- 1Key Laboratory of Poyang Lake Environment and Resource Utilization, Ministry of Education, and School of Resources and Environment, Nanchang University, Nanchang, China
- 2Faculty of Science and Engineering, Soka University, Tokyo, Japan
Anaerobic digestion piggery effluent (ADPE) with a quite high ammonium (NH4+) concentration and turbidity (dark brown color) generally requires high dilution before microalgae cultivation, owing to its NH4+ toxicity and color inhibition to algal growth. An integrated pretreatment strategy of ammonia stripping and chemical flocculation may be a more practical pretreatment procedure for enhancing algae yield and nutrient recovery from anaerobic digestion piggery effluent. In this study, we determined the optimum pretreatment strategy of anaerobic digestion piggery effluent for subsequent microalgae cultivation and nutrient recovery. The results showed that the integrated anaerobic digestion piggery effluent pretreatment strategy of high-temperature ammonia stripping and chemical flocculation at a mixed dosage of 2 g L−1 polyaluminum chloride (PAC) and 40 mg L−1 cationic polyacrylamide (C-PAM), and 50 mg L−1 ammonium nitrogen (NH4+-N) enrichment provided maximum algal yield (optical density = 1.8) and nutrient removal (95.2%, 98.7%, 99.3%, and 78.5% for the removal efficiencies of total nitrogen, NH4+-N, total phosphorus, and chemical oxygen demand, respectively) from anaerobic digestion piggery effluent. The integrated pretreatment strategy is expected to become a more practical pretreatment procedure for enhancing algae yield and nutrient recovery from anaerobic digestion piggery effluent.
1 Introduction
Large-scale piggery wastewater is commonly treated by an anaerobic digestion (AD) process, which results in the intermittent release of AD piggery effluent (ADPE), specifically comprising high levels of nitrogen, phosphorus, and organic matter (Qian et al., 2022b). If ADPE is directly discharged into natural water bodies, it will lead to serious environmental pollution and ecological risk (Zhao et al., 2022). Microalgae-based wastewater treatment is one of the most promising technologies for advanced AD effluent (ADE) treatment and nutrient recovery (Qian et al., 2022c), owing to fast algal growth, high photosynthetic efficiency, and excellent nutrient uptake. Simultaneously, the harvested algal biomass can be developed for high-value products such as animal feed, biogas, biofuel, and valuable chemicals (Qian et al., 2022a; Chen et al., 2022). However, a quite high ammonium (NH4+) concentration and turbidity (dark brown color) are the current barriers to adopting algal cultivation for ADPE remediation because either of the two factors can inhibit algal growth. Algal cells during photosynthesis can result in a high pH level of ADPE, which can easily shift the chemical equilibrium from NH4+ to free ammonia (NH3) (Ayre et al., 2017). NH3 is toxic to most algal strains by diffusing through the cell membrane and accumulating in the algal cytoplasm because of its uncoupling effects on photosynthesis in chloroplasts (Crofts, 1966; Uggetti et al., 2014). In addition, the dark brown color of ADPE easily obstructs the passage of sunlight from water bodies, thus greatly inhibiting algal photosynthesis and growth (Liu et al., 2015). Therefore, ADPE should be properly pretreated to reduce NH3 toxicity and color inhibition for subsequent microalgae cultivation.
In most previous studies, high-dilution pretreatment of ADPE was the most convenient method to reduce NH4+ toxicity and color inhibition prior to microalgae cultivation. For example, Chlorella vulgaris attained the maximum biomass yield in 50-fold diluted ADPE at an NH4+-N concentration of 100 mg L−1 and chromaticity of 170, but its growth was inhibited in 10- and 20-fold diluted ADPE (Kwon et al., 2020). This might be because the NH4+-N concentration of 110 mg L−1 was critical for NH4+ tolerance in green algae, including Chlorella spp. (Collos and Harrison, 2014). Indeed, the high-dilution pretreatment cannot be widely applied in large-scale microalgae-based wastewater treatment because it needs a huge volume of freshwater. Ammonia stripping is a reliable pretreatment process to maintain the NH4+ level of ADPE below the NH4+ tolerance of microalgae, but the dark color of ADPE still inhibits the growth of microalgae (Li et al., 2019). Chemical flocculation can rapidly remove the color with only a small amount of flocculant, and the process is stable and controllable (El Bied et al., 2021). For example, in the study conducted by Depraetere et al. (2013), 0.2 mM ferric chloride and 200 mg L−1 cationic starch could remove chromaticities of 76% and 73% in piggery wastewater, respectively. Therefore, an integrated pretreatment strategy of ammonia stripping and chemical flocculation may be a feasible procedure to eliminate NH4 toxicity and color inhibition from ADPE for subsequent microalgae cultivation.
This study was conducted to solve the technical problems encountered in ADPE pretreatment for subsequent microalgae cultivation. In this study, the integrated ADPE pretreatment strategy of ammonia stripping and chemical flocculation, and subsequent microalgae cultivation was conducted to optimize the most suitable pretreatment condition for microalgae cultivation. Then, the optimal pretreated ADPE was supplemented with each of the different NH4+-N concentrations and used for microalgae cultivation to further enhance algal yield and nutrient removal from ADPE. The integrated pretreatment strategy is expected to become a more practical pretreatment procedure for enhancing algal yield and nutrient recovery from ADPE.
2 Materials and methods
2.1 ADPE preparation and flocculants
ADPE was obtained from an anaerobic digester of the Zhenghe Biogas Plant in Xinyu City, Jiangxi Province, China. The plant collected piggery manure to generate biogas and ADPE through a continuous stirred tank reactor (CSTR). All the collected ADPEs were filtered through a solid–liquid centrifugal separator (LLW800, KAIDI, Suzhou, China) to remove large particles and stored at 4°C before use. Polyaluminum chloride (PAC) and cation polyacrylamide (C-PAM) as flocculants were purchased from Gongyi Xinqi Polymer Co., Ltd., China.
2.2 High-temperature ammonia stripping device
The high-temperature ammonia stripping device mainly consisted of a stripping tower, circulating pump, water tank, air-blower, heating unit, and electronic control unit (Supplementary Figure S1). The stripping tower was made of double-layer stainless steel with an inner diameter of 154 mm, a height of 1,500 mm, and a working volume of 10 L. A porous material was filled inside the stripping tower to adsorb NH3. ADPE was pumped from the water tank along with the pipeline to the top of the inner layer of the stripping tower using the circulating pump at a constant flow rate and then sprayed downward using a nozzle to fully contact the porous material. The outer layer of the stripping tower was filled with 70°C hot water, which was supplied by a heating water tank. Compressed air from the air-blower was injected into the bottom of the stripping tower through a porous diffuser, and the airflow was controlled by a flowmeter. ADPE in the stripping tower was circulated from the bottom back to the water tank, which was equipped with a blender to homogeneously mix ADPE. Ammonia stripped from the top of the stripping tower was collected and absorbed by 10% (v/v) sulfuric acid (H2SO4) solution to recover ammonium sulfate, which was used as supplementation of the culture medium for subsequent microalgae cultivation.
2.3 Algal strain and pre-culture conditions
One algal strain used for ADPE remediation was isolated from a local water body surrounding the Nanchang Maiyuan Landfill Plant and named Nanchang University (NCU)-7, which was identified as Chlorella sorokiniana, based on our previous methods (Li et al., 2022). C. sorokiniana has been widely applied in wastewater remediation because it can achieve high biomass production and nutrient removal in mixotrophic cultivation (León-Vaz et al., 2019; Qian et al., 2020; Qian et al., 2021).
Algal cells were maintained with a 100-mL TAP medium (Supplementary Table S1) in a 250-mL Erlenmeyer flask. They were pre-cultured at 28°C ± 1°C with a continuous light intensity of 3,000 lux and set on an orbital shaker (TS-2102GZ, Tensuc, Shanghai, China) at a rotation speed of 120 rpm.
2.4 Cultivation of microalgae
Algal cells in the TAP medium were centrifuged at 6,000 rpm for 5 min to remove the supernatant, and the residual algal pellets were washed twice and resuspended in distilled water to prevent the influence of the nutrients from the medium. Subsequently, the algal suspension was inoculated into 200 mL culture medium (appropriately treated ADPE) at an initial optical density (OD) of ca. 0.5 in a 500-mL flask and cultivated at the same temperature, light, and rotation speed conditions as those in the algal pre-culture.
2.5 Experimental design
2.5.1 ADPE pretreatment by high-temperature ammonia stripping
A total volume of 40-L ADPE was first pretreated using the high-temperature ammonia stripping device to reduce the ammonium concentration. The ADPE was circulated at a flow rate of 500 mL min−1 and three cycles with a total stripping period of 4 h. The airflow rate was set to 15 m3 h−1. After ammonia stripping, the pretreated ADPE was collected for further experiments.
2.5.2 Flocculation pretreatment for microalgae cultivation
The pretreated ADPE by ammonia stripping was used in the flocculation experiment. The gradient concentrations of PAC (2, 4, 6, and 8 g L−1) and C-PAM (40, 80, and 120 mg L−1) were fully mixed in ADPE for flocculation pretreatment, and the mixed concentration in each treatment is listed in Table 1. Subsequently, the flocculation sediment in each treatment was removed by centrifugation at 2,000 rpm for 2 min to obtain the supernatant, which was used as the culture medium for microalgae cultivation to determine the optimum pretreatment for enhancing algal yield. Simultaneously, ADPE with ammonia stripping pretreatment but without flocculation was used as a control.
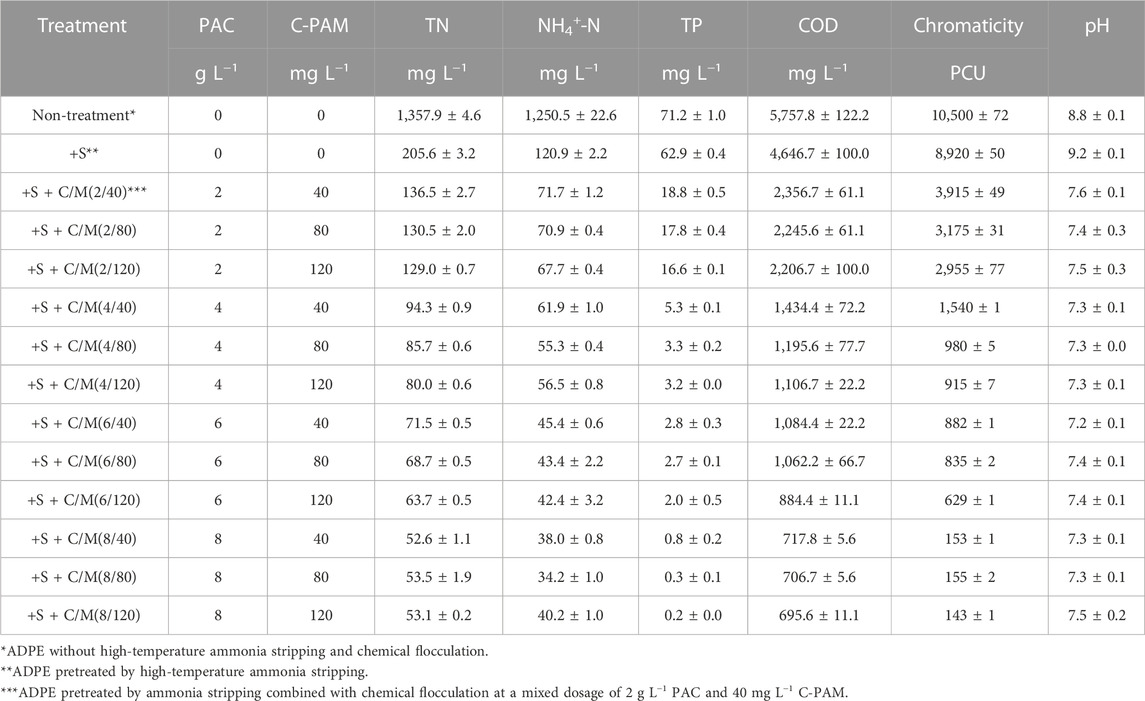
TABLE 1. Chromaticity, pH, and the concentrations of total nitrogen (TN), ammonium nitrogen (NH4+-N), total phosphorus (TP), and chemical oxygen demand (COD) in untreated ADPE; ADPE pretreated by high-temperature ammonia stripping; and ADPE pretreated by the ammonia stripping combined with different chemical flocculant dosages (the different mixed dosages of polyaluminum chloride (PAC) and cationic polyacrylamide (C-PAM)). Data are reported as the mean ± standard deviation (n = 3).
2.5.3 Microalgae cultivation in the optimum pretreated ADPE enriched with different NH4+-N concentrations
To further enhance the algal yield and nutrient removal from ADPE, the algal cells were cultured in the optimum pretreated ADPE enriched with NH4+-N concentrations of 50, 100, and 200 mg L−1. The optimum pretreated ADPE without NH4+-N enrichment was used as a control. These NH4+-N concentrations were provided by the recovered ammonium sulfate from the ammonia stripping device.
2.6 Analytical methods
2.6.1 Algal growth and water quality analysis
OD as algal yield was measured at 680 nm using a spectrophotometer (DR 6000, Hach, Loveland, United States) to determine the growth of microalgae. Chlorophyll a in algal cells was measured based on a previous study (Song et al., 2020).
pH and chromaticity (unit: PCU) were measured using a portable pH meter (PB-10, Sartorius, Beijing, China) and colorimeter (SD9011B, XINRUI, Shanghai, China), respectively. To determine the water quality characteristics, the collected sample was filtered through a 0.45-µm pore-size syringe filter (PES, Jinteng, Tianjin, China) to remove suspended materials. The filtrate was analyzed using a Hach kit (DR 6000 Hach, Loveland, United States) to determine the concentrations of the chemical oxygen demand (COD), total nitrogen (TN), NH4+-N, and total phosphorus (TP). The removal efficiencies (R, %) of nutrients (COD, TN, NH4+-N, and TP) were calculated using the following equation:
where C0i and Cfi represent the concentrations (mg L−1) of nutrient i on the initial and final days of the experimental period, respectively.
2.6.2 Three-dimensional fluorescence excitation–emission matrix (3D-EEM) analysis
The EEM spectra of the ADPE samples were determined by fluorescence spectroscopy (F97pro, Lengguang Tech, Shanghai, China) with a scanning emission (Em) wavelength from 300 to 600 nm (1-nm increment) by increasing the excitation (Ex) wavelength from 300 to 500 nm (5-nm increments).
3 Results and discussion
3.1 ADPE pretreated by the integrated strategy and corresponding EEM analysis
In untreated ADPE, chromaticity, TN, NH4+-N, TP, and COD concentrations were 10,500 PCU, 1,357.9, 1,250.5, 71.2, and 5,757.8 mg L−1, respectively, while they decreased to 8,920 PCU, 205.6, 120.9, 62.9, and 4,646.7 mg L−1 after high-temperature ammonia stripping pretreatment (+s), respectively (Table 1). The slight decrease in COD and chromaticity may be because the volatile organic compounds escape from ADPE through heat degradation and CH4 production (Meng et al., 2023). The NH4+-N content sharply declined, but chromaticity was still quite high after ammonia stripping. However, after the integrated pretreatment of ammonia stripping combined with chemical flocculation, chromaticity, TN, NH4+-N, TP, and COD concentrations further reduced to 2,950–3,920 PCU, 129–137, 67–72, 16–19, and 2,200–2,360 mg L−1 in +S + C/M(2/40-120) treatments, respectively (e.g., +C/M(2/40) represented chemical flocculation at a mixed dosage of 2 g L−1 PAC and 40 mg L−1 C-PAM). With the increasing PAC supplementation amounts from 4 to 8 g L−1, they further declined to 910–1,550 PCU, 80–95, 55–62, 3.2–5.3, and 1,100–1,440 mg L−1 in +S + C/M(4/40-120) treatments; 620–890 PCU, 63–72, 42–46, 2.0–2.8, and 880–1,090 mg L−1 in +S + C/M(6/40-120) treatments; and 140–155 PCU, 52–54, 34–41, 0.2–0.8, and 690–720 mg L−1 in +S + C/M(8/40-120) treatments. These results suggested that the chemical flocculation of PAC and C-PAM addition could not only effectively reduce COD and chromaticity from ADPE but also remove nutrients including nitrogen and phosphorus. Compared to the C-PAM dosage, the increases in PAC dosage could more effectively remove TN, NH4+-N, TP, COD, and chromaticity from ADPE. Furthermore, the increases in PAC dosage had a greater removal effect on COD and chromaticity than TN, NH4+-N, and TP. These results were confirmed by the study conducted by Harif et al. (2023).
It could be found that the color removal effect of the flocculant on ADPE was not good enough when PAC dosage was low, the formed floc was small and suspended in ADPE, and the floc sedimentation performance was poor (Supplementary Figure S2). With the increases in PAC dosages, floc formation was accelerated in ADPE, the floc volume became larger, and the sedimentation performance of floc improved, corresponding to the enhancement of color removal. As known, the flocculation of PAC is mainly related to the charge neutralization process or the adsorption and precipitation of metal hydroxide. The deposition of hydroxyl radicals leads to the possibility of sweep flocculation, in which the impurity particles are involved in the growing sediment, thus being effectively removed (Duan and Gregory, 2003). The addition of PAC to wastewater would destroy the stability of colloidal materials and make small particles agglomerate into large settleable flocs (Wei et al., 2016).
3D-EEM spectroscopy was used to evaluate the structure characterizations of organic matter in ADPE before and after ammonia stripping combined with chemical flocculation pretreatments. Peak A was only identified from ADPE in non-treatment and +s treatment at Ex/Em 390–430/450–500 nm (Figures 1A, B), while peaks B and C were detected in +S + C/M(2/40) treatment at Ex/Em 350–380/430–460 nm and 380–410/460–490 nm, respectively (Figure 1C). These three peaks were all described as polycarboxylate-type humic acid (Wang and Zhang, 2010; Qian et al., 2023).
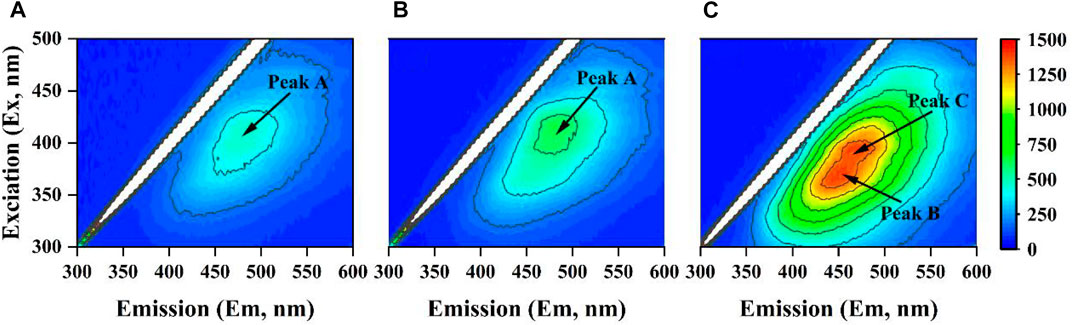
FIGURE 1. Three-dimensional excitation—emission matrix (EEM) spectra of untreated ADPE (A), ADPE pretreated by high-temperature ammonia stripping (B), and ADPE pretreated by high-temperature ammonia stripping and chemical flocculation (C) [where the mixed dosages of flocculants were 2 g L−1 polyaluminum chloride (PAC) and 40 mg L−1 cation polyacrylamide (C-PAM)].
3.2 Microalgae cultivation in pretreated ADPEs by the integrated strategy
Algal growth was inhibited in +S treatment without flocculation (Figure 2) probably because the extremely high chromaticity (ca. 9,000 PCU) still existed in the pretreated ADPE. Very high chromaticity easily leads to the light reduction received by microalgae and inhibits the synthesis of various compounds in algal cells (Maltsev et al., 2021). ODs as algal yields were the highest in +S + C/M(2/40-120) treatment, being ca. 1.6, on the final day among the treatment, followed by ca. 1.3 in +S + C/M(4/40-120) treatment and ca. 1.1 in +S + C/M(6 and 8/40-120) treatment. This suggested that ADPEs in +S + C/M(2/40-120) treatment with a PAC supplementation of 2 g L−1 were more suitable media for microalgae cultivation, maybe due to a large amount of phosphorus existing in the media (Table 1) compared to the other treatment procedures. To reduce C-PAM dosage as much as possible for high cost efficiency, adding the mixed supplementation of 2 g L−1 PAC and 40 mg L−1 C-PAM to the ammonia stripping-pretreated ADPE (i.e., +S + C/M(2/40) treatment) was the most suitable pretreatment for enhancing algal yield from ADPE. Wang et al. (2020) also conducted a similar study, which reported that the COD, total Kjeldahl nitrogen (TKN), and TP contents in dairy manure wastewater were removed by 72.6%, 58.7%, and 43.0% in the optimal flocculation–centrifuge pretreatment, respectively, and their content in the two-fold diluted manure supernatant from this pretreatment was further reduced by 82.2%, 90.1%, and 83.4%, respectively, with a harvested algal yield of ca. 1.2 g L−1 during culturing C. vulgaris UTEX-2714. These results confirmed that the integrated pretreatment strategy of ammonia stripping and chemical flocculation was a reliable procedure to avoid NH3 toxicity and color inhibition from ADPE for subsequent microalgae cultivation.
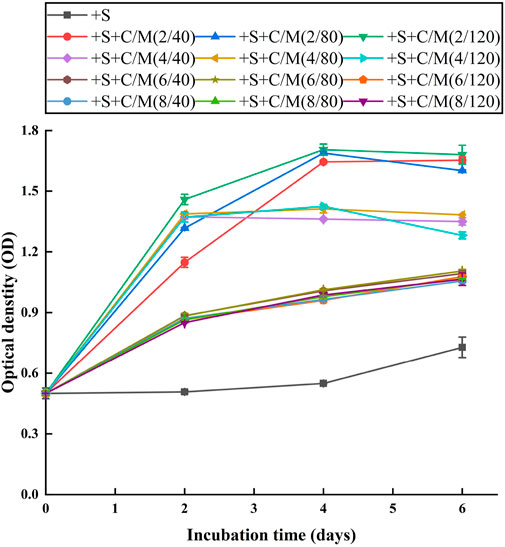
FIGURE 2. Growth curves of NCU-7 in different treatments (detailed information on each treatment is shown in Table 1). Error bars represent standard deviations (n = 3).
3.3 Microalgae cultivation in pretreated ADPEs enriched with different NH4+-N concentrations
Based on the most suitable pretreatment, +S + C/M(2/40)-pretreated ADPEs were enriched with different NH4+-N concentrations and used as culture media for microalgae cultivation to further enhance algal yield and nutrient removal from ADPE. These NH4+-N concentrations were provided by recovered ammonium sulfate from the ammonia stripping device to achieve nitrogen resource recycling and utilization.
Growth curves of NCU-7 showed similar trajectories among all treatments, but the final algal yields were quite different. OD was ca. 1.8 in the 50-mg NH4+-N treatment, being the highest among the treatments, followed by 1.6 in no enrichment and 100-mg NH4+-N treatment (Figure 3A). However, the lowest algal yield (OD = 1.5) was obtained in the 200-mg NH4+-N treatment. The trends of algal yields among the treatments were similar to those of chlorophyll a (Figure 3B). These results suggested that algal growth might be inhibited in 100- and 200-mg NH4+-N treatments compared to the 50-mg NH4+-N treatment. Therefore, the 50-mg NH4+-N enrichment was a suitable treatment for microalgae cultivation.
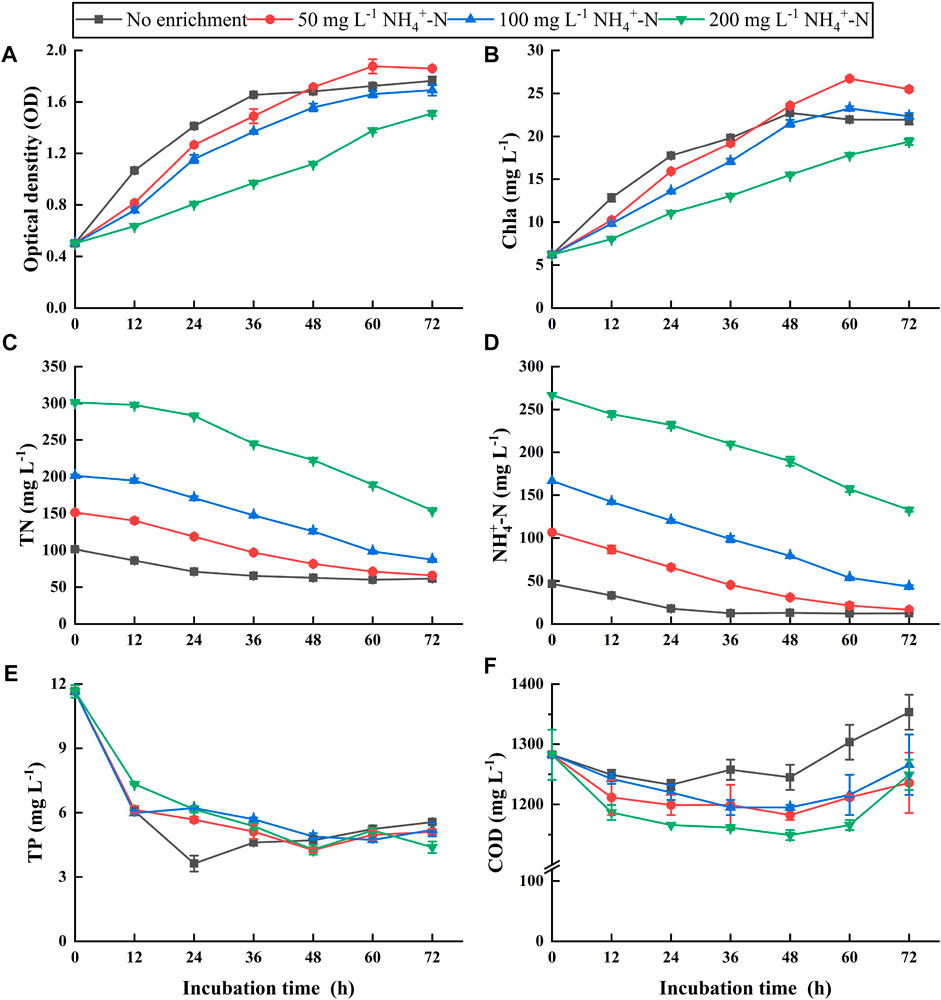
FIGURE 3. Algal growth curves (A), chlorophyll a (B), and the concentrations of total nitrogen (TN) (C), ammonium nitrogen (NH4+-N) (D), total phosphorus (TP) (E), and chemical oxygen demand (COD) (F) in the optimum pretreated ADPE enriched with NH4+-N concentrations of 50, 100, and 200 mg L−1 (“the optimum pretreated ADPE” represents “ADPE was pretreated by the ammonia stripping combined with chemical flocculation at a mixed dosage of 2 g L−1 PAC and 40 mg L−1 C-PAM”). Error bars represent standard deviations (n = 3).
TN, NH4+-N, and TP concentrations in all treatments decreased with increasing incubation times, regardless of the initial concentrations. At the end of the experiment, 50-, 100-, and 200-mg NH4+-N treatments, and no enrichment removed 85.5, 114.1, 133.8, and 34.3 mg L−1 of TN concentrations (Figure 3C), respectively, in response to NH4+-N removal of 90.2, 123.1, 147.3, and 39.8 mg L−1, respectively (Figure 3D). It has been known that high pH levels above 8.0 favor NH4+ volatilization by converting into ammonia (Escudero et al., 2014). Therefore, this TN/NH4+-N removal might include partial volatilization losses during the experiment due to pH levels of 9.0–11.0 (data not shown). TP concentrations decreased to almost the same level in all treatments (Figure 3E). pH levels above 7.0 contributed to enhancing phosphorus removal from wastewater through phosphate precipitation with metals, such as calcium phosphate and/or struvite (Moutin et al., 1992; Le Corre et al., 2009). The level of algal yields in the 50-mg NH4+-N treatment was significantly higher than that in the 100- and 200-mg NH4+-N treatments despite a lower TN/NH4+-N removal amount in the 50-mg NH4+-N treatment and similar levels of TP removal amounts among the three treatments. This suggested that relatively high nitrogen and phosphorus removal was assimilated into the algal biomass in the 50-mg NH4+-N treatment. COD concentrations gradually decreased until 48 h but increased after that point (Figure 3F). The decrease was ascribed to algal heterotrophy, while the increase might be related to algal excretion (Zhuang et al., 2016). Based on the initial nutrient concentrations in raw ADPE without pretreatment, TN, NH4+-N, TP, and COD in the suitable 50-mg NH4+-N treatment with high algal yields achieved high removal efficiencies of 95.2%, 98.7%, 99.3%, and 78.5%, respectively.
4 Conclusion
The integrated ADPE pretreatment strategy of high-temperature ammonia stripping and chemical flocculation at a mixed dosage of 2 g L−1 PAC and 40 mg L−1 C-PAM, and 50 mg L−1 NH4+-N enrichment provided the maximum algal yield (OD = 1.8) and nutrient removal (95.2%, 98.7%, 99.3%, and 78.5% for TN, NH4+-N, TP, and COD removal efficiencies, respectively) from ADPE. The integrated pretreatment strategy could be a promising approach to algal biomass production and nutrient removal from ADPE.
Data availability statement
The original contributions presented in the study are included in the article/Supplementary Material; further inquiries can be directed to the corresponding authors.
Author contributions
JQ: conceptualization, investigation, methodology, formal analysis, data curation, writing—review and editing, and funding acquisition. CX: conceptualization, investigation, methodology, formal analysis, data curation, and writing—review and editing. HS: writing—review and editing. WZ: writing—review and editing, and funding acquisition. TT: writing—review and editing. HL: writing—review and editing. YT: writing—review and editing. MS: writing—review and editing. SK: writing—review and editing. JuL: conceptualization, writing—review and editing, and funding acquisition. JiL: conceptualization, and writing—review and editing. All authors contributed to the article and approved the submitted version.
Funding
This study was supported by the Key Research and Development Program of Jiangxi Province of China (20182ABC28006, 20212BBF61010, and 20214BBG74004) and the National Natural Science Foundation of China (32160867 and 31960734).
Conflict of interest
The authors declare that the research was conducted in the absence of any commercial or financial relationships that could be construed as a potential conflict of interest.
Publisher’s note
All claims expressed in this article are solely those of the authors and do not necessarily represent those of their affiliated organizations, or those of the publisher, the editors, and the reviewers. Any product that may be evaluated in this article, or claim that may be made by its manufacturer, is not guaranteed or endorsed by the publisher.
Supplementary material
The Supplementary Material for this article can be found online at: https://www.frontiersin.org/articles/10.3389/fbioe.2023.1219103/full#supplementary-material
References
Ayre, J. M., Moheimani, N. R., and Borowitzka, M. A. (2017). Growth of microalgae on undiluted anaerobic digestate of piggery effluent with high ammonium concentrations. Algal Res. 24, 218–226. doi:10.1016/j.algal.2017.03.023
Chen, F., Qian, J., He, Y., Leng, Y., and Zhou, W. (2022). Could Chlorella pyrenoidosa be exploited as an alternative nutrition source in aquaculture feed ? A study on the nutritional values and anti-nutritional factors. Front. Nutr. 9, 1069760.
Collos, Y., and Harrison, P. J. (2014). Acclimation and toxicity of high ammonium concentrations to unicellular algae. Mar. Pollut. Bull. 80, 8–23. doi:10.1016/j.marpolbul.2014.01.006
Crofts, A. R. (1966). Uptake of ammonium ion by chloroplasts, and the mechanism of amine uncoupling. Biochem. Biophys. Res. Commun. 24, 127–134. doi:10.1016/0006-291X(66)90420-7
Depraetere, O., Foubert, I., and Muylaert, K. (2013). Decolorisation of piggery wastewater to stimulate the production of Arthrospira platensis. Bioresour. Technol. 148, 366–372. doi:10.1016/j.biortech.2013.08.165
Duan, J., and Gregory, J. (2003). Coagulation by hydrolysing metal salts. Adv. Colloid Interface Sci. 100–102, 475–502. doi:10.1016/S0001-8686(02)00067-2
El Bied, O., Kessler, M., Terrero, M. A., Fechtali, T., Cano, A. F., and Acosta, J. A. (2021). Turbidity and chemical oxygen demand reduction from pig slurry through a coagulation flocculation process. Agronomy 11, 2158. doi:10.3390/agronomy11112158
Escudero, A., Blanco, F., Lacalle, A., and Pinto, M. (2014). Ammonium removal from anaerobically treated effluent by Chlamydomonas acidophila. Bioresour. Technol. 153, 62–68. doi:10.1016/j.biortech.2013.11.076
Harif, S., Aboulhassan, M. A., and Bammou, L. (2023). Multi-response optimization for color removal from cardboard wastewater using polyaluminum chloride and cationic polyacrylamide. Int. J. Environ. Sci. Technol. 20, 4281–4292. doi:10.1007/s13762-022-04267-9
Kwon, G., Nam, J. H., Kim, D. M., Song, C., and Jahng, D. (2020). Growth and nutrient removal of Chlorella vulgaris in ammonia-reduced raw and anaerobically-digested piggery wastewaters. Environ. Eng. Res. 25, 135–146. doi:10.4491/eer.2018.442
Le Corre, K. S., Valsami-Jones, E., Hobbs, P., and Parsons, S. A. (2009). Phosphorus recovery from wastewater by struvite crystallization: A review. Crit. Rev. Environ. Sci. Technol. 39, 433–477. doi:10.1080/10643380701640573
León-Vaz, A., León, R., Díaz-Santos, E., Vigara, J., and Raposo, S. (2019). Using agro-industrial wastes for mixotrophic growth and lipids production by the green microalga Chlorella sorokiniana. N. Biotechnol. 51, 31–38. doi:10.1016/j.nbt.2019.02.001
Li, J., Qian, J., Tang, J., Jin, Z., Lu, Q., Cheng, J., et al. (2022). Enhancement of ammonium removal from landfill leachate using microalgae by an integrated strategy of nutrient balance and trophic mode conversion. Algal Res. 61, 102572. doi:10.1016/j.algal.2021.102572
Li, J., Wang, L., Lu, Q., and Zhou, W. (2019). Toxicity alleviation for microalgae cultivation by cationic starch addition and ammonia stripping and study on the cost assessment. RSC Adv. 9, 38235–38245. doi:10.1039/c9ra03454d
Liu, J., Song, Y., Liu, Y., and Ruan, R. (2015). Fungal pretreatment of effluent from piggery anaerobic digestion by Phanerochaete chrysosporium. Clean-Soil Air Water 43, 1190–1196. doi:10.1002/clen.201400561
Maltsev, Y., Maltseva, K., Kulikovskiy, M., and Maltseva, S. (2021). Influence of light conditions on microalgae growth and content of lipids, carotenoids, and fatty acid composition. Biol. (Basel) 10, 1060. doi:10.3390/biology10101060
Meng, X., Zhu, M., Cai, Y., Wang, Q., Liu, W., and Ren, L. (2023). The greenhouse gas emission potential and phytotoxicity of biogas slurry in static storage under different temperatures. Environ. Sci. Pollut. Res., 46257–46269. doi:10.1007/s11356-023-25645-x
Moutin, T., Gal, J. Y., El Halouani, H., Picot, B., and Bontoux, J. (1992). Decrease of phosphate concentration in a high rate pond by precipitation of calcium phosphate: Theoretical and experimental results. Water Res. 26, 1445–1450. doi:10.1016/0043-1354(92)90063-A
Qian, J., Chen, F., and Zhou, W. (2022a). Advancements of application of microalgae biotechnology in the aquaculture water quality control. Amsterdam, Netherlands: Elsevier Inc. doi:10.1016/bs.aibe.2022.05.002
Qian, J., Liu, X., Ban, S., Fujiwara, M., Kodera, T., Akizuki, S., et al. (2020). pH treatments in continuous cultivation to maximize microalgal production and nutrient removal from anaerobic digestion effluent of aquatic macrophytes. J. Appl. Phycol. 32, 3349–3362. doi:10.1007/s10811-020-02196-z
Qian, J., Shimotori, K., Liu, X., Ban, S., Akizuki, S., Fujiwara, M., et al. (2021). Enhancement of algal growth by Mg2+ released from anaerobic digestion effluent of aquatic macrophytes through photolysis. Biochem. Eng. J. 172, 108065. doi:10.1016/j.bej.2021.108065
Qian, J., Wan, T., Ye, Y., Li, J., Toda, T., Li, H., et al. (2023). Insight into the formation mechanism of algal biofilm in soy sauce wastewater. J. Clean. Prod. 394, 136179. doi:10.1016/j.jclepro.2023.136179
Qian, J., Zhang, J., Jin, Z., Cheng, J., Li, J., Song, H., et al. (2022b). Enhancing algal yield and nutrient removal from anaerobic digestion piggery effluent by an integrated process-optimization strategy of fungal decolorization and microalgae cultivation. Apllied Sci. 12, 4714. doi:10.3390/app12094741
Qian, J., Zhang, X., Cheng, J., Jin, Z., and Zhu, H. (2022c). Enhancing algal growth and nutrient removal from nitrified anaerobic digestion piggery effluent through process optimization. Algal Res. 65, 102746. doi:10.1016/j.algal.2022.102746
Song, C., Liu, Z., Wang, C., Li, S., and Kitamura, Y. (2020). Different interaction performance between microplastics and microalgae: The bio-elimination potential of Chlorella sp. L38 and Phaeodactylum tricornutum MASCC-0025. Sci. Total Environ. 723, 138146. doi:10.1016/j.scitotenv.2020.138146
Uggetti, E., Sialve, B., Latrille, E., and Steyer, J. P. (2014). Anaerobic digestate as substrate for microalgae culture: The role of ammonium concentration on the microalgae productivity. Bioresour. Technol. 152, 437–443. doi:10.1016/j.biortech.2013.11.036
Wang, L., Chen, L., and Wu, S. (2020). Nutrient reduction of dairy manure through solid-liquid separation with flocculation and subsequent microalgal treatment. Appl. Biochem. Biotechnol. 190, 1425–1437. doi:10.1007/s12010-019-03185-w
Wang, Z. P., and Zhang, T. (2010). Characterization of soluble microbial products (SMP) under stressful conditions. Water Res. 44, 5499–5509. doi:10.1016/j.watres.2010.06.067
Wei, Y., Dong, X., Ding, A., and Xie, D. (2016). Characterization and coagulation-flocculation behavior of an inorganic polymer coagulant - poly-ferric-zinc-sulfate. J. Taiwan Inst. Chem. Eng. 58, 351–356. doi:10.1016/j.jtice.2015.06.004
Zhao, G., Wang, X., Hong, Y., Liu, X., Wang, Q., Zhai, Q., et al. (2022). Attached cultivation of microalgae on rational carriers for swine wastewater treatment and biomass harvesting. Bioresour. Technol. 351, 127014. doi:10.1016/j.biortech.2022.127014
Keywords: anaerobic digestion piggery effluent, ammonia stripping, chemical flocculation, microalgae cultivation, nutrient recovery
Citation: Qian J, Xu C, Song H, Zhou W, Toda T, Li H, Takayama Y, Sekine M, Koga S, Li J and Liu J (2023) Enhancing algal growth and nutrient recovery from anaerobic digestion piggery effluent by an integrated pretreatment strategy of ammonia stripping and flocculation. Front. Bioeng. Biotechnol. 11:1219103. doi: 10.3389/fbioe.2023.1219103
Received: 08 May 2023; Accepted: 23 May 2023;
Published: 29 June 2023.
Edited by:
Qian Lu, Jiangsu University of Science and Technology, ChinaReviewed by:
Libin Yang, Tongji University, ChinaGao Huanan, Tsinghua University, China
Xiangyuan Deng, Jiangsu University of Science and Technology, China
Copyright © 2023 Qian, Xu, Song, Zhou, Toda, Li, Takayama, Sekine, Koga, Li and Liu. This is an open-access article distributed under the terms of the Creative Commons Attribution License (CC BY). The use, distribution or reproduction in other forums is permitted, provided the original author(s) and the copyright owner(s) are credited and that the original publication in this journal is cited, in accordance with accepted academic practice. No use, distribution or reproduction is permitted which does not comply with these terms.
*Correspondence: Jun Li, lijunj2j@ncu.edu.cn; Jin Liu, gjinliu@ncu.edu.cn
†These authors have contributed equally to this work