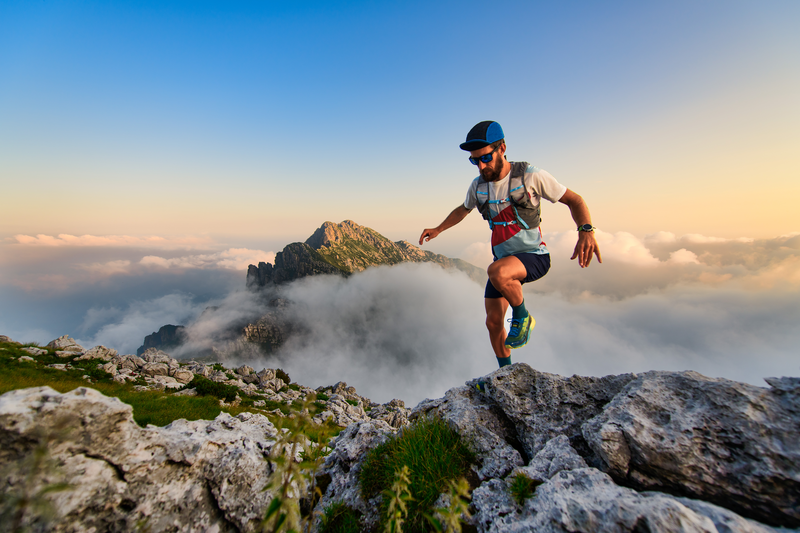
95% of researchers rate our articles as excellent or good
Learn more about the work of our research integrity team to safeguard the quality of each article we publish.
Find out more
REVIEW article
Front. Bioeng. Biotechnol. , 09 May 2023
Sec. Cell and Gene Therapy
Volume 11 - 2023 | https://doi.org/10.3389/fbioe.2023.1148761
Mesenchymal stromal cells (MSCs) are a heterogeneous population containing multipotent adult stem cells with a multi-lineage differentiation capacity, which differentiated into mesodermal derivatives. MSCs are employed for therapeutic purposes and several investigations have demonstrated that the positive effects of MSC transplants are due to the capacity of MSCs to modulate tissue homeostasis and repair via the activity of their secretome. Indeed, the MSC-derived secretomes are now an alternative strategy to cell transplantation due to their anti-inflammatory, anti-apoptotic, and regenerative effects. The cellular senescence is a dynamic process that leads to permanent cell cycle arrest, loss of healthy cells’ physiological functions and acquiring new activities, which are mainly accrued through the release of many factors, indicated as senescence-associated secretory phenotype (SASP). The senescence occurring in stem cells, such as those present in MSCs, may have detrimental effects on health since it can undermine tissue homeostasis and repair. The analysis of MSC secretome is important either for the MSC transplants and for the therapeutic use of secretome. Indeed, the secretome of MSCs, which is the main mechanism of their therapeutic activity, loses its beneficial functions and acquire negative pro-inflammatory and pro-aging activities when MSCs become senescent. When MSCs or their derivatives are planned to be used for therapeutic purposes, great attention must be paid to these changes. In this review, we analyzed changes occurring in MSC secretome following the switch from healthy to senescence status.
The process of senescence induces loss of cellular functions and is associated with permanent cell cycle exit. Senescence may be induced by the impaired activity of lysosomes and mitochondria, the accumulation of unrepaired or misrepaired DNA, presence of nonfunctional/proteins, the oxidation of cellular macromolecules (Campisi and D’adda Di Fagagna, 2007; Lopez-Otin et al., 2023). Senescence may have beneficial and negative consequences on health: it may promote organismal aging through impairment of tissue renewal and function; it may also have an anti-cancer effect since it can arrest cancer growth. Moreover, senescence may promote wound healing (Campisi and D’adda Di Fagagna, 2007; Kuilman and Peeper, 2009).
The senescence occurring in stem cells, such as those present in mesenchymal stromal cells, may have detrimental effects on health since it can undermine tissue homeostasis and repair. This issue must be carefully evaluated to better understand the physiological organismal aging and when stem cells are used for therapeutic purposes.
MSCs are a heterogenous population containing multipotent adult stem cells with a multi-lineage differentiation capacity, which are commonly differentiated into mesodermal derivatives such as adipocytes, chondrocytes, and osteocytes (Muraglia et al., 2000). MSCs can be derived from various human or animal body tissues including bone marrow, adipose tissue, amniotic fluid from the mid-trimester of pregnancy, umbilical cord, human synovial fluid, and human fetal bone tissue of an early-termination pregnancy (Özcan et al., 2016; Alessio et al., 2018; Alessio et al., 2019a; Gnani et al., 2019; Kehl et al., 2019; Alessio et al., 2020; Ratushnyy et al., 2020; Vassilieva et al., 2020; Grigorieva et al., 2021; Kizilay Mancini et al., 2021; Kwon et al., 2021; Liao et al., 2021; Wang B. et al., 2022; Miura et al., 2022). Besides the multipotential differentiation capacity, MSCs play a key role in tissue homeostasis and regeneration through the secretion of hundreds of biologically active factors (cytokines, chemokines, and growth and survival factors), which have a paracrine and long-range action (Galderisi et al., 2022). MSCs are, nowadays, employed for therapeutic purposes (Galderisi and Giordano, 2014).
According to the minimal criteria for defining multipotent mesenchymal stromal cells by the International Society for Cell and Gene Therapy (ISCT), the isolation and characterization of MSCs for different clinical purposes must fulfill the following major benchmarks: they must be able to adhere to plastic well surfaces; express surface markers of CD90, CD73, and CD105; be unable to express CD45, CD34, CD14α or CD11b, CD79 or CD19, and HLA-DR surface molecules; and able to differentiate into adipocytes, chondroblasts, and osteoblasts (Dominici et al., 2006). Although these criteria are basically employed in several stem cell laboratories for the identification and purification of MSCs, non-clonal cultures of bone marrow stromal cells may contain variable percentages of multipotent stem cells where committed progenitor and differentiated cells can also be present (Muraglia et al., 2000; Galderisi U et al., 2014).
The self-renewal, in vitro expandability, multipotent differentiation capacity, being less prone to senescence, and non-immunogenic but still immunomodulatory properties of MSCs from various tissue sources have attracted researchers for their clinical applications in the field of regenerative diseases (Zhang et al., 2015; Alessio et al., 2018; Alessio et al., 2019b). MSCs are candidates for cell-based therapeutic strategies for various disease conditions including neurodegenerative disorders, where clinical stabilization of non-option Parkinsonism was observed for at least 6 months, improvements observed in cardiovascular diseases, perianal fistulas associated with Crohn’s disease, COVID-19, bone disorders, and cancers (Giordano et al., 2014; Canesi et al., 2016; Kastrup et al., 2017; Liu S. et al., 2020; Cheng et al., 2020; Hmadcha et al., 2020; Wang et al., 2021; Johnson et al., 2022; Shi et al., 2022; Sohrabi et al., 2022). A clinical trial on the safety and feasibility of adipose-derived stromal cells also demonstrated a safe and feasible treatment of ischemic heart diseases and heart failure (Kastrup et al., 2017).
However, several factors including the risk of tumorigenicity and immunosuppression makes the reputation of stem cell therapies come into question (Madrid et al., 2021). The other essential concerns are the biological, legal, and societal issues related to the use and application of human-derived stem cells (Moradi et al., 2019). Therapeutic use of MSCs also still needs inclusive characterization guidelines to accommodate various sources including bone marrow, adipose tissue, amniotic membrane, umbilical cord, and others to alleviate the regulatory gaps in stem cell-based therapies (Wright et al., 2021). Safety related issues related to cell-based therapies and their products shall be repeatedly tested before their actual therapeutic application for regenerative disorders (Lu et al., 2011). Hence, various legal and ethical barriers may hinder the full exploitation of stem cells in clinical medicine; the laws and standards may be applied to ensure ethical integrity in the clinical practices of stem cell therapy (Moradi et al., 2019).
In recent years, several investigations have demonstrated that the positive effects of MSC transplants are due to the capacity of MSCs to modulate tissue homeostasis via the activity of their secretome (Galderisi et al., 2022). The administration of MSC-derived conditioned medium, either as direct application of MSC-derived whole lysates or extracellular vesicles or exosomes, are now alternative strategies to cell transplantation due to their anti-inflammatory, anti-apoptotic, and regenerative effects (Gudiño and Salas, 2021). Moreover, novel vehicles of MSC-derived products are also currently in consideration for the delivery of miRNAs in cancer therapy (Sohrabi et al., 2022). The changes occurring in the secretome composition of MSCs during senescence may have negative consequences on the body’s health due to the importance of healthy MSC secretome in tissue repair and homeostasis (Caplan and Dennis, 2006; Hsiao et al., 2012). When MSCs or their derivates have to be used for therapeutic purposes, great attention must be paid to these changes.
Stem cell-based therapies can offer the optional potential to cure various kinds of disorders that are incurable or difficult to treat. However, the only stem cell-based products approved by the United States Food and Drug Administration (FDA) for clinical use consists of hematopoietic stem cells derived from cord blood (FDA, 2022). The hematopoietic stem cell-based therapy was approved for limited use in patients with disorders of the hematopoietic system.
In December 2014, the European Medicines Agency (EMA) recommended the first stem cell-based therapy trademarked as Holoclar, containing stem cells, for approval to treat moderate to severe limbal stem cell deficiency that can result in blindness (EMA, 2014). Later in June 2018, Holoclar, which contains corneal epithelial stem cells, sponsored by Holostem Terapie Avanzate S. r.l. Modena, Italy, was also granted an orphan drug designation by the United States Food and Drug Administration for the treatment of limb stem cell deficiency (FDA, 2018).
The United States FDA and EMA are still cautious of the applications of stem cell product therapy, and both warned against using unapproved and unproven cell-based therapies, which may not be safe and effective. The EMA raised a crucial concern in that patients using unproven or unregulated cell-based therapies have reportedly suffered serious, sometimes fatal, side effects including infections, unwanted immune reactions, tumor formation, loss of vision, and bleeding in the brain (EMA, 2014).
A total of 1,179 MSC cell-based clinical trials (CTs) at different phases (I–III) were registered in the www.ClinicalTrials.gov database as of 15 November 2022, using a search term of “mesenchymal stem cell therapy” for the treatment of various conditions such as diabetic nephropathy, hemorrhagic stroke, hemophilia A and B, rheumatoid arthritis, bronchopulmonary dysplasia, autoimmune disorders, ulcerative colitis, acute myocardial infarction, COVID-19, and many other conditions. Currently, an increased number of registered clinical trials and varieties of diseases/conditions were observed compared to the previous records, where 493 CTs and 767 CTs were registered as of 15 June 2015 (Squillaro et al., 2016) and January 2020 (Liu J. et al., 2020), respectively.
About 30% of the total registered stem cell-based CTs were completed while 40 and 18 registered CTs were on terminated and suspended status, respectively (Figure 1), due to several reasons including the necessity of major revisions of the protocol, recruitment difficulties, inclusion defaults, availability of the vaccine, COVID-19 pandemic, political pressure, updates of NIH operational issues, low predictive probability of achieving postulated results, safety and precaution reasons and other unlisted explanations. The rest of the registered stem cell based CTs were under recruiting, active but not yet recruiting, unknown status, and some other status.
FIGURE 1. Current registered clinical trials of MSC based therapy. Data were retrieved from www.ClinicalTrial.org accessed on 15 November 2022.
MSC secretome or conditioned media-based therapy is also a recently introduced therapeutic strategy although the number of registered clinical trials in this category is much less than whole cell-based stem cell therapy. Only 12 registered MSC secretome/conditioned media therapy clinical trials were retrieved from the www.ClinicalTrials.org database, using a search term of “MSC secretome therapy” at the different statuses of complete 2 (17%), recruiting 6 (50%), active but not recruiting 3 (25%), and unknown status 1 (8%) (Figure 2).
FIGURE 2. Current registered clinical trials of MSC secretome or conditioned media-based therapy. Data were retrieved from www.ClinicalTrial.org accessed on 15 November 2022.
The MSC secretome or conditioned medium-based therapy registered CTs were intended for the treatment of nasopharyngeal cancer, COVID-19, polycystic ovary syndrome or infertility, knee osteoarthritis, skin aging, chronic ulcers, ischemic stroke, corneal defect, and Keloid scars. The MSC secretome or conditioned medium-based therapy clinical trials are based on the fact that this alternative strategy may allow the direct application, easy distribution, and access to tissues of soluble secreted active molecules of MSCs, though getting a sufficient volume of secretome/conditioned media in vitro has not yet been resolved (Gudiño and Salas, 2021; Wang B. et al., 2022).
Senescence is from the Latin root word “senex” meaning “slowing old” (Dodig et al., 2019). Cellular senescence is a dynamic process that leads to permanent cell cycle arrest and the loss of healthy cells’ physiological functions and gaining of new ones, which are mainly accrued through the release of many factors (Coppé et al., 2010). These new activities have been collectively indicated as Senescence-Associated Secretory Phenotype (SASP) (Coppé et al., 2010). Subsequently, the semantic extension of the term SASP refers to the secretome of senescent cells. SASP contains many bioactive molecules, such as growth factors, anti-apoptotic factors, pro-inflammatory cytokines, chemokines, and modulators of extracellular environment. Collectively, these factors can promote senescence of normal cells via paracrine signaling and reinforce the senescence process through autocrine mechanism (Coppé et al., 2010).
A number of nuclear stressors, as indicated in Figure 3, can promote the initiation and progression of cellular senescence and SASP in vitro as well as in vivo experimental models (Parikh et al., 2019; Zlotorynski, 2020; Martini and Passos, 2022; Takasugi et al., 2022).
FIGURE 3. The figure depicts the several classes of stressors that can induce senescence: oxidative and oncogenic stresses; DNA damage; proliferative exhaustion; impairment of cellular function, such as mitochondrial and lysosomal activities. The activation of P53, RB, P21, and P16 pathways promote the onset of senescence. The senescent cells secrete SASP that can act in autocrine way to reinforce senescence and in paracrine way to induce secondary senescence in cells that were not directly hit by stressors. Figure was created with Biorender tools (https://www.biorender.com/) whose licence belongs to UG.
The occurrence of cellular senescence is primarily, as far as we know, dependent on the activation of major tumor-suppression pathways controlled by P53, RB1, and P16-INK4A proteins (Coppé et al., 2010), which have been recently considered to be biomarkers to detect and monitor cellular senescence in culture and ex-vivo specimens (Figure 3). As the cellular senescence is triggered by various DNA stressors, the SASP begins to accumulate in the microenvironment of senescent cells or tissues, where these secreted biomolecules can exert either beneficial effects (i.e., tumor suppression) (Alessio et al., 2023) or deleterious effects such as pro-carcinogenic for secondary tumors, cancer relapse, and cancer chemotherapy-induced side effects (Demaria et al., 2017). SASP is also involved in the aging phenomena by promoting secondary senescence in healthy cells surrounding those directly hit by DNA stressors (paracrine effect of SASP) (Roger et al., 2021).
Given the paramount role of SASP in senescence and senescence-related phenomena, currently there are many studies on SASP obtained from various kinds of cells, including MSCs, fibroblasts, endothelial cells, liver stellate cells and epithelial cells of several human organs and murine MSCs, fibroblasts, and other cells of mouse origin as well as some cancer cells (Fausti et al., 2013; Loaiza and Demaria, 2016; Nishizawa et al., 2016; Abbadie et al., 2017; Caroti et al., 2017; Ferreira et al., 2018; Alessio et al., 2019b; Kotla et al., 2019; Dorronsoro et al., 2021; Kerschbaum et al., 2021; Schwartz et al., 2021; Zhang et al., 2021; Gerasymchuk et al., 2022; Yamada et al., 2022).
The intrinsic dynamic nature of senescence and its variability, depending on cell type, cell status, and animal species, makes it difficult to establish a universal indicator to demonstrate the occurrence and progression of the senescence status. Currently, optimized and combined algorithms of different senescence-related biomarkers are the better option for the identification and isolation of senescent cells within a cell population. The main parameters used to identify senescent cells are: i) cell morphology; ii) evaluation of the expression of cell cycle regulators, such as P53, P21-CIP1, P16-INK4A, P38-MAPK, P14-ARF, at their transcriptional and translational levels; iii) detection of the expression of retinoblastoma gene family (RB1, RB2/P130, P107); iv) determination of senescence associated lysosomal β-galactosidase activity (SA-β-gal); v) identification of critical shorting of telomere DNA sequence length; and vi) lipofuscin detection using GL13 staining (Dimri et al., 1995; Debacq-Chainiaux et al., 2009; Georgakopoulou et al., 2013; Alessio et al., 2017; Evangelou et al., 2017; Salmonowicz and Passos, 2017; Son et al., 2019; Jannone et al., 2020; Chen et al., 2021; Ogrodnik, 2021; Guan et al., 2022; Wagner and Wagner, 2022; Weng et al., 2022). There are also website tools that may help in the identification of senescent cells. For instance, the Tumor Cellular Senescence Estimation Resource (TCSER, http://tcser.bmicc.org) can produce a cellular senescence score for quantification of senescence level and senescence related genes (Wang X. et al., 2022).
The SASP content and senescence–associated extracellular vesicles can also be major components of combined biomarkers for identifying senescent cells (Jeon et al., 2019; Basisty et al., 2020).
In youth many physiological functions have a spare capacity to better cope changes in environmental cues. With the age this spare capacity is lost due to the progressive impairment of many molecular mechanisms and physiological functions. These events lead to progressive loss of muscle mass, accumulation of adipose tissue and can contribute to onset of aging-related diseases (Al-Azab et al., 2022; Lu et al., 2022). A wide range of aging-related disorders are now reported from different corners of the world including osteoarthritis or cartilage degenerative diseases (Liu et al., 2022), reduced muscle regenerative processes (Lu et al., 2022), neurodegenerative diseases, cardiovascular diseases, cancer, immune dysfunction (Li et al., 2021), dementia, cataract, chronic obstructive pulmonary diseases (COPD), diabetes mellitus (Lancaster et al., 2018), and depression (Verhoeven et al., 2014).
As previously described, during aging, several biological and physical changes take place in mammalian organisms: immune aging (changes in number of T cells, loss of ability to respond to antigen, low grade inflammation) and cellular senescence are among the most important ones, though the biological aging or cellular senescence may not necessarily correlate with chronological age and are not used as typical hallmarks to monitor aging. However, since cellular senescence is associated with many types of aging related disorders, eliminating senescent cells and attenuation of their SASP components are currently attractive therapeutic strategies (Childs et al., 2015; Dodig et al., 2019; Lee et al., 2022).
In vitro experiments of MSC aging, the self-renewal, differentiation capacity, and stemness properties are always compromised with age, while the expression of P53, P21-CIP1, and P16-INK4A genes and the levels of reactive oxygen species (ROS) increase with chronological age (Kapetanos et al., 2021). The increased expression of the aforementioned cell cycle regulator genes may be directly associated with different spot DNA damages or accumulated reactive oxygen species that promote the process of cellular senescence. Progressive erosion of telomere length of proliferating cells in vitro triggers the DNA damage response (DDR) where the damage sensor ATM (ataxia telangiectasia mutated) kinase stabilizes the tumor suppressor protein (P53) and upregulates a cyclin-dependent kinase inhibitor (P21-CIP1), which in turn prevents cyclin-dependent kinase 2 (CDK2) mediated inactivation of RB1 and the subsequent entry of cells into the S-phase of the cell cycle (Childs et al., 2015; Mijit et al., 2020; Kumari and Jat, 2021). The ATM-P53-P21-CIP1 axis has also been implicated in other DNA damaging stresses including UV or gamma irradiation, chemotherapeutics, and RAS activations (Childs et al., 2015).
Despite the roles of senescence in maintaining normal tissue development, angiogenesis, tissue hemostasis, wound healing, and tumor inhibition, it is also implicated as one of the major causes of aging-associated disorders (McHugh and Gil, 2018). Hence, senescent cells are currently considered an interesting target for synthetic, natural, and biological therapeutic agents (Childs et al., 2015), which can be either senolytic (selectively targets and eliminates senescent cells) or senomorphic (inhibits the physiologic activities of senescent cells) (Martel et al., 2020). On the other hand, restoring senescence through tumor suppressing signaling or oncogene activation may cause cancer cells to respond to various chemotherapies (Childs et al., 2015), while persistent senescence induction may cause unexpected damage of the surrounding tissues through their secreted proteins, SASP (Childs et al., 2015; Watanabe et al., 2017).
During senescence, the MSC secretome, which contains factors that promote tissue repair and homeostasis, undergoes striking modifications in its composition and becomes SASP. When MSCs or their derivatives have to be used for therapeutic purposes, great attention must be paid to these changes.
We performed a concise literature review and compared the composition of SASP released by senescent MSCs (Table 1; Supplementary Files S1, S3) and secretome of healthy MSC populations (Table 2; Supplementary Files S2, S3).
This comparison may be of interest for the reasons we previously discussed, but it has its own intrinsic limits.
1) MSCs from different sources and animal species may differ in their healthy secretome and SASP composition;
2) Senescence induced by different stressors may produce distinct SASPs;
3) Senescence is a dynamic process, SASP composition of early senescent cells differs from that of late senescent cells;
4) Cited studies did not utilize the same methods to identify the factors present in secretome. Some studies performed the western blot analysis, others used dot blots for cytokine detection, and others performed liquid chromatography and mass spectrometry (LC-MS/MS). In some cases, researchers also detected the transcripts that are associated with factors present in SASP.
The most common sources of senescent MSCs were human and mouse bone marrow and adipose tissue, human umbilical cord, and human desquamated endometrium tissue, in which cellular senescence was induced using various genotoxic agents. Whereas healthy or non-senescent MSCs were originated from human bone marrow, adipose tissue, and umbilical cords (Wharton’s Jelly).
The literature included in the review employed several senescence-inducing agents such as H2O2, x-ray, and gamma-ray irradiations, extensive cultivation, exogenous factors: IGF-I, IGF-II, and IGFBP-4 (insulin-like growth factor I, insulin-like growth factor II, insulin-like growth factor binding protein 4), advanced glycation end products (AGE), WNT3A, MSC-derived exosomes, and Doxorubicin treatment (Supplementary File S1). In the literature we reviewed, the secretory phenotypes (which will be discussed in detail below) of senescent and healthy MSCs were detected using qRT-PCR, Western Blot, high resolution MS, LC-MS/MS, proteome profiler assay, immunoassay, inflammatory cytokine array, ELISA, and whole transcriptomic analysis (Supplementary Files S1, S2).
Large fractions of secreted proteins were observed in various functional groups of proteins of senescent MSCs (Table 1). In particular, secreted proteins in the panels of extracellular matrix (ECM) modulators, immunomodulators (pro- and anti-inflammatory), and cytoskeleton groups of SASPs from the senescent MSCs were more abundant relative to the corresponding proteins in secretome of healthy MSCs (Table 2). On the other hand, growth factors and cell cycle, and signaling proteins were elevated in the secretome of healthy MSCs compared to other groups of proteins of the same cell population (Table 2) while these secreted proteins remained unchanged in the counterparts of senescent MSCs. For the sake of clarity, the components identified in healthy MSC secretomes and in the SASP of senescent MSCs were grouped into 7 classes. This classification, even if it is arbitrary, may help in identifying factors that are exclusively present either in healthy or in aged cells.
In detail, healthy MSCs release factors involved in many biological processes, which have roles in preserving the tissue’s healthiness. Some released proteins are regulators of chondrogenesis, osteogenesis, adipogenesis, and angiogenesis (Özcan et al., 2016; Ayaz-Guner et al., 2020; Acar et al., 2021). Of note, bone marrow derived MSC secretome contains factors promoting the growth and the differentiation of glia and neurons. Other factors have immunomodulation activities and are also implicated in chemotaxis and migration of immune cells. Other secretome components play a key role in neutralizing toxic substances and drugs (detoxification activity) as well as counteracting reactive oxygen species (ROS) through many factors involved in oxidation-reduction reactions. Many factors released by MSCs play roles in the remodeling of ECM through the reshaping of glycosaminoglycans and other ECM components. The MSCs also release regulators of key cellular activities, such as synthesis and degradation of proteins and nucleic acids, regulators of metabolism, and modulators of endoplasmic reticulum stress (Özcan et al., 2016; Ayaz-Guner et al., 2020; Acar et al., 2021).
Senescence almost completely abolishes the release of these “protective factors” involved in tissue repair and homeostasis. The SASP of senescent MSCs still contains factors involved in ECM remodeling, regulation of metabolic processes, ox-redox- and immuno-modulators, regulators of synthesis and degradation of proteins and nucleic acids. Nevertheless, these factors are committed to the acquisition of the typical features of senescent cells and the loss of MSC original functions (Özcan et al., 2016; Ayaz-Guner et al., 2020; Acar et al., 2021).
Gene families of P53, RB, CDK inhibitors, growth factors, IGFBPs (insulin-like growth binding factors), prostaglandins, pro-inflammatory cytokines, chemokines, proteases, receptor proteins, cytoskeletal proteins, heat shock proteins, ribosomal proteins, proteasome activators, proangiogenic and angiogenesis related proteins, ECM proteins, fibronectin, collagens, actin binding proteins, SERPIN, and trans-Golgi network (TGN) proteins were identified from SASPs of senescence induced MSC cells (Table 1). The P53, RB, and CDK inhibitors, such as P16-INK4A, P27, and P21-CIP1, are primary genes that are activated under the cellular pathways responding to several DNA damaging stressors. Hence, promotors of these genes endorse their transcription and translation of effector proteins that can change the molecular functions of the cells themselves (autocrine effect) and overall tissue microenvironment (paracrine effect), propagating the stress responses from the senescent cells to the neighboring cells (Figure 3).
Upregulated expressions of these genes and detection of their downstream products in conditioned media of senescent cell populations usually correlated with the alteration of cell cycles, growth arrest, and occurrence of senescent cells in the heterogeneous population of MSC cells (Mijit et al., 2020; Kumari and Jat, 2021). The presence of increased levels of CDK inhibitors (P16-INK4A and P21-CIP1) in response to DNA damaging stresses maintain the de-phosphorylation of RB family proteins. This arrests cell cycle (Ohtani, 2022; Takasugi et al., 2022) and consequently leads to cellular senescence.
The senescence-associated secretory phenotype (SASP) is comprised of pro-inflammatory cytokines, growth factors, and their binding proteins; ECM components and enzymes may establish inflammatory, immunosuppressive, and catabolic microenvironment of the extracellular matrix of the tissues that lead to deleterious effects such as pro-carcinogenesis, tumorigenesis, and metastasis of the already established tumors (Basisty et al., 2020; Mavrogonatou et al., 2020) or to beneficial effects, such as alarming signals and activation of the tissue repairing machineries (Coppé et al., 2010).
Among the pro-inflammatory cytokines, interleukin-6 (IL-6) has been shown to be correlated with the senescence of different cell types including MSCs, keratinocytes, melanocytes, monocytes, fibroblasts, and epithelial cells, which appears to be secreted by persistent DNA-damaging signaling pathways. It directly affects the neighboring cells in the given tissues where it can adhere to GP80 and GP130 IL-6 receptors of epithelial and endothelial cells of experimental models (Coppé et al., 2010). As a consequence, JAK (Janus tyrosin kinase)/STAT (signal transducers and activators of transcription) and other signaling pathways can be activated inducing a self- and cross-reinforced senescence and strengthening tumorigenic capabilities of some cancer cell lines (Kojima et al., 2013; Ortiz-Montero et al., 2017; Taher et al., 2018; Li et al., 2020).
The growth factors and their inhibiting binding proteins are also components of senescence-associated secretory phenotypes (SASP) in the reviewed literature, where their altered representations were correlated to the occurrence of replicative or acute cellular senescence (Table 1).
For instance, VEGFB (vascular endothelial growth factor B) is among the growth factors that was upregulated in WNT3A induced MSC senescence. This factor may facilitate cancer development through promoting vascular angiogenesis of growing tumors (Coppé et al., 2006). The secretory profile of other growth factors like EGF (epidermal growth factor), HGF (hepatic growth factor), FGF (fibroblast growth factor), NGF (neural growth factors), and growth factor inhibitors: IGFBP-2, 3, 4, 5, 6, and 7 (Box 1), also contributed to the autocrine/paracrine effects of senescent cells exerted on their microenvironment of different senescent endothelial, epithelial, and fibroblast cells (Coppé et al., 2010; Zhao et al., 2020).
The extracellular roles of IGFBP-3, IGFBP-4, IGFBP-5, and IGFBP-7 have previously shown autocrine/paracrine senescence effects on i) human endometrial MSCs, ii) human umbilical endothelial cells, iii) BRAF-positive human primary fibroblasts, and iv) melanocytes, respectively. Increased levels of IGFBPs have been detected in the bloodstream of both irradiated mice and humans, and this may correlate with the release of these factors from senescent MSCs and other cell types. Unlike the other IGF binding protein species, IGFBP-6 overexpression leads to increased cellular life span and proved to be a negative regulator of cellular senescence in human fibroblasts (Kim et al., 2007; Wajapeyee et al., 2008; Micutkova et al., 2011; Severino et al., 2013; Alessio et al., 2020; Vassilieva et al., 2020).
The matrix metalloproteinase (MMP) enzymes are among the SASP components of senescence-induced MSCs (Table 1). These are the most important families of Zinc-dependent proteases involved in the functions and control of ECM, whose alterations can contribute to cellular senescence, the aging process, and aging-related disorders (Freitas-Rodríguez et al., 2017). Aging, replicative, or stress induced cellular senescence of MSCs or other stem cells alters the expressions and functions of MMPs that may affect the self-renewal capacity of stem cells, i. e, stemness characteristics, irreversibly degrade the ECM components, and shed cell surface receptors (Freitas-Rodríguez et al., 2017; Levi et al., 2020).
In addition to the degradation of ECM components, collagens in particular, MMPs can signal via cleavage and activation of different proteinase activated receptors (PARs), which triggers the establishment of pro-inflammatory conditions in Lithium-induced senescent endothelial cells (Struewing et al., 2009). In combination with TGF-β1, the MMP family can be sufficient to induce fibroblast senescence and consequent cancer promotion (Gabasa et al., 2021). Consistent upregulation of MMPs secreted by senescent fibroblast cells regulates soluble factors in the SASP, including CXCL/CCL family members of cytokines originating from surrounding neighboring cells, such as leukocytes, tumor cells, endothelial cells, and other cells (Coppé et al., 2010), that make it more complicated to understand the molecular signatures and functions of SASP in the extracellular microenvironment.
The analysis of MSC secretome is important either for the MSC transplants and for the therapeutic use of secretome. Indeed, the secretome of MSCs, which is the main mechanism of their therapeutic activity, loses its beneficial functions and acquire negative pro-inflammatory and pro-aging activities when MSCs become senescent.
Secreted molecules of senescence-induced MSCs including pro-inflammatory cytokines, chemokines, growth factors, insulin-like growth factor binding proteins, serine proteinase inhibitors, matrix metalloproteinases, proteasomes, and extracellular matrix proteins were the most frequently reported secretome components. These factors could be used as biomarkers of senescence-induced conditions in aging-related or chronic diseases and they may be also useful for preliminary evaluation of MSC samples and their derivatives (extracellular vesicles, secretomes) that have to be used in cell therapy. Indeed, inter-donor variability, ex vivo proliferation, in vitro cryopreservation (Galipeau, 2013), stem cell quantity and quality such as the percentage of stem/progenitor cells, surface marker expression, clonal expansion, multi-differentiating capacity, and the presence of senescent cells may also contribute to the unsuccessful MSC clinical trials.
YS and NA conceptualized and drafted the manuscript and prepared the tables, boxes, and figures. UG, NA, and YA revised the manuscript, tables, boxes, and figures. All authors approved the final manuscript before submission to the journal.
The authors would like to gratitude University of Campania, Luigi Vanvitelli, for funding young researchers, Sper.Rice.Base.Giovani Recercatori, 2022. INNERMOST.
The authors declare that the research was conducted in the absence of any commercial or financial relationships that could be construed as a potential conflict of interest.
All claims expressed in this article are solely those of the authors and do not necessarily represent those of their affiliated organizations, or those of the publisher, the editors and the reviewers. Any product that may be evaluated in this article, or claim that may be made by its manufacturer, is not guaranteed or endorsed by the publisher.
The Supplementary Material for this article can be found online at: https://www.frontiersin.org/articles/10.3389/fbioe.2023.1148761/full#supplementary-material
Abbadie, C., Pluquet, O., and Pourtier, A. (2017). Epithelial cell senescence: An adaptive response to pre-carcinogenic stresses? Cell Mol. Life Sci. 74, 4471–4509. doi:10.1007/s00018-017-2587-9
Acar, M. B., Ayaz-Güner, Ş., Gunaydin, Z., Karakukcu, M., Peluso, G., Di Bernardo, G., et al. (2021). Proteomic and biological analysis of the effects of metformin senomorphics on the mesenchymal stromal cells. Front. Bioeng. Biotechnol. 9, 730813. doi:10.3389/fbioe.2021.730813
Al-Azab, M., Safi, M., Idiiatullina, E., Al-Shaebi, F., and Zaky, M. Y. (2022). Aging of mesenchymal stem cell: Machinery, markers, and strategies of fighting. Cell. Mol. Biol. Lett. 27, 69. doi:10.1186/s11658-022-00366-0
Alessio, N., Acar, M. B., Squillaro, T., Aprile, D., Ayaz-Guner, S., Di Bernardo, G., et al. (2023). Progression of irradiated mesenchymal stromal cells from early to late senescence: Changes in SASP composition and anti-tumor properties. Cell Prolif. press, e13401. doi:10.1111/cpr.13401
Alessio, N., Aprile, D., Squillaro, T., Di Bernardo, G., Finicelli, M., Melone, M. A., et al. (2019a). The senescence-associated secretory phenotype (SASP) from mesenchymal stromal cells impairs growth of immortalized prostate cells but has no effect on metastatic prostatic cancer cells. Aging (Albany NY) 11, 5817–5828. doi:10.18632/aging.102172
Alessio, N., Capasso, S., Ferone, A., Di Bernardo, G., Cipollaro, M., Casale, F., et al. (2017). Misidentified human gene functions with mouse models: The case of the retinoblastoma gene family in senescence. Neoplasia 19, 781–790. doi:10.1016/j.neo.2017.06.005
Alessio, N., Pipino, C., Mandatori, D., Di Tomo, P., Ferone, A., Marchiso, M., et al. (2018). Mesenchymal stromal cells from amniotic fluid are less prone to senescence compared to those obtained from bone marrow: An in vitro study. J. Cell Physiol. 233, 8996–9006. doi:10.1002/jcp.26845
Alessio, N., Squillaro, T., Di Bernardo, G., Galano, G., De Rosa, R., Melone, M. a. B., et al. (2020). Increase of circulating IGFBP-4 following genotoxic stress and its implication for senescence. Elife 9, e54523. doi:10.7554/elife.54523
Alessio, N., Squillaro, T., Monda, V., Peluso, G., Monda, M., Melone, M. A., et al. (2019b). Circulating factors present in the sera of naturally skinny people may influence cell commitment and adipocyte differentiation of mesenchymal stromal cells. World J. Stem Cells 11, 180–195. doi:10.4252/wjsc.v11.i3.180
Ayaz-Guner, S., Alessio, N., Acar, M. B., Aprile, D., Özcan, S., Di Bernardo, G., et al. (2020). A comparative study on normal and obese mice indicates that the secretome of mesenchymal stromal cells is influenced by tissue environment and physiopathological conditions. Cell Commun. Signal 18, 118. doi:10.1186/s12964-020-00614-w
Basisty, N., Kale, A., Jeon, O. H., Kuehnemann, C., Payne, T., Rao, C., et al. (2020). A proteomic atlas of senescence-associated secretomes for aging biomarker development. PLoS Biol. 18, e3000599. doi:10.1371/journal.pbio.3000599
Campisi, J., and D'adda Di Fagagna, F. (2007). Cellular senescence: When bad things happen to good cells. Nat. Rev. Mol. Cell Biol. 8, 729–740. doi:10.1038/nrm2233
Canesi, M., Giordano, R., Lazzari, L., Isalberti, M., Isaias, I. U., Benti, R., et al. (2016). Finding a new therapeutic approach for no-option parkinsonisms: Mesenchymal stromal cells for progressive supranuclear palsy. J. Transl. Med. 14, 127. doi:10.1186/s12967-016-0880-2
Caplan, A. I., and Dennis, J. E. (2006). Mesenchymal stem cells as trophic mediators. J. Cell Biochem. 98, 1076–1084. doi:10.1002/jcb.20886
Caroti, C. M., Ahn, H., Salazar, H. F., Joseph, G., Sankar, S. B., Willett, N. J., et al. (2017). A novel technique for accelerated culture of murine mesenchymal stem cells that allows for sustained multipotency. Sci. Rep. 7, 13334. doi:10.1038/s41598-017-13477-y
Chen, X., Feng, J., Chang, Q., Lu, F., and Yuan, Y. (2021). Senescence of donor cells impairs fat graft regeneration by suppressing adipogenesis and increasing expression of senescence-associated secretory phenotype factors. Stem Cell Res. Ther. 12, 311. doi:10.1186/s13287-021-02383-w
Cheng, F., Huang, Z., and Li, Z. (2020). Efficacy and safety of mesenchymal stem cells in treatment of complex perianal fistulas: A meta-analysis. Stem Cells Int. 2020, 1–11. doi:10.1155/2020/8816737
Childs, B. G., Durik, M., Baker, D. J., and Van Deursen, J. M. (2015). Cellular senescence in aging and age-related disease: From mechanisms to therapy. Nat. Med. 21, 1424–1435. doi:10.1038/nm.4000
Coppé, J. P., Desprez, P. Y., Krtolica, A., and Campisi, J. (2010). The senescence-associated secretory phenotype: The dark side of tumor suppression. Annu. Rev. Pathol. 5, 99–118. doi:10.1146/annurev-pathol-121808-102144
Coppé, J. P., Kauser, K., Campisi, J., and Beauséjour, C. M. (2006). Secretion of vascular endothelial growth factor by primary human fibroblasts at senescence. J. Biol. Chem. 281, 29568–29574. doi:10.1074/jbc.m603307200
Debacq-Chainiaux, F., Erusalimsky, J. D., Campisi, J., and Toussaint, O. (2009). Protocols to detect senescence-associated beta-galactosidase (SA-βgal) activity, a biomarker of senescent cells in culture and in vivo. Nat. Protoc. 4, 1798–1806. doi:10.1038/nprot.2009.191
Demaria, M., O'leary, M. N., Chang, J., Shao, L., Liu, S., Alimirah, F., et al. (2017). Cellular senescence promotes adverse effects of chemotherapy and cancer relapse. Cancer Discov. 7, 165–176. doi:10.1158/2159-8290.cd-16-0241
Dimri, G. P., Lee, X., Basile, G., Acosta, M., Scott, G., Roskelley, C., et al. (1995). A biomarker that identifies senescent human cells in culture and in aging skin in vivo. Proc. Natl. Acad. Sci. U. S. A. 92, 9363–9367. doi:10.1073/pnas.92.20.9363
Dodig, S., Čepelak, I., and Pavić, I. (2019). Hallmarks of senescence and aging. Biochem. medica 29, 483–497. doi:10.11613/bm.2019.030501
Dominici, M., Le Blanc, K., Mueller, I., Slaper-Cortenbach, I., Marini, F., Krause, D., et al. (2006). Minimal criteria for defining multipotent mesenchymal stromal cells. The International Society for Cellular Therapy position statement. Cytotherapy 8, 315–317. doi:10.1080/14653240600855905
Dorronsoro, A., Santiago, F. E., Grassi, D., Zhang, T., Lai, R. C., Mcgowan, S. J., et al. (2021). Mesenchymal stem cell-derived extracellular vesicles reduce senescence and extend health span in mouse models of aging. Aging Cell 20, e13337. doi:10.1111/acel.13337
Ema (2014). First stem-cell therapy recommended for approval in EU. New treatment for rare condition caused by burns to the eye. Available at: https://www.ema.europa.eu/en/documents/press-release/first-stem-cell-therapy-recommended-approval-eu_en.pdf.
Evangelou, K., Lougiakis, N., Rizou, S. V., Kotsinas, A., Kletsas, D., Muñoz-Espín, D., et al. (2017). Robust, universal biomarker assay to detect senescent cells in biological specimens. Aging Cell 16, 192–197. doi:10.1111/acel.12545
Fausti, F., Di Agostino, S., Cioce, M., Bielli, P., Sette, C., Pandolfi, P. P., et al. (2013). ATM kinase enables the functional axis of YAP, PML and p53 to ameliorate loss of Werner protein-mediated oncogenic senescence. Cell Death Differ. 20, 1498–1509. doi:10.1038/cdd.2013.101
Fda (2022). U.S. Food and drug administration. Approved cellular and gene therapy products. Available at: https://www.fda.gov/vaccines-blood-Available at: biologics/cellular-gene-therapy-products/approved-cellular-and-gene-therapy-products (Accessed online on November 16, 2022).
Fda (2018). U.S. Food and drug administration. Orphan drug designations and approvals. Available at: https://www.accessdata.fda.gov/scripts/opdlisting/oopd/detailedIndex.cfm?cfgridkey=632218 (Accessed online on Novemeber 16, 2022).
Ferreira, J. R., Teixeira, G. Q., Santos, S. G., Barbosa, M. A., Almeida-Porada, G., and Gonçalves, R. M. (2018). Mesenchymal Stromal Cell Secretome: Influencing Therapeutic Potential by Cellular Pre-conditioning, 9.
Freitas-Rodríguez, S., Folgueras, A. R., and López-Otín, C. (2017). The role of matrix metalloproteinases in aging: Tissue remodeling and beyond. Biochim. Biophys. Acta Mol. Cell Res. 1864, 2015–2025. doi:10.1016/j.bbamcr.2017.05.007
Gabasa, M., Radisky, E. S., Ikemori, R., Bertolini, G., Arshakyan, M., Hockla, A., et al. (2021). MMP1 drives tumor progression in large cell carcinoma of the lung through fibroblast senescence. Cancer Lett. 507, 1–12. doi:10.1016/j.canlet.2021.01.028
Galderisi U, C. A., Mab, M., and Peluso, G. (2014). Is it possible to improve the success rate of cellular therapy based on mesenchymal stem cells? J Stem Cells Res, Rev Rep. 1, 1017.
Galderisi, U., and Giordano, A. (2014). The gap between the physiological and therapeutic roles of mesenchymal stem cells. Med. Res. Rev. 34, 1100–1126. doi:10.1002/med.21322
Galderisi, U., Peluso, G., and Di Bernardo, G. (2022). Clinical trials based on mesenchymal stromal cells are exponentially increasing: Where are we in recent years? Stem Cell Rev. Rep. 18, 23–36. doi:10.1007/s12015-021-10231-w
Galipeau, J. (2013). The mesenchymal stromal cells dilemma--does a negative phase III trial of random donor mesenchymal stromal cells in steroid-resistant graft-versus-host disease represent a death knell or a bump in the road? Cytotherapy 15, 2–8. doi:10.1016/j.jcyt.2012.10.002
Georgakopoulou, E. A., Tsimaratou, K., Evangelou, K., Fernandez Marcos, P. J., Zoumpourlis, V., Trougakos, I. P., et al. (2013). Specific lipofuscin staining as a novel biomarker to detect replicative and stress-induced senescence. A method applicable in cryo-preserved and archival tissues. Aging (Albany NY) 5, 37–50. doi:10.18632/aging.100527
Gerasymchuk, M., Robinson, G. I., Kovalchuk, O., and Kovalchuk, I. (2022). Modeling of the senescence-associated phenotype in human skin fibroblasts. Int. J. Mol. Sci. 23, 7124. doi:10.3390/ijms23137124
Giordano, R., Canesi, M., Isalberti, M., Isaias, I. U., Montemurro, T., Viganò, M., et al. (2014). Autologous mesenchymal stem cell therapy for progressive supranuclear palsy: Translation into a phase I controlled, randomized clinical study. J. Transl. Med. 12, 14. doi:10.1186/1479-5876-12-14
Gnani, D., Crippa, S., Della Volpe, L., Rossella, V., Conti, A., Lettera, E., et al. (2019). An early-senescence state in aged mesenchymal stromal cells contributes to hematopoietic stem and progenitor cell clonogenic impairment through the activation of a pro-inflammatory program. Aging Cell 18, e12933. doi:10.1111/acel.12933
Grigorieva, O., Arbatskiy, M., Novoseletskaya, E., Dyachkova, U., Ishkin, A., Kalinina, N., et al. (2021). Platelet-derived growth factor induces sasp-associated gene expression in human multipotent mesenchymal stromal cells but does not promote cell senescence. Biomedicines 9, 1290. doi:10.3390/biomedicines9101290
Guan, L., Crasta, K. C., and Maier, A. B. (2022). Assessment of cell cycle regulators in human peripheral blood cells as markers of cellular senescence. Ageing Res. Rev. 78, 101634. doi:10.1016/j.arr.2022.101634
Gudiño, V., and Salas, A. (2021). Promise of mesenchymal stem cell lysates in IBD therapy: Are the parts greater than the whole? Dig. Dis. Sci. 66, 932–934. doi:10.1007/s10620-020-06473-2
Hmadcha, A., Martin-Montalvo, A., Gauthier, B. R., Soria, B., and Capilla-Gonzalez, V. (2020). Therapeutic potential of mesenchymal stem cells for cancer therapy. Front. Bioeng. Biotechnol. 8, 43. doi:10.3389/fbioe.2020.00043
Hsiao, S. T., Asgari, A., Lokmic, Z., Sinclair, R., Dusting, G. J., Lim, S. Y., et al. (2012). Comparative analysis of paracrine factor expression in human adult mesenchymal stem cells derived from bone marrow, adipose, and dermal tissue. Stem Cells Dev. 21, 2189–2203. doi:10.1089/scd.2011.0674
Infante, A., and Rodríguez, C. I. J. S. R. (2018). Secretome analysis of in vitro aged human mesenchymal stem cells reveals IGFBP7 as a putative factor for promoting osteogenesis. Sci. Rep. 8, 4632–4643. doi:10.1038/s41598-018-22855-z
Jannone, G., Rozzi, M., Najimi, M., Decottignies, A., and Sokal, E. M. (2020). An optimized protocol for histochemical detection of senescence-associated beta-galactosidase activity in cryopreserved liver tissue. J. Histochem Cytochem 68, 269–278. doi:10.1369/0022155420913534
Jeon, O. H., Wilson, D. R., Clement, C. C., Rathod, S., Cherry, C., Powell, B., et al. (2019). Senescence cell-associated extracellular vesicles serve as osteoarthritis disease and therapeutic markers. JCI Insight 4, e125019. doi:10.1172/jci.insight.125019
Johnson, S., Hoch, J. S., Halabi, W. J., Ko, J., Nolta, J., and Dave, M. (2022). Mesenchymal stem/stromal cell therapy is more cost-effective than fecal diversion for treatment of perianal Crohn’s disease fistulas. Front. Immunol. 13, 859954. doi:10.3389/fimmu.2022.859954
Kapetanos, K., Asimakopoulos, D., Christodoulou, N., Vogt, A., and Khan, W. (2021). Chronological age affects MSC senescence in vitro-A systematic review. Int. J. Mol. Sci. 22, 7945. doi:10.3390/ijms22157945
Kastrup, J., Haack-Sørensen, M., Juhl, M., Harary Søndergaard, R., Follin, B., Drozd Lund, L., et al. (2017). Cryopreserved off-the-shelf allogeneic adipose-derived stromal cells for therapy in patients with ischemic heart disease and heart failure-A safety study. Stem Cells Transl. Med. 6, 1963–1971. doi:10.1002/sctm.17-0040
Kehl, D., Generali, M., Mallone, A., Heller, M., Uldry, A.-C., Cheng, P., et al. (2019). Proteomic analysis of human mesenchymal stromal cell secretomes: A systematic comparison of the angiogenic potential. npj Regen. Med. 4, 8–13. doi:10.1038/s41536-019-0070-y
Kerschbaum, S., Wegrostek, C., Riegel, E., and Czerny, T. (2021). Senescence in a cell culture model for burn wounds. Exp. Mol. Pathol. 122, 104674. doi:10.1016/j.yexmp.2021.104674
Kim, K. S., Seu, Y. B., Baek, S. H., Kim, M. J., Kim, K. J., Kim, J. H., et al. (2007). Induction of cellular senescence by insulin-like growth factor binding protein-5 through a p53-dependent mechanism. Mol. Biol. Cell 18, 4543–4552. doi:10.1091/mbc.e07-03-0280
Kizilay Mancini, O., Huynh, D. N., Menard, L., Shum-Tim, D., Ong, H., Marleau, S., et al. (2021). Ex vivo Ikkβ ablation rescues the immunopotency of mesenchymal stromal cells from diabetics with advanced atherosclerosis. Cardiovasc Res. 117, 756–766. doi:10.1093/cvr/cvaa118
Kojima, H., Inoue, T., Kunimoto, H., and Nakajima, K. (2013). IL-6-STAT3 signaling and premature senescence. Jakstat 2, e25763. doi:10.4161/jkst.25763
Kotla, S., Le, N. T., Vu, H. T., Ko, K. A., Gi, Y. J., Thomas, T. N., et al. (2019). Endothelial senescence-associated secretory phenotype (SASP) is regulated by Makorin-1 ubiquitin E3 ligase. Metabolism Clin. Exp. 100, 153962. doi:10.1016/j.metabol.2019.153962
Kuilman, T., and Peeper, D. S. (2009). Senescence-messaging secretome: SMS-ing cellular stress. Nat. Rev. Cancer 9, 81–94. doi:10.1038/nrc2560
Kumari, R., and Jat, P. (2021). Mechanisms of cellular senescence: Cell cycle arrest and senescence associated secretory phenotype. 9.
Kwon, J. H., Kim, M., Um, S., Lee, H. J., Bae, Y. K., Choi, S. J., et al. (2021). Senescence-associated secretory phenotype suppression mediated by small-sized mesenchymal stem cells delays cellular senescence through TLR2 and TLR5 signaling. Cells 10, 63–63. doi:10.3390/cells10010063
Lancaster, J. J., Koevary, J. W., Chinyere, I. R., and Goldman, S. (2018). The promise of induced pluripotent stem cell–derived cardiomyocytes to treat heart failure. 11, e005425.
Lee, K. A., Flores, R. R., Jang, I. H., Saathoff, A., and Robbins, P. D. (2022). Immune senescence, immunosenescence and aging. Front. Aging 3, 900028. doi:10.3389/fragi.2022.900028
Lehmann, J., Narcisi, R., Franceschini, N., Chatzivasileiou, D., Boer, C. G., Koevoet, W., et al. (2022). WNT/beta-catenin signalling interrupts a senescence-induction cascade in human mesenchymal stem cells that restricts their expansion. Cell Mol. Life Sci. 79, 82. doi:10.1007/s00018-021-04035-x
Levi, N., Papismadov, N., Solomonov, I., Sagi, I., and Krizhanovsky, V. (2020). The ECM path of senescence in aging: Components and modifiers. Febs J. 287, 2636–2646. doi:10.1111/febs.15282
Li, Y., Lu, L., Xie, Y., Chen, X., Tian, L., Liang, Y., et al. (2020). Interleukin-6 knockout inhibits senescence of bone mesenchymal stem cells in high-fat diet-induced bone loss. Front. Endocrinol. (Lausanne) 11, 622950. doi:10.3389/fendo.2020.622950
Li, Z., Zhang, Z., Ren, Y., Wang, Y., Fang, J., Yue, H., et al. (2021). Aging and age-related diseases: From mechanisms to therapeutic strategies. Biogerontology 22, 165–187. doi:10.1007/s10522-021-09910-5
Liao, C. M., Luo, T., Von Der Ohe, J., De Juan Mora, B., Schmitt, R., and Hass, R. (2021). Human MSC-derived exosomes reduce cellular senescence in renal epithelial cells. Int. J. Mol. Sci. 22, 13562. doi:10.3390/ijms222413562
Liu, J., Ding, Y., Liu, Z., and Liang, X. (2020a). Senescence in mesenchymal stem cells: Functional alterations, molecular mechanisms, and rejuvenation strategies, Front. Cell Dev. Biol., 8 258. doi:10.3389/fcell.2020.00258
Liu, S., Xu, X., Liang, S., Chen, Z., Zhang, Y., Qian, A., et al. (2020b). The application of MSCs-derived extracellular vesicles in bone disorders: Novel cell-free therapeutic strategy. Front. Cell Dev. Biol. 8, 619. doi:10.3389/fcell.2020.00619
Liu, Y., Zhang, Z., Li, T., Xu, H., and Zhang, H. (2022). Senescence in osteoarthritis: From mechanism to potential treatment. Arthritis Res. Ther. 24, 174. doi:10.1186/s13075-022-02859-x
Loaiza, N., and Demaria, M. (2016). Cellular senescence and tumor promotion: Is aging the key? Biochimica Biophysica Acta (BBA) - Rev. Cancer 1865, 155–167. doi:10.1016/j.bbcan.2016.01.007
Lopez-Otin, C., Blasco, M. A., Partridge, L., Serrano, M., and Kroemer, G. (2023). Hallmarks of aging: An expanding universe. Cell 186, 243–278. doi:10.1016/j.cell.2022.11.001
Lu, A., Tseng, C., Guo, P., Gao, Z., Whitney, K. E., Kolonin, M. G., et al. (2022). The role of the aging microenvironment on the fate of PDGFRβ lineage cells in skeletal muscle repair. Stem Cell Res. Ther. 13, 405. doi:10.1186/s13287-022-03072-y
Lu, S.-J., Lee, R. J., Napoli, C., Oh, S., Kimbrel, E. A., and Feng, Q. (2011). The promise and therapeutic potential of human ES and iPS cells. Stem Cells Int. 2011, 1–2. doi:10.4061/2011/959275
Madrid, M., Sumen, C., Aivio, S., and Saklayen, N. (2021). Autologous induced pluripotent stem cell-based cell therapies: Promise, progress, and challenges. Curr. Protoc. 1, e88. doi:10.1002/cpz1.88
Martel, J., Ojcius, D. M., Wu, C. Y., Peng, H. H., Voisin, L., Perfettini, J. L., et al. (2020). Emerging use of senolytics and senomorphics against aging and chronic diseases. Med. Res. Rev. 40, 2114–2131. doi:10.1002/med.21702
Martini, H., and Passos, J. F. (2022). Cellular senescence: All roads lead to mitochondria. FEBS J. n/a 290, 1186–1202. doi:10.1111/febs.16361
Mavrogonatou, E., Pratsinis, H., and Kletsas, D. (2020). The role of senescence in cancer development. Semin. Cancer Biol. 62, 182–191. doi:10.1016/j.semcancer.2019.06.018
Mchugh, D., and Gil, J. (2018). Senescence and aging: Causes, consequences, and therapeutic avenues. J. Cell Biol. 217, 65–77. doi:10.1083/jcb.201708092
Micutkova, L., Diener, T., Li, C., Rogowska-Wrzesinska, A., Mueck, C., Huetter, E., et al. (2011). Insulin-like growth factor binding protein-6 delays replicative senescence of human fibroblasts. Mech. Ageing Dev. 132, 468–479. doi:10.1016/j.mad.2011.07.005
Mijit, M., Caracciolo, V., Melillo, A., Amicarelli, F., and Giordano, A. (2020). Role of p53 in the regulation of cellular senescence. Biomolecules 10, 420. doi:10.3390/biom10030420
Miura, Y., Endo, K., Komori, K., and Sekiya, I. (2022). Clearance of senescent cells with ABT-263 improves biological functions of synovial mesenchymal stem cells from osteoarthritis patients. Stem Cell Res. Ther. 13, 222. doi:10.1186/s13287-022-02901-4
Moradi, S., Mahdizadeh, H., Šarić, T., Kim, J., Harati, J., Shahsavarani, H., et al. (2019). Research and therapy with induced pluripotent stem cells (iPSCs): Social, legal, and ethical considerations. Stem Cell Res. Ther. 10, 341. doi:10.1186/s13287-019-1455-y
Muraglia, A., Cancedda, R., and Quarto, R. (2000). Clonal mesenchymal progenitors from human bone marrow differentiate in vitro according to a hierarchical model. J. Cell Sci. 113 (7), 1161–1166. doi:10.1242/jcs.113.7.1161
Nishizawa, H., Iguchi, G., Fukuoka, H., Takahashi, M., Suda, K., Bando, H., et al. (2016). IGF-I induces senescence of hepatic stellate cells and limits fibrosis in a p53-dependent manner. Sci. Rep. 6, 34605. doi:10.1038/srep34605
O'hagan-Wong, K., Nadeau, S., Carrier-Leclerc, A., Apablaza, F., Hamdy, R., Shum-Tim, D., et al. (2016). Increased IL-6 secretion by aged human mesenchymal stromal cells disrupts hematopoietic stem and progenitor cells' homeostasis. Oncotarget 7, 13285–13296. doi:10.18632/oncotarget.7690
Ogrodnik, M. (2021). Cellular aging beyond cellular senescence: Markers of senescence prior to cell cycle arrest in vitro and in vivo. Aging Cell 20, e13338. doi:10.1111/acel.13338
Ohtani, N. (2022). The roles and mechanisms of senescence-associated secretory phenotype (SASP): Can it be controlled by senolysis? Inflammation and regeneration 42.
Ortiz-Montero, P., Londoño-Vallejo, A., and Vernot, J. P. (2017). Senescence-associated IL-6 and IL-8 cytokines induce a self- and cross-reinforced senescence/inflammatory milieu strengthening tumorigenic capabilities in the MCF-7 breast cancer cell line. Cell Commun. Signal 15, 17. doi:10.1186/s12964-017-0172-3
Özcan, S., Alessio, N., Acar, M. B., Mert, E., Omerli, F., Peluso, G., et al. (2016). Unbiased analysis of senescence associated secretory phenotype (SASP) to identify common components following different genotoxic stresses. Aging 8, 1316–1329. doi:10.18632/aging.100971
Özcan, S., Alessio, N., Acar, M. B., Toprak, G., Gönen, Z. B., Peluso, G., et al. (2015). Myeloma cells can corrupt senescent mesenchymal stromal cells and impair their anti-tumor activity. Oncotarget 6, 39482–39492. doi:10.18632/oncotarget.5430
Parikh, P., Britt, R. D., Manlove, L. J., Wicher, S. A., Roesler, A., Ravix, J., et al. (2019). Hyperoxia-induced cellular senescence in fetal airway smooth muscle cells. Am. J. Respir. Cell Mol. Biol. 61, 51–60. doi:10.1165/rcmb.2018-0176oc
Ratushnyy, A., Ezdakova, M., and Buravkova, L. (2020). Secretome of senescent adipose-derived mesenchymal stem cells negatively regulates angiogenesis. Int. J. Mol. Sci. 21, 1802. doi:10.3390/ijms21051802
Roger, L., Tomas, F., and Gire, V. (2021). Mechanisms and regulation of cellular senescence. Int. J. Mol. Sci. 22, 13173. doi:10.3390/ijms222313173
Salmonowicz, H., and Passos, J. F. (2017). Detecting senescence: A new method for an old pigment. Aging Cell 16, 432–434. doi:10.1111/acel.12580
Schwartz, R. E., Shokhirev, M. N., Andrade, L. R., Gutkind, J. S., Iglesias-Bartolome, R., and Shadel, G. S. (2021). Insights into epithelial cell senescence from transcriptome and secretome analysis of human oral keratinocytes. Aging (Albany NY) 13, 4747–4777. doi:10.18632/aging.202658
Severino, V., Alessio, N., Farina, A., Sandomenico, A., Cipollaro, M., Peluso, G., et al. (2013). Insulin-like growth factor binding proteins 4 and 7 released by senescent cells promote premature senescence in mesenchymal stem cells. Cell Death Dis. 4, e911. doi:10.1038/cddis.2013.445
Shi, L., Yuan, X., Yao, W., Wang, S., Zhang, C., Zhang, B., et al. (2022). Human mesenchymal stem cells treatment for severe COVID-19: 1-year follow-up results of a randomized, double-blind, placebo-controlled trial. eBioMedicine 75, 103789. doi:10.1016/j.ebiom.2021.103789
Sohrabi, B., Dayeri, B., Zahedi, E., Khoshbakht, S., Nezamabadi Pour, N., Ranjbar, H., et al. (2022). Mesenchymal stem cell (MSC)-derived exosomes as novel vehicles for delivery of miRNAs in cancer therapy. Cancer Gene Ther. 29, 1105–1116. doi:10.1038/s41417-022-00427-8
Son, H. N., Chi, H. N. Q., Chung, D. C., and Long, L. T. (2019). Morphological changes during replicative senescence in bovine ovarian granulosa cells. Cell cycleGeorget. Tex.) 18, 1490–1497. doi:10.1080/15384101.2019.1624108
Squillaro, T., Peluso, G., and Galderisi, U. (2016). Clinical trials with mesenchymal stem cells: An update. Cell Transpl. 25, 829–848. doi:10.3727/096368915x689622
Struewing, I. T., Durham, S. N., Barnett, C. D., and Mao, C. D. (2009). Enhanced endothelial cell senescence by lithium-induced matrix metalloproteinase-1 expression. J. Biol. Chem. 284, 17595–17606. doi:10.1074/jbc.M109.001735
Taher, M. Y., Davies, D. M., and Maher, J. (2018). The role of the interleukin (IL)-6/IL-6 receptor axis in cancer. Biochem. Soc. Trans. 46, 1449–1462. doi:10.1042/bst20180136
Takasugi, M., Yoshida, Y., Hara, E., and Ohtani, N. (2022). The role of cellular senescence and SASP in tumour microenvironment. FEBS J. 290, 1348–1361. Epub ahead of print. PMID: 35106956. doi:10.1111/febs.16381
Vassilieva, I., Kosheverova, V., Vitte, M., Kamentseva, R., Shatrova, A., Tsupkina, N., et al. (2020). Paracrine senescence of human endometrial mesenchymal stem cells: A role for the insulin-like growth factor binding protein 3. Aging (Albany NY) 12, 1987–2004. doi:10.18632/aging.102737
Verhoeven, J. E., Revesz, D., Wolkowitz, O. M., and Penninx, B. W. (2014). Cellular aging in depression: Permanent imprint or reversible process?: An overview of the current evidence, mechanistic pathways, and targets for interventions. Bioessays 36, 968–978. doi:10.1002/bies.201400068
Voskamp, C., Anderson, L. A., Koevoet, W. J., Barnhoorn, S., Mastroberardino, P. G., Van Osch, G. J., et al. (2021). TWIST1 controls cellular senescence and energy metabolism in mesenchymal stem cells. Eur. Cell Mater 42, 401–414. doi:10.22203/ecm.v042a25
Wagner, K. D., and Wagner, N. (2022). The Senescence Markers p16INK4A, p14ARF/p19ARF, and p21 in Organ Development and Homeostasis. Cells 11.
Wajapeyee, N., Serra, R. W., Zhu, X., Mahalingam, M., and Green, M. R. (2008). Oncogenic BRAF induces senescence and apoptosis through pathways mediated by the secreted protein IGFBP7. Cell 132, 363–374. doi:10.1016/j.cell.2007.12.032
Wang, B., Pang, M., Song, Y., Wang, H., Qi, P., Bai, S., et al. (2022a). Human fetal mesenchymal stem cells secretome promotes scarless diabetic wound healing through heat-shock protein family, e10354.
Wang, S. H., Shetty, A. K., Jin, K., and Chunhua Zhao, R. (2021). Combating COVID-19 with mesenchymal stem/stromal cell therapy: Promise and challenges. Front. Cell Dev. Biol. 8.
Wang, X., Ma, L., Pei, X., Wang, H., Tang, X., Pei, J. F., et al. (2022b). Comprehensive assessment of cellular senescence in the tumor microenvironment. Brief. Bioinform 23, bbac118. doi:10.1093/bib/bbac118
Watanabe, S., Kawamoto, S., Ohtani, N., and Hara, E. (2017). Impact of senescence-associated secretory phenotype and its potential as a therapeutic target for senescence-associated diseases. Cancer Sci. 108, 563–569. doi:10.1111/cas.13184
Weng, Z., Wang, Y., Ouchi, T., Liu, H., Qiao, X., Wu, C., et al. (2022). Mesenchymal stem/stromal cell senescence: Hallmarks, mechanisms, and combating strategies. Stem Cells Transl. Med. 11, 356–371. doi:10.1093/stcltm/szac004
Wright, A., Arthaud-Day, M. L., and Weiss, M. L. (2021). Therapeutic use of mesenchymal stromal cells: The need for inclusive characterization guidelines to accommodate all tissue sources and species. Front. Cell Dev. Biol. 9, 632717. doi:10.3389/fcell.2021.632717
Yamada, Z., Nishio, J., Motomura, K., Mizutani, S., Yamada, S., Mikami, T., et al. (2022). Senescence of alveolar epithelial cells impacts initiation and chronic phases of murine fibrosing interstitial lung disease. Front. Immunol. 13, 935114. doi:10.3389/fimmu.2022.935114
Zhang, J., Huang, X., Wang, H., Liu, X., Zhang, T., Wang, Y., et al. (2015). The challenges and promises of allogeneic mesenchymal stem cells for use as a cell-based therapy. Stem Cell Res. Ther. 6, 234. doi:10.1186/s13287-015-0240-9
Zhang, M., Serna-Salas, S., Damba, T., Borghesan, M., Demaria, M., and Moshage, H. (2021). Hepatic stellate cell senescence in liver fibrosis: Characteristics, mechanisms and perspectives. Mech. Ageing Dev. 199, 111572. doi:10.1016/j.mad.2021.111572
Zhao, Y., Wu, Z., Chanal, M., Guillaumond, F., Goehrig, D., Bachy, S., et al. (2020). Oncogene-induced senescence limits the progression of pancreatic neoplasia through production of activin A. Cancer Res. 80, 3359–3371. doi:10.1158/0008-5472.can-19-3763
Keywords: mesenchymal stromal cells (MSC), aging, senescence, secretome, SASP
Citation: Siraj Y, Galderisi U and Alessio N (2023) Senescence induces fundamental changes in the secretome of mesenchymal stromal cells (MSCs): implications for the therapeutic use of MSCs and their derivates. Front. Bioeng. Biotechnol. 11:1148761. doi: 10.3389/fbioe.2023.1148761
Received: 20 January 2023; Accepted: 24 April 2023;
Published: 09 May 2023.
Edited by:
Miomir Radosav Knezevic, Educell, SloveniaReviewed by:
Primoz J. Rozman, Blood Transfusion Centre of Slovenia, SloveniaCopyright © 2023 Siraj, Galderisi and Alessio. This is an open-access article distributed under the terms of the Creative Commons Attribution License (CC BY). The use, distribution or reproduction in other forums is permitted, provided the original author(s) and the copyright owner(s) are credited and that the original publication in this journal is cited, in accordance with accepted academic practice. No use, distribution or reproduction is permitted which does not comply with these terms.
*Correspondence: Umberto Galderisi, dW1iZXJ0by5nYWxkZXJpc2lAdW5pY2FtcGFuaWEuaXQ=
Disclaimer: All claims expressed in this article are solely those of the authors and do not necessarily represent those of their affiliated organizations, or those of the publisher, the editors and the reviewers. Any product that may be evaluated in this article or claim that may be made by its manufacturer is not guaranteed or endorsed by the publisher.
Research integrity at Frontiers
Learn more about the work of our research integrity team to safeguard the quality of each article we publish.