- 1Department of Preventive and Rehabilitative Sport Medicine, Institute of Cardiovascular Research and Sport Medicine, German Sport University Cologne, Cologne, Germany
- 2Department of Fitness and Health, IST University of Applied Sciences, Düsseldorf, Germany
- 3AIT Austrian Institute of Technology GmbH, Center for Health and Bioresources, Molecular Diagnostics, Vienna, Austria
The ELSAH (electronic smart patch system for wireless monitoring of molecular biomarkers for healthcare and wellbeing) project has received funding from EU’s Horizon 2020 research and innovation program (grant agreement no. 825549). Its aim is to develop a wearable smart patch-based microneedle sensor system that can simultaneously measure several biomarkers in users’ dermal interstitial fluid. This system could have several use cases based on continuous glucose and lactate monitoring: early detection of (pre-) diabetes mellitus, increasing physical performance through optimal carbohydrate intake, achieving a healthier lifestyle through behavioral changes based on the interpretation of glucose data, performance diagnostics (lactate threshold test), control of optimal training intensities corresponding with certain lactate levels, or warning of diseases/health threats, such as the metabolic syndrome or sepsis associated with increased lactate levels. The ELSAH patch system has a high potential of increasing health and wellbeing in users.
Introduction
Fueled by the growing functionality and affordability of miniaturized electronics, the widespread proliferation of smartphones and increasing consumer desire to monitor health data, wearables for health and wellbeing are on the rise (Heikenfeld et al., 2018). Currently available commercial products such as the Fitbit fitness tracker or the Apple Watch usually monitor several physiological variables and activities including heart rate, heart rate variability, or number of daily steps, which can also help predict maximum oxygen consumption or energy expenditure (Majumder et al., 2017).
An in-depth analysis of the user’s health, performance or stress level requires continuous quantification of molecular biomarkers which, of course, implies direct contact with the user’s biofluids (Heikenfeld et al., 2018). Blood is generally considered the gold reference biofluid to quantify molecular biomarkers. However, blood sampling is an invasive technique that isn’t compatible with the needs of wearable users. To that end, several wearables are currently being developed that use biofluids such as sweat (Sempionatto et al., 2017), saliva (Kim et al., 2014) or ocular fluid (Yao et al., 2011) to measure biomarkers, but these systems often face specific challenges linked to the respective non-invasive sample type. Among others, these challenges include poor correlations of biomarker concentrations to reference levels in blood, contamination or large fluctuations in pH that hamper reliable quantification of the target biomarkers (Mitsubayashi et al., 1994; Heikenfeld, 2016; Heikenfeld et al., 2018).
One way of dealing with this dilemma is to aim for minimally invasive sampling instead. The dermal interstitial fluid (ISF) has proven to be pH stable and highly comparable with the biomarker composition of blood (Tran et al., 2018). Furthermore, ISF can be sampled and analyzed using minimally invasive microneedles that have been rated as pain-free and are not susceptible to contamination (Sazonov, 2014; Ventrelli et al., 2015). Consequently, minimally invasive analysis of the ISF using microneedle-based sensors is the most promising approach for integrating continuous monitoring of biomarkers into wearables for healthcare and wellbeing.
In the ELSAH project, a flexible and integrated smart patch-based wearable sensor system (“ELSAH patch”) is developed to quantitatively monitor several molecular biomarkers via electrochemical detection using a minimally invasive microneedle-based approach (Figure 1). The ELSAH patch will be fully self-sustained by integrating the microneedle biosensor, a microchip, a battery and antenna constructs into the patch, thereby enabling independent measurements and secure wireless data transmission to the user’s mobile phone.
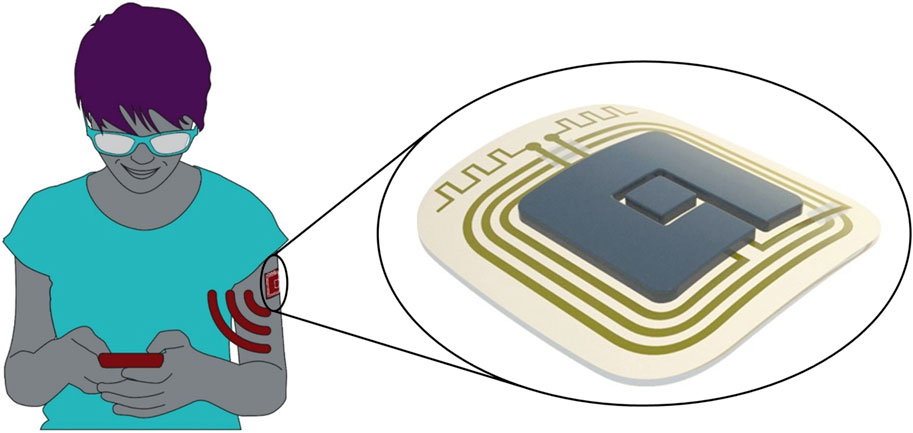
FIGURE 1. ELSAH consists of a smart patch-based microneedle sensor system that measures biomarkers in the user’s interstitial fluid and is connected to a mobile phone. Source and copyright holder: AIT/Purtscher and LEITAT (https://www.elsah.researchproject.at).
In this article, based on the specifications of the ELSAH system, we will present possible applications of the ELSAH patch that we believe can increase health and fitness in users.
ELSAH prototype and measurement technique
The final ELSAH patch will measure the two biomarkers glucose and lactate, which belong to the most reliable metabolic biomarkers to promote a healthy lifestyle (Goodwin et al., 2007; Klonoff et al., 2017). To comply with end user requirements for health and wellbeing monitoring systems (Holzer et al., 2020), the final ELSAH patch aims to feature the following properties:
1) Simultaneous electrochemical quantification of glucose and lactate concentrations in the ISF within a range of 0.3–30 mM;
2) Total monitoring period of 24 h at a measurement interval of 5 min;
3) Total patch area of 4 × 4 cm2 with a maximum patch thickness of 4 mm;
4) Choice of components and integration process resulting in manufacturing costs of a post-project commercial version of no more than EUR 3 per patch.
Various systems are available for determining glucose levels in diabetes care applications (continuous glucose monitoring (CGM) systems) (Rodbard, 2016). Most of them rely on probing the subcutaneous tissue ISF with the use of relatively large needles (5–13 mm in length) (Rodbard, 2016). These needles form electrodes that are functionalized by enzymes (usually glucose oxidase) which catalyze the electrochemical oxidation of glucose, thereby determining glucose concentration (Rodbard, 2016). Owing to the relatively large needles required for this system, it is not always in line with user needs, e.g., due to fear of needles (trypanophobia) and the possibility of subsequent bleeding/bruising (Yeoh et al., 2022). Furthermore, these systems are very expensive and are usually applied for long periods of time (1–2 weeks).
The microneedles used in the ELSAH system (with a microneedle length of approximately 0.5 mm) are pain-free to apply and wear (Ventrelli et al., 2015). Moreover, their production is compatible with mass manufacturing and is therefore inexpensive, they can be regularly exchanged and aren’t susceptible to contamination (Sazonov, 2014). The metallized polymeric microneedles in ELSAH are functionalized by a surface modification protocol involving direct electron transfer enzymes (cellobiose dehydrogenase-based), which enable the oxygen insensitive electrochemical detection of glucose and lactate (Jayakumar et al., 2022). For the quantification of glucose, a research group from the Imperial College London has already demonstrated in human test persons that a clinically acceptable correlation with the glucose level in blood can be obtained using microneedle-based sensing in ISF for an operating period of 24 h (Sharma et al., 2018). Furthermore, the study participants generally reported good tolerability of the microneedles with no long-term skin reactions, thus demonstrating good user comfort (Sharma et al., 2018). The ELSAH microneedles are shown in Figure 2.
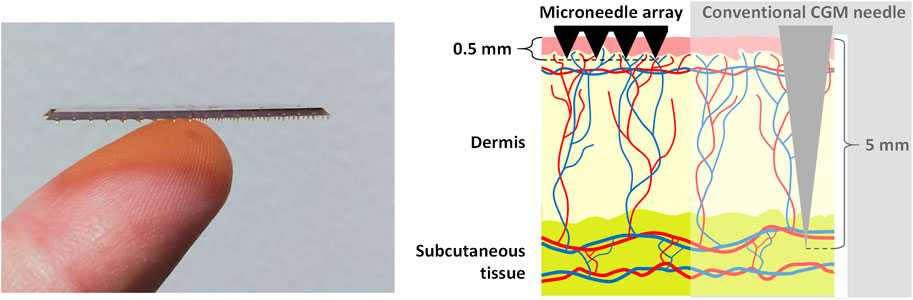
FIGURE 2. ELSAH polymeric microneedles (0.5 mm) compared to a human finger and a conventional CGM needle (≈5 mm) when inserted in the skin. Source and copyright holder: Tyndall National Institute and AIT (https://www.elsah.researchproject.at).
Possible use cases
Glucose is an important energy substrate for the brain, red blood cells, skeletal muscle and other cells and tissues. Hypoglycemia can lead to reduced brain and muscle function and, in the worst case, to hypoglycemic coma and death, while hyperglycemia can induce dehydration and life-threatening hyperglycemic coma (Wintergerst et al., 2006). It is also well-established that chronic increased blood glucose levels can cause cardiovascular complications in the long term (Stratton et al., 2000). Furthermore, hyperglycemia can alter lactate metabolism in the long term (Brinkmann and Brixius, 2015).
Lactate is released when pyruvate produced from glucose via anaerobic glycolysis is converted into lactate. Thus, it is indicative of the body’s metabolic state (Goodwin et al., 2007). Basal lactate levels can be increased in the context of several diseases/infections (e.g., diabetes mellitus, metabolic syndrome, cancer, sepsis) (Levraut et al., 1998; Wouters, 2002; Gustavsson et al., 2012; Brinkmann and Brixius, 2015; Mariappan et al., 2015; Oedorf et al., 2017; Spencer and Stanton, 2019).
Use case 1: early detection of (pre-)diabetes mellitus
The metabolic state (“healthy,” “pre-diabetes” or “type 2 diabetes mellitus”) can be determined using glucose metrics. An early warning system for (pre-) diabetes mellitus could be established using the ELSAH patch. Common diagnostic methods include the fasting plasma glucose (FPG) test and the 75 g oral glucose tolerance test (OGTT) (Khan et al., 2019). According to the American Diabetes Association (ADA), FPG ≥100 mg/dL (5.5 mmol/L) or plasma glucose values 2 h post-75 g glucose ingestion ≥140 mg/dL (7.8 mmol/L) indicate pre-diabetes, while FPG ≥126 mg/dL (7.0 mmol/L) or plasma glucose values 2 h post-75 g glucose ingestion ≥200 mg/dL (11.1 mmol/L) indicate type 2 diabetes mellitus (ElSayed et al., 2023). The patch could be used to either measure fasting glucose values or glucose values during an OGTT in the ISF, with the ELSAH software providing the corresponding interpretation. In case of any indications of an abnormal glucose metabolism, the system could recommend the user to consult a medical doctor for further clarification. The ELSAH software could also evaluate several CGM-derived glycemic metrics such as “time in range” or glycemic variability over a 24-h period to provide a more detailed interpretation of the user’s glucose metabolism. Glucose variability has been discussed as a new option for determining glycemic control which is strongly correlated with vascular complications (Hirsch and Brownlee, 2005; Nalysnyk et al., 2010). However, CGM metrics are not yet well defined in subjects without diabetes, and additional studies are necessary to improve the accuracy of analyses that detect transitions from a healthy to a (pre-) diabetes state (Gottfried et al., 2022). The advantage of the ELSAH system diabetes test compared to others is that it is painless and bloodless. Furthermore, it is cheaper than having a blood sample taken by a medical doctor, which needs to then be analyzed in a laboratory. It is estimated that around 240 million people worldwide are currently living with undiagnosed diabetes (Ogurtsova et al., 2022). By using the ELSAH system for early detection of the disease, many patients could receive quick medical attention to slow down progression of the disease and prevent secondary complications.
Use case 2: optimization of physical performance
Continuous glucose monitoring provides valuable information about athlete’s nutritional state before, during and post-exercise, and can thus influence decisions on how to improve physical performance (Holzer et al., 2022). There is evidence, for example, that the best times in long endurance runs are most likely achieved with only few high glucose fluctuations, and when a sufficient supply of carbohydrates is guaranteed (Oishi et al., 2018; Ishihara et al., 2020). The number of studies involving healthy subjects is very small; to be able to make more detailed recommendations, further studies in this field are urgently needed. The ELSAH software could define upper and lower limits, for example, and warn the athlete if he/she falls below or exceeds these limits. The athlete could, accordingly, increase or limit his/her carbohydrate consumption. The accuracy of personalized recommendations increases as more performance data are being synchronized with CGM data. The software could be further developed and synchronize data with a self-learning system. To our knowledge, such systems do not yet exist. The ELSAH patch allows for 24-h recording, and athletes can therefore use it as needed to monitor their glucose levels. This reduces costs compared to the use of conventional CGM systems.
Use case 3: achieving a healthier lifestyle through behavioral changes based on the interpretation of glucose data
It has been shown that continuous glucose monitoring can help increase awareness of foods with high glycemic loads, thereby improving nutritional choices (Dehghani Zahedani et al., 2021). Continuous glucose monitoring could furthermore motivate people to increase their physical activity which is an effective way to decrease glucose values/postprandial glucose excursions (Liao et al., 2020; Bellini et al., 2021). The ELSAH system could potentially collect and display data on the individual’s “time in range.” The ADA’s glucose target range recommendation is 70–140 mg/dL (3.9–7.8 mmol/L) for patients with diabetes mellitus. A recent study based on CGM data of 4.805 individuals without diabetes mellitus indicates that a higher amount of time spent within an optimized glucose target range (70–100 mg/dL, 3.9–5.6 mmol/L) is associated with a more favorable cardiometabolic risk profile, while no such link was found for the time spent within the ADA’s target range (Berry et al., 2022). Long-term observational studies must be carried out to determine the actual risk of diseases and all-cause mortality in individuals without diabetes mellitus dependent on the different times they spend in different glucose ranges to answer the question whether stricter targets should be recommended for healthy subjects. ELSAH patches can be used as a cost-effective alternative to “classic” CGM systems for the collection of glucose data and can be used in a lifestyle intervention that motivates people to eat healthier or to more regularly be more physically active.
Use case 4: performance diagnostics
Lactate level in blood is elevated (in most people up to ≈8–10 mM) following a progressive incremental exercise test to exhaustion, while the highest lactate levels (≈15–25 mM) are measured in the first few minutes after an “all-out” maximal exertion of 30–120 s (Withers et al., 1991; Goodwin et al., 2007). The ELSAH system could be used as a testing system with instructions for a step endurance test, the measurement of lactate levels and the calculation of individual lactate thresholds (lactate thresholds 1 and 2) beyond which lactate increases more rapidly. (Wackerhage et al., 2022). Lactate thresholds are considered well-established, relatively valid predictors of physical performance (Goodwin et al., 2007). A later increase in lactate levels during exercise testing indicates improved endurance performance (Goodwin et al., 2007). The ELSAH patch is expected to measure lactate more accurately than sensors that measure lactate in other biological fluids, such as sweat, saliva or ocular fluid, because lactate content is more variable in such fluids than in blood or in the ISF (Bollella et al., 2019). There is considerably interest in the development of highly sensitive lactate sensors that can measure lactate in real-time without blood sampling. Hence, lactate performance diagnostics could be carried out not only by sports medicine professionals, but by every coach or by the athlete himself/herself.
Use case 5: determining and controlling training intensities
Exercise intensity is often prescribed by individual’s heart rate based on heart rate formulas which in many cases might be inaccurate (Goldberg et al., 1988). Knowledge of real-time lactate concentrations can be highly relevant for regulating training intensities during exercise as lactate levels adequately reflect the athlete’s metabolic state. (Goodwin et al., 2007). Based on individual performance diagnostics, training can be performed in different intensity ranges at, below or above the individual lactate thresholds. It is expected that training aligned with lactate thresholds provides a more homogenous training stimulus in a group of athletes than training that is based on heart rate formulas (Mann et al., 2013). However, more studies are needed to substantiate the theoretical advantages of threshold-based training programs (Jamnick et al., 2020).
Use case 6: early warning of several diseases/health threats
Lactate can also be co-indicative for several diseases. Studies have shown increased fasting basal lactate levels (at rest), e.g., in patients with metabolic syndrome (≈+25%) (Jones et al., 2019). Basal lactate levels are also increased in the tumor environment in cancer patients (Mariappan et al., 2015; Spencer and Stanton, 2019). More studies are necessary to show the extent to which lactate might increase in neighboring areas in the ISF, and whether lactate in the ISF is a useful marker of tumor progression. Furthermore, lactate levels are associated with the degree of severity of acute infections and are drastically increased in septic patients (Levraut et al., 1998; Gustavsson et al., 2012; Oedorf et al., 2017). Basal lactate >4 mmol/L has been demonstrated to be an independent predictor of deterioration (e.g., acute renal failure, non-elective intubation, vasopressor administration or in-hospital mortality) for intensive care unit patients (Oedorf et al., 2017). Easily applicable continuous monitoring of lactate levels can open up entirely new perspectives for health-conscious end users. The ELSAH system could provide warnings when a certain lactate level is exceeded. The ELSAH system would then recommend to perform a health check by a medical doctor.
Conclusion
The ELSAH patch system has a high potential of increasing health and wellbeing among users. Possible use cases have been presented for further development in the post-project phase. In vivo validation studies on measurement accuracy and further studies on possible lag times (blood→ISF), especially in the case of rapidly changing blood glucose or lactate values (during/post-exercise or meal intake), are needed (Madden et al., 2020). The ELSAH system will be fully self-sustained, allowing for independent measurements and secure wireless data transmission to the user’s mobile phone. Thereby, it assumes a pioneering role in the field of microneedle measuring systems. Microneedle-based technologies have immense potential for use in transdermal diagnostics, and a significant number of academic institutes and companies are developing highly miniaturized, patch-like sensors for biomarker monitoring (Madden et al., 2020; Tasca et al., 2019; Teymourian et al., 2021; biolinq, 2023; nutromics, 2023; sava, 2023). These alternatives to ELSAH are devices that are still being developed, and are manufactured using conventional Printed Circuit Board (PCB) technologies and plastic packaging methods. ELSAH aims to integrate the microneedle-biosensor and microchip into a fully disposable patch by using printed electronics technologies which will provide the antenna for wireless communication and the batteries for energy supply as well. ELSAH patches can be used by individuals who might need it only once in a while, as, for example, for the yearly medical check-up, without the need to invest in expensive electronic equipments, and is therefore affordable for any end user. One important aspect that will have to be addressed in the post-project phase is how to make the ELSAH patch fully environmentally sustainable. To this end, eco-design methodologies and medical device design processes must be incorporated into the future development.
Data availability statement
The original contributions presented in the study are included in the article/supplementary material, further inquiries can be directed to the corresponding author.
Author contributions
CB and GM wrote the manuscript, WB provided intellectual contribution to the manuscript.
Funding
The ELSAH project has received funding from EU’s Horizon 2020 research and innovation program under grant agreement no. 825549.
Acknowledgments
We thank the ELSAH consortium for providing information (https://www.elsah.researchproject.at).
Conflict of interest
CB is a member of the Abbott Advisory Board.
The remaining authors declare that the research was conducted in the absence of any commercial or financial relationships that could be construed as a potential conflict of interest.
Publisher’s note
All claims expressed in this article are solely those of the authors and do not necessarily represent those of their affiliated organizations, or those of the publisher, the editors and the reviewers. Any product that may be evaluated in this article, or claim that may be made by its manufacturer, is not guaranteed or endorsed by the publisher.
References
Bellini, A., Nicolò, A., Bulzomì, R., Bazzucchi, I., and Sacchetti, M. (2021). The effect of different postprandial exercise types on glucose response to breakfast in individuals with type 2 diabetes. Nutrients 13, 1440. doi:10.3390/nu13051440
Berry, S., Bermingham, K., Valdes, A., Franks, P., Wolf, J., and Spector, T. (2022). Optimised glucose “time in range” using continuous glucose monitors in 4,805 non-diabetic individuals is associated with favourable diet and health: The ZOE PREDICT studies. Curr. Dev. Nutr. 6, 1108. doi:10.1093/cdn/nzac078.002
biolinq (2023). biolinq. Available at: https://www.biolinq.com/ (04 15, 2023).
Bollella, P., Sharma, S., Cass, A. E. G., and Antiochia, R. (2019). Microneedle-based biosensor for minimally-invasive lactate detection. Biosens. Bioelectron. 123, 152–159. doi:10.1016/j.bios.2018.08.010
Brinkmann, C., and Brixius, K. (2015). Hyperlactatemia in type 2 diabetes: Can physical training help? J. Diabetes Complicat. 29 (7), 965–969. doi:10.1016/j.jdiacomp.2015.05.018
Dehghani Zahedani, A., Shariat Torbaghan, S., Rahili, S., Karlin, K., Scilley, D., Thakkar, R., et al. (2021). Improvement in glucose regulation using a digital tracker and continuous glucose monitoring in healthy adults and those with type 2 diabetes. Diabetes Ther. 12, 1871–1886. doi:10.1007/s13300-021-01081-3
ElSayed, N. A., Aleppo, G., Aroda, V. R., Bannuru, R. R., Brown, F. M., Bruemmer, D., et al. (2023). 2. Classification and diagnosis of diabetes: Standards of care in diabetes-2023. Diabetes Care 46, S19–S40. doi:10.2337/dc23-s002
Goldberg, L., Elliot, D. L., and Kuehl, K. S. (1988). Assessment of exercise intensity formulas by use of ventilatory threshold. Chest 94 (1), 95–98. doi:10.1378/chest.94.1.95
Goodwin, M. L., Harris, J. E., Hernández, A., and Gladden, L. B. (2007). Blood lactate measurements and analysis during exercise: A guide for clinicians. J. Diabetes Sci. Technol. 1 (4), 558–569. doi:10.1177/193229680700100414
Gottfried, S., Pontiggia, L., Newberg, A., Laynor, G., and Monti, D. (2022). Continuous glucose monitoring metrics for earlier identification of pre-diabetes: Protocol for a systematic review and meta-analysis. BMJ Open 12, e061756. doi:10.1136/bmjopen-2022-061756
Gustavsson, L., Andersson, L-M., Brink, M., Lindh, M., and Westin, J. (2012). Venous lactate levels can be used to identify patients with poor outcome following community-onset norovirus enteritis. Scand. J. Infect. Dis. 44 (10), 782–787. doi:10.3109/00365548.2012.686671
Heikenfeld, J., Jajack, A., Rogers, J., Gutruf, P., Tian, L., Pan, T., et al. (2018). Wearable sensors: Modalities, challenges, and prospects. Lab. Chip 18 (2), 217–248. doi:10.1039/c7lc00914c
Heikenfeld, J. (2016). Non-invasive analyte access and sensing through eccrine sweat: Challenges and outlook circa 2016. Electroanalysis 28 (6), 1242–1249. doi:10.1002/elan.201600018
Hirsch, I. B., and Brownlee, M. (2005). Should minimal blood glucose variability become the gold standard of glycemic control? J. Diabetes Complicat. 19 (3), 178–181. doi:10.1016/j.jdiacomp.2004.10.001
Holzer, R., Bloch, W., and Brinkmann, C. (2022). Continuous glucose monitoring in healthy adults—possible applications in health care, wellness, and sports. Sensors 22 (5), 2030. doi:10.3390/s22052030
Holzer, R., Bloch, W., and Brinkmann, C. (2020). Minimally invasive electrochemical patch-based sensor system for monitoring glucose and lactate in the human body—a survey-based analysis of the end-user’s perspective. Sensors 20 (20), 5761. doi:10.3390/s20205761
Ishihara, K., Uchiyama, N., Kizaki, S., Mori, E., Nonaka, T., and Oneda, H. (2020). Application of continuous glucose monitoring for assessment of individual carbohydrate requirement during ultramarathon race. Nutrients 12 (4), 1121. doi:10.3390/nu12041121
Jamnick, N. A., Pettitt, R. W., Granata, C., Pyne, D. B., and Bishop, D. J. (2020). An examination and critique of current methods to determine exercise intensity. Sports Med. 50, 1729–1756. doi:10.1007/s40279-020-01322-8
Jayakumar, K., Reichhart, T. M. B., Schulz, C., Ludwig, R., Felice, A. K. G., and Leech, D. (2022). An oxygen insensitive amperometric glucose biosensor based on an engineered cellobiose dehydrogenase: Direct versus mediated electron transfer responses. ChemElectroChem 9. doi:10.1002/celc.202200418
Jones, T. E., Pories, W. J., Houmard, J. A., Tanner, C. J., Zheng, D., Zou, K., et al. (2019). Plasma lactate as a marker of metabolic health: Implications of elevated lactate for impairment of aerobic metabolism in the metabolic syndrome. Surgery 166, 861–866. doi:10.1016/j.surg.2019.04.017
Khan, R. M. M., Chua, Z. J. Y., Tan, J. C., Yang, Y., Liao, Z., and Zhao, Y. (2019). From pre-diabetes to diabetes: Diagnosis, treatments and translational research. Medicina: Kaunas).
Kim, J., Valdés-Ramírez, G., Bandodkar, A. J., Jia, W., Martinez, A. G., Ramírez, J., et al. (2014). Non-invasive mouthguard biosensor for continuous salivary monitoring of metabolites. Analyst 139 (7), 1632–1636. doi:10.1039/c3an02359a
Klonoff, D. C., Ahn, D., and Drincic, A. (2017). Continuous glucose monitoring: A review of the technology and clinical use. Diabetes Res. Clin. Pract. 133, 178–192. doi:10.1016/j.diabres.2017.08.005
Levraut, J., Ciebiera, J. P., Chave, S., Rabary, O., Jambou, P., Carles, M., et al. (1998). Mild hyperlactatemia in stable septic patients is due to impaired lactate clearance rather than overproduction. Am. J. Respir. Crit. Care Med. 157 (4), 1021–1026. doi:10.1164/ajrccm.157.4.9705037
Liao, Y., Basen-Engquist, K. M., Urbauer, D. L., Bevers, T. B., Hawk, E., and Schembre, S. M. (2020). Using continuous glucose monitoring to motivate physical activity in overweight and obese adults: A pilot study. Cancer Epidemiol. Biomarkers Prev. 29 (4), 761–768. doi:10.1158/1055-9965.epi-19-0906
Madden, J., O'Mahony, C., Thompson, M., O'Riordan, A., and Galvin, P. (2020). Biosensing in dermal interstitial fluid using microneedle based electrochemical devices. Sens. Biosensing Res. 29, 100348. doi:10.1016/j.sbsr.2020.100348
Majumder, S., Mondal, T., and Deen, M. J. (2017). Wearable sensors for remote health monitoring. Sensors 17 (1), 130. doi:10.3390/s17010130
Mann, T., Lamberts, R. P., and Lambert, M. I. (2013). Methods of prescribing relative exercise intensity: Physiological and practical considerations. Sports Med. 43, 613–625. doi:10.1007/s40279-013-0045-x
Mariappan, R., Venkatraghavan, L., Vertanian, A., Agnihotri, S., Cynthia, S., Reyhani, S., et al. (2015). Serum lactate as a potential biomarker of malignancy in primary adult brain tumours. J. Clin. Neurosci. 22 (1), 144–148. doi:10.1016/j.jocn.2014.06.005
Mitsubayashi, K., Suzuki, M., Tamiya, E., and Karube, I. (1994). Analysis of metabolites in sweat as a measure of physical condition. Anal. Chim. Acta 289 (1), 27–34. doi:10.1016/0003-2670(94)80004-9
Nalysnyk, L., Hernandez-Medina, M., and Krishnarajah, G. (2010). Glycaemic variability and complications in patients with diabetes mellitus: Evidence from a systematic review of the literature. Diabetes Obes. Metab. 12 (4), 288–298. doi:10.1111/j.1463-1326.2009.01160.x
nutromics (2023). nutromics. Available at: https://www.nutromics.com/ 04 15, 2023).
Oedorf, K., Day, D. E., Lior, Y., Novack, V., Sanchez, L., Wolfe, R., et al. (2017). Serum lactate predicts adverse outcomes in emergency department patients with and without infection. West J. Emerg. Med. 18 (2), 258–266. doi:10.5811/westjem.2016.10.31397
Ogurtsova, K., Guariguata, L., Barengo, N. C., Ruiz, P. L-D., Sacre, J. W., Karuranga, S., et al. (2022). IDF Diabetes Atlas: Global estimates of undiagnosed diabetes in adults for 2021. Diabetes Res. Clin. Pract. 183, 109118. doi:10.1016/j.diabres.2021.109118
Oishi, A., Makita, N., Kishi, S., Isogawa, A., and Iiri, T. (2018). Continuous glucose monitoring of a runner during five marathons. Sci. Sports 33, 370–374. doi:10.1016/j.scispo.2018.05.001
Rodbard, D. (2016). Continuous glucose monitoring: A review of successes, challenges, and opportunities. Diabetes Technol. Ther. 18 (2), S2. doi:10.1089/dia.2015.0417
sava (2023). sava. Available at: https://www.sava.health/ (04 15, 2023).
Sazonov, E. (2014). Wearable sensors: Fundamentals, implementation and applications. Burlington: Elsevier Science.
Sempionatto, J. R., Nakagawa, T., Pavinatto, A., Mensah, S. T., Imani, S., Mercier, P., et al. (2017). Eyeglasses based wireless electrolyte and metabolite sensor platform. Lab. Chip 17 (10), 1834–1842. doi:10.1039/c7lc00192d
Sharma, S., El-Laboudi, A., Reddy, M., Jugnee, N., Sivasubramaniyam, S., El Sharkawy, M., et al. (2018). A pilot study in humans of microneedle sensor arrays for continuous glucose monitoring. Anal. Methods 10 (18), 2088–2095. doi:10.1039/c8ay00264a
Spencer, N. Y., and Stanton, R. C. (2019). The Warburg effect, lactate, and nearly a century of trying to cure cancer. Semin. Nephrol. 39, 380–393. doi:10.1016/j.semnephrol.2019.04.007
Stratton, I. M., Adler, A. I., Neil, H. A., Matthews, H. R., Manley, S. E., Cull, C. A., et al. (2000). Association of glycaemia with macrovascular and microvascular complications of type 2 diabetes (UKPDS 35): Prospective observational study. BMJ 321 (7258), 405–412. doi:10.1136/bmj.321.7258.405
Tasca, F., Tortolini, C., Bollella, P., and Antiochia, R. (2019). Microneedle-based electrochemical devices for transdermal biosensing: A review. Curr. Opin. Electrochem 16, 42–49. doi:10.1016/j.coelec.2019.04.003
Teymourian, H., Tehrani, F., Mahato, K., and Wang, J. (2021). Lab under the skin: Microneedle based wearable devices. Adv. Healthc. Mater 10 (17), e2002255. doi:10.1002/adhm.202002255
Tran, B. Q., Miller, P. R., Taylor, R. M., Boyd, G., Mach, P. M., Rosenzweig, C. N., et al. (2018). Proteomic characterization of dermal interstitial fluid extracted using a novel microneedle-assisted technique. J. Proteome Res. 17 (1), 479–485. doi:10.1021/acs.jproteome.7b00642
Ventrelli, L., Marsilio Strambini, L., and Barillaro, G. (2015). Microneedles for transdermal biosensing: Current picture and future direction. Adv. Healthc. Mater 4 (17), 2606–2640. doi:10.1002/adhm.201500450
Wackerhage, H., Gehlert, S., Schulz, H., Weber, S., Ring-Dimitriou, S., and Heine, O. (2022). Lactate thresholds and the simulation of human energy metabolism: Contributions by the cologne sports medicine group in the 1970s and 1980s. Front. Physiol. 13, 899670. doi:10.3389/fphys.2022.899670
Wintergerst, K. A., Buckingham, B., Gandrud, L., Wong, B. J., Kache, S., and Wilson, D. M. (2006). Association of hypoglycemia, hyperglycemia, and glucose variability with morbidity and death in the pediatric intensive care unit. Pediatrics 118 (1), 173–179. doi:10.1542/peds.2005-1819
Withers, R. T., Sherman, W. M., Clark, D. G., Esselbach, P. C., Nolan, S. R., Mackay, M. H., et al. (1991). Muscle metabolism during 30, 60 and 90 s of maximal cycling on an air-braked ergometer. Eur. J. Appl. Physiol. Occup. Physiol. 63, 354–362. doi:10.1007/bf00364462
Wouters, E. F. M. (2002). Chronic obstructive pulmonary disease. 5: Systemic effects of COPD. Thorax 57 (12), 1067–1070. doi:10.1136/thorax.57.12.1067
Yao, H., Shum, A. J., Cowan, M., Lähdesmäki, I., and Parviz, B. A. (2011). A contact lens with embedded sensor for monitoring tear glucose level. Biosens. Bioelectron. 26 (7), 3290–3296. doi:10.1016/j.bios.2010.12.042
Keywords: continuous glucose monitoring, glucose, lactate, microneedle, patch, sensor
Citation: Brinkmann C, Bloch W and Mutinati GC (2023) ELSAH (electronic smart patch system for wireless monitoring of molecular biomarkers for healthcare and wellbeing): definition of possible use cases. Front. Bioeng. Biotechnol. 11:1166857. doi: 10.3389/fbioe.2023.1166857
Received: 15 February 2023; Accepted: 18 April 2023;
Published: 04 May 2023.
Edited by:
Nan Zhu, Dalian University of Technology, ChinaReviewed by:
Yu Jiang, Nanjing Tech University, ChinaCopyright © 2023 Brinkmann, Bloch and Mutinati. This is an open-access article distributed under the terms of the Creative Commons Attribution License (CC BY). The use, distribution or reproduction in other forums is permitted, provided the original author(s) and the copyright owner(s) are credited and that the original publication in this journal is cited, in accordance with accepted academic practice. No use, distribution or reproduction is permitted which does not comply with these terms.
*Correspondence: Christian Brinkmann, Y2guYnJpbmttYW5uQGdteC5uZXQ=