- 1The Research and Application Center of Precision Medicine, The Second Affiliated Hospital of Zhengzhou University, Zhengzhou, China
- 2Department of Pharmacy/Evidence-Based Pharmacy Center, West China Second University Hospital, Sichuan University, Chengdu, Sichuan, China
- 3Department of Psychiatry, Huaian No. 3 People’s Hospital, Huai’an, Jiangsu, China
- 4Department of Electronic Science and Technology, School of Physics and Technology, Wuhan University, Wuhan, China
- 5Blood Purification Center, The People’s Hospital of Zhengzhou University, Zhengzhou, China
- 6Blood Purification Center, Henan Provincial People’s Hospital, Zhengzhou, China
- 7Department of Central Laboratory and Precision Medicine Center, The Affiliated Huai’an Hospital of Xuzhou Medical University and Huai’an Second People’s Hospital, Huai’an, China
- 8Department of Nephrology, The Affiliated Huai’an Hospital of Xuzhou Medical University and Huai’an Second People’s Hospital, Huai’an, China
Introduction: Radiotherapy (RT) is one of the key methods for treating breast cancer. However, the effect of single RT is often poor because of insufficient deposition of X-rays in tumor sites and radiation resistance induced by the abnormal tumor microenvironment (overexpression of glutathione (GSH)). The development of multifunctional RT sensitizers and synergetic therapeutic strategies is, therefore, a promising area for enhancing the anticancer effect of RT.
Methods: In this study, a multifunctional nanozyme hydrogel based on Cu-doped polypyrrole (CuP) was designed to work concertedly with a second near-infrared thermal RT. The CuP-based hydrogel (CH) reached the tumor site when injected in-situ and achieved long-term storage.
Results: Once stimulated with 1064-nm laser irradiation, the heated and softened hydrogel system released CuP nanozyme to provide photothermal therapy, thereby inhibiting the repair of DNA damage caused by RT. In addition, CuP with dual nanozyme activity depleted the intracellular GSH to reduce the antioxidant capacity of the tumor. Moreover, CuP converted H2O2 to produce ·OH to directly kill the tumor cells, thus enhancing the capability of low-dose RT to inhibit tumor growth. In vivo experiments showed that the CH system used in combination with a low-power 1064-nm laser and low-dose RT (4 Gy) exhibited good synergistic anticancer effects and biological safety.
Discussion: As a new light-responsive hydrogel system, CH holds immense potential for radio-sensitization.
Introduction
Cancer is one of the most life-threatening diseases in the world (Li et al., 2022). Approximately 70% of patients with cancer need radiotherapy (RT) (Limoli and Vozenin, 2023; Lin et al., 2023; Wu et al., 2023). RT mainly uses high-energy X-rays or γ-rays to induce DNA damage and cell apoptosis, thus resulting in tumor ablation (Secchi et al., 2023). When an adequate dose of radiation is used to locally irradiate the cancer tissue, it fights against cancer cells and effectively combats the disease. However, high-dose radiation can also cause damage to the normal cells in the vicinity, which is a concern in the development of RT (Ni et al., 2018; Gao et al., 2022). With the advancements in nanomedicine, several new cancer treatments, such as photothermal therapy (PTT), photodynamic therapy (PDT), and gene therapy, have emerged (Wang et al., 2022a; Wang et al., 2022b; Sun et al., 2022; Zhao et al., 2022). Of these, PTT is a kind of treatment method that uses a photothermal agent to convert light energy into heat energy under the irradiation of external light sources, such as near-infrared (NIR) laser (Ye et al., 2019; Zhu Y. et al., 2022). This irradiation increases the temperature of the tumor site, thus ablating the tumor. Owing to its high selectivity, low invasion, and high efficiency, PTT has been employed widely (Zhu et al., 2023a). The heat generated during PTT not only directly kills tumor cells but also acts as an RT sensitizer. First, hyperthermia induces double-strand breaks in the DNA, thus leading to protein aggregation and inhibiting the repair of DNA damage induced by ion radiation (Wang et al., 2021). Second, the high temperature kills S-phase cells that display the lowest sensitivity to radiation (Lyu et al., 2021). Third, the mild photothermal effect accelerates the blood flow in the tumor, thus increasing the oxygenation in the hypoxic part of the tumor and improving the sensitivity of tumor cells to RT (Lyu et al., 2019). Therefore, the concerted use of PTT/RT has good prospects.
The antitumor effect of PTT/RT is limited to a great extent by the complexity of the tumor physiological environment (poor permeability of the radiosensitizer in RT) and its limitation (limited conversion efficiency of the photothermal agent) (Yin et al., 2017; Cheng et al., 2021; Lan et al., 2021). Owing to the rapid growth and metabolism of tumor cells, the tumor microenvironment (TME) tends to be acidic, with low oxygen and elevated H2O2 levels (Ren et al., 2020; Zhu D. et al., 2022). Based on these characteristics, a reasonably designed nanozyme system can generate toxic substances in tumors via endogenous properties or exogenous stimulation to kill tumor cells, thereby improving the effects of thermal RT on tumors (Ji et al., 2021; Jiang et al., 2022). In recent years, nanomaterials have gained widespread attention in the field of tumor therapy (Ye et al., 2019; Ren et al., 2020; Sun et al., 2021; Zhao et al., 2022; Chen et al., 2023). The physicochemical response characteristics of nanomaterials are combined with the catalytic activity of enzymes, such as glutathione oxidase (GSH-OXD) and peroxidase (POD) (Zhu Y. et al., 2021; Zhu et al., 2023a; Zhu et al., 2023b). Hence, the development of multifunctional nanozymes can provide a promising weapon for enhancing tumor treatment. Intracellular GSH can offer protection against radiation, including free radical removal, peroxide reduction, and protein mercaptan, to maintain the reduced state (Chang et al., 2020). Emerging studies have shown that mixed-valence copper-based nanomaterials can specifically catalyze the conversion of GSH into glutathione disulfide in tumors in response to the TME, thereby restoring the sensitivity of cells to X-rays. In addition, a Cu+-mediated pod-like reaction converts H2O2 into highly toxic OH to destroy mitochondria, which makes cells more vulnerable to radiation attack (Zhang et al., 2011).
Photothermal agents are generally injected intravenously to deliver them to tumor tissues, and although the biocompatibility and targeting of most photothermal agents can be improved via modification, their efficacy remains unsatisfactory (Dai et al., 2022; Zhuang et al., 2022). Furthermore, the systemic toxicity of nanomaterials, which often leads to unavoidable side effects, should be considered (Xu et al., 2022). Hydrogel is a three-dimensional network polymer that has been widely used in the biomedical field. The functions of the hydrogel include sustained drug release, cell delivery vehicle, and tissue engineering (Hou et al., 2018). Injectable hydrogels with injectable and in situ gelling properties are of great interest owing to their biocompatibility, ease of handling, and non-invasive mode of administration via injection (Wu et al., 2019). Such hydrogels exhibit properties that are superior to those of preformed hydrogels. The hydrogel carrying the cargo can prolong the sustained release time of the cargo, immensely reduce the toxicity and systemic side effects, centralize the administration concentration, and augment the utilization rate of the drug (Zhu D. et al., 2021; Zhang et al., 2022). Injectable hydrogels are clinically appealing because they significantly reduce patient discomfort, risk of infection, recovery time, and treatment costs (Chen et al., 2020). For example, Zhang designed light-responsive black phosphorus (BP)-based hydrogel to achieve controlled release of the BP and enable safe photothermal therapy (Qiu et al., 2018). Therefore, light-responsive hydrogels are expected to become the preferred medium for controlled-release nanomaterials.
In this study, Cu-doped polypyrrole (CuP), a copper-based multifunctional nanozyme was synthesized and loaded into agarose hydrogel to form a CuP-based hydrogel (CH) to achieve sensitization treatment for breast cancer using a second near-infrared (NIR-II) photothermal synergistic RT (Scheme 1). CH reached the focal area directly when injected intratumorally and accumulated in situ after gelation. After irradiation with 1064-nm laser (0.5 W/cm2), CH converted light energy into heat energy, thereby enabling accurate and deep penetration of NIR-II PTT. Moreover, the thermally responsive hydrogel gradually softened and released the CuP nanozyme. CuP reduced GSH in the TME and converted H2O2 to generate highly toxic OH, destroy the redox steady state, and greatly enhance the effect of subsequent RT. Both in vitro cell experiments and in vivo animal model experiments showed that the designed CH system achieved satisfactory synergistic therapeutic effects. Furthermore, the combination of a relatively low radiation dose (only 4Gy) and a laser power of 0.5 W/cm2 resulted in almost no adverse effects during the treatment cycle. This finding confirmed the long-term reliability of the CH system and provided novel insights for the multiple sensitization study of RT for 4T1 tumors.
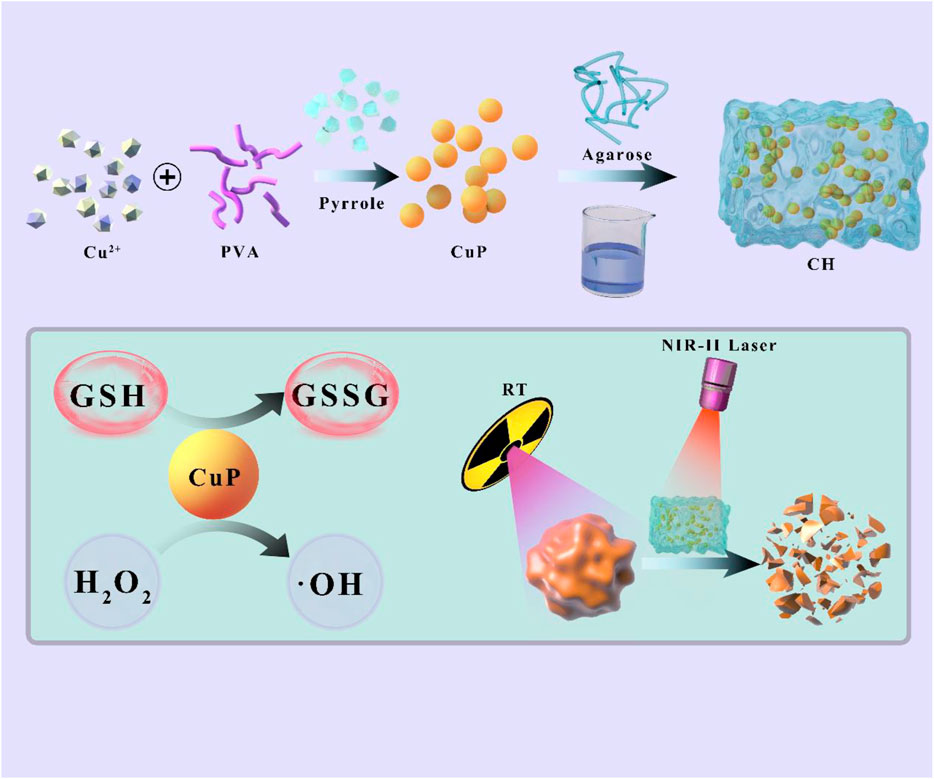
SCHEME 1. Schematic illustration of Cu-Doped polypyrrole hydrogel with tumor catalyst activity for NIR-II thermo-radiotherapy.
Results and discussion
In this study, CuCl2 was used as an oxidation catalyst instead of FeCl3. In situ chemical oxidative polymerization was used to trigger the polymerization of pyrrole monomer at room temperature, and polyvinyl alcohol (PVA) was used as a stabilizer to avoid the infinite growth of CuP. As shown in Figure 1A, transmission electron microscopy (TEM) revealed that the synthesized CuP exhibited good dispersion, uniform size, and spherical characteristics. When the elemental mapping images were analyzed, the major elements Cu, C, and N were distributed (Figure 1B). The size of the CuP nanozyme was controlled to approximately 100 nm by improving the PVA content (Figure 1C). The stability of nanocomposites in solution is critical to achieving a good therapeutic effect (Su et al., 2021; Yang et al., 2022). The zeta potential of the CuP obtained by repeated preparation is almost unchanged (Figure 1D). Hence, the average hydrodynamic diameter change of CuP dispersed in PBS was measured for 1 week using dynamic light scattering (DLS). Figure 1E presents the hydrodynamic diameter of CuP that was measured for seven consecutive days. The results confirmed the long-term stability of the prepared nanozyme. As depicted in Figure 1F, the UV–vis spectra showed that CuP had a broad absorption band in the 1,000–1,100 nm range. The absorption spectrum of CuP irradiated with a 1064-nm laser did not change significantly, which indicates that CuP has excellent photothermal conversion stability. Transition metals with multivalent states, such as Cu and Co, have been proven to exhibit catalytic activity in tumor-specific therapy, thus destroying the redox homeostasis in the TME (Ren et al., 2020). To confirm that CuP displayed POD-like enzymatic activity, methylene blue (MB) was used as a probe for the preliminary evaluation of the degradation ability of CuP for MB at different temperatures. The results demonstrated that the POD-like activity of a given concentration of CuP increased with the increase in temperature. It is worth noting that when the temperature reached 45°C, the degradation rate of MB reached approximately 50% in 20 min (Figure 1G). To adapt to the internal oxidative stress of cell growth and survival, cancer cells regulate their ROS level by upregulating antioxidants such as GSH (Sang et al., 2020). Hence, GSH is highly expressed in tumor cells, which affects their sensitivity to RT to a certain extent. DTNB was used as a probe to test the GSH–OXD-like activity of CuP. The findings suggested that the consumption of GSH by CuP increased with time, which could be attributed to the redox reaction between GSH and the active site of Cu2+ (Figure 1H).
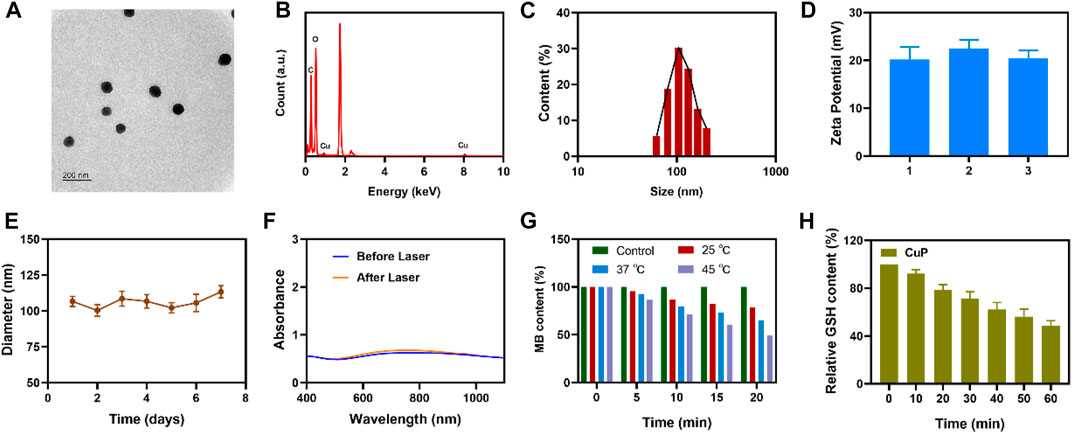
FIGURE 1. (A) TEM image of CuP (B) Energy dispersive spectroscopy (EDS) of CuP (C) Hydrodynamic diameter of CuP in water (D) The zeta potential of CuP suspended in PBS (E) DLS diameter of CuP within 7 days (F) UV-vis-NIR absorbance spectra of CuP aqueous dispersions before and after 1,064 nm laser irradiation for 20 min (G) MB depletion profile treated with or without CuP at different temperature (RT, 37°C, and 45°C) (H) The relative GSH content of the supernatant after the reaction of GSH and CuP.
For further experiments, injectable hydrogels were prepared by mixing CuP and agarose solutions as vehicles for enhancing PTT/RT. The rheological properties of CH were examined at different temperatures. The results showed that the hydrogel gradually softened with the increase in temperature and the storage modulus continued to decrease, thus leading to the controlled release of CuP in the hydrogel (Figure 2A). When the temperature and injection time were controlled, CH gelled rapidly in situ in the tumor. Owing to the excellent optical absorption of CuP in the NIR II region and its ability to respond to the conversion of light energy into heat energy, the photothermal heating capacities of CuP and CH were evaluated comprehensively using the photothermal imaging system. Figure 2B portrays the heating effect of CuP at different concentrations under the irradiation of a 1064-nm laser (0.5 W/cm2). The temperature of the aqueous solution of CuP (20 μg/mL) increased gradually under irradiation for 5 min and finally reached 46 °C (Figure 2B). In contrast, the temperature of the deionized aqueous solution did not increase perceptibly. This finding signifies that CuP, a photothermal agent, plays a key role in photothermal stability under laser irradiation. Furthermore, according to the temperature changes in the four heating–cooling cycles of 1064-nm laser irradiation with a power of 0.5 W/cm2 for 5 min (Figure 2C), the heating effect remained mostly unchanged until the end of the cycle. This result indicates that CuP has good photothermal stability. Therefore, the prepared CuP displays good photothermal conversion ability and photothermal stability under the irradiation of a 1064-nm laser, thus denoting its great potential in the PTT application. Scanning electron microscope (SEM) revealed the three-dimensional network structure of the hydrogel. The complex pore structure can accommodate more carriers (Figure 2D). Hence, the photothermal heating ability of CH was tested. CH was dropped at the center of a Petri dish and irradiated with a 1064-nm laser (Figure 2E). The infrared thermogram and 3D thermogram showed that the heating effect was significant (Figure 2F). The above results demonstrate that CuP has a good heating effect under 1064-nm laser irradiation. The CuP is an excellent photothermal agent that could be used synergistically with multimodal therapy for tumors.
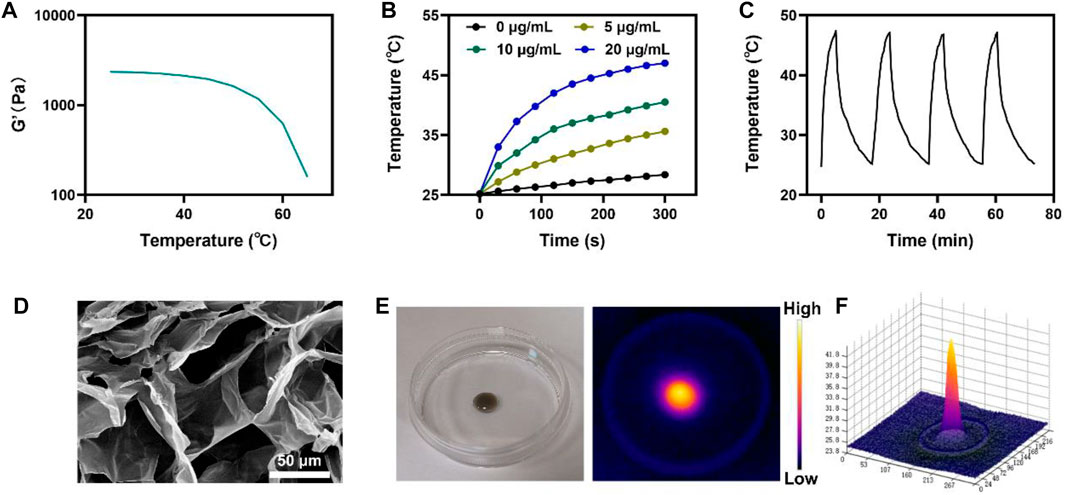
FIGURE 2. (A) Temperature-dependent Rheological curves for the prepared CH (B) Temperature changes of CuP NPs at various concentrations under a 5 min irradiation from a 1,064 nm laser at 0.5 W/cm2 (C) Heating curve of CH for four cycles having a 0.5 W/cm2 power intensity under irradiation by 1,064 nm laser (D) SEM image of the CH (E) The photographic pictures represent the morphology of the prepared CH and IR image during laser irradiation (F) Relevant 3D temperature diagram in 2 E.
In view of the aforementioned properties of CuP, the TME modulation ability and in vitro anticancer outcomes of CH were evaluated. The photothermal therapeutic effect and radio-sensitization ability of CuP were investigated using 4T1 cells as a model. First, the high performance of the CH synergistic multimodal treatment was confirmed using a clone formation experiment. As shown in Figure 3A, PBS + NIR and CH groups had high cell populations, whereas RT and CH + NIR groups formed relatively fewer colonies. Compared with these groups, CH synergistic NIR + RT formed fewer cell clusters and had a lower survival fraction, which was significantly different compared with CH + NIR. Studies have reported that when irradiating cells with X-rays, RT can directly damage DNA molecules in the nucleus or indirectly damage them by producing various reactive oxygen molecules with water molecules. In this study, DCFH-DA probes were used to explore the reactive oxygen species (ROS) induced by various groups. Upon entering the cells, DCFH-DA is hydrolyzed by intracellular esterase to produce DCFH, which is in turn oxidized by ROS to produce DCF that emits green fluorescence (Zhu et al., 2020). The RT group induced weak green fluorescence, as shown in Figures 3B,F. On the contrary, the CH + NIR group produced moderate green fluorescence, which could be attributed to the fact that CH subjected to laser radiation facilitates photothermal therapy by destroying the TME. CuP reduced the intracellular GSH content and converted the abundant H2O2 to produce OH, which led to increased oxidative stress and, eventually, increased ROS level. The brightest green fluorescence was achieved in the CH + NIR + RT group, which indicates that the heating effect of PTT could effectively promote the sensitization of RT to generate more ROS. X-ray induced double-strand breaks in the DNA, and H2AX served as the sensitivity index for monitoring double-strand breaks in the DNA. The red fluorescence in the CH + NIR + RT group was the most significant of all groups, which indicates that its nuclear DNA was most damaged (Figures 3C, Supplementary Figure S1). Furthermore, to investigate whether CH has good biocompatibility, its cytotoxicity was studied in vitro using 4T1 cells. MTT test results showed that even if the concentration of CuP reached 80 μg/mL (Figure 3D), it displayed low cytotoxicity toward 4T1 cells and that the cell viability remained >90%. CH-mediated PTT inhibited the repair of DNA damage after RT. CuP in the hydrogel system not only exhibited POD-like activity and produced highly toxic OH to destroy cell activity but also reduced the GSH content and made tumor cells more sensitive to ROS, thereby accentuating the radio-sensitization effect (Figure 3E). This combined strategy can augment the therapeutic efficacy by transforming non-toxic CH into a toxic therapeutic system via light stimulation and increasing the sensitivity of tumor cells to X-rays.
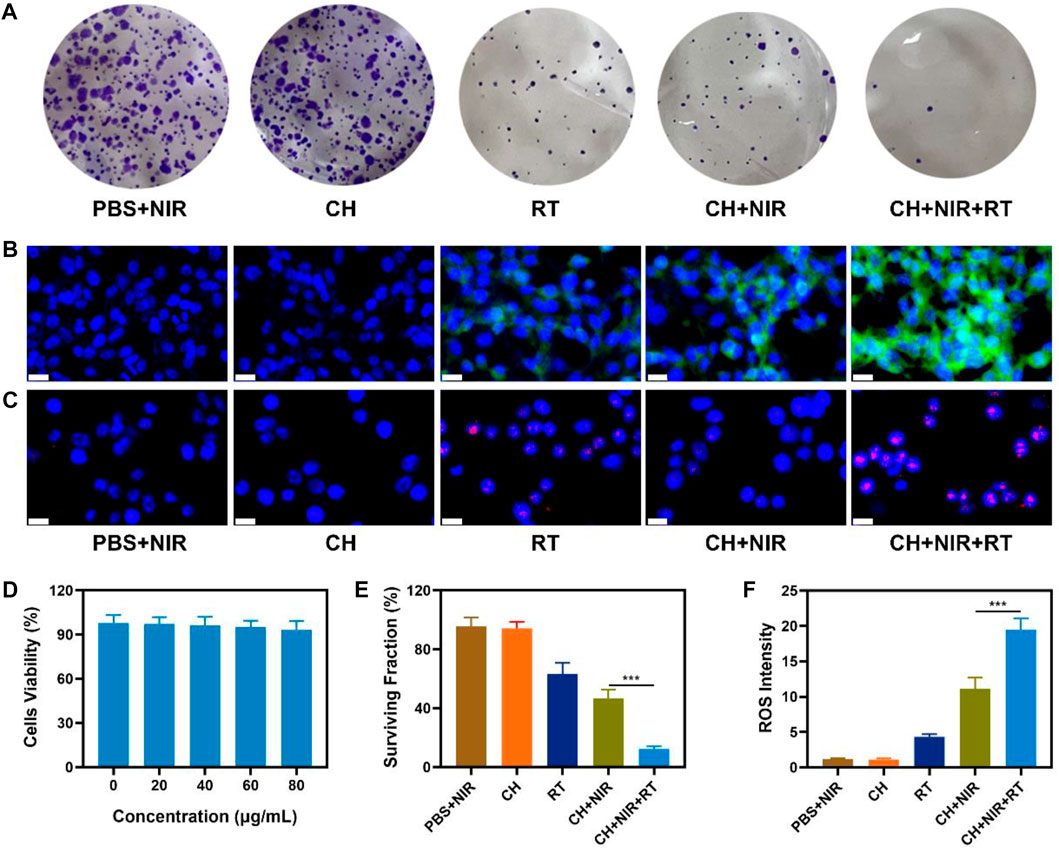
FIGURE 3. (A) Colony of 4T1 cells treated with different formulations and the irradiation dose was 4 Gy (B) CLSM images of DCFH-DA-stained 4T1 cells after treatment with different formulations. Scale bars: 20 μm (C) Detection of 4T1 cells’ DNA damage through immunofluorescence staining of γ-H2AX, induced by different formulations. Scale bars: 20 μm (D) Dark cytotoxicity of CH on 4T1 cells (E) In vitro cytotoxicity of different formulations against 4T1 cells (F) Corresponding quantitative analysis of ROS generation in 3A. ***p < 0.005; Student’s t-test.
Considering the encouraging results for the validation experiments of photothermal agent and multifunctional nanozyme, the in vivo antitumor ability was investigated. 4T1 tumor-bearing mice were constructed via the subcutaneous injection of 4T1 cells in BALB/c mice. The mice were randomly assigned to the following five groups: 1) PBS + NIR, 2) CH, 3) RT, 4) CH + NIR, and 5) CH + NIR + RT. Tumor volumes were recorded every 4 days after providing the corresponding treatment, as shown in Figure 4A. The tumor volumes increased rapidly over time in the PBS + NIR group and were slightly inhibited by RT. On the contrary, the tumor volumes were inhibited during the first week in the CH + NIR-treated mice. Non-etheless, the treatment was not effective in the following week, and there was a rapid increase in the tumor volume. This result suggests that the combination of CH and photothermal therapy was inadequate to destroy tumor cell growth. In the CH + NIR + RT group, the tumor volume was significantly inhibited during the whole treatment cycle. CH was initially enriched in the tumor area, and after irradiation with the highly penetrating 1064-nm laser, the light energy was converted into heat energy, which destroyed the activity of tumor cells to a certain extent. Moreover, the high temperature enhanced the nanozyme activity of CuP, which converted H2O2 to produce more OH. This killed the tumor tissue and depleted GSH, the reducing substance, which made the tumor cells more sensitive to RT. After the 16th day of treatment, the mice were sacrificed and their tumors were weighed. As shown in Figures 4B, Supplementary Figure S2, the tumor weight was significantly reduced in the treatment group compared with that in the control group, which agreed with the tumor growth curve. Notable changes in body weights were not seen in any of the mice during the treatment cycle, which affirms that the regimen was not considered toxic and did not cause substantial harm to the mice (Figure 4C). To further examine the mechanism of killing the tumor cells, the tumor tissue was subjected to various staining analyses at the end of the treatment. The CH + NIR + RT group exhibited the strongest apoptotic signal and the weakest proliferative signal, together with a high content and density of ROS (Figure 4D). These findings allude that this therapeutic regimen achieved the optimal antitumor effects. To further confirm the in vivo safety of the system, H&E staining was performed on the main organs (heart, liver, spleen, lung, and kidney) of the mice. As illustrated in Supplementary Figure S3, compared with the PBS group, there were no significant pathological changes in the main organs of mice treated with CH + NIR + RT. This finding signifies that the treatment system has good biocompatibility and would not cause significant damage to the mice. Based on these observations, we believe that CH is a good photothermal agent and radiosensitizer, with good application potential for treating breast cancer.
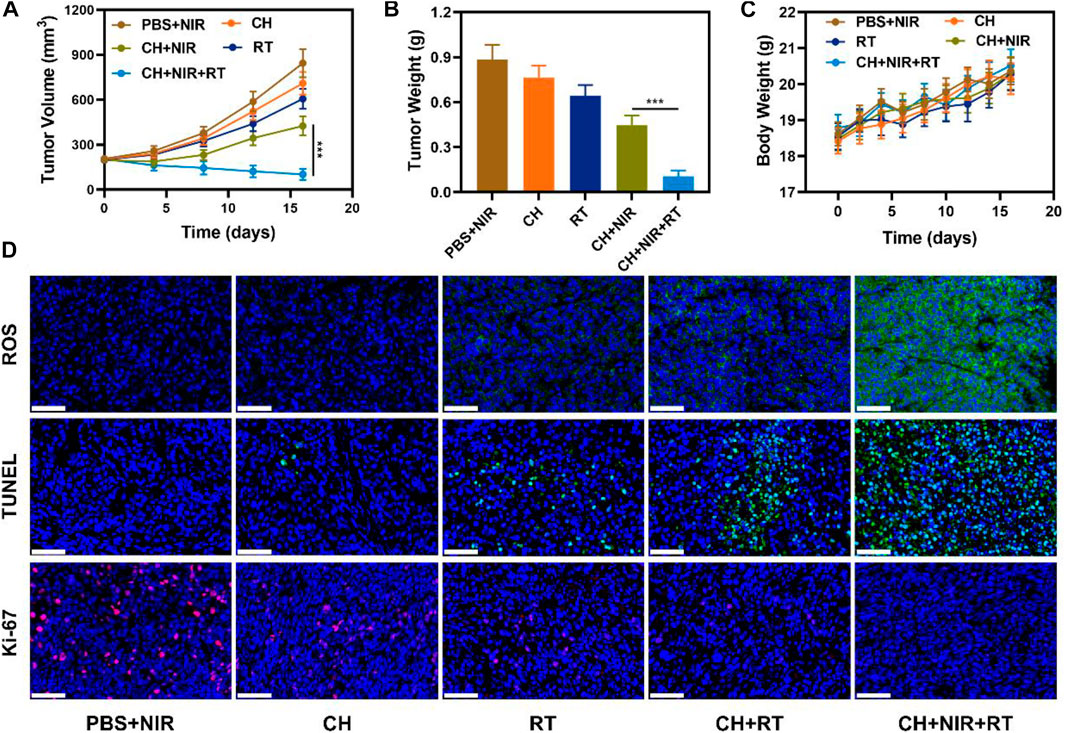
FIGURE 4. (A) Tumor volume changes and (B) tumor weights (C) Body weight changes of treated mice (D) ROS, TUNEL and Ki-67 stained tumor sections from the indicated treatment groups. Scale bars: 50 μm ***p < 0.005; Student’s t-test.
Conclusion
In this study, a Cu-doped polypyrrole-based hydrogel with tumor catalytic activity was designed for enhanced NIR-II PTT and RT. After IR stimulation, the non-toxic CH turned into a toxic treatment system. The softened CH released CuP to deplete the GSH in the TME, thereby alleviating the antioxidant capability and catalyzing the production of OH from intracellular H2O2 to kill tumor cells. The oxidative stress was thus amplified, and the approach worked concertedly with the subsequent RT to achieve a good antitumor effect. This treatment system, when combined with low-dose RT, can commendably inhibit tumor growth during the entire treatment cycle. In addition, the availability and biological safety of CH make it an extremely promising agent in clinical therapy.
Data availability statement
The original contributions presented in the study are included in the article/Supplementary Material, further inquiries can be directed to the corresponding authors.
Ethics statement
The animal experiments were carried out according to the protocol approved by the Ministry of Health in People's Republic of PR China and were approved by the Administrative Committee on Animal Research of the Wuhan University.
Author contributions
SW Methodology, Validation, Formal analysis; HF Conceptualization, Writing—review and editing; YM Writing—original draft, Writing—review and editing; DZ Formalanalysis, Data curation; HZ Formal analysis, Data curation, Writing—review and editing; XL Conceptualization, Writing—review and editing, Project administration; QH: Funding acquisition, Investigation, Writing—original draft. All authors contributed to the article and approved the submitted version.
Funding
The authors are grateful for the financial support from the Cultivation of High-level Internationalized Talents Project, 22180007.
Conflict of interest
The authors declare that the research was conducted in the absence of any commercial or financial relationships that could be construed as a potential conflict of interest.
Publisher’s note
All claims expressed in this article are solely those of the authors and do not necessarily represent those of their affiliated organizations, or those of the publisher, the editors and the reviewers. Any product that may be evaluated in this article, or claim that may be made by its manufacturer, is not guaranteed or endorsed by the publisher.
Supplementary material
The Supplementary Material for this article can be found online at: https://www.frontiersin.org/articles/10.3389/fbioe.2023.1225937/full#supplementary-material
References
Chang, M., Hou, Z., Jin, D., Zhou, J., Wang, M., Wang, M., et al. (2020). Colorectal tumor microenvironment-activated bio-decomposable and metabolizable Cu2 O@CaCO3 nanocomposites for synergistic oncotherapy. Adv. Mater. 32, e2004647. doi:10.1002/adma.202004647
Chen, M., Tan, Y., Dong, Z., Lu, J., Han, X., Jin, Q., et al. (2020). Injectable anti-inflammatory nanofiber hydrogel to achieve systemic immunotherapy post local administration. Nano Lett. 20, 6763–6773. doi:10.1021/acs.nanolett.0c02684
Chen, Z., Zheng, X., Zhao, J., Tang, J., Hu, L., and Wang, S. (2023). Glucose oxidase-loaded colloidal stable WS2 nanobowls for combined starvation/photothermal therapy of colorectal tumors. Int. J. Pharm. 636, 122848. doi:10.1016/j.ijpharm.2023.122848
Cheng, Y., Wen, C., Sun, Y. Q., Yu, H., and Yin, X. B. (2021). Mixed-Metal MOF-derived hollow porous nanocomposite for trimodality imaging guided reactive oxygen species-augmented synergistic therapy. Adv. Funct. Mater. 31 (37), 2104378. doi:10.1002/adfm.202104378
Dai, J., Wu, M., Xu, Y., Yao, H., Lou, X., Hong, Y., et al. (2022). Platelet membrane camouflaged AIEgen-mediated photodynamic therapy improves the effectiveness of anti-PD-L1 immunotherapy in large-burden tumors. Bioeng. Transl. Med. 8, e10417. doi:10.1002/btm2.10417
Gao, J., Wang, Z., Guo, Q., Tang, H., Wang, Z., Yang, C., et al. (2022). Mitochondrion-targeted supramolecular "nano-boat" simultaneously inhibiting dual energy metabolism for tumor selective and synergistic chemo-radiotherapy. Theranostics 12 (3), 1286–1302. doi:10.7150/thno.67543
Hou, M., Yang, R., Zhang, L., Zhang, L., Liu, G., Xu, Z., et al. (2018). Injectable and natural humic acid/agarose hybrid hydrogel for localized light-driven photothermal ablation and chemotherapy of cancer. ACS Biomaterials Sci. Eng. 4 (12), 4266–4277. doi:10.1021/acsbiomaterials.8b01147
Ji, S., Jiang, B., Hao, H., Chen, Y., Dong, J., Mao, Y., et al. (2021). Matching the kinetics of natural enzymes with a single-atom iron nanozyme. Nat. Catal. 4 (5), 407–417. doi:10.1038/s41929-021-00609-x
Jiang, C., He, T., Tang, Q., He, J., Ren, Q., Zhang, D.-Y., et al. (2022). Nanozyme catalyzed cascade reaction for enhanced chemodynamic therapy of low-H2O2 tumor. Appl. Mater. Today 26, 101357. doi:10.1016/j.apmt.2021.101357
Lan, J.-S., Liu, L., Zeng, R.-F., Qin, Y.-H., Hou, J.-W., Xie, S.-S., et al. (2021). Tumor-specific carrier-free nanodrugs with GSH depletion and enhanced ROS generation for endogenous synergistic anti-tumor by a chemotherapy-photodynamic therapy. Chem. Eng. J. 407, 127212. doi:10.1016/j.cej.2020.127212
Li, Q., Wu, T. T., Fan, X. W., Guo, X. B., Jiang, W., and Fan, K. L. (2022). Multifaceted nanozymes for synergistic antitumor therapy: A review. Mater. Des. 224, 111430. doi:10.1016/j.matdes.2022.111430
Limoli, C. L., and Vozenin, M.-C. (2023). Reinventing radiobiology in the light of FLASH radiotherapy. Reinventing Radiobiology Light FLASH Radiotherapy 7 (1), 1–21. doi:10.1146/annurev-cancerbio-061421-022217
Lin, T. A., Wei, L.-J., and Ludmir, E. B. (2023). Radiotherapy alone vs radiotherapy with concurrent chemoradiotherapy and survival of patients with low-risk nasopharyngeal carcinoma. JAMA 329 (3), 261. doi:10.1001/jama.2022.21033
Lyu, M., Chen, M., Liu, L., Zhu, D., Wu, X., Li, Y., et al. (2021). A platelet-mimicking theranostic platform for cancer interstitial brachytherapy. Theranostics 11 (15), 7589–7599. doi:10.7150/thno.61259
Lyu, M., Zhu, D., Li, Y., and Quan, H. (2019). Bimetallic nanodots for tri-modal CT/MRI/PA imaging and hypoxia-resistant thermoradiotherapy in the NIR-II biological windows. Biomaterials 233, 119656. doi:10.1016/j.biomaterials.2019.119656
Ni, K., Lan, G., Veroneau, S. S., Duan, X., Song, Y., and Lin, W. (2018). Nanoscale metal-organic frameworks for mitochondria-targeted radiotherapy-radiodynamic therapy. Nat. Commun. 9 (1), 4321. doi:10.1038/s41467-018-06655-7
Qiu, M., Wang, D., Liang, W., Liu, L., Zhang, Y., Chen, X., et al. (2018). Novel concept of the smart NIR-light-controlled drug release of black phosphorus nanostructure for cancer therapy. Proc. Natl. Acad. Sci. U. S. A. 115 (3), 501–506. doi:10.1073/pnas.1714421115
Ren, S. Z., Wang, B., Zhu, X. H., Zhu, D., Liu, M., Li, S. K., et al. (2020). Oxygen self-sufficient core-shell metal-organic framework-based smart nanoplatform for enhanced synergistic chemotherapy and photodynamic therapy. ACS Appl. Mater. interfaces 12 (22), 24662–24674. doi:10.1021/acsami.0c08534
Sang, Y., Cao, F., Li, W., Zhang, L., You, Y., Deng, Q., et al. (2020). Bioinspired construction of a nanozyme-based H2O2 homeostasis disruptor for intensive chemodynamic therapy. J. Am. Chem. Soc. 142 (11), 5177–5183. doi:10.1021/jacs.9b12873
Secchi, V., Cova, F., Villa, I., Babin, V., Nikl, M., Campione, M., et al. (2023). Energy partitioning in multicomponent nanoscintillators for enhanced localized radiotherapy. ACS Appl. Mater. interfaces 15, 24693–24700. doi:10.1021/acsami.3c00853
Su, X., Cao, Y., Liu, Y., Ouyang, B., Ning, B., Wang, Y., et al. (2021). Localized disruption of redox homeostasis boosting ferroptosis of tumor by hydrogel delivery system. Mater. Today Bio 12, 100154. doi:10.1016/j.mtbio.2021.100154
Sun, J., Wang, J., Hu, W., Wang, Y., Zhang, Q., Hu, X., et al. (2022). A porous bimetallic Au@Pt core-shell oxygen generator to enhance hypoxia-dampened tumor chemotherapy synergized with NIR-II photothermal therapy. ACS Nano 16, 10711–10728. doi:10.1021/acsnano.2c02528
Sun, M., Ye, H., Shi, Q., Xie, J., Yu, X., Ling, H., et al. (2021). Both-in-one hybrid bacteria suppress the tumor metastasis and relapse via tandem-amplifying reactive oxygen species-immunity responses. Adv. Healthc. Mater. 10 (21), 2100950. doi:10.1002/adhm.202100950
Wang, S., Yang, Y., Wu, H., Li, J., Xie, P., Xu, F., et al. (2022a). Thermosensitive and tum or microenvironment activated nanotheranostics for the chemodynamic/photothermal therapy of colorectal tumor. J. Colloid Interface Sci. 612, 223–234. doi:10.1016/j.jcis.2021.12.126
Wang, S., Zeng, N., Zhang, Q., Chen, M., and Huang, Q. (2022b). Nanozyme hydrogels for self-augmented sonodynamic/photothermal combination therapy. Front. Oncol. 12, 888855. doi:10.3389/fonc.2022.888855
Wang, Z., Zeng, W., Chen, Z., Suo, W., Quan, H., and Tan, Z. J. (2021). An intratumoral injectable nanozyme hydrogel for hypoxia-resistant thermoradiotherapy. Colloids surfaces. B, Biointerfaces 207, 112026. doi:10.1016/j.colsurfb.2021.112026
Wu, H., Liu, L., Song, L., Ma, M., Gu, N., and Zhang, Y. (2019). Enhanced tumor synergistic therapy by injectable magnetic hydrogel mediated generation of hyperthermia and highly toxic reactive oxygen species. ACS Nano 13, 14013–14023. doi:10.1021/acsnano.9b06134
Wu, Y., Zhu, K., Zhang, X., Du, W., Song, J., and Yang, H. (2023). Emerging plasmonic nanoparticles and their assemblies for cancer radiotherapy. Adv. drug Deliv. Rev. 194, 114710. doi:10.1016/j.addr.2023.114710
Xu, Y., Feng, K., Zhao, H., Di, L., Wang, L., and Wang, R. (2022). Tumor-derived extracellular vesicles as messengers of natural products in cancer treatment. Theranostics 12 (4), 1683–1714. doi:10.7150/thno.67775
Yang, X., Wang, S., Zhang, X., Ye, C., Wang, S., and An, X. (2022). Development of PVA-based microsphere as a potential embolization agent. Biomater. Adv. 135, 112677. doi:10.1016/j.msec.2022.112677
Ye, H., Wang, K., Wang, M., Liu, R., Song, H., Li, N., et al. (2019). Bioinspired nanoplatelets for chemo-photothermal therapy of breast cancer metastasis inhibition. Biomaterials 206, 1–12. doi:10.1016/j.biomaterials.2019.03.024
Yin, W., Li, J., Ke, W., Zha, Z., and Ge, Z. (2017). Integrated nanoparticles to synergistically elevate tumor oxidative stress and suppress antioxidative capability for amplified oxidation therapy. ACS Appl. Mater. interfaces 9 (35), 29538–29546. doi:10.1021/acsami.7b08347
Zhang, D.-F., Zhang, H., Shang, Y., and Guo, L. (2011). Stoichiometry-controlled fabrication of CuxS hollow structures with Cu2O as sacrificial templates. Cryst. Growth & Des. 11 (9), 3748–3753. doi:10.1021/cg101283w
Zhang, T., Liu, Z., Tang, W., Zhu, D., Lyu, M., Lam, J. W. Y., et al. (2022). Mitochondria-targeting Type I AIE photosensitizer combined with H2S therapy: Uninterrupted hydroxyl radical generation for enhancing tumor therapy. Nano Today 46, 101620. doi:10.1016/j.nantod.2022.101620
Zhao, J., Ye, H., Lu, Q., Wang, K., Chen, X., Song, J., et al. (2022). Inhibition of post-surgery tumour recurrence via a sprayable chemo-immunotherapy gel releasing PD-L1 antibody and platelet-derived small EVs. J. nanobiotechnology 20 (1), 62. doi:10.1186/s12951-022-01270-7
Zhu, D., Chen, H., Huang, C., Li, G., Wang, X., Jiang, W., et al. (2022b). H2O2 self-producing single-atom nanozyme hydrogels as light-controlled oxidative stress amplifier for enhanced synergistic therapy by transforming “cold” tumors. Adv. Funct. Mater. 32, 2110268. doi:10.1002/adfm.202110268
Zhu, D., Lyu, M., Jiang, W., Suo, M., Huang, Q., and Li, K. (2020). A biomimetic nanozyme/camptothecin hybrid system for synergistically enhanced radiotherapy. J. Mater. Chem. B 8 (24), 5312–5319. doi:10.1039/d0tb00676a
Zhu, D., Zheng, Z., Luo, G., Suo, M., Li, X., Duo, Y., et al. (2021b). Single injection and multiple treatments: An injectable nanozyme hydrogel as AIEgen reservoir and release controller for efficient tumor therapy. Nano Today 37, 101091. doi:10.1016/j.nantod.2021.101091
Zhu, Y., Gong, P., Wang, J., Cheng, J., Wang, W., Cai, H., et al. (2023b). Amplification of lipid peroxidation by regulating cell membrane unsaturation to enhance chemodynamic therapy. Angew. Chem. 62 (12), e202218407. doi:10.1002/anie.202218407
Zhu, Y., Pan, Y., Guo, Z., Jin, D., Wang, W., Liu, M., et al. (2023a). Photothermal enhanced and tumor microenvironment responsive nanozyme for amplified cascade enzyme catalytic therapy. Adv. Healthc. Mater. 12 (7), e2202198. doi:10.1002/adhm.202202198
Zhu, Y., Wang, W., Cheng, J., Qu, Y., Dai, Y., Liu, M., et al. (2021a). Stimuli-responsive manganese single-atom nanozyme for tumor therapy via integrated cascade reactions. Angew. Chem. 60 (17), 9566–9574. doi:10.1002/ange.202017152
Zhu, Y., Wang, Z., Zhao, R., Zhou, Y., Feng, L., Gai, S., et al. (2022a). Pt decorated Ti3C2Tx MXene with NIR-II light amplified nanozyme catalytic activity for efficient phototheranostics. ACS Nano 16 (2), 3105–3118. doi:10.1021/acsnano.1c10732
Keywords: radiotherapy, nanozyme, hydrogel, photothermal therapy, ROS
Citation: Wang S, Fei H, Ma Y, Zhu D, Zhang H, Li X and Huang Q (2023) Cu-doped polypyrrole hydrogel with tumor catalyst activity for NIR-II thermo-radiotherapy. Front. Bioeng. Biotechnol. 11:1225937. doi: 10.3389/fbioe.2023.1225937
Received: 20 May 2023; Accepted: 23 June 2023;
Published: 07 July 2023.
Edited by:
Long Bai, East China University of Science and Technology, ChinaReviewed by:
Jiulong Zhao, Naval Medical University, ChinaHao Ye, ETH Zürich, Switzerland
Yang Zhu, Fuzhou University, China
Copyright © 2023 Wang, Fei, Ma, Zhu, Zhang, Li and Huang. This is an open-access article distributed under the terms of the Creative Commons Attribution License (CC BY). The use, distribution or reproduction in other forums is permitted, provided the original author(s) and the copyright owner(s) are credited and that the original publication in this journal is cited, in accordance with accepted academic practice. No use, distribution or reproduction is permitted which does not comply with these terms.
*Correspondence: Qinqin Huang, qqhuang@zzu.edu.cn; Xiang Li, lixiang_suda@126.com; Hongtao Zhang, zhtzzu@zzu.edu.cn
†These authors have contributed equally to this work