- Henan Key Laboratory of Cancer Epigenetics, Cancer Institute, The First Affiliated Hospital, College of Clinical Medicine of Henan University of Science and Technology, Luoyang, China
Esophageal cancer is a malignant tumor with a high incidence worldwide. Currently, there are a lack of effective early diagnosis and treatment methods for esophageal cancer. However, delivery systems based on nanoparticles (NPs) have shown ideal efficacy in real-time imaging and chemotherapy, radiotherapy, gene therapy, and phototherapy for tumors, which has led to their recent widespread design as novel treatment strategies. Compared to traditional drugs, nanomedicine has unique advantages, including strong targeting ability, high bioavailability, and minimal side effects. This article provides an overview of the application of NPs in the diagnosis and treatment of esophageal cancer and provides a reference for future research.
1 Background
As a common malignant tumor of the digestive system, the global incidence and mortality rates of esophageal cancer rank seventh and sixth among all malignant tumors, respectively. The number of male patients is approximately 2–3 times that of female patients (Sung et al., 2021). Currently, there are two main histological subtypes of esophageal cancer, comprising esophageal squamous cell carcinoma (ESCC) and esophageal adenocarcinoma (EAC), each of which have significant geographical differences and different risk factors. EAC, which is highly prevalent in Western countries, is associated with Barrett’s esophagus, gastroesophageal reflux disease, obesity, and smoking. In contrast, ESCC, which is highly prevalent in China and some other East Asian regions, is associated with smoking, alcohol abuse, and poor dietary habits (Morgan et al., 2022). Early symptoms of esophageal cancer are atypical and can be easily overlooked, leading to the majority of patients being diagnosed at an advanced stage, significantly increasing the difficulty of treatment and the chance of recurrence. Despite advances in tumor treatment research in recent years, as well as the emergence of various new drugs, esophageal cancer lacks the targeted therapy options available for lung cancer, which was higher mutation rates (Melosky et al., 2021). Moreover, unlike renal cancer and malignant melanoma, esophageal cancer does not respond well to immunotherapy (Yoneda et al., 2021). Therefore, chemotherapy remains the cornerstone of treatment for esophageal cancer in clinical practice; however, the accompanying toxic side effects and strong drug resistance should not be underestimated.
The rapid development of nanomedicine undoubtedly brings a ray of hope to this problem. Multiple studies have confirmed that nanoparticles (NPs) show good results in tumor imaging, targeted drug delivery, tumor immunotherapy, and tumor photothermal therapy due to their unique structure and properties. To construct an ideal nanomaterial, it is important to consider not only size, shape, and surface charge, but also hydrophilicity and deformability (Lakshmanan et al., 2021). For example, loading of the polymer material poly lactic-co-glycolic acid (PLGA) with polyethylene glycol (PEG) can improve solubility and enhance cell uptake in the tumor microenvironment, while also reducing immunogenicity and prolonging the circulation time of drugs in the blood, ultimately avoiding the recognition of NPs by the reticuloendothelial system (RES) (Ding and Zhu, 2018). Subsequently, due to the damaged endothelial blood vessels and abnormal lymphatic drainage, more NPs accumulate in tumor tissues, known as the enhanced permeability and retention effect (EPR) (Jhaveri and Torchilin, 2014).
Currently, nanomaterial delivery systems used for tumor diagnosis and treatment can be mainly divided into four types (Al-Zoubi and Al-Zoubi, 2022) (Figure 1): 1) organic NPs, such as liposomes, polymers, and nanohydrogels; 2) inorganic NPs, such as gold, carbon, silica, graphene, and other metal NPs; 3) viral NPs (Mellid-Carballal et al., 2023); and 4) hybrid NPs, such as organic-inorganic NPs, and biofilm-coated NPs (Peng and Yao, 2022). This article provides a comprehensive overview of nanomedical research related to esophageal cancer diagnosis and treatment (Table 1), offering different perspectives and a reference basis for future design and exploration of novel nanosystems.
2 Diagnosis
From the histological perspective, the esophagus is a muscular duct that has a certain degree of expansibility and activity. As a result, clinical symptoms of esophageal stenosis or obstruction, such as difficulty in swallowing and pain, only occur when the tumor reaches a relatively advanced local or metastatic stage (Smyth et al., 2017). At this time, endoscopy is undoubtedly the first choice, which not only allows for a biopsy to determine the pathological classification, but also enables the identification of tumor location, lesion length, and surrounding involvement (Schmidlin and Gill, 2021). Imaging examinations, such as computed tomography (CT), magnetic resonance imaging (MRI), positron emission tomography (PET-CT), and single photon emission computed tomography (SPECT), are also used for staging and overall assessment of esophageal cancer. However, these imaging techniques have limitations to varying degrees, including the presence of artifacts, ionizing radiation damage, rapid clearance of contrast agents, and long scanning time (Nagaraju et al., 2021).
To overcome these issues, nanomaterials have been used to develop new methods to optimize tumor imaging (Liu and Grodzinski, 2021). Superparamagnetic iron oxide (SPIO) has been proven to enhance the detection rate of metastatic cervical lymph nodes in patients with esophageal cancer during MRI scans (Motoyama et al., 2012). Furthermore, studies by Pultrum et al. (2009) have shown that ultrasmall superparamagnetic iron oxide (USPIO) combined with MRI can detect most mediastinal and abdominal lymph nodes, providing a significant reference for regional staging and pre-operative assessment. Additionally, a gold nanoprobe labeled with a heterobivalent (HB) peptide ligand, HB-Au-NPs, has demonstrated good results in esophageal cancer imaging, where it can specifically target overexpressed epidermal growth factor receptor (EGFR) and tyrosine kinase receptor 2 (ErbB2) in cancer cells. After injection into xenograft mouse models, a tumor uptake peak can be observed within 8 h, showing strong contrast in photoacoustic and CT imaging, while also exhibiting good stability and biocompatibility (Chen et al., 2021).
Nanomaterials have also received wide attention in the early diagnosis of esophageal cancer. Gai et al. (2018) prepared a novel chitosan-Fe3O4 NP, CNFV, encapsulated with bispecific antibodies against fibroblast growth factor receptor (FGFR) and vascular endothelial growth factor receptor (VEGFR) using a covalent bonding method. The CNFV was used for enhanced CT imaging of patients with suspected esophageal cancer. The results showed that compared to patients who underwent CT imaging alone, CECT-CNFV not only enhanced the resolution of the captured images but also significantly improved the accuracy and sensitivity for diagnosing patients with suspected early stage esophageal cancer. Notably, patients who were diagnosed early with CECT-CNFV had a higher median overall survival and median progression-free survival. As mentioned above, the use of NP-based techniques contributes to the early diagnosis and prognostic evaluation of esophageal cancer, thus guiding personalized treatment.
3 Chemotherapy
Chemotherapy has been used to treat cancer for more than a century. Most chemotherapeutic drugs act on cells during their division phase, inhibiting tumor cell growth by affecting the functions of microtubules, proteins, or DNA synthesis. However, they also exhibit side effects and toxicity to normal human tissues, some of which are even irreversible (Amreddy et al., 2018). Multidrug resistance (MDR) also poses a significant challenge and greatly reduces the effectiveness of chemotherapy (De Jong and Borm, 2008). However, with the help of NPs, chemotherapeutic drugs can achieve targeted delivery (Figure 2), increasing drug bioavailability and improving the therapeutic effects, with an effective cycle time and minimal side effects (Al Bostami et al., 2022).
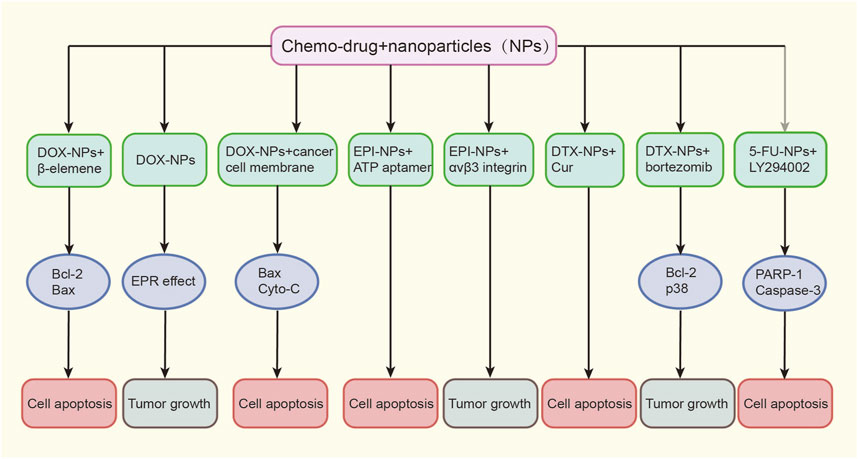
FIGURE 2. Various nanoformulations of chemo-drugs on EC growth. Doc, docetaxel; EPI, epirubicin; DTX, docetaxel; 5-FU, 5-fluorouracil; EC, esophageal cancer.
Doxorubicin (DOX) has been widely used to treat various cancers including esophageal cancer. Liposomal doxorubicin was the first liposome-encapsulated anticancer drug to gain clinical approval (Rivankar, 2014). Research on the combination of DOX and NPs for the treatment of esophageal cancer is ongoing, with the aim to achieve lower drug toxicity and better tolerance. Zhan et al. (2020) used mesoporous silica as a carrier, loaded DOX and β-elemene simultaneously, and absorbed hyaluronic acid on its surface to construct a dual-drug co-delivery nanosystem termed bMED NPs. Through a series of in vitro and in vivo experiments, bMED NPs have been proven to have not only good antitumor effects, but also accumulate in tumor tissues to achieve drug release and prolonged circulation in the body. Zhang et al. (2017) developed novel hollow carbon spheres (HCSs) for the efficient delivery of DOX. Their results showed that these spheres were easily engulfed by cancer cells and unaffected by environmental pH. Moreover, due to their ability to alter drug distribution and prolong circulation time, they exhibited significant inhibitory effects on esophageal cancer cells. Additionally, a nanocomposite composed of the polymer material PLGA connecting DOX and curcumin has been used for the treatment of drug-resistant esophageal cancer cell lines after being wrapped by TE10 cell membranes. Both in vitro cell studies and in vivo animal models have demonstrated the strong targeting and anti-tumor activity of these NPs, which also showed good biocompatibility, effectively avoiding the side effects of chemotherapeutic drugs and providing a novel strategy to treat multidrug-resistant esophageal cancer (Gao et al., 2021).
Epirubicin (EPI) is an isomer of DOX, which has received favorable attention from many researchers. In a study conducted by Wang et al. (2020), EPI was first inserted into an ATP adapter (Ap) to form a double-stranded DNA, before being compressed using polyethyleneimine (PEI) to successfully construct the PEI-Ap-EPI nanosystem. After being ingested by esophageal cancer cells, PEI-Ap-EPI was shown to open and release EPI in an ATP-enriched environment within the cells, significantly improving the inhibition efficiency against cancer cells. Fan et al. (2018) also proposed and synthesized fluorescent peptide-assembled NPs (f-PNPs) for the delivery of EPI and imaging of tumor tissues. To achieve tumor targeting, f-PNPs were first conjugated with the RGD peptide to selectively target esophageal cancer cells through the αvβ3 integrin, before embedding the NPs with EPI to obtain RGD-fPNPs/EPI. This system not only demonstrated the enhanced EPR effect of RGD-fPNPs/EPI at the cellular level, resulting in greater EPI accumulation in tumor tissue, but also proved the targeted delivery and tracking of EPI in vivo using a xenograft mouse model, achieving drug delivery to the tumor site and facilitating monitoring of the treatment response with the help of NIR fluorescence.
As a kind of natural cytotoxic drug, the taxane family changes the carcinogenic process of multiple cells, including mitosis, angiogenesis, inflammatory reaction, and cell apoptosis (Mosca et al., 2021), and its second-generation product docetaxel (DTX) is a frontline drug for treating esophageal cancer. To further improve the efficacy of DTX, Deng et al. (2020) loaded DTX and Cur together onto NPs to form T7-NP-DC. T7 functioned to enhance the targeted distribution of the nanomedicine to tumor tissues and significantly increased the release of DTX and Cur through the pH response, effectively exerting a safe and efficient anti-tumor effect. Additionally, Wang et al. (2017) prepared nanoscale formulations of DTX and bortezomib (BTZ) using a disulfide-bond-crosslinked micelle (DCM) platform (DTX-DCMs and BTZ-DCMs), further revealing that NPs loaded with chemotherapeutic drugs not only have the advantages of a small diameter and high loading rate, but can also be effectively internalized by esophageal cancer cells to induce apoptosis in a dose-dependent manner.
The 5-fluorouracil (5-FU) is another commonly used chemotherapy drug for treating esophageal cancer. The 5-FU works by inhibiting the synthesis of thymidylate synthase, thus blocking DNA synthesis and ultimately affecting tumor growth and proliferation. However, toxicity and MDR remain major factors limiting its effectiveness (Longley et al., 2003). Previous studies have reported that autophagy inhibitors can overcome the MDR of cancer cells (Kumar et al., 2012). Therefore, Feng et al. (2018) designed a PEG nano-liposome loaded with both 5-FU and the autophagy inhibitor LY294002 (LY) for targeted therapy of esophageal cancer. The results confirmed that this unique drug delivery system achieved controlled release of both components, with a relatively faster release rate of LY compared to 5-FU. Moreover, when autophagy was inhibited, the cancer cells showed significantly increased sensitivity to 5-FU, leading to higher levels of apoptosis.
4 Radiotherapy
Radiation therapy is a commonly used method for treating esophageal cancer. Concurrent chemoradiotherapy (CCRT) is of vital importance for patients with inoperable or pre-operative esophageal cancer, while radiation therapy alone provides a good choice for patients who cannot tolerate or have shown a limited response to chemotherapy (Li et al., 2016). However, due to the presence of intrinsically and radiation-induced resistant tumor cells, radiation therapy is not completely effective. Radiation resistance is mainly caused by hypoxia, DNA damage repair, cell cycle arrest, related gene alterations, and tumor stem cells, and in certain cases, it can even promote tumor invasion and metastasis (Chen et al., 2020a). Therefore, it is crucial to research and develop safe and effective radiosensitizers.
The level of miR181a in the serum of patients with esophageal cancer can be used to predict the sensitivity of locally advanced ESCC to radiotherapy and has a radiosensitizing effect (Xiang et al., 2014). Zhou et al. (2021) designed a multifunctional nanocomposite material to effectively deliver miR181a to tumor tissue. They first anchored CeOs NPs to two-dimensional graphdiyne (GDY) with sp2-and sp-hybridized carbon atoms to enhance its catalase (CAT) activity, and then connected miR181a to it. Finally, they encapsulated the above composite material with PEG-iRGD to improve its dispersibility and stability. When combined with radiotherapy, GDY-CeO2-miR181a-PEG-iRGD showed significant radiosensitizing effects in both cell-derived xenograft and PDX (patient-derived tumor xenograft) models of ESCC. Moreover, Ren et al. (2018) took advantage of the common high expression of EGFR in esophageal cancer and coupled the recombinant protein anti-EGFR-iRGD to the surface of PTX wrapped in red blood cell membranes to form a new nanodrug termed iEPRNPs. In vitro experiments showed that compared to free PTX, iE-PRNPs had significantly enhanced radiosensitizing effects in EGFR-overexpressing esophageal cancer cells and exhibited good targeting, high penetration, and ideal sustained release characteristics.
Microbubbles (MBs) are materials composed of gas as a core (usually perfluorocarbon) and lipids, polymers, or proteins as a shell membrane, which can be used as a contrast agent for ultrasound imaging after intravenous injection (Czarnota et al., 2012). Ultrasound-stimulated microbubbles (USMBs) have been proven to induce endothelial cell apoptosis to achieve anti-angiogenesis effects. USMBs have also shown synergistic effects with radiotherapy for treating various malignant tumors, such as colon cancer, breast cancer, and prostate cancer (Huang et al., 2013; Al-Mahrouki et al., 2014; Lai et al., 2016). Similarly, the enhanced effect of radiotherapy mediated by USMBs has been observed in esophageal cancer, which was accompanied by the inhibition of proliferation, migration, and invasion ability of esophageal cancer cells, as well as the promotion of their apoptosis (Shi et al., 2021).
Metal nanomaterials also exhibit good radio enhancement effects. Zhou et al. (2022) synthesized UiO-66-NH2 (Hf) with a diameter < 100 nm based on metal-organic frameworks (MOFs) under atmospheric pressure conditions, which maintain stability in physiological environments. In vivo and in vitro experiments demonstrated that UiO-66-NH2 (Hf) enhanced X-ray absorption leading to DNA breakage in cancer cells, increased generation of reactive oxygen species (ROS), and ultimately induced apoptosis. Furthermore, Chang et al. (2015) used rhenium-188 (188Re) liposomes in combination with radiotherapy for treating esophageal cancer. The results showed that the inhibition rate of tumor growth achieved by this combination therapy reached 53%, which was much higher than that achieved with individual treatments, without increasing biotoxicity and side effects.
5 Gene therapy
The siRNA is a type of double-stranded RNA molecule consisting of 20–23 nucleotides (Alshaer et al., 2021). In 1998, by conducting an antisense RNA inhibition experiment in the nematode Caenorhabditis elegans, Fire et al. (1998) discovered that double-stranded RNA was 10 times more efficient at inducing gene silencing compared to single-stranded or antisense RNA. The siRNA was speculated to play a central role in RNA silencing in a phenomenon termed RNA interference (RNAi). As a result of this discovery, Andrew Z. Fire and Craig C. Mello were awarded the Nobel Prize in Physiology or Medicine in 2006 (Dong et al., 2019). Since then, siRNA-based therapeutic strategies have been widely used in the research of various diseases, including cancer, viral infections, and genetic inherited diseases. However, the application of siRNA in vivo poses challenges due to its instability, off-target effects, and susceptibility to degradation by nucleases (Nikam and Gore, 2018). The emergence of nanodelivery systems undoubtedly provides new solutions to this problem. Zhang et al. (2022) designed a multifunctional carboxymethyl chitosan-based NP (CEAMB NPs) capable of simultaneously delivering DOX and siRNAs targeting major vault protein (MVP) and B-cell lymphoma-2 (BCL2) to explore its anti-tumor effect in esophageal cancer. The results showed that CEAMB NPs not only effectively targeted the tumor site and suppressed the expression of target genes but also greatly enhanced the ability of DOX to induce apoptosis in tumor cells, significantly improving the therapeutic effect. Moreover, Jun et al. (2020) developed mEYLNs-Dox/siLPCAT1, a liposome nanocarrier with a layer of white blood cell membrane wrapped on its surface carrying both DOX and siRNA targeting the lipid synthesis metabolic gene LPCAT1. Studies have shown that this combination of chemotherapy and gene therapy exhibited a strong synergistic effect, achieving a therapeutic effect greater than the sum of individual treatments, while the encapsulation of the white blood cell membrane significantly prolonged the circulation time of the drugs, making it an ideal medium for targeted delivery.
As a type of single-stranded non-coding RNA, microRNAs (miRNAs) play a key role at the post-transcriptional level, participating in the regulation of a series of biological activities in vivo, including cell growth, tissue differentiation, and angiogenesis (Diener et al., 2022). The miRNAs are also abnormally expressed in various cancers and regulate the growth, invasion, and metastasis of tumors through functions similar to oncogenes or tumor suppressor genes (Ganju et al., 2017). Therefore, miRNAs have become important therapeutic targets for various cancers. The miR-375 is a tumor suppressor factor that is lowly expressed in ESCC tissues. Increased levels of miR-375 induce the expression of Bax, Caspase-3, and E-cadherin, thereby limiting the occurrence and development of esophageal cancer by promoting apoptosis and inhibiting epithelial-mesenchymal transition (EMT). To facilitate targeted delivery of miR-375, Li et al. (2021) designed a novel four-way junction RNA nanocarrier and loaded it with paclitaxel (PTX) and EGFR-specific aptamer (EGFRapt) to form a 4WJ-EGFRapt-miR-375-PTX nanosystem. Under the modification of EGFRapt, esophageal cancer cells showed significantly enhanced endocytosis of the nanodrug, allowing selective accumulation of miR-375 and PTX at the tumor site, demonstrating stronger therapeutic effects and lower systemic toxicity (Figure 3). Furthermore, Deng et al. (2017) used a simple two-step assembly method to connect the tumor suppressor miRNA-203 and near-infrared (NIR) fluorescent imaging agent cyanine-5 (Cy-5) on the basis of nanodiamond clusters (NDs). They further confirmed that this nanosystem (miR-203/F-PNDs) not only significantly inhibited the proliferation of Ec-109 cells in vitro but also achieved precise imaging of the tumor site after intravenous injection for 24 h.
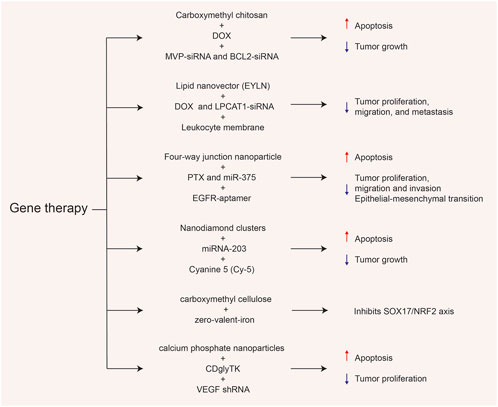
FIGURE 3. Various gene-related nanosystems and their therapeutic role in esophageal cancer (EC). DOX, doxorubicin; MVP, major vault protein; BCL2, B-cell lymphoma-2; PTX paclitaxel; NRF2, Nuclear factor-erythroid 2-related factor 2; VEGF, vascular endothelial growth factor.
Nuclear factor-erythroid 2-related factor 2 (NRF2), derived from the redox system, is the main regulatory factor in the cellular antioxidant response, with increasing evidence suggesting that NRF2 plays a role in the progression, metastasis, and therapeutic resistance of various cancers (Rojo de la Vega et al., 2018). Hsieh et al. (2022) found that in ESCC, NRF2 was negatively correlated with the expression of a tumor suppressor factor, SOX17, which is lowly expressed due to promoter hypermethylation. They also proved that SOX17 acted as an upstream inhibitor of NRF2 and that its downregulation was associated with a poor response to CCRT and low survival rate. Based on this, they designed carboxymethyl cellulose-coated zero-valent-iron (ZVI@CMC) NPs to inhibit the activity of DNA methyltransferases (DNMTs), thereby restoring the expression of SOX17 and overcoming the tumor resistance caused by NRF2 upregulation. Coincidentally, in order to treat carboplatin-resistant ovarian cancer, researchers have also designed a hyaluronic acid (HA)-decorated metal-organic framework (MOF) to specifically deliver GSK-J, the JMJD3 demethylase inhibitor. The negatively charged HA and positively charged MOF can form a stable nano-shell through strong surface affinity. It has been verified that the formed nanosystem HA@MOF@GSK-J1 can target multiple receptors, such as CD44 and HER2, to treat carboplatin-resistant ovarian cancer cells (Yang et al., 2022).
Suicide gene therapy is a novel approach to treating malignant tumors, in which genes from bacteria or viruses are introduced into target cells, and the enzymes they express are used to activate drug precursors or encode toxic substances for direct gene therapy. This approach induces tumor cell suicide without affecting normal cells (Navarro et al., 2016). Currently, cytosine deaminase (CD) and herpes simplex virus thymidine kinase (HSV-TK) are the two most extensively studied suicide genes (Uckert et al., 1998). Previous studies have shown that the fusion gene (CDglyTK) of yeast cytosine deaminase (yCD) and thymidine kinase (TK) was more effective than a single suicide gene. Therefore, Liu et al. (2016) constructed calcium phosphate NPs (CPNP) to deliver CDglyTK and shRNA against vascular endothelial growth factor (VEGF). CPNP efficiently delivered CDglyTK into EC9706 cells, converting 5-fluorocytosine (5-FC) to the cytotoxic drug 5-fluorouracil (5-FU) locally, leading to death of surrounding tumor cells. Meanwhile, VEGF-targeted shRNA further enhanced the antitumor effect, demonstrating a more effective anti-esophageal cancer effect both in vitro and in vivo.
6 Phytochemical therapy
In recent years, researchers have gradually discovered that certain natural chemical substances found in plants have anticancer activities, which can affect the biological activities of tumor cells through targeting signaling pathways, promoting apoptosis, blocking cell cycle progression, and regulating antioxidant reactions (Jang and Lee, 2023). However, their clinical applications are limited due to issues such as low solubility, poor permeability, high hepatotoxicity, and unsuitable pharmacokinetic parameters. To address these problems, NPs have been used to deliver plant chemical substances to specific tumor cells or tissues, while also improving their water solubility and bioavailability (Rizwanullah et al., 2018).
Cur is a yellow pigment extracted from the rhizomes of the ginger plant, a member of the ginger family. As a lipophilic polyphenol, Cur exhibits anti-inflammatory, anti-cancer, antiviral, and antioxidant properties (Kotha and Luthria, 2019). Martin et al. (2015) used polylactic-co-glycolic-co-polyethylene glycol acid (PLGA-b-PEG-COOH) to simultaneously load Cur and lipophilic gold nanorods (GNRs), forming a new nanosystem termed GNRs-1/Curc@PMs, and verified its anti-proliferative effect in esophageal adenocarcinoma OE-19 cells. Additionally, to overcome the poor water solubility of Cur, Niu et al. (2020) prepared Cur NPs based on class I hydrophobin recombinant HGFI (rHGFI) using a freeze-drying method. The results showed that with the help of rHGFI, the solubility and stability of Cur significantly improved, and it exhibited stronger anti-esophageal cancer effects than the free Cur.
Oridonin is another well-known phytochemical agent with potential anti-cancer activity. An increasing number of studies have demonstrated that oridonin can play a role for treating various malignant tumors, including lung cancer, cervical cancer, breast cancer, gastric cancer, and colorectal cancer (Liu X. et al., 2021). Pi et al. (2017) synthesized selenium element-based NPs conjugated with the EGFR-binding peptide GE11 to encapsulate and deliver oridonin to treat esophageal cancer. GE11-Ori-Se NPs taken-up by KYSE-150 cells (an EGFR-overexpressing esophageal cancer cell line) were found to not only accumulate in lysosomes and release oridonin, but also induce cancer cell apoptosis through inducing ROS production, activating the mitochondrial-dependent pathway, and inhibiting the EGFR-mediated PI3K/AKT and Ras/Raf/MEK/ERK pathways. Similar results were observed in the study by Jiang et al. (2018), where they functionalized graphene oxides (GO) with the GE11 peptide to create a novel nanomedicine (Ori@GE11-GO) for targeted delivery of oridonin. This modification of the GE11 peptide allowed oridonin to achieve specific recognition of EGFR-overexpressing esophageal cancer cells and improve anticancer efficiency through pathways such as cell cycle blockade, disruption of mitochondrial membrane potential, and activation of apoptosis signaling pathways.
7 Phototherapy
Recently, phototherapy has attracted much attention from researchers due to its minimally invasive and repeatable advantages, which have led to its gradual use for treating various malignant tumors. Phototherapy includes photodynamic therapy (PDT) and photothermal therapy (PTT) (Li et al., 2020) (Figure 4). PDT mainly relies on the cell death and apoptosis induced by ROS produced by photosensitizers under laser irradiation. The combination of photosensitizers and nanomaterials can improve the efficiency of PDT and reduce toxic side effects (Kwiatkowski et al., 2018). Importantly, this therapy can also help drugs effectively cross the blood-brain barrier (BBB) sand has broad prospects in the treatment of some brain tumors. Mo and his team designed both nanosystems to help the drug better target glioblastoma. One is a combination of yolk-shell nanoparticles, cyclic arginine-glycine-aspartate, and hydroxychloroquine, encapsulated by cancer cell membranes (Mo et al., 2022). The other is exosome-modified combination of zinc sulfide (ZnS), iRGD peptide and hydroxychloroquine (Liu et al., 2023). Under laser irradiation, both types of NPs demonstrate excellent ability to target glioblastoma cells. Hydroxychloroquine effectively inhibits autophagy, significantly improving the therapeutic effect and reducing systemic toxicity. Similarly, PDT also exhibits promising therapeutic effects in esophageal cancer. Ren et al. (2022) used green fluorescence carbon dots (GCDs), which have excellent optical properties as drug carriers, and simultaneously loaded the photosensitizer Ce6, chemotherapeutic drug cisplatin, and targeting ligand EGF to prepare GCD-Ce6/Pt-EGF nanocomposites. Through in vitro and in vivo experiments, these NPs were found to actively target EGF, avoiding non-specific uptake by normal tissues, increasing the content of Ce6 and cisplatin at the tumor site. Moreover, under 660 nm laser irradiation, these NPs exhibited stronger induction of apoptosis and a stable treatment effect.
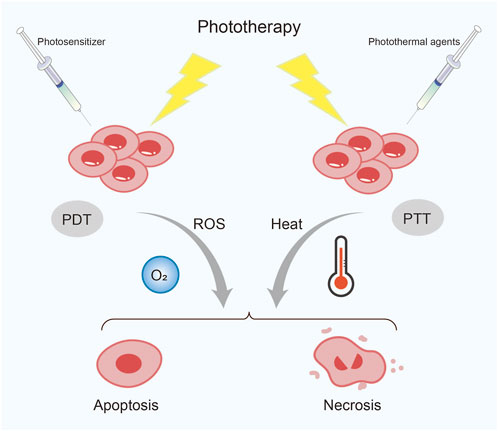
FIGURE 4. Classification of phototherapy. PDT, photodynamic therapy; PTT, photothermal therapy; ROS, reactive oxygen species.
PTT is a treatment method that uses the photothermal effect of photothermal agents (PTAs) to convert an external light source, usually near-infrared light (NIR), into heat energy, causing local high temperatures that kill tumor cells (Zhao et al., 2021). Therefore, the selection of PTAs with high photothermal conversion efficiency and good biocompatibility are prerequisites for PTT. Various types of nanomaterial-based PTAs have shown great potential and prospects in tumor PTT, currently mainly classified into five categories: noble metal materials, carbon-based materials, transition metal-based materials, organic small molecule materials, and semiconductor polymer materials (Chen et al., 2022).
Gold nanomaterials do not have inherent therapeutic effects, but their surface plasmon resonance (SPR) characteristics allow them to convert the oscillation of electrons on the particle surface into heat under NIR light irradiation, thereby triggering photothermal effects (Liu XY. et al., 2021). Li et al. (2013) used chitosan coated gold/gold sulfide (CS-GGS) NPs to treat EAC through endoscopic NIR irradiation. The results showed that this treatment method could selectively target cancer cells to achieve therapeutic effects without harming normal esophageal tissue. Chu et al. (2013) also demonstrated that Fe3O4 NPs significantly inhibited the viability and tumor growth of esophageal cancer cells both in vitro and in vivo after 808 nm NIR irradiation. Similarly, Wang et al. (2019) coated Cu9S5 NPs with silica to form a Cu9S5@MS core-shell nanostructure and investigated its anti-cancer activity on esophageal cancer EC109 and TE8 cell lines. The results showed that Cu9S5@MS could inhibit the growth of esophageal cancer cells by promoting apoptosis after 808 nm NIR irradiation and exhibited good affinity and biocompatibility. Some natural substances have also shown good photothermal conversion performance in photothermal therapy for esophageal cancer. Indeed, Chu et al. (2016) reported that natural black sesame melanin (BSM) extracted from black sesame could be assembled into 20–200 nm flaky NPs in aqueous solution, while a liposome layer was encapsulated on the surface of BSM to improve its water solubility. In a mouse model with Eca-109 cell-induced esophageal cancer, liposome-BSM not only absorbed NIR light and rapidly converted it into heat energy to achieve therapeutic effects on esophageal cancer, but also accurately located the sentinel lymph node (SLN), providing a reference for the surgical treatment of esophageal cancer.
Despite these successes, single PTT therapy still has limitations. To further enhance the treatment effect, many researchers are attempting to co-load chemotherapeutic drugs and PTAs onto NPs to achieve synergistic therapy of chemotherapy and PTT. For example, Jin et al. (2017) simultaneously encapsulated DOX and polypyrrole (PPy) in the core of hollow tantalum oxide (TaOx) NPs, before coupling NIR fluorescent dye (NIRDye800) onto their shells to form the PPy&DOX@TaOx-NIRDye800-PEG nanosystem. As tantalum has a similar X-ray attenuation coefficient to gold and higher than iodine, it can provide strong contrast in CT imaging, facilitating initial tumor localization. When treating KYSE30 tumor-bearing mice, the NPs significantly increased the temperature of the tumor site after laser irradiation, achieving a tumor growth inhibition rate of up to 100%, with no cases of recurrence. They also demonstrated good photoacoustic and fluorescence imaging capabilities, which assisted with real-time monitoring of tumor location and anatomy. The combination of these three imaging modalities provides new directions for early diagnosis and precise treatment of tumors.
In many PTT-based combination therapies, the combination of PDT and PTT also exhibits a synergistic effect of “1 + 1 > 2”. Zmerli et al. (2021) took advantage of the good photothermal conversion performance of polydopamine (PDA) to conjugate photosensitizers and synthesize polyethylene glycolated PDA NPs. By combining two phototherapy modalities under different wavelengths, a synergistic phototoxic effect was achieved, which significantly inhibited the growth of esophageal cancer KYSE-30 and HET-1A cells. Additionally, Yu et al. (2013) prepared a nanocomposite of thermosensitizer gold selenide (Au2Se/Au) and photosensitizer zinc phthalocyanine (ZnPc), which was irradiated with a 655-nm laser to generate ROS and thermotherapy effects on esophageal cancer Eca-109 cells. After 20 min of treatment, the cell survival rate was only about 20%, which was significantly better than treatment using PDT or PTT alone. The photosensitizer IR820 has good tissue penetration and excellent photothermal conversion efficiency, but it is easily metabolized in the body and cannot accumulate in large quantities in tumor sites. To overcome this problem, Liu et al. (2020) chose to deliver IR820 using hollow carbon nanocages (CNCs) and crosslink bovine serum albumin-manganese dioxide (BSA-MnO2) nanozyme on their surface to form the BMIOC nanosystem. In the BALB/c nude mouse esophageal cancer model, BMIOC not only rapidly produced O2 in the tumor tissue, enhancing the PDT efficacy, but also achieved high concentrations of IR820 at the tumor site; this system improved the PTT effect and realized real-time magnetic resonance (MR) imaging and NIR fluorescence imaging. Similarly, Chen et al. (2020b) used BSA-MnO2 as an oxygen generator and connected PDA encapsulated with porphyrin porous amine (PZM) to load the chemotherapeutic drug irinotecan to successfully prepare the FA-PDA@PZM/SN38@BSA-MnO2 (FA-PPSM) nanosystem for the treatment of esophageal cancer. The results showed significant tumor suppression effects of FA-PPSM under 580 nm and 808 nm laser irradiation. Compared to free irinotecan, the side effects, such as diarrhea and bone marrow suppression, were greatly reduced, achieving synergistic treatment of PTT, PDT, and chemotherapy.
8 Conclusion
NPs have potential value in improving the current diagnosis and treatment methods for esophageal cancer, showing definite effects in real-time imaging, drug delivery, radiosensitization, and photothermal and photodynamic therapy. Compared to traditional therapies, NPs have unique advantages in terms of targeted drug delivery and sustained release, improving drug pharmacokinetics, overcoming tumor cell resistance mechanisms, and reducing toxicity to normal tissues. However, there are still some key problems to be solved in order to realize clinical transformation. First, efficacy and safety are important evaluation indicators for the superiority of drugs. Currently, research on NPs is mainly focused on in vitro and animal experiments. However, existing animal models, including subcutaneous and orthotopic xenograft models based on tumor cell lines, patient-derived xenograft models (PDXs), and genetically engineered mouse models (GEMMs) (Gonzalez-Valdivieso et al., 2021), cannot fully simulate the physiological barriers between organisms and nanomedicines. The complexity of the human immune system makes the acute toxicity and potential long-term side effects of NPs difficult to predict. The Phase I clinical trial of the liposomal drug, MRX34, was terminated early due to severe immune-related adverse reactions occurring in 20% of patients (Hong et al., 2020). Secondly, the synthesis process of most NPs is complex, difficult, and relatively unstable for long-term preservation. It requires a significant investment of manpower, resources, and finances, which poses great challenges for pharmaceutical companies and quality control departments. How to optimize manufacturing processes and achieve large-scale and reproducible preparation while ensuring drug quality is a question that researchers must consider. In addition, the inherent pathways and mechanisms of NPs in tumor diagnosis and treatment have not been fully explored and elucidated. Only by further exploring key issues such as the interaction mechanism between NPs and the mononuclear macrophage system, the key conditions for NPs to be engulfed and taken up by tumor cells, and the environmental requirements for NPs to target tumor tissues, can we design nanosystems that are more suitable for clinical practical applications. Last but not least, the ethical issues brought about by new materials and new processes, including biological membranes, should not be ignored in the application of nanomedicine.
Therefore, in the process of translating nanomedicine from preclinical stage to clinical stage, multiple efforts are still needed. Currently, several anti-tumor nanomedicines have already been marketed or are in clinical research stages, mainly involving solid tumors, lung cancer, breast cancer, pancreatic cancer, etc. (Schmidt et al., 2020; Yoneshima et al., 2021; Kosaka et al., 2022; Rojas et al., 2023) However, there are still very few clinical trials specifically targeting esophageal cancer, and low effectiveness remains the main reason for trial failures. Thus, researchers need to further investigate from multiple disciplines such as materials science, physical chemistry, and pharmacology, taking into account key performance factors such as drug metabolism, biocompatibility, tissue distribution, and biological safety. They should design nanosystems that can overcome a series of biological barriers in the body, target tumor cells effectively and have good safety. More importantly, its effects were validated by developing novel animal models capable of simulating the heterogeneity and specific physiological environment of human tumors, accelerating clinical transformation. In addition, pharmaceutical companies need to establish quality standards and evaluation methods in advance during the mass production process, and strict implementation is ensured through collaboration between different operational units to guarantee product quality. At the same time, regulatory authorities should also develop relevant guidelines and identification technologies to promote the research and development process of nanomedicine. It is believed that in the near future, multifunctional NPs will provide a realistic basis for the integration of diagnosis and treatment, as well as individualized treatment of esophageal cancer, becoming another effective weapon for precise treatment of tumors.
Author contributions
QX: Writing–original draft. YZ: Writing–review and editing. AZ: Writing–review and editing, Funding acquisition. ZD: Writing–review and editing. JY: Supervision, Writing–review and editing.
Funding
The author(s) declare financial support was received for the research, authorship, and/or publication of this article. This work was financially supported by the National Natural Science Foundation of China (82202644).
Conflict of interest
The authors declare that the research was conducted in the absence of any commercial or financial relationships that could be construed as a potential conflict of interest.
Publisher’s note
All claims expressed in this article are solely those of the authors and do not necessarily represent those of their affiliated organizations, or those of the publisher, the editors and the reviewers. Any product that may be evaluated in this article, or claim that may be made by its manufacturer, is not guaranteed or endorsed by the publisher.
References
Al Bostami, R. D., Abuwatfa, W. H., and Husseini, G. A. (2022). Recent advances in nanoparticle-based Co-delivery systems for cancer therapy. Nanomater. (Basel) 12 (15), 2672. doi:10.3390/nano12152672
Al-Mahrouki, A. A., Iradji, S., Tran, W. T., and Czarnota, G. J. (2014). Cellular characterization of ultrasound-stimulated microbubble radiation enhancement in a prostate cancer xenograft model. Dis. Model Mech. 7 (3), 363–372. doi:10.1242/dmm.012922
Alshaer, W., Zureigat, H., Al Karaki, A., Al-Kadash, A., Gharaibeh, L., Hatmal, M. M., et al. (2021). siRNA: mechanism of action, challenges, and therapeutic approaches. Eur. J. Pharmacol. 905, 174178. doi:10.1016/j.ejphar.2021.174178
Al-Zoubi, M. S., and Al-Zoubi, R. M. (2022). Nanomedicine tactics in cancer treatment: challenge and hope. Crit. Rev. Oncol. Hematol. 174, 103677. doi:10.1016/j.critrevonc.2022.103677
Amreddy, N., Babu, A., Muralidharan, R., Panneerselvam, J., Srivastava, A., Ahmed, R., et al. (2018). Recent advances in nanoparticle-based cancer drug and gene delivery. Adv. Cancer Res. 137, 115–170. doi:10.1016/bs.acr.2017.11.003
Chang, C. H., Liu, S. Y., Chi, C. W., Yu, H. L., Chang, T. J., Tsai, T. H., et al. (2015). External beam radiotherapy synergizes 1⁸⁸Re-liposome against human esophageal cancer xenograft and modulates 1⁸⁸Re-liposome pharmacokinetics. Int. J. Nanomedicine 10, 3641–3649. doi:10.2147/IJN.S80302
Chen, J., Nguyen, V. P., Jaiswal, S., Kang, X., Lee, M., Paulus, Y. M., et al. (2021). Thin layer-protected gold nanoparticles for targeted multimodal imaging with photoacoustic and CT. Pharm. (Basel) 14 (11), 1075. doi:10.3390/ph14111075
Chen, J., Zhou, L., Wang, C., Sun, Y., Lu, Y., Li, R., et al. (2020b). A multifunctional SN38-conjugated nanosystem for defeating myelosuppression and diarrhea induced by irinotecan in esophageal cancer. Nanoscale 12 (41), 21234–21247. doi:10.1039/d0nr06266a
Chen, Y., Gao, P., Wu, T., Pan, W., Li, N., and Tang, B. (2020a). Organelle-localized radiosensitizers. Chem. Commun. (Camb). 56 (73), 10621–10630. doi:10.1039/d0cc03245j
Chen, Y., Zhou, F., Wang, C., Hu, L., and Guo, P. (2022). Nanostructures as photothermal agents in tumor treatment. Molecules 28 (1), 277. doi:10.3390/molecules28010277
Chu, M., Hai, W., Zhang, Z., Wo, F., Wu, Q., Zhang, Z., et al. (2016). Melanin nanoparticles derived from a homology of medicine and food for sentinel lymph node mapping and photothermal in vivo cancer therapy. Biomaterials 91, 182–199. doi:10.1016/j.biomaterials.2016.03.018
Chu, M., Shao, Y., Peng, J., Dai, X., Li, H., Wu, Q., et al. (2013). Near-infrared laser light mediated cancer therapy by photothermal effect of Fe3O4 magnetic nanoparticles. Biomaterials 34 (16), 4078–4088. doi:10.1016/j.biomaterials.2013.01.086
Czarnota, G. J., Karshafian, R., Burns, P. N., Wong, S., Al Mahrouki, A., Lee, J. W., et al. (2012). Tumor radiation response enhancement by acoustical stimulation of the vasculature. Proc. Natl. Acad. Sci. U. S. A. 109 (30), E2033–E2041. doi:10.1073/pnas.1200053109
De Jong, W. H., and Borm, P. J. (2008). Drug delivery and nanoparticles:applications and hazards. Int. J. Nanomedicine 3 (2), 133–149. doi:10.2147/ijn.s596
Deng, L., Zhu, X., Yu, Z., Li, Y., Qin, L., Liu, Z., et al. (2020). <p>Novel T7-modified pH-responsive targeted nanosystem for Co-delivery of docetaxel and curcumin in the treatment of esophageal cancer</p>. Int. J. Nanomedicine 15, 7745–7762. doi:10.2147/ijn.s257312
Deng, X., Yin, Z., Lu, J., Xia, Y., Shao, L., Hu, Q., et al. (2017). Two-step assembling of near-infrared "OFF-ON" fluorescent nanohybrids for synchronous tumor imaging and MicroRNA modulation-based therapy. ACS Appl. Mater Interfaces 9 (4), 3294–3305. doi:10.1021/acsami.6b11438
Diener, C., Keller, A., and Meese, E. (2022). Emerging concepts of miRNA therapeutics: from cells to clinic. Trends Genet. 38 (6), 613–626. doi:10.1016/j.tig.2022.02.006
Ding, D., and Zhu, Q. (2018). Recent advances of PLGA micro/nanoparticles for the delivery of biomacromolecular therapeutics. Mater Sci. Eng. C Mater Biol. Appl. 92, 1041–1060. doi:10.1016/j.msec.2017.12.036
Dong, Y., Siegwart, D. J., and Anderson, D. G. (2019). Strategies, design, and chemistry in siRNA delivery systems. Adv. Drug Deliv. Rev. 144, 133–147. doi:10.1016/j.addr.2019.05.004
Fan, Z., Chang, Y., Cui, C., Sun, L., Wang, D. H., Pan, Z., et al. (2018). Near infrared fluorescent peptide nanoparticles for enhancing esophageal cancer therapeutic efficacy. Nat. Commun. 9 (1), 2605. doi:10.1038/s41467-018-04763-y
Feng, Y., Gao, Y., Wang, D., Xu, Z., Sun, W., and Ren, P. (2018). Autophagy inhibitor (LY294002) and 5-fluorouracil (5-FU) combination-based nanoliposome for enhanced efficacy against esophageal squamous cell carcinoma. Nanoscale Res. Lett. 13 (1), 325. doi:10.1186/s11671-018-2716-x
Fire, A., Xu, S., Montgomery, M. K., Kostas, S. A., Driver, S. E., and Mello, C. C. (1998). Potent and specific genetic interference by double-stranded RNA in Caenorhabditis elegans. Nature 391 (6669), 806–811. doi:10.1038/35888
Gai, J., Gao, Z., Song, L., Xu, Y., Liu, W., and Zhao, C. (2018). Contrast-enhanced computed tomography combined with Chitosan-Fe(3)O(4) nanoparticles targeting fibroblast growth factor receptor and vascular endothelial growth factor receptor in the screening of early esophageal cancer. Exp. Ther. Med. 15 (6), 5344–5352. doi:10.3892/etm.2018.6087
Ganju, A., Khan, S., Hafeez, B. B., Behrman, S. W., Yallapu, M. M., Chauhan, S. C., et al. (2017). miRNA nanotherapeutics for cancer. Drug Discov. Today 22 (2), 424–432. doi:10.1016/j.drudis.2016.10.014
Gao, Y., Zhu, Y., Xu, X., Wang, F., Shen, W., Leng, X., et al. (2021). Surface PEGylated cancer cell membrane-coated nanoparticles for codelivery of curcumin and doxorubicin for the treatment of multidrug resistant esophageal carcinoma. Front. Cell Dev. Biol. 9, 688070. doi:10.3389/fcell.2021.688070
Gonzalez-Valdivieso, J., Girotti, A., Schneider, J., and Arias, F. J. (2021). Advanced nanomedicine and cancer: challenges and opportunities in clinical translation. Int. J. Pharm. 599, 120438. doi:10.1016/j.ijpharm.2021.120438
Hong, D. S., Kang, Y. K., Borad, M., Sachdev, J., Ejadi, S., Lim, H. Y., et al. (2020). Phase 1 study of MRX34, a liposomal miR-34a mimic, in patients with advanced solid tumours. Br. J. Cancer 122 (11), 1630–1637. doi:10.1038/s41416-020-0802-1
Hsieh, C. H., Kuan, W. H., Chang, W. L., Kuo, I. Y., Liu, H., Shieh, D. B., et al. (2022). Dysregulation of SOX17/NRF2 axis confers chemoradiotherapy resistance and emerges as a novel therapeutic target in esophageal squamous cell carcinoma. J. Biomed. Sci. 29 (1), 90. doi:10.1186/s12929-022-00873-4
Huang, P., You, X., Pan, M., Li, S., Zhang, Y., Zhao, Y., et al. (2013). A novel therapeutic strategy using ultrasound mediated microbubbles destruction to treat colon cancer in a mouse model. Cancer Lett. 335 (1), 183–190. doi:10.1016/j.canlet.2013.02.011
Jang, J. H., and Lee, T. J. (2023). Mechanisms of phytochemicals in anti-inflammatory and anti-cancer. Int. J. Mol. Sci. 24 (9), 7863. doi:10.3390/ijms24097863
Jhaveri, A. M., and Torchilin, V. P. (2014). Multifunctional polymeric micelles for delivery of drugs and siRNA. Front. Pharmacol. 5, 77. doi:10.3389/fphar.2014.00077
Jiang, J. H., Pi, J., Jin, H., and Cai, J. Y. (2018). Functional graphene oxide as cancer-targeted drug delivery system to selectively induce oesophageal cancer cell apoptosis. Artif. Cells Nanomed Biotechnol. 46 (Suppl. 3), S297–S307. doi:10.1080/21691401.2018.1492418
Jin, Y., Ma, X., Zhang, S., Meng, H., Xu, M., Yang, X., et al. (2017). A tantalum oxide-based core/shell nanoparticle for triple-modality image-guided chemo-thermal synergetic therapy of esophageal carcinoma. Cancer Lett. 397, 61–71. doi:10.1016/j.canlet.2017.03.030
Jun, Y., Tang, Z., Luo, C., Jiang, B., Li, X., Tao, M., et al. (2020). Leukocyte-mediated combined targeted chemo and gene therapy for esophageal cancer. ACS Appl. Mater Interfaces 12 (42), 47330–47341. doi:10.1021/acsami.0c15419
Kosaka, Y., Saeki, T., Takano, T., Aruga, T., Yamashita, T., Masuda, N., et al. (2022). Multicenter randomized open-label phase II clinical study comparing outcomes of NK105 and paclitaxel in advanced or recurrent breast cancer. Int. J. Nanomedicine 17, 4567–4578. doi:10.2147/ijn.s372477
Kotha, R. R., and Luthria, D. L. (2019). Curcumin: biological, pharmaceutical, nutraceutical, and analytical aspects. Molecules 24 (16), 2930. doi:10.3390/molecules24162930
Kumar, P., Zhang, D. M., Degenhardt, K., and Chen, Z. S. (2012). Autophagy and transporter-based multi-drug resistance. Cells 1 (3), 558–575. doi:10.3390/cells1030558
Kwiatkowski, S., Knap, B., Przystupski, D., Saczko, J., Kędzierska, E., Knap-Czop, K., et al. (2018). Photodynamic therapy - mechanisms, photosensitizers and combinations. Biomed. Pharmacother. 106, 1098–1107. doi:10.1016/j.biopha.2018.07.049
Lai, P., Tarapacki, C., Tran, W. T., Kaffas, A. E., Lee, J., Hupple, C., et al. (2016). Breast tumor response to ultrasound mediated excitation of microbubbles and radiation therapy in vivo. Oncoscience 3 (3-4), 98–108. doi:10.18632/oncoscience.299
Lakshmanan, V. K., Jindal, S., Packirisamy, G., Ojha, S., Lian, S., Kaushik, A., et al. (2021). Nanomedicine-based cancer immunotherapy: recent trends and future perspectives. Cancer Gene Ther. 28 (9), 911–923. doi:10.1038/s41417-021-00299-4
Li, M., Zhang, X., Zhao, F., Luo, Y., Kong, L., and Yu, J. (2016). Involved-field radiotherapy for esophageal squamous cell carcinoma: theory and practice. Radiat. Oncol. 11, 18. doi:10.1186/s13014-016-0589-7
Li, X., Lovell, J. F., Yoon, J., and Chen, X. (2020). Clinical development and potential of photothermal and photodynamic therapies for cancer. Nat. Rev. Clin. Oncol. 17 (11), 657–674. doi:10.1038/s41571-020-0410-2
Li, X., Zhang, L., Guo, X., Xie, F., Shen, C., Jun, Y., et al. (2021). Self-assembled RNA nanocarrier-mediated chemotherapy combined with molecular targeting in the treatment of esophageal squamous cell carcinoma. J. Nanobiotechnology 19 (1), 388. doi:10.1186/s12951-021-01135-5
Li, Y., Gobin, A. M., Dryden, G. W., Kang, X., Xiao, D., Li, S. P., et al. (2013). Infrared light-absorbing gold/gold sulfide nanoparticles induce cell death in esophageal adenocarcinoma. Int. J. Nanomedicine 8, 2153–2161. doi:10.2147/IJN.S37140
Liu, C. H., and Grodzinski, P. (2021). Nanotechnology for cancer imaging: advances, challenges, and clinical opportunities. Radiol. Imaging Cancer 3 (3), e200052. doi:10.1148/rycan.2021200052
Liu, J., Gao, J., Zhang, A., Guo, Y., Fan, S., He, Y., et al. (2020). Carbon nanocage-based nanozyme as an endogenous H(2)O(2)-activated oxygenerator for real-time bimodal imaging and enhanced phototherapy of esophageal cancer. Nanoscale 12 (42), 21674–21686. doi:10.1039/d0nr05945e
Liu, T., Wu, H. J., Liang, Y., Liang, X. J., Huang, H. C., Zhao, Y. Z., et al. (2016). Tumor-specific expression of shVEGF and suicide gene as a novel strategy for esophageal cancer therapy. World J. Gastroenterol. 22 (23), 5342–5352. doi:10.3748/wjg.v22.i23.5342
Liu, W., Wei, L., Li, M., and Mo, J. (2023). Zinc sulfide-based hybrid exosome-coated nanoplatform for targeted__treatment of glioblastoma in an orthotopic mouse glioblastoma model. Mater. Today Adv. 17 (1), 100327. doi:10.1016/j.mtadv.2022.100327
Liu, X., Xu, J., Zhou, J., and Shen, Q. (2021a). Oridonin and its derivatives for cancer treatment and overcoming therapeutic resistance. Genes Dis. 8 (4), 448–462. doi:10.1016/j.gendis.2020.06.010
Liu, X. Y., Wang, J. Q., Ashby, C. R., Zeng, L., Fan, Y. F., and Chen, Z. S. (2021b). Gold nanoparticles: synthesis, physiochemical properties and therapeutic applications in cancer. Drug Discov. Today 26 (5), 1284–1292. doi:10.1016/j.drudis.2021.01.030
Longley, D. B., Harkin, D. P., and Johnston, P. G. (2003). 5-fluorouracil: mechanisms of action and clinical strategies. Nat. Rev. Cancer 3 (5), 330–338. doi:10.1038/nrc1074
Martin, R. C., Locatelli, E., Li, Y., Zhang, W., Li, S., Monaco, I., et al. (2015). Gold nanorods and curcumin-loaded nanomicelles for efficient in vivo photothermal therapy of Barrett's esophagus. Nanomedicine (Lond). 10 (11), 1723–1733. doi:10.2217/nnm.15.25
Mellid-Carballal, R., Gutierrez-Gutierrez, S., Rivas, C., and Garcia-Fuentes, M. (2023). Viral protein nanoparticles (Part 1): pharmaceutical characteristics. Eur. J. Pharm. Sci. 187, 106460. doi:10.1016/j.ejps.2023.106460
Melosky, B., Wheatley-Price, P., Juergens, R. A., Sacher, A., Leighl, N. B., Tsao, M. S., et al. (2021). The rapidly evolving landscape of novel targeted therapies in advanced non-small cell lung cancer. Lung Cancer 160, 136–151. doi:10.1016/j.lungcan.2021.06.002
Mo, J., Chen, X., Li, M., Liu, W., Zhao, W., Lim, L. Y., et al. (2022). Upconversion nanoparticle-based cell membrane-coated cRGD peptide bioorthogonally labeled nanoplatform for glioblastoma treatment. ACS Appl. Mater Interfaces 14, 49454–49470. doi:10.1021/acsami.2c11284
Morgan, E., Soerjomataram, I., Rumgay, H., Coleman, H. G., Thrift, A. P., Vignat, J., et al. (2022). The global landscape of esophageal squamous cell carcinoma and esophageal adenocarcinoma incidence and mortality in 2020 and projections to 2040: new estimates from GLOBOCAN 2020. Gastroenterology 163 (3), 649–658.e2. doi:10.1053/j.gastro.2022.05.054
Mosca, L., Ilari, A., Fazi, F., Assaraf, Y. G., and Colotti, G. (2021). Taxanes in cancer treatment: activity, chemoresistance and its overcoming. Drug Resist Updat 54, 100742. doi:10.1016/j.drup.2020.100742
Motoyama, S., Ishiyama, K., Maruyama, K., Narita, K., Minamiya, Y., and Ogawa, J. (2012). Estimating the need for neck lymphadenectomy in submucosal esophageal cancer using superparamagnetic iron oxide-enhanced magnetic resonance imaging: clinical validation study. World J. Surg. 36 (1), 83–89. doi:10.1007/s00268-011-1322-1
Nagaraju, G. P., Srivani, G., Dariya, B., Chalikonda, G., Farran, B., Behera, S. K., et al. (2021). Nanoparticles guided drug delivery and imaging in gastric cancer. Semin. Cancer Biol. 69, 69–76. doi:10.1016/j.semcancer.2020.01.006
Navarro, S. A., Carrillo, E., Griñán-Lisón, C., Martín, A., Perán, M., Marchal, J. A., et al. (2016). Cancer suicide gene therapy: a patent review. Expert Opin. Ther. Pat. 26 (9), 1095–1104. doi:10.1080/13543776.2016.1211640
Nikam, R. R., and Gore, K. R. (2018). Journey of siRNA: clinical developments and targeted delivery. Nucleic Acid. Ther. 28 (4), 209–224. doi:10.1089/nat.2017.0715
Niu, B., Li, M., Jia, J., Zhang, C., Fan, Y. Y., and Li, W. (2020). Hydrophobin-enhanced stability, dispersions and release of curcumin nanoparticles in water. J. Biomater. Sci. Polym. Ed. 31 (14), 1793–1805. doi:10.1080/09205063.2020.1775761
Peng, T., and Yao, J. (2022). Development and application of bionic systems consisting of tumor-cell membranes. J. Zhejiang Univ. Sci. B 23 (9), 770–777. doi:10.1631/jzus.b2200156
Pi, J., Jiang, J., Cai, H., Yang, F., Jin, H., Yang, P., et al. (2017). GE11 peptide conjugated selenium nanoparticles for EGFR targeted oridonin delivery to achieve enhanced anticancer efficacy by inhibiting EGFR-mediated PI3K/AKT and Ras/Raf/MEK/ERK pathways. Drug Deliv. 24 (1), 1549–1564. doi:10.1080/10717544.2017.1386729
Pultrum, B. B., van der Jagt, E. J., van Westreenen, H. L., van Dullemen, H. M., Kappert, P., Groen, H., et al. (2009). Detection of lymph node metastases with ultrasmall superparamagnetic iron oxide (USPIO)-enhanced magnetic resonance imaging in oesophageal cancer: a feasibility study. Cancer Imaging 9 (1), 19–28. doi:10.1102/1470-7330.2009.0004
Ren, G., Wang, Z., Tian, Y., Li, J., Ma, Y., Zhou, L., et al. (2022). Targeted chemo-photodynamic therapy toward esophageal cancer by GSH-sensitive theranostic nanoplatform. Biomed. Pharmacother. 153, 113506. doi:10.1016/j.biopha.2022.113506
Ren, W., Sha, H., Yan, J., Wu, P., Yang, J., Li, R., et al. (2018). Enhancement of radiotherapeutic efficacy for esophageal cancer by paclitaxel-loaded red blood cell membrane nanoparticles modified by the recombinant protein anti-EGFR-iRGD. J. Biomater. Appl. 33 (5), 707–724. doi:10.1177/0885328218809019
Rivankar, S. (2014). An overview of doxorubicin formulations in cancer therapy. J. Cancer Res. Ther. 10 (4), 853–858. doi:10.4103/0973-1482.139267
Rizwanullah, M., Amin, S., Mir, S. R., Fakhri, K. U., and Rizvi, M. (2018). Phytochemical based nanomedicines against cancer: current status and future prospects. J. Drug Target 26 (9), 731–752. doi:10.1080/1061186x.2017.1408115
Rojas, L. A., Sethna, Z., Soares, K. C., Olcese, C., Pang, N., Patterson, E., et al. (2023). Personalized RNA neoantigen vaccines stimulate T cells in pancreatic cancer. Nature 618 (7963), 144–150. doi:10.1038/s41586-023-06063-y
Rojo de la Vega, M., Chapman, E., and Zhang, D. D. (2018). NRF2 and the hallmarks of cancer. Cancer Cell 34 (1), 21–43. doi:10.1016/j.ccell.2018.03.022
Schmidlin, E. J., and Gill, R. R. (2021). New frontiers in esophageal radiology. Ann. Transl. Med. 9 (10), 904. doi:10.21037/atm-20-2909
Schmidt, K. T., Huitema, A., Dorlo, T., Peer, C. J., Cordes, L. M., Sciuto, L., et al. (2020). Population pharmacokinetic analysis of nanoparticle-bound and free camptothecin after administration of NLG207 in adults with advanced solid tumors. Cancer Chemother. Pharmacol. 86 (4), 475–486. doi:10.1007/s00280-020-04134-9
Shi, J., Fu, C., Su, X., Feng, S., and Wang, S. (2021). Ultrasound-stimulated microbubbles inhibit aggressive phenotypes and promotes radiosensitivity of esophageal squamous cell carcinoma. Bioengineered 12 (1), 3000–3013. doi:10.1080/21655979.2021.1931641
Smyth, E. C., Lagergren, J., Fitzgerald, R. C., Lordick, F., Shah, M. A., Lagergren, P., et al. (2017). Oesophageal cancer. Nat. Rev. Dis. Prim. 3, 17048. doi:10.1038/nrdp.2017.48
Sung, H., Ferlay, J., Siegel, R. L., Laversanne, M., Soerjomataram, I., Jemal, A., et al. (2021). Global cancer statistics 2020: GLOBOCAN estimates of incidence and mortality worldwide for 36 cancers in 185 countries. CA Cancer J. Clin. 71 (3), 209–249. doi:10.3322/caac.21660
Uckert, W., Kammertöns, T., Haack, K., Qin, Z., Gebert, J., Schendel, D. J., et al. (1998). Double suicide gene (cytosine deaminase and herpes simplex virus thymidine kinase) but not single gene transfer allows reliable elimination of tumor cells in vivo. Hum. Gene Ther. 9 (6), 855–865. doi:10.1089/hum.1998.9.6-855
Wang, J., Xu, L., Liu, X., Yang, R., and Wang, D. (2020). A facile adenosine triphosphate-responsive nanoplatform for efficacious therapy of esophageal cancer. Oncol. Lett. 20 (4), 1. doi:10.3892/ol.2020.11969
Wang, S., Liu, J., Qiu, S., and Yu, J. (2019). Facile fabrication of Cu(9)-S(5) loaded core-shell nanoparticles for near infrared radiation mediated tumor therapeutic strategy in human esophageal squamous carcinoma cells nursing care of esophageal cancer patients. J. Photochem Photobiol. B 199, 111583. doi:10.1016/j.jphotobiol.2019.111583
Wang, X. S., Kong, D. J., Lin, T. Y., Li, X. c., Izumiya, Y., Ding, X. z., et al. (2017). A versatile nanoplatform for synergistic combination therapy to treat human esophageal cancer. Acta Pharmacol. Sin. 38 (6), 931–942. doi:10.1038/aps.2017.43
Xiang, Z., Dong, X., Sun, Q., Li, X., and Yan, B. (2014). Clinical significance of up-regulated miR-181a in prognosis and progression of esophageal cancer. Acta Biochim. Biophys. Sin. (Shanghai). 46 (11), 1007–1010. doi:10.1093/abbs/gmu083
Yang, B., Liu, W., Li, M., and Mo, J. (2022). GSK-J1-loaded, hyaluronic acid-decorated metal-organic frameworks for the treatment of ovarian cancer. Front. Pharmacol. 13, 1023719. doi:10.3389/fphar.2022.1023719
Yoneda, A., Kuroki, T., and Eguchi, S. (2021). Immunotherapeutic advances in gastric cancer. Surg. Today 51 (11), 1727–1735. doi:10.1007/s00595-021-02236-2
Yoneshima, Y., Morita, S., Ando, M., Nakamura, A., Iwasawa, S., Yoshioka, H., et al. (2021). Phase 3 trial comparing nanoparticle albumin-bound paclitaxel with docetaxel for previously treated advanced NSCLC. J. Thorac. Oncol. 16 (9), 1523–1532. doi:10.1016/j.jtho.2021.03.027
Yu, C., Wo, F., Shao, Y., Dai, X., and Chu, M. (2013). Bovine serum albumin nanospheres synchronously encapsulating "gold selenium/gold" nanoparticles and photosensitizer for high-efficiency cancer phototherapy. Appl. Biochem. Biotechnol. 169 (5), 1566–1578. doi:10.1007/s12010-012-0078-x
Zhan, W., Li, H., Guo, Y., Du, G., Wu, Y., and Zhang, D. (2020). Construction of biocompatible dual-drug loaded complicated nanoparticles for in vivo improvement of synergistic chemotherapy in esophageal cancer. Front. Oncol. 10, 622. doi:10.3389/fonc.2020.00622
Zhang, L., Yao, M., Yan, W., Liu, X., Jiang, B., Qian, Z., et al. (2017). Delivery of a chemotherapeutic drug using novel hollow carbon spheres for esophageal cancer treatment. Int. J. Nanomedicine 12, 6759–6769. doi:10.2147/ijn.s142916
Zhang, X., Wang, M., Feng, J., Qin, B., Zhang, C., Zhu, C., et al. (2022). Multifunctional nanoparticles co-loaded with Adriamycin and MDR-targeting siRNAs for treatment of chemotherapy-resistant esophageal cancer. J. Nanobiotechnology 20 (1), 166. doi:10.1186/s12951-022-01377-x
Zhao, L., Zhang, X., Wang, X., Guan, X., Zhang, W., and Ma, J. (2021). Recent advances in selective photothermal therapy of tumor. J. Nanobiotechnology 19 (1), 335. doi:10.1186/s12951-021-01080-3
Zhou, W., Liu, Z., Wang, N., Chen, X., Sun, X., and Cheng, Y. (2022). Hafnium-based metal-organic framework nanoparticles as a radiosensitizer to improve radiotherapy efficacy in esophageal cancer. ACS Omega 7 (14), 12021–12029. doi:10.1021/acsomega.2c00223
Zhou, X., You, M., Wang, F., Wang, Z., Gao, X., Jing, C., et al. (2021). Multifunctional graphdiyne-cerium oxide nanozymes facilitate MicroRNA delivery and attenuate tumor hypoxia for highly efficient radiotherapy of esophageal cancer. Adv. Mater 33 (24), e2100556. doi:10.1002/adma.202100556
Zmerli, I., Ibrahim, N., Cressey, P., Denis, S., and Makky, A. (2021). Design and synthesis of new PEGylated polydopamine-based nanoconstructs bearing ROS-responsive linkers and a photosensitizer for bimodal photothermal and photodynamic therapies against cancer. Mol. Pharm. 18 (9), 3623–3637. doi:10.1021/acs.molpharmaceut.1c00597
Keywords: esophageal cancer, nanoparticles, anti-cancer, diagnosis, treatment
Citation: Xiao Q, Zhang Y, Zhao A, Duan Z and Yao J (2023) Application and development of nanomaterials in the diagnosis and treatment of esophageal cancer. Front. Bioeng. Biotechnol. 11:1268454. doi: 10.3389/fbioe.2023.1268454
Received: 28 July 2023; Accepted: 24 October 2023;
Published: 03 November 2023.
Edited by:
Maoquan Chu, Tongji University, ChinaReviewed by:
Jingxin Mo, University of New South Wales, AustraliaZixiu Du, Shanghai Jiao Tong University, China
Copyright © 2023 Xiao, Zhang, Zhao, Duan and Yao. This is an open-access article distributed under the terms of the Creative Commons Attribution License (CC BY). The use, distribution or reproduction in other forums is permitted, provided the original author(s) and the copyright owner(s) are credited and that the original publication in this journal is cited, in accordance with accepted academic practice. No use, distribution or reproduction is permitted which does not comply with these terms.
*Correspondence: Jun Yao, yaojun74@163.com