S. B. (2023). Harnessing growth factor interactions to optimize articular cartilage repair. Adv. Exp. Med. Biol. 1402, 135–143. doi:10.1007/978-3-031-25588-5_10
Tsai, S. Y., Huang, Y. C., Chueh, L. L., Yeh, L. S., and Lin, C. S. (2014). Intra-articular transplantation of porcine adipose-derived stem cells for the treatment of canine osteoarthritis: a pilot study. World J. Transpl. 4, 196–205. doi:10.5500/wjt.v4.i3.196
Ude, C. C., Shamsul, B. S., Ng, M. H., Chen, H. C., Ohnmar, H., Amaramalar, S. N., et al. (2018). Long-term evaluation of osteoarthritis sheep knee, treated with TGF-β3 and BMP-6 induced multipotent stem cells. Exp. Gerontol. 104, 43–51. doi:10.1016/j.exger.2018.01.020
Ude, C. C., Sulaiman, S. B., Min-Hwei, N., Hui-Cheng, C., Ahmad, J., Yahaya, N. M., et al. (2014). Cartilage regeneration by chondrogenic induced adult stem cells in osteoarthritic sheep model. PLoS One 9, e98770. doi:10.1371/journal.pone.0098770
van Buul, G. M., Siebelt, M., Leijs, M. J., Bos, P. K., Waarsing, J. H., Kops, N., et al. (2014). Mesenchymal stem cells reduce pain but not degenerative changes in a mono-iodoacetate rat model of osteoarthritis. J. Orthop. Res. 32, 1167–1174. doi:10.1002/jor.22650
Vega, A., Martin-Ferrero, M. A., Del Canto, F., Alberca, M., Garcia, V., Munar, A., et al. (2015). Treatment of knee osteoarthritis with allogeneic bone marrow mesenchymal stem cells: a randomized controlled trial. Transplantation 99, 1681–1690. doi:10.1097/tp.0000000000000678
Venosa, M., Calafiore, F., Mazzoleni, M., Romanini, E., Cerciello, S., and Calvisi, V. (2022). Platelet-rich plasma and adipose-derived mesenchymal stem cells in association with arthroscopic microfracture of knee articular cartilage defects: a pilot randomized controlled trial. Adv. Orthop. 2022, 1–9. doi:10.1155/2022/6048477
Vinod, E., James, J. V., Sabareeswaran, A., Amirtham, S. M., Thomas, G., Sathishkumar, S., et al. (2019). Intraarticular injection of allogenic chondroprogenitors for treatment of osteoarthritis in rabbit knee model. J. Clin. Orthop. Trauma 10, 16–23. doi:10.1016/j.jcot.2018.07.003
Wang, A. T., Zhang, Q. F., Wang, N. X., Yu, C. Y., Liu, R. M., Luo, Y., et al. (2020). Cocktail of hyaluronic acid and human amniotic mesenchymal cells effectively repairs cartilage injuries in sodium iodoacetate-induced osteoarthritis rats. Front. Bioeng. Biotechnol. 8, 87. doi:10.3389/fbioe.2020.00087
Wang, G., Xing, D., Liu, W., Zhu, Y., Liu, H., Yan, L., et al. (2022). Preclinical studies and clinical trials on mesenchymal stem cell therapy for knee osteoarthritis: a systematic review on models and cell doses. Int. J. Rheum. Dis. 25, 532–562. doi:10.1111/1756-185x.14306
Wang, W., He, N., Feng, C., Liu, V., Zhang, L., Wang, F., et al. (2015). Human adipose-derived mesenchymal progenitor cells engraft into rabbit articular cartilage. Int. J. Mol. Sci. 16, 12076–12091. doi:10.3390/ijms160612076
Wang, X., Song, X., Li, T., Chen, J., Cheng, G., Yang, L., et al. (2019). Aptamer-functionalized bioscaffold enhances cartilage repair by improving stem cell recruitment in osteochondral defects of rabbit knees. Am. J. Sports Med. 47, 2316–2326. doi:10.1177/0363546519856355
Wang, X., Zhao, Y., Li, S., Wang, Y., Jia, C., Yang, X., et al. (2023). Activation of the kynurenine-aryl hydrocarbon receptor axis impairs the chondrogenic and chondroprotective effects of human umbilical cord-derived mesenchymal stromal cells in osteoarthritis rats. Hum. Cell 36, 163–177. doi:10.1007/s13577-022-00811-4
Wu, Y., Yang, Z., Denslin, V., Ren, X., Lee, C. S., Yap, F. L., et al. (2020). Repair of osteochondral defects with predifferentiated mesenchymal stem cells of distinct phenotypic character derived from a nanotopographic platform. Am. J. Sports Med. 48, 1735–1747. doi:10.1177/0363546520907137
Xing, D., Wang, K., Wu, J., Zhao, Y., Liu, W., Li, J. J., et al. (2021). Clinical-grade human embryonic stem cell-derived mesenchymal stromal cells ameliorate the progression of osteoarthritis in a rat model. Molecules 26, 604. doi:10.3390/molecules26030604
Xing, D., Wu, J., Wang, B., Liu, W., Liu, W., Zhao, Y., et al. (2020). Intra-articular delivery of umbilical cord-derived mesenchymal stem cells temporarily retard the progression of osteoarthritis in a rat model. Int. J. Rheum. Dis. 23, 778–787. doi:10.1111/1756-185x.13834
Xu, J., Feng, Q., Lin, S., Yuan, W., Li, R., Li, J., et al. (2019). Injectable stem cell-laden supramolecular hydrogels enhance in situ osteochondral regeneration via the sustained co-delivery of hydrophilic and hydrophobic chondrogenic molecules. Biomaterials 210, 51–61. doi:10.1016/j.biomaterials.2019.04.031
Yang, X., Zhu, T. Y., Wen, L. C., Cao, Y. P., Liu, C., Cui, Y. P., et al. (2015). Intraarticular injection of allogenic mesenchymal stem cells has a protective role for the osteoarthritis. Chin. Med. J. Engl. 128, 2516–2523. doi:10.4103/0366-6999.164981
Ye, K., Traianedes, K., Robins, S. A., Choong, P. F. M., and Myers, D. E. (2018). Osteochondral repair using an acellular dermal matrix-pilot in vivo study in a rabbit osteochondral defect model. J. Orthop. Res. 36, 1919–1928. doi:10.1002/jor.23837
Yilmaz, B., Tahmasebifar, A., and Baran, E. T. (2020). Bioprinting technologies in tissue engineering. Adv. Biochem. Eng. Biotechnol. 171, 279–319. doi:10.1007/10_2019_108
Yoon, I. S., Chung, C. W., Sung, J. H., Cho, H. J., Kim, J. S., Shim, W. S., et al. (2011). Proliferation and chondrogenic differentiation of human adipose-derived mesenchymal stem cells in porous hyaluronic acid scaffold. J. Biosci. Bioeng. 112, 402–408. doi:10.1016/j.jbiosc.2011.06.018
You, Q., Liu, Z., Zhang, J., Shen, M., Li, Y., Jin, Y., et al. (2020). Human amniotic mesenchymal stem cell sheets encapsulating cartilage particles facilitate repair of rabbit osteochondral defects. Am. J. Sports Med. 48, 599–611. doi:10.1177/0363546519897912
Yun, S., Ku, S. K., and Kwon, Y. S. (2016). Adipose-derived mesenchymal stem cells and platelet-rich plasma synergistically ameliorate the surgical-induced osteoarthritis in Beagle dogs. J. Orthop. Surg. Res. 11, 9. doi:10.1186/s13018-016-0342-9
Zheng, K., Ma, Y., Chiu, C., Pang, Y., Gao, J., Zhang, C., et al. (2022). Co-culture pellet of human Wharton’s jelly mesenchymal stem cells and rat costal chondrocytes as a candidate for articular cartilage regeneration: in vitro and in vivo study. Stem Cell Res. Ther. 13, 386. doi:10.1186/s13287-022-03094-6
Zhi, Z., Zhang, C., Kang, J., Wang, Y., Liu, J., Wu, F., et al. (2020). The therapeutic effect of bone marrow-derived mesenchymal stem cells on osteoarthritis is improved by the activation of the KDM6A/SOX9 signaling pathway caused by exposure to hypoxia. J. Cell Physiol. 235, 7173–7182. doi:10.1002/jcp.29615
Keywords: osteoarthritis, cartilage, stem cells, musculoskeletal health, regenerative therapy
Citation: Campbell TM and Trudel G (2024) Protecting the regenerative environment: selecting the optimal delivery vehicle for cartilage repair—a narrative review. Front. Bioeng. Biotechnol. 12:1283752. doi: 10.3389/fbioe.2024.1283752
Received: 27 August 2023; Accepted: 15 January 2024;
Published: 25 January 2024.
Edited by:
Lei Zhang, First Affiliated Hospital of Wenzhou Medical University, ChinaReviewed by:
William Serrano, University of Puerto Rico at Humacao, Puerto RicoShibu Chameettachal, Indian Institute of Technology Hyderabad, India
Copyright © 2024 Campbell and Trudel. This is an open-access article distributed under the terms of the Creative Commons Attribution License (CC BY). The use, distribution or reproduction in other forums is permitted, provided the original author(s) and the copyright owner(s) are credited and that the original publication in this journal is cited, in accordance with accepted academic practice. No use, distribution or reproduction is permitted which does not comply with these terms.
*Correspondence: T. Mark Campbell, dGNhbXBiZWxsQGJydXllcmUub3Jn