- Nursing Teaching and Research Department, The Fourth Affiliated Hospital of China Medical University, Shenyang, China
In the past few decades, great progress has been made in the field of nanomaterials against bacterial infection. However, with the widespread emergence of drug-resistant bacteria, people try their best to explore and develop new antibacterial strategies to fight bacteria without obtaining or increasing drug resistance. Recently, multi-mode synergistic therapy has been considered as an effective scheme for the treatment of bacterial infections, especially the combination of photothermal therapy (PTT) and photodynamic therapy (PDT) with controllable, non-invasive, small side effects and broad-spectrum antibacterial characteristics. It can not only improve the efficiency of antibiotics, but also do not promote antibiotic resistance. Therefore, multifunctional nanomaterials which combine the advantages of PTT and PDT are more and more used in the treatment of bacterial infections. However, there is still a lack of a comprehensive review of the synergistic effect of PTT and PDT in anti-infection. This review first focuses on the synthesis of synergistic photothermal/photodynamic nanomaterials and discusses the ways and challenges of photothermal/photodynamic synergism, as well as the future research direction of photothermal/photodynamic synergistic antibacterial nanomaterials.
1 Introduction
Bacterial infection is a very common disease in people’s daily life, which is a serious threat to human health (Wu et al., 2018; Liu et al., 2019; Wu et al., 2019). Antibiotics are the main clinical treatment for pathogenic bacterial infections. However, in recent decades, due to unwisdom or abuse of antibiotics, drug-sensitive bacteria continue to mutate, leading to the emergence and prevalence of bacterial drug resistance (Crofts et al., 2017; Gross et al., 2019; Scheres and Kuszewski, 2019; Shang et al., 2020). It is necessary to focus on the development of treatments that can quickly and effectively overcome pathogenic bacteria without producing drug resistance (Laxminarayan et al., 2013; Wang et al., 2019; Sun et al., 2019; Yuan et al., 2020).
With the development of nanomedicine, more and more nanomaterials and new antibacterial therapy are used in antibacterial therapy (Huang et al., 2009; Shan et al., 2019; Yang et al., 2019; Murugesan and Scheibel, 2020). For example, silver nanoparticles (Zawadzka et al., 2021), metal oxides (Shi et al., 2018; Xie et al., 2020), carbon-based materials (Xi et al., 2019), and metal-organic frameworks (MOF) (Liu et al., 2019; Tao et al., 2023) have broad-spectrum antibacterial activity and can be sterilized by physical or chemical methods. Emerging antimicrobial methods, including PTT, PDT, and chemodynamic therapy, have recently been identified as effective antimicrobial methods and have attracted great attention in anti-infective therapy (Wang et al., 2020; Wu et al., 2023). Among them, compared with other treatments, phototherapy is favored because of its controllable, non-invasive, few side effects and broad-spectrum antibacterial properties (Liu et al., 2017, Liu, Guo, Li, Xiao, Zhang, Bu, Jia, Zhe, Wang and interfaces 2019; Ma et al., 2020). PTT uses photothermal agent near infrared (NIR) light to convert into local high temperature, thus destroying the cell membrane and denaturing bacterial proteins, thus achieving bacterial death (Mao et al., 2017; Wang et al., 2019; Qing et al., 2019). PDT uses photosensitizers to absorb energy under laser irradiation and transfer it to molecular oxygen to produce cytotoxic reactive oxygen species (ROS): hydroxyl radical (OH), superoxide anion (O2−), hydrogen peroxide (H2O2), and singlet oxygen (1O2) (Xia et al., 2017; Sun et al., 2019; Wang et al., 2020b). ROS can oxidize and destroy biomolecules, such as lipids, proteins, and nucleic acids, thus inducing cell apoptosis (Tan et al., 2018).
However, bacterial infection is a very complex process, including initial bacterial adhesion, biofilm formation and infection (Li et al., 2017). Therefore, the use of a single antibacterial method may not be enough, so the nanomaterials combined with a variety of antibacterial methods were studied to enhance the antibacterial effect. For example, hyperthermia and long-term exposure to single-mode PTT therapy may lead to inflammation and thermal damage to nearby normal tissue (Rizwan et al., 2014; Richter and Kietzmann, 2016; Zhu et al., 2018; Gao et al., 2019). Using single-mode PDT therapy to kill bacteria requires a large amount of ROS; while excessive ROS can damage normal tissue by inducing inflammation and necrosis (Choi et al., 2009; Ma et al., 2022; Wu et al., 2022). In addition, the short life of ROS will limit the role of PDT. Integrating PTT and PDT on a single platform can bring the advantages of both high fever and ROS to the treatment of infected sites under light irradiation, overcoming the inherent limitations of a single PTT or PDT (Mao et al., 2018; Jiang et al., 2020; Wang et al., 2021). PTT/PDT synergistic therapy shows great potential in overcoming the shortcomings of individual therapies to achieve enhanced antibacterial properties (Zhang et al., 2022). Based on the fact that the complex interactions between host and bacteria during bacterial infection lead to specific microenvironments, including low pH, hypoxia, toxins, enzymes and so on, scientists have developed multi-functional synergistic nanomaterials with the responsiveness of bacterial infection microenvironment (Koo et al., 2017; Lv et al., 2020; Li et al., 2022).
This review introduces the latest progress of various nanomaterials used in the combination of PDT and PTT in the treatment of bacterial infections, such as hydrogels, multifunctional nanoplatforms, fiber membranes, nanosheets, and other nanomaterials, with emphasis on the loading and pathways of the materials driving the action of PDT and PTT, as shown in Figure 1. The recent overview of PDT and PTT strategies for the treatment of bacterial infections can provide clues to multiple ways of synergistic antimicrobial therapy and contribute to the development of new collaborative treatment systems to improve the efficacy of bacterial infection treatment, reduce side effects and avoid drug resistance.
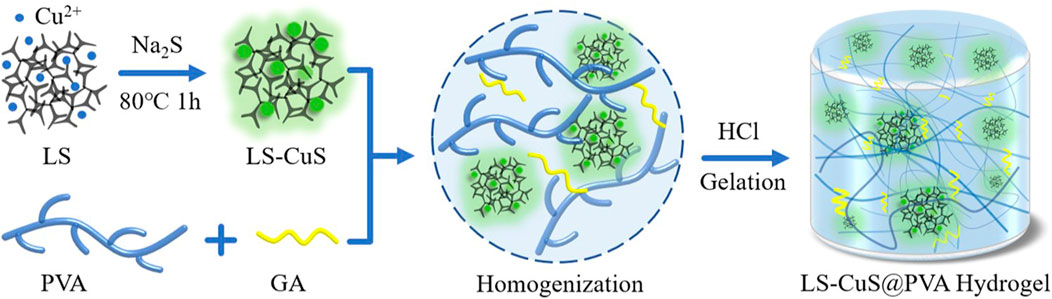
FIGURE 1. Photos of the LS-CuS@PVA hydrogel before and after gelation. Reproduced with permission from Ref (Xie et al., 2022).
2 Hydrogels for antibacterial therapy
Their porosity, biocompatibility, biodegradability, mechanical strength and stability are all adjustable, and provide excellent renewable 3D networks to simulate local tissue (Cheng et al., 2022). The porous structure of hydrogel is usually prepared by electrostatic interaction/hydrogen bond or covalent bond between the polymer chains, and their porosity, biocompatibility, biodegradability, mechanical strength and stability are all adjustable (Khurana et al., 2019; Sang et al., 2020; Maleki et al., 2021; Tao et al., 2022). It is suitable for the research and development of various materials (Liang et al., 2019a).
2.1 CuS -contained photothermal/photodynamic synergistic therapy
Copper sulfide (CuS) can show a significant photodynamic/photothermal effect under near-infrared light, produce hydroxyl radical (OH) in the presence of H2O2, and have excellent peroxidase-like activity. Because of its inherent advantages of near infrared absorption, efficient heat generation, low cost, biodegradability, and high cost, CuS has become an ideal material for antibacterial therapy (Wang et al., 2015; Wang et al., 2020c).
Recently, it has been reported that a LS-CuS@PVA composite hydrogel with near-infrared activated photothermal, photodynamic, and peroxidase-like activity was synthesized by introducing lignin copper sulfide (LS-CuS) nanocomposites into polyvinyl alcohol (PVA) hydrogels. The biodegradability of PVA polymer makes it a suitable scaffold for the construction of multi-functional antibacterial platform. The nano-gel can effectively kill bacteria through the synergistic antibacterial effect of photothermal, photodynamic and peroxidase-like activity, which is attributed to the local heat caused by photothermal effect, which destroys bacteria and makes them sensitive to ROS and accelerates the catalytic reaction to produce more OH in vivo and in vitro. It is proved that LS-CuS@PVA (Figure 1) has good efficacy in the treatment of antibiotic resistant bacteria and can inhibit the formation of biofilm (Xie et al., 2022).
Some CuS-treated hybrid hydrogels were synthesized. Trimethoxysilyl methacrylate (MPS, 97%) and mesoporous silica (MSiO2) modified CuS nanoparticles were synthesized by free radical polymerization. CuS nanoparticles can not only be used as photosensitizer of PTT, but also produce ROS in PDT under near infrared radiation. Under the irradiation of 808 nm near-infrared light, the near-infrared light of the mixed hydrogel is absorbed and converted into heat, then the copper ion formed by CuS NPs dissociation is released, and the OH produced by the reaction between the free carrier and water molecules under near-infrared light. The combined effects of high temperature, free radical oxygen species, and released copper ions under near infrared radiation make it have good antibacterial activity (Li et al., 2018).
The high surface activity of CuS nanoparticles makes it easy to agglomerate in the preparation process. Xiong et al. prepared uniformly dispersed CuS nanoparticles using corn straw as template and stabilizer, and then crosslinked with chitin to prepare CuS@cornstalk/chitin composite hydrogel. Under light, CuS nanoparticles embedded in hydrogel are released while producing light and heat, and hydrogen peroxide is decomposed to form strong oxidant OH, so as to realize the synergistic treatment of PDT and PTT (Xiong et al., 2019).
2.2 MoS2-contained photothermal/photodynamic synergistic therapy
Molybdenum sulfide nanosheets have their elemental abundance, electrochemical stability, high catalytic activity, and unique optical properties, and they have excellent photodetection capabilities in a variety of spectral responses, which can generate hyperthermia and ROS(Mak et al., 2010; Liang et al., 2018).
Zhang et al. prepared a composite hydrogel containing Ag3PO4 and MoS2. Ag3PO4/MoS2 composites were prepared by liquid phase reaction, and then dissolved in PVA to form the final product. Under 660 nm visible light (VL) irradiation, the hydrogel can be triggered to produce more ROS, while under 808 nm near infrared (NIR) irradiation, the hydrogel can produce more heat. Among them, Ag3PO4 can produce a large number of ROS, which can significantly improve the antibacterial activity and reduce the toxicity through the synergistic action of PDT and PTT, which also shows the advantage of synergistic antibacterial activity of PDT and PDT (Figure 2). However, it needs two kinds of light sources to achieve better results, which limits its application (Zhang et al., 2019).
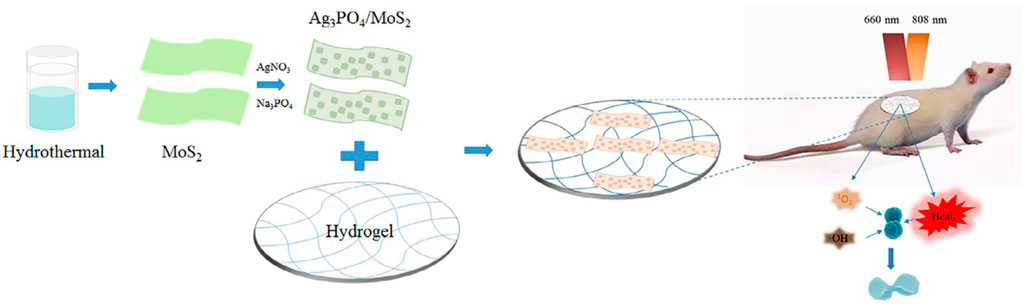
FIGURE 2. Schematic illustration of the synthesis of the Ag3PO4/MoS2 HD and its potential application in treatment of wound infection by the combination of PDT and PTT. Reproduced with permission from Ref (Zhang et al., 2019).
A kind of hydrogel containing CuS and MOS2 is manufactured. The main step is to mix CuS@MoS2 microspheres into porous polyvinyl alcohol (PVA) hydrogels. The hybrid hydrogel reduces the excessive temperature of CuS@MoS2 microspheres under energy light irradiation to about 50 C, and the mixed hydrogel produces thermotherapy and ROS under double light (660 nm + 808 nm) irradiation. Based on the synergistic action of PDT and PTT, 99.3% of Escherichia coli and 99.5% of Staphylococcus aureus were killed in 15min due to the synergistic effect of photodynamic and photothermal antibacterial treatment under the irradiation of 660 nm VL and 808 NIR(Zhang et al., 2020).
2.3 ZnO-contained photothermal/photodynamic synergistic therapy
Zinc oxide not only has good biocompatibility and low cost but also has good performance in anti-inflammatory, antibacterial, antifungal, and other biomedical applications (Hahn et al., 2012; Sehmi et al., 2015; Surendra et al., 2016). Zinc oxide has the ability to produce ROS and can also be used to promote PDT therapy (Xiang et al., 2019; Xiang et al., 2020).
By introducing ZnO quantum dots@GO carbon nanotubes into the hydrogel structure, a zinc oxide quantum dots@GO nanocomposites (NCS) with good antibacterial activity was prepared. Graphene oxide (GO), as a new type of carbon material, has been widely used in the biomedical field because of its high light absorption in the NIR region (Gong et al., 2018; Zhang et al., 2018; Liang et al., 2019b). Under near-infrared light irradiation, GO in the nanocomposite can be used as a photosensitizer for PTT, while zinc ion can inhibit the action of respiratory enzymes and produce ROS, which irreversibly destroys bacterial cell membrane, mitochondria, and DNA, resulting in bacterial cell death, so as to achieve the combined effect of PDT and PTT (Liang et al., 2019a).
Xiang et al. selected zinc ions in transition metal ions, and mixed hydrogels (DFT-hydrogel) were prepared with Folic acid (FA) and dopamine (DA). Firstly, carbon quantum dots (CQD) modified zinc oxide (C/ZnO) composites were selected as functional nanoparticles. PDA can be grafted onto its surface, which has good biocompatibility and excellent photothermal retention (Wu et al., 2018b; Chen et al., 2018; Liu et al., 2018; Nam et al., 2018). Two carboxyl groups in FA molecules or catechol in PDA can easily form metal-ligand coordination with zinc ions to form DFT-C/ZnO-hydrogel. Under the excitation of infrared or visible light, C/ethanol can produce reactive oxygen species (ROS), which can oxidize proteins, phospholipids and DNA/RNA in a very short time to achieve sterilization (Figure 3). In addition, CQD and PDA have good photothermal properties under near-infrared light, which is also helpful to sterilization. Therefore, the double irradiation of 808 nm near infrared light and 660 nm red light can enhance the treatment of PDT and PTT, and significantly improve the rapid antibacterial performance of the hydrogel (Xiang, Mao, Liu, Cui, Jing, Yang, Liang, Li, Zhu and Zheng, 2019).
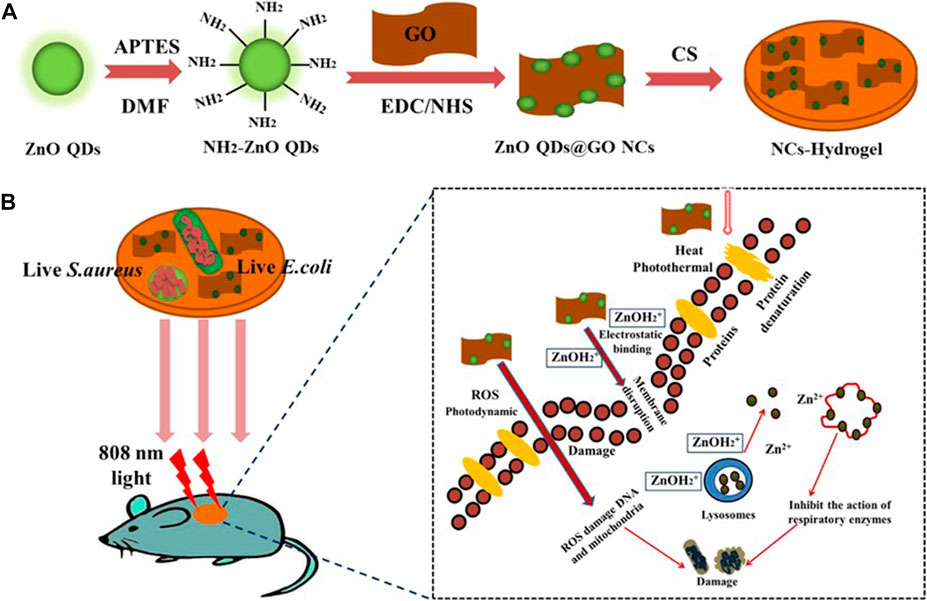
FIGURE 3. Schematic of the synthetic route of (A) ZnO QDs@GO-CS hydrogel and (B) bacteria killing processes. Reproduced with permission from Ref (Liang et al., 2019b).
2.4 Other photothermal/photodynamic synergistic therapy
With the development of nanomedicine, the hydrogel can be used as a platform to explore the research and development of multifunctional therapeutic materials, not only in the treatment of bacterial infection but also in the treatment of tumors. For example, Yin et al. synthesized palladium nanoparticles (PdNPs) with PTT and PDT capabilities (Figure 4). Then the chemotherapeutic drug doxorubicin (DOX) was loaded on Pd nanoparticles to form hydrogel (Pd/DOX@hydrogel). Under the irradiation of near infrared light (808Nm), Pd/DOX@hydrogel produces enough heat to PTT, regulate drug release, and produce ROS, so as to further kill residual cancer cells and prevent wound infection (Fan et al., 2023).
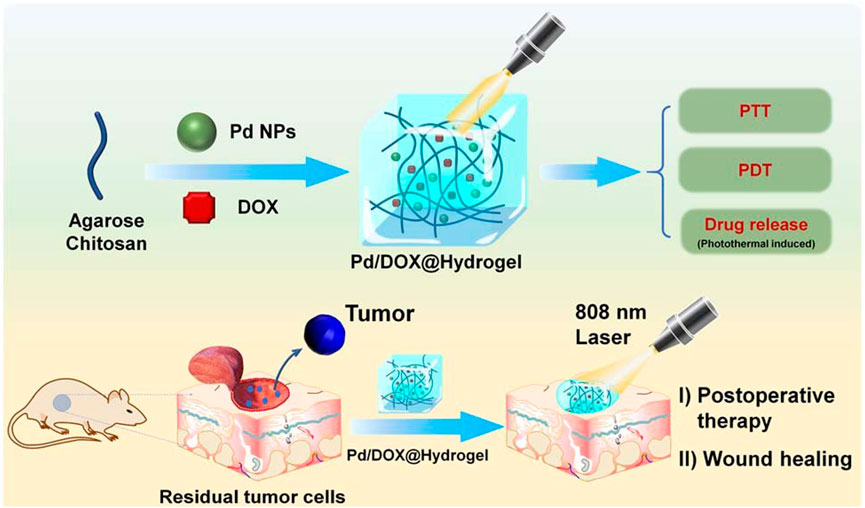
FIGURE 4. Schematic construction of Pd/DOX@hydrogel for post-operative therapy through NIR-light triggered photothermal/photo-dynamic therapy and drug release with wound healing capability. Reproduced with permission from Ref (Fan et al., 2023).
3 Nanoplatforms for antibacterial therapy
Scientists have explored multifunctional materials based on PTT and PDT antibacterial models, such as metal materials carbon nanomaterials (carbon nanotubes, graphene, carbon dots, and nanocrystals), aggregation-induced luminescence materials (supramolecules based on metal rings) and halogen fluorescein (Zou et al., 2016; Xin et al., 2019; Wang et al., 2021; Niu et al., 2021). The antibacterial models of PTT and PDT show good application prospects in the field of sterilization and phototherapy (Han et al., 2020).
3.1 MoS2-contained photothermal/photodynamic synergistic therapy
Recently, Ge and his colleagues have developed a nanoplatform MoS2-QPEI/Ce6/PNS@ZIF-8 with dual response to pH and near-infrared light (NIR), which enables the synergistic antibacterial effect of PDT and PTT to promote wound healing. Firstly, quaternized polyethylenimine (QPEI) was added to the suspension of molybdenum disulfide (MoS2) nanosphere powder to form QPEI-Modified MoS2 Nanospheres and then mixed with dihydroporphyrin e6 (Ce6) and Panax notoginseng saponins (PNS), followed by the addition of zinc nitrate hexahydrate and 2-methylimidazole solution to form nano-platform MoS2-QPEI/Ce6/PNS@ZIF-8. The nanoplatform shows good dispersion and uniform nanometer size (120–150 nm) and has high photothermal conversion efficiency and good photodynamic effect. In the low acid microenvironment of the biofilm, the acid-sensitive zeolite imidazolium frame-8 (ZIF-8) decomposes and releases photosensitizer Ce6, MoS2; QPEI, and PNS. Photosensitizer MoS2 is used in photothermal therapy. The long positively charged carbon chain in the released QPEI structure adsorbs on the cell membrane surface through ion interaction and then destroys the cell membrane structure to release oxygen, alleviate the hypoxia state of the biofilm, and enhance Ce6-mediated PDT (produce1O2). PNS contains a variety of active ingredients to achieve antibacterial, hemostasis, and wound healing (Figure 5). In vitro antibacterial and live/death experiments showed that MQC@ZIF-8 achieved superior antibacterial activity through combined therapy (Jin et al., 2022).
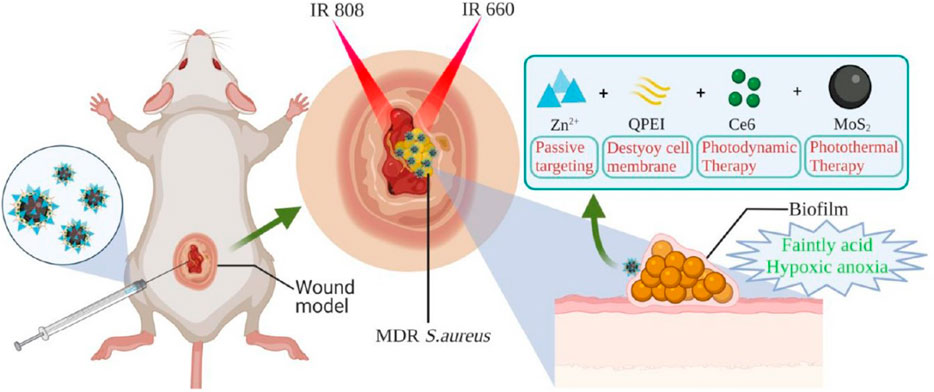
FIGURE 5. Schematic diagram of wound infection treatment using a combination of PTT/PDT/antibacterial active ingredients. Reproduced with permission from Ref (Jin et al., 2022).
A photothermally activated multifunctional nano-antibacterial platform was constructed by introducing indocyanine green (ICG) photosensitizer and silver nanoparticles (AgNPs) into the surface of molybdenum disulfide (MoS2) nanosheets. Photon hyperthermia produced by MoS2 nanoparticles can not only kill bacteria directly but also accelerate the release of ICG and silver ions, which are commonly used chemical antimicrobial agents. The released ICG can be converted into singlet oxygen with the help of photocatalytic oxygen of 808 nm, thus realizing photodynamic sterilization. And loaded ICG and AgNPs can in turn increase calories, which is a mutually reinforcing effect that can produce a synergistic therapeutic effect (Figure 6). The anti-infection experiment in vivo strongly proved that MoS2/ICG/Ag has significant anti-biofilm properties and low biological toxicity (Li et al., 2022).
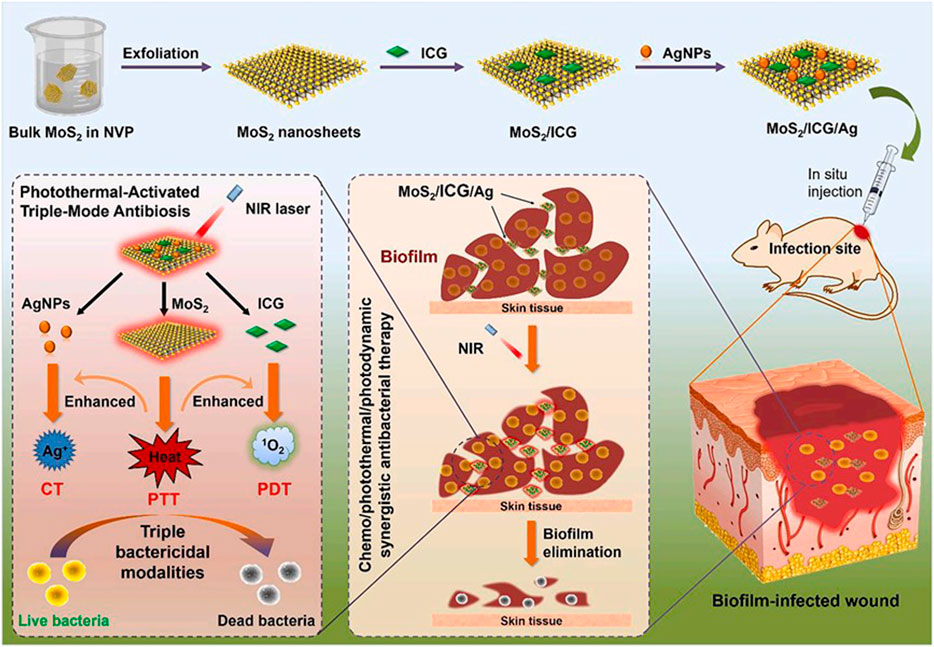
FIGURE 6. Schematic illustration for the preparation of multifunctional MoS2/ICG/Ag nanocomposites for the photothermally activated triple-mode synergistic antibacterial therapy. Reproduced with permission from Ref (Li et al., 2022).
Different Fe3O4@MoS2@sodium dodecyl sulfate nanocomposites were prepared on Fe3O4@MoS2 by ultrasound-assisted sodium dodecyl sulfate coating. The synergistic effect of Fe3O4@MoS2@sodium dodecyl sulfate and near infrared radiation eliminated almost all the biofilms of MRSA, thus improving the germicidal ability of Fe3O4@MoS2sodium dodecyl sulfate under near infrared radiation (Wang et al., 2022).
3.2 Cu-contained photothermal/photodynamic synergistic therapy
Copper ion not only has the ability to destroy bacterial membranes, but also has been proven to promote skin regeneration as a trace element.
A near-infrared (NIR)-activated chemical/photodynamic/photothermal composite therapeutic agent is loaded with fluorescein isothiocyanate (FITC) on mesoporous silica nanoparticles (MSN), super Small copper sulfide nanoparticles (Cu2−xSNPs) and polylysine (ε-Polylysine, PLL) were prepared. The biodegradable PLL can not only enhance the adhesion to the bacterial surface and increase the effect of phototherapy but also destroy the cells through electrostatic interaction. NIR-activated Cu2-xSNPs are used as popular PTT and PDT reagents due to their excellent photostability, negligible cytotoxicity, and biodegradability (Dai et al., 2021).
A hollow Cu2-XS nano-homojunction (nano-HJ) platform was developed by to effectively eradicate bacteria and tumors under tissue permeable near infrared (NIR) light. Copper ions released from Cu2-XSnano-HJs, Cu2-XS nano-HJS have the ability to destroy bacterial membranes, thus achieving the enhanced antibacterial effect in coordination with phototherapy. The nanocomposite material can not only detect bacteria and biofilm rapidly through fluorescence imaging but also ablate bacteria and biofilm through chemical/photothermal/photodynamic combined effects under near-infrared light irradiation (Gao et al., 2021).
Chu and his colleagues successfully prepared a novel quaternary ammonium salted copper-RCDS by coupling the quaternary ammonium compound CAB-35 with copper RCDS through a simple preparation route. The quaternary ammonium group and long hydrocarbon chain in CAB-35 can destroy the cell membrane and enhance the sensitivity of bacterial cells to high temperatures and ROS. Under the irradiation of 808 nm laser, the synergistic antibacterial effect of PPT, PDT, and quaternary ammonium salt can be realized (Figure 7). The nanomaterials triggered by a single near infrared laser have PTT/PDT synergistic antibacterial properties, which can overcome the complexity of multiple light sources (Chu et al., 2021).
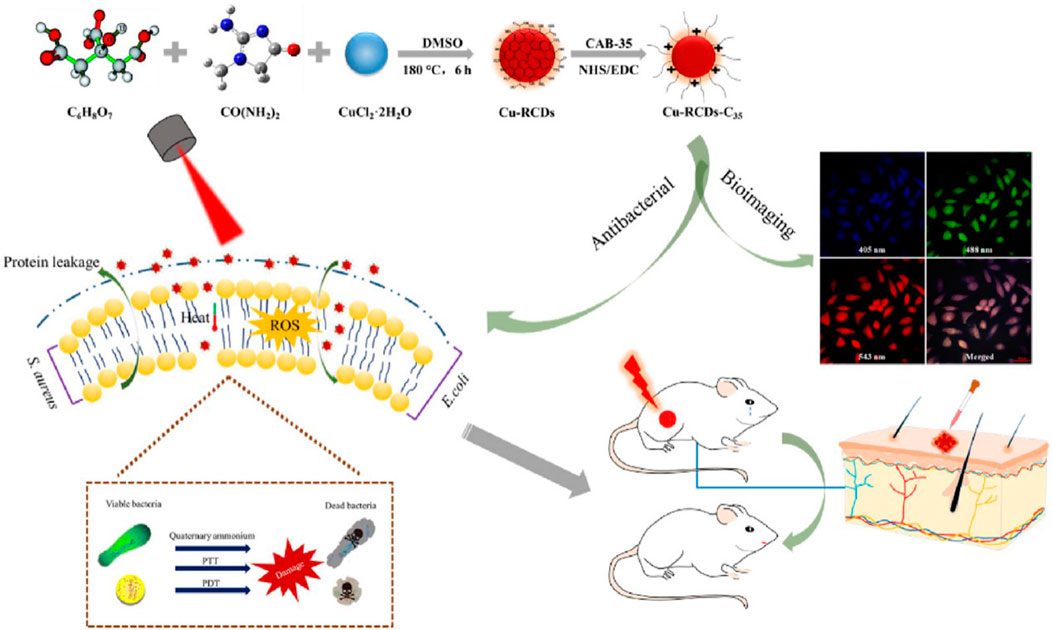
FIGURE 7. Schematic illustration of the preparation of Cu-RCDs-C35 and related biological applications. Reproduced with permission from Ref (Chu et al., 2021).
3.3 ICG-contained photothermal/photodynamic synergistic therapy
The development of a mesoporous carbon nano-platform (CIL@ICG/PFH@O2) modified with cationic cations is reported. Cationic liquids attract anion ICG to provide a near-infrared triggered O2 diffusion enhanced PTT/PDT synergistic antibacterial therapy (Figure 8). Under the irradiation of single wavelength (808Nm) near infrared laser, carbon nanoparticles have a wide wavelength absorption range and high photothermal conversion efficiency, and their local temperature increases rapidly, which may promote the gasification of PFH and significantly accelerate the release of O2 from CIL@ICG/PFH@O2, thus rapidly activating and enhancing the photodynamic effect of CIL@ICG/PFH@O2 (Zhou et al. 2022).
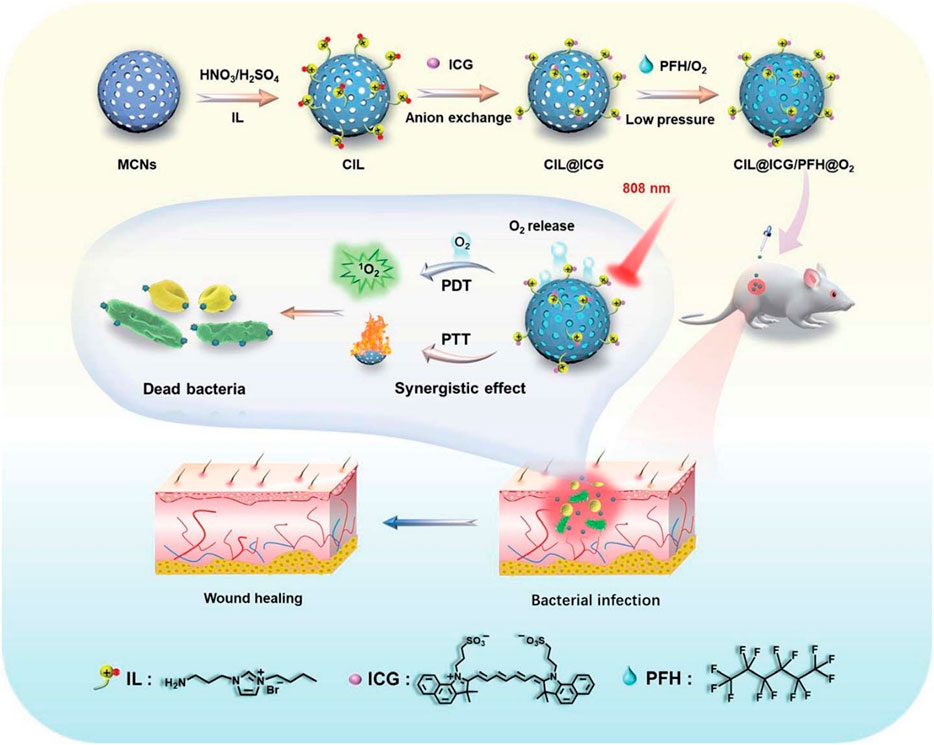
FIGURE 8. Schematic illustration of the synthesis of CIL@ICG/PFH@O2 nanoparticles and their corresponding synergistic antibacterial mech-anism under single wavelength (808 nm) NIR irradiation. CIL@ICG/PFH@O2 showed bactericidal activities against drug-resistant bacteria both in vitro and in vivo Reproduced with permission from Ref (Zhou et al., 2022).
3.4 Other photothermal/photodynamic synergistic therapy
Black phosphorus (BP) is found to have good photoluminescence properties, near-infrared photothermal absorption properties, which is the same as graphene (Li et al., 2014; Ling et al., 2015; Wu et al., 2018c; Zhao et al., 2018). Using this property, Liu and colleagues studied BP and AuNP nanocomposites (BPs@AuNPs). BPS has the ability to produce a large amount of 1O2 under the stimulation of 650 nm laser, which enables BPS@AuNPs to PTT/PDT under a single light source and has a synergistic therapeutic effect on bacteria. The nanocomposites have high antibacterial activity against S. aureus and E. coli in vitro and inhibit the growth of bacteria in the wound model of S. aureus infection in vivo (Liu et al., 2021).
Obeng et al. synthesized ZnO@Ag nanocomposites with good biocompatibility by doping ZnONPs with silver nanoparticles (AgNPs). Zinc oxide @ 8% Ag + PDT + PTT has a significantly destructive effect on biofilm. It has high antibacterial, antimicrobial membrane, and wound healing effects, and can be combined with PTT or PDT alone (Obeng et al., 2022).
4 Fiber membrane for antibacterial therapy
An intelligent fiber membrane with multi-synergistic therapy has been developed to deal with drug-resistant bacterial infections. They first encapsulated curcumin and ICG in the large cavity of ZIF-8/PLA by chemical and electrostatic interaction and then coated the composite Cur-ICG@ZIF-8/PLA/PCM (CIZPP) with non-covalent interaction of phase change material (PCM). PCM has the mechanism of near-infrared induced phase transition and the dissociation of ZIF-8 in the acidic microenvironment of bacterial infection, which realizes the double stimulus response (NIR and pH) release of curcumin in CIZPP. In addition, due to the limiting effect, the photothermal stability and singlet oxygen (1O2) production of CIZPP were higher than those of ICG adsorbed directly on polylactic acid/PCM scaffolds. Indocyanine green (ICG) is an attractive PTT therapeutic agent to build a multi-functional treatment platform. The interaction between ICG and moderate ZIF-8/ICG strengthens the formation of ROS and promotes PDT (Figure 9). As an antibiotic-free antibacterial component, curcumin enhances chemotherapy. Through multi-synergistic anti-infective therapy, it can stimulate collagen deposition, promote the formation of the dermis and skin accessories, and effectively improve the healing rate of infected wounds (Zhang et al., 2022).
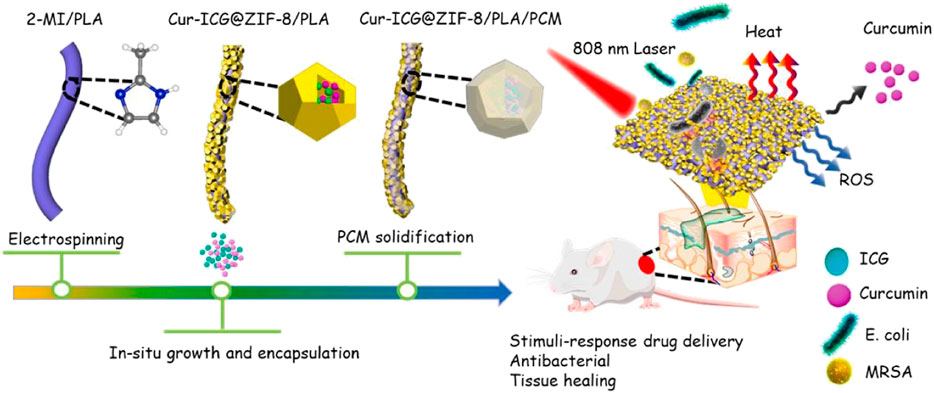
FIGURE 9. Schematic diagram of synthetic procedure and application of CIZPP. Reproduced with permission from Ref (Zhang et al., 2022).
Zang et al. prepared titanium carbide (MXene)/imidazole framework-8 zeolite (ZIF-8)/PLA composite membranes by in situ growth of ZIF-8 molecular sieves on MXene and electrospinning. After MZ-8/PLA was compounded into electrospun scaffolds, it exhibited strong PTT and PDT properties under 808 nm laser irradiation. MZ-8 can promote the generation of ROS, and its photothermal conversion efficiency is 80.5%. In vitro experiments confirmed that the synthesized MZ-8 could generate hyperthermia and ROS based on the PTT-PDT effect, realizing the synergistic antibacterial effect of PDT and PTT (Zhang et al., 2021).
5 Nanosheets for antibacterial therapy
A new phototherapy nanoscale (MHCNSs) with the functions of hyaluronidase (HAase) response fluorescence imaging (FLI) and PTT/PDT was prepared. The Ce6 released by MHCNSs can produce ROS for antibacterial PDT, MoS2NSs and contribute to the bactericidal effect of MHCNSs, which makes MHCNSs a dual-mode (PTT/PDT) antibacterial nanoscale. The results of in vitro and in vivo experiments showed that MHCNSs had good biocompatibility. The study of antibacterial activity further showed that MHCNSS (40 μg mL−1) had an obvious killing effect on methicillin-resistant S. aureus in infected wounds of mice compared with other groups, and the reduction rate was 99.9% (Yuwen et al., 2021).
Recently, a new type of indocyanine green (ICG) functionalized hexagonal Mn3O4 nanoparticles (Mn3O4HNSs@ICG) has been designed to cooperate in the fight against bacterial infection. ICG as a photosensitizer, manganese oxide can convert light energy into high heat, and the released Mn3+ and Mn2+ ions participate in Mn3O4HNSs, which is conducive to the electron transfer in the Fenton-like reaction, thus promoting the production of ROS for treatment. Secondly, the flake structure with a rough surface and rich defects makes Mn3O4HNSs@ICG easy to adhere to the surface of bacteria, thus destroying its membrane system. Carry on the synergistic action of many ways to achieve good antibacterial. In vitro and in vivo toxicity evaluation showed that the material had good biological safety and was expected to be used in clinical anti-infective therapy (Zhang et al., 2022).
6 Other nanomaterials for antibacterial therapy
With the development of nanotechnology, more and more nanomaterials have been reported. There are other nanomaterials that can realize synergistic antibacterial effects of PDT and PTT have been developed.
Inspired by the morphology and infection mode of the COVID-19 coronavirus, Ni and his colleagues designed porous graphite nitride carbon (g-C3N4) with “artificial virus” embedded cobalt nanoparticles by self-assembling transpeptide transactivators with three layers of shell. Firstly, three layers of porous graphitized carbonitride (TCNCo) loaded with cobalt nanoparticles were prepared by the template method. The cobalt (Co) nanoparticles have an additional magnetic targeting function, which can enhance the ability of photothermal conversion. Then three-shell porous graphite carbon nanoparticles (TCNCo) coated with transduction peptide (TAT)were prepared by electrostatic self-assembly. TAT has a good ability to penetrate bacterial cell membranes because of its rich positively charged amino acids and stable secondary structure (Gao et al., 2019; Mookherjee et al., 2020). By imitating the coronal morphology and infection mode of COVID-19 cells, TCNCoT showed a tentacle-like structure on its surface, overcome the bottleneck of the bacterial membrane, successfully penetrated the bacterial cell membrane, and then released TCNCo with photothermal and photodynamic effects into the bacteria. In vitro experiments showed that the germicidal efficiency of the nanoparticles in 20min was as high as 99.99%, which was 18.6 times that of g-C3N4, and the germicidal efficacy remained 99.99% after 3 rounds of repeated use (Ni et al., 2022).
Self-assembled aggregation-induced emission (AIE) nanospheres (AIE-PEG1000 NPs) with near-infrared II (NIR-II) fluorescence emission, photothermal and photodynamic properties were prepared using multi-functional AIE luminescence (AIE-4COOH). AIE-PEG1000 nanoparticles were encapsulated in lipid nanoparticles with teicoplanin (Tei) and ammonium bicarbonate (AB) to form laser-activated nanoparticles (AIE-Tei@AB Nvs). (AIE) photothermal agent or AIE photosensitizer is one of the multifunctional materials with fluorescence imaging properties and PTT or PDT functions, so it can diagnose and treat diseases at the same time (Li et al., 2020; Zhang et al., 2021; Ni et al., 2021; Yan et al., 2021). Under the irradiation of 660 nm laser, AIE-Tei@AB NVS successfully realized the near infrared-II fluorescence and infrared (IR) thermal imaging of focus by loading the photoluminescence and photothermal properties of AIE-PEG1000 nanoparticles. At the same time, during the photothermal process, the loaded AB is thermally decomposed to produce a large number of CO2/NH3 bubbles (efficient ultrasound contrast agent), thus achieving high-performance ultrasound imaging of the infected focus The efficient photothermal and photodynamic characteristics of AIE-Tei@AB Nvs, combined with the decomposition and rapid release of NV during bubble formation. Synergistic treatment of bacterial infections through a variety of treatments (Li et al., 2023).
Using water as solvent and molybdenum trichloride (MoCl3) as the precursor, MoO3−xNDS nanozyme was prepared by a one-pot hydrothermal method. Based on the combination of photodynamic, photothermal, and peroxidase-like enzyme activities modulated by a single near-infrared irradiation (808 nm), MoO3−xNDS alone has a triple therapeutic synergistic efficiency. motivated by. Both photodynamic and nanozyme activities lead to the production of reactive oxygen species (ROS). The photothermal effect can adjust the MoO3−xNDS to their optimal enzymatic temperature (50 C), which can generate sufficient ROS even at a low concentration of H2O2 (100 µm). In vitro and in vivo experiments demonstrate the excellent antibacterial efficiency of MoO3-xNDs against drug-resistant extended-spectrum β-lactamases producing E. coli and methicillin-resistant S. aureus (MRSA) (Zhang et al., 2021).
6 Conclusions and prospects
This paper reviews the application of anti-infective materials containing PDT and PTT synergistic therapy in recent years. We discuss how various components in different materials play their roles in synergy. At the same time, we provide some latest examples that can overcome the shortcomings of a single treatment model by combining multiple approaches such as PDT and PTT, and achieve complementary multiple therapeutic effects. However, due to some challenges, the clinical application of these systems is still difficult to use. First of all, in order to improve the therapeutic effect and reduce the side effects, the combination of PTT and PDT has attracted much attention because of its low systemic toxicity, non-invasive, and excellent therapeutic effect. However, most PTT/PDT collaborative strategies are based on multi-component therapeutic agents prepared by complex processes and require different light sources to stimulate PTT and PDT. Therefore, it is very necessary to develop effective single-component drugs for PTT/PDT synergistic therapy. At the same time, simplification should be borne in mind when designing new synergistic therapeutic materials so that they can be used clinically by simplifying, expanding scale, and reducing costs. Secondly, the molecular mechanism of bacterial infection and the mechanism of drug resistance are not very clear. We need to evaluate the different characteristics of different bacteria in order to achieve accurate and efficient treatment. Then, the drugs that play the synergistic effect of PDT and PTT need to be released in an orderly manner and will not have harmful effects on normal cells, which requires an in-depth exploration of the synergistic therapy involved, which can make use of the differences between infected bacteria and normal cells for specific targeting. With the development of chemistry, material technology, and nanomedicine, the emergence of new design concepts and new treatments, as well as an in-depth understanding of the molecular and cellular mechanisms of bacterial infection, we believe that a new synergistic system that can meet the above challenges will be developed to further improve antimicrobial activity and reduce side effects and promote its clinical transformation in infection treatment.
Author contributions
ZY: Formal analysis, Resources, Writing—Original Draft, Visualization, Writing—Review and Editing. DW: Formal analysis, Resources, Writing—Original Draft. YG: Conceptualization, Review and Editing, Supervision, Funding acquisition, Writing—Review and Editing. All authors contributed to the article and approved the submitted version.
Conflict of interest
The authors declare that the research was conducted in the absence of any commercial or financial relationships that could be construed as a potential conflict of interest.
Publisher’s note
All claims expressed in this article are solely those of the authors and do not necessarily represent those of their affiliated organizations, or those of the publisher, the editors and the reviewers. Any product that may be evaluated in this article, or claim that may be made by its manufacturer, is not guaranteed or endorsed by the publisher.
References
Chen, W., Wang, Y., Qin, M., Zhang, X., Zhang, Z., Sun, X., et al. (2018). Bacteria-driven hypoxia targeting for combined biotherapy and photothermal therapy. ACS Nano 12, 5995–6005. doi:10.1021/acsnano.8b02235
Cheng, Z., Li, Y., Zhao, D., Zhao, W., Wu, M., Zhang, W., et al. (2022). Nanocarriers for intracellular co-delivery of proteins and small-molecule drugs for cancer therapy. Biotechnology 10, 994655. doi:10.3389/fbioe.2022.994655
Choi, K., Kim, J., Kim, G. W., and Choi, C. J. C. (2009). Oxidative stress-induced necrotic cell death via mitochondira-dependent burst of reactive oxygen species. Curr. Neurovasc. Res. 6, 213–222. doi:10.2174/156720209789630375
Chu, X., Zhang, P., Wang, Y., Sun, B., Liu, Y., Zhang, Q., et al. (2021). Near-infrared carbon dot-based platform for bioimaging and photothermal/photodynamic/quaternary ammonium triple synergistic sterilization triggered by single NIR light source. Carbon N. Y. 176, 126–138. doi:10.1016/j.carbon.2021.01.119
Crofts, T. S., Gasparrini, A. J., and Dantas, G. J. N. R. M. (2017). Next-generation approaches to understand and combat the antibiotic resistome. Nat. Rev. Microbiol. 15, 422–434. doi:10.1038/nrmicro.2017.28
Dai, X., Ma, J., Chen, N., Cai, Y., He, Y., Li, X., et al. (2021). MSNs-based nanocomposite for biofilm imaging and NIR-activated chem/photothermal/photodynamic combination therapy. ACS Appl. Bio Mat. 4, 2810–2820. doi:10.1021/acsabm.1c00034
Fan, T., Zheng, N. N., Yan, L., He, S., Ai, F., and Hu, J. J. N. A. (2023). Palladium nanoparticles based smart hydrogels for NIR light-triggered photothermal/photodynamic therapy and drug release with wound healing capability. Nanoscale Adv. 5, 45. doi:10.1039/D2NA00925K
Gao, G., Jiang, Y. W., Jia, H. R., and Wu, F. G. J. B. (2019a). Near-infrared light-controllable on-demand antibiotics release using thermo-sensitive hydrogel-based drug reservoir for combating bacterial infection. Biomaterials 188, 83–95. doi:10.1016/j.biomaterials.2018.09.045
Gao, S., Yan, X., Xie, G., Zhu, M., Ju, X., Stang, P. J., et al. (2019b). Membrane intercalation-enhanced photodynamic inactivation of bacteria by a metallacycle and TAT-decorated virus coat protein. Proc. Natl. Acad. Sci. U. S. A. 116, 23437–23443. doi:10.1073/pnas.1911869116
Gao, X., Wei, M., Ma, D., Yang, X., Zhang, Y., Zhou, X., et al. (2021). Engineering of a hollow-structured Cu 2−<sub>X</sub> S nano-homojunction platform for near infrared-triggered infected wound healing and cancer therapy. Adv. Funct. Mat. 31, 2106700. doi:10.1002/adfm.202106700
Gong, P., Wang, F., Guo, F., Liu, J., Wang, B., Ge, X., et al. (2018). Fluorescence turn-off Ag/fluorinated graphene composites with high NIR absorption for effective killing of cancer cells and bacteria. J. Mat. Chem. B 6, 7926–7935. doi:10.1039/c8tb02211a
Gross, T. M., Lahiri, J., Golas, A., Luo, J., Verrier, F., Kurzejewski, J. L., et al. (2019). Copper-containing glass ceramic with high antimicrobial efficacy. Nat. Commun. 10, 1979. doi:10.1038/s41467-019-09946-9
Hahn, Y. B., Ahmad, R., and Tripathy, N. J. C. C. (2012). Chemical and biological sensors based on metal oxide nanostructures. Chem. Commun. 48, 10369–10385. doi:10.1039/c2cc34706g
Han, D., Li, Y., Liu, X., Li, B., Han, Y., Zheng, Y., et al. (2020). Rapid bacteria trapping and killing of metal-organic frameworks strengthened photo-responsive hydrogel for rapid tissue repair of bacterial infected wounds. Chem. Eng. J. 396, 125194. doi:10.1016/j.cej.2020.125194
Huang, W. C., Tsai, P. J., and Chen, Y. C. J. S. (2009). Multifunctional Fe3O4@ Au nanoeggs as photothermal agents for selective killing of nosocomial and antibiotic-resistant bacteria. Small 5, 51–56. doi:10.1002/smll.200801042
Jiang, Q. E. F., Tian, J., Yang, J., Zhang, J., and Cheng, Y. J. (2020). Light-excited antibiotics for potentiating bacterial killing via reactive oxygen species generation. ACS Appl. Mat. Interfaces 12, 16150–16158. doi:10.1021/acsami.0c02647
Jin, W., Song, P., Wu, Y., Tao, Y., Yang, K., Gui, L., et al. (2022). Biofilm microenvironment-mediated MoS2 nanoplatform with its photothermal/photodynamic synergistic antibacterial molecular mechanism and wound healing study. ACS Biomater. Sci. Eng. 8, 4274–4288. doi:10.1021/acsbiomaterials.2c00856
Khurana, B., Gierlich, P., Meindl, A., Gomes-da-Silva, L. C., Senge, M. O. J. P., and Sciences, P. (2019). Hydrogels: Soft matters in photomedicine. Photochem. Photobiol. Sci. 18, 2613–2656. doi:10.1039/c9pp00221a
Koo, H., Allan, R. N., Howlin, R. P., Stoodley, P., and Hall-Stoodley, L. J. N. R. M. (2017). Targeting microbial biofilms: Current and prospective therapeutic strategies. Nat. Rev. Microbiol. 15, 740–755. doi:10.1038/nrmicro.2017.99
Laxminarayan, R., Duse, A., Wattal, C., Zaidi, A. K., Wertheim, H. F., Sumpradit, N., et al. (2013). Antibiotic resistance—The need for global solutions. Lancet Infect. Dis. 13, 1057–1098. doi:10.1016/S1473-3099(13)70318-9
Li, B., Wang, W., Zhao, L., Yan, D., Li, X., Gao, Q., et al. (2023). Multifunctional AIE nanosphere-based “nanobomb” for trimodal imaging-guided photothermal/photodynamic/pharmacological therapy of drug-resistant bacterial infections. ACS Nano 17 (5), 4601–4618. doi:10.1021/acsnano.2c10694
Li, H., Gong, M., Xiao, J., Hai, L., Luo, Y., He, L., et al. (2022a). Photothermally activated multifunctional MoS2 bactericidal nanoplatform for combined chemo/photothermal/photodynamic triple-mode therapy of bacterial and biofilm infections. Chem. Eng. J. 429, 132600. doi:10.1016/j.cej.2021.132600
Li, H., Wen, H., Zhang, Z., Song, N., Kwok, R. T., Lam, J. W., et al. (2020). Reverse thinking of the aggregation-induced emission principle: Amplifying molecular motions to boost photothermal efficiency of nanofibers. Angew. Chem. Int. Ed. 59, 20371–20375. doi:10.1002/anie.202008292
Li, L., Yu, Y., Ye, G. J., Ge, Q., Ou, X., Wu, H., et al. (2014). Black phosphorus field-effect transistors. Nat. Nanotechnol. 9, 372–377. doi:10.1038/nnano.2014.35
Li, M., Liu, X., Tan, L., Cui, Z., Yang, X., Li, Z., et al. (2018). Noninvasive rapid bacteria-killing and acceleration of wound healing through photothermal/photodynamic/copper ion synergistic action of a hybrid hydrogel. Biomater. Sci. 6, 2110–2121. doi:10.1039/c8bm00499d
Li, S., Cui, S., Yin, D., Zhu, Q., Ma, Y., Qian, Z., et al. (2017). Dual antibacterial activities of a chitosan-modified upconversion photodynamic therapy system against drug-resistant bacteria in deep tissue. Nanoscale 9, 3912–3924. doi:10.1039/c6nr07188k
Li, Z., Xu, W., Yang, J., Wang, J., Wang, J., Zhu, G., et al. (2022b). A tumor microenvironments-adapted polypeptide hydrogel/nanogel composite boosts antitumor molecularly targeted inhibition and immunoactivation. Adv. Mat. May 34, e2200449. doi:10.1002/adma.202200449
Liang, H., Shuang, W., Zhang, Y., Chao, S., Han, H., Wang, X., et al. (2018). Graphene-like multilayered CuS nanosheets assembled into flower-like microspheres and their electrocatalytic oxygen evolution properties. ChemElectroChem 5, 494–500. doi:10.1002/celc.201701074
Liang, Y., Wang, M., Zhang, Z., Ren, G., Liu, Y., Wu, S., et al. (2019a). Facile synthesis of ZnO QDs@ GO-CS hydrogel for synergetic antibacterial applications and enhanced wound healing. Chem. Eng. J. 378, 122043. doi:10.1016/j.cej.2019.122043
Liang, Y., Zhao, X., Hu, T., Chen, B., Yin, Z., Ma, P. X., et al. (2019b). Adhesive hemostatic conducting injectable composite hydrogels with sustained drug release and photothermal antibacterial activity to promote full-thickness skin regeneration during wound healing. Small 15, 1900046. doi:10.1002/smll.201900046
Ling, X., Wang, H., Huang, S., Xia, F., and Dresselhaus, M. S. (2015). The renaissance of black phosphorus. renaissance black phosphorus 112, 4523–4530. doi:10.1073/pnas.1416581112
Liu, B., Li, C., Yang, P., Hou, Z., and Lin, J. J. A. (2017). 808-nm-Light-excited lanthanide-doped nanoparticles: Rational design, luminescence control and theranostic applications. Adv. Mat. 29, 1605434. doi:10.1002/adma.201605434
Liu, B., Su, Y., Wu, S., and Shen, J. J. N. J. (2021). Two dimensional BP@ AuNP nanocomposites for photothermal/photodynamic therapy mediated wound disinfection and infected wound healing under a single light source. New J. Chem. 45, 18124–18130. doi:10.1039/d1nj03137f
Liu, S., Pan, J., Liu, J., Ma, Y., Qiu, F., Mei, L., et al. (2018). Dynamically PEGylated and borate-coordination-polymer-coated polydopamine nanoparticles for synergetic tumor-targeted, chemo-photothermal combination therapy. Small 14, 1703968. doi:10.1002/smll.201703968
Liu, Y., Guo, Z., Li, F., Xiao, Y., Zhang, Y., Bu, T., et al. (2019a). Multifunctional magnetic copper ferrite nanoparticles as fenton-like reaction and near-infrared photothermal agents for synergetic antibacterial therapy. ACS Appl. Mat. Interfaces 11, 31649–31660. doi:10.1021/acsami.9b10096
Liu, Z., Wang, F., Ren, J., and Qu, X. J. B. (2019b). A series of MOF/Ce-based nanozymes with dual enzyme-like activity disrupting biofilms and hindering recolonization of bacteria. Biomaterials 208, 21–31. doi:10.1016/j.biomaterials.2019.04.007
Lv, X., Zhang, J., Yang, D., Shao, J., Wang, W., Zhang, Q., et al. (2020). Recent advances in pH-responsive nanomaterials for anti-infective therapy. J. Mat. Chem. B 8, 10700–10711. doi:10.1039/d0tb02177f
Ma, M., Gao, N., Li, X., Liu, Z., Pi, Z., Du, X., et al. (2020). A biocompatible second near-infrared nanozyme for spatiotemporal and non-invasive attenuation of amyloid deposition through scalp and skull. ACS Nano 14, 9894–9903. doi:10.1021/acsnano.0c02733
Ma, Y., Jiang, K., Chen, H., Shi, Q., Liu, H., Zhong, X., et al. (2022). Liquid exfoliation of V8C7 nanodots as peroxidase-like nanozymes for photothermal-catalytic synergistic antibacterial treatment. Acta Biomater. 149, 359–372. doi:10.1016/j.actbio.2022.06.031
Mak, K. F., Lee, C., Hone, J., Shan, J., and Heinz, T. F. J. P. (2010). Atomically thin MoS 2: A new direct-gap semiconductor. Phys. Rev. Lett. 105, 136805. doi:10.1103/PhysRevLett.105.136805
Maleki, A., He, J., Bochani, S., Nosrati, V., Shahbazi, M. A., and Guo, B. J. A. (2021). Multifunctional photoactive hydrogels for wound healing acceleration. ACS Nano 15, 18895–18930. doi:10.1021/acsnano.1c08334
Mao, C., Xiang, Y., Liu, X., Cui, Z., Yang, X., Li, Z., et al. (2018). Repeatable photodynamic therapy with triggered signaling pathways of fibroblast cell proliferation and differentiation to promote bacteria-accompanied wound healing. ACS Nano 12, 1747–1759. doi:10.1021/acsnano.7b08500
Mao, C., Xiang, Y., Liu, X., Cui, Z., Yang, X., Yeung, K. W. K., et al. (2017). Photo-Inspired antibacterial activity and wound healing acceleration by hydrogel embedded with Ag/Ag@AgCl/ZnO nanostructures. Ag@ AgCl/ZnO nanostructures 11, 9010–9021. doi:10.1021/acsnano.7b03513
Mookherjee, N., Anderson, M. A., Haagsman, H. P., and Davidson, D. J. J. (2020). Antimicrobial host defence peptides: Functions and clinical potential. Nat. Rev. Drug Discov. 19, 311–332. doi:10.1038/s41573-019-0058-8
Murugesan, S., and Scheibel, T. J. A. F. M. (2020). Copolymer/clay nanocomposites for biomedical applications. Adv. Funct. Mater. 30, 1908101. doi:10.1002/adfm.201908101
Nam, J., Son, S., Ochyl, L. J., Kuai, R., Schwendeman, A., and Moon, J. J. J. N. (2018). Chemo-photothermal therapy combination elicits anti-tumor immunity against advanced metastatic cancer. Nat. Commun. 9, 1074. doi:10.1038/s41467-018-03473-9
Ni, J., Wang, Y., Zhang, H., Sun, J. Z., and Tang, B. Z. J. M. (2021). Aggregation-induced generation of reactive oxygen species: Mechanism and photosensitizer construction. Molecules 26, 268. doi:10.3390/molecules26020268
Ni, Y., Wang, J., Wang, M., Liu, L., Nie, H., Wang, Q., et al. (2022). COVID-19-inspired “artificial virus” to combat drug-resistant bacteria by membrane-intercalation-photothermal-photodynamic multistage effects. Chem. Eng. J. 446, 137322. doi:10.1016/j.cej.2022.137322
Niu, J., Wang, L., Cui, T., Wang, Z., Zhao, C., Ren, J., et al. (2021). Antibody mimics as bio-orthogonal catalysts for highly selective bacterial recognition and antimicrobial therapy. ACS Nano 15, 15841–15849. doi:10.1021/acsnano.1c03387
Obeng, E., Feng, J., Wang, D., Zheng, D., Xiang, B., and Shen, J. J. F. (2022). Multifunctional phototheranostic agent ZnO@ Ag for anti-infection through photothermal/photodynamic therapy Front. Chem. 10, 1054739. doi:10.3389/fchem.2022.1054739
Qing, G., Zhao, X., Gong, N., Chen, J., Li, X., Gan, Y., et al. (2019). Thermo-responsive triple-function nanotransporter for efficient chemo-photothermal therapy of multidrug-resistant bacterial infection. Nat. Commun. 10, 4336. doi:10.1038/s41467-019-12313-3
Richter, K., and Kietzmann, T. J. C. (2016). Reactive oxygen species and fibrosis: Further evidence of a significant liaison. Cell. Tissue Res. 365, 591–605. doi:10.1007/s00441-016-2445-3
Rizwan, S., ReddySekhar, P., and MalikAsrar, B. J. A. (2014). Reactive oxygen species in inflammation and tissue injury. Antioxid. Redox Signal 20, 1126–1167. doi:10.1089/ars.2012.5149
Sang, Y., Cao, F., Li, W., Zhang, L., You, Y., Deng, Q., et al. (2020). Bioinspired construction of a nanozyme-based H2O2 homeostasis disruptor for intensive chemodynamic therapy. J. Am. Chem. Soc. Mar. 18 (142), 5177–5183. doi:10.1021/jacs.9b12873
Scheres, J., and Kuszewski, K. J. (2019). The Ten Threats to Global Health in 2018 and 2019. A welcome and informative communication of WHO to everybody. Public Health Management/Zdrowie Publiczne i Zarzadzanie 17 (1).
Sehmi, S. K., Noimark, S., Bear, J. C., Peveler, W. J., Bovis, M., Allan, E., et al. (2015). Lethal photosensitisation of Staphylococcus aureus and Escherichia coli using crystal violet and zinc oxide-encapsulated polyurethane. J. Mat. Chem. B 3, 6490–6500. doi:10.1039/c5tb00971e
Shan, J., Li, X., Yang, K., Xiu, W., Wen, Q., Zhang, Y., et al. (2019). Efficient bacteria killing by Cu2WS4 nanocrystals with enzyme-like properties and bacteria-binding ability. ACS Nano 13, 13797–13808. doi:10.1021/acsnano.9b03868
Shang, Z., Chan, S. Y., Song, Q., Li, P., and Huang, W. J. R. (2020). The strategies of pathogen-oriented therapy on circumventing antimicrobial resistance. Res. (Wash D C) 2020, 2016201. doi:10.34133/2020/2016201
Shi, S., Wu, S., Shen, Y., Zhang, S., Xiao, Y., He, X., et al. (2018). Iron oxide nanozyme suppresses intracellular Salmonella Enteritidis growth and alleviates infection in vivo. Theranostics 8, 6149–6162. doi:10.7150/thno.29303
Sun, J., Song, L., Fan, Y., Tian, L., Luan, S., Niu, S., et al. (2019). Synergistic photodynamic and photothermal antibacterial nanocomposite membrane triggered by single NIR light source. ACS Appl. Mat. Interfaces 11, 26581–26589. doi:10.1021/acsami.9b07037
Surendra, T., Roopan, S. M., Al-Dhabi, N. A., Arasu, M. V., Sarkar, G., and Suthindhiran, K. J. (2016). Vegetable peel waste for the production of ZnO nanoparticles and its toxicological efficiency, antifungal, hemolytic, and antibacterial activities. Nanoscale Res. Lett. 11, 546–610. doi:10.1186/s11671-016-1750-9
Tan, L., Li, J., Liu, X., Cui, Z., Yang, X., Yeung, K. W. K., et al. (2018). In situ disinfection through photoinspired radical oxygen species storage and thermal-triggered release from black phosphorous with strengthened chemical stability. Small 14, 1703197. doi:10.1002/smll.201703197
Tao, B., Lin, C., Qin, X., Yu, Y., Guo, A., Li, K., et al. (2022). Fabrication of gelatin-based and Zn2+-incorporated composite hydrogel for accelerated infected wound healing. Mat. Today Bio 13, 100216. doi:10.1016/j.mtbio.2022.100216
Tao, B., Yi, W., Qin, X., Wu, J., Li, K., Guo, A., et al. (2023). Improvement of antibacterial, anti-inflammatory, and osteogenic properties of OGP loaded Co-MOF coating on titanium implants for advanced osseointegration. J. Mat. Sci. Technol. 146, 131–144. doi:10.1016/j.jmst.2022.11.013
Wang, H., Gong, S., Li, X., Chong, Y., Ge, Q., Wang, J., et al. (2022). SDS coated Fe3O4@ MoS2 with NIR-enhanced photothermal-photodynamic therapy and antibiotic resistance gene dissemination inhibition functions. Colloids Surfaces B Biointerfaces 214, 112457. doi:10.1016/j.colsurfb.2022.112457
Wang, H., Song, Z., Li, S., Wu, Y., and Han, H. J. A. (2019a). One stone with two birds: Functional gold nanostar for targeted combination therapy of drug-resistant Staphylococcus aureus infection. ACS Appl. Mat. Interfaces 11, 32659–32669. doi:10.1021/acsami.9b09824
Wang, S., Riedinger, A., Li, H., Fu, C., Liu, H., Li, L., et al. (2015). Plasmonic copper sulfide nanocrystals exhibiting near-infrared photothermal and photodynamic therapeutic effects. ACS Nano 9, 1788–1800. doi:10.1021/nn506687t
Wang, W., Cheng, X., Liao, J., Lin, Z., Chen, L., Liu, D., et al. (2019b). Synergistic photothermal and photodynamic therapy for effective implant-related bacterial infection elimination and biofilm disruption using Cu9S8 nanoparticles. ACS Biomater. Sci. Eng. 5, 6243–6253. doi:10.1021/acsbiomaterials.9b01280
Wang, W., Li, B., Yang, H., Lin, Z., Chen, L., Li, Z., et al. (2020a). Efficient elimination of multidrug-resistant bacteria using copper sulfide nanozymes anchored to graphene oxide nanosheets. Nano Res. 13, 2156–2164. doi:10.1007/s12274-020-2824-7
Wang, X., Shi, Q., Zha, Z., Zhu, D., Zheng, L., Shi, L., et al. (2021a). Copper single-atom catalysts with photothermal performance and enhanced nanozyme activity for bacteria-infected wound therapy. Bioact. Mat. 6, 4389–4401. doi:10.1016/j.bioactmat.2021.04.024
Wang, Y., Chen, C., Zhang, D., and Wang, J. J. A. C. B. E. (2020b). Bifunctionalized novel Co-V MMO nanowires: Intrinsic oxidase and peroxidase like catalytic activities for antibacterial application. Appl. Catal. B Environ. 261, 118256. doi:10.1016/j.apcatb.2019.118256
Wang, Y., Yang, Y., Shi, Y., Song, H., and Yu, C. J. A. M. (2020c). Antibiotic-free antibacterial strategies enabled by nanomaterials: Progress and perspectives. Adv. Mater. 32, 1904106. doi:10.1002/adma.201904106
Wang, Z., Niu, J., Zhao, C., Wang, X., Ren, J., and Qu, X. J. A. C. (2021b). A bimetallic metal–organic framework encapsulated with DNAzyme for intracellular drug synthesis and self-sufficient gene therapy. Angew. Chem. Int. Ed. 133, 12431–12437. doi:10.1002/anie.202016442
Wu, J., Huang, S., Jin, Z., Chen, J., Hu, L., Long, Y., et al. (2018a). Black phosphorus: An efficient co-catalyst for charge separation and enhanced photocatalytic hydrogen evolution. J. Mat. Sci. 53, 16557–16566. doi:10.1007/s10853-018-2830-2
Wu, J., Shen, P., Qin, X., Yang, Y., Lin, C., Li, X., et al. (2023). Self-supply of H2O2 and O2 by a composite nanogenerator for chemodynamic therapy/hypoxia improvement and rapid therapy of biofilm-infected wounds. Chem. Eng. J. 459, 41507. doi:10.1016/j.cej.2023.141507
Wu, K., Zhu, D., Dai, X., Wang, W., Zhong, X., Fang, Z., et al. (2022). Bimetallic oxide Cu1 5Mn1 5O4 cage-like frame nanospheres with triple enzyme-like activities for bacterial-infected wound therapy. Nano Today 43, 101380. doi:10.1016/j.nantod.2022.101380
Wu, Q., Niu, M., Chen, X., Tan, L., Fu, C., Ren, X., et al. (2018b). Biocompatible and biodegradable zeolitic imidazolate framework/polydopamine nanocarriers for dual stimulus triggered tumor thermo-chemotherapy. Biomaterials 162, 132–143. doi:10.1016/j.biomaterials.2018.02.022
Wu, Q., Wei, G., Xu, Z., Han, J., Xi, J., Fan, L., et al. (2018c). Mechanistic insight into the light-irradiated carbon capsules as an antibacterial agent. ACS Appl. Mat. Interfaces 10, 25026–25036. doi:10.1021/acsami.8b04932
Wu, S., Li, A., Zhao, X., Zhang, C., Yu, B., Zhao, N., et al. (2019). Silica-coated gold–silver nanocages as photothermal antibacterial agents for combined anti-infective therapy. ACS Appl. Mat. Interfaces 11, 17177–17183. doi:10.1021/acsami.9b01149
Xi, J., Wei, G., An, L., Xu, Z., Xu, Z., Fan, L., et al. (2019). Copper/carbon hybrid nanozyme: Tuning catalytic activity by the copper state for antibacterial therapy. Nano Lett. 19, 7645–7654. doi:10.1021/acs.nanolett.9b02242
Xia, L. Y., Zhang, X., Cao, M., Chen, Z., and Wu, F. G. J. B. (2017). Enhanced fluorescence emission and singlet oxygen generation of photosensitizers embedded in injectable hydrogels for imaging-guided photodynamic cancer therapy. Biomacromolecules 18, 3073–3081. doi:10.1021/acs.biomac.7b00725
Xiang, Y., Mao, C., Liu, X., Cui, Z., Jing, D., Yang, X., et al. (2019). Rapid and superior bacteria killing of carbon quantum dots/ZnO decorated injectable folic acid-conjugated PDA hydrogel through dual-light triggered ROS and membrane permeability. Small 15, 1900322. doi:10.1002/smll.201900322
Xiang, Y., Zhou, Q., Li, Z., Cui, Z., Liu, X., Liang, Y., et al. (2020). A Z-scheme heterojunction of ZnO/CDots/C3N4 for strengthened photoresponsive bacteria-killing and acceleration of wound healing. J. Mat. Sci. Technol. 57, 1–11. doi:10.1016/j.jmst.2020.05.016
Xie, Y., Gan, C., Li, Z., Liu, W., Yang, D., and Qiu, X. J. A. B. S. (2022). Fabrication of a lignin-copper sulfide-incorporated PVA hydrogel with near-infrared-activated photothermal/photodynamic/peroxidase-like performance for combating bacteria and biofilms, ACS Biomater. Sci. Eng. 8, 560–569. doi:10.1021/acsbiomaterials.1c01406
Xie, Y., Qu, X., Li, J., Li, D., Wei, W., Hui, D., et al. (2020). Ultrafast physical bacterial inactivation and photocatalytic self-cleaning of ZnO nanoarrays for rapid and sustainable bactericidal applications. Sci. Total Environ. 738, 139714. doi:10.1016/j.scitotenv.2020.139714
Xin, Q., Shah, H., Nawaz, A., Xie, W., Akram, M. Z., Batool, A., et al. (2019). Antibacterial carbon-based nanomaterials. Antibact. carbon-based Nanomater. 31, 1804838. doi:10.1002/adma.201804838
Xiong, Y., Luo, B., Chen, G., Cai, J., Jiang, Q., Gu, B., et al. (2019). CuS@ Corn stalk/chitin composite hydrogel for photodegradation and antibacterial. Polym. (Basel). 11, 1393. doi:10.3390/polym11091393
Yan, D., Xie, W., Zhang, J., Wang, L., Wang, D., and Tang, B. Z. J. A. C. (2021). Donor/π-Bridge manipulation for constructing a stable NIR-II aggregation-induced emission luminogen with balanced phototheranostic performance. Angew. Chem. Int. Ed. 133, 26769–26776. doi:10.1002/anie.202111767
Yang, D., Lv, X., Xue, L., Yang, N., Hu, Y., Weng, L., et al. (2019). A lipase-responsive antifungal nanoplatform for synergistic photodynamic/photothermal/pharmaco-therapy of azole-resistant Candida albicans infections. Chem. Commun. 55, 15145–15148. doi:10.1039/c9cc08463k
Yuan, Z., Lin, C., He, Y., Tao, B., Chen, M., Zhang, J., et al. (2020). Near-infrared light-triggered nitric-oxide-enhanced photodynamic therapy and low-temperature photothermal therapy for biofilm elimination. ACS Nano 14, 3546–3562. doi:10.1021/acsnano.9b09871
Yuwen, L., Qiu, Q., Xiu, W., Yang, K., Li, Y., Xiao, H., et al. (2021). Hyaluronidase-responsive phototheranostic nanoagents for fluorescence imaging and photothermal/photodynamic therapy of methicillin-resistant Staphylococcus aureus infections. Biomater. Sci. 9, 4484–4495. doi:10.1039/d1bm00406a
Zawadzka, K., Felczak, A., Nowak, M., Kowalczyk, A., Piwoński, I., and Lisowska, K. J. (2021). Antimicrobial activity and toxicological risk assessment of silver nanoparticles synthesized using an eco-friendly method with Gloeophyllum striatum. J. Hazard. Mat. 418, 126316. doi:10.1016/j.jhazmat.2021.126316
Zhang, M., Wang, W., Mohammadniaei, M., Zheng, T., Zhang, Q., Ashley, J., et al. (2021a). Upregulating aggregation-induced-emission nanoparticles with blood–tumor-barrier permeability for precise photothermal eradication of brain tumors and induction of local immune responses. Adv. Mat. 33, 2008802. doi:10.1002/adma.202008802
Zhang, M., Wu, F., Wang, W., Shen, J., Zhou, N., and Wu, C. J. (2018). Multifunctional nanocomposites for targeted, photothermal, and chemotherapy. photothermal, Chemother. 31, 1847–1859. doi:10.1021/acs.chemmater.8b00934
Zhang, S., Ye, J., Liu, X., Wang, G., Qi, Y., Wang, T., et al. (2022a). Dual stimuli-responsive smart fibrous membranes for efficient photothermal/photodynamic/chemo-therapy of drug-resistant bacterial infection. Chem. Eng. J. 432, 134351. doi:10.1016/j.cej.2021.134351
Zhang, S., Ye, J., Liu, X., Wang, Y., Li, C., Fang, J., et al. (2021b). Titanium carbide/zeolite imidazole framework-8/polylactic acid electrospun membrane for near-infrared regulated photothermal/photodynamic therapy of drug-resistant bacterial infections. J. Colloid Interface Sci. 599, 390–403. doi:10.1016/j.jcis.2021.04.109
Zhang, X., Min, Y., Zhang, Q., Wu, S., Fu, W., Wu, J., et al. (2022b). Functionalized Mn3O4 nanosheets with photothermal, photodynamic, and oxidase-like activities triggered by low-powered near-infrared light for synergetic combating multidrug-resistant bacterial infections. Adv. Healthc. Mat. 11, 2200121. doi:10.1002/adhm.202200121
Zhang, X., Zhang, C., Yang, Y., Huang, X., Hang, R., Yao, X. J. C. E. J., et al. (2019). Light-assisted rapid sterilization by a hydrogel incorporated with Ag3PO4/MoS2 composites for efficient wound disinfection. Chem. Eng. J. 374, 596–604. doi:10.1016/j.cej.2019.05.229
Zhang, X., Zhang, G., Zhang, H., Liu, X., Shi, J., Shi, H., et al. (2020). A bifunctional hydrogel incorporated with CuS@ MoS2 microspheres for disinfection and improved wound healing. Chem. Eng. J. 382, 122849. doi:10.1016/j.cej.2019.122849
Zhang, Y., Li, D., Tan, J., Chang, Z., Liu, X., Ma, W., et al. (2021c). Near-infrared regulated nanozymatic/photothermal/photodynamic triple-therapy for combating multidrug-resistant bacterial infections via oxygen-vacancy molybdenum trioxide nanodots. Small 17, 2005739. doi:10.1002/smll.202005739
Zhao, Y., Zhang, Y. H., Zhuge, Z., Tang, Y. H., Tao, W., and Chen, Y. J. A. (2018). Synthesis of a poly-L-lysine/black phosphorus hybrid for biosensors. Anal. Chem. 90, 3149–3155. doi:10.1021/acs.analchem.7b04395
Zhou, J., Wang, W., Zhang, Q., Zhang, Z., Guo, J., and Yan, F. J. C. S. (2022). Oxygen-supplied mesoporous carbon nanoparticles for enhanced photothermal/photodynamic synergetic therapy against antibiotic-resistant bacterial infections. Chem. Sci. 13, 6967–6981. doi:10.1039/d2sc01740g
Zhu, Y., Matsumura, Y., Velayutham, M., Foley, L. M., Hitchens, T. K., and Wagner, W. R. J. B. (2018). Reactive oxygen species scavenging with a biodegradable, thermally responsive hydrogel compatible with soft tissue injection. Biomaterials 177, 98–112. doi:10.1016/j.biomaterials.2018.05.044
Keywords: nanomaterials, photothermal, photodynamic, Synergistic therapy, bacterial infections
Citation: Yan Z, Wang D and Gao Y (2023) Nanomaterials for the treatment of bacterial infection by photothermal/photodynamic synergism. Front. Bioeng. Biotechnol. 11:1192960. doi: 10.3389/fbioe.2023.1192960
Received: 24 March 2023; Accepted: 28 April 2023;
Published: 11 May 2023.
Edited by:
Dongfang Zhou, Southern Medical University, ChinaReviewed by:
Xianwen Wang, Anhui Medical University, ChinaBailong Tao, The First Affiliated Hospital of Chongqing Medical University, China
Yupeng Wang, Southern Medical University, China
Copyright © 2023 Yan, Wang and Gao. This is an open-access article distributed under the terms of the Creative Commons Attribution License (CC BY). The use, distribution or reproduction in other forums is permitted, provided the original author(s) and the copyright owner(s) are credited and that the original publication in this journal is cited, in accordance with accepted academic practice. No use, distribution or reproduction is permitted which does not comply with these terms.
*Correspondence: Yun Gao, Nzc3MDQ2NDVAY211LmVkdS5jbg==
†These authors have contributed equally to this work