- 1Tianjin Key Laboratory of Retinal Functions and Diseases, Tianjin International Joint Research and Development Centre of Ophthalmology and Vision Science, Eye Institute and School of Optometry, Tianjin Medical University Eye Hospital, Tianjin, China
- 2Tianjin Fourth Central Hospital, Department of Ophthalmology, Tianjin, China
Rhegmatogenous retinal detachment (RRD) is the most common retinological emergency that can cause blindness without surgical treatment. RRD occurs when liquefied vitreous accumulates between the neurosensory retina and the retinal pigment epithelium via retinal breaks, which are caused by the separation of the vitreous from the retina with aging. Currently, the main treatment option is pars plana vitrectomy, which involves surgical removal of the vitreous and laser photocoagulation around retinal breaks to generate firm chorioretinal adhesion, as well as subsequent filling of the vitreous cavity with long-lasting substitutes (expansile gas or silocone oil) to prevent the connection between the subretinal space and the vitreous cavity via the breaks before the chorioretinal adhesion firm enough. However, the postoperative face-down position and the not very satisfactory first retinal reattachment rate place a heavy burden on patients. With the development of technology and materials engineering, researchers have developed biomaterials that can be used as a retinal patch to seal retinal breaks and prevent the connection of subretinal space and vitreous cavity via breaks, thus replacing the long-lasting vitreous substitutes and eliminating the postoperative face-down position. Preclinical studies have demonstrated that biomaterial sealants have enough biocompatibility and efficacy in the in vitro and in vivo experiments. Some sealants have been used in clinical trials on a small scale, and the results indicate promising application prospects of the biomaterial sealants as retinal patches in the repair of RRD. Herein, we review the recent advances in biomaterials as retinal patches for the repair of RRD, focusing on the biomaterial categories, methods, and procedures for sealing retinal breaks, as well as their biocompatibility and efficacy, current limitations, and development perspectives.
Introduction
The vitreous body is situated between the lens and retina, and accounts for approximately 80% of the volume of the eye globe. It aids in keeping the retina in place and provides nourishment to the eye. The vitreous humor is a transparent gel-like substance that is predominantly composed of water (98–99%), long fine collagen fibers, and hyaluronic acid (HA). Aging leads to homogeneous vitreous liquefaction into a heterogeneous mixture, including aggregated collagen fibrils and dissociated hyaluronans, because of various factors such as oxidative damage, digestion by enzymes, and collagen mutations. (Tram and Swindle-Reilly, 2018) As the gel network collapses, the vitreous separates from the retina, which may induce retinal breaks at the site if the forces of separation are significantly strong or if there is abnormal adhesion between the vitreous gel and the retina. The flow of liquefied vitreous into the subretinal space via these retinal breaks held open by vitreoretinal traction leads to separation of the neurosensory retina from the underlying retinal pigment epithelium (RPE), that is, rhegmatogenous retinal detachment (RRD) (Figure 1). (Feltgen and Walter, 2014; Lumi et al., 2015) In the pathological process of RRD, not only does the liquefied vitreous enter the subretina through retinal breaks, but the RPE cells also lose cell-cell contact and diffuse into the vitreous cavity via retinal breaks, undergo epithelial-mesenchymal transition (EMT), and eventually transform into myofibroblasts. This causes the development of proliferative fibrocellular membranes with the ability to contract, which facilitates the progression of tractional retinal detachment (Figure 2). (Pastor et al., 2016; Zheng et al., 2022).
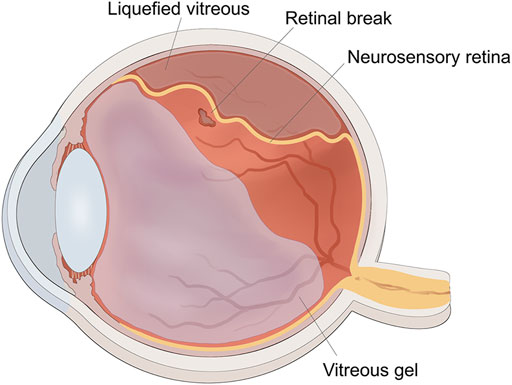
FIGURE 1. The schematic diagram of rhegmatogenous retinal detachment. The traction of vitreous gel onto retina created the retinal break, liquified vitreous penetrated into the subretinal space through the retinal break to induce retinal detachment.
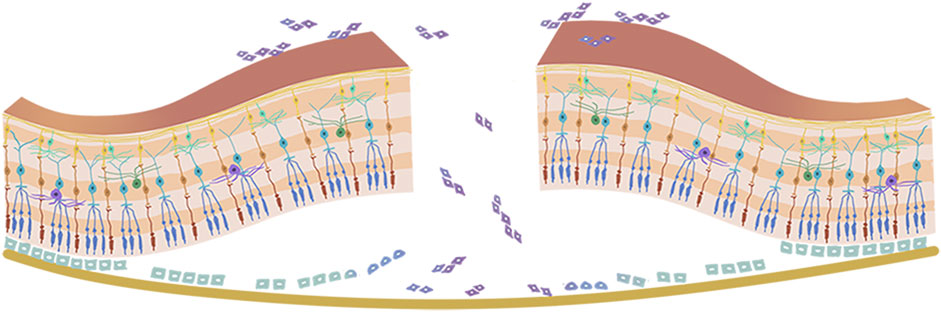
FIGURE 2. Pathophysiology of proliferative vitreoretinopathy. The retinal pigment epithelium cells lose cell-cell contact, and diffuse into the vitreous cavity via retinal breaks, then go through epithelial-mesenchymal transition (EMT) and eventually transform into myofibroblasts.
RRD is one of the most common retinological crises, and must be treated as quickly as possible. (Yorston et al., 2021) In view of the fact that the three necessary pre-requisites for the progression of RRD are the flow of liquefied vitreous, vitreoretinal tractional forces, and retinal breaks, the management principles are to treat all the retinal breaks and weaken or eliminate vitreoretinal traction to prevent the penetration of fluid into the subretinal space via retinal breaks. Invasive procedures include single pneumatic retinopexy (PR), scleral buckling (SB), and pars plana vitrectomy (PPV), as well as any combination of these. (Kuhn and Aylward, 2014) PR is the intravitreal injection of an expanding gas bubble to act as a tamponade to re-attach the retina. Although this technique is simple and causes minimal damage, its indications are limited. It has only been recommended for individuals with small, anterior, superior retinal breaks and little or no proliferative vitreoretinopathy (PVR). (Kunikata et al., 2019; Chronopoulos et al., 2021) SB is performed after accurate localization of all retinal breaks and precise cryotherapy around retinal breaks for scar induction, and by suturing foam sponges or silicone bands to the sclera to cause an inward indentation of the sclera and choroid to neutralize the traction of the vitreous on the retina. (Kuhn and Aylward, 2014; Kunikata et al., 2019) PPV begins with a thorough removal of the vitreous, which eliminates vitreoretinal tractional force and resets the detached retina, followed by laser photocoagulation around the edge of the retinal breaks to generate chorioretinal adhesion, and injection of the vitreous cavity with long-acting alternatives (expansile gas or silocone oil) to act as a tamponade. The fundamental role of long-lasting vitreous substitutes is to keep the neurosensory retina attached to the RPE and prevent liquid flow between the vitreous cavity and the subretinal space until the chorioretinal adhesion becomes firm enough to seal the retinal breaks. (Feltgen and Walter, 2014; Zheng et al., 2022) However, the tamponade property of long-lasting vitreous substitutes requires a long-term postoperative face-down position, given that their gravity is lower than that of the aqueous humor. Moreover, the visual quality is poor during this period because the refractive index of vitreous substitutes is lower or higher (1.00 in expansile gas and 1.40 in silocone oil) than in the natural human vitreous (1.33). (Hoshi et al., 2019) The refractive status returns to normal after the expansile gas is absorbed within 2 weeks to 2 months, or after the silicone oil is removed via a second surgical procedure, because the refractive index of the aqueous fluid produced by the eye itself and filled in the vitreous cavity is identical to that of the natural vitreous. (Wagenfeld et al., 2010; Chen et al., 2015; Kontos et al., 2017; Raczynska et al., 2018; Tetsumoto et al., 2020; Popovic et al., 2022) For simple RRD with anterior retinal breaks, there were no significant differences between RRD and SB in terms of the primary success rate, visual acuity gain, and final anatomical success. (Znaor et al., 2019) However, for some more challenging situations, such as giant retinal breaks, multiple large breaks, bullous detachment, and cases complicated by severe PVR, isolated PPV or PPV and SB combined with long-lasting vitreous substitutes are the first-line treatment options. (Wang and Snead, 2020).
With evident medical and technical breakthroughs, PPV has increased in popularity and has become the most commonly performed surgery for the treatment of RRD, albeit with an initial retinal reattachment rate ranging from 74% to 96.3%. (Minihan et al., 2001; Bourla et al., 2010; Oshima et al., 2010; Kobashi et al., 2014; Haugstad et al., 2017; Romano et al., 2017) The most prevalent cause of surgical failure in retinal detachment is PVR. RPE cells are crucial contributors in triggering PVR. Therefore, it would make sense to prevent PVR by inhibiting RPE cell migration, proliferation, and EMT through retinal breaks by patching the breaks with an appropriate substance. Likewise, the application of such a material could effectively block communication between the two sides of the breaks, thereby eliminating the need for long-lasting vitreous substitutes and the postoperative face-down position. This review summarizes the materials, methods, and procedures for patching retinal breaks as well as their biocompatibility and efficacy in the repair of RRD, which could provide basic and comprehensive information.
Clinical biomaterials
Surgical glues are useful adjuncts to surgical care and are classified as hemostats, adhesives, and sealants, based on their function. Hemostats act as hemostatic agents to clot the blood. While adhesives bond two surfaces together, like wounds or incisions, sealants mostly develop a barrier layer to prevent fluid or air leakage. It is noteworthy that the same glue can perform numerous functions. For instance, fibrin glue can function as a sealant, adhesive, or hemostat. (Jain and Wairkar, 2019) A retinal sealant is utilized in the vitrectomy repair of RRD to build a seal over the retinal breaks before the chorioretinal adhesion becomes firm enough to avoid the migration of RPE into the vitreous, thus reducing the subsequent PVR. The general surgical steps are complete PPV followed by fluid-air exchange to remove subretinal fluid and achieve retinal reattachment, laser photocoagulation around retinal breaks under air conditions, full coverage of retinal breaks with retinal sealants, and finally leaving sterile air to fill the vitreous cavity or proceeding with fluid-air exchange again to allow balanced salt solution (BSS) to fill the vitreous cavity (Figure 3). An ideal retinal sealant must have certain characteristics to create a stable and safe seal around the retinal breaks: (A) Biocompatibility indicates that it does not cause local irritation, inflammation, toxicity, or antigenicity. (B) Strong and stable persistent adhesion means that it must remain firmly attached and adherent to the retina for an appropriate time frame to permit the establishment of chorioretinal adhesion induced by laser photocoagulation. (C) Owing to its flexibility, it should be delivered to the target surface via a simple procedure. (D) Biodegradability: It should be naturally absorbable to avoid the long-term negative effects of persistent foreign bodies and the need for a second surgery to remove it.
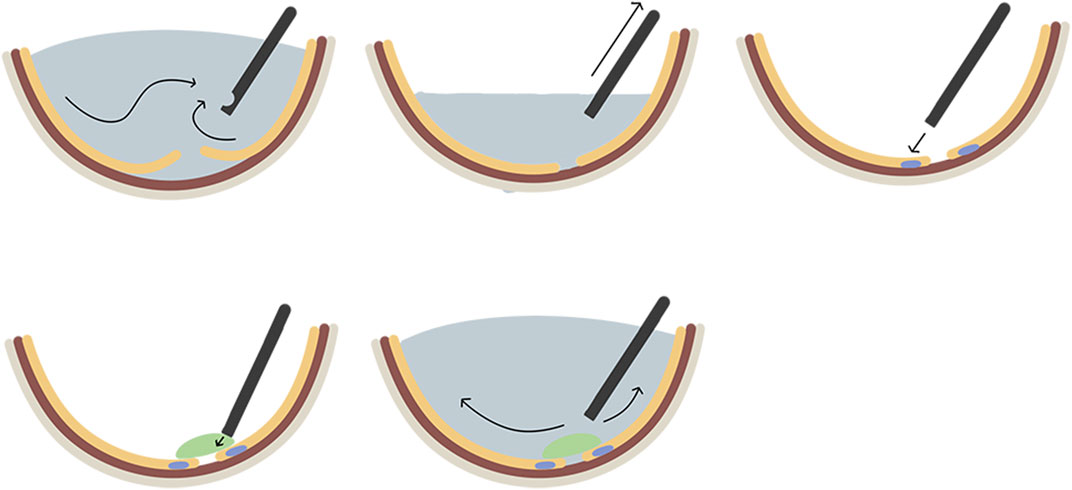
FIGURE 3. The general surgical steps of applying biomaterials as retinal patches for the repair of rhegmatogenous retinal detachment. A. complete pars plana vitrectomy; B. fluid-air exchange to remove subretinal fluid and achieve retinal reattachment; C. laser photocoagulation around retinal break; D. full coverage of retinal breaks with biomaterial sealants; E, fluid-air exchange again to fill the vitreous cavity with the balanced salt solution.
Currently, there are two major categories of tissue sealants used in ophthalmic surgery: natural compound-based sealants (such as protein derivatives and polysaccharide derivatives) and synthetic material-based sealants [such as cyanoacrylate and polyethylene glycol (PEG) derivatives]. (Guhan et al., 2018; Jain and Wairkar, 2019) Sealants derived from natural biopolymers are obtained from biological constituents, which provide many benefits over synthetic materials, such as greater biocompatibility, lower immunological response, in vivo degradability, and the potential to guide and promote endogenous reparative processes. Conversely, sealants derived from synthetic polymeric materials also offer some advantages over natural biomaterials because of their well-defined chemical compositions, such as reproducibility, more precise control over gel time, swelling behavior, mechanical properties, adjustable mechanical strength, degradation rate, and byproducts. These advantages make it possible to tailor the materials for specific biomedical and biotechnological applications. (Heher et al., 2018) Table 1 summarizes the studies on different sealants for RRD surgery.
Sealants of natural origin
Sealants of natural origin are classified into two types: those based on proteins (such as fibrin and gelatin-based sealants) and those based on polysaccharides (such as HA-based and chondroitin sulfate-based sealants). Nevertheless, the use of original natural sealants is hindered by some limitations, such as poor mechanical properties, weak manipulability, and rapid degradation. Cross-linking can compensate for these undesirable deficiencies, while maintaining the biocompatibility and biodegradability of the original materials. Cross-linking is a polymer stabilization procedure that results in multidimensional extension of polymer chains by attaching one polymer to another, consequently forming a stable network structure. (Poudel et al., 2022) Sealants are divided into two categories according to the type of cross-linking junctions: physically and chemically cross-linked. Physically cross-linked networks have transient junctions between polymeric chains that are mediated by chain entanglements or physical interactions such as hydrophobic interactions, hydrogen bonding, or ionic interactions. Chemically cross-linked networks have permanent junctions, which refer to the intermolecular or intramolecular joining of two or more molecules by a covalent bond via the introduced cross-linkers. (Arora et al., 2017; Lu et al., 2018) As no extra chemical components are added to the physically cross-linked networks, they ensure the favorable biocompatibility of the original materials. However, some added cross-linkers in chemically cross-linked networks may cause cytotoxicity that the human body cannot endure at high concentrations. (Jeon et al., 2007; Lai, 2014).
Protein-based materials are more often employed, whereas polysaccharide-based systems are very popular and desirable. The representative sealants are described below. Table 2 and Table 3 summarizes these two different materials as retinal patches for RRD surgery.
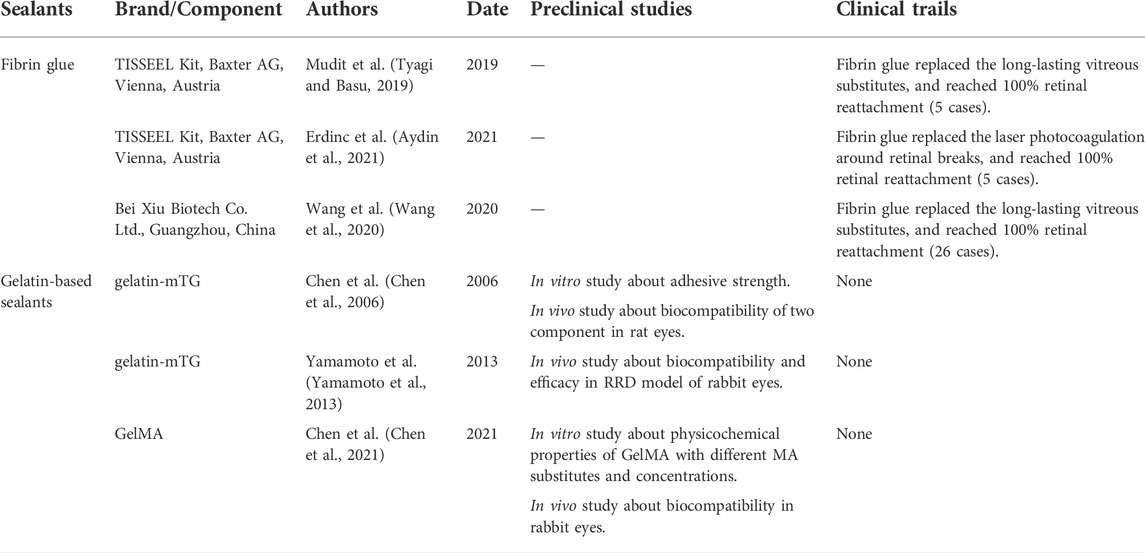
TABLE 2. The summaries of studies about sealants based on proteins as retinal patches for the treatment of RRD.
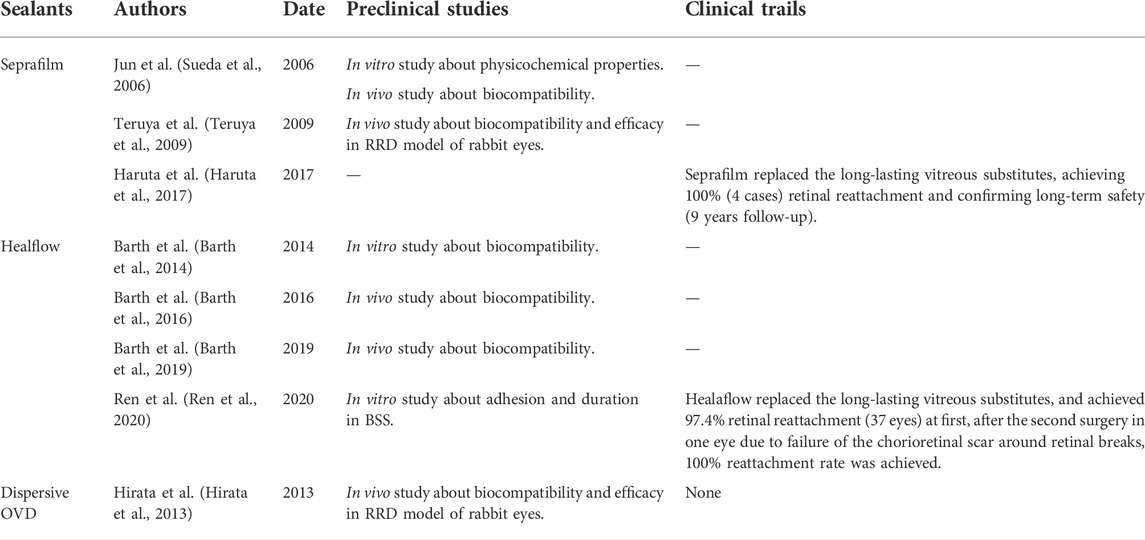
TABLE 3. The summaries of studies about sealants based on polysaccharides as retinal patches for the treatment of RRD.
Sealants based on protein
Fibrin glue
Fibrin is a protein found in the human blood that assists in blood clotting. Fibrin glue is a biological sealant derived from blood products, and its working principle is to imitate the final stages of the coagulation cascade, wherein the fibrin clot is formed. Fibrin glue consists of two components drawn in separate syringes. The first is primarily composed of human plasma proteins, including fibrinogen, factor XIII, fibronectin, and plasminogens. The second is the combination of thrombin and calcium chloride. The transformation of fibrinogen to fibrin glue after the mixture of the two solutions consists of three main steps: 1) thrombin cleaves soluble fibrinogen into insoluble tiny fibrinopeptides; 2) thrombin also stimulates factor XIII to form transglutaminase-factor XIIIa in the presence of calcium ions; and 3) factor XIIIa catalyzes the transformation of fibrinopeptides to fibrin network via the formation of amide bonds between the lysine (Lys) and glutamine (Gln) residues (Figure 4). Cross-linking contributes to the stability and deformation resistance of fibrin clots as well as resistance to solubilization. Hence, transglutaminases are recognized as natural biological crosslinkers. The conversion of the solution to a milky white color can be observed when the glue begins to gel. Moreover, the concentration of the thrombin activation solution determines the formation rate of fibrin glue, while the adhesive strength of fibrin glue is directly proportional to the concentration of available fibrinogen. (Brennan, 1991; Scognamiglio et al., 2016) Finally, fibrin glue is progressively absorbed by a biological process in which the tissue plasminogen activator (tPA) cleaves plasminogen into plasmin, which subsequently lyses fibrin into soluble fibrin degradation products. (Brennan, 1991).
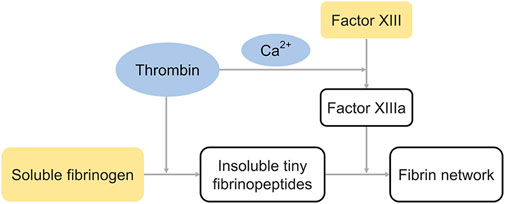
FIGURE 4. The cross-linking process of fibrin glue. Thrombin catalyzes the formation of insoluble tiny fibrinopeptides and factor XIIIa, and factor XIIIa catalyzes the transformation of fibrinopeptides to fibrin network. The blue and yellow represent components in different syringes.
With the advancement of technology, biologically derived fibrin glue can now be mass-produced with meticulousness to eliminate any possibility of contamination or inclusion of infectious organisms. The sealing properties of fibrin glue have been extensively described in the fields of pulmonary and anorectal surgery, where it is particularly effective in preventing alveolar air leakage and closing fistula tracts. (Zmora et al., 2003; Kawamura et al., 2005) Furthermore, fibrin glue has been shown to be safe and effective as an ocular tissue sealant in ocular surface surgeries as well as in vitreoretinal surgeries. It has been used in ocular surface surgery for sealing corneal and conjunctival wound leaks, corneal stem cell transplantation, pterygium surgery, conjunctival closure after surgery, etc., (Pfister and Sommers, 2005; Srinivasan et al., 2009; Sonmez and Beden, 2011; Yang et al., 2013; Romano et al., 2016; Scalcione et al., 2018) Furthermore, isolated case reports have presented excellent results of fibrin glue as an adjunctive option in the surgical treatment of optic disc pit-associated macular detachment. (de Oliveira et al., 2017; Almeida et al., 2018) Therefore, it is theoretically feasible to apply fibrin glue to seal retinal breaks in patients with RRD based on favorable biocompatibility and sealing properties. Mudit et al. (Tyagi and Basu, 2019) reported that the initial clinical results of fibrin glue-assisted repair for RRD patients. Following the completion of PPV, fluid-air exchange, and laser photocoagulation around retinal breaks, the breaks were subsequently covered with 0.1–0.2 ml of fibrin glue (TISSEEL Kit, Baxter AG, Vienna, Austria), and subsequently the vitreous cavity was filled with the BSS after the glue formed a thick fibrin clot. At the end of the process, there was no evidence of glue migration or displacement and the patients were not instructed to maintain any specific postoperative body positioning. All the five cases (100%) had successful retinal reattachment, which was maintained after 3–8 months of follow-up. No eye showed any increase in postoperative inflammation or intraocular pressure (IOP), and electroretinography (ERG) revealed no remarkable alterations in retinal function. The milky-white color of the fibrin gel was visible during the follow-up period. The clump of fibrin glue significantly decreased in size after 1 week and completely dissolved after 2 weeks. However, the authors recommended avoiding using the dual-chambered syringe provided with the product during the fibrin glue application procedure because the glue tends to gel once there is contact between the two components (sealer protein and thrombin solutions) within the 25-gauge needle, which would block it. Preferably, the two components of the glue should be separately prepared in 1 ml insulin syringes. The procedure involves injecting a more viscous sealer protein solution to cover the retinal breaks completely, and then applying thrombin solution to mix them to form a gel. Likewise, the clinical trial conducted by Wang et al. (Wang et al., 2020) included a slightly larger sample size (26 eyes) and presented a 100% retinal reattachment rate during the entire follow-up period using the same surgical procedures as mentioned above. Commercially available fibrin gel was obtained from the Beixiu Porcine Fibrin Sealant Kit (Bei Xiu Biotech Co., Ltd., Guangzhou, China). Both pilot trials demonstrated that fibrin glue could be used as a substitute for endotamponades in certain RRD cases. Moreover, Erdinc et al. (Aydin et al., 2021) explored the use of fibrin glue as an alternative to laser photocoagulation for RRD treatment. After applying fibrin glue (TISSEEL Kit; Baxter AG, Vienna, Austria) on retinal breaks for 5 min, the vitreous cavity was filled with long-lasting vitreous substitutions. No laser photocoagulation was performed. All the five patients had successful retinal reattachment, and no postoperative complications were detected during the following 10–24 months.
These findings provide preliminary evidence to support the favorable biocompatibility and efficacy of commercially available fibrin glues as a prospective retinal patch for sealing retinal breaks in clinical RRD patients, and may potentially replace the use of endotamponades or laser photocoagulation. Nevertheless, all the clinical data available to date have been derived from small-scale clinical trials. More validation is needed, including longer follow-up, larger sample sizes, and multicenter clinical trials.
Gelatin-based sealants
Gelatin is a mixture of peptides and proteins obtained by the partial hydrolysis of collagen, and the peptide bonds link them together to form polymers with molecular weights ranging from 15,000 to 400,000 Da. (Echave et al., 2017) Gelatin has target sequences for the enzymatic degradation of matrix metalloproteinases (MMP), so the degradation products are biocompatible. (Backstrom and Tokes, 1995) The US Food and Drug Administration (FDA) has allowed the use of gelatin in biomedical applications because of its desirable qualities such as bioactive moieties, biocompatibility, biodegradability, and relatively low antigenicity. (Kang and Park, 2021) It is used clinically as a plasma expander and stabilizer in a variety of protein formulations, vaccinations, and gelatin sponges. (Elzoghby, 2013) However, owing to the relative instability of gelatin hydrogels in aqueous solutions (they swell and often dissolve above 35°C), several cross-linking methods, including chemical, photochemical, and enzymatic methods, have been utilized to provide stability under biological circumstances. (Scognamiglio et al., 2016; Echave et al., 2017) Traditionally, aldehydes are well-known chemical cross-linkers for peptides; however, they are hazardous to mammalian cells at high doses in the millimolar range because of the cytotoxicity, immunogenicity, and inflammatory effects of their degradation products. (Esterbauer et al., 1991) Therefore, there has been an increasing interest in using natural cross-linkers, such as transglutaminase or tyrosinase, to cross-link gelatin by enzymatic methods under physiological conditions. Nevertheless, direct cross-linking methods (without prior modification of gelatin) exhibit limited tailorability in the design of hydrogels, mainly because of poor control of the cross-link density and the resulting stiffness. Therefore, adding functional groups to the gelatin backbone before cross-linking provides a great degree of control over the design and properties of hydrogels. For these reasons, functionalized gelatin, followed by cross-linking, has overtaken direct cross-linking as the favored approach.
Enzymatically crosslinked gelatin (gelatin-mTG)
The gelatin microbial transglutaminase (gelatin-mTG) is generated by cross-linking gelatin molecules via the cross-linker of calcium-independent mTG enzyme, which covalent bond between a free amine group of a peptide-bound Lys and the acyl group at the end of the side chain of a peptide-bound Gln. (Scognamiglio et al., 2016) Gelatin-mTG could form a gel in 30 min under moist conditions, and this gel could withstand pressures of 200 mmHg in the in vitro test. The tensile static and dynamic loading of the adhesive hydrogels in bulk revealed that the Young’s modulus of gelatin-mTG ranged from 15 kPa to 120 kPa, and these bulk parameters were equivalent to those of fibrin-based sealant hydrogels. (McDermott et al., 2004; Liu et al., 2009) Therefore, based on the substantial adhesive and cohesive strength of gelatin-mTG, Chen et al. (Chen et al., 2006) first examined the potential of gelatin-mTG sealants (gelatin was obtained from Sigma Chemicals and mTG was purchased from Ajinomoto USA) for ophthalmic applications, especially for retinal reattachment. Gelatin-mTG sealants were prepared by mixing gelatin and mTG solutions for 1 min. The in vitro experiment demonstrated that the gelatin-mTG sealants could attach to moist retinal tissue, and the adhesive strengths achieved lap-shear strengths of 15–45 kPa over 30 min, which is comparable to strengths found with other soft-tissue adhesives. The in vivo histological study indicated that the components of gelatin-mTG are potentially biocompatible, since they did not induce cellular damage in the retinal tissues of rats after gelatin and mTG diffusion into the retina following vitreous cavity injection. Subsequently, Yamamoto et al. (Yamamoto et al., 2013) investigated the effects of using gelatin-mTG sealants for treating experimental RRD in rabbits. Reattachment of the retina occurred in all four eyes after retinal breaks were covered with gelatin-mTG sealants. Simultaneously, optical coherence tomography (OCT) revealed that the gelatin-mTG sealants were still adhered to the retina at least 1 week after the initial application, and ERG demonstrated that gelatin-mTG sealants had no negative impact on retinal function.
Photocrosslinked gelatin derivatives (GelMA)
Methacrylic anhydride (MA) is a well-known functional group that can partially substitute methacryloyl groups on reactive amines to obtain modified gelatin, that is, gelatin methacrylate (GelMA). GelMA is photoreactive and can be photocrosslinked via ultraviolet or visible light to achieve high physiological stability. In particular, the physical properties can be optimized for different applications by tuning the degree of gelatin modification, photoinitiator concentration, and cross-linking conditions. The chemical modification of gelatin by MA involves no more than 5% of the amino acid residues in the molar ratio, which implies that most of the functional amino acid domains of gelatin (such as MMP-degradable motifs) will be retained; thus, the GelMA hydrogels preserve the remarkable biocompatibility and bioactivity of gelatin. (Yue et al., 2015; Klotz et al., 2016) In terms of adhesion and sealing properties, GelMA outperforms clinically used fibrin glues and PEG-based glues in vitro, and is biocompatible enough to adequately seal large lung leaks and tracheal injuries in animal models. (Assmann et al., 2017; Gasek et al., 2021) Therefore, Chen et al. (Chen et al., 2021) engineered GelMA with varying degrees of substitution (25, 50, and 75%) and concentrations (10, 20, and 30%), and then tested and compared their adhesion, stability at 37°C, degradation time, swelling ratio, photo-crosslinking time, pH, and physical conditions before photo-crosslinking. In the in vitro study, the GelMA hydrogel with a 75% degree of substitution and a 20% concentration (75/20) displayed greater adhesion and stability than the other nine GelMA hydrogels (substitution/concentration) during the first 2 weeks. Furthermore, the GelMA hydrogel (75/20) was in gel condition at 37°C and could be injected with a 25-gauge needle before photo-crosslinking. The degradation time was reasonable, the swelling ratio was low, the photo-crosslinking time was short, and the pH was steady. Thus, the GelMA hydrogel (75/20) had the ideal formula. Histological examination showed that the retinal layer was not damaged 28 days after vitreous injection of the GelMA hydrogel (75/20). Although the animal RRD model has not been established to assess its effectiveness in vivo, preliminary conclusions could be drawn that GelMA (75/20) exhibits favorable intraocular biocompatibility, and it may be a suitable formulation for use as a patch to seal retinal breaks in RRD surgery.
In summary, gelatin-based sealants show great promise in preclinical in vitro and in vivo studies, indicating that they would be efficient with excellent prospects for clinical application. However, there are no clinical data regarding gelatin-based sealants for the treatment of RRD. Clearly, clinical trials in humans are necessary to completely evaluate the efficacy and safety of gelatin-based sealants for treating human RRD.
Sealants based on polysaccharides
HA-based sealants
HA is a natural linear anionic polysaccharide with many distinct properties such as good biocompatibility and biodegradability, native biofunctionality, hydrophilicity, and non-immunoreactivity. (Ding et al., 2022) These properties contribute to its utilization as a promising candidate in clinical ophthalmology. HA has been used as an artificial tear ingredient to treat dry eyes, as well as a viscoelastic agent for cataract surgery. However, under natural conditions, numerous inherent limitations, including poor mechanical qualities and restricted cell adhesion, have hindered its wider utilization. These challenges can be solved by cross-linking HA chains to generate a gel-like structure that varies from its raw sol-like nature. Covalent cross-linking of HA can be achieved in two ways: by directly adding a cross-linker, or by first modifying the carboxyl, hydroxyl, or N-acetyl groups of HA chains with functional groups, followed by cross-linking. (Perez et al., 2021)
Seprafilm
Seprafilm is a translucent bioresorbable membrane composed of carboxymethylcellulose (CMC) and HA that has been chemically modified to slow the rate of degradation and clearance after implantation in the body. This membrane transforms the form of thin sheets into a hydrophilic gel and binds strongly to the target surface of tissues within 24–48 h after placement in a wet environment in the body. Animal experiments have shown that Seprafilm is absorbed from the application site within 7 days and is completely cleared within 28 days after implantation. Seprafilm is primarily used in abdominal and pelvic laparotomies asan adhesive physicochemical barrier to prevent adhesion between adjacent tissues and was approved by the FDA in 1996. (Diamond et al., 2012a; Diamond et al., 2012b; Guo et al., 2021) Afterwards, Seprafilm was utilized and investigated in an “off-label” capacity at the discretion of individual surgeons. Therefore, Tsurumaru et al. (Tsurumaru et al., 2009) investigated its efficacy in preventing postoperative adhesion between the conjunctiva and sclera during glaucoma filtering surgery. Animal studies have shown that it is effective in reducing adhesion and maintaining low eye pressure, and may be a desirable antifibrotic agent for trabeculectomy in the early stages of wound repair. Similarly, based on its adhesion and barrier properties, Jun et al. (Sueda et al., 2006) evaluated the safety and efficacy of Seprafilm (Genzyme Corporation, Cambridge, MA, USA) for sealing retinal breaks in animals. The in vitro investigation indicated that Seprafilm remained solid in BSS for 30 days before dissolving and that the pH of this solution was between 7.2 and 8.0, which is neutral for intraocular use. It also adhered well to the retina and effectively inhibited the permeation of methylene blue. After crushing and powdering the Seprafilm and mixing it with BSS, 0.1 ml of this solution was injected into the vitreous cavity of rabbits. This in vivo study demonstrated that there was no postoperative ocular inflammatory reaction during the 6-week follow-up, and ERG and histology proved that the morphology and function of the retina were normal before and after injection. As a result of technological advancements and these promising preliminary findings, Teruya et al. (Teruya et al., 2009) further investigated the short-term (14-day) effects of Seprafilm® for patching retinal breaks in rabbit eyes with experimental RRD. The retina was reattached using Seprafilm as retinal patches during the 14-day observation period. Funduscopic examination and OCT revealed that the Seprafilm adhered tightly to the retina, the border of the breaks was indistinct on the 7th postoperative day, and Seprafilm dissolved within 14 days. Simultaneously, histological examination revealed that the Seprafilm application sites showed no inflammatory changes. Hence, the favorable biocompatibility and efficacy of seprifilm indicate that it is a potential retinal patch for repairing retinal breaks in clinical RRD cases. Subsequently, Haruta et al. (Haruta et al., 2017) confirmed the long-term safety of intraocular Seprafilm (Sanofi, Bridgewater, NJ, USA) in clinical RRD patients. Following laser photocoagulation around retinal breaks in four patients, a 5 × 2 mm sheet of Seprafilm was placed over the retinal breaks after being delivered into the eye through one of the sclerotomy sites that had been enlarged to 3 mm. The retina remained reattached in all the four eyes at 1, 3, and 6 months and 9 years postoperatively, with no immediate or late adverse events. Electroretinography and specular microscopy revealed no evidence of Seprafilm toxicity 9 years after the surgery.
Healaflow
Healaflow (Anteis S.A., Plan Les Ouates, Switzerland) is a commercially available translucent hydrogel made of over 97% water and reticulated HA of non-animal origin (22.5 mg/ml) using 1,4-butanediol diglycidyl ether (BDDE) as a cross-linker. BDDE is a well-known industry-standard cross-linker of market-leading cross-linked HA products. Its stability and metabolism have been thoroughly examined, and long-term safety has been confirmed by various studies and clinical trials over the years. (De Boulle et al., 2013) Healaflow is initially used in glaucoma filtration procedures as a space-filler to minimize postoperative fibrosis, and it usually takes about 3 months for the body to completely degrade. Its effect on maintaining functional filtration blebs and controlling and stabilizing intraocular pressure is comparable to that of mitomycin-C. (Bettin et al., 2016; Mudhol and Bansal, 2021; Wu et al., 2021) The composition of Healaflow is similar to that of natural vitreous: a reinforced hydrogel composed of HA with similar light transmittance as well as physical and mechanical properties. Therefore, it is a plausible candidate for vitreous substitution. Accordingly, Barth et al. (Barth et al., 2014) introduced the retinal explant assay as a valuable technique for early biocompatibility assessment, prior to more expensive and time-consuming in vivo testing. The neurosensory retina of the rat eyes was dissected from the RPE and vitreous, explanted onto culture plate inserts, and co-cultured after being covered with hydrogel. In this setting, retinal explants cultured with Healaflow outperformed standard-cultured specimens and appeared to reduce the trauma induced by the culture process. Further in vivo biocompatibility testing was conducted. After central vitrectomy and fluid-air exchange, approximately 0.5–1 ml Healaflow was injected into the vitreous cavity via a 25-gauge needle. Postoperative clinical evaluation, ERG, histology, and immunohistochemistry clearly showed that Healaflow displayed good biocompatibility with no morphological or functional alterations to the retina. (Barth et al., 2016; Barth et al., 2019) Ren et al. (Ren et al., 2020) described the in vitro testing of Healaflow adhesion. That is, Healaflow was applied to three walls of a culture flask filled with BSS (the inside surface of the lid, side wall, and bottom), and 14 days later, Healaflow remained adherent on the three walls with no change in size. On the premise that Healaflow combines good biocompatibility and adhesion, the authors further explored the potential of Healaflow as a retinal patch for the treatment of clinical primary RRD. After complete vitrectomy, fluid-air exchange, and laser photocoagulation around the retinal breaks, Healaflow was applied to the surface of all breaks with a 27-gauge needle in 37 patients (38 eyes). Primary reattachment was achieved in 37 eyes (97.4%) due to failure of the chorioretinal scar around the retinal breaks in one eye. Final reattachment was achieved in all eyes (100%) after the second surgery, with no significant adverse complications.
To summarize, these two commercially available HA-based sealants were tested in preclinical in vitro and in vivo experiments, as well as in clinical RRD patients, and showed good efficacy and safety as retinal patches for RRD treatment. HA-based sealants sealing retinal breaks could replace long-standing vitreous substitutes, allowing for earlier visual recovery and fewer complications. However, unlike fibrin glue, neither of the two HA-based sealants displayed alternative properties to laser photocoagulation. Ren et al. (Ren et al., 2020) described a patient with failed retinal reattachment due to the lack of chorioretinal scarring around retinal breaks. However, Healaflow is better than Seprifilm in terms of flexibility. The sheet shape of Seprafilm necessitates expansion of the sclerotomy sites to deliver it into the eye, and the conditions must be kept moist to transform it into a hydrophilic gel. In contrast, the gel nature and injectable properties of Healaflow make it easy to deliver into the eye and cover retinal breaks.
Chondroitin sulfate-based sealants
Ophthalmic viscosurgical devices (OVDs) with both solid and fluid properties are commonly used in anterior segment surgery, such as in challenging cataracts, flat anterior chambers, pseudoexfoliation syndrome, intraoperative floppy iris syndrome, or glaucoma surgery. Its main physical properties not only create and maintain anterior chamber depth and visibility but also protect corneal endothelial cells and other intraocular tissues. (Bissen-Miyajima, 2008; Borkenstein et al., 2021) The commonly used OVDs are composed of the following three building blocks: sodium HA, chondroitin sulfate, and hydroxypropyl methylcellulose. Chondroitin sulfate is a sulfated glycosaminoglycan that is composed of alternating sugar chains. It is often associated with proteins as a component of proteoglycans. OVDs containing chondroitin sulfate are known as dispersive OVDs, which have a stronger adhesive ability than other cohesive OVDs. (Hirata et al., 2013) Hirata et al. (Hirata et al., 2013) investigated the efficiency of retinal reattachment in four experimental rabbit RRD eyes by short-term patching of retinal breaks with a dispersive OVD (Viscoat, Alcon, Japan), based on the premise of its strong adhesive ability. After temporarily flattening the detached retina and performing laser photocoagulation around the retinal breaks, then the dispersive OVD was injected on the retinal surface to patch retinal breaks. Postoperative inflammation did not differ between rabbit eyes received dispersive OVD as a retinal patch and those who did not (the control group). OCT revealed that the dispersive OVD was slightly decreased, however, it was still adequate to patch the retinal break 3 days after surgery. Seven days later, the dispersive OVD disappeared, the retina was repositioned, and no re-detachment was observed. Alternatively, retinal detachment persisted in the control group. Furthermore, scanning electron microscopy demonstrated that in the control group, the edge of the retinal breaks was clearly identifiable and the surrounding retina was floating, while in the eyes with dispersive OVD, the breaks were indistinct and the exposed areas were covered with glial cells. Measurements showed a significant reduction in the exposed RPE area in the dispersed OVD group.
These results suggest that OVD is a promising new treatment option for RRD, however, further clinical trials are needed to verify its effectiveness and safety.
Sealants of synthetic materials
The performance inefficacy, safety issues (immune responses, viral transmission, possible cytotoxicity triggered by residual chemical cross-linkers, etc.), and limitations related to the application of some natural-based surgical sealants have pushed researchers to develop synthetic sealants, whose precise control over their mechanical properties via modification of a variety of functional groups makes them perform better in the biological environment, and have more application areas and fewer drawbacks. Synthetic adhesives are composed of synthetic chemicals, typically monomers, pre-polymers, or non-cross-linked polymers, which undergo in situ polymerization or cross-linking to form insoluble adhesive matrices when applied to tissues. (Mehdizadeh and Yang, 2013) Various types of synthetic sealant materials have been used for general surgical applications. Generally, synthetic sealant materials fall into one of the three categories: cyanoacrylates, PEGs, or polyurethane. However, in terms of retinal patches for RRD treatment, there are no studies on polyurethane-based sealants. Meanwhile, after the localized but definite retinal toxicity was validated by histological examinations in rabbit eyes 1 month after cyanoacrylate implantation, (Hida et al., 1988) further clinical studies were prevented. Currently, the synthetic sealants used as retinal patches for the treatment of RRD are PEG-based sealants.
PEG-based sealants
PEG is a synthetic, inert, water-soluble polymer that is widely used in the biomedical field owing to its excellent biocompatibility and non-immunogenicity. Modification by degradable functionalities or copolymerization with biodegradable polymers can mask the lack of biodegradability, which enables its successful use in a variety of biomedical applications, such as acting as a hemostat or a fluid barrier via different end groups. (Scognamiglio et al., 2016) For example, the PEG hydrogel (DuraSeal Dural Sealant System) has been approved by the US FDA for obtaining watertight dural closure when applied after standard dural suturing. (Osbun et al., 2012) In addition, in the field of ophthalmology, the PEG-based synthetic sealant could also act as an adhesion barrier to prevent leakage of filtration blebs and as an adhesive to close sclerotomies of PPV in rabbit eyes. (Hoshi et al., 2016; Nagata et al., 2020).
In preclinical studies of RRD, PEG-based sealants from different brands showed excellent biocompatibility and efficacy in both in vitro and in vivo investigations when applied as retinal patches to close retinal breaks for the treatment of RRD. Table 4 summarizes the studies on different brands. However, no clinical data are available to date. The brand of DuraSeal® (Confluent Surgical, Waltham, MA, USA) is composed of two synthetic liquids: a PEG solution with a gel-like consistency (>90% water) and an amine component that serves as a precursor. Polymerization occurred within 20 s of mixing the two components without heat formation. (Sueda et al., 2007; Barliya et al., 2018) The brand of Medicus polymer (Medicus Biosciences, San José, California, USA) comprised solutions of PEG 8-arm acetate amine combined with solutions of PEGylated 8-arm ester dissolved in physiological buffer solution and a viscosity enhancer. In mature market commodities, the component is kept in powder form in a syringe and must be combined with a viscosity enhancer (methylcellulose) before being used in hydrogel conditions. (Kerr, 2014; Sarfare et al., 2015; Hubschman et al., 2017) One thing that makes this brand of compound unique compared to other PEG-based sealants is that the kinetics of its degradation polymerization can be customized by the surgeons themselves via different formulations. (Hubschman et al., 2017) The brand of FocalSeal® (Genzyme Corporation, Cambridge, MA, USA) is an ethylene glycol-oligotrimethylene carbonate copolymer that has been end-capped with acrylate esters. Triethanolamine (90 mM) and eosin Y were also present in the solution, acting as photoinitiators. A transparent, flexible, and securely adhering hydrogel was created by polymerizing the solution for 40–60 s with visible illumination from a xenon arc lamp (450–500 nm, blue-green). (Hoshi et al., 2015; Hoshi et al., 2018).
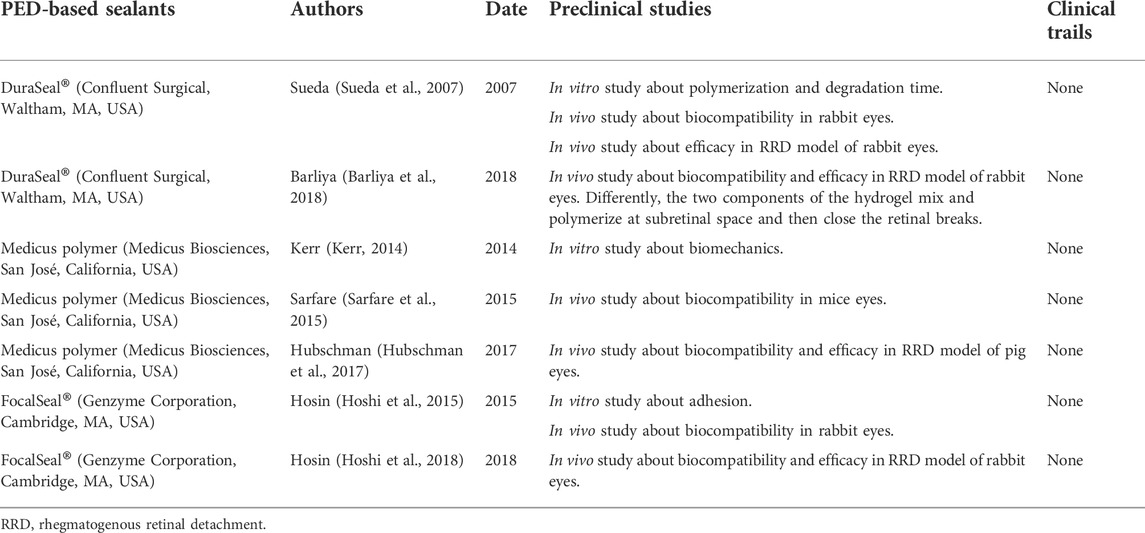
TABLE 4. The summaries of studies about PEG-based sealants as a retinal patch for the treatment of RRD.
Similar to the safety and efficacy reports of PEG hydrogel as a dural sealant in multicenter, single-blind, prospective randomized cranial surgery, (Osbun et al., 2012) it is believed that multicenter and large-scale clinical trials on PEG-based sealants as retinal patches will also be reported shortly.
Outlook
In the natural biological world, a range of species, such as mussels and barnacles, secrete substances that assist them in underwater attachment. The strong adhesion of mussels to a surface is based on the mussel adhesive protein (MAP), in which 3,4 dihydroxy-L-phenyalanine (DOPA) has been identified as the key factor that can penetrate water boundary layers and subsequently bond strongly with surfaces. (Zhang et al., 2020) The MAP model is well known for its promising adhesive performance to wet surfaces; therefore, mussel-inspired adhesives have been designed and tested in preclinical studies. (Lee et al., 2011; Rahimnejad and Zhong, 2017; Kianersi et al., 2020; Pandey et al., 2020) Now the research field is moving towards the use of nanoparticles to create next-generation mussel-inspired adhesives with increased adhesive strength and drug delivery for tissue regeneration. A low concentration of MAP is biocompatible with the retina. (Liggett et al., 1990) Moreover, magnetic nanoparticle bioadhesive compounds can effectively seal retinal breaks in rabbit eyes. (Ji et al., 2018) Although there has been a surge in research on mussel-inspired adhesives in recent years, none of these adhesives have been reported in clinical trials. However, they have received great interest from the research community. Therefore, mussel-inspired adhesives may be another potential option for retinal patches in the treatment of RRD.
Conclusion
The progress of technology has made biomaterials progress from simple original material to the current cross-linked or polymerized materials, and from single modification to the coexistence of multiple modifications. These advances have reduced the retinal damage caused by the toxicity of biomaterials, difficulties in delivery, as well as enhanced biocompatibility and efficacy, while simultaneously making significant contributions to the treatment alteration of RRD, quick recovery of vision, and avoidance of postoperative face-down position. Although research on biomaterials as retinal patches for RRD repair is in full swing, most are in the primary stage of research, and few materials have entered clinical applications. There is still a long way to go and carry further exploration before biomaterials become a standard procedure. The challenges in the translation to clinical studies are summarized as follows. First, in experimental eyes with RRD, small round retinal breaks are usually generated at the inferior region, resulting in a localized retinal detachment that lasts only a few seconds. Conversely, the location and morphology of retinal breaks in patients with RRD remain uncertain. Importantly, retinal detachment lasts significantly longer and has more microenvironmental alterations; hence, experimental RRD could not fully imitate the pathological processes of clinical RRD. Therefore, some patients and ophthalmologists are disinclined to undergo initial trials based on the preclinical outcomes. Second, the precise application of sealants over retinal breaks is challenging. Visualization through air is difficult for transparent sealants, such as the clinically used Healaflow. In addition, there is a risk of sealant migration to the subretinal space owing to fluidity, such as the two solution components of fibrin glue. Additionally, if there are remaining retinal folds across which the sealant flows, it might result in retino-retinal adhesions. For superior retinal breaks, it is difficult to attach sealants successfully because of the spherical shape of the eyeball and the effect of gravity; especially the sealant requires a few seconds to gel, such as the clinically used fibrin glue, whose two components need to be applied one by one. Therefore, a learning curve exists. Third, the indication of biomaterials as retinal patches overlaps with that of SB; therefore, some patients and ophthalmologists prefer SB with a long history and no difference in the success rate with PPV, which would limit the application and dissemination of biomaterials as retinal patches. Finally, the cost of sealants must be considered in terms of the surgical cost. All of these factors contribute to the fact that, despite encouraging results, some sealants are currently only available for preclinical testing, or that, while the remaining sealants have undergone clinical trials, all clinical studies are on small scales, and the condition of RRD patients is simple. To date, whether these biomaterials as retinal patches can completely replace long-lasting vitreous substitutions in cases of complex RRD has not been reported. Therefore, more randomized, prospective, large-scale clinical trials of biomaterials as retinal patches need to be conducted to definitively determine their biocompatibility, efficacy, and potential for complete replacement of long-lasting vitreous substitutes in RRD patients.
Author contributions
XR and XL contributed to conception and design of the study. CZ wrote the manuscript. KX draw figures and polished English statement. XZ and DW edited the manuscript. All authors contributed to manuscript revision, read, and approved the submitted version.
Funding
This work was supported by the grants from the Fund for Less Developed Regions of the National Natural Science Foundation of China (82160202).
Conflict of interest
The authors declare that the research was conducted in the absence of any commercial or financial relationships that could be construed as a potential conflict of interest.
Publisher’s note
All claims expressed in this article are solely those of the authors and do not necessarily represent those of their affiliated organizations, or those of the publisher, the editors and the reviewers. Any product that may be evaluated in this article, or claim that may be made by its manufacturer, is not guaranteed or endorsed by the publisher.
References
Almeida, D. R. P., Chin, E. K., Arjmand, P., Velez, G., Evans, L. P., and Mahajan, V. B. (2018). Fibrin glue and internal limiting membrane abrasion for optic disc pit maculopathy. Ophthalmic Surg. Lasers Imaging Retina 49 (12), e271–e277. doi:10.3928/23258160-20181203-18
Arora, B., Tandon, R., Attri, P., and Bhatia, R. (2017). Chemical crosslinking: Role in protein and peptide science. Curr. Protein Pept. Sci. 18 (9), 946–955. doi:10.2174/1389203717666160724202806
Assmann, A., Vegh, A., Ghasemi-Rad, M., Bagherifard, S., Cheng, G., Sani, E. S., et al. (2017). A highly adhesive and naturally derived sealant. Biomaterials 140, 115–127. doi:10.1016/j.biomaterials.2017.06.004
Aydin, E., Eris, E., Kazanci, L., and Uyar, O. M. (2021). Reattachment of rhegmatogenous retinal detachment via fibrin tissue adhesive. Korean J. Ophthalmol. 35 (3), 173–178. doi:10.3341/kjo.2020.0020
Backstrom, J. R., and Tokes, Z. A. (1995). The 84-kDa form of human matrix metalloproteinase-9 degrades substance P and gelatin. J. Neurochem. 64 (3), 1312–1318. doi:10.1046/j.1471-4159.1995.64031312.x
Barliya, T., Sandalon, S., Ofri, R., Livnat, T., and Weinberger, D. (2018). Transcleral approach for closing retinal tears using DuraSeal hydrogel sealant. Indian J. Ophthalmol. 66 (2), 238–243. doi:10.4103/ijo.ijo_758_17
Barth, H., Crafoord, S., O'Shea, T. M., Pritchard, C. D., Langer, R., and Ghosh, F. (2014). A new model for in vitro testing of vitreous substitute candidates. Graefes Arch. Clin. Exp. Ophthalmol. 252 (10), 1581–1592. doi:10.1007/s00417-014-2714-3
Barth, H., Crafoord, S., Andreasson, S., and Ghosh, F. (2016). A cross-linked hyaluronic acid hydrogel (Healaflow®) as a novel vitreous substitute. Graefes Arch. Clin. Exp. Ophthalmol. 254 (4), 697–703. doi:10.1007/s00417-015-3256-z
Barth, H., Crafoord, S., Arner, K., and Ghosh, F. (2019). Inflammatory responses after vitrectomy with vitreous substitutes in a rabbit model. Graefes Arch. Clin. Exp. Ophthalmol. 257 (4), 769–783. doi:10.1007/s00417-019-04242-0
Bettin, P., Di Matteo, F., Rabiolo, A., Fiori, M., Ciampi, C., and Bandello, F. (2016). Deep sclerectomy with mitomycin C and injectable cross-linked hyaluronic acid implant: Long-term results. J. Glaucoma 25 (6), e625–e629. doi:10.1097/ijg.0000000000000309
Bissen-Miyajima, H. (2008). Ophthalmic viscosurgical devices. Curr. Opin. Ophthalmol. 19 (1), 50–54. doi:10.1097/icu.0b013e3282f14db0
Borkenstein, A. F., Borkenstein, E. M., and Malyugin, B. (2021). Ophthalmic viscosurgical devices (OVDs) in challenging cases: a review. Ophthalmol. Ther. 10 (4), 831–843. doi:10.1007/s40123-021-00403-9
Bourla, D. H., Bor, E., Axer-Siegel, R., Mimouni, K., and Weinberger, D. (2010). Outcomes and complications of rhegmatogenous retinal detachment repair with selective sutureless 25-gauge pars plana vitrectomy. Am. J. Ophthalmol. 149 (4), 630–634. doi:10.1016/j.ajo.2009.11.003
Chen, T., Janjua, R., McDermott, M. K., Bernstein, S. L., Steidl, S. M., and Payne, G. F. (2006). Gelatin-based biomimetic tissue adhesive. Potential for retinal reattachment. J. Biomed. Mat. Res. 77 (2), 416–422. doi:10.1002/jbm.b.30439
Chen, X., Yan, Y., Hong, L., and Zhu, L. (2015). A comparison of strict face-down positioning with adjustable positioning after pars plana vitrectomy and gas tamponade for rhegmatogenous retinal detachment. Retina 35 (5), 892–898. doi:10.1097/iae.0000000000000413
Chen, J., Wang, C., Wen, W., Ni, J., Jiang, J., Ge, Z., et al. (2021). Feasibility studies of intraocular use of gelatin methacryloyl hydrogel to patch retinal tears. Polym. Adv. Technol. 32 (8), 3160–3166. doi:10.1002/pat.5328
Chronopoulos, A., Hattenbach, L. O., and Schutz, J. S. (2021). Pneumatic retinopexy: A critical reappraisal. Surv. Ophthalmol. 66 (4), 585–593. doi:10.1016/j.survophthal.2020.12.007
De Boulle, K., Glogau, R., Kono, T., Nathan, M., Tezel, A., Roca-Martinez, J. X., et al. (2013). A review of the metabolism of 1, 4-butanediol diglycidyl ether-crosslinked hyaluronic acid dermal fillers. Dermatol. Surg. 39 (12), 1758–1766. doi:10.1111/dsu.12301
de Oliveira, P. R. C., Berger, A. R., and Chow, D. R. (2017). Use of evicel fibrin sealant in optic disc pit-associated macular detachment. Ophthalmic Surg. Lasers Imaging Retina 48 (4), 358–363. doi:10.3928/23258160-20170329-13
Diamond, M. P., Burns, E. L., Accomando, B., Mian, S., and Holmdahl, L. (2012). Seprafilm® adhesion barrier: (1) a review of preclinical, animal, and human investigational studies. Gynecol. Surg. 9 (3), 237–245. doi:10.1007/s10397-012-0741-9
Diamond, M. P., Burns, E. L., Accomando, B., Mian, S., and Holmdahl, L. (2012). Seprafilm® adhesion barrier: (2) a review of the clinical literature on intraabdominal use. Gynecol. Surg. 9 (3), 247–257. doi:10.1007/s10397-012-0742-8
Ding, Y. W., Wang, Z. Y., Ren, Z. W., Zhang, X. W., and Wei, D. X. (2022). Advances in modified hyaluronic acid-based hydrogels for skin wound healing. Biomater. Sci. 10 (13), 3393–3409. doi:10.1039/d2bm00397j
Echave, M. C., Saenz del Burgo, L., Pedraz, J. L., and Orive, G. (2017). Gelatin as biomaterial for tissue engineering. Curr. Pharm. Des. 23 (24), 3567–3584. doi:10.2174/0929867324666170511123101
Elzoghby, A. O. (2013). Gelatin-based nanoparticles as drug and gene delivery systems: reviewing three decades of research. J. Control. Release 172 (3), 1075–1091. doi:10.1016/j.jconrel.2013.09.019
Esterbauer, H., Schaur, R. J., and Zollner, H. (1991). Chemistry and biochemistry of 4-hydroxynonenal, malonaldehyde and related aldehydes. Free Radic. Biol. Med. 11 (1), 81–128. doi:10.1016/0891-5849(91)90192-6
Feltgen, N., and Walter, P. (2014). Rhegmatogenous retinal detachment-an ophthalmologic emergency. Dtsch. Arztebl. Int. 111 (1-2), 12–21. doi:10.3238/arztebl.2014.0012
Gasek, N., Park, H. E., Uriarte, J. J., Uhl, F. E., Pouliot, R. A., Riveron, A., et al. (2021). Development of alginate and gelatin-based pleural and tracheal sealants. Acta Biomater. 131, 222–235. doi:10.1016/j.actbio.2021.06.048
Guhan, S., Peng, S. L., Janbatian, H., Saadeh, S., Greenstein, S., Al Bahrani, F., et al. (2018). Surgical adhesives in ophthalmology: history and current trends. Br. J. Ophthalmol. 102 (10), 1328–1335. doi:10.1136/bjophthalmol-2017-311643
Guo, Y., Zhu, Q., Chen, S., Li, Y., Fu, D., Qiao, D., et al. (2021). Effect of sodium hyaluronate-arboxycellulose membrane (Seprafilm®) on postoperative small bowel obstruction: A meta-analysis. Surgery 169 (6), 1333–1339. doi:10.1016/j.surg.2020.12.004
Haruta, M., Arai, M., Sueda, J., Hirose, T., and Yamakawa, R. (2017). Patching retinal breaks with Seprafilm for treating retinal detachments in humans: 9 years of follow-up. Eye 31 (5), 776–780. doi:10.1038/eye.2016.329
Haugstad, M., Moosmayer, S., and Bragadomicronttir, R. (2017). Primary rhegmatogenous retinal detachment - surgical methods and anatomical outcome. Acta Ophthalmol. 95 (3), 247–251. doi:10.1111/aos.13295
Heher, P., Ferguson, J., Redl, H., and Slezak, P. (2018). An overview of surgical sealant devices: current approaches and future trends. Expert Rev. Med. Devices 15 (10), 747–755. doi:10.1080/17434440.2018.1526672
Hida, T., Sheta, S. M., Proia, A. D., and McCuen, B. W. (1988). Retinal toxicity of cyanoacrylate tissue adhesive in the rabbit. Retina 8 (2), 148–153. doi:10.1097/00006982-198808020-00013
Hirata, A., Yamamoto, S., and Okinami, S. (2013). Use of an ophthalmic viscosurgical device for experimental retinal detachment in rabbit eyes. J. Funct. Biomater. 4 (1), 6–13. doi:10.3390/jfb4010006
Hoshi, S., Okamoto, F., Arai, M., Hirose, T., Sugiura, Y., Kaji, Y., et al. (2015). In vivo and in vitro feasibility studies of intraocular use of polyethylene glycol–based synthetic sealant to close retinal breaks in porcine and rabbit eyes. Invest. Ophthalmol. Vis. Sci. 56 (8), 4705–4711. doi:10.1167/iovs.14-15349
Hoshi, S., Okamoto, F., Arai, M., Hirose, T., Fukuda, S., Sugiura, Y., et al. (2016). Polyethylene glycol-based synthetic hydrogel sealant for closing vitrectomy wounds: An in vivo and histological study. Transl. Vis. Sci. Technol. 5 (3), 7. doi:10.1167/tvst.5.3.7
Hoshi, S., Okamoto, F., Arai, M., Hirose, T., Sugiura, Y., Murakami, T., et al. (2018). Patching retinal breaks with polyethylene glycol-based synthetic hydrogel sealant for retinal detachment in rabbits. Exp. Eye Res. 177, 117–121. doi:10.1016/j.exer.2018.08.004
Hoshi, S., Okamoto, F., Murakami, T., Sakai, T., Shinohara, Y., Fujii, T., et al. (2019). Ability of nonswelling polyethylene glycol-based vitreous hydrogel to maintain transparency in the presence of vitreous hemorrhage. Transl. Vis. Sci. Technol. 8 (6), 33. doi:10.1167/tvst.8.6.33
Hubschman, J. P., Govetto, A., Farajzadeh, M., Sato, T., Askari, S., and Glasgow, B. (2017). Feasibility of a polyethylene glycol-derived polymer as retinal patch to seal retinal breaks during vitrectomy for rhegmatogenous retinal detachment: a prospective, in vivo pilot study in a porcine model. Clin. Exp. Ophthalmol. 45 (7), 708–716. doi:10.1111/ceo.12942
Jain, R., and Wairkar, S. (2019). Recent developments and clinical applications of surgical glues: An overview. Int. J. Biol. Macromol. 137, 95–106. doi:10.1016/j.ijbiomac.2019.06.208
Jeon, O., Song, S. J., Lee, K-J., Park, M. H., Lee, S-H., Hahn, S. K., et al. (2007). Mechanical properties and degradation behaviors of hyaluronic acid hydrogels cross-linked at various cross-linking densities. Carbohydr. Polym. 70 (3), 251–257. doi:10.1016/j.carbpol.2007.04.002
Ji, Y-S., Jang, J. Y., Yoon, K. C., and Park, S. W. (2018). Feasibility of using magnetic nanoparticle-bioadhesive compound to repair experimental retinal detachment. Investigative Ophthalmol. Vis. Sci. 59 (9), 4228. https://iovs.arvojournals.org/article.aspx?articleid=2691889
Kang, J. I., and Park, K. M. (2021). Advances in gelatin-based hydrogels for wound management. J. Mat. Chem. B 9 (6), 1503–1520. doi:10.1039/d0tb02582h
Kawamura, M., Gika, M., Izumi, Y., Horinouchi, H., Shinya, N., Mukai, M., et al. (2005). The sealing effect of fibrin glue against alveolar air leakage evaluated up to 48 h; comparison between different methods of application. Eur. J. Cardio-Thoracic Surg. 28 (1), 39–42. doi:10.1016/j.ejcts.2005.02.044
Kerr, M. (2014) Biomechanical testing of an adhesive polymer intended for the treatment of retinal detachment 2014. California: UCLA. ProQuest ID: Kerr_ucla_0031N_12664.
Kianersi, S., Varjani, A. A. A., Solouk, A., Ai, J., and Lee, B. P. (2020). Mussel-inspired polydopamine-coated silk fibroin as a promising biomaterial. Bioinspired, Biomim. Nanobiomaterials 9 (3), 147–154. doi:10.1680/jbibn.19.00045
Klotz, B. J., Gawlitta, D., Rosenberg, A., Malda, J., and Melchels, F. P. W. (2016). Gelatin-methacryloyl hydrogels: Towards biofabrication-based tissue repair. Trends Biotechnol. 34 (5), 394–407. doi:10.1016/j.tibtech.2016.01.002
Kobashi, H., Takano, M., Yanagita, T., Shiratani, T., Wang, G., Hoshi, K., et al. (2014). Scleral buckling and pars plana vitrectomy for rhegmatogenous retinal detachment: an analysis of 542 eyes. Curr. Eye Res. 39 (2), 204–211. doi:10.3109/02713683.2013.838270
Kontos, A., Tee, J., Stuart, A., Shalchi, Z., and Williamson, T. H. (2017). Duration of intraocular gases following vitreoretinal surgery. Graefes Arch. Clin. Exp. Ophthalmol. 255 (2), 231–236. doi:10.1007/s00417-016-3438-3
Kuhn, F., and Aylward, B. (2014). Rhegmatogenous retinal detachment: a reappraisal of its pathophysiology and treatment. Ophthalmic Res. 51 (1), 15–31. doi:10.1159/000355077
Kunikata, H., Abe, T., and Nakazawa, T. (2019). Historical, current and future approaches to surgery for rhegmatogenous retinal detachment. Tohoku J. Exp. Med. 248 (3), 159–168. doi:10.1620/tjem.248.159
Lai, J. Y. (2014). Relationship between structure and cytocompatibility of divinyl sulfone cross-linked hyaluronic acid. Carbohydr. Polym. 101, 203–212. doi:10.1016/j.carbpol.2013.09.060
Lee, B. P., Messersmith, P. B., Israelachvili, J. N., and Waite, J. H. (2011). Mussel-inspired adhesives and coatings. Annu. Rev. Mat. Res. 41, 99–132. doi:10.1146/annurev-matsci-062910-100429
Liggett, P. E., Cano, M., Robin, J. B., Green, R. L., and Lean, J. S. (1990). Intravitreal biocompatibility of mussel adhesive protein. A preliminary study. Retina 10 (2), 144–147. doi:10.1097/00006982-199004000-00011
Liu, Y., Kopelman, D., Wu, L. Q., Hijji, K., Attar, I., Preiss-Bloom, O., et al. (2009). Biomimetic sealant based on gelatin and microbial transglutaminase: an initial in vivo investigation. J. Biomed. Mat. Res. 91 (1), 5–16. doi:10.1002/jbm.b.31368
Lu, L., Yuan, S., Wang, J., Shen, Y., Deng, S., Xie, L., et al. (2018). the formation mechanism of hydrogels. Curr. Stem Cell Res. Ther. 13 (7), 490–496. doi:10.2174/1574888x12666170612102706
Lumi, X., Hawlina, M., Glavac, D., Facsko, A., Moe, M. C., Kaarniranta, K., et al. (2015). Ageing of the vitreous: From acute onset floaters and flashes to retinal detachment. Ageing Res. Rev. 21, 71–77. doi:10.1016/j.arr.2015.03.006
McDermott, M. K., Chen, T., Williams, C. M., Markley, K. M., and Payne, G. F. (2004). Mechanical properties of biomimetic tissue adhesive based on the microbial transglutaminase-catalyzed crosslinking of gelatin. Biomacromolecules 5 (4), 1270–1279. doi:10.1021/bm034529a
Mehdizadeh, M., and Yang, J. (2013). Design strategies and applications of tissue bioadhesives. Macromol. Biosci. 13 (3), 271–288. doi:10.1002/mabi.201200332
Minihan, M., Tanner, V., and Williamson, T. H. (2001). Primary rhegmatogenous retinal detachment: 20 years of change. Br. J. Ophthalmol. 85 (5), 546–548. doi:10.1136/bjo.85.5.546
Mudhol, R., and Bansal, R. (2021). Cross-linked hyaluronic acid viscoelastic scleral implant in trabeculectomy. Indian J. Ophthalmol. 69 (5), 1135–1141. doi:10.4103/ijo.ijo_2462_20
Nagata, T., Harada, Y., Arai, M., Hirose, T., and Kondo, H. (2020). Polyethylene glycol-based synthetic hydrogel sealant for filtration bleb leaks: An in vivo and histologic study. Transl. Vis. Sci. Technol. 9 (6), 24. doi:10.1167/tvst.9.6.24
Osbun, J. W., Ellenbogen, R. G., Chesnut, R. M., Chin, L. S., Connolly, P. J., Cosgrove, G. R., et al. (2012). A multicenter, single-blind, prospective randomized trial to evaluate the safety of a polyethylene glycol hydrogel (Duraseal Dural Sealant System) as a dural sealant in cranial surgery. World Neurosurg. x. 78 (5), 498–504. doi:10.1016/j.wneu.2011.12.011
Oshima, Y., Wakabayashi, T., Sato, T., Ohji, M., and Tano, Y. (2010). A 27-gauge instrument system for transconjunctival sutureless microincision vitrectomy surgery. Ophthalmology 117 (1), 93–102. doi:10.1016/j.ophtha.2009.06.043
Pandey, N., Soto-Garcia, L. F., Liao, J., Philippe, Z., Nguyen, K. T., and Hong, Y. (2020). Mussel-inspired bioadhesives in healthcare: design parameters, current trends, and future perspectives. Biomater. Sci. 8 (5), 1240–1255. doi:10.1039/c9bm01848d
Pastor, J. C., Rojas, J., Pastor-Idoate, S., Di Lauro, S., Gonzalez-Buendia, L., and Delgado-Tirado, S. (2016). Proliferative vitreoretinopathy: A new concept of disease pathogenesis and practical consequences. Prog. Retin. Eye Res. 51, 125–155. doi:10.1016/j.preteyeres.2015.07.005
Perez, L. A., Hernandez, R., Alonso, J. M., Perez-Gonzalez, R., and Saez-Martinez, V. (2021). Hyaluronic acid hydrogels crosslinked in physiological conditions: Synthesis and biomedical applications. Biomedicines 9 (9), 1113. doi:10.3390/biomedicines9091113
Pfister, R. R., and Sommers, C. I. (2005). Fibrin sealant in corneal stem cell transplantation. Cornea 24 (5), 593–598. doi:10.1097/01.ico.0000157402.89032.ad
Popovic, M. M., Muni, R. H., Nichani, P., and Kertes, P. J. (2022). Pars plana vitrectomy, scleral buckle, and pneumatic retinopexy for the management of rhegmatogenous retinal detachment: a meta-analysis. Surv. Ophthalmol. 67 (1), 184–196. doi:10.1016/j.survophthal.2021.05.008
Poudel, H., Sanford, K., Szwedo, P. K., Pathak, R., and Ghosh, A. (2022). Synthetic matrices for intestinal organoid culture: Implications for better performance. ACS Omega 7 (1), 38–47. doi:10.1021/acsomega.1c05136
Raczynska, D., Mitrosz, K., Raczynska, K., and Glasner, L. (2018). The influence of silicone oil on the ganglion cell complex after pars plana vitrectomy for rhegmatogenous retinal detachment. Curr. Pharm. Des. 24 (29), 3476–3493. doi:10.2174/1381612824666180813115438
Rahimnejad, M., and Zhong, W. (2017). Mussel-inspired hydrogel tissue adhesives for wound closure. RSC Adv. 7 (75), 47380–47396. doi:10.1039/c7ra06743g
Ren, X. J., Bu, S. C., Wu, D., Liu, B. S., Yang, F. H., Hu, B. J., et al. (2020). Patching retinal breaks with Healaflow in 27-gauge vitrectomy for the treatment of rhegmatogenous retinal detachment. Retina 40 (10), 1900–1908. doi:10.1097/iae.0000000000002701
Romano, V., Cruciani, M., Conti, L., and Fontana, L. (2016). Fibrin glue versus sutures for conjunctival autografting in primary pterygium surgery. Cochrane Database Syst. Rev. 12, CD011308. doi:10.1002/14651858.CD011308.pub2
Romano, M. R., Cennamo, G., Ferrara, M., Cennamo, M., and Cennamo, G. (2017). Twenty-seven-gauge versus 25-gauge vitrectomy for primary rhegmatogenous retinal detachment. Retina 37 (4), 637–642. doi:10.1097/iae.0000000000001215
Sarfare, S., Dacquay, Y., Askari, S., Nusinowitz, S., and Hubschman, J. P. (2015). Biocompatibility of a synthetic biopolymer for the treatment of rhegmatogenous retinal detachment. J. Clin. Exp. Ophthalmol. 6 (5), 475. doi:10.4172/2155-9570.1000475
Scalcione, C., Ortiz-Vaquerizas, D., Said, D. G., and Dua, H. S. (2018). Fibrin glue as agent for sealing corneal and conjunctival wound leaks. Eye 32 (2), 463–466. doi:10.1038/eye.2017.227
Scognamiglio, F., Travan, A., Rustighi, I., Tarchi, P., Palmisano, S., Marsich, E., et al. (2016). Adhesive and sealant interfaces for general surgery applications. J. Biomed. Mat. Res. 104 (3), 626–639. doi:10.1002/jbm.b.33409
Sonmez, B., and Beden, U. (2011). Fibrin glue-assisted sutureless limbal stem cell transplantation surgery for the treatment of severe ocular chemical injury. Cornea 30 (3), 296–300. doi:10.1097/ico.0b013e3181eeb2af
Srinivasan, S., Dollin, M., McAllum, P., Berger, Y., Rootman, D. S., and Slomovic, A. R. (2009). Fibrin glue versus sutures for attaching the conjunctival autograft in pterygium surgery: a prospective observer masked clinical trial. Br. J. Ophthalmol. 93 (2), 215–218. doi:10.1136/bjo.2008.145516
Sueda, J., Sakuma, T., Nakamura, H., Usumoto, N., Okuno, T., Arai, M., et al. (2006). In vivo and in vitro feasibility studies of intraocular use of Seprafilm to close retinal breaks in bovine and rabbit eyes. Invest. Ophthalmol. Vis. Sci. 47 (3), 1142–1148. doi:10.1167/iovs.05-0931
Sueda, J., Fukuchi, T., Usumoto, N., Okuno, T., Arai, M., and Hirose, T. (2007). Intraocular use of hydrogel tissue adhesive in rabbit eyes. Jpn. J. Ophthalmol. 51 (2), 89–95. doi:10.1007/s10384-006-0405-2
Teruya, K., Sueda, J., Arai, M., Tsurumaru, N., Yamakawa, R., Hirata, A., et al. (2009). Patching retinal breaks with Seprafilm in experimental rhegmatogenous retinal detachment of rabbit eyes. Eye 23 (12), 2256–2259. doi:10.1038/eye.2008.403
Tetsumoto, A., Imai, H., Hayashida, M., Otsuka, K., Matsumiya, W., Miki, A., et al. (2020). The comparison of the surgical outcome of 27-gauge pars plana vitrectomy for primary rhegmatogenous retinal detachment between air and SF6 gas tamponade. Eye 34 (2), 299–306. doi:10.1038/s41433-019-0726-2
Tram, N. K., and Swindle-Reilly, K. E. (2018). Rheological properties and age-related changes of the human vitreous humor. Front. Bioeng. Biotechnol. 6, 199. doi:10.3389/fbioe.2018.00199
Tsurumaru, N., Arai, M., Teruya, K., Sueda, J., and Yamakawa, R. (2009). Seprafilm as a new antifibrotic agent following trabeculectomy in rabbit eyes. Jpn. J. Ophthalmol. 53 (2), 164–170. doi:10.1007/s10384-008-0638-3
Tyagi, M., and Basu, S. (2019). Glue-assisted retinopexy for rhegmatogenous retinal detachments (GuARD): A novel surgical technique for closing retinal breaks. Indian J. Ophthalmol. 67 (5), 677–680. doi:10.4103/ijo.ijo_1943_18
Wagenfeld, L., Zeitz, O., Skevas, C., and Richard, G. (2010). Long-lasting endotamponades in vitreoretinal surgery. Ophthalmologica 224 (5), 291–300. doi:10.1159/000298749
Wang, A., and Snead, M. P. (2020). Scleral buckling-a brief historical overview and current indications. Graefes Arch. Clin. Exp. Ophthalmol. 258 (3), 467–478. doi:10.1007/s00417-019-04562-1
Wang, Q., Zhao, J., Xu, Q., Han, C., and Hou, B. (2020). Intraocular application of fibrin glue as an adjunct to pars plana vitrectomy for rhegmatogenous retinal detachment. Retina 40 (4), 718–724. doi:10.1097/iae.0000000000002584
Wu, L., Liu, J., Chang, X., and Zheng, Y. (2021). The therapeutic effect of Healaflow in glaucoma surgery. Am. J. Transl. Res. 13 (8), 9729–9735.
Yamamoto, S., Hirata, A., Ishikawa, S., Ohta, K., Nakamura, K., and Okinami, S. (2013). Feasibility of using gelatin-microbial transglutaminase complex to repair experimental retinal detachment in rabbit eyes. Graefes Arch. Clin. Exp. Ophthalmol. 251 (4), 1109–1114. doi:10.1007/s00417-012-2245-8
Yang, M. B., Melia, M., Lambert, S. R., Chiang, M. F., Simpson, J. L., and Buffenn, A. N. (2013). Fibrin glue for closure of conjunctival incision in strabismus surgery: a report by the American academy of ophthalmology. Ophthalmology 120 (9), 1935–1941. doi:10.1016/j.ophtha.2013.05.008
Yorston, D., Donachie, P. H. J., Laidlaw, D. A., Steel, D. H., Sparrow, J. M., Aylward, G. W., et al. (2021). Factors affecting visual recovery after successful repair of macula-off retinal detachments: findings from a large prospective UK cohort study. Eye 35 (5), 1431–1439. doi:10.1038/s41433-020-1021-y
Yue, K., Trujillo-de Santiago, G., Alvarez, M. M., Tamayol, A., Annabi, N., and Khademhosseini, A. (2015). Synthesis, properties, and biomedical applications of gelatin methacryloyl (GelMA) hydrogels. Biomaterials 73, 254–271. doi:10.1016/j.biomaterials.2015.08.045
Zhang, W., Wang, R., Sun, Z., Zhu, X., Zhao, Q., Zhang, T., et al. (2020). Catechol-functionalized hydrogels: biomimetic design, adhesion mechanism, and biomedical applications. Chem. Soc. Rev. 49 (2), 433–464. doi:10.1039/c9cs00285e
Zheng, C., Xi, H., Wen, D., Ke, Y., Zhang, X., Ren, X., et al. (2022). Biocompatibility and efficacy of a linearly cross-linked sodium hyaluronic acid hydrogel as a retinal patch in rhegmatogenous retinal detachment repairment. Front. Bioeng. Biotechnol. 10, 914675. doi:10.3389/fbioe.2022.914675
Zmora, O., Mizrahi, N., Rotholtz, N., Pikarsky, A. J., Weiss, E. G., Nogueras, J. J., et al. (2003). Fibrin glue sealing in the treatment of perineal fistulas. Dis. Colon Rectum 46 (5), 584–589. doi:10.1007/s10350-004-6612-3
Keywords: rhegmatogenous retinal detachment, biomaterials, biocompatibility, categories, synthetic material, polyethylene glycol
Citation: Zheng C, Wen D, Xu K, Zhang X, Ren X and Li X (2022) Advances in biomaterials as a retinal patch for the repair of rhegmatogenous retinal detachment. Front. Bioeng. Biotechnol. 10:997243. doi: 10.3389/fbioe.2022.997243
Received: 18 July 2022; Accepted: 04 October 2022;
Published: 17 October 2022.
Edited by:
Xiaoyuan Li, Northeast Normal University, ChinaReviewed by:
Peiquan Zhao, Shanghai Jiao Tong University, ChinaFengyuan Zhao, Peking University Third Hospital, China
Nikhil Pandey, University of Maryland, United States
Haijie Han, Zhejiang University, China
Edmund Wong, Singapore National Eye Center, Singapore
Copyright © 2022 Zheng, Wen, Xu, Zhang, Ren and Li. This is an open-access article distributed under the terms of the Creative Commons Attribution License (CC BY). The use, distribution or reproduction in other forums is permitted, provided the original author(s) and the copyright owner(s) are credited and that the original publication in this journal is cited, in accordance with accepted academic practice. No use, distribution or reproduction is permitted which does not comply with these terms.
*Correspondence: Xinjun Ren, zlrxjrsy@126.com; Xiaorong Li, xiaorli@163.com