- 1Institute for Advanced Study, Research Center of Composites and Surface and Interface Engineering, Chengdu University, Chengdu, China
- 2School of Mechanical Engineering, Chengdu University, Chengdu, China
- 3Luzhou Key Laboratory of Oral & Maxillofacial Reconstruction and Regeneration, The Affiliated Stomatological Hospital of Southwest Medical University, Luzhou, China
- 4Institute of Stomatology, Southwest Medical University, Luzhou, China
Fouling, including inorganic, organic, bio-, and composite fouling seriously affects our daily life. To reduce these effects, antifouling strategies including fouling resistance, release, and degrading, have been proposed. Superhydrophobicity, the most widely used characteristic for antifouling that relies on surface wettability, can provide surfaces with antifouling abilities owing to its fouling resistance and/or release effects. PDMS shows valuable and wide applications in many fields, and due to the inherent hydrophobicity, superhydrophobicity can be achieved simply by roughening the surface of pure PDMS or its composites. In this review, we propose a versatile “3M” methodology (materials, methods, and morphologies) to guide the fabrication of superhydrophobic PDMS-based materials for antifouling applications. Regarding materials, pure PDMS, PDMS with nanoparticles, and PDMS with other materials were introduced. The available methods are discussed based on the different materials. Materials based on PDMS with nanoparticles (zero-, one-, two-, and three-dimensional nanoparticles) are discussed systematically as typical examples with different morphologies. Carefully selected materials, methods, and morphologies were reviewed in this paper, which is expected to be a helpful reference for future research on superhydrophobic PDMS-based materials for antifouling applications.
Introduction
There are four main types of fouling according to the nature of the foulant, namely, inorganic, organic, bio-, and composite fouling (He et al., 2021a; He et al., 2021b). Fouling seriously affects daily life. For example, in biofouling (Callow and Callow, 2011; Bixler et al., 2014; Manolakis and Azhar, 2020), various unwanted organisms attach to the surfaces of metallic, ceramic, polymeric, or composite products; this leads to increased fuel consumption and corrosion in marine biofouling (Dobretsov et al., 2013; Mieszkin et al., 2013; Gaw et al., 2017; Hu et al., 2020), hospital-acquired infections in medical biofouling (Jorge et al., 2012; Ammons and Copié, 2013; Leslie et al., 2014), or function decline in industrial biofouling (Bixler and Bhushan, 2012; Liang et al., 2020; Obotey Ezugbe and Rathilal, 2020). Various antifouling strategies involving fouling resistance, release, and degrading, have been proposed (Zhao et al., 2018; Maan et al., 2020). Inspired by nature, such as the anti-wettability of lotus leaf, rice leaf, and shark skin effects, scientists have developed many well-known bionic antifouling coatings with different surface wettability properties (Ball, 1999; Roach et al., 2008; Zhu et al., 2010; Scardino and de Nys, 2011; Wu et al., 2011; Bixler and Bhushan, 2013; Bixler and Bhushan, 2014; Azemar et al., 2015; Jiang et al., 2015; Zhang et al., 2016; Pan et al., 2019; Zarghami et al., 2019; Basu et al., 2020).
In our previous publications, we discussed the relationship between antifouling and surface wettability (He et al., 2021a; He et al., 2021b; Lan et al., 2021; Lei et al., 2021). For example, we summarized the frequent strategies to achieve anti-biofouling polymers for biomedical applications based on different types of surface wettability (He et al., 2021a), including superhydrophilicity (Figure 1A), hydrophilicity (Figure 1B), hydrophobicity (Figure 1C), and superhydrophobicity (Figure 1D). Examples with suitable polymers were introduced for specific applications in vivo and in vitro, such as cardiological (bioprosthetic heart valves, polymeric heart valves, etc.), ophthalmological (intraocular lenses, contact lenses, etc.), nephrological (urinary catheters, hemodialysis membranes, etc.), and other applications (surgical products, sutures, dressings, biosensors, respirators, etc.).
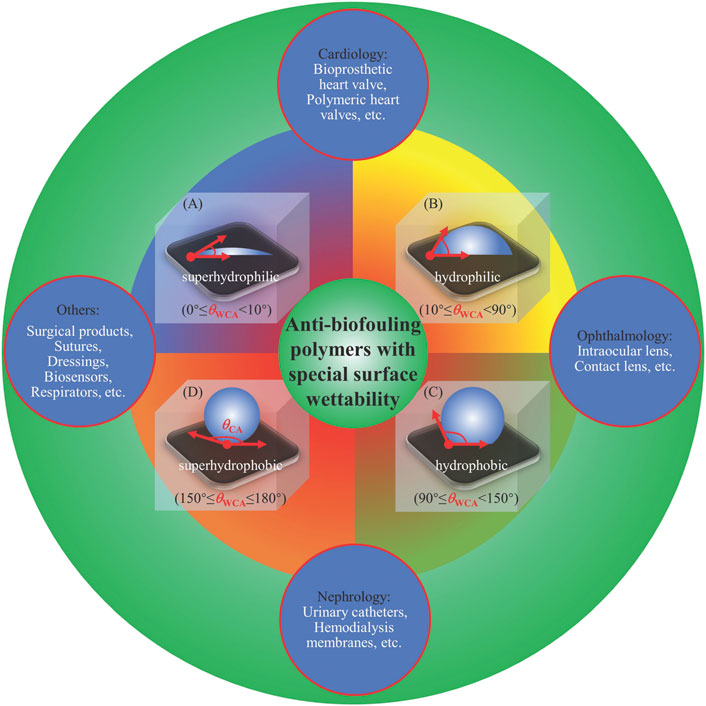
FIGURE 1. Anti-biofouling polymers with different surface wettability for various biomedical applications. Reprinted with permission from Ref. (He et al., 2021a).
Among the four mentioned types of surface wettability, superhydrophobicity (which is the most common research focus in the field) can confer antifouling abilities to various surfaces owing to its fouling-resistant and/or fouling-release properties (Wang and Jiang, 2007; Xia and Jiang, 2008; Ma et al., 2015; Liu et al., 2020a; Simovich et al., 2020). Similarly, other kinds of super-phobicity, such as superoleophobicity, underwater superoleophobicity, and superhemophobicity, can lead to adequate antifouling effects owing to a decreasing adhesion strength between the foulant and substrate (He et al., 2021b). Notably, different types of super-phobicity could be effective against different types of fouling (Figure 2). Superhydrophobicity and superoleophobicity are the most applied super-phobicity strategies in antifouling fields.
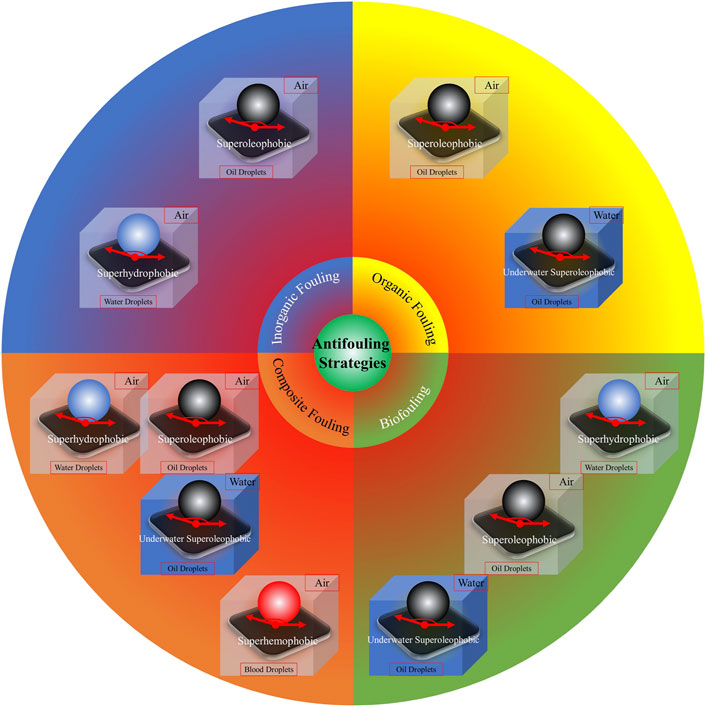
FIGURE 2. Antifouling strategies based on super-phobic surfaces. Reprinted with permission from Ref. (He et al., 2021b). Copyright 2021, Elsevier B.V.
It is known that surface wettability is a result of the surface chemical composition and physical structure (Young, 1805; Zhu et al., 2012; Tian et al., 2014; Yu et al., 2015; Kuang et al., 2016; Martin et al., 2017; Lee et al., 2022; Luo et al., 2022; Zhang et al., 2022). Silicone- or fluoro-based polymers are the main polymeric materials used to achieve superhydrophobicity or superoleophobicity (Dobretsov and Thomason, 2011), and silicone- and fluoro-based polymers with fouling release properties are suitable for achieving antifouling abilities (Carl et al., 2012; Lejars et al., 2012; Liu et al., 2017; Liang et al., 2020). However, fluoro-based materials are expensive and may result in irreversible pollution due to fluoride toxicity (Cao et al., 2022). By contrast, silicone-based polymers, such as polydimethylsiloxane (PDMS), are advantageous owing to their acceptable costs, chemical stability, biocompatibility, and weatherability (Liu et al., 2021). There are 1,025 publications related to superhydrophobic PDMS (Supplementary Figure S1, searched in all fields in Web of Science with “superhydrophobic” and “PDMS” on July 14th, 2022), but only 7 review papers based on this topic are searchable (Supplementary Figure S2). After checked these 7 review papers one by one, there is no review paper focused on the antifouling applications based on superhydrophobic PDMS materials. Therefore, it is necessary to summarize this topic in order to provide a helpful reference for future research on superhydrophobic PDMS-based materials for antifouling applications.
“3M” methodology to obtain superhydrophobic polydimethylsiloxane-based materials
“3M” methodology
A versatile “3M” (materials, methods, and morphologies) methodology to obtain superhydrophobicity easily and universally is proposed in this review as a guide for future research. The “3M” methodology (Figure 3) underlies the strategies for obtaining all types of PDMS-based superhydrophobic materials (pure PDMS, PDMS with nanoparticles, and PDMS with other materials), although each type has its own focus and character. For example, for pure PDMS-based superhydrophobicity, the material is PDMS, but the chosen fabrication method must consider the expected final morphology. Similarly, for PDMS with nanoparticles-based superhydrophobicity, the nanoparticle morphology together with its specific material, and the fabrication method of PDMS with nanoparticles should be considered simultaneously. The “3M” methodology also works for the third superhydrophobicity type (based on PDMS with other materials). Thus, the proposed “3M” methodology can be summarized in the following sentence: “The use of specific materials and methods to construct special morphologies for surface superhydrophobicity;” thus, it can be extrapolated to various fields that require surfaces with superhydrophobicity or other special surface wettability properties.
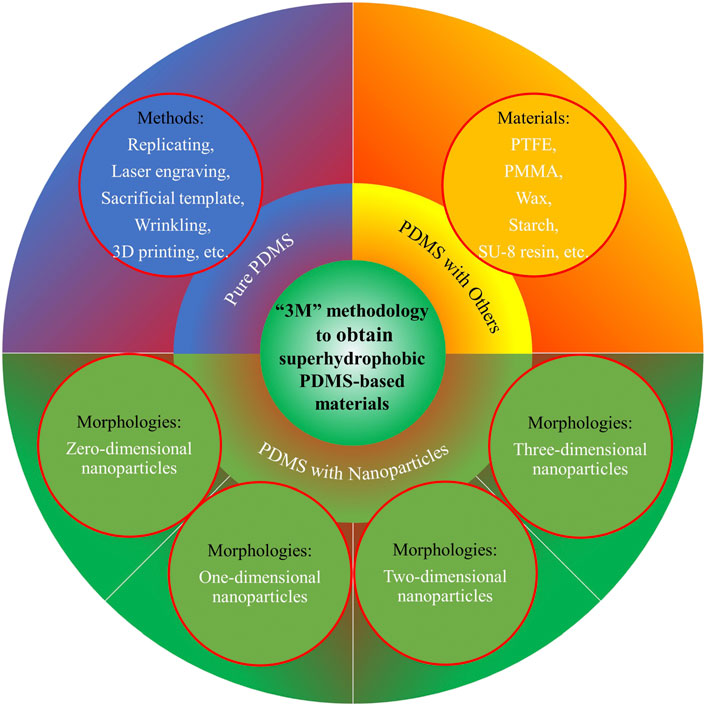
FIGURE 3. A versatile “3M” (materials, methods, and morphologies) methodology to obtain superhydrophobicity on PDMS-based materials.
Superhydrophobicity based on different polydimethylsiloxane materials
PDMS is an optically clear, inert, nontoxic material that is widely applied in medical devices, cosmetics, elastomers, antifoaming agents, flexible sensors, stretchable electronics, and other valuable domestic applications (Das et al., 2018; Zaman et al., 2019; Wang et al., 2021a; Liu et al., 2021; Qi et al., 2021). Due to the inherent hydrophobicity of PDMS, superhydrophobicity can be achieved simply by roughening the surface of pure PDMS or its composites (Figure 3).
Pure PDMS can be roughened to obtain superhydrophobicity via replication (Liu et al., 2006; Cho and Choi, 2008; Park et al., 2011; Dai et al., 2019; Liu et al., 2019; Schultz et al., 2020; Siddiquie et al., 2020), laser engraving (Yong et al., 2013; Yong et al., 2017; Zhao et al., 2019a; Zhang et al., 2020; Chen et al., 2021), introducing a sacrificial template (Yu et al., 2017; Davis et al., 2018), wrinkling (Zhao et al., 2013), 3D printing (He et al., 2017; Chen et al., 2019), and other methods (Zimmermann et al., 2008a; Zimmermann et al., 2008b; Artus and Seeger, 2014; Seo et al., 2016; Wang et al., 2021b; Mazaltarim et al., 2021; Siddiqui et al., 2021; Park et al., 2022). As an example, for the PDMS to achieve superhydrophobicity, the replication methods use different molds, including natural morphologies (lotus leaves (Liu et al., 2006), rose petals (Dai et al., 2019), shark skin surfaces (Liu et al., 2019), etc.), and artificial morphologies (periodic or multiscale structures produced via femtosecond laser processing on stainless-steel substrates (Siddiquie et al., 2020), cylindrical silicon trenches produced via reactive ion etching (Park et al., 2011), polycarbonate spherulite networks produced via a controlled solvent treatment (Schultz et al., 2020), nanoporous anodic aluminum oxides produced via two-step anodization (Cho and Choi, 2008), etc.). Although the methods or morphologies may differ, the purpose is to introduce micro- and nano-scale or hierarchical roughness into the hydrophobic PDMS material to obtain superhydrophobicity.
The case of materials based on PDMS with nanoparticles is different from that of pure PDMS materials because nanoparticles possess inherently rough structures that can be directly exploited to fabricate PDMS-based superhydrophobic surfaces. Nanoparticle materials can be classified by morphology into four types: zero-dimensional nanoparticles (He et al., 2011; He et al., 2012; Zhao et al., 2015; Aslanidou et al., 2016; Selim et al., 2018a; Davis et al., 2018; Liu et al., 2018; Saharudin et al., 2018; Lu et al., 2019; Liu et al., 2020b; Gu et al., 2020; Han and Gong, 2021; Xiong et al., 2022a; Rin Yu et al., 2022; Yu et al., 2022) (such as spherical silicon dioxide (SiO2) (Aslanidou et al., 2016; Yu et al., 2022), titanium dioxide (TiO2) (Liu et al., 2020b), polypyrrole nanoparticles (Xiong et al., 2022a), core-shell spherical composite nanoparticles (Selim et al., 2018a), or hollow spherical nanoclusters (Han and Gong, 2021)), one-dimensional nanoparticles (Wang et al., 2019a; Dai et al., 2019; Selim et al., 2019; Li et al., 2021) (such as linear nanorods (Selim et al., 2019) and carbon nanotubes (CNTs) (Li et al., 2021)), two-dimensional nanoparticles (Wang et al., 2019b; Saharudin et al., 2019; Li and Guo, 2020; Cao et al., 2021) (such as laminar graphene and its derivatives (Li and Guo, 2020), iron oxide (Fe3O4) nanoplates (Cao et al., 2021)), and three-dimensional nanoparticles (single material nanoparticles such as tetrapod-shaped zinc oxide (ZnO) (Yamauchi et al., 2019) and flower-like calcium titanium (CaTiO3) structures (Wang et al., 2007), and composite nanoparticles (Nine et al., 2015; Shi et al., 2015; Barthwal et al., 2020; Zhu et al., 2020; Zhang et al., 2021a; Zhang et al., 2021b; Wu et al., 2021; Selim et al., 2022a; Selim et al., 2022b; Xiong et al., 2022b; Cheng et al., 2022; Miao et al., 2022), among which are dual-sized SiO2 with micropowder and nanofumed morphologies (Zhang et al., 2021a), polydopamine clusters integrated with SiO2 to create micro-nano composite structures (Miao et al., 2022), TiO2 and SiO2 composite nanoparticles (Xiong et al., 2022b), and CNT and Fe3O4 composites (Wu et al., 2021)). For example, SiO2 nanoparticles themselves have nano-scale roughness and the aggregates formed by the particles provide additional hierarchical roughness (He et al., 2011; Gao and Yan, 2012; He et al., 2012; He et al., 2013; Yu et al., 2018). Therefore, materials based on PDMS and nanoparticles easily satisfy the roughness requirements for superhydrophobicity.
For PDMS with other materials, various methods can be used to obtain superhydrophobicity, such as spin coating with PDMS and polytetrafluoroethylene (PTFE) powder (Ruan et al., 2017), electrospinning to produce PDMS and poly (methyl methacrylate) (PMMA) composites (Lu et al., 2021), drop casting or spray coating with PDMS and wax (Zhao et al., 2019b; Torun et al., 2019; Celik et al., 2021), spray coating with PDMS and starch (Wang et al., 2021c), preparing PDMS films with SU-8 resin (Wu et al., 2018), and other methods (Děkanovský et al., 2019; Pakzad et al., 2020; Cao et al., 2022; Zhao et al., 2022). The combined action of the used materials and methods leads to specific morphologies that result in superhydrophobicity.
The comparison of different typical superhydrophobic PDMS-based materials is listed in Table 1. For different types of superhydrophobic PDMS-based materials, materials, methods, and morphologies are summarized and sorted to compare with each other.
Among these three types of superhydrophobic materials based on PDMS, the PDMS with nanoparticles type has many advantages with respect to the other two types. First, the nanoparticles with different morphologies can be obtained easily and inexpensively and may confer other functional properties to the materials, such as photocatalytic (Liu et al., 2020b; Chen et al., 2022), electrical conductivity (Wang et al., 2019c; Li and Guo, 2020), thermochromic (Cheng et al., 2022), and self-illuminous properties (Shi et al., 2015). Second, the methods to achieve superhydrophobicity with PDMS with nanoparticles are based on one-step coating strategies (Steele et al., 2009), which are easier and less costly than strategies such as replicating, laser engraving, and electrospinning. Third, hierarchical roughness can be obtained via the spontaneous formation of nanoparticle aggregates (He et al., 2011; Gao and Yan, 2012; He et al., 2012; He et al., 2013; Yu et al., 2018). Here, we will use materials based on PDMS with nanoparticles to exemplify the “3M” methodology in the following sections.
Superhydrophobicity based on polydimethylsiloxane with nanoparticle aggregates
Nanoparticles with different morphologies form different aggregates; the typical aggregate morphologies are shown in Figure 4. Zero-dimensional nanoparticles may be made of a single, two, or more types of materials with core–shell structures. One-dimensional nanoparticles can have many different morphologies, such as nanorods, nanowires and nanotubes. The morphologies of two-dimensional nanoparticles are usually simple laminar or layered structures. Three-dimensional nanoparticles can consist of single materials (such as the shown tetrapod-shaped or flower-like particles) or composite nanoparticles. The latter can be combinations of differently sized nanoparticles with the same dimensional morphology (for example, raspberry-like structures) or combinations of nanoparticles with different dimensional morphologies (for example, linear one-dimensional nanoparticles on the surface of laminar two-dimensional nanoparticles). Regardless of their specific morphology, nanoparticles aggregate spontaneously to form various hierarchical structures. Usually, aggregates of PDMS with nanoparticles are similar in morphology to those without PDMS. In this context, the nanoparticle aggregates usually provide the necessary hierarchical roughness to achieve superhydrophobicity and the PDMS provides a low surface energy and binds the aggregates together.
Polydimethylsiloxane with zero-dimensional nanoparticles
A facile and universal strategy to fabricate superhydrophobic surfaces via spin-coating a mixture of PDMS and SiO2 nanoparticles on a target substrate was proposed in our previous publications (He et al., 2011; He et al., 2012). As shown in Figure 5A, multi-scale physical structures with micro-scale nanoparticle aggregates and nano-scale single nanoparticles were obtained via one-step coating; this was attributed to spontaneous nanoparticle aggregates (Liu et al., 2008; Wang et al., 2008; Xu et al., 2010). Owing to the low surface energy of PDMS and the hydrophobicity of SiO2 nanoparticles, the final coating exhibited superhydrophobicity with water contact angles (WCA) higher than 150° (Figure 5B).
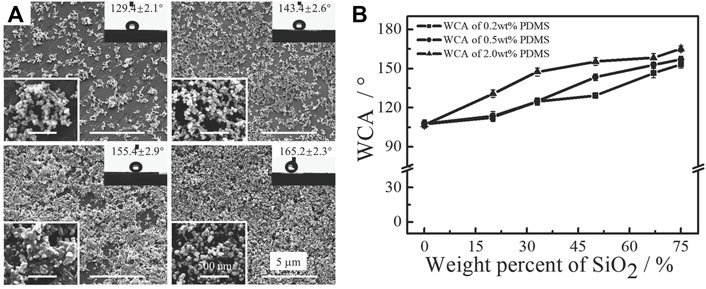
FIGURE 5. Superhydrophobic coatings fabricated with PDMS and SiO2 nanoparticles. SEM images of the physical morphologies (A), and WCA changes with the weight percent of nanoparticles (B). Reprinted with permission from Ref. (He et al., 2012). Copyright 2012, Elsevier B.V.
Similar coating methods have been studied, such as casting, spray-coating, dip-coating, and other methods (Garcia et al., 2010; Zhou et al., 2012; Li et al., 2013; Selim et al., 2018b; Elzaabalawy et al., 2019; Kamelian et al., 2019; Saadatbakhsh et al., 2020), which demonstrates the efficiency of this strategy. Superhydrophobic coatings can be obtained using PDMS and hydrophilic SiO2 nanoparticles (owing to the migration of PDMS molecular chains to the surface (Ju et al., 2017; Su et al., 2017; Davis et al., 2018)) and using PDMS and other types of nanoparticles, such as carbon black (Zhai et al., 2019), TiO2 (Qing et al., 2019; Chen et al., 2022), flame soot (Shen et al., 2013), and other materials (Su et al., 2018; Wang et al., 2018; Li et al., 2022a; Pakdel et al., 2022). Additionally, other functionalities can be combined with superhydrophobicity. For example, Esfandiar Pakdel et al. reported that coatings of PDMS and natural yak hair melanin particles prepared via a dip–pad–dry–cure process exhibited superhydrophobicity, UV protection, and personal thermal management properties (Pakdel et al., 2022).
In addition to single-material spherical nanoparticles, there is another type of zero-dimensional nanoparticles consisting of two or more materials and denominated composite zero-dimensional nanoparticles. Their typical morphologies are core–shell structures (Selim et al., 2018a). Yong Huang et al. fabricated Ag@SiO2 core–shell composite zero-dimensional nanoparticles via a modified Stöber method and obtained a superhydrophobic PDMS and Ag@SiO2 coating via a solution casting method (Figure 6A) (Selim et al., 2018a). The superhydrophobic coating exhibited excellent antifouling abilities against various bio-foulants (Figure 6B).
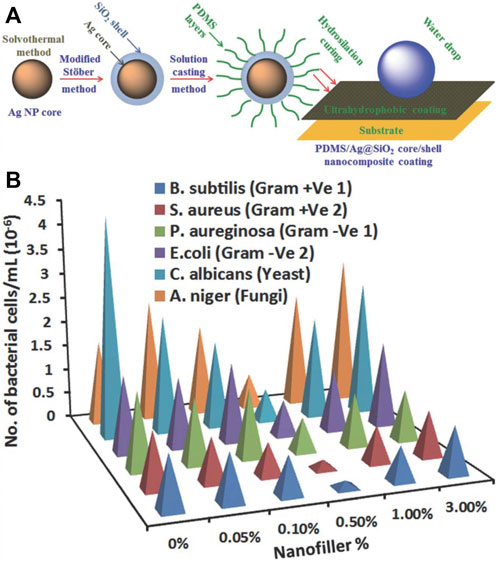
FIGURE 6. Superhydrophobic coatings fabricated by PDMS and Ag@SiO2 core–shell composite zero-dimensional nanoparticles (A). Antifouling behavior of bacteria, yeast, and fungi strains on PDMS materials with different contents of Ag@SiO2 nanoparticles (B). Reprinted with permission from Ref. (Selim et al., 2018a).
Polydimethylsiloxane with one-dimensional nanoparticles
One-dimensional nanoparticles can exhibit nanorod (Selim et al., 2019; Selim et al., 2022a; Selim et al., 2022b), nanowire (Zhang et al., 2013; Li et al., 2020), nanofilament (Zhou et al., 2022), nanotube (Wang et al., 2010), and nanofiber structures (Chen et al., 2009; Liang et al., 2020), among other morphologies. Mohamed S. Selim et al. fabricated a superhydrophobic nanocoating based on PDMS and ZnO nanorods; the coating exhibited long-term antifouling abilities for marine applications (Selim et al., 2019). Zhiguang Guo et al. performed spray-coating of a silk fibroin membrane using a mixture of PDMS and Ag nanowires (Figure 7) (Li et al., 2020). SEM and atomic force microscopy images shown in Figures 7A and 7B revealed the hierarchical structures of a PDMS and Ag nanowire membrane. The prepared membranes exhibited superhydrophobicity, self-cleaning, and antifouling properties (Figures 7C and 7D). Superhydrophobic coatings with similar morphologies have also been obtained via a simple coating process with PDMS and TiO2 nanowires (Zhang et al., 2013) or CNTs (Wang et al., 2010).
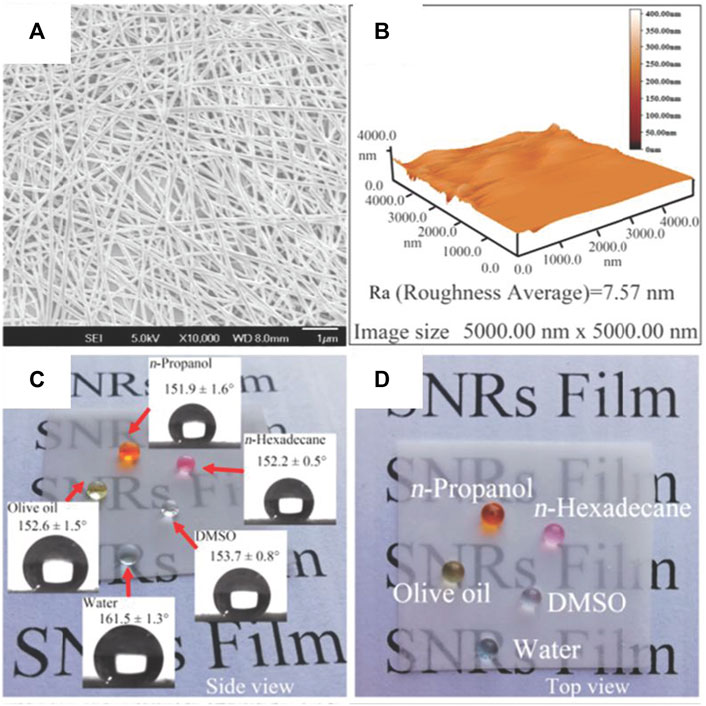
FIGURE 7. SEM (A), atomic force microscopy (B) images, and antiwetting behavior (C,D) of a PDMS and Ag nanowire membrane. Reprinted with permission from Ref. (Li et al., 2020). Copyright 2020, American Chemical Society.
Polydimethylsiloxane with two-dimensional nanoparticles
Two-dimensional nanoparticles can be made of materials such as montmorillonite (Song et al., 2015; Kancı Bozoğlan et al., 2021; Peng et al., 2021), layered silicates (Ho et al., 2012), mica (Han et al., 2019; Miyamoto et al., 2019), graphite (Li and Guo, 2020), graphene, and GO (Wang et al., 2019b; Saharudin et al., 2019; Wang et al., 2021d; Selim et al., 2022a; Selim et al., 2022b). Here, we use the emerging graphene materials as an example to introduce the fabrication of superhydrophobic materials made with PDMS with two-dimensional nanoparticles. Owing to the multi-functionality of graphene, excellent superhydrophobic and photo-responsive properties can be achieved by pouring PDMS and graphene mixtures into templates (Figure 8A) (Wang et al., 2019b). The surface morphologies of PDMS and graphene composites are determined by the hierarchical structures of the two-dimensional graphene nanoparticles (Figures 8B and 8C). The displacement of the beluga whale robot is shown in Figure 8D, and this phenomenon is attributed to the superhydrophobicity of PDMS and graphene composites.
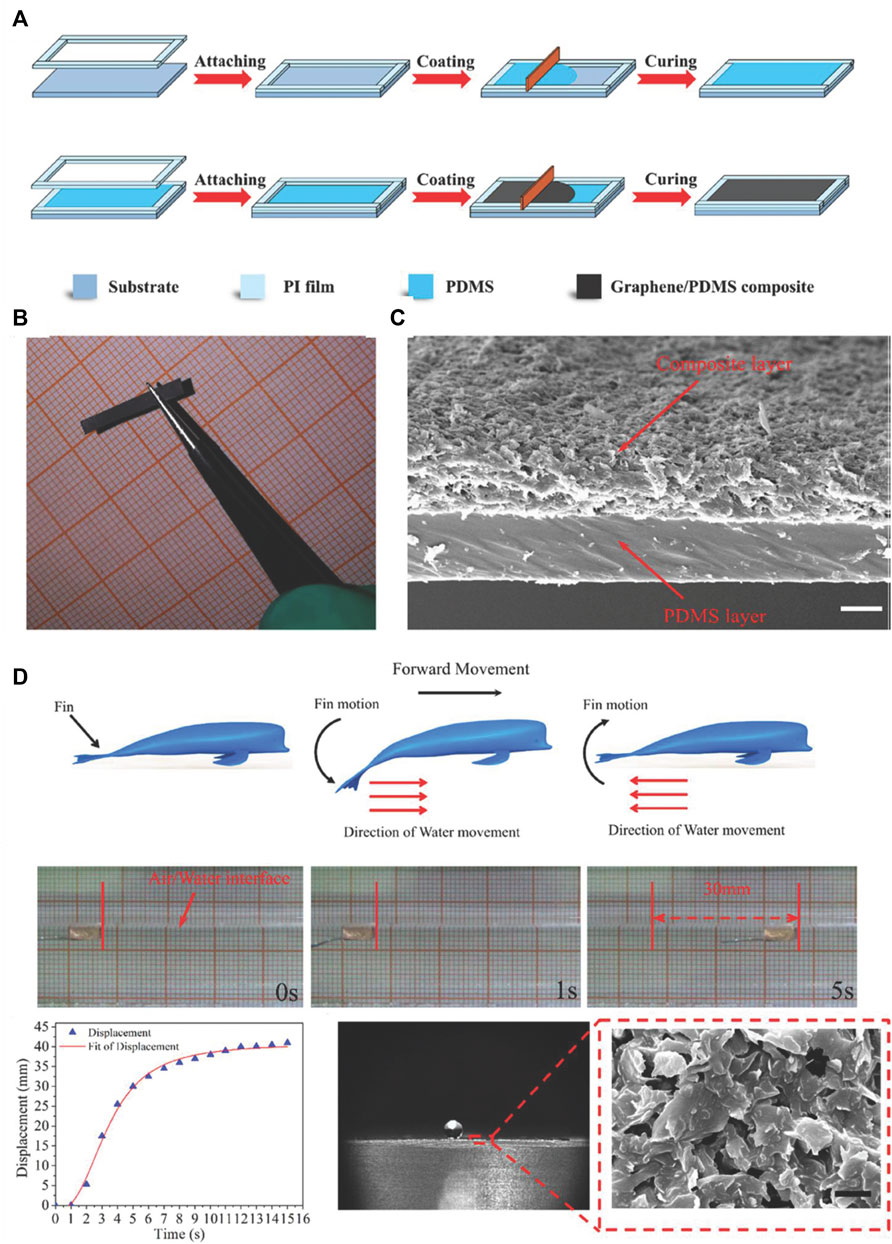
FIGURE 8. Fabrication process of PDMS and graphene materials (A). Optical (B) and SEM (C) images of PDMS and graphene materials. Photo-responsive and superhydrophobic properties of PDMS and graphene materials (D). Reprinted with permission from Ref. (Wang et al., 2019b). Copyright 2019, American Chemical Society.
Polydimethylsiloxane with three-dimensional nanoparticles
Three-dimensional nanoparticles can be categorized into two types. The first type are particles made of single materials, such as tetrapod-shaped ZnO (Yamauchi et al., 2019) and flower-like CaTiO3 structures (Wang et al., 2007). As shown in Figures 9A–9D, Yoshihiro Yamauchi et al. reported superhydrophobic materials made of PDMS and tetrapod-shaped ZnO with porcupinefish-like structures obtained by pouring the composite into a template (Yamauchi et al., 2019). The composite materials exhibited superhydrophobicity not only on the surface but also inside; thus, the superhydrophobicity can be stable even under material abrasion, bending, or twisting deformation (Figures 9E–9G).
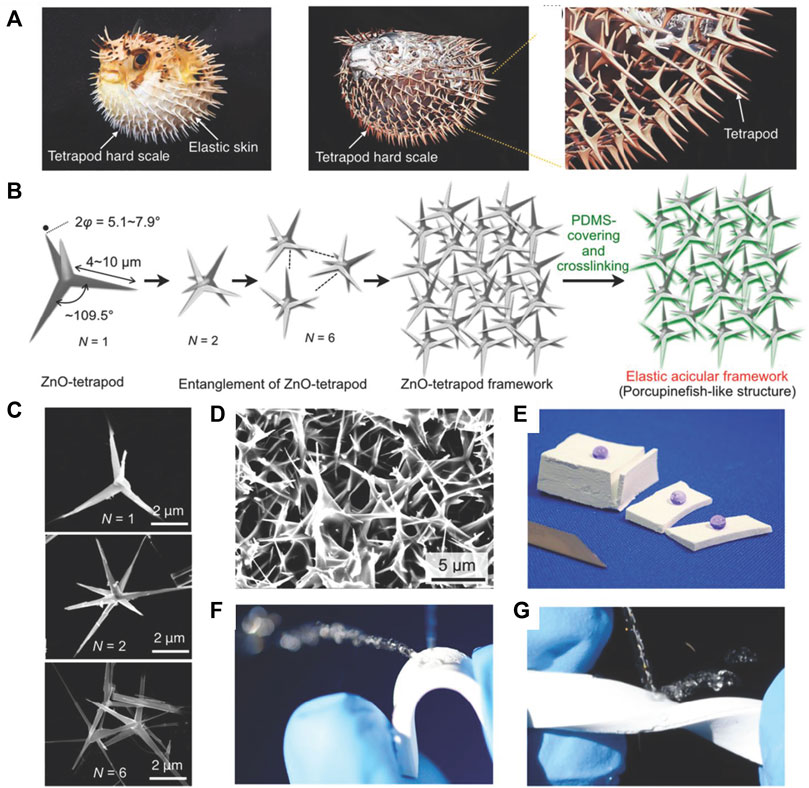
FIGURE 9. Photograph and computer tomography scan images of a porcupinefish and its skeleton (A). Schematic representation of independent tetrapod-shaped ZnO and its composite with PDMS (B). SEM images of tetrapod-shaped ZnO (C) and elastic acicular frameworks (D). Photographs of the materials showing superhydrophobicity with slicing resistance (E), bending resistance (F), and twisting resistance (G). Reprinted with permission from Ref. (Yamauchi et al., 2019). Copyright 2019, American Chemical Society.
The second type of three-dimensional nanoparticles are those consisting of a combination of two or more materials. As shown in Figure 10, three-dimensional nanoparticles with a raspberry-like morphology have been obtained via the aggregation of spherical SiO2 nanoparticles on the surface of spherical thermochromic pigment (TP) particles (Cheng et al., 2022). A thermochromic superhydrophobic coating has been fabricated by brushing a mixture of PDMS and the three-dimensional nanoparticles onto a substrate (Figure 10A). SEM images of a blue TP powder and various coatings are shown in Figure 10B. Coatings made with different TP particles show similar superhydrophobicity, and surface contaminants can easily be washed with water (Figure 10C). A similar superhydrophobic surface made of nanoparticles with zero-dimensional morphology and different sizes was obtained by combining PDMS and CaCO3/SiO2 composite particles with a raspberry-like morphology (Yang et al., 2009). Sumit Barthwal et al. reported a stable superhydrophobic coating based on PDMS and three-dimensional composite nanoparticles assembled with one-dimensional multi-walled CNTs and ZnO nanorods (Barthwal et al., 2020). The multi-walled CNT and ZnO composite nanoparticles were prepared via a sol-gel method, and superhydrophobic coatings were obtained by dip-coating various substrates.
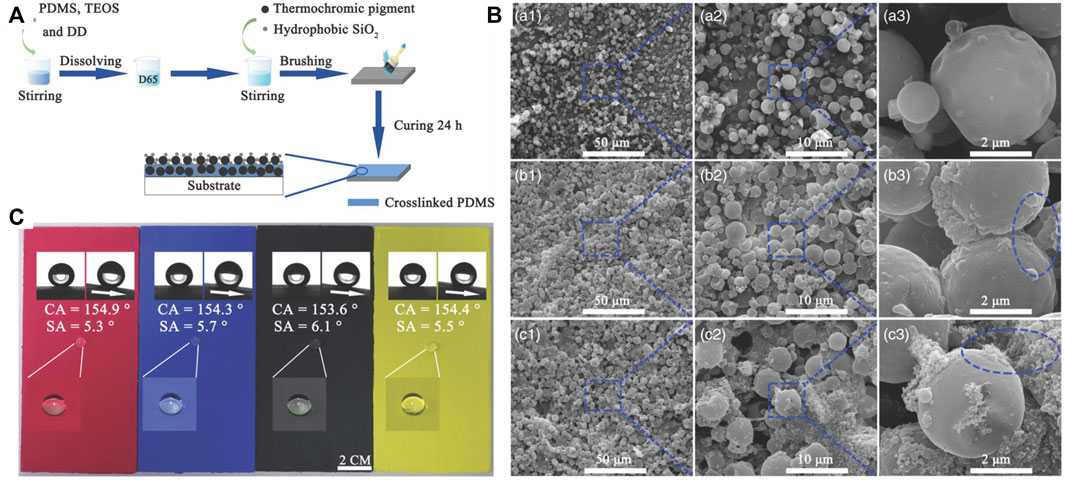
FIGURE 10. Schematic diagram of the fabrication process of thermochromic superhydrophobic coatings (A). SEM images of a blue TP powder and various coatings (B): blue TP powder (a1–a3); blue TP/coating without SiO2 (b1–b3); blue TP/coating containing SiO2 (c1–c3). Surface wettability measurements for different coatings (C): the red, blue, black, and yellow TP/coating. Reprinted with permission from Ref. (Cheng et al., 2022). Copyright 2021, Wiley-VCH GmbH.
Three-dimensional composite nanoparticles can also consist of combinations of nanoparticles with different dimensional morphologies. Mohamed S. Selim et al. developed a simple two-phase process to obtain three-dimensional composite nanoparticles with one-dimensional anatase TiO2 nanorods (Selim et al., 2022a) or boehmite nanorods (c-AlOOH) (Selim et al., 2022b) on the surface of two-dimensional GO sheets. PDMS and three-dimensional composite nanoparticles consisting of nanorods on the surface of GO sheets can be coated onto substrates such as a hull to confer superhydrophobicity and antifouling abilities to the surface. Dusan Losic et al. prepared graphene-based superhydrophobic composite coatings with diatomaceous earth (DE), reduced GO (rGO) and TiO2 (P25) nanoparticles via spraying, brush painting, and dip coating (Nine et al., 2015). The morphologies of DE, TiO2 (P25) and GO particles can be found in Figures 11A and 11B. Due to the hydrophilicity of GO (Figure 11B, WCA 45°), the final superhydrophobic coating was prepared with rGO to avoid the effect of hydrophilic GO. Finally, the coatings with PDMS and DE, DE/TiO2, or DE/TiO2/rGO composite particles showed rough surface morphologies and superhydrophobicity (Figure 11C).
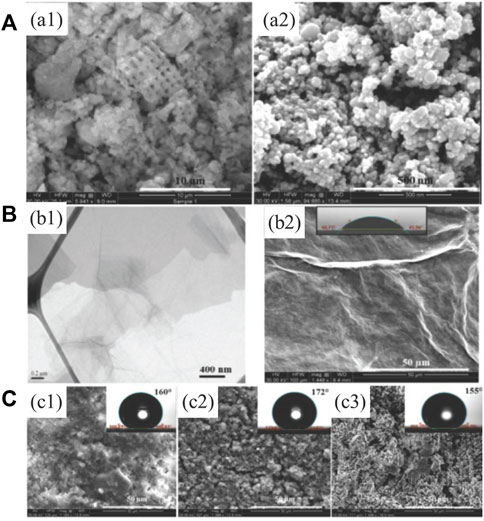
FIGURE 11. SEM images of DE and TiO2 (P25) nanoparticles (A). TEM image of exfoliated GO and SEM image of dried GO flakes (B). SEM images and WCAs on superhydrophobic coating fabricated with PDMS and DE, DE/TiO2, or DE/TiO2/rGO particles (C). Reprinted with permission from Ref. (Nine et al., 2015). Copyright 2015, American Chemical Society.
Owing to the wide variety of potential morphologies of three-dimensional composite nanoparticles, it is impossible to discuss them thoroughly in this review. Nonetheless, other morphologies of three-dimensional composite nanoparticles can be reasonably conceived based on the “3M” methodology and the previous discussion (Dai et al., 2021; Li et al., 2022b; Li et al., 2022c). For example, zero-dimensional carbon black nanoparticles and one-dimensional carbon nanotubes have been mixed to obtain conductive composite nanoparticles, and superhydrophobic materials could be obtained by mixing PDMS and the conductive composite nanoparticles in a solution and curing the PDMS (Li et al., 2022b).
Methods to obtain superhydrophobicity based on polydimethylsiloxane and nanoparticles
The “3M” methodology can be applied to prepare a variety of superhydrophobic coatings. In addition to the previous examples, in which superhydrophobic materials for antifouling applications were obtained based on a combination of PDMS with nanoparticle aggregates (shown for zero-dimensional nanoparticles as an example in Figure 12A), two other strategies can be used to obtain superhydrophobic materials using PDMS and nanoparticles (Figures 12B and 12C).

FIGURE 12. Strategies to obtain superhydrophobicity using PDMS and nanoparticles. Mixing PDMS and nanoparticle aggregates (A); PDMS on the surface of the nanoparticle aggregates (B); Nanoparticle aggregates on a PDMS surface (C).
When PDMS is added on the surface of nanoparticle aggregates (Figure 12B), the surface of the final superhydrophobic material consists of PDMS. Therefore, hydrophilic particles could be used without hydrophobic modifications because the PDMS provides the required hydrophobicity. Figure 13A shows an example in which Ag nanoparticles (AgNPs) were first applied on a rubber band (RB) substrate, and PDMS was then coated on the substrate with AgNPs to confer superhydrophobicity to the material (Wang et al., 2019c). Owing to the existence of a continuous PDMS film on the surface, the superhydrophobicity can be maintained under cyclic stretching–releasing and abrasion tests (Figure 13B).
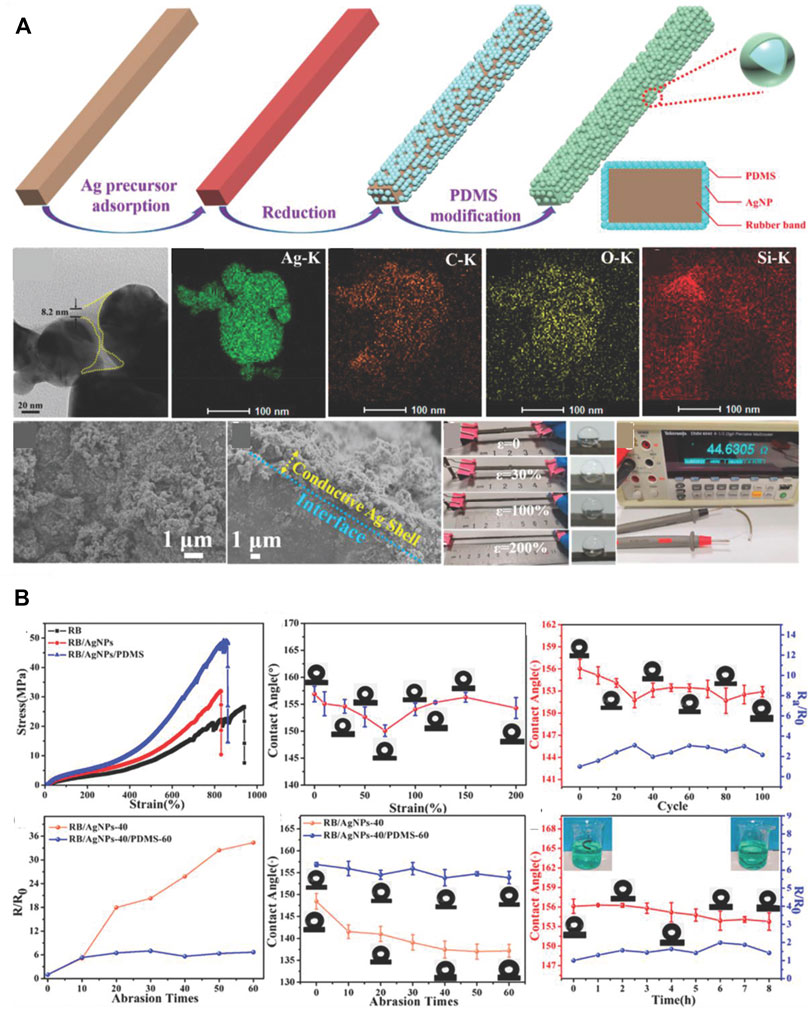
FIGURE 13. Preparation of superhydrophobic composites PDMS on the surface of AgNPs (A). Durable superhydrophobicity of the composites under cyclic stretching–releasing and abrasion tests (B). Reprinted with permission from Ref. (Wang et al., 2019c). Copyright 2019, American Chemical Society.
When nanoparticle aggregates are added on a PDMS surface (Figure 12C), the surface of the final superhydrophobic material consists of nanoparticles. Therefore, the nanoparticles must be hydrophobic to avoid conferring a hydrophilic or superhydrophilic character to the surface. Figure 14A shows an example in which hydrophilic SiO2 nanoparticles hydrophobized with PDMS are closely laid on a PDMS and carbonyl iron particle (CIP) microcilia array to form a superhydrophobic coating (Dai et al., 2021). The nanoparticle aggregates provide the necessary roughness to achieve superhydrophobicity (Figure 14B). For this type of superhydrophobic materials, the substrate may be a thick PDMS layer, which would expand the applications of PDMS-based superhydrophobic materials (Figure 14A).
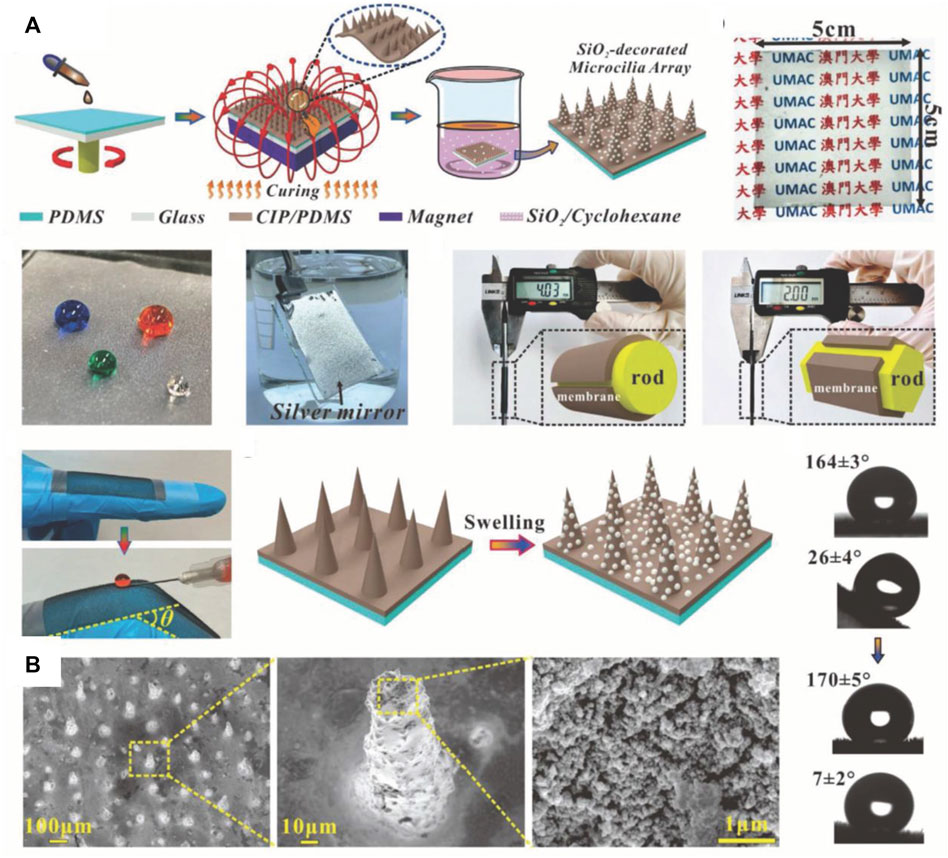
FIGURE 14. Preparation of superhydrophobic coatings by adding hydrophobized SiO2 nanoparticles on a PDMS and CIP microcilia array (A). SEM images of the surfaces (B). Reprinted with permission from Ref. (Dai et al., 2021). Copyright 2020, Wiley-VCH GmbH.
Conclusion and outlook
In this review, according to the mechanism of superhydrophobicity based on dual micro-scale and nano-scale structures, or hierarchical roughness, we propose a versatile “3M” methodology (materials, methods, and morphologies) that can be defined as “The use of specific materials and methods to construct special morphologies for surface superhydrophobicity” to guide the fabrication of superhydrophobic PDMS-materials for antifouling applications. Three types of PDMS-based materials were introduced: pure PDMS materials, materials consisting of PDMS and nanoparticles, and combinations of PDMS and other materials. Furthermore, the methods that can be chosen were discussed based on the different types of materials. Because materials made of PDMS and nanoparticles are advantageous, they were discussed to exemplify various morphologies and explain the “3M” methodology to obtain superhydrophobicity. Owing to the wide variety of potential morphologies of zero-, one-, two-, and three-dimensional nanoparticles, it is impossible to discuss them thoroughly in this review. Nonetheless, typical materials, methods, and morphologies were carefully selected and reviewed. Based on this “3M” methodology, in future research, people can design various novel morphologies, and obtain necessary dual micro-scale and nano-scale structures, or hierarchical roughness by adopting novel materials or methods. Therefore, numerous novel superhydrophobic materials will be explored. This paper is expected to serve as a helpful reference to future research on the fabrication of superhydrophobic materials based on PDMS and other polymers for antifouling applications. Moreover, superhydrophobic antifouling materials with multifunctions, such as optical, electrical, magnetic, thermo function, will have extensive applications in biomedical devices, lab-on-a-chip devices, sensors, etc. The challenges in future research should be focused on developing novel cheap and safe raw materials, versatile and covenitent fabrication methods, and designable but easily achieveable and stable enough morphologies.
Author contributions
ZH and XL conceived and designed this review paper; ZH and XL wrote this review paper; XY, LM, and NW revised this review paper.
Acknowledgments
The authors would like to acknowledge the financial support from the National Natural Science Foundation of China (No: 51873240), the Talent Introduction Program of The Affiliated Stomatological Hospital of Southwest Medical University (No: 2022BS02), the Talent Introduction Program of Chengdu University (2081920001), and Innovative leading talents program of The Affiliated Stomatological Hospital of Southwest Medical University (Host: X L).
Conflict of interest
The authors declare that the research was conducted in the absence of any commercial or financial relationships that could be construed as a potential conflict of interest.
Publisher’s note
All claims expressed in this article are solely those of the authors and do not necessarily represent those of their affiliated organizations, or those of the publisher, the editors and the reviewers. Any product that may be evaluated in this article, or claim that may be made by its manufacturer, is not guaranteed or endorsed by the publisher.
Supplementary material
The Supplementary Material for this article can be found online at: https://www.frontiersin.org/articles/10.3389/fbioe.2022.998852/full#supplementary-material
References
Ammons, M. C., and Copié, V. (2013). Mini-review: Lactoferrin: a bioinspired, anti-biofilm therapeutic. Biofouling 29 (4), 443–455. doi:10.1080/08927014.2013.773317
Artus, G. R. J., and Seeger, S. (2014). One-dimensional silicone nanofilaments. Advances in Colloid and Interface Science 209, 144–162. doi:10.1016/j.cis.2014.03.007
Aslanidou, D., Karapanagiotis, I., and Panayiotou, C. (2016). Tuning the wetting properties of siloxane-nanoparticle coatings to induce superhydrophobicity and superoleophobicity for stone protection. Materials & Design 108, 736–744. doi:10.1016/j.matdes.2016.07.014
Azemar, F., Faÿ, F., Réhel, K., and Linossier, I. (2015). Development of hybrid antifouling paints. Progress in Organic Coatings 87, 10–19. doi:10.1016/j.porgcoat.2015.04.007
Ball, P. (1999). Engineering Shark skin and other solutions. Nature 400 (6744), 507–509. doi:10.1038/22883
Barthwal, S., Barthwal, S., Singh, B., and Bahadur Singh, N. (2020). Multifunctional and fluorine-free superhydrophobic composite coating based on PDMS modified MWCNTs/ZnO with self-cleaning, oil-water separation, and flame retardant properties. Colloids and Surfaces A: Physicochemical and Engineering Aspects 597, 124776. doi:10.1016/j.colsurfa.2020.124776
Basu, S., Hanh, B. M., Isaiah Chua, J. Q., Daniel, D., Ismail, M. H., Marchioro, M., Amini, S., Rice, S. A., and Miserez, A. (2020). Green biolubricant infused slippery surfaces to combat marine biofouling. J. Colloid Interface Sci. 568, 185–197. doi:10.1016/j.jcis.2020.02.049
Bixler, G. D., and Bhushan, B. (2012). Biofouling: lessons from nature. Phil. Trans. R. Soc. A. 370 (1967), 2381–2417. doi:10.1098/rsta.2011.0502
Bixler, G. D., and Bhushan, B. (2013). Fluid drag reduction and efficient self-cleaning with rice leaf and butterfly wing bioinspired surfaces. Nanoscale 5 (17), 7685–710. doi:10.1039/c3nr01710a
Bixler, G. D., and Bhushan, B. (2014). Rice- and butterfly-wing effect inspired self-cleaning and low drag micro/nanopatterned surfaces in water, oil, and air flow. Nanoscale 6 (1), 76–96. doi:10.1039/c3nr04755e
Bixler, G. D., Theiss, A., Bhushan, B., and Lee, S. C. (2014). Anti-fouling properties of microstructured surfaces bio-inspired by rice leaves and butterfly wings. J. Colloid Interface Sci. 419, 114–33. doi:10.1016/j.jcis.2013.12.019
Callow, J. A., and Callow, M. E. (2011). Trends in the development of environmentally friendly fouling-resistant marine coatings. Nat. Commun. 2, 244. doi:10.1038/ncomms1251
Cao, X., Pan, J., Cai, G., Xiao, S., Ma, X., Zhang, X., and Dong, Z. (2022). A chemically robust and self-healing superhydrophobic polybenzoxazine coating without fluorocarbon resin modification: Fabrication and failure mechanism. Progress in Organic Coatings 163, 106630. doi:10.1016/j.porgcoat.2021.106630
Cao, X., Zhang, Y., Hu, W., Zheng, H., Dan, Y., Hu, J., and Chen, Z. (2021). Preparation of superhydrophobic nanoplate iron oxide surface on a carbon steel for anti-wetting applications. Materials & Design 211, 110169. doi:10.1016/j.matdes.2021.110169
Carl, C., Poole, A. J., Sexton, B. A., Glenn, F. L., Vucko, M. J., Williams, M. R., Whalan, S., and de Nys, R. (2012). Enhancing the settlement and attachment strength of pediveligers of Mytilus galloprovincialis bychanging surface wettability and microtopography. Biofouling 28 (2), 175–186. doi:10.1080/08927014.2012.662676
Celik, N., Sahin, F., Ruzi, M., Yay, M., Unal, E., and Onses, M. S. (2021). Blood repellent superhydrophobic surfaces constructed from nanoparticle-free and biocompatible materials. Colloids and Surfaces B: Biointerfaces 205, 111864. doi:10.1016/j.colsurfb.2021.111864
Chen, L., Guo, F., Yang, T., Hu, T., Bennett, P., Yang, Q., and Liu, D. (2021). Aging characteristics and self-healing properties of laser-textured superhydrophobic silicone rubber for composite insulators. Polymer Degradation and Stability 192, 109693. doi:10.1016/j.polymdegradstab.2021.109693
Chen, Q., Zhao, J., Ren, J., Rong, L., Cao, P. F., and Advincula, R. C. (2019). 3D Printed Multifunctional, Hyperelastic Silicone Rubber Foam. Adv. Funct. Mater. 29 (23), 1900469. doi:10.1002/adfm.201900469
Chen, R., Zhang, X., Su, Z., Gong, R., Ge, X., Zhang, H., and Wang, C. (2009). Perfectly Hydrophobic Silicone Nanofiber Coatings: Preparation from Methyltrialkoxysilanes and Use as Water-Collecting Substrate. J. Phys. Chem. C 113 (19), 8350–8356. doi:10.1021/jp8114622
Chen, Z., Su, X., Wu, W., Chen, S., Zhang, X., Wu, Y., Xie, H., and Li, K. (2022). Superhydrophobic PDMS@TiO2 wood for photocatalytic degradation and rapid oil-water separation. Surface and Coatings Technology 434, 128182. doi:10.1016/j.surfcoat.2022.128182
Cheng, H., Wang, F., Liu, H., Ou, J., Li, W., and Xue, R. (2022). Fabrication and Properties of Thermochromic Superhydrophobic Coatings. Adv. Eng. Mater. 24 (1), 2100647. doi:10.1002/adem.202100647
Cho, W. K., and Choi, I. S. (2008). Fabrication of hairy polymeric films inspired by geckos: Wetting and high adhesion properties. Adv. Funct. Mater. 18 (7), 1089–1096. doi:10.1002/adfm.200701454
Dai, S., Zhu, Y., Gu, Y., and Du, Z. (2019). Biomimetic fabrication and photoelectric properties of superhydrophobic ZnO nanostructures on flexible PDMS substrates replicated from rose petal. Appl. Phys. A 125 (2), 138. doi:10.1007/s00339-019-2438-7
Dai, Z., Chen, G., Ding, S., Lin, J., Li, S., Xu, Y., and Zhou, B. (2021). Facile Formation of Hierarchical Textures for Flexible, Translucent, and Durable Superhydrophobic Film. Adv. Funct. Mater. 31 (7), 2008574. doi:10.1002/adfm.202008574
Das, S., Kumar, S., Samal, S. K., Mohanty, S., and Nayak, S. K. (2018). A Review on Superhydrophobic Polymer Nanocoatings: Recent Development and Applications. Ind. Eng. Chem. Res. 57 (8), 2727–2745. doi:10.1021/acs.iecr.7b04887
Davis, A., Surdo, S., Caputo, G., Bayer, I. S., and Athanassiou, A. (2018). Environmentally Benign Production of Stretchable and Robust Superhydrophobic Silicone Monoliths. ACS Appl. Mater. Interfaces 10 (3), 2907–2917. doi:10.1021/acsami.7b15088
Děkanovský, L., Elashnikov, R., Kubiková, M., Vokatá, B., Švorčík, V., and Lyutakov, O. (2019). Dual‐Action Flexible Antimicrobial Material: Switchable Self‐Cleaning, Antifouling, and Smart Drug Release. Adv. Funct. Mater. 29 (31), 1901880. doi:10.1002/adfm.201901880
Dobretsov, S., Abed, R. M. M., and Teplitski, M. (2013). Mini-review: Inhibition of biofouling by marine microorganisms. Biofouling 29 (4), 423–441. doi:10.1080/08927014.2013.776042
Dobretsov, S., and Thomason, J. C. (2011). The development of marine biofilms on two commercial non-biocidal coatings: a comparison between silicone and fluoropolymer technologies. Biofouling 27 (8), 869–880. doi:10.1080/08927014.2011.607233
Elzaabalawy, A., Verberne, P., and Meguid, S. A. (2019). Multifunctional Silica-Silicone Nanocomposite with Regenerative Superhydrophobic Capabilities. ACS Appl. Mater. Interfaces 11 (45), 42827–42837. doi:10.1021/acsami.9b15445
Gao, N., and Yan, Y. (2012). Characterisation of surface wettability based on nanoparticles. Nanoscale 4 (7), 2202–18. doi:10.1039/c2nr11736c
Garcia, N., Benito, E., Tiemblo, P., Hasan, M. M. B., Synytska, A., and Stamm, M. (2010). Chemically guided topography in alkylsilane- and oligosiloxane-modified silica nanoparticle coatings: from very hydrophobic surfaces to "pearl" bouncing droplets. Soft Matter 6 (19), 4768–4776. doi:10.1039/c0sm00119h
Gaw, S. L., Sarkar, S., Nir, S., Schnell, Y., Mandler, D., Xu, Z. J., Lee, P. S., and Reches, M. (2017). Electrochemical Approach for Effective Antifouling and Antimicrobial Surfaces. ACS Appl. Mater. Interfaces 9 (31), 26503–26509. doi:10.1021/acsami.7b03761
Gu, H., Li, G., Li, P., Liu, H., Chadyagondo, T. T., Li, N., and Xiong, J. (2020). Superhydrophobic and breathable SiO2/polyurethane porous membrane for durable water repellent application and oil-water separation. Applied Surface Science 2020, 144837. doi:10.1016/j.apsusc.2019.144837
Han, M., Silva, S. M., Lei, W., Quigley, A., Kapsa, R. M. I., Moulton, S. E., and Greene, G. W. (2019). Adhesion and Self-Assembly of Lubricin (PRG4) Brush Layers on Different Substrate Surfaces. Langmuir 35 (48), 15834–15848. doi:10.1021/acs.langmuir.9b01809
Han, X., and Gong, X. (2021). In situIn Situ, One-Pot Method to Prepare Robust Superamphiphobic Cotton Fabrics for High Buoyancy and Good Antifouling. ACS Appl. Mater. Interfaces 13 (26), 31298–31309. doi:10.1021/acsami.1c08844
He, Z., Chen, Y., Yang, J., Tang, C., Lv, J., Liu, Y., Mei, J., Lau, W.-M., and Hui, D. (2017). Fabrication of Polydimethylsiloxane films with special surface wettability by 3D printing. Composites Part B: Engineering 129, 58–65. doi:10.1016/j.compositesb.2017.07.025
He, Z., Lan, X., Chen, F., Wang, K., Deng, H., Zhang, Q., and Fu, Q. (2013). Effect of surface wettability on transparency in different water conditions. J. Coat. Technol. Res. 10 (5), 641–647. doi:10.1007/s11998-013-9490-6
He, Z., Lan, X., Hu, Q., Li, H., Li, L., and Mao, J. (2021). Antifouling strategies based on super-phobic polymer materials. Progress in Organic Coatings 157, 106285. doi:10.1016/j.porgcoat.2021.106285
He, Z., Ma, M., Lan, X., Chen, F., Wang, K., Deng, H., Zhang, Q., and Fu, Q. (2011). Fabrication of a transparent superamphiphobic coating with improved stability. Soft Matter 7 (14), 6435–6443. doi:10.1039/c1sm05574g
He, Z., Ma, M., Xu, X., Wang, J., Chen, F., Deng, H., Wang, K., Zhang, Q., and Fu, Q. (2012). Fabrication of superhydrophobic coating via a facile and versatile method based on nanoparticle aggregates. Applied Surface Science 258 (7), 2544–2550. doi:10.1016/j.apsusc.2011.10.090
He, Z., Yang, X., Wang, N., Mu, L., Pan, J., Lan, X., Li, H., and Deng, F. (2021). Anti-Biofouling Polymers with Special Surface Wettability for Biomedical Applications. Front. Bioeng. Biotechnol. 9 (1260), 807357. doi:10.3389/fbioe.2021.807357
Ho, T. T., Zimmermann, T., Ohr, S., and Caseri, W. R. (2012). Composites of cationic nanofibrillated cellulose and layered silicates: water vapor barrier and mechanical properties. ACS Appl. Mater. Interfaces 4 (9), 4832–40. doi:10.1021/am3011737
Hu, P., Xie, Q., Ma, C., and Zhang, G. (2020). Silicone-Based Fouling-Release Coatings for Marine Antifouling. Langmuir 36 (9), 2170–2183. doi:10.1021/acs.langmuir.9b03926
Jiang, T., Guo, Z., and Liu, W. (2015). Biomimetic superoleophobic surfaces: focusing on their fabrication and applications. J. Mater. Chem. A Mater. 3 (5), 1811–1827. doi:10.1039/C4TA05582A
Jorge, P., Lourenço, A., and Pereira, M. O. (2012). New trends in peptide-based anti-biofilm strategies: a review of recent achievements and bioinformatic approaches. Biofouling 28 (10), 1033–1061. doi:10.1080/08927014.2012.728210
Ju, J., Yao, X., Hou, X., Liu, Q., Zhang, Y. S., and Khademhosseini, A. (2017). A Highly Stretchable and Robust Non-fluorinated Superhydrophobic Surface. J. Mater. Chem. A Mater. 5 (31), 16273–16280. doi:10.1039/C6TA11133E
Kamelian, F. S., Mohammadi, T., and Naeimpoor, F. (2019). Fast, facile and scalable fabrication of novel microporous silicalite-1/PDMS mixed matrix membranes for efficient ethanol separation by pervaporation. Separation and Purification Technology 229, 115820. doi:10.1016/j.seppur.2019.115820
Kancı Bozoğlan, B., Duman, O., and Tunç, S. (2021). Smart antifungal thermosensitive chitosan/carboxymethylcellulose/scleroglucan/montmorillonite nanocomposite hydrogels for onychomycosis treatment. Colloids and Surfaces A: Physicochemical and Engineering Aspects 610, 125600. doi:10.1016/j.colsurfa.2020.125600
Kuang, M., Wang, J., and Jiang, L. (2016). Bio-inspired photonic crystals with superwettability. Chem. Soc. Rev. 45 (24), 6833–6854. doi:10.1039/c6cs00562d
Lan, X., Lei, Y., He, Z., Yin, A., Li, L., Tang, Z., Li, M., and Wang, Y. (2021). A transparent hydrophilic anti-biofouling coating for intraocular lens materials prepared by "bridging" of the intermediate adhesive layer. J. Mater. Chem. B 9 (17), 3696–3704. doi:10.1039/d1tb00065a
Lee, J.-W., Kim, K., Ryoo, G., Kim, J., Vinoth Kumar, J., and Hwang, W. (2022). Super-hydrophobic/hydrophilic patterning on three-dimensional objects. Applied Surface Science 576, 151849. doi:10.1016/j.apsusc.2021.151849
Lei, Y., Lan, X., He, Z., Yin, A., Jin, W., Hu, Q., and Wang, Y. (2021). Multifarious anti-biofouling bioprosthetic heart valve materials with the formation of interpenetrating polymer network structures. Materials & Design 206, 109803. doi:10.1016/j.matdes.2021.109803
Lejars, M., Margaillan, A., and Bressy, C. (2012). Fouling Release Coatings: A Nontoxic Alternative to Biocidal Antifouling Coatings. Chem. Rev. 112 (8), 4347–4390. doi:10.1021/cr200350v
Leslie, D. C., Waterhouse, A., Berthet, J. B., Valentin, T. M., Watters, A. L., Jain, A., Kim, P., Hatton, B. D., Nedder, A., Donovan, K., Super, E. H., Howell, C., Johnson, C. P., Vu, T. L., Bolgen, D. E., Rifai, S., Hansen, A. R., Aizenberg, M., Super, M., Aizenberg, J., and Ingber, D. E. (2014). A bioinspired omniphobic surface coating on medical devices prevents thrombosis and biofouling. Nat. Biotechnol. 32, 1134–1140. Available at: https://www.nature.com/articles/nbt.3020#supplementary-information. doi:10.1038/nbt.3020
Li, D., Fan, Y., Han, G., and Guo, Z. (2020). Superomniphobic Silk Fibroin/Ag Nanowires Membrane for Flexible and Transparent Electronic Sensor. ACS Appl. Mater. Interfaces 12 (8), 10039–10049. doi:10.1021/acsami.9b23378
Li, K., Zeng, X., Li, H., Lai, X., Ye, C., and Xie, H. (2013). Study on the wetting behavior and theoretical models of polydimethylsiloxane/silica coating. Applied Surface Science 279, 458–463. doi:10.1016/j.apsusc.2013.04.137
Li, Q., Zhao, X., Li, L., Hu, T., Yang, Y., and Zhang, J. (2021). Facile preparation of polydimethylsiloxane/carbon nanotubes modified melamine solar evaporators for efficient steam generation and desalination. Journal of Colloid and Interface Science 584, 602–609. doi:10.1016/j.jcis.2020.10.002
Li, R., Yang, L., Zhou, C., Zhang, G., Tian, J., and Wu, W. (2022). Fast self-healing superhydrophobic sponge with all-weather heating and anti-fouling properties. Materials Today Chemistry 23, 100730. doi:10.1016/j.mtchem.2021.100730
Li, S., Xu, R., Wang, J., Yang, Y., Fu, Q., and Pan, C. (2022). Ultra-stretchable, super-hydrophobic and high-conductive composite for wearable strain sensors with high sensitivity. Journal of Colloid and Interface Science 617, 372–382. doi:10.1016/j.jcis.2022.03.020
Li, X., Yan, J., Yu, T., and Zhang, B. (2022). Versatile nonfluorinated superhydrophobic coating with self-cleaning, anti-fouling, anti-corrosion and mechanical stability. Colloids and Surfaces A: Physicochemical and Engineering Aspects 642, 128701. doi:10.1016/j.colsurfa.2022.128701
Li, Z., and Guo, Z. (2020). Flexible 3D porous superhydrophobic composites for oil-water separation and organic solvent detection. Materials & Design 196, 109144. doi:10.1016/j.matdes.2020.109144
Liang, Y., Kim, S., Yang, E., and Choi, H. (2020). Omni-Directional Protected Nanofiber Membranes by Surface Segregation of PDMS-Terminated Triblock Copolymer for High-Efficiency Oil/Water Emulsion Separation. ACS Appl. Mater. Interfaces 12 (22), 25324–25333. doi:10.1021/acsami.0c05559
Liu, B., He, Y., Fan, Y., and Wang, X. (2006). Fabricating Super-Hydrophobic Lotus-Leaf-Like Surfaces through Soft-Lithographic Imprinting. Macromol. Rapid Commun. 27 (21), 1859–1864. doi:10.1002/marc.200600492
Liu, C., Ma, C., Xie, Q., and Zhang, G. (2017). Self-repairing silicone coatings for marine anti-biofouling. J. Mater. Chem. A Mater. 5 (30), 15855–15861. doi:10.1039/C7TA05241C
Liu, H.-D., Gu, B., Yuan, W.-F., and He, Q. (2018). Fabrication of a superhydrophobic polyurethane foam and its application for continuous oil removal. Mater. Res. Express 5 (2), 025005. doi:10.1088/2053-1591/aaa995
Liu, J., Yao, Y., Li, X., and Zhang, Z. (2021). Fabrication of advanced polydimethylsiloxane-based functional materials: Bulk modifications and surface functionalizations. Chemical Engineering Journal 408, 127262. doi:10.1016/j.cej.2020.127262
Liu, J., Ye, L., Sun, Y., Hu, M., Chen, F., Wegner, S., Mailander, V., Steffen, W., Kappl, M., and Butt, H. J. (2020). Elastic Superhydrophobic and Photocatalytic Active Films Used as Blood Repellent Dressing. Adv. Mater. 32 (11), e1908008. doi:10.1002/adma.201908008
Liu, S., Zheng, J., Hao, L., Yegin, Y., Bae, M., Ulugun, B., Taylor, T. M., Scholar, E. A., Cisneros-Zevallos, L., Oh, J. K., and Akbulut, M. (2020). Dual-Functional, Superhydrophobic Coatings with Bacterial Anticontact and Antimicrobial Characteristics. ACS Appl. Mater. Interfaces 12 (19), 21311–21321. doi:10.1021/acsami.9b18928
Liu, Y., Gu, H., Jia, Y., Liu, J., Zhang, H., Wang, R., Zhang, B., Zhang, H., and Zhang, Q. (2019). Design and Preparation of Biomimetic Polydimethylsiloxane (PDMS) Films with Superhydrophobic, Self-Healing and Drag Reduction Properties via Replication of Shark Skin and SI-ATRP. Chem. Eng. J. 356, 318–328. doi:10.1016/j.cej.2018.09.022
Liu, Y., Tan, T., Wang, B., Zhai, R., Song, X., Li, E., Wang, H., and Yan, H. (2008). Fabrication of CdS films with superhydrophobicity by the microwave assisted chemical bath deposition. Journal of Colloid and Interface Science 320 (2), 540–547. doi:10.1016/j.jcis.2007.10.066
Lu, N., Hu, Z., Wang, F., Yan, L., Sun, H., Zhu, Z., Liang, W., and Li, A. (2021). Superwetting Electrospun PDMS/PMMA Membrane for PM2.5 Capture and Microdroplet Transfer. Langmuir 37 (44), 12972–12980. doi:10.1021/acs.langmuir.1c02038
Lu, Z., Xu, L., He, Y., and Zhou, J. (2019). One-step facile route to fabricate functionalized nano-silica and silicone sealant based transparent superhydrophobic coating. Thin Solid Films 692, 137560. doi:10.1016/j.tsf.2019.137560
Luo, Q., Peng, J., Chen, X., Zhang, H., Deng, X., Jin, S., and Zhu, H. (2022). Recent Advances in Multifunctional Mechanical–Chemical Superhydrophobic Materials. Front. Bioeng. Biotechnol. 10, 947327. doi:10.3389/fbioe.2022.947327
Ma, S., Ye, Q., Pei, X., Wang, D., and Zhou, F. (2015). Antifouling on Gecko's Feet Inspired Fibrillar Surfaces: Evolving from Land to Marine and from Liquid Repellency to Algae Resistance. Adv. Mater. Interfaces 2 (13), 1500257. doi:10.1002/admi.201500257
Maan, A. M. C., Hofman, A. H., de Vos, W. M., and Kamperman, M. (2020). Recent Developments and Practical Feasibility of Polymer-Based Antifouling Coatings. Adv. Funct. Mater. 30 (32), 2000936. doi:10.1002/adfm.202000936
Manolakis, I., and Azhar, U. (2020). Recent Advances in Mussel-Inspired Synthetic Polymers as Marine Antifouling Coatings. Coatings 10 (7), 653. doi:10.3390/coatings10070653
Martin, S., Brown, P. S., and Bhushan, B. (2017). Fabrication techniques for bioinspired, mechanically-durable, superliquiphobic surfaces for water, oil, and surfactant repellency. Advances in Colloid and Interface Science 241, 1–23. doi:10.1016/j.cis.2017.01.004
Mazaltarim, A. J., Torres, A., and Morin, S. A. (2021). Mechanically Tunable Superhydrophobic Surfaces Enabled by the Rational Manipulation of Microcrack Networks in Nanoporous Films. Adv. Mater. Interfaces 8 (17), 2100869. doi:10.1002/admi.202100869
Miao, S., Xiong, Z., Zhang, J., Wu, Y., and Gong, X. (2022). Polydopamine/SiO2 Hybrid Structured Superamphiphobic Fabrics with Good Photothermal Behavior. Langmuir 38, 9431–9440. doi:10.1021/acs.langmuir.2c01629
Mieszkin, S., Callow, M. E., and Callow, J. A. (2013). Interactions between microbial biofilms and marine fouling algae: a mini review. Biofouling 29 (9), 1097–1113. doi:10.1080/08927014.2013.828712
Miyamoto, T., Yamazaki, N., Watanabe, S., and Yamada, S. (2019). Aqueous Lubrication with the Molecularly Confined Films of Silicone-Based Amphiphilic Block Copolymer Aggregates. Langmuir 35 (48), 15784–15794. doi:10.1021/acs.langmuir.9b03212
Nine, M. J., Cole, M. A., Johnson, L., Tran, D. N. H., and Losic, D. (2015). Robust Superhydrophobic Graphene-Based Composite Coatings with Self-Cleaning and Corrosion Barrier Properties. ACS Appl. Mater. Interfaces 7 (51), 28482–28493. doi:10.1021/acsami.5b09611
Obotey Ezugbe, E., and Rathilal, S. (2020). Membrane Technologies in Wastewater Treatment: A Review. Membranes (Basel) 10 (5), 89. doi:10.3390/membranes10050089
Pakdel, E., Xie, W., Wang, J., Kashi, S., Sharp, J., Zhang, Q., Varley, R. J., Sun, L., and Wang, X. (2022). Superhydrophobic natural melanin-coated cotton with excellent UV protection and personal thermal management functionality. Chemical Engineering Journal 433, 133688. doi:10.1016/j.cej.2021.133688
Pakzad, H., Liravi, M., Moosavi, A., Nouri-Borujerdi, A., and Najafkhani, H. (2020). Fabrication of durable superhydrophobic surfaces using PDMS and beeswax for drag reduction of internal turbulent flow. Applied Surface Science 513, 145754. doi:10.1016/j.apsusc.2020.145754
Pan, S., Guo, R., Richardson, J. J., Berry, J. D., Besford, Q. A., Bjornmalm, M., Yun, G., Wu, R., Lin, Z., Zhong, Q. Z., Zhou, J., Sun, Q., Li, J., Lu, Y., Dong, Z., Banks, M. K., Xu, W., Jiang, J., Jiang, L., and Caruso, F. (2019). Ricocheting Droplets Moving on Super-Repellent Surfaces. Adv. Sci. (Weinh). 6 (21), 1901846. doi:10.1002/advs.201901846
Park, S., Song, S., and Yoon, S.-H. (2022). Ultrasonication-induced and diluent-assisted suspension polymerization for size-controllable synthesis of polydimethylsiloxane droplets. Colloids and Surfaces A: Physicochemical and Engineering Aspects 644, 128827. doi:10.1016/j.colsurfa.2022.128827
Park, Y.-B., Im, H., Im, M., and Choi, Y.-K. (2011). Self-cleaning effect of highly water-repellent microshell structures for solar cell applications. J. Mater. Chem. 21, 633–636. doi:10.1039/c0jm02463e
Peng, J., Tomsia, A. P., Jiang, L., Tang, B. Z., and Cheng, Q. (2021). Stiff and tough PDMS-MMT layered nanocomposites visualized by AIE luminogens. Nat. Commun. 12 (1), 4539. doi:10.1038/s41467-021-24835-w
Qi, D., Zhang, K., Tian, G., Jiang, B., and Huang, Y. (2021). Stretchable Electronics Based on PDMS Substrates. Adv. Mater. 33 (6), 2003155. doi:10.1002/adma.202003155
Qing, Y., Long, C., An, K., Hu, C., and Liu, C. (2019). Sandpaper as template for a robust superhydrophobic surface with self-cleaning and anti-snow/icing performances. J. Colloid Interface Sci. 548, 224–232. doi:10.1016/j.jcis.2019.04.040
Rin Yu, C., Shanmugasundaram, A., and Lee, D.-W. (2022). Nanosilica coated polydimethylsiloxane mushroom structure: A next generation flexible, transparent, and mechanically durable superhydrophobic thin film. Applied Surface Science 583, 152500. doi:10.1016/j.apsusc.2022.152500
Roach, P., Shirtcliffe, N. J., and Newton, M. I. (2008). Progess in superhydrophobic surface development. Soft Matter 4 (2), 224–240. doi:10.1039/b712575p
Ruan, M., Zhan, Y., Wu, Y., Wang, X., Li, W., Chen, Y., Wei, M., Wang, X., and Deng, X. (2017). Preparation of PTFE/PDMS Superhydrophobic Coating and Its Anti-Icing Performance. RSC Adv. 7, 41339–41344. doi:10.1039/c7ra05264b
Saadatbakhsh, M., Jamali Asl, S., Kiani, M. J., and Nouri, N. M. (2020). Slip length measurement of pdms/hydrophobic silica superhydrophobic coating for drag reduction application. Surface and Coatings Technology 404, 126428. doi:10.1016/j.surfcoat.2020.126428
Saharudin, K. A., Karim, M. A., and Sreekantan, S. (2019). Preparation of a Polydimethylsiloxane (PDMS)/Graphene-based Super-hydrophobic Coating. Materials Today: Proceedings 17, 752–760. doi:10.1016/j.matpr.2019.06.359
Saharudin, K. A., Sreekantan, S., Basiron, N., Chun, L. K., Kumaravel, V., Abdullah, T. K., and Ahmad, Z. A. (2018). Improved super-hydrophobicity of eco-friendly coating from palm oil fuel ash (POFA) waste. Surface and Coatings Technology 337, 126–135. doi:10.1016/j.surfcoat.2017.11.070
Scardino, A. J., and de Nys, R. (2011). Mini review: Biomimetic models and bioinspired surfaces for fouling control. Biofouling 27 (1), 73–86. doi:10.1080/08927014.2010.536837
Schultz, C. W., Ng, C. L. W., and Yu, H. Z. (2020). Superhydrophobic Polydimethylsiloxane via Nanocontact Molding of Solvent Crystallized Polycarbonate: Optimized Fabrication, Mechanistic Investigation, and Application Potential. ACS Appl. Mater. Interfaces 12 (2), 3161–3170. doi:10.1021/acsami.9b18041
Selim, M. S., Azzam, A. M., Higazy, S. A., El-Safty, S. A., and Shenashen, M. A. (2022). Novel graphene-based ternary nanocomposite coatings as ecofriendly antifouling brush surfaces. Progress in Organic Coatings 167, 106803. doi:10.1016/j.porgcoat.2022.106803
Selim, M. S., Elmarakbi, A., Azzam, A. M., Shenashen, M. A., El-Saeed, A. M., and El-Safty, S. A. (2018). Eco-friendly design of superhydrophobic nano-magnetite/silicone composites for marine foul-release paints. Progress in Organic Coatings 116, 21–34. doi:10.1016/j.porgcoat.2017.12.008
Selim, M. S., Fatthallah, N. A., Higazy, S. A., Hao, Z., and Jing Mo, P. (2022). A comparative study between two novel silicone/graphene-based nanostructured surfaces for maritime antifouling. Journal of Colloid and Interface Science 606, 367–383. doi:10.1016/j.jcis.2021.08.026
Selim, M. S., Yang, H., Wang, F. Q., Fatthallah, N. A., Huang, Y., and Kuga, S. (2019). Silicone/ZnO nanorod composite coating as a marine antifouling surface. Applied Surface Science 466, 40–50. doi:10.1016/j.apsusc.2018.10.004
Selim, M. S., Yang, H., Wang, F. Q., Li, X., Huang, Y., and Fatthallah, N. A. (2018). Silicone/Ag@SiO2 core–shell nanocomposite as a self-cleaning antifouling coating material. RSC Adv. 8 (18), 9910–9921. doi:10.1039/C8RA00351C
Seo, K., Kim, M., Seok, S., and Kim, D. H. (2016). Transparent superhydrophobic surface by silicone oil combustion. Colloids and Surfaces A: Physicochemical and Engineering Aspects 492, 110–118. doi:10.1016/j.colsurfa.2015.12.022
Shen, L., Wang, W., Ding, H., and Guo, Q. (2013). Flame soot stably deposited on silicone coatings possess superhydrophobic surface. Applied Surface Science 284, 651–656. doi:10.1016/j.apsusc.2013.07.149
Shi, X., Dou, R., Ma, T., Liu, W., Lu, X., Shea, K. J., Song, Y., and Jiang, L. (2015). Bioinspired Lotus-like Self-Illuminous Coating. ACS Appl. Mater. Interfaces 7 (33), 18424–18428. doi:10.1021/acsami.5b04499
Siddiqui, A. R., Li, W., Wang, F., Ou, J., and Amirfazli, A. (2021). One-step fabrication of transparent superhydrophobic surface. Applied Surface Science 542, 148534. doi:10.1016/j.apsusc.2020.148534
Siddiquie, R. Y., Gaddam, A., Agrawal, A., Dimov, S. S., and Joshi, S. S. (2020). Anti-Biofouling Properties of Femtosecond Laser-Induced Submicron Topographies on Elastomeric Surfaces. Langmuir 36 (19), 5349–5358. doi:10.1021/acs.langmuir.0c00753
Simovich, T., Rosenhahn, A., and Lamb, R. N. (2020). Thermoregeneration of Plastrons on Superhydrophobic Coatings for Sustained Antifouling Properties. Adv. Eng. Mater. 22 (3), 1900806. doi:10.1002/adem.201900806
Song, E. H., Kang, B. H., Kim, T. Y., Lee, H. J., Park, Y. W., Kim, Y. C., and Ju, B. K. (2015). Highly oriented gold/nanoclay-polymer nanocomposites for flexible gas barrier films. ACS Appl. Mater. Interfaces 7 (8), 4778–83. doi:10.1021/am508641g
Steele, A., Bayer, I., and Loth, E. (2009). Inherently Superoleophobic Nanocomposite Coatings by Spray Atomization. Nano Lett. 9 (1), 501–505. doi:10.1021/nl8037272
Su, X., Li, H., Lai, X., Zhang, L., Liao, X., Wang, J., Chen, Z., He, J., and Zeng, X. (2018). Dual-Functional Superhydrophobic Textiles with Asymmetric Roll-Down/Pinned States for Water Droplet Transportation and Oil-Water Separation. ACS Appl. Mater. Interfaces 10 (4), 4213–4221. doi:10.1021/acsami.7b15909
Su, X., Li, H., Lai, X., Zhang, L., Wang, J., Liao, X., and Zeng, X. (2017). Vapor–Liquid Sol–Gel Approach to Fabricating Highly Durable and Robust Superhydrophobic Polydimethylsiloxane@Silica Surface on Polyester Textile for Oil–Water Separation. ACS Appl. Mater. Interfaces 9 (33), 28089–28099. doi:10.1021/acsami.7b08920
Tian, Y., Su, B., and Jiang, L. (2014). Interfacial material system exhibiting superwettability. Adv. Mater. 26 (40), 6872–97. doi:10.1002/adma.201400883
Torun, I., Ruzi, M., Er, F., and Onses, M. S. (2019). Superhydrophobic coatings made from biocompatible polydimethylsiloxane and natural wax. Progress in Organic Coatings 136, 105279. doi:10.1016/j.porgcoat.2019.105279
Wang, C.-F., Chen, W.-Y., Cheng, H.-Z., and Fu, S.-L. (2010). Pressure-Proof Superhydrophobic Films from Flexible Carbon Nanotube/Polymer Coatings. J. Phys. Chem. C 114 (37), 15607–15611. doi:10.1021/jp1047985
Wang, D., Guo, Z., Chen, Y., Hao, J., and Liu, W. (2007). In situIn Situ Hydrothermal Synthesis of Nanolamellate CaTiO3 with Controllable Structures and Wettability. Inorg. Chem. 46 (19), 7707–7709. doi:10.1021/ic700777f
Wang, F., Qiu, L., and Tian, Y. (2021). Super Anti-Wetting Colorimetric Starch-Based Film Modified with Poly(dimethylsiloxane) and Micro-/Nano-Starch for Aquatic-Product Freshness Monitoring. Biomacromolecules 22 (9), 3769–3779. doi:10.1021/acs.biomac.1c00588
Wang, F., Tay, T. E., Sun, Y., Liang, W., and Yang, B. (2019). Low-voltage and -surface energy SWCNT/poly(dimethylsiloxane) (PDMS) nanocomposite film: Surface wettability for passive anti-icing and surface-skin heating for active deicing. Composites Science and Technology 184, 107872. doi:10.1016/j.compscitech.2019.107872
Wang, G., Li, A., Zhao, W., Xu, Z., Ma, Y., Zhang, F., Zhang, Y., Zhou, J., and He, Q. (2021). A Review on Fabrication Methods and Research Progress of Superhydrophobic Silicone Rubber Materials. Adv. Mater. Interfaces 8 (1), 2001460. doi:10.1002/admi.202001460
Wang, H. X., Fang, J., Cheng, T., Ding, J., Qu, L. T., Dai, L. M., Wang, X. G., and Lin, T. (2008). One-step coating of fluoro-containing silica nanoparticles for universal generation of surface superhydrophobicity. Chem. Commun. (7), 877–879. doi:10.1039/b714352d
Wang, L., Luo, J., Chen, Y., Lin, L., Huang, X., Xue, H., and Gao, J. (2019). Fluorine-free Superhydrophobic and Conductive Rubber Composite with Outstanding Deicing Performance for Highly Sensitive and Stretchable Strain Sensors. ACS Appl. Mater. Interfaces 11 (19), 17774–17783. doi:10.1021/acsami.9b03545
Wang, Q., Sun, G., Tong, Q., Yang, W., and Hao, W. (2021). Fluorine-free superhydrophobic coatings from polydimethylsiloxane for sustainable chemical engineering: Preparation methods and applications. Chemical Engineering Journal 426, 130829. doi:10.1016/j.cej.2021.130829
Wang, S., and Jiang, L. (2007). Definition of superhydrophobic states. Adv. Mater. 19 (21), 3423–3424. doi:10.1002/adma.200700934
Wang, X., Dai, L., Jiao, N., Tung, S., and Liu, L. (2021). Superhydrophobic photothermal graphene composites and their functional applications in microrobots swimming at the air/water interface. Chemical Engineering Journal 422, 129394. doi:10.1016/j.cej.2021.129394
Wang, X., Jiao, N., Tung, S., and Liu, L. (2019). Photoresponsive Graphene Composite Bilayer Actuator for Soft Robots. ACS Appl. Mater. Interfaces 11 (33), 30290–30299. doi:10.1021/acsami.9b09491
Wang, Z., Shen, X., Yan, Y., Qian, T., Wang, J., Sun, Q., and Jin, C. (2018). Facile fabrication of a PDMS @ stearic acid-Al(OH)3 coating on lignocellulose composite with superhydrophobicity and flame retardancy. Applied Surface Science 450, 387–395. doi:10.1016/j.apsusc.2018.04.220
Wu, D., Wang, J.-N., Wu, S.-Z., Chen, Q.-D., Zhao, S., Zhang, H., Sun, H.-B., and Jiang, L. (2011). Three-Level Biomimetic Rice-Leaf Surfaces with Controllable Anisotropic Sliding. Adv. Funct. Mater. 21 (15), 2927–2932. doi:10.1002/adfm.201002733
Wu, H., Luo, J., Huang, X., Wang, L., Guo, Z., Liang, J., Zhang, S., Xue, H., and Gao, J. (2021). Superhydrophobic, mechanically durable coatings for controllable light and magnetism driven actuators. Journal of Colloid and Interface Science 603, 282–290. doi:10.1016/j.jcis.2021.06.106
Wu, Y., Zeng, J., Si, Y., Chen, M., and Wu, L. (2018). Large-Area Preparation of Robust and Transparent Superomniphobic Polymer Films. ACS Nano 12 (10), 10338–10346. doi:10.1021/acsnano.8b05600
Xia, F., and Jiang, L. (2008). Bio-inspired, smart, multiscale interfacial materials. Adv. Mater. 20 (15), 2842–2858. doi:10.1002/adma.200800836
Xiong, Z., Huang, J., Wu, Y., and Gong, X. (2022). Robust multifunctional fluorine-free superhydrophobic fabrics for high-efficiency oil–water separation with ultrahigh flux. Nanoscale 14 (15), 5840–5850. doi:10.1039/D2NR00337F
Xiong, Z., Yu, H., and Gong, X. (2022). Designing Photothermal Superhydrophobic PET Fabrics via in situIS Polymerization and 1, 4-Conjugation Addition Reaction. Langmuir 38 (28), 8708–8718. doi:10.1021/acs.langmuir.2c01366
Xu, Q. F., Wang, J. N., and Sanderson, K. D. (2010). Organic−Inorganic Composite Nanocoatings with Superhydrophobicity, Good Transparency, and Thermal Stability. Acs Nano 4 (4), 2201–2209. doi:10.1021/nn901581j
Yamauchi, Y., Tenjimbayashi, M., Samitsu, S., and Naito, M. (2019). Durable and Flexible Superhydrophobic Materials: Abrasion/Scratching/Slicing/Droplet Impacting/Bending/Twisting-Tolerant Composite with Porcupinefish-Like Structure. ACS Appl. Mater. Interfaces 11 (35), 32381–32389. doi:10.1021/acsami.9b09524
Yang, J., Pi, P., Wen, X., Zheng, D., Xu, M., Cheng, J., and Yang, Z. (2009). A novel method to fabricate superhydrophobic surfaces based on well-defined mulberry-like particles and self-assembly of polydimethylsiloxane. Applied Surface Science 255 (6), 3507–3512. doi:10.1016/j.apsusc.2008.09.092
Yong, J., Chen, F., Fang, Y., Huo, J., Yang, Q., Zhang, J., Bian, H., and Hou, X. (2017). Bioinspired Design of Underwater Superaerophobic and Superaerophilic Surfaces by Femtosecond Laser Ablation for Anti- or Capturing Bubbles. ACS Appl. Mater. Interfaces 9 (45), 39863–39871. doi:10.1021/acsami.7b14819
Yong, J., Chen, F., Yang, Q., Zhang, D., Du, G., Si, J., Yun, F., and Hou, X. (2013). Femtosecond Laser Weaving Superhydrophobic Patterned PDMS Surfaces with Tunable Adhesion. J. Phys. Chem. C 117 (47), 24907–24912. doi:10.1021/jp408863u
Young, T. (1805). An Essay on the Cohesion of Fluids. Philosophical Transactions of the Royal Society of London 95 (0), 65–87. doi:10.1098/rstl.1805.0005
Yu, C., Yu, C., Cui, L., Song, Z., Zhao, X., Ma, Y., and Jiang, L. (2017). Facile Preparation of the Porous PDMS Oil-Absorbent for Oil/Water Separation. Adv. Mater. Interfaces 4 (3), 1600862. doi:10.1002/admi.201600862
Yu, H., Wu, M., Duan, G., and Gong, X. (2022). One-step fabrication of eco-friendly superhydrophobic fabrics for high-efficiency oil/water separation and oil spill cleanup. Nanoscale 14 (4), 1296–1309. doi:10.1039/D1NR07111D
Yu, S., Guo, Z., and Liu, W. (2015). Biomimetic transparent and superhydrophobic coatings: from nature and beyond nature. Chem. Commun. 51 (10), 1775–1794. doi:10.1039/C4CC06868H
Yu, Y., Shao, H., He, Z., Tang, C., Yang, J., Li, Y., Wang, C., Li, X., Shuai, M., and Mei, J. (2018). Patternable Poly(chloro-p-xylylene) Film with Tunable Surface Wettability Prepared by Temperature and Humidity Treatment on a Polydimethylsiloxane/Silica Coating. Materials 11 (4), 486. doi:10.3390/ma11040486
Zaman, Q., Zia, K. M., Zuber, M., Mabkhot, Y. N., Almalki, F., and Hadda, T. B. (2019). A comprehensive review on synthesis, characterization, and applications of polydimethylsiloxane and copolymers. Int. J. Plast. Technol. 23 (2), 261–282. doi:10.1007/s12588-019-09259-y
Zarghami, S., Mohammadi, T., Sadrzadeh, M., and Van der Bruggen, B. (2019). Superhydrophilic and underwater superoleophobic membranes - A review of synthesis methods. Progress in Polymer Science 98, 101166. doi:10.1016/j.progpolymsci.2019.101166
Zhai, W., Xia, Q., Zhou, K., Yue, X., Ren, M., Zheng, G., Dai, K., Liu, C., and Shen, C. (2019). Multifunctional flexible carbon black/polydimethylsiloxane piezoresistive sensor with ultrahigh linear range, excellent durability and oil/water separation capability. Chemical Engineering Journal 372, 373–382. doi:10.1016/j.cej.2019.04.142
Zhang, J., Zhang, L., and Gong, X. (2021). Large-Scale Spraying Fabrication of Robust Fluorine-Free Superhydrophobic Coatings Based on Dual-Sized Silica Particles for Effective Antipollution and Strong Buoyancy. Langmuir 37 (19), 6042–6051. doi:10.1021/acs.langmuir.1c00706
Zhang, L., Uzoma, P. C., Xiaoyang, C., Penkov, O. V., and Hu, H. (2022). Bio-Inspired Hierarchical Micro/Nanostructured Surfaces for Superhydrophobic and Anti-Ice Applications. Front. Bioeng. Biotechnol. 10, 872268. doi:10.3389/fbioe.2022.872268
Zhang, M., Guo, C., and Hu, J. (2020). One-step fabrication of flexible superhydrophobic surfaces to enhance water repellency. Surface and Coatings Technology 400, 126155. doi:10.1016/j.surfcoat.2020.126155
Zhang, P., Lin, L., Zang, D., Guo, X., and Liu, M. (2016). Designing Bioinspired Anti-Biofouling Surfaces based on a Superwettability Strategy. Small 13 (4), 1503334. doi:10.1002/smll.201503334
Zhang, X., Chen, J., Chen, Y., Lin, X., Wang, B., Liu, Y., Jiang, Y., and Zhang, H. (2021). Studies on Hydrophobic Silica/Silicone Rubber Composite Microspheres with Dual-Size Microstructures. Langmuir 37 (50), 14668–14678. doi:10.1021/acs.langmuir.1c02398
Zhang, X., Guo, Y., Zhang, Z., and Zhang, P. (2013). Self-cleaning superhydrophobic surface based on titanium dioxide nanowires combined with polydimethylsiloxane. Applied Surface Science 284, 319–323. doi:10.1016/j.apsusc.2013.07.100
Zhao, M., Li, W., Wu, Y., Zhao, X., Tan, M., and Xing, J. (2019). Performance Investigation on Different Designs of Superhydrophobic Surface Texture for Composite Insulator. Materials (Basel) 12 (7), 1164. doi:10.3390/ma12071164
Zhao, S., Xia, H., Wu, D., Lv, C., Chen, Q.-D., Ariga, K., Liu, L.-Q., and Sun, H.-B. (2013). Mechanical stretch for tunable wetting from topological PDMS film. Soft Matter 9 (16), 4236. doi:10.1039/c3sm27871a
Zhao, X., Zhang, R., Liu, Y., He, M., Su, Y., Gao, C., and Jiang, Z. (2018). Antifouling membrane surface construction: Chemistry plays a critical role. Journal of Membrane Science 551, 145–171. doi:10.1016/j.memsci.2018.01.039
Zhao, Y., Hao, T., Wu, W., Meng, Y., Cao, X., Zhang, Q., She, W., You, J., Shi, D., and Jiang, T. (2022). A novel moisture-controlled siloxane-modified hyperbranched waterborne polyurethane for durable superhydrophobic coatings. Applied Surface Science 587, 152446. doi:10.1016/j.apsusc.2022.152446
Zhao, Y., Liu, E., Fan, J., Chen, B., Hu, X., He, Y., and He, C. (2019). Superhydrophobic PDMS/wax coated polyester textiles with self-healing ability via inlaying method. Progress in Organic Coatings 132, 100–107. doi:10.1016/j.porgcoat.2019.03.043
Zhao, Y., Liu, Y., Xu, Q., Barahman, M., and Lyons, A. M. (2015). Catalytic, Self-Cleaning Surface with Stable Superhydrophobic Properties: Printed Polydimethylsiloxane (PDMS) Arrays Embedded with TiO2Nanoparticles. ACS Appl. Mater. Interfaces 7 (4), 2632–2640. doi:10.1021/am5076315
Zhou, H., Wang, H., Niu, H., Gestos, A., Wang, X., and Lin, T. (2012). Fluoroalkyl Silane Modified Silicone Rubber/Nanoparticle Composite: A Super Durable, Robust Superhydrophobic Fabric Coating. Adv. Mater. 24 (18), 2409–2412. doi:10.1002/adma.201200184
Zhou, X., Liu, J., Liu, W., Steffen, W., and Butt, H.-J. (2022). Fabrication of Stretchable Superamphiphobic Surfaces with Deformation-Induced Rearrangeable Structures. Advanced Materials 34 (10), 2107901. doi:10.1002/adma.202107901
Zhu, D., Li, X., Zhang, G., Zhang, X., Zhang, X., Wang, T., and Yang, B. (2010). Mimicking the rice leaf-from ordered binary structures to anisotropic wettability. Langmuir 26 (17), 14276–83. doi:10.1021/la102243c
Zhu, R., Liu, M., Hou, Y., Zhang, L., Li, M., Wang, D., and Fu, S. (2020). One-Pot Preparation of Fluorine-Free Magnetic Superhydrophobic Particles for Controllable Liquid Marbles and Robust Multifunctional Coatings. ACS Appl. Mater. Interfaces 12 (14), 17004–17017. doi:10.1021/acsami.9b22268
Zhu, X., Zhang, Z., Ren, G., Yang, J., Wang, K., Xu, X., Men, X., and Zhou, X. (2012). A novel superhydrophobic bulk material. J. Mater. Chem. 22 (38), 20146–20148. doi:10.1039/C2JM33769J
Zimmermann, J., Rabe, M., Verdes, D., and Seeger, S. (2008). Functionalized silicone nanofilaments: A novel material for selective protein enrichment. Langmuir 24 (3), 1053–1057. doi:10.1021/la702977v
Keywords: superhydrophobic, PDMS, nanoparticles, aggregates, antifouling
Citation: He Z, Yang X, Mu L, Wang N and Lan X (2022) A versatile “3M” methodology to obtain superhydrophobic PDMS-based materials for antifouling applications. Front. Bioeng. Biotechnol. 10:998852. doi: 10.3389/fbioe.2022.998852
Received: 20 July 2022; Accepted: 11 August 2022;
Published: 29 August 2022.
Edited by:
Huihua Yuan, Nantong University, ChinaCopyright © 2022 He, Yang, Mu, Wang and Lan. This is an open-access article distributed under the terms of the Creative Commons Attribution License (CC BY). The use, distribution or reproduction in other forums is permitted, provided the original author(s) and the copyright owner(s) are credited and that the original publication in this journal is cited, in accordance with accepted academic practice. No use, distribution or reproduction is permitted which does not comply with these terms.
*Correspondence: Xiaorong Lan, xiaoronglancdm@163.com