- 1Department of Forest Biomaterials Engineering, College of Forest and Environmental Sciences, Kangwon National University, Kangwon, South Korea
- 2Key Lab of Agricultural Resources and Ecology of Poyang Lake Basin, College of Land Resources and Environment, Jiangxi Agricultural University, Nanchang, Jiangxi, China
- 3Jiangxi Academy of Forestry, Nanchang, Jiangxi, China
- 4Tianjin Key Laboratory of Pulp and Paper, Tianjin University of Science and Technology, Tianjin, China
- 5Department of Emergency Medicine and BioMedical Science Graduate Program (BMSGP), Chonnam National University, Hwasun, South Korea
- 6Department of Emergency Medical Rescue, Nambu University, Gwangju, South Korea
Flavonoid aglycones possess biological activities, such as antioxidant and antidiabetic activities compared to glycosides. Taxifolin, a flavonoid aglycones, is detected only in trace amounts in nature and is not easily observed. Therefore, in this study, to investigate the hair tonic and hair loss inhibitors effect of taxifolin, high content of taxifolin aglycone extract was prepared by enzymatic hydrolysis. Taxifolin effectively regulates the apoptosis of dermal papilla cells, which is associated with hair loss, based on its strong antioxidant activities. However, inhibition of dihydrotestosterone (DHT), which is a major cause of male pattern hair loss, was significantly reduced with taxifolin treatment compared with minoxidil, as a positive control. It was also confirmed that a representative factor for promoting hair growth, IGF-1, was significantly increased, and that TGF-β1, a representative biomarker for hair loss, was significantly reduced with taxifolin treatment. These results suggest that taxifolin from enzymatic hydrolysis of RM is a potential treatment for hair loss and a hair growth enhancer.
Introduction
Rhododendron mucronulatum (RM) is a deciduous shrub with a height of approximately 2 m and is distributed in some parts of China and Japan and all over Korea (Chung and Lee, 1991). In Korea, petals are commonly used in food, such as in making flower-patterned griddle cakes. In addition, its roots have been used extensively in oriental traditional medicine as remedies for fever, dysuria, and tonic in Korea and China (Lee et al., 2007; Choi et al., 2011; Si et al., 2011; Si et al., 2013a, 2013b). Flavonoids, which are phenolic compounds (Si et al., 2008a; Si et al., 2008b; Si et al., 2009a; Si et al., 2009c), have been found in the RM flowers, leaves, and stems. The phenolic compounds of RM roots have outstanding antioxidant, anti-inflammatory effects and inhibit allergic inflammation (Lee et al., 2005; Lee et al., 2007; Cho et al., 2008; Choi et al., 2011; Jang et al., 2022).
Taxifolin aglycone has been reported to have strong anti-inflammatory activities in the exudative and proliferative phases of inflammation in albino rats (Gupta et al., 1971). In addition, it has been proven to be effective in diabetic cardiomyopathy by inhibiting oxidative stress to regulate mitochondrial function and inhibit cell apoptosis (Sun et al., 2014; Zheng et al., 2021). Moreover, taxifolin exerts cardioprotective effects against diazinon-induced myocardial injury in rats (Najeb et al., 2022). However, it has been reported that taxifolin aglycone is found in complex preparations such as silymarin (LegalonTM), is presented in very small amounts, and is rarely used alone (Kroll et al., 2007; Weidmann, 2012).
Many researchers use hydrolysis to change the structure of a compound (Xie et al., 2018; Xie et al., 2019; Du et al., 2019; Du et al., 2021a; Lu et al., 2019; Yang et al., 2019; Wang et al., 2021; Wang et al., 2020; Liu et al., 2020a; Xu et al., 2020b; Xu et al., 2020c; Liu et al., 2021a; Liu et al., 2021b; Liu et al., 2022a; Xiong et al., 2021a; Xiong et al., 2021b; Liu et al., 2021e; Liu et al., 2021f; Ma et al., 2021; Zhang et al., 2021). For instance, acid hydrolysis and enzymatic hydrolysis are used to separate sugars (El-Zawawy et al., 2011; Jang et al., 2012; Chen et al., 2016; Dai et al., 2020; Dai et al., 2017; Liu et al., 2017b; An et al., 2020; An et al., 2019; Li et al., 2020; Li et al., 2019; Liu et al., 2020b; Du et al., 2021b; Du et al., 2022; Xu et al., 2021b; Xu et al., 2022; Liu et al., 2022b; Liu et al., 2022c; Liu et al., 2022d; Liu et al., 2022f; Li et al., 2022). Compared to acid hydrolysis, enzymatic hydrolysis is an environmentally friendly method, can be performed under mild reaction conditions, and does not require expensive corrosion protection equipment and neutralization after hydrolysis (Fan et al., 2012; Liu et al., 2017a; Liu et al., 2017b; Chen et al., 2020; Lu et al., 2020; Xu et al., 2020a; Xu et al., 2021a; Liu et al., 2021c, 2021d; Liu et al., 2022e). In a previous study on flavonoid compounds using enzymatic hydrolysis, it was reported that 90% of flavonoid glycosides could be converted into aglycone by hydrolyzing flavonoids and pectic oligosaccharides present in the bark of the bergamot (Mandalari et al., 2006). And the bio-activities could be improved by increasing the total soluble phenolic contents and the flavonoid aglycones content of guava leaves tea by enzymatic hydrolysis (Hu et al., 2017; Wang et al., 2018; Yu et al., 2021; Huang et al., 2021). In a previous study, we conducted a study on the treatment of atopic dermatitis using hirsutenonone which was obtained by enzymatic hydrolysis of oregonin (Jeong et al., 2010). Therefore, based on the success of enzymatic hydrolysis in previous studies, in this study, the effect of taxifolin, prepared by enzymatic hydrolysis, on hair loss, which is an intractable skin disease, was investigated.
Hair loss can be caused by various and complex factors such as aging, excessive stress, and genetic factors (Lei and Chuong, 2016). Various factors, such as insulin-like growth factors (IGF-1), B-cell lymphoma 2 (Bcl-2), Bcl-2-associated X protein (Bax), Poly ADP-ribose polymerase 1 (PARP), and Caspase-3 protein, are involved in cell apoptosis (Muller et al., 1994; Philpott et al., 1994; Zhao et al., 2001). To suppress the death of hair dermal papilla cells, it is necessary to understand how to effectively control these factors. In particular, testosterone, which is a type of androgen, is oxidized by 5α-reductase in the hair dermal papilla cells to produce DHT (Dihydrotestosterone). DHT is known to induce male pattern hair loss by inhibiting hair growth by reducing the growth phase of hair dermal papilla cells (Kaufman, 2002; Dhariwala and Ravikumar, 2019). Hair loss treatment targeting cell apoptosis includes minoxidil, a topical agent, and Propecia, an oral agent. However, these treatments are associated with side effects such as cardiac lesions, irritative dermatitis, and serious deformities (Herman et al., 1996; Nguyen et al., 2003; Hagemann et al., 2005).
Notably, among Asians, especially in Korea, hair loss is emerging as a serious social problem that is leading to stress and depression; hair loss lowers self-esteem and interferes with daily life (Kim et al., 2006; Lee and Lee, 2010). Therefore, it is necessary to develop safer treatments for hair loss using natural ingredients.
In this study, to develop hair tonic and hair loss inhibitors with no side effects, high content of taxifolin extract was prepared by enzymatic hydrolysis. In addition, oxidative stress was induced with H2O2 to investigate the oxidative stress effects on hair loss and the apoptosis control effect of the enzymatic hydrolysis of RM on dermal papilla cells. Further, we studied the effects of taxifolin separated and purified from enzymatic hydrolysis on the production of DHT, which is the direct cause of male pattern hair loss, hair growth-promoting factor IGF-1, and hair loss promoting factor TGF-β control effect.
Materials and methods
Preparation of enzymatic hydrolysis of RM
Rhododendron mucronulatum root (60 kg) from the Seoul Yangnyeongsi Medicine Market, Republic of Korea, was soaked in 60% EtOH at room temperature for 1 week and then filtered. The filtrate was concentrated and freeze-dried (4.964 kg, RM 60E). Then, 10 g of RM 60E was diluted in distilled water (150 ml, 95%, w/w) and Pectinex XXL, Ultra SP-L (Nobozymes Co. Ltd., Bagsvaerd, Denmark) (4 ml each, total 8 ml, 5%, w/w) were added. The mixture was shaken aerobically at 300 rpm for 24 h at 40°C, heated for 5 min at 85°C to inactivate the enzyme, and centrifuged for 10 min at 10,000 rpm and filtered. Then, the filtrate was fractionated with ethyl acetate, and the ethyl acetate layer extract was concentrated and freeze-dried to obtain 5.38 g.
Isolation of taxifolin by the enzymatic hydrolysis of RM
To isolate taxifolin (aglycone), Yamazen AI-580S system Medium Pressure Liquid Chromatography (Yamazen Corp., Osaka, Japan) was used. The ethyl acetate layer (4.5 g), and taxifolin (aglycone) were isolated. Silica gel column (30 µm, Yamazen Corp., Osaka, Japan) and ODS column (50 µm, Yamazen Corp., Osaka, Japan) were used. Silica gel column was used with CM solution (chloroform: methanol/5:1) and applied as an isocratic condition. After that, the isolating layer was concentrated and freeze-dried to a powder. Then powder (210 mg) was applied to the ODS column and eluted with distilled water. MW solution (methanol: water) was applied as an gradient condition (30%→35% MeOH; 10 min, 35%→45% MeOH; 20 min, 45%→50% MeOH; 20 min, 50%→0% MeOH; 15 min, 0% MeOH; 5 min). These conditions were repeated to obtain a final 103 mg of single taxifolin. A flowchart of taxifolin isolation is presented in Figure 1.
Chemical separation and identification
Thin layer chromatography (TLC) was carried out using a pre-coated silica gel 60 F254 plate (Merck, Darmstadt, Germany) with chloroform and methanol (5:1, v/v) and chloroform, methanol and water (70:30:4, v/v). The spots were detected under UV radiation (254 nm) and by spraying with
DPPH radical scavenging activity
DPPH radical scavenging assay was measured by modifying the method of Hatano et al. (1989). After preparing the DPPH (Sigma-Aldrich, United States) reagent in 0.1 mM DPPH solution (99.5% methanol, Daejung Chemical & Metals CO., LTD., Gyeonggi, Korea), the reaction ratio between the sample prepared in a varying concentrations in a 96 well plate (NUNC, United States), the sample and DPPH reaction ratio were performed to 1:9 (v/v), The reaction was then performed in the dark for 30 min and absorbance was measured at 517 nm wavelength. The radical scavenging activity was indicated as half-maximal inhibitory concentration (IC50) (Re et al., 1999).
ABTS radical scavenging activity
ABTS radical scavenging assay was measured by modifying the method of Re et al., 1999. ABTS (Sigma-Aldrich, Co., United States) reagent was dissolved in distilled water at a concentration of 7.0 mM, and potassium persulfate (Samchun Chemical Corporation, Korea) was dissolved in H2O at a concentration of 2.45 mM. The two solutions were mixed in a 1:1 ratio and left in the dark for 12–16 h to prepare a radical stock solution. The solution was diluted with phosphate-buffered saline, (Welgene, INC., Korea), and absorbance was measured with INNO Microplate Spectrophotometer (LTEK, Seongnam, South Korea) at 750 nm so that an absorbance value between 0.68 and 0.72 was obtained. In a 96 well plate (NUNC, United States), the sample and ABTS reaction ratio were performed to 1:9 (v/v), and the reaction was performed in the dark for 15 min at room temperature. After the reaction, absorbance was measured at 750 nm wavelength. The radical scavenging activity was indicated as half-maximal inhibitory concentration (IC50).
Quantitative chromatographic analysis
High-pressure liquid chromatography (HPLC) was analyzed using a Waters 2,695 system (United States) by modifying the method of Jeon et al., 2011. Experiments were conducted with a combination of 2,487 dual rhamda absorbance detector, run time 40 min, Phenomenex KJ0-4282 Guard column, Sky Pak C18 column (120 Å, 4.6*250 mm, 5 µm), and 25°C of the column oven temperature. A gradient mobile phase consisting of 1% formic acid in distilled water (A) and CH3CN (B) was used to run the separation. The elution program was set as follows: from 10% CH3CN (B) to 35% CH3CN (B) in 20 min, from 35% CH3CN (B) to 100% CH3CN (B) in 5 min, from 100% CH3CN (B) to 10% CH3CN (B) in 10 min, and 10% CH3CN (B) for 5 min; temperature, room temperature; flow rate, 1.0 ml/min; injection volume, 20 μl; UV detection, 280 nm. Taxifolin (Chengdu Biopurify Phytochemicals Ltd., Chengdu, China) was used for standard. Taxifolin, compound 1, enzymatic hydrolysis of RM and RM 60E were weighed to 1 mg and dissolved in 1 ml of methanol, respectively. Then, taxifolin was diluted to 500, 250, 125, 62.5, and 31.25 ppm and compound 1 was dilluted to 500 ppm.
Cell culture and treatment
Human follicle dermal papilla cells (HFDPC, # PCS-201-012) and the appropriate media (fibroblast basal medium, #PCS-201-030) and serum-free fibroblast growth kit (#PCS- 201-040 contained human serum albumin, linoleic acid, lecithin), rh FGF β, rh EGF/TGF β-1 supplement, rh insulin and ascorbic acid were obtained from the American Type Culture Collection (ATCC, Manassas, VA, United States). Cells were maintained following by manufacturer’s instructions. The humidified incubator was supplied with 5% CO2 maintained at 37°C and different complete media were supplemented with 1% antibiotics and growth factors (ATCC). The cells were used for in vitro experiments, after at least 14 days of proliferation.
MTT assay
Cells were pre-incubated with the indicated doses of taxifolin and treated with H2O2 for 12 h to investigate the effects of each dose on oxidative-stress-induced cyotoxicity in HDFPC. After incubation, the MTT stock solution (20 μl, final concentration 0.5 mg/ml) of 3-(4,5-dimetylthiazol-2-yl)-2,5-diphenyltetrazolium bromide (MTT, Sigma-Aldrich, MO, United States) was added to each well, and further incubated for 20 min in CO2 incubator. After removal of the supernatant, dimethylsulfoxide (100 μl) (DMSO, Sigma-Aldrich, MO, United States) were added to each well. The solubilized formazan after 20 min, the absorbance were measured at an 570 nm using a microplate reader (SPECTROstar Nano, BMG LABTECH, Berlin, Germany).
Enzyme-linked immunosorbent assay
Enzyme-linked immunosorbent assays (ELISA) were performed for IGF-I (ELH-IGF1-1, RayBiotech, Peachtree Corners, GA, United States), TGF-β (ADI-900-155, Enzo Biochem, New York, NY, United States) and DHT (11-DHTHU-E01, ALPCO, Salem, NH, United States). The sample collection and assay protocols were adapted from the manufacturer’s instructions.
Statistical analysis
All the experiments were repeated three times, and the mean value and standard deviation were presented. To compare each group, a one-way analysis of variance (ANOVA) followed by Tukey’s multiple range test was used and Student’s t-test. SPSS for Windows software (ver. 10.0, Chicago, IL, United States) was used to conduct the statistical analyses. Significance was set at p < 0.05.
Results
Chemical separation and identification
Taxifolin: White yellow amorphous powder, Negative LC-MS/MS m/z: 303.0 [M-H]−; 1H-NMR (700 MHz, MeOH-d4 + D2O): δ 6.80–6.96 (3H in total, m, H-2′, H-5′, and H-6′), 5.92 (1H, d, J = 2.1 Hz, H-8), 5.88 (1H, d, J = 2.1 Hz, H-6), 4.92 (1H, d, J = 11.1 Hz, H-2), 4.51 (1H, d, J = 11.1 Hz, H-3); 13C-NMR (175 MHz, MeOH-d4 + D2O): 198.47 (C-4), 168.74 (C-7), 165.35 (C-5), 164.55 (C-9), 147.18 (C-4′), 146.35 (C-3′), 120.94 (C-6′), 115.91 (C-5′), 116.11 (C-2′), 101.87 (C-10), 97.33 (C-6), 96.30 (C-8), 85.16 (C-2), 73.71 (C-3). The defined chemical structure of purified taxifolin (Figure 2) was made with ChemDraw (Perkinelmer, MA, United States).
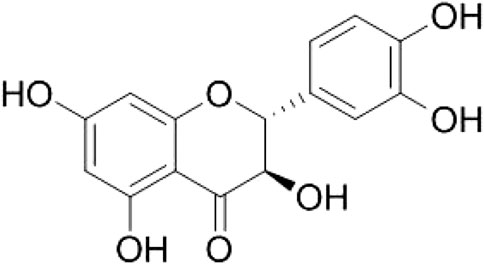
FIGURE 2. Chemical structures of compound 1. This figure was made with ChemDraw (http://www.perkinelmer.com/category/chemdraw).
Taxifolin was a white yellow amorphous powder. In TLC monitoring, spots were observed under UV radiation at 254 nm. Orange and red spots were detected by heating after spraying with 10% H2SO4 and anisaldehyde-H2SO4, respectively. And black spot was confirmed by spraying FeCl3. In LC-MS/MS, taxifolin showed 303.0 [M-H]− (Figure 3A) and compound 1 showed m/z: 302.9 [M-H]− (Figure 3B). Therefore, we confirmed that taxifolin and compound had same molecular weight. In 1H-NMR spectrum, δ 6.80–6.96 (3H in total, m, H-2′, 5′, 6′), a peak presumed to have the ABX type 3,4-dihydroxylation pattern of the B ring appeared. The signals of H-8 and H-6, which are meta-coupled through δ 5.92 (1H, d, J = 2.1 Hz, H-8), and 5.88 (1H, d, J = 2.1 Hz, H-6), respectively, were analyzed. Therefore, it was confirmed that the structure of the A-ring was a 5,7-dihydroxylation pattern. In addition, through δ 4.92 (1H, d, J = 11.1 Hz, H-2) and δ 4.51 (1H, d, J = 11.1 Hz, H-3), it was flavanonol structure that had no double bond in the C ring (Supplementary Figure S1). In the 13C-NMR spectrum, C-2 and C-3 were 85.16 and 73.71 ppm, respectively, indicating that glycosylation shift had not appeared (Supplementary Figure S2). Therefore, it was confirmed as an aglycone form. Thus, compound 1 was identified as taxifolin aglycone based on the spectral data compared with the values reported in the previous studies (Harborne and Mabry, 1982; Ishimaru et al., 1995; Lee et al., 2005).
In TLC monitoring, compared with taxifolin, spot was shown in compound 1 (Figure 4A). Therefore, we compared taxifolin spot was not confirmed in compound 1, taxifolin glycoside, RM60E and enzymatic hydrolysis of RM (Figure 4B). In enzymatic hydrolysis of RM, taxifolin spot was detected. However, taxifolin spot was not shown in RM60E and only taxifolin glycoside spot was identified.
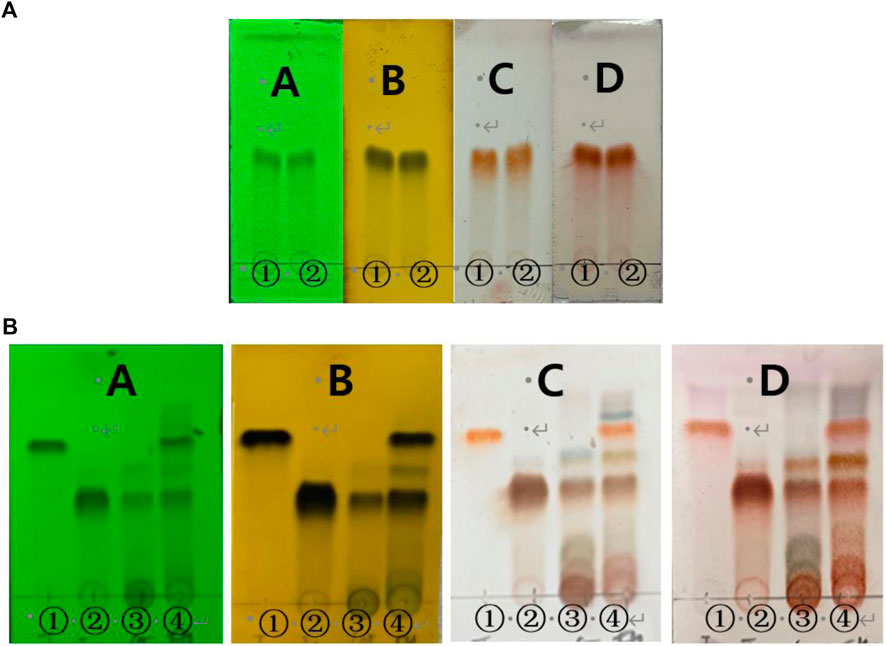
FIGURE 4. TLC monitoring of (A) taxifolin and compound 1. A = UV radiation (254 nm), B = FeCl3, C = 10% H2SO4 D = ρ-anisaldehyde H2SO4. 1 = taxifolin, 2 = compound 1. Chloroform:Methanol = 5:1 and (B) compound 1, taxifolin glycoside, RM60E, enzymatic hydrolysis of RM. A = UV radiation (254 nm), B = FeCl3, C = 10% H2SO4 D = ρ-anisaldehyde H2SO4. 1 = compound 1, 2 = taxifolin glycoside, 3 = RM60E, 4 = enzymatic hydrolysis of RM. Chloroform:Methanol:Water = 70:30:4.
DPPH and ABTS radical scavenging activity
Figure 5A is a graph showing the DPPH radical scavenging activity of compound 1, RM60E and enzymatic hydrolysis of RM. The IC50 of compound 1, RM60E and enzymatic hydrolysis of RM was 7.23 ± 0.03 µg/ml, 20.19 ± 0.34 µg/ml, and 11.21 ± 0.73 µg/ml, respectively. The IC50 for positive control (vitamin C) was 4.95 ± 0.05 µg/ml.
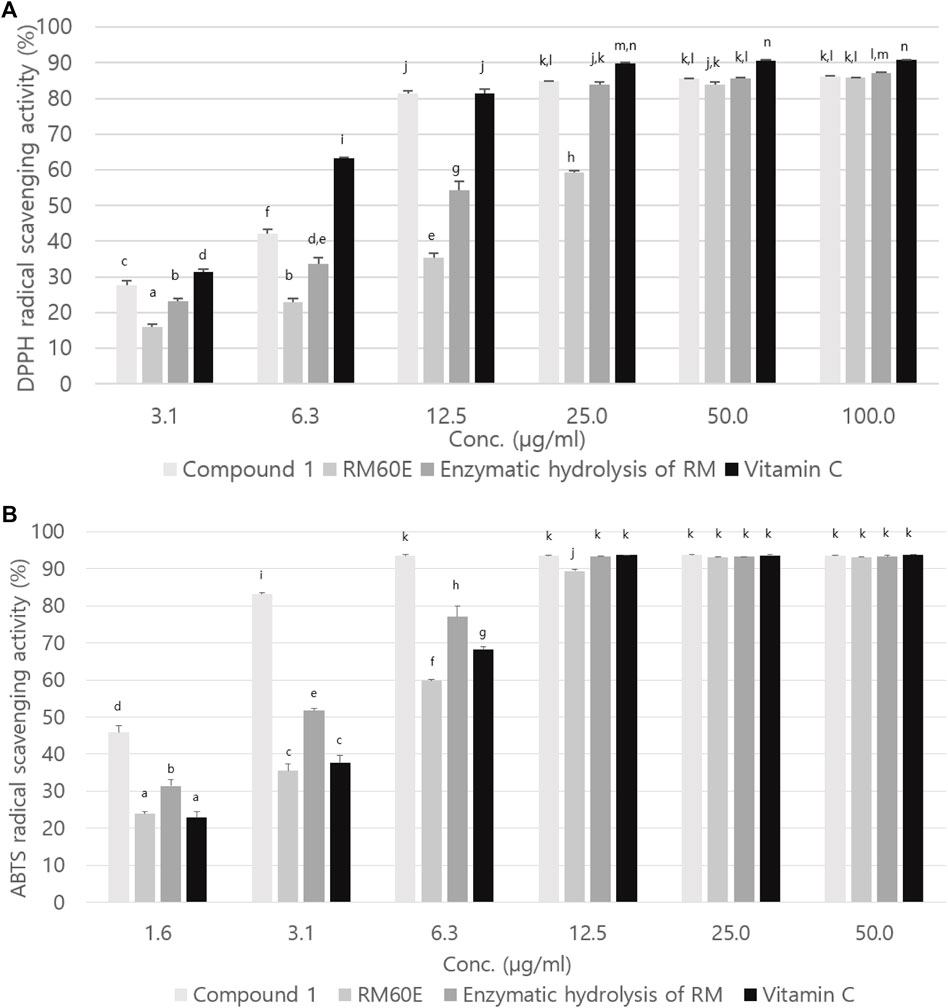
FIGURE 5. (A) DPPH and (B) ABTS radical scavenging activities of compound 1, RM60E, and enzymatic hydrolysis of RM. Values were expressed as mean ± S.D. of three determinations. Values bearing different superscripts in the same column are significantly different at p < 0.05 by Tukey’s test.
ABTS radical scavenging activity of compound 1, RM60E and enzymatic hydrolysis of RM is shown in Figure 5B. The IC50 of vitamin C, compound 1, RM60E, and enzymatic hydrolysis of RM were 4.36 ± 0.12 µg/ml, 1.68 ± 0.07 µg/ml, 4.98 ± 0.08 µg/ml, and2.99 ± 0.06 µg/ml, respectively.
Quantitative chromatographic analysis
The relationship between the concentration and the peak-area was measured by the minimum square method (R2 value). The standard calibration curve was obtained with concentrations of five increments for taxifolin, Y = 79768x − 191089 (R2 = 0.9985) as shown in Supplementary Figure S3. Good linearity (correlation coefficient 0.998) is shown for a calibration curve. The retention time of taxifolin was 15.12 ± 0.02 min (Figure 6A). The average content of taxifolin in compound 1 (Figure 6B) and the enzymatic hydrolysis of RM (Figure 6C) and were calculated at 217.82 ± 1.12 µg/ml and 480.27 ± 7.61 µg/ml (n = 3) by the above formula. However, taxifolin was not detected in RM 60E (Figure 6D).
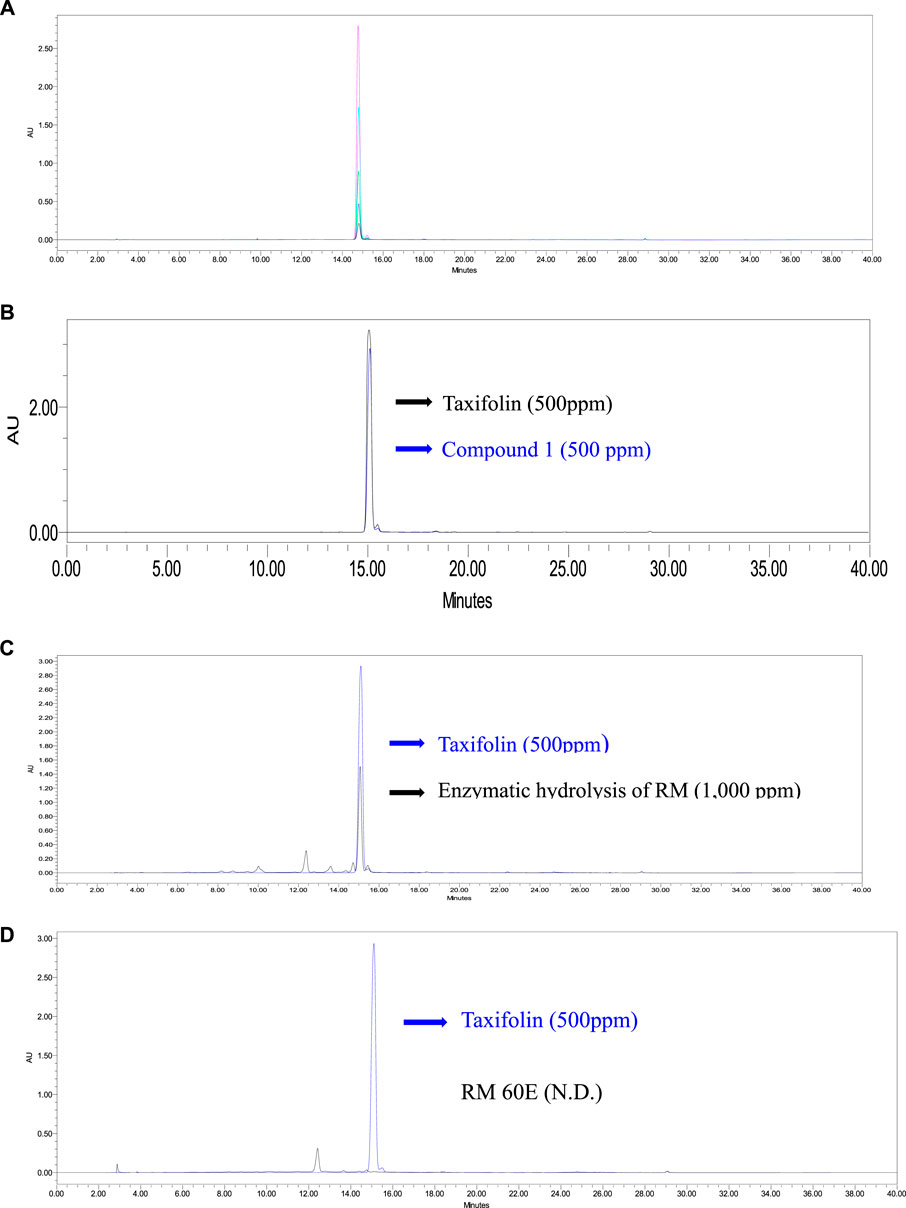
FIGURE 6. HPLC chromatogram of (A) taxifolin. Black (31.25 ppm), blue (62.5 ppm), green (125 ppm), skyblue (250 ppm), pink (500 ppm), (B) compound 1 (500 ppm). Black (taxifolin; 500 ppm), blue (compound 1; 500 ppm) (C) enzymatic hydrolysis of RM (1,000 ppm). Black (enzymatic hydrolysis of RM; 500 ppm), blue (taxifolin; 500 ppm) and (D) RM 60E. Black (RM 60E; 1,000 ppm), blue (taxifolin; 500 ppm).
Cell viability of human dermal follicle papilla cell after treatment with taxifolin
A cytotoxicityeffect of taxifolin was performed on human dermal follicle papilla cells (HDFPC). The HDFPCs were treated with various concentrations of taxifolin, for 12 h. Cell viability was determined by the MTT assay. The endogenous cytotoxicity of taxifolin is presented in Figure 7A. The cell viability of taxifolin (µg/ml) was higher than 80% at nearly 50 µg/ml. The results showed that there was no cytotoxicity observed at all the concentrations.
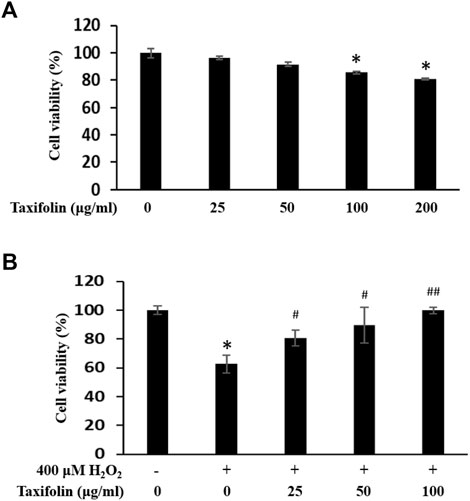
FIGURE 7. (A) Endogenous cytotoxicity of taxifolin in HDFPC. Cells were incubated with the indicated doses of taxifolin. Cell viability was calculated as described in Materials and methods. Data are expressed as the Mean ± SD. *p < 0.05 vs. non-treated group. (B) Inhibitory effect of taxifolin on hydrogen peroxide-induced cytotoxicity in HDFPC. Cells were pre-incubated with the indicated doses of taxifolin for 20 min and further treated 400 μM H2O2 for 12 h. Cell viability was calculated as described in Materials and methods. Data are expressed as the Mean ± SD. *p < 0.005 vs. non-treated group. #p < 0.05 and ##p < 0.005 vs. control group (H2O2 treated only).
To investigate the effects of anti-oxidative stress by taxifolin, we examined recovering abilities of taxifolin from enzymatic hydrolysis of RM on H2O2-induced cytotoxicity model with HDFPC (Figure 7B). Cell viability was markedly decreased to 60% by 400 μM H2O2 whereas the taxifolin from enzymatic hydrolysis of RM -treated HDFPCs showed a statistically significant increase in antioxidant activities in a dose-dependent manner compared with the control group.
Efficacy in increasing IGF-1 expression
Changes in IGF-1 level on oxidative stress-induced HDFPC damage and recovery effects of taxifolin from enzymatic hydrolysis of RM are shown in Figure 8A. IGF, one of the key maintenance factors involved in the anagen stage of hair growth cycle, was secreted at significantly lower levels by H2O2 compared normal control group on the intracellular region of HDFPC, whereas pretreatment of taxifolin from enzymatic hydrolysis of RM rescued IGF-1 level in a dose-dependent manner compared to H2O2 alone. These results suggested that taxifolin from enzymatic hydrolysis of RM was interrupt the oxidative stress-induced malfunction of hair growth cycles.
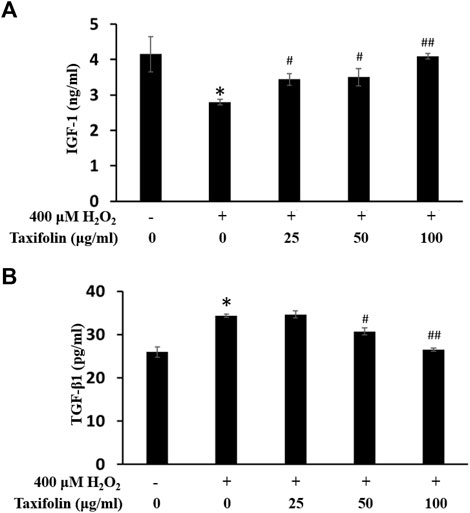
FIGURE 8. Recovery effects of taxifolin on oxidative stress-induced (A) IGF-1 formation and (B) TGF-β1 production. Cells were pre-incubated with indicated concentration of taxifolin (μg/mL, w/v) and treated with hydrogen peroxide for 12 h. IGF-1 production as measured as described in Materials and methods. Data are expressed as the mean ± SD. *p < 0.001 vs. non-treated group. #p < 0.05 and ##p < 0.005 vs. control group (hydrogen peroxide treated only). TGF-β1 production was measured as described in Materials and methods. Data are expressed as the mean ± SD. *p < 0.01 vs. non-treated group. #p < 0.05 and ##p < 0.01 vs. control group (hydrogen peroxide treated only).
TGF-β1 expression inhibition efficacy
As TGF-β families are a well-known inhibitory factors on hair growth and we examined the effect of oxidative stress on the production of TGF-β1. The effect of taxifolin from enzymatic hydrolysis of RM on TGF-β1 expression in the intracellular region was determined (Figure 8B). Treatment with H2O2 significantly increased TGF-β1 expression compared to the normal controls. However, taxifolin from enzymatic hydrolysis of RM treatment abrogated these effects in a dose-dependent manner, resulting in a significant reduction in TGF-β1 expression compared to H2O2 treatment alone. These results suggested that taxifolin from enzymatic hydrolysis of RM was regulates the oxidative stress-induced breakdown of hair growth cycles.
Dihydrotestosterone production inhibitory effect
In hair follicle papillae, 5α-reductase, DHT producing enzyme, normally predominated and known androgenic hormone response oi hair follicles depends on an intrafollicular androgenic signaling. The inhibition of DHT was investigated with various concentrations of taxifolin, and 10 µM minoxidil was used in the positive control group (Figure 9). The positive control group treated with minoxidil had a significant and strong inhibitory ability on DHT production. Although a taxifolin from enzymatic hydrolysis of RM was not as potent as minoxidil, it showed a dose-dependent DHT inhibitory effect, and a statistically significant and definite DHT production inhibitory ability when compared with the normal control group.
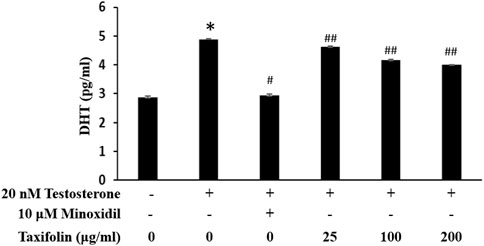
FIGURE 9. Inhibitory effect of taxifolin on testosterone-induced DHT production in HDFPC. Cells were pre-incubated with the indicated concentration of taxifolin for 20 min and further treated 20 nM testosterone for 12 h. The concentration of DHT were analyzed from cell lysate as described in Materials and methods. Data are expressed as the Mean ± SD. *p < 0.001 vs. non-treated group. #p < 0.001 and ##p < 0.05 vs. control group (testosterone treated only).
Discussion
Taxifolin is known to have various pharmacological properties such as antioxidants, anticancer, cardiovascular, and effective inhibition of oxidative stress, cell apoptosis, malignancies, microbial infection, cardiovascular disease, and liver disease. And it has an anti-cancer activity with a little or no side effects to the normal cells (Si et al., 2009b; Das et al., 2021). In this study, we compared taxifolin with vitamin C, which is positive control. In DPPH radical scavenging activity, especially in low concentration, taxifolin showed significantly high antioxidant activity than RM60E and enzymatic hydrolysis of RM. And in 12.5 µg/ml, taxifolin has antioxidant activity as same as vitamin. Moreover, at a low concentration, the antioxidant activity of taxifolin was higher than that of vitamin C and from 12.5 and above, the results were statistically equivalent to those of vitamin C. Also, it was confirmed that taxifolin has high antioxidants compared with other flavonoids in a previous studies (Lee et al., 2006; Li et al., 2014; Chanput et al., 2016). Therefore, based on the excellent antioxidant activities, we conducted experiments about apoptosis modulation of taxifolin. However, taxifolin is rarely been used alone; it usually used in complex preparations and it is found in small amounts (Weidmann, 2012; Sunil and Xu, 2019). In the previous study, taxifolin barely discernable in the HPLC chromatogram of silymarin (Kroll et al., 2007). According to the quantitative chromatographic analysis, we confirmed that taxifolin was not detected in 60% EtOH extracts from RM. However, high content of taxifolin 217.82 ± 1.12 µg/ml showed in the enzymatic hydrolysis of RM which 217% higher than RM60E. Therefore, it was confirmed that a high content of taxifolin aglycone was produced by enzymatic hydrolysis.
Classically, hair loss is caused by various and complex factors, and one of the causes is oxidative stress. Oxidative stress is known to induce apoptosis then stimulate various cell types within the scalp and hair components; these cell types are keratinocytes, hair follicle cells, papilla cells, and various immune cells. Particularly, apoptosis is mediated by various signal cascades including Bcl-2, Bax, caspase family, and PARP (Tsujimoto et al., 1984; Cleary et al., 1986; Oltval et al., 1993; Muller et al., 1994; Sedlak et al., 1995; Zhao et al., 2001). In our previous reports, when interrupt the oxidative stress can modulate HFDPCs apoptosis (Ha et al., 2021; Kwon et al., 2022). In this study, in H2O2-induced apoptosis, taxifolin showed statistically significant antioxidant activity. Therefore, it can be expected that taxifolin may modulate the cell death signaling and required further experiments.
IGF-1 is known to be an important growth factor that protects against oxidative stress-induced epithelial cell apoptosis and promotes hair growth (Philpott et al., 1994; Baregamian et al., 2006). Therefore, under the presence of IGF-1, hair follicle morphology was maintained to normal. Furthermore, IGF-1 plays an important role in androgen-dependent alopecia because it is involved in the action of testosterone by affecting the androgen hormone (Price, 1999; Randall, 2008). Thus, we investigated the effect of promoting the expression of growth factors in a dose-dependent manner by treating the dermal papilla cells with taxifolin. The results confirmed that taxifolin will modulate IGF-1 in HDFPC.
TGF-β inhibits the proliferation of hair follicles and it can regulate hair follicles as a negative growth factor. Androgen is related to ROS production, and ROS is involved in TGF-β secretion. Particularly, TGF-β1 secretion is closely related to androgenetic alopecia (Trüeb, 2002) and it focused ROS/antioxidative reaction as crucial role in dermal papilla cells (DPCs) (Shin et al., 2013) as by hair follicle dermal papilla cells (DPCs) in bald scalp. Traditionally, TGF-β was well known as potent inhibitor of the many various cell types (Tucker et al., 1984; Barnard et al., 1988), particularly TGF-β1 was most often mentioned as major factor on hair growth inhibition (Mori et al., 1996; Inui et al., 2003; Shin et al., 2013). Similarly, TGF-β2 (Hibino and Nishiyama, 2004) and predictively TGF-β3 also suggested as important key factor on hair follicle development, growth and cycles (Choi et al., 2018). Moreover, particularly in the epithelial cells with respect to reactive oxygen species (ROS) homeostasis, androgens may be significant in regulating the cellular redox state. In the DP-6 cell line, it is reported that ROS formation was increased after androgen administration, and TGF-β1 secretion was increased by androgen administration (Mori et al., 1996; Park et al., 2001; Olsen et al., 2006). Thus, in hair follicle cells, interruption of the ROS-mediated signaling pathway will inhibit TGF-β1 signals. In this study, we confirmed that taxifolin inhibited TGF-β1 expression in HDFPC. Therefore, taxifolin could be an excellent new natural ingredient for hair growth promotion.
DHT is the representative factor in male pattern hair loss. When testosterone, a male hormone, is oxidized by 5α-reductase, it changes to DHT (Kaufman, 2002; Dhariwala and Ravikumar, 2019). The results of this study showed that with inhibition of DHT production, which is the main cause of male pattern hair loss, with taxifolin, DHT production was modulated in the non-toxic ranges of taxifolin, and, the effects were statistically significant when compared with the positive and negative controls. The positive control group in this experiment, minoxidil, is a common treatment for hair loss. However, minoxidil is only effective for initial hair loss and crown of ongoing hair loss. In the early stages of male pattern baldness, minoxidil can prevent the progression of hair loss. In addition, it was reported that if minoxidil is not applied to the scalp twice a day consistently for 6–12 months, it is not effective. Moreover, minoxidil has side effects, including cardiovascular side effects in children who use minoxidil and irritative dermatitis. And diffuse facial, forearm, cheek and neck hypertrichosis, redness, and contact dermatitis have been reported, and hirsutism can be induced in 0.5%–1% of the users (Hagemann et al., 2005; Georgala et al., 2006; Jimenez-Cauhe et al., 2020). Moreover, there is the disadvantage that hair returns to the state before treatment when minoxidil treatment is stopped (do Nascimento et al., 2020). Therefore, it is important to find effective and safe hair loss treatments. In this study, taxifolin was confirmed to be an effective anti-hair loss agent and a new molecule that promotes hair growth in male pattern hair loss.
Conclusion
In this study, experiments were conducted to confirm that new molecules derived from natural sources can provide an objective basis for scientifically controlling hair loss and have a low risk of side effects. In general, flavonoid aglycone has stronger biological activities, such as antioxidant activity and antidiabetic activitiesthan glycosides. Taxifolin is detected only in trace amounts in nature and is not easily observed (Weidmann, 2012; Sunil and Xu, 2019). Therefore, after preparing high-content taxifolin aglycone extracts from enzymatic hydrolysis of RM, and separating and purifying taxifolin from the extracts, experiments were conducted. In conclusion, it was confirmed that taxifolin effectively regulates the apoptosis of dermal papilla cells, which are closely related to hair loss, based on its strong antioxidant activity. In addition, DHT, a major cause of male pattern hair loss, was statistically significantly reduced in taxifolin compared to minoxidil treatment. It was also confirmed that taxifolin treatment significantly increased the representative factor for promoting hair growth, IGF-1, and significantly reduced TGF-β1, a representative biomarker for promoting hair loss. These results demonstrated taxifolin as a potential treatment for hair loss resulting from the death of the dermal papilla cells and male pattern hair loss.
Data availability statement
All relevant data is contained within the article: The original contributions presented in the study are included in the article/Supplementary Material, further inquiries can be directed to the corresponding authors.
Author contributions
Investigation, S-MP and Y-CH; Supervision, CS, K-HP, and S-EC; Writing—original draft, S-EC, K-HP, and CS; Writing—review and editing, CS, CG, WG, and Y-SB.
Funding
This research was supported by the Technology Development Program (S3030198) funded by the Ministry of SMEs and Startups (MSS, Korea) and Key Technology Research and Development Program of Tianjin (19YFZCSN00950) from Tianjin Municipal Science and Technology Bureau, China. This work was also partially supported by the R&D program for Forest Science Technology (2019151D10-2223-0301) provided by the Korea Forest Service (Korea Forestry Promotion Institute) to S-EC and National Research Foundation of Korea (NRF) grant funded by the Korean government (MSIT) (2021R1A2C1091322) to K-HP.
Conflict of interest
The authors declare that the research was conducted in the absence of any commercial or financial relationships that could be construed as a potential conflict of interest.
Publisher’s note
All claims expressed in this article are solely those of the authors and do not necessarily represent those of their affiliated organizations, or those of the publisher, the editors and the reviewers. Any product that may be evaluated in this article, or claim that may be made by its manufacturer, is not guaranteed or endorsed by the publisher.
Supplementary material
The Supplementary Material for this article can be found online at: https://www.frontiersin.org/articles/10.3389/fbioe.2022.995238/full#supplementary-material
Supplementary Figure S1 | 1H-NMR spectrum of compound 1 (700 MHz, MeOH-d4+D2O).
Supplementary Figure S2 | 13C-NMR spectrum of compound 1 (175 MHz, MeOH-d4+D2O).
Supplementary Figure S3 | Calibration curve and equation of taxifolin. Y = 79768x − 191089 (R2 = 0.9985).
Abbreviations
RM, Rhododendron mucrotulatum; RM 60E, 60% EtOH extract from Rhododendron mucrotulatum; DHT, dihydrotestosterone; MTT, 3-(4,5-Dimethylthiazol-2-yl)-2,5-Diphenyltetrazolium Bromide; HFDPC, Human follicle dermal papilla cells; ELISA, Enzyme-linked immunosorbent assay; IGF-I, Insulin-like growth factor-I; TGF-β, Transforming growth factor-beta; Bax, Bcl-2-associated X protein; Bcl-2, B-cell lymphoma 2; PARP-1, Poly ADP-ribose polymerase 1.
References
An, L., Si, C., Bae, J. H., Jeong, H., and Kim, Y. S. (2020). One-step silanization and amination of lignin and its adsorption of Congo red and Cu (II) ions in aqueous solution. Int. J. Biol. Macromol. 159, 222–230. doi:10.1016/j.ijbiomac.2020.05.072
An, L., Si, C., Wang, G., Sui, W., and Tao, Z. (2019). Enhancing the solubility and antioxidant activity of high-molecular-weight lignin by moderate depolymerization via in Situ ethanol/acid catalysis. Ind. Crops Prod. 128, 177–185. doi:10.1016/j.indcrop.2018.11.009
Baregamian, N., Song, J., Jeschke, M. G., Evers, B. M., and Chung, D. H. (2006). IGF-1 protects intestinal epithelial cells from oxidative stress-induced apoptosis. J. Surg. Res. 136, 31–37. doi:10.1016/j.jss.2006.04.028
Barnard, J. A., Bascom, C. C., Lyons, R. M., Sipes, N. J., and Moses, H. L. (1988). Transforming growth factor β in the control of epidermal proliferation. Am. J. Med. Sci. 31, 159–163. doi:10.1097/00000441-198809000-00003
Chanput, W., Krueyos, N., and Ritthiruangdej, P. (2016). Anti-oxidative assays as markers for anti-inflammatory activity of flavonoids. Int. Immunopharmacol. 40, 170–175. doi:10.1016/j.intimp.2016.08.038
Chen, S., Wang, G., Sui, W., Parvez, A. M., Dai, L., and Si, C. (2020). Novel lignin-based phenolic nanosphere supported palladium nanoparticles with highly efficient catalytic performance and good reusability. Ind. Crops Prod. 145, 112164. doi:10.1016/j.indcrop.2020.112164
Chen, X., Yang, Q., Si, C.-L., Wang, Z., Huo, D., Hong, Y., et al. (2016). Recovery of oligosaccharides from prehydrolysis liquors of poplar by microfiltration/ultrafiltration membranes and anion exchange resin. ACS Sustain. Chem. Eng. 4, 937–943. doi:10.1021/acssuschemeng.5b01029
Cho, Y. J., Ju, I. S., Chun, S. S., An, B. J., Kim, J. H., Kim, M. U., et al. (2008). Screening of biological activities of extracts from Rhododendron mucronulatum Turcz. Flowers. J. Korean Soc. Food Sci. Nutr. 37, 276–281. doi:10.3746/jkfn.2008.37.3.276
Choi, S. E., Park, K. H., Han, B. H., Jeong, M. S., Seo, S. J., Lee, D. I., et al. (2011). Inhibition of inducible nitric oxide synthase and cyclooxygenase-2 expression by phenolic compounds from roots of Rhododendron mucronulatum. Phytother. Res. 25, 1301–1305. doi:10.1002/ptr.3376
Choi, Y. M., Choi, S. Y., Kim, H., Kim, J., Ki, M. S., An, I., et al. (2018). TGFβ family mimetic peptide promotes proliferation of human hair follicle dermal papilla cells and hair growth in C57BL/6 mice. Biomed. Dermatol. 2, 23–26. doi:10.1186/s41702-018-0033-8
Chung, T. Y., and Lee, S. E. (1991). Volatile flavor components of Jindalrae flower (Korean azalea flower, Rhododendron mucronulatum Turczaninow). J. Korean Agric. Chem. Soc. 34, 344–352.
Cleary, M. L., Smith, S. D., and Sklar, J. (1986). Cloning and structural analysis of cDNAs for bcl-2 and a hybrid bcl-2/immunoglobulin transcript resulting from the t (14;18) translocation. Cell 47, 19–28. doi:10.1016/0092-8674(86)90362-4
Dai, L., Cao, Q., Wang, K., Han, S., Si, C., Liu, D., et al. (2020). High efficient recovery of L-lactide with lignin-based filler by thermal degradation. Ind. Crops Prod. 143, 111954. doi:10.1016/j.indcrop.2019.111954
Dai, L., Liu, R., Hu, L.-Q., Zou, Z.-F., and Si, C.-L. (2017). Lignin nanoparticle as a novel green carrier for the efficient delivery of resveratrol. ACS Sustain. Chem. Eng. 5, 8241–8249. doi:10.1021/acssuschemeng.7b01903
Das, A., Baidya, R., Chakraborty, T., Samanta, A. K., and Roy, S. (2021). Pharmacological basis and new insights of taxifolin: A comprehensive review. Biomed. Pharmacother. 142, 112004. doi:10.1016/j.biopha.2021.112004
Dhariwala, M. Y., and Ravikumar, P. (2019). An overview of herbal alternatives in androgenetic alopecia. J. Cosmet. Dermatol. 18, 966–975. doi:10.1111/jocd.12930
do Nascimento, I. J. B., Harries, M., Rocha, V. B., Thompson, J. Y., Wong, C. H., Varkaneh, H. K., et al. (2020). Effect of oral minoxidil for alopecia: Systematic review. Int. J. Trichol. 12, 147. doi:10.4103/ijt.ijt_19_20
Du, H., Liu, W., Zhang, M., Si, C., Zhang, X., and Li, B. (2019). Cellulose nanocrystals and cellulose nanofibrils based hydrogels for biomedical applications. Carbohydr. Polym. 209, 130–144. doi:10.1016/j.carbpol.2019.01.020
Du, H., Parit, M., Liu, K., Zhang, M., Jiang, Z., Huang, T.-S., et al. (2021a). Engineering cellulose nanopaper with water resistant, antibacterial, and improved barrier properties by impregnation of chitosan and the followed halogenation. Carbohydr. Polym. 270, 118372. doi:10.1016/j.carbpol.2021.118372
Du, H., Parit, M., Liu, K., Zhang, M., Jiang, Z., Huang, T.-S., et al. (2021b). Multifunctional cellulose nanopaper with superior water-resistant, conductive, and antibacterial properties functionalized with chitosan and polypyrrole. ACS Appl. Mat. Interfaces 13, 32115–32125. doi:10.1021/acsami.1c06647
Du, H., Zhang, M., Liu, K., Parit, M., Jiang, Z., Zhang, X., et al. (2022). Conductive PEDOT:PSS/cellulose nanofibril paper electrodes for flexible supercapacitors with superior areal capacitance and cycling stability. Chem. Eng. J. 428, 131994. doi:10.1016/j.cej.2021.131994
El-Zawawy, W. K., Ibrahima, M. M., Abdel-Fattahb, Y. R., Soliman, N. A., and Mahmoud, M. M. (2011). Acid and enzyme hydrolysis to convert pretreated lignocellulosic materials into glucose for ethanol production. Carbohydr. Polym. 84, 865–871. doi:10.1016/j.carbpol.2010.12.022
Georgala, S., Befon, A., Maniatopoulou, E., and Georgala, C. (2006). Topical use of minoxidil in children and systemic side effects. Dermatology 214, 101–102. doi:10.1159/000096924
Gupta, M. B., Bhalla, T. N., Gupta, G. P., Mitra, C. R., and Bhargava, K. P. (1971). Anti-inflammatory activity of taxifolin. Jpn. J. Pharmacol. 21, 377–382. doi:10.1016/S0021-5198(19)36228-6
Ha, E. J., Yun, J. H., Si, C., Bae, Y. S., Jeong, Y. H., Park, K. H., et al. (2021). Application of ethanol extracts from Alnus sibirica Fisch. ex Turcz in hair growth promotion. Front. Bioeng. Biotechnol. 9, 673314. doi:10.3389/fbioe.2021.673314
Hagemann, T., Schlutter-Bohmer, B., Allam, J. P., Bieber, T., and Novak, N. (2005). Positive lymphocyte transformation test in a patient with allergic contact dermatitis of the scalp after short-term use of topical minoxidil solution. Contact Dermat. 53, 53–55. doi:10.1111/j.0105-1873.2005.00456b.x
Hatano, T., Edamatsu, R., Hiramatsu, M., Mori, A., Fujita, Y., Yasuhara, T., et al. (1989). Effects of the interaction of tannins with Co-existing substances. VI. Effects of tannins and related polyphenols on superoxide anion radical, and on 1, 1-diphenyl-2-picrylhydrazyl radical. Chem. Pharm. Bull. 37, 2016–2021. doi:10.1248/cpb.37.2016
Herman, E. H., Zhang, J., Chadwick, D. P., and Ferrans, V. J. (1996). Age dependence of the cardiac lesions induced by minoxidil in the rat. Toxicology 110, 71–83. doi:10.1016/0300-483X(96)03337-9
Hibino, T., and Nishiyama, T. (2004). Role of TGF-β2 in the human hair cycle. J. Dermatol. Sci. 35, 9–18. doi:10.1016/j.jdermsci.2003.12.003
Hu, L., Wang, K., Li, G., Zhang, R., Luo, Y., Si, C.-L., et al. (2017). Isolation and structural elucidation of heartwood extractives of Juglans sigillata. Holzforschung 71, 785–791. doi:10.1515/hf-2017-0036
Huang, L.-Z., Ma, M.-G., Ji, X.-X., Choi, S.-E., and Si, C. (2021). Recent developments and applications of hemicellulose from wheat straw: A review. Front. Bioeng. Biotechnol. 9, 690773. doi:10.3389/fbioe.2021.690773
Inui, S., Fukuzato, Y., Nakajima, T., Yoshikawa, K., and Itami, S. (2003). Identification of androgen-inducible TGF-β1 derived from dermal papilla cells as a key mediator in androgenetic alopecia. J. Investig. Dermatol. Symp. Proc. 8, 69–71. doi:10.1046/j.1523-1747.2003.12174.x
Ishimaru, K., Omoto, T., Asai, I., Ezaki, K., and Shimomura, K. (1995). Taxifolin 3-arabinoside from Fragaria x ananassa. Phytochemistry 40, 345–347. doi:10.1016/0031-9422(95)00204-K
Jang, S. S., Lee, D., Song, J., Park, D. H., Jeon, C. Y., and Hwang, G. S. (2022). Inhibitory effect of Rhododendron mucronulatum root extract on allergic inflammation. J. Int. Korean Med. 43, 68–78. doi:10.22246/jikm.2022.43.1.68
Jang, S. S., Shirai, Y., Uchida, M., and Wakisaka, M. (2012). Production of mono sugar from acid hydrolysis of seaweed. Afr. J. Biotechnol. 11, 1953. doi:10.5897/AJB10.1681
Jeon, J. S., Kim, H. G., Um, B. H., and Kim, C. Y. (2011). Rapid detection of antioxidant flavonoids in azalea (Rhododendron mucronulatum) flowers using on-line HPLC-ABTS+ system and preparative isolation of three flavonoids by centrifugal partition chromatography. Sep. Sci. Technol. 46, 518–524. doi:10.1080/01496395.2010.520296
Jeong, M. S., Choi, S. E., Kim, J. Y., Kim, J. S., Kim, E. J., Park, K. H., et al. (2010). Atopic dermatitis-like skin lesions reduced by topical application and intraperitoneal injection of Hirsutenone in NC/Nga mice. Clin. Dev. Immunol. 618517, 1–7. doi:10.1155/2010/618517
Jimenez-Cauhe, J., Saceda-Corralo, D., Rodrigues-Barata, R., Moreno-Arrones, O. M., Ortega-Quijano, D., Fernandez-Nieto, D., et al. (2020). Safety of low-dose oral minoxidil treatment for hair loss. A systematic review and pooled-analysis of individual patient data. Dermatol. Ther. 33, e14106. doi:10.1111/dth.14106
Kaufman, K. D. (2002). Androgens and alopecia. Mol. Cell. Endocrinol. 198, 89–95. doi:10.1016/S0303-7207(02)00372-6
Kim, S. H., Kim, J. D., and Lee, H. K. (2006). A statistical study on hair loss and the actual conditions of its care. J. Korean Soc. Cosmetol. 12, 71–83.
Kroll, D. J., Shaw, H. S., and Oberlies, N. H. (2007). Milk thistle nomenclature: Why it matters in cancer research and pharmacokinetic studies. Integr. Cancer Ther. 6, 110–119. doi:10.1177/1534735407301825
Kwon, Y. E., Choi, Sun. Eun., and Park, K. H. (2022). Regulation of cytokines and dihydrotestosterone production in human hair follicle papilla cells by supercritical extraction-residues extract of Ulmus davidiana. Molecules 27, 1419. doi:10.3390/molecules27041419
Lee, B. W., Lee, J. H., Gal, S. W., Moon, Y. H., and Park, K. H. (2006). Selective ABTS radical-scavenging activity of prenylated flavonoids from Cudrania tricuspidata. Biosci. Biotechnol. Biochem. 70, 427–432. doi:10.1271/bbb.70.427
Lee, J. H., Jeon, W. J., Yoo, E. S., Kim, C. M., and Kwon, Y. S. (2005). The chemical constituents and their antioxidant activity of the stem of Rhododendron mucronulatum. Nat. Prod. Sci. 11, 97–102. doi:10.1162/0011526053124343
Lee, S. H., and Lee, J. R. (2010). Association of diffuse hair loss of adult male on stress, self-confidence, and depression. J. Korean Soc. Cosmetol. 16, 1171–1179.
Lee, S. W., Choi, S. H., Hong, Y. S., and Lim, S. I. (2007). Grayanotoxin poisoning from flower of Rhododendron mucronulatum in humans. Bull. Environ. Contam. Toxicol. 78, 11–12. doi:10.1007/s00128-007-9006-0
Lei, M., and Chuong, C. M. (2016). Aging, alopecia, and stem cells. Science 351, 559–560. doi:10.1126/science.aaf1635
Li, Q., Wei, Q., Yuan, E., Yang, J., and Ning, Z. (2014). Interaction between four flavonoids and trypsin: Effect on the characteristics of trypsin and antioxidant activity of flavonoids. Int. J. Food Sci. Technol. 49, 1063–1069. doi:10.1111/ijfs.12401
Li, W., Wang, G., Sui, W., Xu, T., Li, Z., Parvez, A. M., et al. (2022). Facile and scalable preparation of cage-like mesoporous carbon from lignin-based phenolic resin and its application in supercapacitor electrodes. Carbon 196, 819–827. doi:10.1016/j.carbon.2022.05.053
Li, X., Lu, X., Nie, S., Liang, M., Yu, Z., Duan, B., et al. (2020). Efficient catalytic production of biomass-derived levulinic acid over phosphotungstic acid in deep eutectic solvent. Ind. Crops Prod. 145, 112154. doi:10.1016/j.indcrop.2020.112154
Li, X., Xu, R., Yang, J., Nie, S., Liu, D., Liu, Y., et al. (2019). Production of 5-hydroxymethylfurfural and levulinic acid from lignocellulosic biomass and catalytic upgradation. Ind. Crops Prod. 130, 184–197. doi:10.1016/j.indcrop.2018.12.082
Liu, C., Wang, G., Sui, W., An, L., and Si, C. (2017a). Preparation and characterization of chitosan by a novel deacetylation approach using glycerol as green reaction solvent. ACS Sustain. Chem. Eng. 5, 4690–4698. doi:10.1021/acssuschemeng.7b00050
Liu, H., Du, H., Zheng, T., Liu, K., Ji, X., Xu, T., et al. (2021a). Cellulose based composite foams and aerogels for advanced energy storage devices. Chem. Eng. J. 426, 130817. doi:10.1016/j.cej.2021.130817
Liu, H., Xu, T., Cai, C., Liu, K., Liu, W., Zhang, M., et al. (2022a). Multifunctional superelastic, superhydrophilic, and ultralight nanocellulose-based composite carbon aerogels for compressive supercapacitor and strain sensor. Adv. Funct. Mat. 32, 2113082. doi:10.1002/adfm.202113082
Liu, H., Xu, T., Liang, Q., Zhao, Q., Zhao, D., and Si, C. (2022b). Compressible cellulose nanofibrils/reduced graphene oxide composite carbon aerogel for solid-state supercapacitor. Adv. Compos. Hybrid. Mat. 5, 1168–1179. doi:10.1007/s42114-022-00427-0
Liu, H., Xu, T., Liu, K., Zhang, M., Liu, W., Li, H., et al. (2021b). Lignin-based electrodes for energy storage application. Ind. Crops Prod. 165, 113425. doi:10.1016/j.indcrop.2021.113425
Liu, K., Du, H., Liu, W., Liu, H., Zhang, M., Xu, T., et al. (2022c). Cellulose nanomaterials for oil exploration applications. Polym. Rev. 62 (3), 585–625. doi:10.1080/15583724.2021.2007121
Liu, K., Du, H., Zheng, T., Liu, H., Zhang, M., Zhang, R., et al. (2021c). Recent advances in cellulose and its derivatives for oilfield applications. Carbohydr. Polym. 259, 117740. doi:10.1016/j.carbpol.2021.117740
Liu, K., Du, H., Zheng, T., Liu, W., Zhang, M., Liu, H., et al. (2021d). Lignin-containing cellulose nanomaterials: Preparation and applications. Green Chem. 23, 9723–9746. doi:10.1039/D1GC02841C
Liu, K., Liu, W., Li, W., Duan, Y., Zhou, K., Zhang, S., et al. (2022d). Strong and highly conductive cellulose nanofibril/silver nanowires nanopaper for high performance electromagnetic interference shielding. Adv. Compos. Hybrid. Mat. 5, 1078–1089. doi:10.1007/s42114-022-00425-2
Liu, L., Wen, W., Zhang, R., Wei, Z., Deng, Y., Xiao, J., et al. (2017b). Complex enzyme hydrolysis releases antioxidative phenolics from rice bran. Food Chem. 214, 1–8. doi:10.1016/j.foodchem.2016.07.038
Liu, S., Du, H., Liu, K., Ma, M.-G., Kwon, Y.-E., Si, C., et al. (2021e). Flexible and porous Co3O4-carbon nanofibers as binder-free electrodes for supercapacitors. Adv. Compos. Hybrid. Mat. 4, 1367–1383. doi:10.1007/s42114-021-00344-8
Liu, W., Du, H., Liu, H., Xie, H., Xu, T., Zhao, X., et al. (2020a). Highly efficient and sustainable preparation of carboxylic and thermostable cellulose nanocrystals via FeCl3-catalyzed innocuous citric acid hydrolysis. ACS Sustain. Chem. Eng. 8, 16691–16700. doi:10.1021/acssuschemeng.0c06561
Liu, W., Du, H., Liu, K., Liu, H., Xie, H., Si, C., et al. (2021f). Sustainable preparation of cellulose nanofibrils via choline chloride-citric acid deep eutectic solvent pretreatment combined with high-pressure homogenization. Carbohydr. Polym. 267, 118220. doi:10.1016/j.carbpol.2021.118220
Liu, W., Du, H., Zhang, M., Liu, K., Liu, H., Xie, H., et al. (2020b). Bacterial cellulose-based composite scaffolds for biomedical applications: A review. ACS Sustain. Chem. Eng. 8, 7536–7562. doi:10.1021/acssuschemeng.0c00125
Liu, W., Liu, K., Du, H., Zheng, T., Zhang, N., Xu, T., et al. (2022e). Cellulose nanopaper: Fabrication, functionalization, and applications. Nano-Micro Lett. 14, 104. doi:10.1007/s40820-022-00849-x
Liu, W., Liu, K., Wang, Y., Lin, Q., Liu, J., Du, H., et al. (2022f). Sustainable production of cellulose nanofibrils from Kraft pulp for the stabilization of oil-in-water Pickering emulsions. Ind. Crops Prod. 185, 115123. doi:10.1016/j.indcrop.2022.115123
Lu, J., Han, X., Dai, L., Li, C., Wang, J., Zhong, Y., et al. (2020). Conductive cellulose nanofibrils-reinforced hydrogels with synergetic strength, toughness, self-adhesion, flexibility and adjustable strain responsiveness. Carbohydr. Polym. 250, 117010. doi:10.1016/j.carbpol.2020.117010
Lu, J., Zhu, W., Dai, L., Si, C., and Ni, Y. (2019). Fabrication of thermo- and pH-sensitive cellulose nanofibrils-reinforced hydrogel with biomass nanoparticles. Carbohydr. Polym. 215, 289–295. doi:10.1016/j.carbpol.2019.03.100
Ma, C., Ma, M.-G., Si, C., Ji, X.-X., and Wan, P. (2021). Flexible MXene-based composites for wearable devices. Adv. Funct. Mat. 31, 2009524. doi:10.1002/adfm.202009524
Mandalari, G., Bennett, R. N., Kirby, A. R., Lo Curto, R. B., Bisignano, G., Waldron, K. W., et al. (2006). Enzymatic hydrolysis of flavonoids and pectic oligosaccharides from bergamot (Citrus bergamia Risso) peel. J. Agric. Food Chem. 54, 8307–8313. doi:10.1021/jf0615799
Mori, O., Hachisuka, H., and Sasai, Y. (1996). Effects of transforming growth factor β1 in the hair cycle. J. Dermatology 23, 89–94. doi:10.1111/j.1346-8138.1996.tb03976.x
Muller, S., Briand, J. P., Barakat, S., Lagueux, J., Poirier, G. G., De Murcia, G., et al. (1994). Autoantibodies reacting with poly(ADP-ribose) and with a zinc-finger functional domain of poly(ADP-ribose) polymerase involved in the recognition of damaged DNA. Clin. Immunol. Immunopathol. 73, 187–196. doi:10.1006/clin.1994.1187
Najeb, S. M., Jaccob, A. A., Al-Moziel, M. S., and Abdulhameed, H. M. (2022). Cardioprotective and antioxidant effects of taxifolin and vitamin C against diazinone-induced myocardial injury in rats. Environ. Anal. Health Toxicol. 37, e2022002. doi:10.5620/eaht.2022002
Nguyen, K. H., and Marks, J. G. (2003). Pseudoacromegaly induced by the long-term use of minoxidil. J. Am. Acad. Dermatol. 48, 962–965. doi:10.1067/mjd.2003.325
Olsen, E. A., Hordinsky, M., Whiting, D., Stough, D., Hobbs, S., Ellis, M. L., et al. (2006). The importance of dual 5α-reductase inhibition in the treatment of male pattern hair loss: Results of a randomized placebo-controlled study of dutasteride versus finasteride. J. Am. Acad. Dermatol. 55, 1014–1023. doi:10.1016/j.jaad.2006.05.007
Oltval, Z. N., Milliman, C. L., and Korsmeyer, S. J. (1993). Bcl-2 heterodimerizes in vivo with a conserved homolog, Bax, that accelerates programed cell death. Cell 74, 609–619. doi:10.1016/0092-8674(93)90509-O
Park, S. K., Kim, J., Seomun, Y., Choi, J. k., Kim, D. H., Han, I. O., et al. (2001). Hydrogen peroxide is a novel inducer of connective tissue growth factor. Biochem. Biophys. Res. Commun. 284, 966–971. doi:10.1006/bbrc.2001.5058
Philpott, M. P., Sanders, D. A., and Kealey, T. (1994). Effects of insulin and insulin-like growth factors on cultured human hair follicles: IGF-I at physiologic concentrations is an important regulator of hair follicle growth in vitro. J. Invest. Dermatol. 102, 857–861. doi:10.1111/1523-1747.ep12382494
Price, V. H. (1999). Treatment of hair loss. N. Engl. J. Med. 341, 964–973. doi:10.1056/NEJM199909233411307
Randall, V. A. (2008). Androgens and hair growth. Dermatol. Ther. 21, 314–328. doi:10.1111/j.1529-8019.2008.00214.x
Re, R., Pellegrini, N., Proteggente, A., Pannala, A., Yang, M., Rice-Evans, C., et al. (1999). Antioxidant activity applying an improved ABTS radical cation decolorization assay. Free Rad. Biol. Med. 26, 1231–1237. doi:10.1016/s0891-5849(98)00315-3
Sedlak, T. W., Oltvai, Z. N., Yang, E., Wang, K., Boise, L. H., Thompson, C. B., et al. (1995). Multiple Bcl-2 family members demonstrate selective dimerizations with Bax. Proc. Natl. Acad. Sci. U. S. A. 92, 7834–7838. doi:10.1073/pnas.92.17.7834
Shin, H., Yoo, H. G., Inui, S., Itami, S., Kim, I. G., Cho, A.-R., et al. (2013). Induction of transforming growth factor-beta 1 by androgen is mediated by reactive oxygen species in hair follicle dermal papilla cells. BMB Rep. 46, 460–464. doi:10.5483/BMBRep.2013.46.9.228
Si, C.-L., Deng, X.-J., Liu, Z., Kim, J.-K., and Bae, Y.-S. (2008a). Studies on the phenylethanoid glycosides with anti-complement activity from Paulownia tomentosa var. tomentosa wood. J. Asian Nat. Prod. Res. 10, 1003–1008. doi:10.1080/10286020802242364
Si, C.-L., Jiang, J.-Z., Liu, S.-C., Hu, H.-Y., Ren, X.-D., Yu, G.-J., et al. (2013a). A new lignan glycoside and phenolics from the branch wood of Pinus banksiana Lambert. Holzforschung 67, 357–363. doi:10.1515/hf-2012-0137
Si, C.-L., Kim, J.-K., Bae, Y.-S., and Li, S.-M. (2009a). Phenolic compounds in the leaves of Populus ussuriensis and their antioxidant activities. Planta Med. 75, 1165–1167. doi:10.1055/s-0029-1185476
Si, C.-L., Liu, Z., Kim, J.-K., and Bae, Y.-S. (2008b). Structure elucidation of phenylethanoid glycosides from Paulownia tomentosa Steud. var. tomentosa wood. Holzforschung 62, 197–200. doi:10.1515/HF.2008.047
Si, C.-L., Shen, T., Jiang, Y.-Y., Wu, L., Yu, G.-J., Ren, X.-D., et al. (2013b). Antioxidant properties and neuroprotective effects of isocampneoside II on hydrogen peroxide-induced oxidative injury in PC12 cells. Food Chem. Toxicol. 59, 145–152. doi:10.1016/j.fct.2013.05.051
Si, C.-L., Wu, L., Zhu, Z.-Y., Kim, J.-K., Kwon, D.-J., and Bae, Y.-S. (2009b). Apigenin derivatives from Paulownia tomentosa Steud. var. tomentosa stem barks. Holzforschung 63 (4), 440–442. doi:10.1515/HF.2009.063
Si, C.-L., Wu, L., and Zhu, Z.-Y. (2009c). Phenolic glycosides from Populus davidiana bark. Biochem. Syst. Ecol. 37, 221–224. doi:10.1016/j.bse.2009.01.007
Si, C.-L., Xu, J., Kim, J.-K., Bae, Y.-S., Liu, P.-T., and Liu, Z. (2011). Antioxidant properties and structural analysis of phenolic glucosides from bark of Populus ussuriensis Kom. Wood Sci. Technol. 45, 5–13. doi:10.1007/s00226-009-0286-x
Sun, X., Chen, R. C., Yang, Z. H., Sun, G. B., Min, W., Ma, X. J., et al. (2014). Taxifolin prevents diabetic cardiomyopathy in vivo and in vitro by inhibition of oxidative stress and cell apoptosis. Food Chem. Toxicol. 63, 221–232. doi:10.1016/j.fct.2013.11.013
Sunil, C., and Xu, B. (2019). An insight into the health-promoting effects of taxifolin (dihydroquercetin). Phytochemistry 166, 112066. doi:10.1016/j.phytochem.2019.112066
Trüeb, R. M. (2002). Molecular mechanisms of androgenetic alopecia. Exp. Gerontol. 37, 981–990. doi:10.1016/S0531-5565(02)00093-1
Tsujimoto, Y., Finger, L. R., Yunis, J., Nowell, P. C., and Croce, C. M. (1984). Cloning of the chromosome breakpoint of neoplastic B cells with the t (14;18) chromosome translocation. Science 226, 1097–1099. doi:10.1126/science.6093263
Tucker, R. F., Shipley, G. D., Moses, H. L., and Holley, R. W. (1984). Growth inhibitor from BSC-1 cells closely related to platelet type β transforming growth factor. Science 226, 705–707. doi:10.1126/science.6093254
Wang, H., Du, H., Liu, K., Liu, H., Xu, T., Zhang, S., et al. (2021). Sustainable preparation of bifunctional cellulose nanocrystals via mixed H2SO4/formic acid hydrolysis. Carbohydr. Polym. 266, 118107. doi:10.1016/j.carbpol.2021.118107
Wang, H., Xie, H., Du, H., Wang, X., Liu, W., Duan, Y., et al. (2020). Highly efficient preparation of functional and thermostable cellulose nanocrystals via H2SO4 intensified acetic acid hydrolysis. Carbohydr. Polym. 239, 116233. doi:10.1016/j.carbpol.2020.116233
Wang, L., Luo, Y., Wu, Y., Liu, Y., and Wu, Z. (2018). Fermentation and complex enzyme hydrolysis for improving the total soluble phenolic contents, flavonoid aglycones contents and bio-activities of guava leaves tea. Food Chem. x. 264, 189–198. doi:10.1016/j.foodchem.2018.05.035
Weidmann, A. E. (2012). Dihydroquercetin: MoMinre than just an impurity? Eur. J. Pharmacol. 684, 19–26. doi:10.1016/j.ejphar.2012.03.035
Xie, H., Du, H., Yang, X., and Si, C. (2018). Recent strategies in preparation of cellulose nanocrystals and cellulose nanofibrils derived from raw cellulose materials. Int. J. Polym. Sci. 2018, 1–25. doi:10.1155/2018/7923068
Xie, H., Zou, Z., Du, H., Zhang, X., Wang, X., Yang, X., et al. (2019). Preparation of thermally stable and surface-functionalized cellulose nanocrystals via mixed H2SO4/Oxalic acid hydrolysis. Carbohydr. Polym. 223, 115116. doi:10.1016/j.carbpol.2019.115116
Xiong, R., Hua, D., Hoeck, J. V., Berdecka, D., Léger, M., Munter, S. D., et al. (2021a). Photothermal nanofibres enable safe engineering of therapeutic cells. Nat. Nanotechnol. 16, 1281–1291. doi:10.1038/s41565-021-00976-3
Xiong, R., Xu, R. X., Huang, C., De Smedt, S., and Braeckmans, K. (2021b). Stimuli-responsive nanobubbles for biomedical applications. Chem. Soc. Rev. 50, 5746–5776. doi:10.1039/C9CS00839J
Xu, J., Li, C., Dai, L., Xu, C., Zhong, Y., Yu, F., et al. (2020a). Biomass fractionation and lignin fractionation towards lignin valorization. Chemsuschem 13, 4284–4295. doi:10.1002/cssc.202001491
Xu, R., Du, H., Liu, C., Liu, H., Wu, M., Zhang, X., et al. (2021a). An efficient and magnetic adsorbent prepared in a dry process with enzymatic hydrolysis residues for wastewater treatment. J. Clean. Prod. 313, 127834. doi:10.1016/j.jclepro.2021.127834
Xu, R., Liu, K., Du, H., Liu, H., Cao, X., Zhao, X., et al. (2020b). Falling leaves return to their roots: A review on the preparation of gamma-valerolactone from lignocellulose and its application in the conversion of lignocellulose. Chemsuschem 13, 6461–6476. doi:10.1002/cssc.202002008
Xu, R., Si, C., Kong, F., and Li, X. (2020c). Synthesis of γ-valerolactone and its application in biomass conversion. J. For. Eng. 5, 20–28. doi:10.13360/j.issn.2096-1359.201904004
Xu, T., Du, H., Liu, H., Liu, W., Zhang, X., Si, C., et al. (2021b). Advanced nanocellulose-based composites for flexible functional energy storage devices. Adv. Mat. 33, 2101368. doi:10.1002/adma.202101368
Xu, T., Liu, K., Sheng, N., Zhang, M., Liu, W., Liu, H., et al. (2022). Biopolymer-based hydrogel electrolytes for advanced energy storage/conversion devices: Properties, applications, and perspectives. Energy Storage Mat. 48, 244–262. doi:10.1016/j.ensm.2022.03.013
Yang, X., Xie, H., Du, H., Zhang, X., Zou, Z., Zou, Y., et al. (2019). Facile extraction of thermally stable and dispersible cellulose nanocrystals with high yield via a green and recyclable FeCl3-catalyzed deep eutectic solvent system. ACS Sustain. Chem. Eng. 7, 7200–7208. doi:10.1021/acssuschemeng.9b00209
Yu, Y., Li, Y., Lou, Y., Liu, Y., Yu, H., and Zhong, Y. (2021). Effect of lignin condensation on cellulose enzymatic hydrolysis during deep eutectic solvent fractionation of lignocellulose. J. Forest. Eng. 6 (06), 101–108. doi:10.13360/j.issn.2096-1359.202104022
Zhang, M., Du, H., Liu, K., Nie, S., Xu, T., Zhang, X., et al. (2021). Fabrication and applications of cellulose-based nanogenerators. Adv. Compos. Hybrid. Mat. 4, 865–884. doi:10.1007/s42114-021-00312-2
Zheng, X., Zhao, Y., Pan, B., and Wang, X. (2021). Extraction, distribution and composition of tannin in Picea sitchensis. J. Forest. Eng. 6 (02), 108–113. doi:10.13360/j.issn.2096-1359.202007026
Keywords: Rhododendron mucrotulatum, taxifolin, enzyme hydrolysis, dihydrotestosterone, human follicle dermal papilla cells
Citation: Park S-M, He Y-C, Gong C, Gao W, Bae Y-S, Si C, Park K-H and Choi S-E (2022) Effects of taxifolin from enzymatic hydrolysis of Rhododendron mucrotulatum on hair growth promotion. Front. Bioeng. Biotechnol. 10:995238. doi: 10.3389/fbioe.2022.995238
Received: 15 July 2022; Accepted: 04 August 2022;
Published: 08 September 2022.
Edited by:
Weijun Yang, Jiangnan University, ChinaReviewed by:
Weicheng Hu, Huaiyin Normal University, ChinaGuiming Fu, Nanchang University, China
Rui Xu, Max Planck Institute of Colloids and Interfaces, Germany
Copyright © 2022 Park, He, Gong, Gao, Bae, Si, Park and Choi. This is an open-access article distributed under the terms of the Creative Commons Attribution License (CC BY). The use, distribution or reproduction in other forums is permitted, provided the original author(s) and the copyright owner(s) are credited and that the original publication in this journal is cited, in accordance with accepted academic practice. No use, distribution or reproduction is permitted which does not comply with these terms.
*Correspondence: Chuanling Si, c2ljaGxpQHR1c3QuZWR1LmNu; Kwang-Hyun Park, a2hwYXJrQG5hbWJ1LmFjLmty; Sun-Eun Choi, b3JlZ29uaW5Aa2FuZ3dvbi5hYy5rciYjeDAyMDBhOw==
†These authors have contributed equally to this work and share first authorship