- 1Experimental Ophthalmology Group, IMIB-Arrixaca, University of Murcia, Murcia, Spain
- 2Institute of Biophysics and Institute for Clinical and Biomedical Research (iCBR), Area of Environment Genetics and Oncobiology (CIMAGO), Faculty of Medicine, University of Coimbra, Coimbra, Portugal
- 3Center for Innovative Biomedicine and Biotechnology (CIBB), University of Coimbra, Coimbra, Portugal
- 4Clinical and Academic Centre of Coimbra (CACC), Coimbra, Portugal
- 5Department of Obstetrics and Feto-maternal Medicine, Inselspital, Bern University Hospital, University of Bern, Bern, Switzerland
- 6Department for BioMedical Research (DBMR), University of Bern, Bern, Switzerland
- 7Ophthalmology Department, Centro Hospitalar e Universitário de Coimbra, Coimbra, Portugal
- 8Ophtalmology Universitary Clinic and Institute for Clinical and Biomedical Research (iCBR), Faculty of Medicine, University of Coimbra, Coimbra, Portugal
- 9Laboratory of Experimental Hematology, Vaccine and Infectious Disease Institute (Vaxinfectio), University of Antwerp, Antwerp, Belgium
Perinatal derivatives (PnD) are gaining interest as a source for cell-based therapies. Since the eye is easily accessible to local administration, eye diseases may be excellent candidates to evaluate novel therapeutic approaches. With this work, we performed a systematic review of published preclinical and clinical studies addressing PnD in the treatment of ocular diseases. We have set two specific objectives: (i) to investigate the current level of standardization in applied technical procedures in preclinical studies and (ii) to assess clinical efficacy in clinical trials. Hereto, we selected studies that applied amniotic membrane (hAM) and mesenchymal stromal cells derived from amniotic membrane (hAMSC), placenta (hPMSC), umbilical cord (hUC-MSC) and Wharton’s Jelly (hUC-WJ-MSC), excluding those where cells were not transplanted individually, following a systematic PubMed search for preclinical studies and consultation of clinical studies on https://clinicaltrials.gov and https://www.clinicaltrialsregister.eu/. Our bibliographic search retrieved 26 pre-clinical studies and 27 clinical trials. There was a considerable overlap regarding targeted ocular structures. Another common feature is the marked tendency towards (i) locally administered treatments and (ii) the PnD type. In the cornea/ocular surface, hAM was preferred and usually applied directly covering the ocular surface. For neuroretinal disorders, intra-ocular injection of umbilical or placental-derived cells was preferred. In general, basic research reported favourable outcomes. However, due to lack of standardization between different studies, until now there is no clear consensus regarding the fate of administered PnD or their mode of action. This might be accountable for the low index of clinical translation. Regarding clinical trials, only a minority provided results and a considerable proportion is in “unknown status”. Nevertheless, from the limited clinical evidence available, hAM proved beneficial in the symptomatic relief of bullous keratopathy, treating dry eye disease and preventing glaucoma drainage device tube exposure. Regarding neuroretinal diseases, application of Wharton’s Jelly MSC seems to become a promising future approach. In conclusion, PnD-based therapies seem to be beneficial in the treatment of several ocular diseases. However, much is yet to be done both in the pre-clinical and in the clinical setting before they can be included in the daily ophthalmic practice.
1 Introduction
Cell therapy encompasses a wide range of treatment strategies that use cells as therapeutic agents. Although cellular therapies have multiple beneficial properties, the rationale supporting the application of cellular medicines varies depending on the source of cells, the target organ or disease. Focusing on research using stem cells, based on their developmental status they can be classified into (i) embryonic stem cells (ESC) and (ii) adult stem cells (ASC). ESCs are extracted from the inner cell mass of blastocysts. They actively divide and applied as such are tumorigenic in vivo, which, together with their implicit ethical problems, means that they are of little benefit to patients in an undifferentiated stage. ASC encompass a wide variety of (stem) cell types that can be obtained postnatal from neonate to adulthood, but also from extra-embryonic perinatal tissues. Several examples of well-known ASC are those derived from bone marrow, dental pulp or adipose tissue (AT). They are widely investigated in novel regenerative medicine strategies because they can be isolated from the patient with little discomfort, and there are no ethical concerns. However, the efficacy of ASC therapy, apart from bone marrow transplantation for hematological malignancies, varies depending on their source, the age of the donors and, importantly, their health (Torre and Flores, 2020). On the other hand, perinatal derivatives (PnD) are prepared from fetal discarded annexes (i.e., amniotic membrane, placenta, umbilical cord). These tissues not only have been less exposed to infections and diseases, come from “young tissue” and are not burdened with ethical concerns, but also could be used as biological structure or scaffold (Abbaspanah et al., 2018).
Three decades ago, the first therapeutic mesenchymal stromal/stem cells (MSC) were isolated from bone-marrow (BM) (Moll et al., 2019). However, throughout the years, MSC originating in other locations have gained increasing interest, with PnD representing 27% and AT up to 22% of the chosen MSCs in registered clinical trials between 2008 and 2018 (Moll et al., 2019). But in contrast to BM, whose safety has been well established, AT and PnD have been associated with severe adverse effects when administered intra-vascularly, some of them fatal. These adverse reactions involve pro-thrombotic and pro-inflammatory mechanisms that were demonstrated to be linked with the presence of high levels of procoagulant Tissue Factor (TF) in those cells (Caplan et al., 2019; Moll et al., 2019; Moll et al., 2022). In addition, intra-vascular injection activates the host immune system, which on the one hand seems to convey the MSC immunomodulatory function, but on the other hand, promotes the sequestration and inactivation of MSC, thereby decreasing their potential therapeutic effect (Moll et al., 2019).
There is an increasing body of evidence from pre-clinical and clinical studies showing that those deleterious effects can be minimized with several strategies, such as modifying MSC manufacture, improving MSC characterization, establishing optimal concentrations and administering simultaneous anticoagulant therapy (Moll et al., 2019; Caplan et al., 2019; Moll et al., 2022). In this context, local administration via direct injection in the target organ/tissue, when possible, presents some advantages. In theory, it can eliminate the need for hemocompatibility and minimize the risk of life-threatening complications [thrombosis and severe systemic inflammatory reaction—Instant Blood-Mediated Inflammatory Reaction (IBMIR)]. Over the years, there has been a tendency among clinical trials to move from intra-vascular delivery to locally administered treatments, with the latter being the choice in about half of the clinical trials currently performed (Moll et al., 2019).
The eye is, per se, considered a sanctuary, protected from the bloodstream by hemato-ocular barriers, rendering him a target difficult to reach endovenously, similarly to central nervous system (Caplan et al., 2019). On the other hand, it is easily accessible, either topically or by intra-ocular administration (intra-vitreal, intra-cameral, subconjunctival, subtenon injection). These surgical procedures are widely and routinely performed worldwide, in an outpatient basis and are well tolerated, with little discomfort. Local administration to the eye easily bypasses the ocular barriers, probably increasing cell delivery efficiency and lowering the incidence of complications (Caplan et al., 2019), which makes the eye a suitable candidate for PnD-based therapies.
With this work, we aimed to perform a systematic review of published preclinical and clinical studies involving therapeutic approaches of PnD in the field of ocular diseases. We have set forward two specific objectives: (i) to investigate the current level of standardization in applied technical procedures, regarding cell therapeutic interventions in preclinical studies, and (ii) to assess how clinical efficacy of PnD was evaluated in view of current and future clinical relevance
This work was performed in the framework of The International Network for Translating Research on Perinatal Derivatives into Therapeutic Approaches (SPRINT, CA17116), funded by COST (European Cooperation in Science and Technology).
2 Materials and methods
We performed a review of published preclinical and clinical studies selecting those that used perinatal derivatives (PnD). We included amniotic membrane and mesenchymal stromal cells derived from amniotic membrane (hAMSC), placenta (hPMSC), umbilical cord (hUC-MSC) and Wharton’s jelly (hUC-WJ-MSC), excluding those where cells were not transplanted individually or were not used in combination with other derivatives such as conditioned medium.
For preclinical studies, the SPRINT consortium performed a systematic search of the PubMed database (Linares-Espinós et al., 2018) using a Boolean search string including perinatal derivatives such as tissues, membranes, cells, and secretome derived from them, but excluding cord blood and hematopoietic products, as well as non-original publications. The search string was supplemented with terms covering preclinical animal or in vivo models, and publications from 2004 to the present were collected in a searchable database as described in detail elsewhere (https://doi.org/10.5281/zenodo.6334077). The filter “ophthalmology OR eye OR vision OR retina OR cornea” was then used to select preclinical studies in the ophthalmology field, that were manually curated to exclude adult MSC.
The search for Clinical Trials (CTs) using PnD in the treatment of ocular conditions was carried out in the https://clinicaltrials.gov and https://www.clinicaltrialsregister.eu/databases, using the following terms: “Ophthalmopathy OR ocular OR vision OR eye OR ophthalmic OR Eye Disease OR Eye OR Ocular OR optic OR retina AND mesenchymal”. Next, the retrieved CTs (n = 41) were manually curated to exclude those testing adult MSCs (n = 14) (Supplementary Figure S1). In addition, CTs that used hAM as a carrier for other cell types, such as in limbal cell transplant, were excluded from this search, since their primary goal was not the use of AM. We also excluded CTs that had been withdrawn. Data were analyzed and plotted using GraphPad Prism 9 (GraphPad, San Diego, CA, United States).
3 Results
Our bibliographic search, as outlined in Materials and Methods section, retrieved 26 pre-clinical studies and 27 randomized clinical trials (CTs), that are listed in Tables 1, 2.
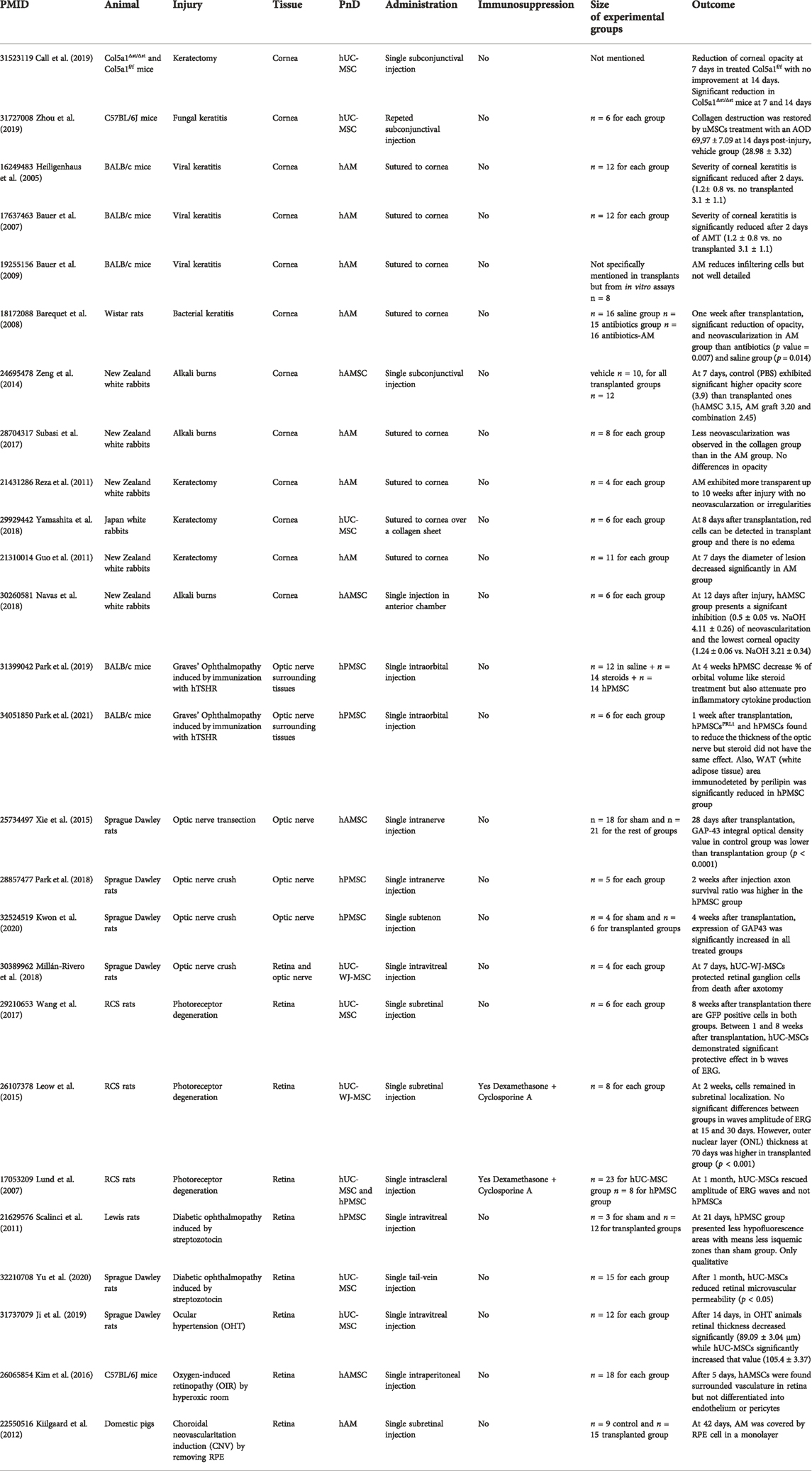
TABLE 1. Papers selected for pre-clinical studies and PnD analysis. (PMID: PubMed ID; PnD: Perinatal Derivates; RCS: Royal College Surgeons; hUC-MSC: human Umbilical Cord Mesenchymal Stromal Cells; hAM: human Amniotic Membrane; hAMSC: human Amniotic Membrane Mesenchymal Stromal Cells; hPMSC: human Placenta Mesenchymal Stromal Cells; hUC-WJ-MSC: human Umbilical Cord Wharton’s Jelly Mesenchymal Stromal Cells).
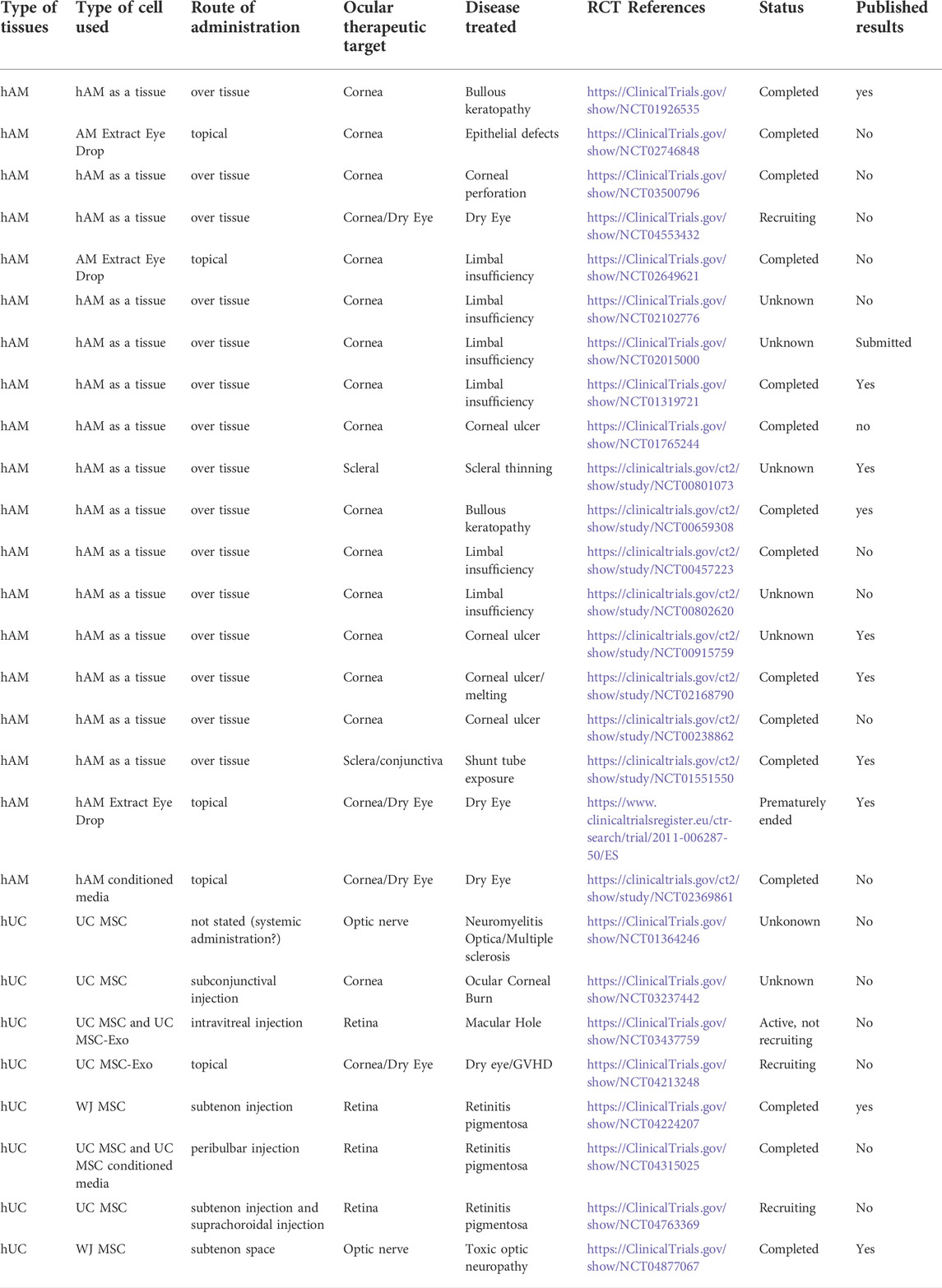
TABLE 2. Clinical trials selected for the present review. (hAM: human Amniotic Membrane; hAMSC: human Amniotic Membrane Mesenchymal Stromal Cells; hUC-MSC: human Umbilical Cord Mesenchymal Stromal Cells; hUC-WJ-MSC: human Umbilical Cord Wharton’s Jelly Mesenchymal Stromal Cells).
Both pre-clinical studies and CTs addressed diseases of the cornea/ocular surface and the retina/optic nerve (Figure 1). No other ocular structure was object of study.
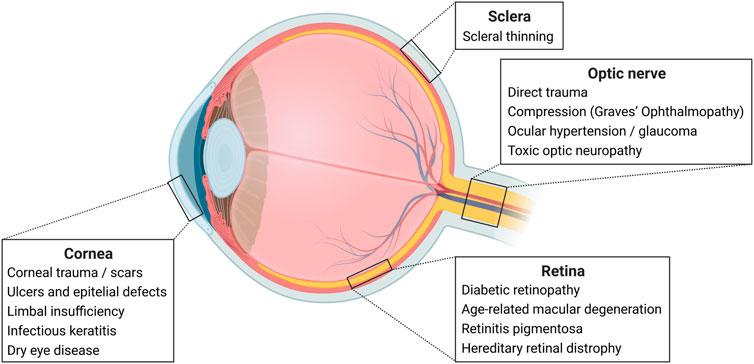
FIGURE 1. Ocular Anatomy and Diseases. Examples of eye diseases per ocular structure. Created with BioRender.com.
3.1 Preclinical studies: Animal models
Most of the reports studied the effects of PnD in rats (n = 11), mice (n = 8) and rabbits (n = 6). Only one of them used higher mammals, namely domestic pigs, to assess the effect of amniotic membrane in retinal neovascularization (Kiilgaard et al., 2012). The used species and applied disease models are summarized in Table 1 and Figure 2.
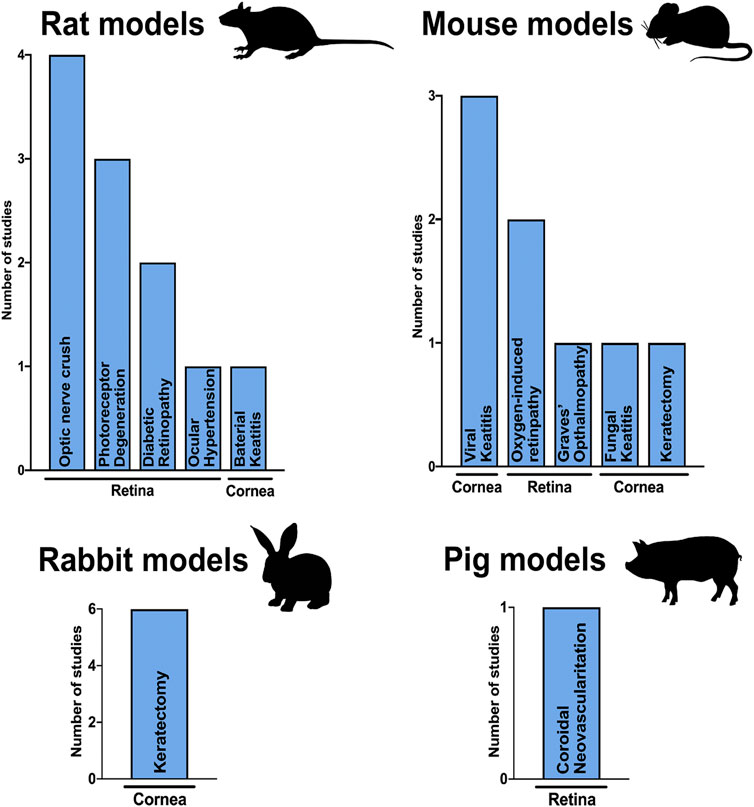
FIGURE 2. Animal models. Column graphs showing the species used to test PnDs in ophthalmology and the number of articles published for every injury model in the cornea and retina.
Rabbits are exclusively used as keratectomy model (Figure 2), where corneal ablation is performed by placing a NaOH soaked disk over the cornea (Guo et al., 2011; Reza et al., 2011; Zeng et al., 2014; Subasi et al., 2017; Navas et al., 2018; Yamashita et al., 2018).
Rodents are the most common model to study either keratitis using bacterial (Barequet et al., 2008), fungal (Zhou et al., 2019) or viral infection (Heiligenhaus et al., 2005; Bauer et al., 2007; Barequet et al., 2008) and retinal injuries or diseases such as photoreceptor degeneration (Lund et al., 2007; Leow et al., 2015; Wang et al., 2017), optic nerve trauma (Xie et al., 2015; Millán-Rivero et al., 2018; Park et al., 2018; Kwon et al., 2020), hypoxia (Kim et al., 2016), ocular hypertension (Ji et al., 2019), Grave’s ophthalmopathy (Park et al., 2019, 2021) and diabetic retinopathy (Scalinci et al., 2011; Yu et al., 2020).
All selected papers analyzed a large enough sampling size (12 papers had a “number of animals” higher than 10) to perform consistent statistical analyses. Furthermore, according to the ethical committees’ recommendations, most of the studies explicitly mentioned the age or weight of animals, although neither explored how transplantation effects differ among interindividual variables.
3.2 Preclinical studies: Type of cells and route of administration
As we expected for their translational potential to the clinic, all the selected studies used human PnDs (Figure 3); thus, in these works, PnDs were xenotransplanted. Surprisingly, among all 26 studies, only two applied an immunosuppressive regimen (Lund et al., 2007; Leow et al., 2015).
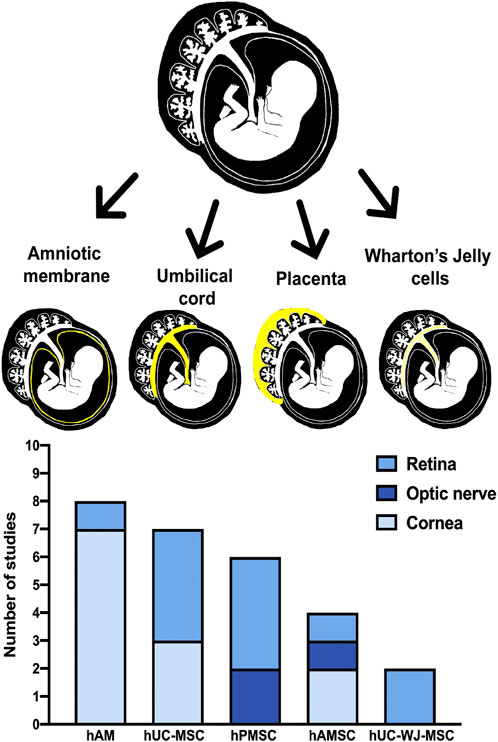
FIGURE 3. Types of PnD. Top, a schematic picture of different origins of fetal tissue for each PnD selected in this review. Below, grouped column graph showing the number of publications using each PnD type in retina, optic nerve, and cornea. (hAM: human Amniotic Membrane; hUC-MSC: human Umbilical Cord Mesenchymal Stromal Cells; hPMSC: human Placenta Mesenchymal Stroma Cells; hAMSC: human Amniotic Membrane Mesenchymal Stromal Cells; hUC-WJ-MSC: human Umbilical Cord Wharton’s Jelly Mesenchymal Stromal Cells).
Regarding corneal diseases, we identified 12 studies using PnD: five aiming to treat corneal infections, four evaluating corneal epithelialization in keratectomy models and three evaluating its effect in alkali burns. The majority (n = 8) was treated with hAM as an over tissue, three with subconjunctival injections of hUC-MSC or hAMSC and one with an intra-cameral injection of hAMSC (Heiligenhaus et al., 2005; Bauer et al., 2007, 2009; Barequet et al., 2008; Guo et al., 2011; Reza et al., 2011; Kiilgaard et al., 2012; Subasi et al., 2017).
There were 14 studies focusing on retinal and optic nerve disorders. In contrast to corneal pathologies, umbilical cord derived cells were preferred (n = 6), followed by hPMSC (n = 5) and hAMSC (n = 2).
In retinal diseases (n = 8), subretinal injections (n = 3) and intra-vitreal injections (n = 2) were the most frequently performed procedures. Of notice, there were two studies where cells were systemically administered, either via the tail or injected in intra-peritoneal space. In none of those two studies systemic immunosuppression was administered. (Lund et al., 2007; Wang et al., 2017; Yamashita et al., 2018; Call et al., 2019; Ji et al., 2019; Zhou et al., 2019; Yu et al., 2020) (Lund et al., 2007; Scalinci et al., 2011; Park et al., 2018, 2019, 2021; Kwon et al., 2020).
In optic nerve disorders (n = 6), cells were injected directly in the nerve (n = 2), in the orbit (n = 2), in the subtenon space (n = 1) and in the vitreous (n = 1).
3.3 Pre-clinical studies: Cell fate and outcome reports
A major strength of preclinical animal models is that they allow to determine the cell fate of transplanted cells. However, only ten studies identified the graft post-mortem, using diverse techniques that include immunofluorescence (Millán-Rivero et al., 2018), membrane staining with lipophilic fluorophores (Scalinci et al., 2011; Yamashita et al., 2018; Kwon et al., 2020) or nuclei stains (Xie et al., 2015; Navas et al., 2018). Of notice, Kim et al., 2016 proved that intraperitoneally injected hAMSCs can migrate into the retina, resulting in supressed neovascularization in the retina. The rest of studies that identified cells agreed on transplanted cells remaining in the local of injection (Millán-Rivero et al., 2018; Kwon et al., 2020), and their effects are locally potentiated but detectable all over retina.
Regarding the route of administration, most of the studies report beneficial effects of PnD when applied locally. The two studies that investigated systemic administration also report beneficial effects, with no severe adverse reactions (Kim et al., 2016; Yu et al., 2020). More concerning is the absence of consensus regarding the optimal time points for analyses, so that positive effects can be reported as early as 2 days post-administration (Heiligenhaus et al., 2005; Bauer et al., 2007) or 2 months (Reza et al., 2011; Wang et al., 2017) after transplantation. Furthermore, the approach for measuring the beneficial effect of PnD transplants also lacks consensus. As shown in Figure 4, we selected those studies that assessed functional or anatomical outcomes in the same animal model and matched time points: for cornea, the clinical score for improvement was the corneal opacity after transplant (Heiligenhaus et al., 2005; Zeng et al., 2014; Navas et al., 2018; Yamashita et al., 2018) and for the retina, recordings of the b-wave in electroretinograms that measure post photoreceptors synapsis (Lund et al., 2007; Leow et al., 2015; Wang et al., 2017). In the studies graphed in Figure 4, PnD displayed a beneficial impact in decreasing corneal opacity, increasing the b-wave amplitude and ONL thickness, but results differed depending on PnD tissue origin. However, no clear statement can be made due to the low number of studies.
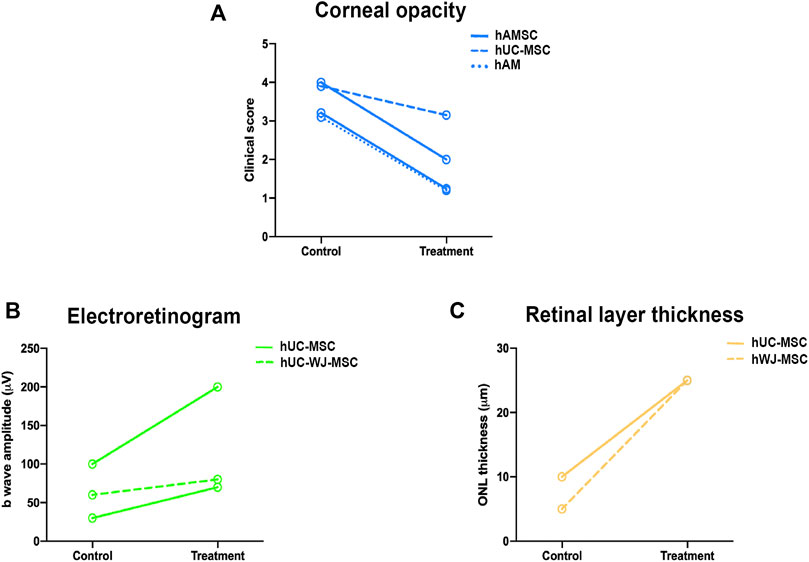
FIGURE 4. Preclinical scores to measure PnD therapeutic success. Graphs representing the functional or anatomical changes after PnD treatment between control and treated groups. Clinical score measuring corneal opacity in keratitis corneal model 15 days after the transplant (A), b wave amplitude (µV) recorded from rats with retinal dystrophy measured 30 days after injection (B) and measurement of the thickness of outer nuclear layer (ONL) (µm) in the same model but measured at 60 days after injection (C). (hAM: human Amniotic Membrane; hUC-MSC: human Umbilical Cord Mesenchymal Stromal Cells; hAMSC: human Amniotic Membrane Mesenchymal Stromal Cells; hUC-WJ-MSC: human Umbilical Cord Wharton’s Jelly Mesenchymal Stromal Cells).
3.4 PnD in clinical trials
Our curated search for Clinical Trials (CT) retrieved 27 registries, that are summarized in Table 2. The vast majority addresses the use of human amniotic membrane, either as a tissue (n = 16) or as an extract/conditioned medium eye drop (n = 3). The remaining trials evaluate the use of umbilical cord mesenchymal stromal cells (hUC-MSC) (n = 3), Wharton’s Jelly MSC (hUC-WJ-MSC) (n = 2), hUC-MSC-derived extracellular vesicles (n = 1), hUC-MSC plus hUC-MSC-derived extracellular vesicles (n = 1) and hUC-MSC plus hUC-MSC-conditioned media (n = 1) (Figure 5).
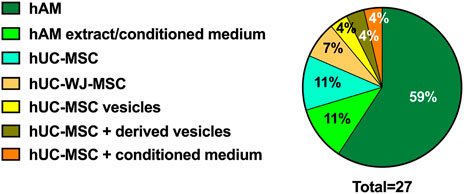
FIGURE 5. Randomized Clinical Trials (RCT) and PnD used. Pie graph representing the type of PnD used in RCTs.
The majority of CTs have used the cornea as a therapeutic target (n = 19) and are designed to treat limbal insufficiency (n = 7), epithelial defects and ulcers (n = 5), bullous keratopathy (n = 2), dry eye disease (n = 3), and corneal perforations (n = 1). The usefulness of hAM in treating scleral/conjunctival thinning is the object of two CTs (Figure 6).
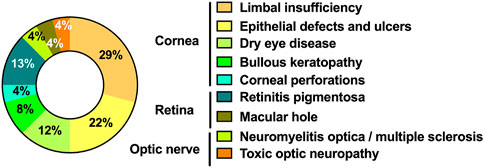
FIGURE 6. Ophtalmic diseases object of RCT grouped by ocular target. Circular graph representing the distribution of ocular diseases that were object of RCT.
The remaining six CT are designed to treat retinal diseases (n = 4) and optic neuropathies (n = 2): retinitis pigmentosa (n = 3), macular hole (n = 1), neuromyelitis optica/multiple sclerosis (n = 1) and toxic optic neuropathy (n = 1) (Figure 6). Of notice, all these six RCT are designed to evaluate the therapeutic value of umbilical cord and Wharton’s Jelly-derived products, rather than hAM, which is not a choice in treating these diseases (Figure 7).
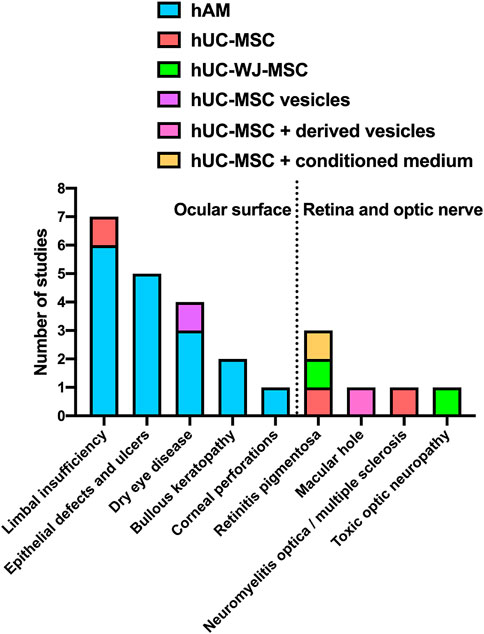
FIGURE 7. Type of PnD used in every disease. Column graph representing the type of PnD used in each pathology; the dashed line separates pathologies associated with the ocular surface on the left and the retina or optic nerve on the right.
In fact, it becomes apparent from Figure 7 that hAM is the PnD of choice to treat ocular surface conditions (the columns to the left of the dashed line), while retinal and optic nerve diseases are predominantly treated with hUC and hUC-WJ derivatives. The route of administration is inherent to that choice: while ocular surface conditions are treated with hAM applied to the surface or with PnD eyedrops, the retina and the optic nerve are not that easily accessible and therefore require PnD to be delivered through subconjunctival, subtenon, periocular or intravitreal injection (Table 2).
Fifteen of those 27 RCT are currently completed, three are still recruiting, one is active but not recruiting, and seven have an unknown recruiting status (Figure 8). Surprisingly, only ten RCT have published results.
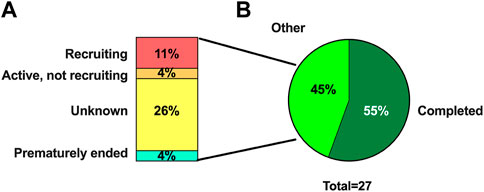
FIGURE 8. Recruiting status of RCT. Column graph (A) representing percentage of RCT not completed and circular graph (B) with percentage of RCT completed and not completed of twenty-seven RCT included.
Available results are compiled in Table 3 and detailed below, by pathology.
3.4.1 Bullous keratopathy
Two RCT evaluate the use of transplanted hAM in the management of BK (NCT01926535; NCT00659308). Both seem to favour hAM: hAM outperforms therapeutic contact lenses in pain relief up to 6 months and is more effective in achieving a regular epithelium at 6 months, compared to anterior stromal puncture.
3.4.2 Pterygium
One randomized, interventional, and comparative study on recurrent pterygium surgery compared conjunctival-limbal autograft (CLAU) with hAM and concluded the superiority of CLAU in all parameters evaluated (healing of epithelial defect, post-operative conjunctival inflammation, and recurrence rate at 1 year) (NCT01319721).
3.4.3 Scleral thinning
A randomized, interventional, comparative study compared hAM with pericardial graft covering to prevent glaucoma drainage device tube exposure and found that hAM was more effective at the 2-year time point (NCT01551550).
Another RCT compared the use of multilayer amniotic membrane transplantation (AMT) with lamellar corneal transplantation (LCT) and lamellar scleral transplantation (LST) for the treatment of scleral thinning after pterygium surgery (NCT00801073). It found that AMT performed significantly worse than LCT or LST. A high rate of reabsorption was noted with AMT, which was the least effective of the three therapeutic options and should not be used for this condition.
3.4.4 Corneal ulcer
Two RCTs evaluated the role of sutureless hAM in the treatment of corneal ulcers. One was designed to prove its safety and wearability as a prerequisite to obtaining regulatory approval. Eight eyes of seven patients were enrolled and showed good tolerance with no complications (NCT02168790).
The largest RCT compared hAM and bandage contact lens (BCL) in aiding corneal re-epithelialization after photorefractive keratectomy in 40 patients. One eye of each patient was treated with hAM, and the other eye with BCL. Published results show shorter healing times for BCL, but no statistical analysis was performed, so clear conclusions cannot be drawn (NCT00915759).
3.4.5 Dry eye disease
One phase III comparative clinical trial evaluated the efficacy of amniotic membrane extract for treating severe dry eye disease compared with autologous serum eyedrops (2011-006287-50). Among the group of 12 patients enrolled, no statistical differences were noted between the two treatments. Since autologous serum eyedrops are a world-wide accepted treatment option for dry eye disease, this RCT proved the non-inferiority of hAM extract.
3.4.6 Neuroretinal diseases
There are two RCTs with the focus on neuroretinal disease that have published results, both using hUC-WJ-MSC.
One prospective, sequential, open-label phase III clinical study enrolled 34 eyes of 32 patients with retinitis pigmentosa (RP) (NCT04224207). In this RCT, subtenon transplantation of WJ-MSCs was effective and safe in the treatment of RP during the first year.
Similarly, the beneficial effect of hUC-WJ-MSC combined with electromagnetic stimulation in toxic optic neuropathy was evidenced in another RCT involving 36 eyes of 18 patients (NCT04877067).
4 Discussion
Our thorough review of pre-clinical studies and clinical trials has shown a considerable overlap with regard to the ocular structures being targeted. The cornea, the retina and the optic nerve are the main targets of PnD therapeutic interventions. Another common feature is the marked tendency towards locally administered treatments, as opposed to systemic delivery. This is in agreement with the current world-wide trends in mesenchymal stromal/stem cell clinical applications (Moll et al., 2019).
Another similarity between pre-clinical and clinical studies is the preferred cell type used: in the cornea and ocular surface, hAM is the PnD of choice, while retinal and optic nerve diseases are predominantly treated with umbilical cord derived and placenta mesenchymal stromal cells. This also implicitly conditions the route of administration, ocular surface conditions being treated with hAM applied as a tissue or with PnD eyedrops, while the retina and the optic nerve are treated with injected cells.
The existence of clinical trials register platforms is a scientific, ethical and moral responsibility (https://www.who.int/clinical-trials-registry-platform/network/trial-registration). Databases for clinical trials registration are a valuable source of information. However, we noticed that the follow-up is frequently missing and results are not published; it is very common to find clinical trials in “unknown status”. Having a follow-up from clinical failure studies could also help to re-drive pre-clinical and clinical works to better targets.
The cornea is a specialized, transparent tissue placed in the anterior part of the eye, that allows the passage of light to the retina, the light sensory tissue (Figure 1). Thus, corneal integrity and transparency are essential for a clear vision. Once damaged, the cornea’s ability to heal relies on stem cells located around its periphery, the so-called limbal stem cells. Not unfrequently, this healing process leads to scar formation and transparency loss, especially in extensive lesions and long-standing disease. PnD-based therapies have immunoregulatory properties that may be useful in modulating wound healing (Bukowiecki et al., 2017). Both pre-clinical studies and CTs have addressed this subject, and demonstrated that PnDs are useful in corneal re-epithelialization in keratectomy animal models. In the clinical setting, hAM was proven useful in the symptomatic relief of bullous keratopathy and was well tolerated and safe in treating corneal ulcers and epithelial defects; however, it did not seem to outperform bandage contact lenses—only one CT with published results was performed, and it seemed to favour contact lens wear compared to hAM, but did not present statistical analysis.
Limbal insufficiency is an ocular surface disease involving the cornea that has attracted the attention of basic and clinical researchers. It is a devastating condition often leading to blindness, that is mostly caused by chemical injuries and typically affects young males, that are more prone to that kind of accidents (Deng et al., 2019). In limbal insufficiency, the cornea becomes opaque and neovascularized, which erodes ocular immune privilege (Bukowiecki et al., 2017) and therefore turns corneal grafts into very high-risk procedures due to the increased risk of rejection. The potential beneficial effect of PnD was evaluated in animal alkali burn models, with favourable results. Regarding clinical trials, there were seven registered studies. Of those, only one had their results published, and it evaluated pterygium, a localized form of limbal insufficiency that does not entail the severity of chemical burns. In pterygia surgery, hAM presented poor results, but the comparative treatment was conjunctival-limbal autograft, whose superiority is well known since it contains limbal stem cells.
There are other diseases of the ocular surface where the treatment with biological products has proven benefits. That is the case of dry eye disease, where the treatment with blood derived products is beneficial, due to the presence of growth factors, among other molecules, and is part of the current treatment guidelines (Jones et al., 2017). The discovery of other biological options that might be even more effective and wider available could be advantageous. In fact, hAM extract can be an option in treating dry eye disease, since a CT proved its non-inferiority compared to autologous serum eyedrops, a gold-standard treatment for this condition. hAM also proved helpful in preventing glaucoma drainage device tube exposure. On the contrary, a CT has shown that in the case of scleral thinning, the use of hAM should be discouraged since there are better alternatives (corneal or scleral grafts).
The optic nerve and retina are projections of the brain and therefore part of the central nervous system. They are highly specialized tissues where neuronal death can occur as a result of varied diseases: age-related macular degeneration (AMD), retinitis pigmentosa (RP), diabetic retinopathy (DR), trauma, ocular hypertension/glaucoma, etc. Regardless of their primary cause (mutations, aging, systemic disorder), these diseases share common underlying mechanisms, such as oxidative stress, excitotoxicity, inflammation, or cytokine imbalance (Holan et al., 2021). The current treatment for these diseases is varied, depending on the disease itself, but of limited success in visual recovery: it encompasses antiangiogenic intravitreal injections (for AMD and DR), laser applied to the retina and metabolic control (in DR), lowering intra-ocular pressure (in glaucoma), etc. There is currently no treatment available for end-stage retinal/optic nerve diseases, where the discovery of nerve cell regeneration therapies remains the holy grail. Of notice, two CT addressing neuroretinal diseases showed very promising results on the use of Wharton’s Jelly MSC, bringing hope to otherwise irreversible diseases. However, one must say that the current belief is that these cells do not differentiate into neurons, rather they facilitate neurorestorative mechanisms (Caplan et al., 2019).
When clinical trials and pre-clinical studies are compared, it becomes evident that research does not easily translate to the clinic, despite reported beneficial effects. That is especially noticeable in neuroretinal diseases, where the amount of CTs is considerably lower. Likely, this is related to the lack of standardization in terms of models, techniques, doses, optimal time points for evaluation and outcome measures of efficacy definition. The lack of similarity of the preclinical and the clinical settings, immunosuppressive treatments, or animal models that do not fully reflect human disease could also contribute to that discrepancy. Also noteworthy is that ophthalmic diseases are multifactorial and progress over time, so more longitudinal or chronic preclinical studies are needed, exploring diverse analyses such as visual tests, functional assessment, and cellular fate in the tissue, to support their use in patients. Other of the greatest advantages of preclinical studies is to be able to document the consequences of an intervention, both in vivo and ex vivo. In these types of therapies, it is very important to determine cell fate and histological changes that may occur, and that is only possible in the preclinical setting.
Furthermore, based on the data extracted from the included studies, it is difficult to extract a unique working mechanism to explain PnD advantages in the ophthalmology field. For some authors, the primary molecular mechanism involved is immunomodulation by the production of cytokines such as TGF-β or IL-10 (Barequet et al., 2008; Guo et al., 2011), the upregulation of essential survival pathways such as NF-κB (Park et al., 2018), and modifying cellular adhesion and motility (Lee et al., 2004). However, each PnD has different properties and that needs to be addressed.
In order to gather all available data on PnD, the SPRINT consortium established a publication database on the Mendeley Reference Manager platform (https://www.mendeley.com/) and collected outcome data from selected publications in an Access database (Microsoft, Redmont WA, United States of America), linking PnD types, dosage, route and time point of applications to functional effects. However, both databases remain to be further implemented in the scientific community (e.g. by the International Placenta Stem Cell Society, IPLASS).
Recently, also new non-cellular therapies have emerged to replace certain limitations of the use of live cells, such as maintaining viability upon allogeneic administration. In particular, extracellular vesicles derived from mesenchymal stromal cells are showing promising results (Thomi et al., 2019). However, it is difficult to draw conclusions about a common beneficial dose to treat a disease. For that, advancing our knowledge of cell-based regenerative mechanisms is still critical to reaching success in eye diseases.
5 Conclusion
The eye is a good candidate for PnD-based therapies and ocular diseases have gained attention from both pre-clinical and clinical researchers. However, the number of studies is considerably low, a sign that much work is still to be done.
Selected pre-clinical studies and clinical trials converge in a number of features. They targeted the same ocular structures (cornea/ocular surface and retina/optic nerve). They tended to choose the same type of PnD according to the target tissue and also their route of administration. In the cornea/ocular surface, hAM was preferred and usually applied directly covering the ocular surface. In neuroretinal disorders, cells of umbilical or placental origin were injected in the eye.
In general, basic research reported favourable outcomes in all animal models of disease treated with PnD. From the clinical perspective, hAM proved useful in the symptomatic relief of bullous keratopathy, treating dry eye disease and in preventing glaucoma drainage device tube exposure. hAM was well tolerated and safe in treating corneal ulcers and epithelial defects; however, it did not seem to outperform bandage contact lenses. In pterygia surgery and in scleral thinning, the use of isolated hAM should be discouraged. Regarding neuroretinal diseases, the use of Wharton’s Jelly MSC seems very promising, giving hope to otherwise irreversible diseases.
Pre-clinical studies are very important because they ought to provide necessary evidence supporting translation to the clinic. This includes efficacy and safety data, as well as insights into mechanisms of action. What stands out from our selection of pre-clinical studies is a lack of standardization among them. Also, until now, they have failed to provide an explanation on how exactly do these PnD work and their fate.
Clinical trials register in dedicated platforms is a valuable source of information. Surprisingly, the effort to register clinical trials is not accompanied by the effort to keep theirs records updated. Only a minority of CT provided results, despite displaying “complete status” and there are some whose status is “unknown”. Also, in the case of “withdrawn” CT, providing an explanation for this fact should be encouraged. Negative results are as important as positive results and becoming aware of them contributes to evolving knowledge.
In conclusion, PnD-based therapies seem to be beneficial in the treatment of several ocular diseases. However, much needs to be done both in the pre-clinical and in the clinical setting before they can be included in the daily ophthalmic practice.
Author contributions
MN-M, EC, MA-B, and AS analyzed the data and drafted the manuscript. PP, MB, and JN contributed to revising and editing the article and all authors approved the submitted version.
Funding
This review is based on work from the COST Action CA17116 “International Network for Translating Research on Perinatal Derivatives into Therapeutic Approaches (SPRINT)”, supported by COST (European Cooperation in Science and Technology). This study was supported by the Spanish Ministry of Economy and Competitiveness, Instituto de Salud Carlos III, Fondo Europeo de Desarrollo Regional “Una manera de hacer Europa” project: PI19/00071 (MA-B).
Conflict of interest
The authors declare that the research was conducted in the absence of any commercial or financial relationships that could be construed as a potential conflict of interest.
Publisher’s note
All claims expressed in this article are solely those of the authors and do not necessarily represent those of their affiliated organizations, or those of the publisher, the editors and the reviewers. Any product that may be evaluated in this article, or claim that may be made by its manufacturer, is not guaranteed or endorsed by the publisher.
Supplementary material
The Supplementary Material for this article can be found online at: https://www.frontiersin.org/articles/10.3389/fbioe.2022.969927/full#supplementary-material
References
Abbaspanah, B., Momeni, M., Ebrahimi, M., and y Mousavi, S. H. (2018). Advances in perinatal stem cells research: A precious cell source for clinical applications. Regen. Med. 13, 595–610. doi:10.2217/rme-2018-0019
Barequet, I. S., Habot-Wilner, Z., Keller, N., Smollan, G., Ziv, H., Belkin, M., et al. (2008). Effect of amniotic membrane transplantation on the healing of bacterial keratitis. Invest. Ophthalmol. Vis. Sci. 49, 163–167. doi:10.1167/iovs.07-1005
Bauer, D., Wasmuth, S., Hennig, M., Baehler, H., Steuhl, K.-P., and y Heiligenhaus, A. (2009). Amniotic membrane transplantation induces apoptosis in T lymphocytes in murine corneas with experimental herpetic stromal keratitis. Invest. Ophthalmol. Vis. Sci. 50, 3188–3198. doi:10.1167/iovs.08-3041
Bauer, D., Wasmuth, S., Hermans, P., Hennig, M., Meller, K., Meller, D., et al. (2007). On the influence of neutrophils in corneas with necrotizing HSV-1 keratitis following amniotic membrane transplantation. Exp. Eye Res. 85, 335–345. doi:10.1016/j.exer.2007.05.009
Bukowiecki, A., Hos, D., Cursiefen, C., and y Eming, S. A. (2017). Wound-healing studies in cornea and skin: Parallels, differences and opportunities. Int. J. Mol. Sci. 18, 1257. doi:10.3390/ijms18061257
Call, M., Elzarka, M., Kunesh, M., Hura, N., Birk, D. E., and y Kao, W. W. (2019). Therapeutic efficacy of mesenchymal stem cells for the treatment of congenital and acquired corneal opacity. Mol. Vis. 25, 415–426.
Caplan, H., Olson, S. D., Kumar, A., George, M., Prabhakara, K. S., Wenzel, P., et al. (2019). Mesenchymal stromal cell therapeutic delivery: Translational challenges to clinical application. Front. Immunol. 10, 1645. doi:10.3389/fimmu.2019.01645
Deng, S. X., Borderie, V., Chan, C. C., Dana, R., Figueiredo, F. C., Gomes, J. A. P., et al. (2019). Global consensus on definition, classification, diagnosis, and staging of limbal stem cell deficiency. Cornea 38, 364–375. doi:10.1097/ICO.0000000000001820
Guo, Q., Hao, J., Yang, Q., Guan, L., Ouyang, S., and Wang, y. J. (2011). A comparison of the effectiveness between amniotic membrane homogenate and transplanted amniotic membrane in healing corneal damage in a rabbit model. Acta Ophthalmol. 89, e315–e319. doi:10.1111/j.1755-3768.2010.02097.x
Heiligenhaus, A., Li, H. F., Yang, Y., Wasmuth, S., Steuhl, K. P., and y Bauer, D. (2005). Transplantation of amniotic membrane in murine herpes stromal keratitis modulates matrix metalloproteinases in the cornea. Invest. Ophthalmol. Vis. Sci. 46, 4079–4085. doi:10.1167/iovs.05-0192
Holan, V., Palacka, K., and y Hermankova, B. (2021). Mesenchymal stem cell-based therapy for retinal degenerative diseases: Experimental models and clinical trials. Cells 10 (3), 588. doi:10.3390/cells10030588
Ji, S., Xiao, J., Liu, J., and y Tang, S. (2019). Human umbilical cord mesenchymal stem cells attenuate ocular hypertension-induced retinal neuroinflammation via toll-like receptor 4 pathway. Stem Cells Int. 2019, 1–17. doi:10.1155/2019/9274585
Jones, L., Downie, L. E., Korb, D., Benitez-Del-Castillo, J. M., Dana, R., Deng, S. X., et al. (2017). TFOS DEWS II management and therapy report. Ocul. Surf. 15, 575–628. doi:10.1016/j.jtos.2017.05.006
Kiilgaard, J. F., Scherfig, E., Prause, J. U., and y la Cour, M. (2012). Transplantation of amniotic membrane to the subretinal space in pigs. Stem Cells Int. 2012, 1–5. doi:10.1155/2012/716968
Kim, K.-S., Park, J.-M., Kong, T., Kim, C., Bae, S.-H., Kim, H. W., et al. (2016). Retinal angiogenesis effects of TGF-β1 and paracrine factors secreted from human placental stem cells in response to a pathological environment. Cell Transpl. 25, 1145–1157. doi:10.3727/096368915X688263
Kwon, H., Park, M., Nepali, S., and y Lew, H. (2020). Hypoxia-preconditioned placenta-derived mesenchymal stem cells rescue optic nerve axons via differential roles of vascular endothelial growth factor in an optic nerve compression animal model. Mol. Neurobiol. 57, 3362–3375. doi:10.1007/s12035-020-01965-8
Lee, J. H., Lee, H. K., Kim, J. K., Chang, J. H., Kim, S. E., Kim, E. K., et al. (2004). Expression of laminin-5 with amniotic membrane transplantation in excimer laser ablated rat corneas. J. Cataract. Refract. Surg. 30, 2192–2199. doi:10.1016/j.jcrs.2004.02.021
Leow, S. N., Luu, C. D., Hairul Nizam, M. H., Mok, P. L., Ruhaslizan, R., Wong, H. S., et al. (2015). Safety and efficacy of human Wharton’s jelly-derived mesenchymal stem cells therapy for retinal degeneration. PLoS One 10, e0128973. doi:10.1371/journal.pone.0128973
Linares-Espinós, E., Hernández, V., Domínguez-Escrig, J. L., Fernández-Pello, S., Hevia, V., Mayor, J., et al. (2018). Methodology of a systematic review. Actas Urol. Espanolas 42, 499–506. doi:10.1016/j.acuroe.2018.07.002
Lund, R. D., Wang, S., Lu, B., Girman, S., Holmes, T., Sauvé, Y., et al. (2007). Cells isolated from umbilical cord tissue rescue photoreceptors and visual functions in a rodent model of retinal disease. Stem Cells 25, 602–611. doi:10.1634/stemcells.2006-0308
Millán-Rivero, J. E., Nadal-Nicolás, F. M., García-Bernal, D., Sobrado-Calvo, P., Blanquer, M., Moraleda, J. M., et al. (2018). Human Wharton’s jelly mesenchymal stem cells protect axotomized rat retinal ganglion cells via secretion of anti-inflammatory and neurotrophic factors. Sci. Rep. 8, 16299. doi:10.1038/s41598-018-34527-z
Moll, G., Ankrum, J. A., Kamhieh-Milz, J., Bieback, K., Ringdén, O., Volk, H.-D., et al. (2019). Intravascular mesenchymal stromal/stem cell therapy product diversification: Time for new clinical guidelines. Trends Mol. Med. 25, 149–163. doi:10.1016/j.molmed.2018.12.006
Moll, G., Ankrum, J. A., Olson, S. D., and y Nolta, J. A. (2022). Improved MSC minimal criteria to maximize patient safety: A Call to embrace tissue factor and hemocompatibility assessment of MSC products. Stem Cells Transl. Med. 11, 2–13. doi:10.1093/stcltm/szab005
Navas, A., Magaña-Guerrero, F. S., Domínguez-López, A., Chávez-García, C., Partido, G., Graue-Hernández, E. O., et al. (2018). Anti-inflammatory and anti-fibrotic effects of human amniotic membrane mesenchymal stem cells and their potential in corneal repair. Stem Cells Transl. Med. 7, 906–917. doi:10.1002/sctm.18-0042
Park, M., Banga, J. P., Kim, G. J., Kim, M., and y Lew, H. (2019). Human placenta-derived mesenchymal stem cells ameliorate orbital adipogenesis in female mice models of Graves’ ophthalmopathy. Stem Cell Res. Ther. 10, 246. doi:10.1186/s13287-019-1348-0
Park, M., Kim, H. C., Kim, O., and y Lew, H. (2018). Human placenta mesenchymal stem cells promote axon survival following optic nerve compression through activation of NF-κB pathway. J. Tissue Eng. Regen. Med. 12, e1441–e1449. doi:10.1002/term.2561
Park, M., Kim, J. Y., Kang, J. M., Lee, H. J., Banga, J. P., Kim, G. J., et al. (2021). PRL-1 overexpressed placenta-derived mesenchymal stem cells suppress adipogenesis in Graves’ ophthalmopathy through SREBP2/HMGCR pathway. Stem Cell Res. Ther. 12, 304. doi:10.1186/s13287-021-02337-2
Reza, H. M., Ng, B.-Y., Gimeno, F. L., Phan, T. T., and y Ang, L. P.-K. (2011). Umbilical cord lining stem cells as a novel and promising source for ocular surface regeneration. Stem Cell Rev. Rep. 7, 935–947. doi:10.1007/s12015-011-9245-7
Scalinci, S. Z., Scorolli, L., Corradetti, G., Domanico, D., Vingolo, E. M., Meduri, A., et al. (2011). Potential role of intravitreal human placental stem cell implants in inhibiting progression of diabetic retinopathy in type 2 diabetes: Neuroprotective growth factors in the vitreous. Clin. Ophthalmol. 5, 691–696. doi:10.2147/OPTH.S21161
Subasi, S., Altintas, O., Yardimoglu, M., Yazir, Y., Karaman, S., Rencber, S. F., et al. (2017). Comparison of collagen cross-linking and amniotic membrane transplantation in an experimental alkali burn rabbit model. Cornea 36, 1106–1115. doi:10.1097/ICO.0000000000001276
Thomi, G., Surbek, D., Haesler, V., Joerger-Messerli, M., and y Schoeberlein, A. (2019). Exosomes derived from umbilical cord mesenchymal stem cells reduce microglia-mediated neuroinflammation in perinatal brain injury. Stem Cell Res. Ther. 10, 105. doi:10.1186/s13287-019-1207-z
Torre, P. D. I., and Flores, A. I. (2020). Current status and future prospects of perinatal stem cells. Genes (Basel) 12, 6. doi:10.3390/genes12010006
Wang, L., Li, P., Tian, Y., Li, Z., Lian, C., Ou, Q., et al. (2017). Human umbilical cord mesenchymal stem cells: Subpopulations and their difference in cell biology and effects on retinal degeneration in RCS rats. Curr. Mol. Med. 17, 421–435. doi:10.2174/1566524018666171205140806
Xie, J.-X., Feng, Y., Yuan, J.-M., You, Z.-D., Lin, H.-Y., Lu, C.-L., et al. (2015). Positive effects of bFGF modified rat amniotic epithelial cells transplantation on transected rat optic nerve. PLoS One 10, e0119119. doi:10.1371/journal.pone.0119119
Yamashita, K., Inagaki, E., Hatou, S., Higa, K., Ogawa, A., Miyashita, H., et al. (2018). Corneal endothelial regeneration using mesenchymal stem cells derived from human umbilical cord. Stem Cells Dev. 27, 1097–1108. doi:10.1089/scd.2017.0297
Yu, C., Yang, K., Meng, X., Cao, B., and Wang, y. F. (2020). Downregulation of long noncoding RNA MIAT in the retina of diabetic rats with tail-vein injection of human umbilical-cord mesenchymal stem cells. Int. J. Med. Sci. 17, 591–598. doi:10.7150/ijms.38078
Zeng, W., Li, Y., Zeng, G., Yang, B., and y Zhu, Y. (2014). Transplantation with cultured stem cells derived from the human amniotic membrane for corneal alkali burns: An experimental study. Ann. Clin. Lab. Sci. 44, 73–81.
Keywords: perinatal derivates, ophthalmology, preclinical models, clinical trials and database search, mesenchymal stromal cells
Citation: Norte-Muñoz M, Botelho MF, Schoeberlein A, Chaves J, Neto Murta J, Ponsaerts P, Agudo-Barriuso M and Costa E (2022) Insights and future directions for the application of perinatal derivatives in eye diseases: A critical review of preclinical and clinical studies. Front. Bioeng. Biotechnol. 10:969927. doi: 10.3389/fbioe.2022.969927
Received: 15 June 2022; Accepted: 17 October 2022;
Published: 08 November 2022.
Edited by:
Guei-Sheung Liu, Centre for Eye Research Australia, AustraliaReviewed by:
Guido Moll, Charité Universitätsmedizin Berlin, GermanyLa-ongsri Atchaneeyasakul, Mahidol University, Thailand
Copyright © 2022 Norte-Muñoz, Botelho, Schoeberlein, Chaves, Neto Murta, Ponsaerts, Agudo-Barriuso and Costa. This is an open-access article distributed under the terms of the Creative Commons Attribution License (CC BY). The use, distribution or reproduction in other forums is permitted, provided the original author(s) and the copyright owner(s) are credited and that the original publication in this journal is cited, in accordance with accepted academic practice. No use, distribution or reproduction is permitted which does not comply with these terms.
*Correspondence: Esmeralda Costa, esmeraldaguedes@gmail.com; Marta Agudo-Barriuso, martabar@um.es