- 1Department of Otorhinolaryngology, Longgang Otorhinolaryngology Hospital & Shenzhen Key Laboratory of Otorhinolaryngology, Shenzhen Institute of Otorhinolaryngology, Shenzhen, China
- 2Shanghai Ninth People’s Hospital Hainan Branch, Hainan Western Central Hospital, Danzhou, China
- 3Affiliated Cancer Hospital and Institute of Guangzhou Medical University, Guangzhou, China
- 4School of Computer Science and Engineering, Yulin Normal University, Yulin, China
Cancer is one of the most lethal diseases in human society, and its incidence is gradually increasing. However, the current tumor treatment often meets the problem of poor efficacy and big side effects. The unique physical and chemical properties of nanomaterials can target the delivery of drugs to tumors, which can improve the therapeutic effect while reducing the damage of drugs to normal cells. This makes nanomaterials become a hot topic in the field of biomedicine. This review summarizes the recent progress of nanomaterials in tumor targeted therapy.
1 Introduction
Malignant tumor is a new organism, that is seriously disturbed in the regulation of cell growth under the action of various tumorigenic factors, resulting in the abnormal proliferation of cells in the body. It often presents as abnormal tissue clumps in the body. Tumors can escape the surveillance of the immune system, grow without limit, and metastasize through blood, lymphatic, or implantation (Robert, 2013). There are still great challenges in the treatment of malignant tumors. In 2022, 1918030 new cancer cases and 609360 cancer deaths are projected to occur in the United States. Although the incidence of lung cancer is gradually slowing down, the incidence of breast cancer and advanced stage prostate cancer is still increasing (Siegel and Miller, 2022). According to the National Cancer Center of China, the number of new cases of malignant tumor in China in 2016 was 4.0640 million, of which 2.2343 million were men and 1.829,600 were women. The crude and age-standardized incidence rates (ASIR) were 293.91 and 186.46 per 100,000 population, respectively (Zheng et al., 2022). Nowadays, the traditional treatment methods are mainly surgery, chemotherapy and radiotherapy. However, the therapeutic effect is still not satisfactory. The main reasons include side effects, drug resistance and insensitivity of tumor cells to radiation (Zhang et al., 2018). Therefore, the search for a highly targeted, efficient and low toxicity therapy has become an important direction in cancer therapy research.
At present, many targeted drugs have been applied in clinical practice, which have the advantages of good targeting and few side effects. Targeted therapy can inhibit the growth of tumor cells by targeting drugs on key genes of tumor growth and division, such as EGFR, HER-2, KRAS, ALK, etc (Rouviere et al., 2015; Saito et al., 2018; Meric-Bernstam et al., 2019). However, the mutation rate of target genes in patient population is not high, so many patients are not suitable for using targeted drugs. In addition, in clinical practice, targeted drugs may miss the target. Long-term use is easy to resist drugs, and the price is expensive. Therefore, it has broad application prospect to find a new therapeutic method which is applicable to a wide population and stable targeting performance. At present, the commonly used targeted drugs in clinical practice include Bevacizumab, Trastuzumab, Cetuximab, Pertuzumab, Osimertinib, Lenvatinib, etc. These targeted drugs can mainly be used in the treatment of lung cancer, bowel cancer, breast cancer, liver cancer, lymphoma.
Nanoparticles exhibit unique physical, chemical, and biological properties. They find wide-ranging applications in fields such as biomedical research (Schmalz et al., 2017). The characteristics of small size and large area to volume ratio of nanoparticles enable them to efficiently bind, absorb and deliver small molecules of drugs (Yetisgin and Cetinel, 2020). Their variable size, shape and surface characteristics also enable them to have high stability, high carrying capacity, properties of binding hydrophilic or hydrophobic substances, and compatibility of different drug delivery routes. Because of their own advantages, nanomaterials can play a certain advantage in tumor treatment. Nanomaterials can wrap drugs or combine drugs to be targeted and delivered near the tumor area, so that drugs can act on tumor cells more accurately. The targeted therapy of nanomaterials can be divided into passive and active targeting according to different targeting pathways (Alavi and Hamidi, 2019). The former refers to that after the combination of nanomaterials and therapeutic drugs enters the human body, taking into account the differences in the specific biochemical microenvironment of organs, tissues, cells and specific lesions, the drugs will stop, stay or accumulate in special sites, so as to increase the efficacy and concentration of drugs at the tumor site, so as to achieve targeted treatment. The latter uses artificial means to deliver nanomaterials and drugs near the tumor site for selective treatment (Figure 1).
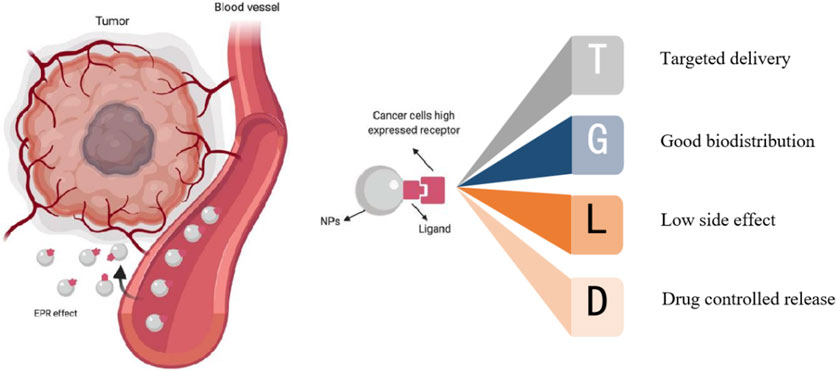
FIGURE 1. Targeting of nanoparticles: active targeting and passive targeting (Sanità et al., 2020).
There is a difference between targeted drugs and nanomaterial targeted therapy, targeted drugs are targeted to the tumor site through the specific binding of drug molecules to specific receptors on the surface of tumor cells, while nanomaterial is targeted to the tumor site through the unique physical and chemical properties of nanomaterials, such as magnetic properties, EPR effects, and binding tumor recognition ligands. Compared with direct intravenous drug input, encapsulation or combination of nanomaterials has the following advantages (Hou et al., 2020; Barani et al., 2021; Dutta et al., 2021; Kashyap et al., 2023): 1) It can improve drug targeting for tumor and reduce the damage to normal tissues and organs. 2) It can improve drug stability, reduce drug delivery by enzymatic hydrolysis, resulting in drug destruction. It can reduce the side effect of anticancer agents. 3) It can make the drug in the body controlled release. For example, the prodrug nanoassemblies (LPNAs) and the pH-reduction dual responsive drug delivery system designed by Ding et al. (2019) can precisely control drug release, resulting in better therapeutic effects (Ding et al., 2019; Ding et al., 2023). In addition, while delivering drugs, nanomaterials can also produce photothermal effects and enhance the killing effect on tumors. Based on the numerous advantages of nanomaterials, nanomaterials have been widely used in the drug-loaded targeted therapy of cancer. This paper will review the research progress in this direction, and provide certain reference value for the subsequent research.
2 Commonly used nanomaterials
Due to the numerous advantages, nanomaterials have been wildly used in clinical and laboratory researches (Figure 2). In this section, we summary the commonly used nanomaterials in cancer therapy, including organic nanomaterials and inorganic nanomaterials (Khalid et al., 2020). At present, some nano-drugs have been applied in clinical practice. For example, Abraxane and Paclitaxel liposome are commonly used in the treatment of breast cancer, ovarian cancer, and non-small cell lung cancer. Adriamycin liposomes is often used in the treatment of ovarian cancer, non-Hodgkin lymphoma, breast cancer, and uterine tumors.
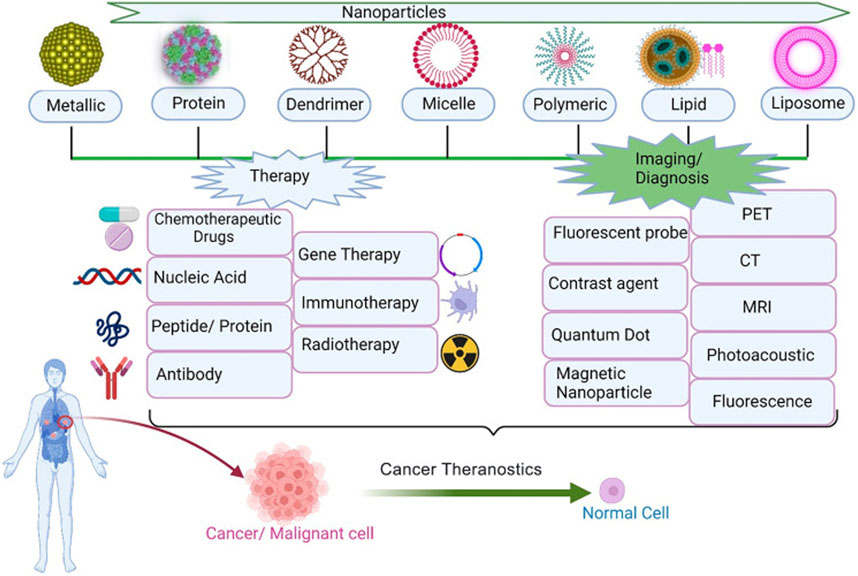
FIGURE 2. Application of nanoparticles in tumor therapy and diagnosis (Kashyap et al., 2023).
2.1 Organic nanomaterials
Organic nanomaterials are substances that mostly naturally existing in organisms or synthesized by chemicals. Such nanomaterials are less cytotoxic and biodegradable, so they are currently a research hotspot and widely used (Khizar et al., 2023). Organic nanomaterials have the advantages of controllable size, large specific surface area, easy surface modification, high permeability and retention effect, and good biocompatibility (López-Dávila et al., 2012; Hussein Kamareddine et al., 2019). Organic nanomaterials can carry both hydrophilic and hydrophobic drugs. Modification of endogenous molecules of some receptors on the surface of organic nanomaterials can significantly improve the targeting of tumor tissue. At present, organic nanomaterials mainly include liposome, protein and polymeric nanomaterials (Palazzolo et al., 2018; Wang et al., 2021).
2.1.1 Liposome nanomaterials
Liposomes are nanocapsules with closed spherical vesicle structure surrounded by phospholipid bilayer and contain hydrophilic water nuclei, which can bind both hydrophilic and hydrophobic drugs (Yang et al., 2021). Compared with other nanocarriers, liposome show good biocompatibility and high drug loading capacity, showing lower toxicity, low immunogenicity, and can be cleared through normal metabolism.
At present, the clinical application of liposome preparations contain different chemotherapy drugs, such as Doxil1/Caelyx1/Myocet1, DaunoXome1, and DepoCyte1, respectively, were used to treat patients with ovarian cancer, AIDS-associated Kaposi’s sarcoma, multiple myeloma, lymphoma, or leukemia combined with meningeal diffusion (Kaspers et al., 2013; Bhowmik et al., 2018; Le Rhun et al., 2020). In addition, there are a number of drugs are being studied. Anthracyclines (ANT) are aromatic polyketo antitumor drugs, representative of which are doxorubicin (DOX), daunorubicin (DNR), aclarubicin, epirubicin, pirarubicin, etc. Doxil is FDA approved for the treatment of ovarian cancer and Kaposi’s sarcoma (Addeo et al., 2008). One of the most common dose-limiting toxicities of anthracyclines is cardiotoxicity, especially in the elderly and in patients with severe complications such as diabetes and coronary heart disease. However, cardiotoxic events with anthracyclines encapsulated in liposomes are significantly reduced during tumor therapy (Fassas et al., 2002). As is shown in Figure 3A, Yoshizaki et al. (2016) a pH-sensitive fusogenic polymer-modified liposomes. The liposomes were loaded with antigenic peptides derived from ovalbumin (OVA) OVA-I (SIINFEKL), and OVA-II, which can significantly enhance the activation efficiency of cytotoxic T lymphocyte (ctl), so as to achieve efficient cancer immunotherapy. Matbou Riahi et al. (2018) developed celecoxib (CLX) liposome nanomaterials to overcome the shortcomings of poor water solubility and low anti-tumor titer, and found that CLX liposome preparation had the slowest release curve and the strongest anti-tumor effect in vivo. Compared with free CLX, the accumulation of CLX liposomes in tumor sites increased by 3 times. It is proved that CLX liposome is a safe and effective antitumor agent and can release the drug slowly. Wang S. et al. (2020b) used the copper acetate gradient method to prepare As2O3 liposomes. By forming a complex of aqueous copper acetate and As2O3 in the liposomes, the encapsulation rate reached 83.1%, the tumor inhibition rate reached 61.2%, and the toxicity of arsenic trioxide (ATO) was greatly reduced. In vivo pharmacokinetic experiments showed that plasma clearance rate of As2O3 liposomes was significantly reduced, and the in vivo circulation time was doubled compared with intravenous injection of As2O3 solution, which improved drug distribution in tumor tissues. Further research showed that the strong skeleton formed by arsenic and metal ions increased the stability of the drug, and the liposome lasted in the body for 6 months longer than the original.
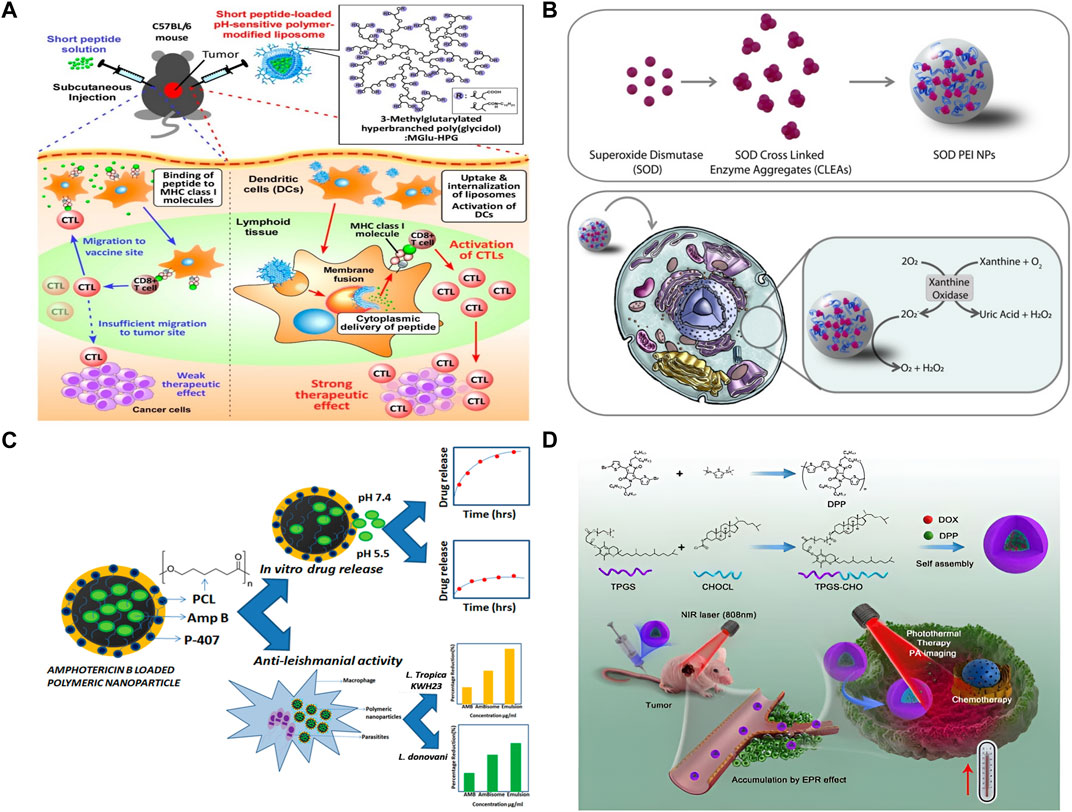
FIGURE 3. Organic nanomaterial. (A) Antigen delivery of pH-sensitive polymer-modified liposomes (Yoshizaki et al., 2016). (B) The synthesis, in vitro assay, and activity in cell of SOD NPs (Galliani et al., 2019). (C) From Amphotericin B (Amp B) loaded polymeric nanoparticles preparation to in vitro drug release (Saqib et al., 2020). (D) Polymer-Based nanocarriers for Co-Delivery and combination of diverse therapies against cancers (Yan et al., 2018).
2.1.2 Protein nanomaterials
Protein nanomaterials is a kind of nano-drug loading system composed of drugs and animal, plant or recombinant proteins as carriers. Protein nanomaterials have the advantages of biodegradability, good biocompatibility, low immunogenicity, high drug-loading and stability, and can accumulate in tumor through EPR effect (Ding et al., 2020; Carrion et al., 2021). The types of protein nanomaterials commonly used in tumor therapy include albumin, whey protein, lipoprotein, zein, soy protein, recombinant ferritin and filamentin (Katouzian and Jafari, 2019).
Wamel’s (van Wamel et al., 2016) study showed that paclitaxel albumin nanoparticle (Abraxane) uses human serum albumin as a carrier, and through the natural transport pathway of albumin in the body, it can rapidly distribute and aggregate in tumor tissues, improve the efficacy of chemotherapy and reduce toxicity. Jayaprakasha et al. (2016) prepared whey protein-coated curcumin nanoparticles by solvent removal method. Compared with free curcumin, the uptake of colon cancer cells and prostate cancer cells increased significantly. The cytotoxicity and bioavailability of nano curcumin were significantly improved. Wu et al. (2013) prepared paclitaxel-fibroin nanoparticles (PTX-SF-NPs) in aqueous solution at room temperature for the treatment of local gastric cancer. The anti-tumor effect of PTX-SF-NPs was evaluated on the in vitro model of gastric cancer in nude mice, and the results showed that PTX-SF-NPs significantly enhanced the effect of delaying tumor growth and reducing tumor weight, with high safety. Sonekar et al. (2016) prepared folic acid conjugated curcumin-melololin nanoparticles by desolvation method, which can be taken orally and targeted for delivery to colon cancer cells, significantly improving the bioavailability and targeting of drugs.
2.1.3 Polymeric nanomaterials
Polymeric nanoparticles are colloidal systems with a wide size range (10–1000 nm). Polymeric nanoparticles have high immunogenicity and stability, and can effectively encapsulate and display antigens. Polymeric nanoparticles can effectively protect drugs from in vitro and in vivo degradation, cross the blood-brain barrier, control drug release sites and improve drug targeting, and thus have a better therapeutic prospect in the targeted delivery of anti-tumor drugs (Elzoghby, 2017). In general, polymeric nanocarriers are more stable than liposomes. Common polymeric nanomedicine delivery carriers include polymeric micelles, dendritic macromolecules, polymeric nanogels, polymeric nanospheres, etc (Salari et al., 2022).
As is shown in Figure 3B, Galliani et al. (2019) developed a PLGA-based nanostructure, which can protect the structure of the enzymes from being destroyed and accurately deliver it to the cytoplasm. In the Figure 3C, Polyacaprolactone (PCL) nanoparticles loaded with Amp B were developed. The IC50 of the nanoformulations was significantly lower as compared to free Amp B, so it can greatly enhance the effect of Amp B. Wang et al. (2017) prepared gemcitabine (GEM) chitosan nanoparticles (FA-PEG-GEM-NPs) with surface modification of folic acid (FA) and polyethylene glycol (PEG). It was confirmed by cell experiments that FA-PEG-GEM-NPs had high selectivity and high toxicity to human non-small cell lung cancer A549 cells. Wu et al. (2018) designed a stimulus-responsive drug delivery system combining radiotherapy and chemotherapy. They modified L-cysteine (L-Cys) on the surface of polyamidoamine (PAMAM) polymer. On the one hand, the sulfhydryl group of L-Cys can act as a radiation protection agent by removing free radicals. On the other hand, disulfide bonds formed by sulfhydryl groups can be used to trigger the release of anti-cancer drugs. Low doses of gamma rays (5 Gy) trigger reactive oxygen species (ROS) production, which breaks disulfide bonds and releases anticancer drugs DOX. Gu et al. (Wang et al., 2018) designed a therapeutic nano hydrogel. When injected in situ into the tumor, the coated gemcitabine (GEM) and immune checkpoint inhibitor anti-PD-L1 antibody (aPDL1) were released in response, realizing the synergistic tumor suppression by chemotherapy and immunization. This gel can achieve ROS responsive degradation and programmed release of therapeutic drugs in ROS rich tumor microenvironments. The results showed that nano gel containing aPDL1-GEM significantly promoted immune-mediated tumor killing in tumor-bearing mice, thus it greatly prevented the recurrence of tumor after resection. Docetaxel (DTX) is a first-line chemotherapy drug for the treatment of metastatic breast cancer. Encapsulated in alendronate (AlN)-modified micelles, docetaxel can achieve sustained release and improve pharmacokinetics. DTX micelles can inhibit tumor growth and significantly prolong animal survival in a model of advanced disseminated breast cancer with bone metastases (Liu, 2019). Yan et al. Repoated a polymer-Based nanocarrier for Co-Delivery and combination of diverse therapies against cancers (Figure 3D).
2.2 Inorganic nanomaterials
Inorganic nanomaterials are nanocarriers synthesized by metallic and semi-metallic materials, which can be used for drug delivery. At present, inorganic nanomaterials used in tumor therapy mainly include metal nanomaterials, non-metallic nanomaterials, magnetic nanomaterials, etc, (Pei et al., 2023). Inorganic nanomaterials are easy to prepare and modify, which has become one of the important research directions of nanocarriers.
2.2.1 Metallic nanomaterials
Metallic nanomaterials, which are synthesized based on metallic elements, not only have the function of targeted drug delivery of conventional carriers, but also enhance the ability of chemotherapy drugs to interfere with cell metabolism, inhibit proliferative cell signal transduction and induce cell apoptosis (Jin et al., 2018).
In the Figure 4A, a hydrogen peroxide (H2O2)-triggered nanomaterial (LV-TAX/Au@Ag) was developed. When the nanomaterial reach the tumor tissues, it will release taxol and recovere the photothermal properties of AuNRs. With this combined chemo-photothermal therapy, tumor growth was significantly inhibited. Sun et al. (2015) prepared BioMOF ZnBTCA as a drug delivery vector to promote the uptake and release of anticancer drugs, which showed strong cytotoxicity to A2780cis (ovarian cancer cells) in vitro. ZnBTCA itself is not significantly cytotoxic to cancer cells, while drug@ZnBTCA will release the drug continuously and slowly to inhibit the cell growth of A2780cis. Compared with free drugs, drug@ZnBTCA reduces toxicity to normal cells. Tomuleasa et al. (2012) studied the therapeutic effects of different Au NPs loaded with adriamycin, cisplatin or capecitabine respectively on liver cancer, and the drug molecules were non-covalently combined with aspartic acid coated Au NPs. Compared with free drugs, Au NP-drug conjugations significantly improved the therapeutic efficacy of tumor cells, and were equally effective on drug-resistant cells. Rotello et al. (Zhu et al., 2008) demonstrated that Au NPs with surface charge and hydrophobicity are very conducive to cell uptake. Silver nanomaterials (Ag NPs) exhibit dose-dependent toxicity to tumor cells, killing tumor cells by inducing oxidative stress and DNA damage. TNBC tumor cells are sensitive to oxidative stress and DNA damage. Ag NPs have high selectivity for TNBC tumor cells, and have little damage to normal breast tissue cells, liver, kidney, and monocyte system cells (Simard et al., 2016). Curcumin and its derivatives are effective on TNBC tumor cells cultured in vitro, and it has been found that curcumin has greater damage to normal cells in vivo experiments. Li et al. (2017) found that in TNBC mouse model, the use of copper nanocomplex coating curcumin can improve its anticancer activity without significant adverse reactions (Li et al., 2017).
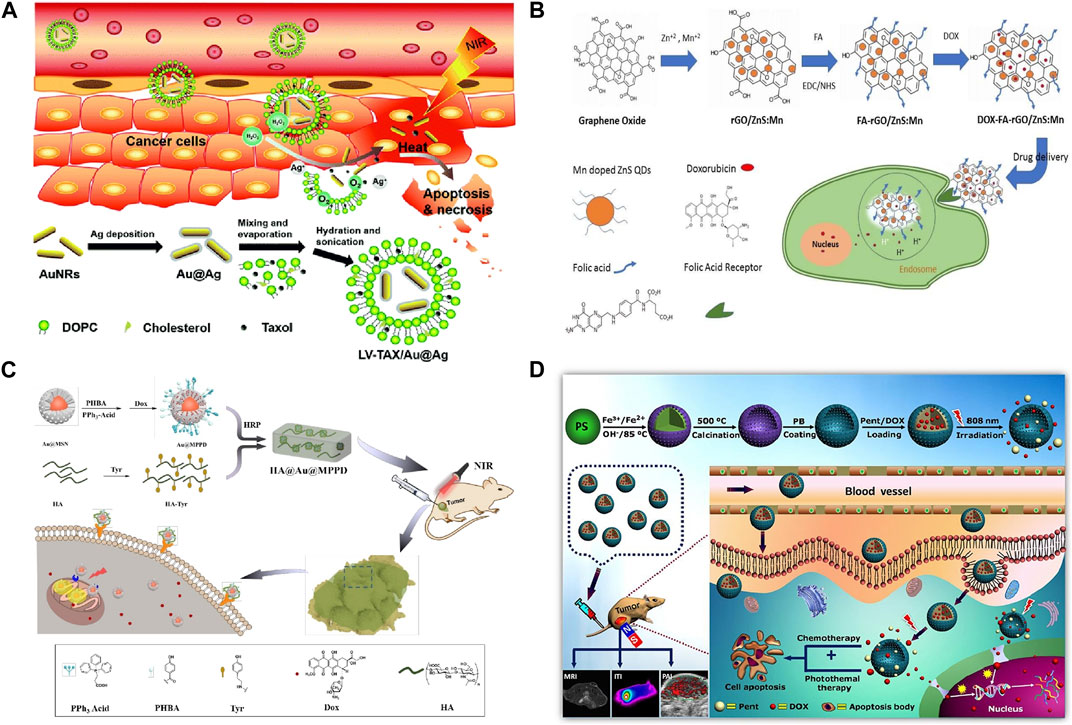
FIGURE 4. (A) Combination therapy of LV-TAX/Au@Ag (Wang K. et al., 2020a). (B) FA-rGO/ZnS:Mn as a drug delivery system to release DOX into the cancer cell nucleus (Diaz-Diestra et al., 2018). (C) FA-rGO/ZnS:Mn as a drug delivery system to release DOX into the cancer cell nucleus (Zhou et al., 2020). (D) Magnetic nanoparticles enhance drug targeting through magnetic fields (Estelrich et al., 2015; Gul et al., 2019).
2.2.2 Nonmetallic nanomaterials
At present, the most widely used nonmetallic nanomaterials mainly include carbon nanomaterials and silica nanomaterials. Mesoporous silica nanoparticles (MSN) have a larger specific surface area due to their unique mesoporous structure, and thus have better drug loading capacity.
In the Figure 4B; Diaz-Diestra et al. (2018) developed a nanocomposite (FA-rGO/ZnS:Mn). This nanocomposite can increase the drug loading rate and enhance the anti-tumor effect without increasing the toxicity to normal cells. Xiong et al. (2015) designed a novel MSN modified with two ligand (folic acid and dexamethasone), as a targeted delivery system for tumor nuclei. Folic acid modification can increase the targeting ability of MSN to tumor cells. Dexamethasone is a glucocorticoid, which can bind to the nuclear receptor of tumor cells, namely glucocorticoid receptor, and the formed dexamethasone-glucocorticoid receptor complex can be actively transported from cytoplasm to nucleus. By combining these two ligand to MSN, the novel delivery vector will target DOX into the tumor nucleus and increase its accumulation in the nucleus, thereby improving the inhibition of HeLa (human cervical cancer cells) and reducing the toxic and side effects on normal cells through receptor-mediated cell selectivity. Kumar et al. (2017) successfully prepared DOX-FA-MSNPs nanomaterials loaded with doxorubicin based on MSNs, and examined the uptake at the level of breast cancer cells by confocal microscopy and flow cytometry, and the results indicated that the uptake of nanomaterials in cell lines was high. Cytotoxic results showed that DOX-FA-MSNPs had higher cytotoxic effects on breast cancer cells. Khodadadei et al. (2017) loaded methotrexate (MTX) onto nitrogen-containing graphene quantum dots (GQDs) and found that its entry into tumor cells was slower than that of free MTX, but its effect time was longer and it had strong cytotoxicity. Liu et al. (2022) prepared nano-scale functional hydroxyapatite particles by reversibly combining hydroxyapatite with adriamycin. The nanoparticle is applied locally to the lesion area and can enter the lysosome of tumor cells. In acidic environment, the combination of hydroxyapatite and adriamycin is destroyed, and the released adriamycin accumulates in mitochondria. Further tests on osteosarcoma mice showed that local delivery of adriamycin via hydroxyapatite granules had a stronger tumor eradication effect than traditional application of adriamycin. Mesoporous silica is also a commonly used nanomaterial. A hyaluronic acid (HA) hydrogel covalently embedded with doxorubicin loaded and triphenylphosphine (TPP) modified core-shell gold mesoporous silica nanoparticles was developed by Zhou et al. (2020) This nanomaterial can accurately target tumor cells via CD44 and generate chemophotothermal synergistic cancer therapy (Figure 4C).
2.2.3 Magnetic nanomaterials
Magnetic nanoparticles (MNPs) have magnetic properties and can be targeted to the target area by an external magnetic field. MNPs are generally magnetic composite materials composed of iron, nickel, cobalt and other metals and their oxides, which have been extensively studied in tumor targeted drug delivery. At present, magnetic nanocarriers used for tumor therapy mainly include core-shell structure nanoparticles (made from silica or polymeric micelles wrapped magnetic nuclei and drugs) (Oh and Park, 2011), magnetic liposomes (Song et al., 2020), and magnetic nanoparticles (transport modes are determined by particle size, morphology, surface charge, surface-to-coupled drugs or targeted molecules) (de Smet et al., 2011). Magnetic ferric oxide nanoparticles (MNP-Fe3O4) are magnetic nanomaterials approved by FDA for clinical application, which have attracted wide attention in the field of tumor targeted therapy (Zhao et al., 2018). In addition, multifunctional magnetic nanoagents (MMNs) is of great value for cancer precision therapy (Ding et al., 2021).
Figure 4D shows the process of a magnetic nanomaterial accurately delivering drugs to tumor tissues through magnetic fields, and killing tumors in coordination with photothermal effects. Wu et al. (2020) developed) a novel chitosan superparamagnetic ferric oxide nanocrystals (PECs), loaded with indocyanine green (ICG) fluorescent dye and irinotecan (IRT). By applying magnetic field, real-time fluorescence monitoring of the efficiency of magnetic targeted drug delivery to the tumour was realized. Centelles et al. (2018) designed imaged thermosensitive liposomes (iTSLs) for targeted Hycamtin delivery. iTSL accumulation at the tumor site can be detected by near infrared fluorescence imaging (NIRF), and its release at the tumor site can be detected by topotecan enhanced fluorescence. High intensity focused ultrasound (HIFU) can promote drug release from liposomes in vivo, induce subablative hyperthermia, change the permeability of tumor vessels and enhance the absorption of nanoparticles. Shao et al. (2016) designed a kind of magnetic Fe3O4/mesoporous silicon “nano bullet” particles. The magnetic Fe3O4 nanoparticles of “nano bullet” can provide magnetic targeting ability, and mesoporous silicon can adsorb chemotherapy drugs Dox. Therefore, the designed “nanobullets” can be targeted and enriched to the liver cancer area under the effect of magnetic targeting to realize the chemotherapy effect on tumors. Lu et al. (2018) constructed a PH-sensitive dual-targeting magnetic nanoparticle, and prepared graphene oxide (MGO) by deposition of Fe3O3 magnetic nanoparticles on graphene oxide (GO) by chemical coprecipitation. MGO- PEG-CET was obtained by modifying MGO with polyethylene glycol (PEG) and cetuximab (CET). EGFR is highly expressed on tumor cell surface, so MGO-PEG-CET can be used for dual targeted delivery of DOX.
3 Summary and outlook
Drug loading and targeted delivery of nanomaterials is one of the hot spots in biomedical research. Although many studies have demonstrated their advantages, most nanomaterials are still in the experimental stage and need to be improved in many aspects: 1) The biological toxicity of materials should be reduced as much as possible; 2) The pharmacokinetic characteristics, metabolism, distribution and cumulative effects in vivo need to be clarified. 3) How to improve the drug loading rate and the controllability of drug release of nanomaterials more effectively; 4) To improve the targeting of nanomaterials and reduce the toxicity to normal cells as much as possible. The real clinical use of nanomaterials requires more interdisciplinary and cross-field collaborative research. With the deepening of various researches, the application of nanomaterials will continue to make breakthrough progress.
Author contributions
PZ, JL, XZ, and WJ: conceptualization, project administration, funding acquisition and editing. PZ, GY, and GX: writing—Review and editing. All authors contributed to the article and approved the submitted version.
Funding
The present study was supported in part by the Guangdong Basic and Applied Basic Research Foundation (2021A1515010970); Shenzhen Innovation of Science and Technology Commission (Nos. JCYJ20210324132407019, LGKCYLWS2022002, LGKCYLWS2021000027, and LGKCYLWS2020099); Shenzhen Key Medical Discipline Construction Fund (No. SZXK039).
Conflict of interest
The authors declare that the research was conducted in the absence of any commercial or financial relationships that could be construed as a potential conflict of interest.
Publisher’s note
All claims expressed in this article are solely those of the authors and do not necessarily represent those of their affiliated organizations, or those of the publisher, the editors and the reviewers. Any product that may be evaluated in this article, or claim that may be made by its manufacturer, is not guaranteed or endorsed by the publisher.
References
Addeo, R., Faiola, V., Guarrasi, R., Montella, L., Vincenzi, B., Capasso, E., et al. (2008). Liposomal pegylated doxorubicin plus vinorelbine combination as first-line chemotherapy for metastatic breast cancer in elderly women ≥65 years of age. Cancer Chemother. Pharmacol. 62 (2), 285–292. doi:10.1007/s00280-007-0605-6
Alavi, M., and Hamidi, M. (2019). Passive and active targeting in cancer therapy by liposomes and lipid nanoparticles. Drug Metab. Pers. Ther. 34 (1). doi:10.1515/dmpt-2018-0032
Barani, M., Bilal, M., Sabir, F., Rahdar, A., and Kyzas, G. Z. (2021). Nanotechnology in ovarian cancer: Diagnosis and treatment. Life Sci. 266, 118914. doi:10.1016/j.lfs.2020.118914
Bhowmik, S., Bhowmick, S., Maiti, K., Chakra, A., Shahi, P., Jain, D., et al. (2018). Two multicenter Phase I randomized trials to compare the bioequivalence and safety of a generic doxorubicin hydrochloride liposome injection with Doxil(®) or Caelyx(®) in advanced ovarian cancer. Cancer Chemother. Pharmacol. 82 (3), 521–532. doi:10.1007/s00280-018-3643-3
Carrion, C. C., Nasrollahzadeh, M., Sajjadi, M., Jaleh, B., Soufi, G. J., and Iravani, S. (2021). Lignin, lipid, protein, hyaluronic acid, starch, cellulose, gum, pectin, alginate and chitosan-based nanomaterials for cancer nanotherapy: Challenges and opportunities. Int. J. Biol. Macromol. 178, 193–228. doi:10.1016/j.ijbiomac.2021.02.123
Centelles, M. N., Wright, M., So, P. W., Amrahli, M., Xu, X. Y., Stebbing, J., et al. (2018). Image-guided thermosensitive liposomes for focused ultrasound drug delivery: Using NIRF-labelled lipids and topotecan to visualise the effects of hyperthermia in tumours. J. Control Release 280, 87–98. doi:10.1016/j.jconrel.2018.04.047
de Smet, M., Heijman, E., Langereis, S., Hijnen, N. M., and Grüll, H. (2011). Magnetic resonance imaging of high intensity focused ultrasound mediated drug delivery from temperature-sensitive liposomes: An in vivo proof-of-concept study. J. Control Release 150 (1), 102–110. doi:10.1016/j.jconrel.2010.10.036
Diaz-Diestra, D., Thapa, B., Badillo-Diaz, D., Beltran-Huarac, J., Morell, G., and Weiner, B. (2018). Graphene oxide/ZnS:Mn nanocomposite functionalized with folic acid as a nontoxic and effective theranostic platform for breast cancer treatment. Nanomater. (Basel). 8 (7), 484. doi:10.3390/nano8070484
Ding, Y., Hu, X., Piao, Y., Huang, R., Xie, L., Yan, X., et al. (2023). Lipid prodrug nanoassemblies via dynamic covalent boronates. ACS Nano 17 (7), 6601–6614. doi:10.1021/acsnano.2c12233
Ding, Y., Liu, J., Li, X., Xu, L., Li, C., Ma, L., et al. (2019). Rational design of drug delivery systems for potential programmable drug release and improved therapeutic effect. Mater. Chem. Front. 3 (6), 1159–1167. doi:10.1039/C9QM00178F
Ding, Y., Xu, Y., Yang, W., Niu, P., Li, X., Chen, Y., et al. (2020). Investigating the EPR effect of nanomedicines in human renal tumors via ex vivo perfusion strategy. Nano Today 35, 100970. doi:10.1016/j.nantod.2020.100970
Ding, Y., Zeng, L., Xiao, X., Chen, T., and Pan, Y. (2021). Multifunctional magnetic nanoagents for bioimaging and therapy. ACS Appl. Bio Mater. 4 (2), 1066–1076. doi:10.1021/acsabm.0c01099
Dutta, B., Barick, K. C., and Hassan, P. A. (2021). Recent advances in active targeting of nanomaterials for anticancer drug delivery. Adv. Colloid Interface Sci. 296, 102509. doi:10.1016/j.cis.2021.102509
Elzoghby, A. O. (2017). Editorial: Polymeric nanocarriers as robust platforms for cancer therapy. Curr. Pharm. Des. 23 (35), 5211–5212. doi:10.2174/1381612823999171106125801
Estelrich, J., Escribano, E., Queralt, J., and Busquets, M. A. (2015). Iron oxide nanoparticles for magnetically-guided and magnetically-responsive drug delivery. Int. J. Mol. Sci. 16 (4), 8070–8101. doi:10.3390/ijms16048070
Fassas, A., Buffels, R., Anagnostopoulos, A., Gacos, E., Vadikolia, C., Haloudis, P., et al. (2002). Safety and early efficacy assessment of liposomal daunorubicin (DaunoXome) in adults with refractory or relapsed acute myeloblastic leukaemia: A phase I-ii study. Br. J. Haematol. 116 (2), 308–315. doi:10.1046/j.0007-1048.2001.03292.x
Galliani, M., Tremolanti, C., and Signore, G. (2019). Nanocarriers for protein delivery to the cytosol: Assessing the endosomal escape of poly(lactide-co-glycolide)-poly(ethylene imine) nanoparticles. Nanomater. (Basel) 9 (4), 652. doi:10.3390/nano9040652
Gul, S., Khan, S. B., Rehman, I. U., Khan, M. A., and Khan, M. I. (2019). A comprehensive review of magnetic nanomaterials modern day theranostics. Front. Mater. 6. doi:10.3389/fmats.2019.00179
Hou, J., Sun, X., Huang, Y., Yang, S., Liu, J., Feng, C., et al. (2020). The design and application of nanomaterials as drug carriers in cancer treatment. Curr. Med. Chem. 27 (36), 6112–6135. doi:10.2174/0929867326666190816231409
Hussein Kamareddine, M., Ghosn, Y., Tawk, A., Elia, C., Alam, W., Makdessi, J., et al. (2019). Organic nanoparticles as drug delivery systems and their potential role in the treatment of chronic myeloid leukemia. Technol. Cancer Res. Treat. 18, 153303381987990. doi:10.1177/1533033819879902
Jayaprakasha, G. K., Chidambara Murthy, K. N., and Patil, B. S. (2016). Enhanced colon cancer chemoprevention of curcumin by nanoencapsulation with whey protein. Eur. J. Pharmacol. 789, 291–300. doi:10.1016/j.ejphar.2016.07.017
Jin, G., He, R., Liu, Q., Dong, Y., Lin, M., Li, W., et al. (2018). Theranostics of triple-negative breast cancer based on conjugated polymer nanoparticles. ACS Appl. Mat. Interfaces 10 (13), 10634–10646. doi:10.1021/acsami.7b14603
Kashyap, B. K., Singh, V. V., Solanki, M. K., Kumar, A., Ruokolainen, J., and Kesari, K. K. (2023). Smart nanomaterials in cancer theranostics: Challenges and opportunities. ACS Omega 8 (16), 14290–14320. doi:10.1021/acsomega.2c07840
Kaspers, G. J., Zimmermann, M., Reinhardt, D., Gibson, B. E., Tamminga, R. Y., Aleinikova, O., et al. (2013). Improved outcome in pediatric relapsed acute myeloid leukemia: Results of a randomized trial on liposomal daunorubicin by the international BFM study group. J. Clin. Oncol. 31 (5), 599–607. doi:10.1200/jco.2012.43.7384
Katouzian, I., and Jafari, S. M. (2019). Protein nanotubes as state-of-the-art nanocarriers: Synthesis methods, simulation and applications. J. Control Release 303, 302–318. doi:10.1016/j.jconrel.2019.04.026
Khalid, K., Tan, X., Mohd Zaid, H. F., Tao, Y., Lye Chew, C., Chu, D. T., et al. (2020). Advanced in developmental organic and inorganic nanomaterial: A review. Bioengineered 11 (1), 328–355. doi:10.1080/21655979.2020.1736240
Khizar, S., Alrushaid, N., Alam Khan, F., Zine, N., Jaffrezic-Renault, N., Errachid, A., et al. (2023). Nanocarriers based novel and effective drug delivery system. Int. J. Pharm. 632, 122570. doi:10.1016/j.ijpharm.2022.122570
Khodadadei, F., Safarian, S., and Ghanbari, N. (2017). Methotrexate-loaded nitrogen-doped graphene quantum dots nanocarriers as an efficient anticancer drug delivery system. Mater Sci. Eng. C Mater Biol. Appl. 79, 280–285. doi:10.1016/j.msec.2017.05.049
Kumar, P., Tambe, P., Paknikar, K. M., and Gajbhiye, V. (2017). Folate/N-acetyl glucosamine conjugated mesoporous silica nanoparticles for targeting breast cancer cells: A comparative study. Colloids Surf. B Biointerfaces 156, 203–212. doi:10.1016/j.colsurfb.2017.05.032
Le Rhun, E., Wallet, J., Mailliez, A., Le Deley, M. C., Rodrigues, I., Boulanger, T., et al. (2020). Intrathecal liposomal cytarabine plus systemic therapy versus systemic chemotherapy alone for newly diagnosed leptomeningeal metastasis from breast cancer. Neuro Oncol. 22 (4), 524–538. doi:10.1093/neuonc/noz201
Li, Y., Jiang, C., Zhang, D., Wang, Y., Ren, X., Ai, K., et al. (2017). Targeted polydopamine nanoparticles enable photoacoustic imaging guided chemo-photothermal synergistic therapy of tumor. Acta Biomater. 47, 124–134. doi:10.1016/j.actbio.2016.10.010
Liu, T., Romanova, S., Wang, S., Hyun, M. A., Zhang, C., Cohen, S. M., et al. (2019). Alendronate-modified polymeric micelles for the treatment of breast cancer bone metastasis. Bone Metastasis 16 (7), 2872–2883. doi:10.1021/acs.molpharmaceut.8b01343
Liu, Y., Nadeem, A., Sebastian, S., Olsson, M. A., Wai, S. N., Styring, E., et al. (2022). Bone mineral: A trojan horse for bone cancers. Efficient mitochondria targeted delivery and tumor eradication with nano hydroxyapatite containing doxorubicin. Mater Today Bio 14, 100227. doi:10.1016/j.mtbio.2022.100227
López-Dávila, V., Seifalian, A. M., and Loizidou, M. (2012). Organic nanocarriers for cancer drug delivery. Curr. Opin. Pharmacol. 12 (4), 414–419. doi:10.1016/j.coph.2012.02.011
Lu, Y. J., Lin, P. Y., Huang, P. H., Kuo, C. Y., Shalumon, K. T., Chen, M. Y., et al. (2018). Magnetic graphene oxide for dual targeted delivery of doxorubicin and photothermal therapy. Nanomater. (Basel). 8 (4), 193. doi:10.3390/nano8040193
Matbou Riahi, M., Sahebkar, A., Sadri, K., Nikoofal-Sahlabadi, S., and Jaafari, M. R. (2018). Stable and sustained release liposomal formulations of celecoxib: In vitro and in vivo anti-tumor evaluation. Int. J. Pharm. 540 (1-2), 89–97. doi:10.1016/j.ijpharm.2018.01.039
Meric-Bernstam, F., Johnson, A. M., Dumbrava, E. E. I., Raghav, K., Balaji, K., Bhatt, M., et al. (2019). Advances in HER2-targeted therapy: Novel agents and opportunities beyond breast and gastric cancer. Clin. Cancer Res. 25 (7), 2033–2041. doi:10.1158/1078-0432.ccr-18-2275
Oh, J. K., and Park, J. M. (2011). Iron oxide-based superparamagnetic polymeric nanomaterials: Design, preparation, and biomedical application. Prog. Polym. Sci. 36 (1), 168–189. doi:10.1016/j.progpolymsci.2010.08.005
Palazzolo, S., Bayda, S., Hadla, M., Caligiuri, I., Corona, G., Toffoli, G., et al. (2018). The clinical translation of organic nanomaterials for cancer therapy: A focus on polymeric nanoparticles, micelles, liposomes and exosomes. Curr. Med. Chem. 25 (34), 4224–4268. doi:10.2174/0929867324666170830113755
Pei, Z., Lei, H., and Cheng, L. (2023). Bioactive inorganic nanomaterials for cancer theranostics. Chem. Soc. Rev. 52 (6), 2031–2081. doi:10.1039/d2cs00352j
Robert, J. (2013). Biology of cancer metastasis. Bull. Cancer 100 (4), 333–342. doi:10.1684/bdc.2013.1724
Rouviere, D., Bousquet, E., Pons, E., Milia, J. D., Guibert, N., and Mazieres, J. (2015). New targets and new drugs in thoracic oncology. Rev. Mal. Respir. 32 (8), 867–876. doi:10.1016/j.rmr.2015.02.091
Saito, M., Suzuki, H., Kono, K., Takenoshita, S., and Kohno, T. (2018). Treatment of lung adenocarcinoma by molecular-targeted therapy and immunotherapy. Surg. Today 48 (1), 1–8. doi:10.1007/s00595-017-1497-7
Salari, N., Faraji, F., Torghabeh, F. M., Faraji, F., Mansouri, K., Abam, F., et al. (2022). Polymer-based drug delivery systems for anticancer drugs: A systematic review. Cancer Treat. Res. Commun. 32, 100605. doi:10.1016/j.ctarc.2022.100605
Sanità, G., Carrese, B., and Lamberti, A. (2020). Nanoparticle surface functionalization: How to improve biocompatibility and cellular internalization. Front. Mol. Biosci. 7, 587012. doi:10.3389/fmolb.2020.587012
Saqib, M., Ali Bhatti, A. S., Ahmad, N. M., Ahmed, N., Shahnaz, G., Lebaz, N., et al. (2020). Amphotericin B loaded polymeric nanoparticles for treatment of leishmania infections. Nanomater. (Basel) 10 (6), 1152. doi:10.3390/nano10061152
Schmalz, G., Hickel, R., van Landuyt, K. L., and Reichl, F. X. (2017). Nanoparticles in dentistry. Dent. Mater 33 (11), 1298–1314. doi:10.1016/j.dental.2017.08.193
Shao, D., Li, J., Zheng, X., Pan, Y., Wang, Z., Zhang, M., et al. (2016). Janus "nano-bullets" for magnetic targeting liver cancer chemotherapy. Biomaterials 100, 118–133. doi:10.1016/j.biomaterials.2016.05.030
Siegel, R. L., Miller, K. D., Fuchs, H. E., and Jemal, A. (2022). Cancer statistics, 2022. CA Cancer J. Clin. 72 (1), 7–33. doi:10.3322/caac.21708
Simard, J. C., Durocher, I., and Girard, D. (2016). Silver nanoparticles induce irremediable endoplasmic reticulum stress leading to unfolded protein response dependent apoptosis in breast cancer cells. Apoptosis 21 (11), 1279–1290. doi:10.1007/s10495-016-1285-7
Sonekar, S., Mishra, M. K., Patel, A. K., Nair, S. K., Singh, C. S., and Singh, A. K. (2016). Formulation and evaluation of folic acid conjugated gliadin nanoparticles of curcumin for targeting colon cancer cells. J. Appl. Pharm. Sci. 6 (10), 068–074. doi:10.7324/japs.2016.601009
Song, Y., Li, D., Lu, Y., Jiang, K., Yang, Y., Xu, Y., et al. (2020). Ferrimagnetic mPEG-b-PHEP copolymer micelles loaded with iron oxide nanocubes and emodin for enhanced magnetic hyperthermia–chemotherapy. hyperthermia-chemotherapy 7 (4), 723–736. doi:10.1093/nsr/nwz201
Sun, R. W., Zhang, M., Li, D., Zhang, Z. F., Cai, H., Li, M., et al. (2015). Dinuclear gold(I) pyrrolidinedithiocarbamato complex: Cytotoxic and antimigratory activities on cancer cells and the use of metal-organic framework. Chemistry 21 (51), 18534–18538. doi:10.1002/chem.201503656
Tomuleasa, C., Soritau, O., Orza, A., Dudea, M., Petrushev, B., Mosteanu, O., et al. (2012). Gold nanoparticles conjugated with cisplatin/doxorubicin/capecitabine lower the chemoresistance of hepatocellular carcinoma-derived cancer cells. J. Gastrointestin Liver Dis. 21 (2), 187–196.
van Wamel, A., Sontum, P. C., Healey, A., Kvåle, S., Bush, N., Bamber, J., et al. (2016). Acoustic Cluster Therapy (ACT) enhances the therapeutic efficacy of paclitaxel and Abraxane® for treatment of human prostate adenocarcinoma in mice. J. Control Release 236, 15–21. doi:10.1016/j.jconrel.2016.06.018
Wang, C., Wang, J., Zhang, X., Yu, S., Wen, D., Hu, Q., et al. (2018). In situ formed reactive oxygen species-responsive scaffold with gemcitabine and checkpoint inhibitor for combination therapy. Sci. Transl. Med. 10 (429), eaan3682. doi:10.1126/scitranslmed.aan3682
Wang, F., Wang, Y., Ma, Q., Cao, Y., and Yu, B. (2017). Development and characterization of folic acid-conjugated chitosan nanoparticles for targeted and controlled delivery of gemcitabinein lung cancer therapeutics. Artif. Cells Nanomed Biotechnol. 45 (8), 1530–1538. doi:10.1080/21691401.2016.1260578
Wang, K., Cai, Z., Fan, R., Yang, Q., Zhu, T., Jiang, Z., et al. (2020a). A tumor-microenvironment-responsive nanomaterial for cancer chemo-photothermal therapy. RSC Adv. 10 (37), 22091–22101. doi:10.1039/d0ra04171h
Wang, S., Liu, C., Wang, C., Ma, J., Xu, H., Guo, J., et al. (2020b). Arsenic trioxide encapsulated liposomes prepared via copper acetate gradient loading method and its antitumor efficiency. Asian J. Pharm. Sci. 15 (3), 365–373. doi:10.1016/j.ajps.2018.12.002
Wang, Z., Zhi, K., Ding, Z., Sun, Y., Li, S., Li, M., et al. (2021). Emergence in protein derived nanomedicine as anticancer therapeutics: More than a tour de force. Semin. Cancer Biol. 69, 77–90. doi:10.1016/j.semcancer.2019.11.012
Wu, D., Zhu, L., Li, Y., Wang, H., Xu, S., Zhang, X., et al. (2020). Superparamagnetic chitosan nanocomplexes for colorectal tumor-targeted delivery of irinotecan. Int. J. Pharm. 584, 119394. doi:10.1016/j.ijpharm.2020.119394
Wu, P., Liu, Q., Li, R., Wang, J., Zhen, X., Yue, G., et al. (2013). Facile preparation of paclitaxel loaded silk fibroin nanoparticles for enhanced antitumor efficacy by locoregional drug delivery. ACS Appl. Mater Interfaces 5 (23), 12638–12645. doi:10.1021/am403992b
Wu, S. Y., Chou, H. Y., Yuh, C. H., Mekuria, S. L., Kao, Y. C., and Tsai, H. C. (2018). Radiation-sensitive dendrimer-based drug delivery system. Adv. Sci. (Weinh) 5 (2), 1700339. doi:10.1002/advs.201700339
Xiong, L., Du, X., Kleitz, F., and Qiao, S. Z. (2015). Cancer-cell-specific nuclear-targeted drug delivery by dual-ligand-modified mesoporous silica nanoparticles. Small 11 (44), 5919–5926. doi:10.1002/smll.201501056
Yan, G., Li, A., Zhang, A., Sun, Y., and Liu, J. (2018). Polymer-based nanocarriers for Co-delivery and combination of diverse therapies against cancers. Nanomater. (Basel) 8 (2), 85. doi:10.3390/nano8020085
Yang, B., Song, B. P., Shankar, S., Guller, A., and Deng, W. (2021). Recent advances in liposome formulations for breast cancer therapeutics. Cell Mol. Life Sci. 78 (13), 5225–5243. doi:10.1007/s00018-021-03850-6
Yetisgin, A. A., Cetinel, S., Zuvin, M., Kosar, A., and Kutlu, O. (2020). Therapeutic nanoparticles and their targeted delivery applications. Molecules 25 (9), 2193. doi:10.3390/molecules25092193
Yoshizaki, Y., Yuba, E., Komatsu, T., Udaka, K., Harada, A., and Kono, K. (2016). Improvement of peptide-based tumor immunotherapy using pH-sensitive fusogenic polymer-modified liposomes. Molecules 21 (10), 1284. doi:10.3390/molecules21101284
Zhang, Q. Y., Wang, F. X., Jia, K. K., and Kong, L. D. (2018). Natural product interventions for chemotherapy and radiotherapy-induced side effects. Front. Pharmacol. 9, 1253. doi:10.3389/fphar.2018.01253
Zhao, Y., Zhao, X., Cheng, Y., Guo, X., and Yuan, W. (2018). Iron oxide nanoparticles-based vaccine delivery for cancer treatment. Mol. Pharm. 15 (5), 1791–1799. doi:10.1021/acs.molpharmaceut.7b01103
Zheng, R., Zhang, S., Zeng, H., Wang, S., Sun, K., Chen, R., et al. (2022). Cancer incidence and mortality in China, 2016. J. Natl. Cancer Cent. 2 (1), 1–9. doi:10.1016/j.jncc.2022.02.002
Zhou, J., Wang, M., Han, Y., Lai, J., and Chen, J. (2020). Multistage-targeted gold/mesoporous silica nanocomposite hydrogel as in situ injectable drug release system for chemophotothermal synergistic cancer therapy. ACS Appl. Bio Mat. 3 (1), 421–431. doi:10.1021/acsabm.9b00895
Keywords: nanomaterials, tumor, targeted therapy, drug delivery, tumorigenic factors
Citation: Zhang P, Ye G, Xie G, Lv J, Zeng X and Jiang W (2023) Research progress of nanomaterial drug delivery in tumor targeted therapy. Front. Bioeng. Biotechnol. 11:1240529. doi: 10.3389/fbioe.2023.1240529
Received: 15 June 2023; Accepted: 14 July 2023;
Published: 24 July 2023.
Edited by:
Zhanzhan Zhang, Tianjin Medical University, ChinaReviewed by:
Guotao Yuan, Sun Yat-sen University, ChinaJingyu Wang, Tianjin Medical University, China
Chunxiong Zheng, Sun Yat-sen University, China
Copyright © 2023 Zhang, Ye, Xie, Lv, Zeng and Jiang. This is an open-access article distributed under the terms of the Creative Commons Attribution License (CC BY). The use, distribution or reproduction in other forums is permitted, provided the original author(s) and the copyright owner(s) are credited and that the original publication in this journal is cited, in accordance with accepted academic practice. No use, distribution or reproduction is permitted which does not comply with these terms.
*Correspondence: Jie Lv, bHZqaWVAeWx1LmVkdS5jbg==; Xianhai Zeng, enhoa2x3eEAxNjMuY29t; Wei Jiang, MTM2Njc0NTI5MTFAMTYzLmNvbQ==
†These authors have contributed equally to this work