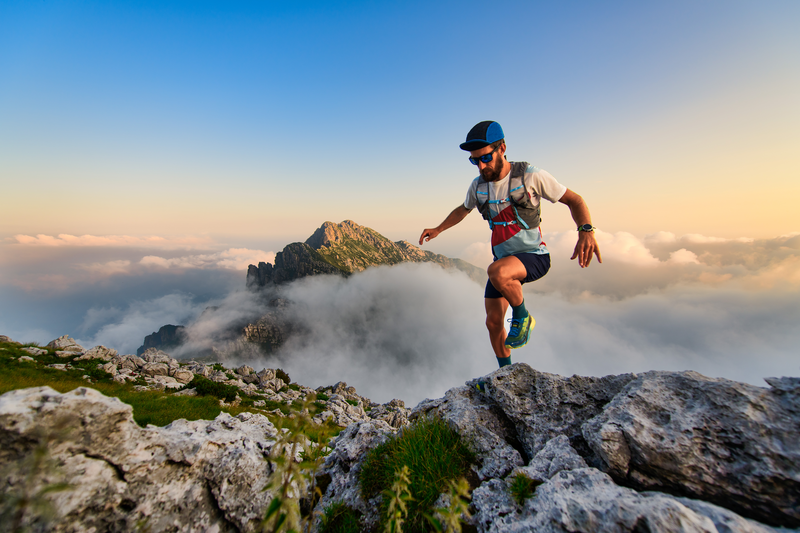
94% of researchers rate our articles as excellent or good
Learn more about the work of our research integrity team to safeguard the quality of each article we publish.
Find out more
MINI REVIEW article
Front. Bioeng. Biotechnol. , 05 August 2022
Sec. Nanobiotechnology
Volume 10 - 2022 | https://doi.org/10.3389/fbioe.2022.958095
This article is part of the Research Topic Preparation and Application of Intelligent Bioactive Nanocolloids View all 17 articles
As an emerging fringe science, bionics integrates the understanding of nature, imitation of nature, and surpassing nature in one aspect, and it organically combines the synergistic complementarity of function and structure–function integrated materials which is of great scientific interest. By imitating the microstructure of a natural biological surface, the bionic superhydrophobic surface prepared by human beings has the properties of self-cleaning, anti-icing, water collection, anti-corrosion and oil–water separation, and the preparation research methods are increasing. The preparation methods of superhydrophobic surface include vapor deposition, etching modification, sol–gel, template, electrostatic spinning, and electrostatic spraying, which can be applied to fields such as medical care, military industry, ship industry, and textile. The etching modification method can directly modify the substrate, so there is no need to worry about the adhesion between the coating and the substrate. The most obvious advantage of this method is that the obtained superhydrophobic surface is integrated with the substrate and has good stability and corrosion resistance. In this article, the different preparation methods of bionic superhydrophobic materials were summarized, especially the etching modification methods, we discussed the detailed classification, advantages, and disadvantages of these methods, and the future development direction of the field was prospected.
Nature is always the source of our inspiration. By observing the structural diversity, functional specificity, and environmental responsiveness of natural organisms, human beings have discovered many different biological structures and functions, thus creating more and more new materials and structures through technological innovation and cross-fertilization across disciplines. Bionic materials are materials prepared by simulating the unique structure or characteristics of organisms (Suresh Kumar et al., 2020; Koch et al., 2008). Biomimetic superhydrophobic surfaces, which are prepared by using the superhydrophobic phenomena related to biological structures in nature (Table 1), such as lotus leaves (Sun and Guo, 2019; Lv et al., 2020), butterfly wings (Zheng et al., 2007; Shao et al., 2019) and rice leaves (Wu et al., 2011; Lee et al., 2013; Rius-Ayra et al., 2018), have attracted wide attention and research because of outstanding self-cleaning (Barthlott and Neinhuis, 1997; Fürstner et al., 2005; Ming et al., 2005), anti-icing (Liu et al., 2020; Sun et al., 2020), anti-corrosion (Liu et al., 2014; Wei et al., 2021a; Zhang et al., 2022), and oil–water separation (Wang et al., 2015a; Saleh et al., 2020; Rasouli et al., 2021; Yao et al., 2021) properties. In recent years, with the deepening of the research in the micro-field, micro-nano materials have developed rapidly, and they have been developed into intelligent responsiveness (Li et al., 2017a; Chang et al., 2018; Li et al., 2021a), environmental remediation (Kumari et al., 2019; Sajjadi et al., 2021), biodegradability (Li et al., 2021b; Li et al., 2022a; Li et al., 2022b), nano-probe imaging (Li et al., 2016; Li et al., 2017b; Li et al., 2019a; Li et al., 2022c), and other characteristics, which are widely used in many fields (Waked, 2011; Khandelwal et al., 2016; Scalisi, 2017; Li et al., 2019b; Siddiqui et al., 2019), especially medicine (Li et al., 2021c; Lu et al., 2021). Biomimetic superhydrophobic surface combines the cutting-edge technologies of bionics and micro-nano fields, and has great development prospects.
The study of superhydrophobic principle can be traced back to 1805, when T. Young (Young, 1805) established Young’s equation of ideal smooth solid surface state, which set a theoretical precedent for studying the wettability of materials. Later, Wensel and Cassie summarized Wensel model (Wenzel, 1936) and Cassie–Baxter model (Cassie and Baxter, 1944) by studying the relationship between surface roughness and wettability. Recent further research shows that superhydrophobic surfaces can be divided into five types (Wang and Jiang, 2007). This classification includes steady-state and transition state, which can explain the phenomena that were difficult to explain by previous theories (Yilgor et al., 2012; Liu et al., 2017a; Chen et al., 2021).
Contact angle and rolling angle are important parameters to characterize the wettability of droplets on solid surfaces, and are also the initial evaluation indexes of biomimetic superhydrophobic surfaces (Aussillous and Quéré, 2001; Richard et al., 2002; Michael and Bhushan, 2007; Nosonovsky and Bhushan, 2008). With the development of research, researchers have made biomimetic superhydrophobic surfaces with multiple functions. Since then, the application fields of biomimetic superhydrophobic surfaces have been expanded by leaps and bounds, included adhesive-responsive superhydrophobic surfaces for sensors (Gao et al., 2019; Liu et al., 2019), industrial anticorrosive superhydrophobic surfaces which can effectively slow down the damage of metal oxide layers and resist strong acid/alkali corrosion (Li et al., 2019c; Ran et al., 2019; Ijaola et al., 2020), superhydrophilic/superhydrophobic surfaces which can realize industrial wastewater treatment and offshore oil spill treatment by using oil–water separation characteristics (Feng et al., 2004; Jayaramulu et al., 2016; Yang et al., 2018; Song et al., 2022), and superhydrophobic coatings with anti-icing and light transmission properties for outdoor glass and photovoltaic converters (Li et al., 2009; Liu et al., 2015a; Cui and Pakkanen, 2020; Zhu et al., 2021). In addition, diversified bionic superhydrophobic surfaces were widely used in modern military (Dong et al., 2013; Wang et al., 2015b; Jiaqiang et al., 2018), microfluidic control (Stratakis et al., 2011; Kong, 2021), fabric and textile industry (Hoefnagels et al., 2007; Xing et al., 2022) and other extended fields.
In this mini review, we reviewed the superhydrophobic surfaces and principles in nature. Section 2 introduced several different preparation methods, with emphasis on the preparation of biomimetic superhydrophobic surfaces by etching modification. Particularly, we discussed the unique advantages and disadvantages of etching modification. Finally, the conclusion of this review and the prospect of the research field in the future were described (Section 3).
With the deepening of research, the preparation methods of bionic superhydrophobic surfaces were gradually diversified. Figure 1 shows the common preparation methods of superhydrophobic surfaces. Generally speaking, the core idea of preparing biomimetic superhydrophobic surface is to imitate the microstructure of biological surface and modify it with low surface energy substances. According to the order of construction, it can be classified into two categories. The first category is to construct micro-nano rough structures on smooth surfaces, and then decorate them with low surface energy materials. The second category is to directly sketch micro-nano rough structures on low surface energy materials.
FIGURE 1. Etching modification (A), electrospinning and electrospraying (B), vapor phase deposition (C), sol–gel (D), template (E) and layer-by-layer self-assembly (F) methods developed to fabricate superhydrophobic surfaces.
In Table 2, we also show the characteristics of typical preparation methods of bionic superhydrophobic materials to understand their advantages and disadvantages. From the table, we can systematically understand the characteristics of various methods, including preparation principle, production cost, production speed, equipment requirement, environmental friendliness, and mechanical durability. It should be emphasized that the characteristics here are for most experiments that use this method to construct bionic superhydrophobic surfaces.
Among these methods, the etching modification method will be described in detail later. In addition, it is not difficult to find that the above-mentioned methods all have a common feature, namely, self-cleaning. At present, self-cleaning has basically become a common property of superhydrophobic surfaces (Parkin and Palgrave, 2005; Sethi and Manik, 2018). The most direct way to achieve self-cleaning effect is to use extremely low rolling angle. The accumulated dirt particles can be effectively cleaned by fast-sliding water droplets (Liu et al., 2015b; Sharma et al., 2021). Photocatalytic reaction can effectively decompose pollutants and achieve the purpose of more efficient self-cleaning (Afzal et al., 2014; Wang et al., 2021a). Compared with simply using droplets to roll away dirt, this more active self-cleaning method is more suitable for places with more pollutants such as oil pollution and organic matter (Mor et al., 2004; Rus et al., 2013; Moghaddasi and Mohammadizadeh, 2022).
In modern research, before the formulation and product development of self-cleaning superhydrophobic materials, molecular dynamics is more and more used for simulation to predict the target performance, which can take into account the self-cleaning performance and the durability brought by the adhesion between film and substrate (Sethi et al., 2022). Through molecular dynamics simulation optimization and experimental verification, Sethi’s research team comprehensively predicted and explained the surface behavior, substrate adhesion, and overall performance of the blend, which was beneficial to determine the volume, surface, and interface characteristics of the best formula before the preparation of bionic superhydrophobic surface coating, reducing the workload in actual preparation, improving work efficiency and product performance (Sethi et al., 2019; Sethi et al., 2020a; Sethi et al., 2020b).
Etching is a simple and effective method to achieve bionic superhydrophobic effect. This method is through selective etching to realize the processing of micro-nano double-ordered structure on the substrate surface (Ellinas et al., 2021).
Wet etching and dry etching are two main ways of etching. In wet etching, the etching substrate is soaked or coated with chemical reagents (such as acid, alkali, etc.), and the etching solution reacts with the material to remove specific surface materials (Huang et al., 2011; Yeganeh and Mohammadi, 2018; Jayarama et al., 2021). Dry etching is a process that uses laser or plasma to react with the substrate surface to form volatile substances, or directly bombards the substrate surface to corrode it (Lee, 1979; Elhadj et al., 20122012; Wang et al., 2021b; Fan et al., 2021). The following section will introduce wet chemical etching and dry laser etching, as well as their application scope, advantages and disadvantages.
Generally, metals and alloys are the most suitable substrates for chemical etching, especially magnesium alloys or aluminum alloys (Panda, 2021; Peng et al., 2021). By controlling the concentration of etching solution and etching time, the structural characteristics of superhydrophobic surface, such as roughness, can be effectively changed (Wei et al., 2021b; Guo et al., 2021; Krishnan et al., 2021; Zheng et al., 2021) (Figure 2).
Strong acids, such as HCl and H2SO4 are common and effective etching solutions. Kumar et al. (Kumar and Gogoi, 2018) used HNO3-HCl mixture to etch aluminum plate, and then treated it with high-density hexadecyl trimethoxysilane (HDTMS) toluene solution to construct a superhydrophobic surface with the static contact angle of 162.0 ± 4.2° and the rolling angle of 4 ± 0.5°. Besides good superhydrophobic self-cleaning performance, the surface also showed good thermal stability, chemical stability, and mechanical stability. Nguyen et al. (Nguyen et al., 2021) etched with HCl solution and deposited with FOTS (fluorooctatrichlorosilane) to prepare superhydrophobic aluminum plates with low ice adhesion strength and long freezing time. In addition, a model for calculating the freezing time was put forward, and the comparison between the experimental and theoretical calculation showed excellent consistency, which provided guidance for understanding the icing phenomenon and designing the ice-repellent surface. Campbell et al. (Gray-Munro and Campbell, 2017) combined the chemical etching of H2SO4-H2O2 with the deposition of silicone coating to construct a lotus leaf-like structure on the surface of magnesium alloy, which had excellent stability in aqueous solution. By this way, the surface degradation rate of biodegradable superhydrophobic magnesium alloy with biocompatibility was controlled, which was significant in medical application. Saleh et al. (Saleh and Baig, 2019) functionalized stainless steel with octadecyl trichlorosilane (ODTCS) after structural treatment with H2SO4, and obtained superhydrophobic and superhydrophilic properties. Experiments showed that the separation efficiency of various nonpolar organic components from water was over 99%, which was expected to be used to separate oil from water. In addition, HF in inorganic acid is also a good etching solution (Qu et al., 2007; Zhang et al., 2015; Du et al., 2018; Kim et al., 2018; Xu et al., 2019; Zhang et al., 2020b; Shaikh et al., 2021).
Compared with inorganic acids, organic acids are less used for etching, because most inorganic acids are relatively more stable and lower in cost. Ou et al. (Ou et al., 2019) sprayed cold galvanized coating on iron substrate, then etched with acetic acid, and finally modified with stearic acid. The superhydrophobic sample surface with an apparent contact angle of 168.4 ± 1.5° and a rolling angle of 3.5 ± 1.2° was obtained. This sample combined the respective characteristics of zinc coating and superhydrophobic surface in terms of metal corrosion resistance, which had rapid manufacturing process, good mechanical durability, and easy repair. Wu et al. (Wu et al., 2012) compared the etching effects of aluminum alloy samples in three different acid solutions (acetic acid, hydrochloric acid, and oxalic acid), and found that the mixed solution of oxalic acid and hydrochloric acid was the better etching combination. Similarly, they found that better surface roughness can be obtained by adjusting the concentration of Cl− ion and oxalate ion in acid solution. This provided a new strategy for controllable preparation of superhydrophobic films on aluminum alloys, which could be used in practical industrial applications. Chen et al. (Chen et al., 2010) proposed a superhydrophobic surface preparation method without hydrophobic treatment, which was directly obtained by soaking aluminum in the mixed solution of HCl and acetic acid. In addition, they also studied the effect of acetic acid content on the surface structure.
Besides acidic solutions, other kinds of solutions have been widely used. NaOH is the most commonly used alkaline etchant, which is usually used to etch aluminum alloy (Saleema et al., 2010; Huang et al., 2015; Lomga et al., 2017; Nguyen-Tri et al., 2019a; Tudu et al., 2019; Yang et al., 2022). In Peng’s work (Peng and Deng, 2015), NH3 was selected as the etchant of aluminum, and the superamphiphobic sample obtained by hot ammonia solution etching and fluorosilane modification had excellent chemical stability. This simple, economical, environment-friendly, and efficient method could be used in the fields of oil-proof and water-proof. Wan et al. (Wan et al., 2018) combined ammonia etching and hydrothermal treatment to construct superhydrophobic surface on copper substrate modified by 1H,1H,2H,2H-perfluorodecyl triethoxysilane (PFDTES), which showed good waterproof, anti-corrosion and anti-adhesion properties in simulated seawater and humid air. Parin et al. (Parin et al., 2018) used three different salt solution etchants, namely AlCl3, FeCl3 and CuCl2, to obtain superhydrophobic aluminum surfaces by chemical etching and fluorosilane deposition, respectively, which confirmed that different etchants would produce different surface micro-nanostructures. Rodič et al. (Rodič et al., 2020) etched aluminum in FeCl3 solution, and then grafted at ambient temperature directly in an ethanol solution of 1H,1H,2H,2H-perfluorodecyltriethoxysilane. The prepared superhydrophobic surface has the characteristics of self-cleaning and anti-icing. Moreover, the promotion of dropwise condensation significantly improved the heat transfer coefficient, so that the sample could be widely used in heat transfer industry. Wang et al. (Wang et al., 2016b) chemically etched magnesium in CuCl2 solution, and then modified it with oleic acid to prepare superhydrophobic surface with contact angle of 155°. Song et al. (Song et al., 2012) developed a rapid preparation method of superhydrophobic materials, in which aluminum was immersed in CuCl2 solution and then modified with ethanol solution of fluoroalkyl silane, and the whole process only took a few seconds. This convenient and efficient method may have the potential of large-scale preparation.
Chemical etching can be divided into two-step method (Milosev et al., 2017; Milošev et al., 2019; Rodič et al., 2022) and one-step method (Chen et al., 2010) in addition to the classification of etching solution. This is classified according to the operation steps. The two-step method is chemical etching and then coating, while the one-step method is chemical etching and coating in the same step (Varshney et al., 2016).
Chemical etching is a simple, quick and low-cost method, which can be used in a large scale. However, the etching solution used in the operation process is toxic to human health and the environment. In addition, the specific shape and thickness of the micro-nano double-ordered structure are difficult to control, and the mechanical durability of the surface is not high. Under the condition of over-etching, the microsurface roughness of the substrate decreases, and even the basic mechanical properties of the substrate are destroyed, which is also an important problem. Therefore, the preparation of green etchant, the optimization of etching process, the precise control of substrate surface morphology and thickness, and the reduction of environmental pollution risk are the key development paths that chemical etching needs to explore in the future.
As shown in Figure 3, laser etching can be divided into heat treatment and cold treatment according to its reaction principle (He et al., 2019; Ehrhardt et al., 2021). Irradiating the substrate surface with high-energy laser beam, the generated high temperature melts and vaporizes the material in a short time. After cooling, the superhydrophobic surface is constructed, which is called laser heat treatment. Cold treatment is a method of breaking chemical bonds in materials through photochemical reaction and constructing superhydrophobic surfaces after cooling. This technology has been widely used in various materials, including metal, glass, and polymer (Fadeeva et al., 2011; Lu et al., 2017; Dinh et al., 2018; Kostal et al., 2018; Li et al., 2019d; Ma et al., 2019; Stratakis et al., 2020).
Boinovich et al. (Boinovich et al., 2017) used Al–Mg alloy as the substrate, and proved that laser treatment can endow the surface with multi-peak roughness and change the composition of the surface layer. This research had overcome the obstacles of metal materials in industrial application, such as easy corrosion, poor cold resistance or weak thermal shock stress resistance. Li et al. (Li et al., 2018) prepared periodic microscale papillary pit microstructure on magnesium alloy surface by laser ablation. After chemical etching with AgNO3 and surface modification with stearic acid, the prepared surface has superhydrophobic property, and the maximum contact angle reaches 158.2°. By adjusting the microstructure, such as the center distance of pits, the surface can change from low adhesion to high adhesion. In addition, due to the high degree of independent selection and accuracy of laser etching, the surface structure that meets the requirements of experimenters can be perfectly reproduced. Using this characteristic of laser etching, superhydrophobic surfaces with excellent anisotropy can be easily prepared (Yong et al., 2014; Fang et al., 2018; Lasagni et al., 2018; Cai et al., 2019; Tuo et al., 2019; Bai et al., 2020; Rong et al., 2021; Yang et al., 2021). In another study, femtosecond laser and picosecond laser were used to construct nanostructures of aluminum, copper, and galvanized steel, respectively, and then aging in vacuum was used to replace low surface energy modification. This method of combining ultrafast laser surface nanostructures with vacuum aging was suitable for a wide range of self-cleaning applications (Khan et al., 2021). In addition to the metal matrix, Chen et al. (Chen et al., 2018) showed a method of manufacturing bionic reed leaves by laser treating the surface of structured polydimethylsiloxane (PDMS). Du et al. (Du et al., 2022) proposed a one-step laser-etching method for manufacturing superhydrophobic silicone rubber with bionic layered micro/nano structure, whose contact angle and sliding angle can be adjusted according to the number of laser-etching cycles, which is beneficial to different application requirements. It was a potential candidate to protect flexible electronics equipment in rainy days and acid/alkali environments. Inspired by the hexagonal microstructure array of mosquito’s compound eye, He et al. (He et al., 2021) made multifunctional superhydrophobic self-cleaning glass with anti-fog and anti-icing by laser texturing. Jing et al. (Jing et al., 2022) used picosecond laser to etch glass substrate and chemically modified it by silanization to prepare superhydrophobic surface with high adhesion. They also pointed out that laser-induced micro/nano structure depends on laser energy to a great extent and significantly affects adhesion, while scanning times have a slight effect on surface morphology and adhesion.
Laser-etching method has high precision and controllability. By controlling the laser type, irradiation time and light intensity, the surface microstructure with controllable direction can be obtained on different substrates (Figure 4), which is suitable for most materials. In addition, no harmful substances are produced during the experiment, which is undoubtedly environment-friendly. In recent years, with the rapid development of 3D laser printing technology and femtosecond laser technology, researchers have been able to complete custom etching of various complex microstructures. However, because the laser synthesis equipment is very complex, expensive, energy-intensive, and the action area of a single laser beam is relatively small, it is not suitable for industrial large-scale preparation of superhydrophobic materials, and it is currently in the laboratory stage.
The etching methods mentioned in the above two chapters usually use different substrates (Table 3), but they can actually be used in combination. For example, Xia et al. (Xia et al., 2022) compared three methods: chemical etching, laser etching, and chemical-laser mixed etching, and pointed out that specific chemical-laser mixed etching parameters can effectively prepare uniform hierarchical structure on aluminum alloy surface, achieving excellent hydrophobicity, and low ice adhesion. When this method was applied to the wing manufacturing, the obtained wing may prevent the aircraft from freezing and protect the aerospace safety. Dong et al. (Dong et al., 2011) also prepared a hydrophobic copper surface with tunable regular microstructure and random nanostructure whose water contact angle is about 153°. The regular microstructure was obtained by nanosecond pulse laser etching, while the random nanostructure was determined by chemical etching. The regular microstructure was obtained by nanosecond pulse laser etching, while the random nanostructure was determined by chemical etching. Liu et al. (Liu et al., 2013) dipped the aluminum alloy in HNO3 and Cu(NO3)2 after laser processing, and finally modified it with DTS (CH3(CH2)11Si(OCH3)3) to prepare the bionic superhydrophobic surface with high adhesion. It is easy to find that these two methods can also be used together with other etching methods to achieve better bionic superhydrophobic effect (Li et al., 2014; Gu et al., 2017; Tian et al., 2017; Feng et al., 2022a; Feng et al., 2022b). Rodič et al. (Rodič and Milošev, 2019) made highly hydrophobic aluminum surface in NaOH solution containing various alkoxysilanes by one-step ultrasonic process, which had corrosion resistance, self-cleaning, and anti-icing characteristics. These mixed preparation processes provided a new way for the preparation of various materials.
This article summarized the basic principle and model of superhydrophobic, epitomized the structure and wetting characteristics of biological superhydrophobic surfaces in nature, reviewed various preparation methods of bionic superhydrophobic materials, emphasized the etching method, and discussed the research status and challenges of superhydrophobic applications. As described in detail above, the chemical etching method is simple, fast, low-cost and has the potential for large-scale application. Its disadvantage is that it is harmful to the environment and human body, and excessive etching will cause the mechanical properties of samples to be destroyed. Laser etching has controllable and customizable surface morphology, but it is expensive and inefficient. The mixed use of the two methods has indeed achieved the effect of complementing each other’s strengths to a certain extent, but it is undeniable that the equipment price and pollution have not been improved.
Science and technology originates from nature and is superior to nature. The research of bionic superhydrophobic surface started from the early imitation of natural animal and plant surface structures, and now it has expanded to the creative behavior of designing structures and modifying materials independently, especially the birth of laser etching, which greatly improved the structural accuracy. The related properties of superhydrophobic surfaces have also developed from simple hydrophobicity to many directions, including anti-icing, water collection, directional transportation and wetting behavior transformation, which has brought about significant changes in the fields of industrial life and national defense science and technology development. The single bionic superhydrophobic surface can no longer meet the actual needs, and the research of multifunctional bionic superhydrophobic surface has become a hot topic discussed by scholars. However, with the continuous expansion and deepening of the research in the field of etching, the existing problems in the process of preparing bionic superhydrophobic surfaces by etching are also exposed. For example, the environmental friendliness and mechanical properties of chemical etching need to be improved, and laser etching needs to consider how to improve efficiency, reduce equipment costs and achieve mass production. In view of the research hotspots and existing problems, we put forward the following prospects:
(1) Superhydrophobic surface should be further developed toward multifunction. Based on the superhydrophobic function, the surface has many functions, such as antibacterial (Zhan et al., 2022), anti-ultraviolet, anti-radiation, underwater drag reduction, and performance change. It improves the applicability of superhydrophobic materials in many fields and environments, and has far-reaching significance for industrial application. Additionally, if the micro-nano structure is directly built on stealth coating materials (wave absorbing materials, light transmitting materials, light guiding materials, etc.), it will have the characteristics of anti-reflection, anti-radiation, and drag reduction, further increasing its concealment and maneuverability. The stealth surface made by this method is likely to make a major breakthrough in modern national defense and military fields.
(2) To improve the chemical and mechanical stability, the appearance of self-healing superhydrophobic coating provides a new development direction for related research (Sam et al., 2019; Chang et al., 2020). The mechanism of intrinsic self-healing superhydrophobic coating is to introduce dynamic chemical bonds into the internal structure of the material. When the material is damaged, the damaged chemical bonds will be restored to the initial state due to dynamic equilibrium, so that the structure and state of the material can be restored. The external self-repairing super-hydrophobic coating can be stimulated by changing the conditions, such as light and temperature, so that the repairing agent inside the material can be released and migrated to the damaged part, and thus the damaged surface can be healed. However, considering the complex environment in practical engineering application, besides self-healing, the wear resistance, acid and alkali resistance and long-term weather resistance of superhydrophobic coating itself need to be further improved.
(3) For the materials with weak hydrophobicity and easy to be polluted by oily substances, it is necessary to further study the super-double hydrophobic materials that are both hydrophobic and oleophobic, and make them into responsive materials, that is, to switch or switch the surface free energy by external stimulation. In addition, perfluoro silanes, which has both hydrophobic and oleophobic properties, is a target worthy of consideration.
(4) Aiming at the problems of strong pollution and high-energy consumption, the development of new biomaterials and new energy sources provides some guiding ideas for the preparation of green, environmentally friendly, low-cost, and reliable superhydrophobic surfaces.
(5) The effects of surface geometry size, wettability, and surface composition on superhydrophobic properties, especially the quantitative research directly related to hysteresis angle, need to be deepened, which is not only to be studied by etching method, but also to be explored by all methods.
(6) It is still necessary to study the preparation of superhydrophobic materials at low cost to enhance the practicability and expand the application fields.
To sum up, the future development direction of preparing bionic superhydrophobic surface by etching method is to combine the advantages of the two methods and develop a set of durable, energy-saving, low-cost, and mass-production preparation methods that meet the multifunctional application requirements of superhydrophobic surface. Only by realizing the industrial production of superhydrophobic surfaces can we really get out of the laboratory and into life.
SG-Z: writing—original draft preparation, reviewing and editing, and drawing the graphs and schematic. HY: methodology, investigation, reviewing and editing, and drawing the graphs and schematic. HN: reviewing and editing. HM: supervision and reviewing and editing. MZ: supervision and reviewing and editing.
This work was supported by the Fundamental Research Funds for the Central Universities (No. 2572020BC07) and the National Natural Science Foundation of China.
The authors declare that the research was conducted in the absence of any commercial or financial relationships that could be construed as a potential conflict of interest.
All claims expressed in this article are solely those of the authors and do not necessarily represent those of their affiliated organizations, or those of the publisher, the editors, and the reviewers. Any product that may be evaluated in this article, or claim that may be made by its manufacturer, is not guaranteed or endorsed by the publisher.
Afzal, S., Daoud, W. A., and Langford, S. J. (2014). Superhydrophobic and photocatalytic self-cleaning cotton. J. Mat. Chem. A 2 (42), 18005–18011. doi:10.1039/c4ta02764g
Alizadeh-Birjandi, E., Tavakoli-Dastjerdi, F., Leger, J. S., Faull, K. F., Davis, S. H., Rothstein, J. P., et al. (2020). Delay of ice formation on penguin feathers. Eur. Phys. J. Spec. Top. 229 (10), 1881–1896. doi:10.1140/epjst/e2020-900273-x
Aljumaily, M. M., Alsaadi, M. A., Das, R., Hashim, N. A., AlOmar, M. K., Alayan, H. M., et al. (2018). Optimization of the synthesis of superhydrophobic carbon nanomaterials by chemical vapor deposition. Sci. Rep. 8 (1), 2278. doi:10.1038/s41598-018-21051-3
Attar, M. R., Khajavian, E., Hosseinpour, S., and Davoodi, A. (2020). Fabrication of micro–nano-roughened surface with superhydrophobic character on an aluminium alloy surface by a facile chemical etching process. Bull. Mat. Sci. 43 (1), 31. doi:10.1007/s12034-019-1998-7
Aussillous, P., and Quéré, D. (2001). Liquid marbles. Nature 411 (6840), 924–927. doi:10.1038/35082026
Autumn, K., Sitti, M., Liang, Y. A., Peattie, A. M., Hansen, W. R., Sponberg, S., et al. (2002). Evidence for van der Waals adhesion in gecko setae. Proc. Natl. Acad. Sci. U. S. A. 99 (19), 12252–12256. doi:10.1073/pnas.192252799
Bai, X., Yang, Q., Fang, Y., Yong, J., Bai, Y., Zhang, J., et al. (2020). Anisotropic, adhesion-switchable, and thermal-responsive superhydrophobicity on the femtosecond laser-structured shape-memory polymer for droplet manipulation. Chem. Eng. J. 400, 125930. doi:10.1016/j.cej.2020.125930
Ball, P. (1999). Engineering Shark skin and other solutions. Nature 400 (6744), 507–509. doi:10.1038/22883
Barthlott, W., and Neinhuis, C. (1997). Purity of the sacred lotus, or escape from contamination in biological surfaces. Planta 202 (1), 1–8. doi:10.1007/s004250050096
Basak, S. (2020). Walking through the biomimetic bandages inspired by Gecko’s feet. Biodes. Manuf. 3 (2), 148–154. doi:10.1007/s42242-020-00069-5
Bechert, D. W., Bruse, M., Hage, W., and Meyer, R. (2000). Fluid mechanics of biological surfaces and their technological application. Naturwissenschaften 87 (4), 157–171. doi:10.1007/s001140050696
Bixler, G. D., and Bhushan, B. (2013). Fluid drag reduction with shark-skin riblet inspired microstructured surfaces. Adv. Funct. Mat. 23 (36), 4507–4528. doi:10.1002/adfm.201203683
Bohn, H. F., and Federle, W. (2004). Insect aquaplaning: Nepenthes pitcher plants capture prey with the peristome, a fully wettable water-lubricated anisotropic surface. Proc. Natl. Acad. Sci. U. S. A. 101 (39), 14138–14143. doi:10.1073/pnas.0405885101
Boinovich, L. B., Modin, E. B., Sayfutdinova, A. R., Emelyanenko, K. A., Vasiliev, A. L., and Emelyanenko, A. M. (2017). Combination of functional nanoengineering and nanosecond laser texturing for design of superhydrophobic aluminum alloy with exceptional mechanical and chemical properties. ACS Nano 11 (10), 10113–10123. doi:10.1021/acsnano.7b04634
Böke, F., Giner, I., Keller, A., Grundmeier, G., and Fischer, H. (2016). Plasma-Enhanced chemical vapor deposition (PE-CVD) yields better hydrolytical stability of biocompatible SiOx thin films on implant alumina ceramics compared to rapid thermal evaporation physical vapor deposition (PVD). ACS Appl. Mat. Interfaces 8 (28), 17805–17816. doi:10.1021/acsami.6b04421
Brown, P. S., and Bhushan, B. (2015). Mechanically durable, superoleophobic coatings prepared by layer-by-layer technique for anti-smudge and oil-water separation. Sci. Rep. 5 (1), 8701. doi:10.1038/srep08701
Cai, Y., Xu, Z., Wang, H., Lau, K. H. A., Ding, F., Sun, J., et al. (2019). A sequential process for manufacturing nature-inspired anisotropic superhydrophobic structures on AISI 316L stainless steel. Nanomanuf. Metrol. 2 (3), 148–159. doi:10.1007/s41871-019-00046-2
Cassie, A. B. D., and Baxter, S. (1944). Wettability of porous surfaces. Trans. Faraday Soc. 40, 546. doi:10.1039/tf9444000546
Chang, J., Zhang, L., and Wang, P. (2018). Intelligent environmental nanomaterials. Environ. Sci. Nano 5 (4), 811–836. doi:10.1039/c7en00760d
Chang, T., Panhwar, F., and Zhao, G. (2020). Flourishing self‐healing surface materials: Recent progresses and challenges. Adv. Mat. Interfaces 7 (6), 1901959. doi:10.1002/admi.201901959
Chen, C., Pan, L., Li, H., Liu, Q., Li, F., Tu, J., et al. (2021). Conversion of superhydrophilicity to superhydrophobicity by changing the microstructure of carbon-high fly ash. Mater. Lett. 299, 130051. doi:10.1016/j.matlet.2021.130051
Chen, D. L., Mao, J. W., Chen, Z. D., Yu, K. X., Han, D. D., and Sun, H. B. (2018). Fabrication of bionic reed leaf superhydrophobic surface by laser processing. Chin. Sci. Bull. 64 (12), 1303–1308. doi:10.1360/n972018-00872
Chen, H., Zhang, P., Zhang, L., Liu, H., Jiang, Y., Zhang, D., et al. (2016). Continuous directional water transport on the peristome surface of Nepenthes alata. Nature 532 (7597), 85–89. doi:10.1038/nature17189
Chen, Z., Guo, Y., and Fang, S. (2010). A facial approach to fabricate superhydrophobic aluminum surface. Surf. Interface Analysis Int. J. Devoted Dev. Appl. Tech. Analysis Surfaces, Interfaces Thin Films 42 (1), 1–6. doi:10.1002/sia.3126
Cui, W., and Pakkanen, T. A. (2020). Fabrication of transparent icephobic surfaces with self-reparability: Effect of structuring and thickness of the lubricant-elastomer layer. Appl. Surf. Sci. 504, 144061. doi:10.1016/j.apsusc.2019.144061
Deo, D., Singh, S. P., Mohanty, S., Guhathakurata, S., Pal, D., and Mallik, S. (2022). Biomimicking of phyto-based super-hydrophobic surfaces towards prospective applications: A review. J. Mater. Sci. 57, 8569–8596. doi:10.1007/s10853-022-07172-1
Dimitrakellis, P., and Gogolides, E. (2018). Atmospheric plasma etching of polymers: A palette of applications in cleaning/ashing, pattern formation, nanotexturing and superhydrophobic surface fabrication. Microelectron. Eng. 194, 109–115. doi:10.1016/j.mee.2018.03.017
Dinh, T. H., Ngo, C. V., and Chun, D. M. (2018). Controlling the wetting properties of superhydrophobic titanium surface fabricated by UV nanosecond-pulsed laser and heat treatment. Nanomater. (Basel). 8 (10), 766. doi:10.3390/nano8100766
Dong, C., Gu, Y., Zhong, M., Li, L., Sezer, K., Ma, M., et al. (2011). Fabrication of superhydrophobic Cu surfaces with tunable regular micro and random nano-scale structures by hybrid laser texture and chemical etching. J. Mater. Process. Technol. 211 (7), 1234–1240. doi:10.1016/j.jmatprotec.2011.02.007
Dong, H., Cheng, M., Zhang, Y., Wei, H., and Shi, F. (2013). Extraordinary drag-reducing effect of a superhydrophobic coating on a macroscopic model ship at high speed. J. Mat. Chem. A Mat. 1 (19), 5886. doi:10.1039/c3ta10225d
Du, X., Gao, B., Li, Y., Song, Z., Zhu, X., and Chen, J. (2020). Fabrication of multiscale structured hydrophobic NiCrZrN coating with high abrasion resistance using multi-arc ion plating. J. Alloys Compd. 812, 152140. doi:10.1016/j.jallcom.2019.152140
Du, X., Xin, B., Xu, J., and Wang, C. (2021). Biomimetic superhydrophobic membrane with multi-scale porous microstructure for waterproof and breathable application. Colloids Surfaces A Physicochem. Eng. Aspects 612, 125924. doi:10.1016/j.colsurfa.2020.125924
Du, Y., Wu, T., Xie, H., and Qu, J. P. (2022). One-step laser etching of a bionic hierarchical structure on a silicone rubber surface with thermal and acid/alkali resistance and tunable wettability. Soft Matter 18 (17), 3412–3421. doi:10.1039/d2sm00242f
Du, Z., Ding, P., Tai, X., Pan, Z., and Yang, H. (2018). Facile preparation of Ag-coated superhydrophobic/superoleophilic mesh for efficient oil/water separation with excellent corrosion resistance. Langmuir 34 (23), 6922–6929. doi:10.1021/acs.langmuir.8b00640
Ehrhardt, M., Lorenz, P., Bauer, J., Heinke, R., Hossain, M. A., Han, B., et al. (2021). Dry etching of germanium with laser induced reactive micro plasma. Lasers Manuf. Mat. Process. 8 (3), 237–255. doi:10.1007/s40516-021-00147-1
Elhadj, S., Guss, G., Matthews, M. J., and Bass, L. (20122012). Laser-induced gas plasma etching of fused silica under ambient conditions[C]//Laser-Induced Damage in Optical Materials. SPIE 8530, 457–468. doi:10.1117/12.979814
Ellinas, K., Dimitrakellis, P., Sarkiris, P., and Gogolides, E. (2021). A review of fabrication methods, properties and applications of superhydrophobic metals. Process. (Basel). 9 (4), 666. doi:10.3390/pr9040666
Fadeeva, E., Truong, V. K., Stiesch, M., Chichkov, B. N., Crawford, R. J., Wang, J., et al. (2011). Bacterial retention on superhydrophobic titanium surfaces fabricated by femtosecond laser ablation. Langmuir 27 (6), 3012–3019. doi:10.1021/la104607g
Fan, Y., Li, S., Wei, D., Fang, Z., Han, Z., and Liu, Y. (2021). Bioinspired superhydrophobic cilia for droplets transportation and microchemical reaction. Adv. Mat. Interfaces 8 (24), 2101408. doi:10.1002/admi.202101408
Fang, Y., Sun, G., Cong, Q., Chen, G. h., and Ren, L. q. (2008). Effects of methanol on wettability of the non-smooth surface on butterfly wing. J. Bionic Eng. 5 (2), 127–133. doi:10.1016/s1672-6529(08)60016-5
Fang, Y., Yong, J., Chen, F., Huo, J., Yang, Q., Zhang, J., et al. (2018). Bioinspired fabrication of Bi/tridirectionally anisotropic sliding superhydrophobic PDMS surfaces by femtosecond laser. Adv. Mat. Interfaces 5 (6), 1701245. doi:10.1002/admi.201701245
Feng, L., Li, S., Li, Y., Li, H., Zhang, L., Zhai, J., et al. (2002). Super-hydrophobic surfaces: From natural to artificial. Adv. Mat. 14 (24), 1857–1860. doi:10.1002/adma.200290020
Feng, L., Zhang, Y., Xi, J., Zhu, Y., Wang, N., Xia, F., et al. (2008). Petal Effect: A superhydrophobic state with high adhesive force. Langmuir 24 (8), 4114–4119. doi:10.1021/la703821h
Feng, L., Zhang, Z., Mai, Z., Ma, Y., Liu, B., Jiang, L., et al. (2004). A super‐hydrophobic and super‐oleophilic coating mesh film for the separation of oil and water. Angew. Chem. Int. Ed. Engl. 116 (15), 2046–2048. doi:10.1002/ange.200353381
Feng, X., Sun, P., and Tian, G. (2022). Recent developments of superhydrophobic surfaces (SHS) for underwater drag reduction opportunities and challenges. Adv. Mat. Interfaces 9 (2), 2101616. doi:10.1002/admi.202101616
Feng, X., Zhang, X., and Tian, G. (2022). Recent advances in bioinspired superhydrophobic ice-proof surfaces: Challenges and prospects. Nanoscale 14 (16), 5960–5993. doi:10.1039/d2nr00964a
Fürstner, R., Barthlott, W., Neinhuis, C., and Walzel, P. (2005). Wetting and self-cleaning properties of artificial superhydrophobic surfaces. Langmuir 21 (3), 956–961. doi:10.1021/la0401011
Gao, D., Cao, J., and Guo, Z. (2019). Underwater manipulation of oil droplets and bubbles on superhydrophobic surfaces via switchable adhesion. Chem. Commun. 55 (23), 3394–3397. doi:10.1039/c9cc00271e
Gao, J., Wu, Y., Zhang, Z., Zhao, D., Zhu, H., Xu, K., et al. (2022). Achieving amorphous micro-nano superhydrophobic structures on quartz glass with a PTFE coating by laser back ablation. Opt. Laser Technol. 149, 107927. doi:10.1016/j.optlastec.2022.107927
Gao, X., Yan, X., Yao, X., Xu, L., Zhang, K., Zhang, J., et al. (2007). The dry-style antifogging properties of mosquito compound eyes and artificial analogues prepared by soft lithography. Adv. Mat. 19 (17), 2213–2217. doi:10.1002/adma.200601946
Gou, X., and Guo, Z. (2018). Superhydrophobic plant leaves with micro-line structures: An optimal biomimetic objective in bionic engineering. J. Bionic Eng. 15 (5), 851–858. doi:10.1007/s42235-018-0072-2
Gray-Munro, J., and Campbell, J. (2017). Mimicking the hierarchical surface topography and superhydrophobicity of the lotus leaf on magnesium alloy AZ31. Mater. Lett. 189, 271–274. doi:10.1016/j.matlet.2016.11.102
Gu, Y., Zhang, W., Mou, J., Zheng, S., Jiang, L., Sun, Z., et al. (2017). Research progress of biomimetic superhydrophobic surface characteristics, fabrication, and application. Adv. Mech. Eng. 9 (12), 168781401774685. doi:10.1177/1687814017746859
Guo, F., Duan, S., Wu, D., Matsuda, K., Wang, T., and Zou, Y. (2021). Facile etching fabrication of superhydrophobic 7055 aluminum alloy surface towards chloride environment anticorrosion. Corros. Sci. 182, 109262. doi:10.1016/j.corsci.2021.109262
Guo, X. J., Xue, C. H., Sathasivam, S., Page, K., He, G., Guo, J., et al. (2019). Fabrication of robust superhydrophobic surfaces via aerosol-assisted CVD and thermo-triggered healing of superhydrophobicity by recovery of roughness structures. J. Mat. Chem. A Mat. 7 (29), 17604–17612. doi:10.1039/c9ta03264a
Guo, Z., and Liu, W. (2007). Biomimic from the superhydrophobic plant leaves in nature: Binary structure and unitary structure. Plant Sci. 172 (6), 1103–1112. doi:10.1016/j.plantsci.2007.03.005
He, T., Wang, Z., Zhong, F., Fang, H., Wang, P., and Hu, W. (2019). Etching techniques in 2D materials. Adv. Mat. Technol. 4 (8), 1900064. doi:10.1002/admt.201900064
He, X., Li, G., Zhang, Y., Lai, X., Zhou, M., Xiao, L., et al. (2021). Bioinspired functional glass integrated with multiplex repellency ability from laser-patterned hexagonal texturing. Chem. Eng. J. 416, 129113. doi:10.1016/j.cej.2021.129113
Hoefnagels, H. F., Wu, D., De With, G., and Ming, W. (2007). Biomimetic superhydrophobic and highly oleophobic cotton textiles. Langmuir 23 (26), 13158–13163. doi:10.1021/la702174x
Huang, Y., Sarkar, D. K., and Chen, X. G. (2015). Superhydrophobic aluminum alloy surfaces prepared by chemical etching process and their corrosion resistance properties. Appl. Surf. Sci. 356, 1012–1024. doi:10.1016/j.apsusc.2015.08.166
Huang, Z., Geyer, N., Werner, P., de Boor, J., and Gosele, U. (2011). Metal-Assisted chemical etching of silicon: A review. Adv. Mat. 23 (2), 285–308. doi:10.1002/adma.201001784
Ijaola, A. O., Farayibi, P. K., and Asmatulu, E. (2020). Superhydrophobic coatings for steel pipeline protection in oil and gas industries: A comprehensive review. J. Nat. Gas Sci. Eng. 83, 103544. doi:10.1016/j.jngse.2020.103544
Jayarama, A., Kannarpady, G. K., Kale, S., Prabhu, S., and Pinto, . R (2021). Chemical etching of glasses in hydrofluoric acid: A brief review. Mater. Today Proc. 55, 46–51. doi:10.1016/j.matpr.2021.12.110
Jayaramulu, K., Datta, K. K. R., Rösler, C., Petr, M., Otyepka, M., Zboril, R., et al. (2016). Biomimetic superhydrophobic/superoleophilic highly fluorinated graphene oxide and ZIF-8 composites for oil-water separation. Angew. Chem. Int. Ed. 55 (3), 1178–1182. doi:10.1002/anie.201507692
Jiang, H. B., Zhang, Y. L., Liu, Y., Fu, X. Y., Li, Y. F., Liu, Y. Q., et al. (2016). Bioinspired few-layer graphene prepared by chemical vapor deposition on femtosecond laser-structured Cu foil. Laser & Photonics Rev. 10 (3), 441–450. doi:10.1002/lpor.201500256
Jiaqiang, E., Jin, Y., Deng, Y., Zuo, W., Zhao, X., Han, D., et al. (2018). Wetting models and working mechanisms of typical surfaces existing in nature and their application on superhydrophobic surfaces: A review. Adv. Mater. Interfaces 5, 1701052. doi:10.1002/admi.201701052
Jing, X., Xia, Y., Chen, F., Yang, C., Yang, Z., and Jaffery, S. H. I. (2022). Preparation of superhydrophobic glass surface with high adhesion. Colloids Surfaces A Physicochem. Eng. Aspects 633, 127861. doi:10.1016/j.colsurfa.2021.127861
Ke, P., Jiao, X. N., Ge, X. H., Xiao, W. M., and Yu, B. (2014). From macro to micro: Structural biomimetic materials by electrospinning. RSC Adv. 4 (75), 39704–39724. doi:10.1039/c4ra05098c
Khan, S. A., Boltaev, G. S., Iqbal, M., Kim, V., Ganeev, R. A., and Alnaser, A. S. (2021). Ultrafast fiber laser-induced fabrication of superhydrophobic and self-cleaning metal surfaces. Appl. Surf. Sci. 542, 148560. doi:10.1016/j.apsusc.2020.148560
Khandelwal, N., Barbole, R. S., Banerjee, S. S., Chate, G. P., Biradar, A. V., Khandare, J. J., et al. (2016). Budding trends in integrated pest management using advanced micro- and nano-materials: Challenges and perspectives. J. Environ. Manag. 184, 157–169. doi:10.1016/j.jenvman.2016.09.071
Kim, J. H., Mirzaei, A., Kim, H. W., and Kim, S. S. (2018). Facile fabrication of superhydrophobic surfaces from austenitic stainless steel (AISI 304) by chemical etching. Appl. Surf. Sci. 439, 598–604. doi:10.1016/j.apsusc.2017.12.211
Koch, K., Bhushan, B., and Barthlott, W. (2008). Diversity of structure, morphology and wetting of plant surfaces. Soft Matter 4 (10), 1943. doi:10.1039/b804854a
Kong, L. H. (2021). An environmentally friendly method for fabrication of superhydrophobic “pipe” with loss-free liquid transportation properties. Surf. Coatings Technol. 407, 126777. doi:10.1016/j.surfcoat.2020.126777
Kostal, E., Stroj, S., Kasemann, S., Matylitsky, V., and Domke, M. (2018). Fabrication of biomimetic fog-collecting superhydrophilic–superhydrophobic surface micropatterns using femtosecond lasers. Langmuir 34 (9), 2933–2941. doi:10.1021/acs.langmuir.7b03699
Krishnan, A., Krishnan, A. V., Ajith, A., and Shibli, S. (2021). Influence of materials and fabrication strategies in tailoring the anticorrosive property of superhydrophobic coatings. Surfaces Interfaces 25, 101238. doi:10.1016/j.surfin.2021.101238
Kumar, A., and Gogoi, B. (2018). Development of durable self-cleaning superhydrophobic coatings for aluminium surfaces via chemical etching method. Tribol. Int. 122, 114–118. doi:10.1016/j.triboint.2018.02.032
Kumari, S., Tyagi, M., and Jagadevan, S. (2019). Mechanistic removal of environmental contaminants using biogenic nano-materials. Int. J. Environ. Sci. Technol. (Tehran). 16 (11), 7591–7606. doi:10.1007/s13762-019-02468-3
Lasagni, A. F., Alamri, S., Aguilar-Morales, A. I., RoBler, F., Voisiat, B., and Kunze, T. (2018). Biomimetic surface structuring using laser based interferometric methods. Appl. Sci. 8 (8), 1260. doi:10.3390/app8081260
Lee, D. H., Park, J. S., Hwang, J. H., Kang, D., Yim, S. Y., and Kim, J. H. (2018). Fabrication of hollow nanoporous gold nanoshells with high structural tunability based on the plasma etching of polymer colloid templates. J. Mat. Chem. C Mat. 6 (23), 6194–6199. doi:10.1039/c8tc01658e
Lee, R. E. (1979). Microfabrication by ion‐beam etching. J. Vac. Sci. Technol. 16 (2), 164–170. doi:10.1116/1.569897
Lee, S. G., Lim, H. S., Lee, D. Y., Kwak, D., and Cho, K. (2013). Tunable anisotropic wettability of rice leaf-like wavy surfaces. Adv. Funct. Mat. 23 (5), 547–553. doi:10.1002/adfm.201201541
Lee, Y., Ju, K. Y., and Lee, J. K. (2010). Stable biomimetic superhydrophobic surfaces fabricated by polymer replication method from hierarchically structured surfaces of Al templates. Langmuir 26 (17), 14103–14110. doi:10.1021/la102057p
Li, D., Wang, H., Luo, D., Liu, Y., Han, Z., and Ren, L. (2018). Corrosion resistance controllable of biomimetic superhydrophobic microstructured magnesium alloy by controlled adhesion. Surf. Coatings Technol. 347, 173–180. doi:10.1016/j.surfcoat.2018.04.078
Li, J., Liu, J., Huo, W., Yu, J., Liu, X., Haslinger, M., et al. (2022). Micro and nano materials and processing techniques for printed biodegradable electronics. Mater. Today Nano 18, 100201. doi:10.1016/j.mtnano.2022.100201
Li, K. K., Lei, J., Xie, Y. X., Lu, L., Zhang, S., Zhou, P., et al. (2021). An easy-to-implement method for fabricating superhydrophobic surfaces inspired by taro leaf. Sci. China Technol. Sci. 64 (12), 2676–2687. doi:10.1007/s11431-021-1855-2
Li, M. T., Liu, M., Yu, Y. H., Li, A. W., and Sun, H. B. (2019). Laser-structured graphene/reduced graphene oxide films towards bio-inspired superhydrophobic surfaces. Bull. Chem. Soc. Jpn. 92 (2), 283–289. doi:10.1246/bcsj.20180255
Li, P., Chen, X., Yang, G., Yu, L., and Zhang, P. (2014). Fabrication of a superhydrophobic etched copper–silver/stearic acid composite coating and evaluation of its friction-reducing and anticorrosion abilities. Mat. Express 4 (4), 309–316. doi:10.1166/mex.2014.1172
Li, X., Hetjens, L., Wolter, N., Li, H., Shi, X., and Pich, A. (2022b). Charge-reversible and biodegradable chitosan-based microgels for lysozyme-triggered release of vancomycin. J. Adv. Res. In Press. doi:10.1016/j.jare.2022.02.014
Li, X., Kong, L. D., Hu, W., Zhang, C., Pich, A., Shi, X., et al. (2022). Safe and efficient 2D molybdenum disulfide platform for cooperative imaging-guided photothermal-selective chemotherapy: A preclinical study. J. Adv. Res. 37, 255–266. doi:10.1016/j.jare.2021.08.004
Li, X., Li, H., Zhang, C., Pich, A., Xing, L., and Shi, X. (2021). Intelligent nanogels with self-adaptive responsiveness for improved tumor drug delivery and augmented chemotherapy. Bioact. Mater. 6 (10), 3473–3484. doi:10.1016/j.bioactmat.2021.03.021
Li, X., Lu, S., Xiong, Z., Hu, Y., Ma, D., Lou, W., et al. (2019). Light‐addressable nanoclusters of ultrasmall iron oxide nanoparticles for enhanced and dynamic magnetic resonance imaging of arthritis. Adv. Sci. (Weinh). 6 (19), 1901800. doi:10.1002/advs.201901800
Li, X., Ouyang, Z., Li, H., Hu, C., Saha, P., Xing, L., et al. (2021). Dendrimer-decorated nanogels: Efficient nanocarriers for biodistribution in vivo and chemotherapy of ovarian carcinoma. Bioact. Mater. 6 (10), 3244–3253. doi:10.1016/j.bioactmat.2021.02.031
Li, X., Shi, T., Li, B., Chen, X., Zhang, C., Guo, Z., et al. (2019). Subtractive manufacturing of stable hierarchical micro-nano structures on AA5052 sheet with enhanced water repellence and durable corrosion resistance. Mater. Des. 183, 108152. doi:10.1016/j.matdes.2019.108152
Li, X., Sun, H., Li, H., Hu, C., Luo, Y., Shi, X., et al. (2021). Multi‐responsive biodegradable cationic nanogels for highly efficient treatment of tumors. Adv. Funct. Mat. 31 (26), 2100227. doi:10.1002/adfm.202100227
Li, X., Xing, L., Hu, Y., Xiong, Z., Wang, R., Xu, X., et al. (2017). An RGD-modified hollow silica@Au core/shell nanoplatform for tumor combination therapy. Acta Biomater. 62, 273–283. doi:10.1016/j.actbio.2017.08.024
Li, X., Xing, L., Zheng, K., Wei, P., Du, L., Shen, M., et al. (2017). Formation of gold nanostar-coated hollow mesoporous silica for tumor multimodality imaging and photothermal therapy. ACS Appl. Mat. Interfaces 9 (7), 5817–5827. doi:10.1021/acsami.6b15185
Li, X., Xiong, Z., Xu, X., Luo, Y., Peng, C., Shen, M., et al. (2016). 99mTc-Labeled multifunctional low-generation dendrimer-entrapped gold nanoparticles for targeted SPECT/CT dual-mode imaging of tumors. ACS Appl. Mat. Interfaces 8 (31), 19883–19891. doi:10.1021/acsami.6b04827
Li, Y., Hu, J., Yang, K., Cao, B., Li, Z., Yang, L., et al. (2019). Synthetic control of Prussian blue derived nano-materials for energy storage and conversion application. Mater. Today Energy 14, 100332. doi:10.1016/j.mtener.2019.07.003
Li, Y., Liu, F., and Sun, J. (2009). A facile layer-by-layer deposition process for the fabrication of highly transparent superhydrophobic coatings. Chem. Commun. (19), 2730–2732. doi:10.1039/b900804g
Liao, R., Zuo, Z., Guo, C., Yuan, Y., and Zhuang, A. (2014). Fabrication of superhydrophobic surface on aluminum by continuous chemical etching and its anti-icing property. Appl. Surf. Sci. 317, 701–709. doi:10.1016/j.apsusc.2014.08.187
Liu, M., Wang, S., and Jiang, L. (2017). Nature-inspired superwettability systems. Nat. Rev. Mat. 2 (7), 17036. doi:10.1038/natrevmats.2017.36
Liu, R., Chi, Z., Cao, L., Weng, Z., Wang, L., Li, L., et al. (2020). Fabrication of biomimetic superhydrophobic and anti-icing Ti6Al4V alloy surfaces by direct laser interference lithography and hydrothermal treatment. Appl. Surf. Sci. 534, 147576. doi:10.1016/j.apsusc.2020.147576
Liu, S., Latthe, S. S., Yang, H., Liu, B., and Xing, R. (2015). Raspberry-like superhydrophobic silica coatings with self-cleaning properties. Ceram. Int. 41 (9), 11719–11725. doi:10.1016/j.ceramint.2015.05.137
Liu, S., Liu, X., Latthe, S. S., Gao, L., An, S., Yoon, S. S., et al. (2015). Self-cleaning transparent superhydrophobic coatings through simple sol–gel processing of fluoroalkylsilane. Appl. Surf. Sci. 351, 897–903. doi:10.1016/j.apsusc.2015.06.016
Liu, Y., Li, X., Jin, J., Liu, J., Yan, Y., Han, Z., et al. (2017). Anti-icing property of bio-inspired micro-structure superhydrophobic surfaces and heat transfer model. Appl. Surf. Sci. 400, 498–505. doi:10.1016/j.apsusc.2016.12.219
Liu, Y., Liu, J., Li, S., Han, Z., and Ren, L. (2013). Biomimetic superhydrophobic surface of high adhesion fabricated with micronano binary structure on aluminum alloy. ACS Appl. Mat. Interfaces 5 (18), 8907–8914. doi:10.1021/am4014715
Liu, Y., Yin, X., Zhang, J., Yu, S., Han, Z., and Ren, L. (2014). A electro-deposition process for fabrication of biomimetic super-hydrophobic surface and its corrosion resistance on magnesium alloy. Electrochimica Acta 125, 395–403. doi:10.1016/j.electacta.2014.01.135
Liu, Z., Yang, X., Pang, G., Zhang, F., Han, Y., Wang, X., et al. (2019). Temperature-based adhesion tuning and superwettability switching on superhydrophobic aluminum surface for droplet manipulations. Surf. Coatings Technol. 375, 527–533. doi:10.1016/j.surfcoat.2019.07.041
Lomga, J., Varshney, P., Nanda, D., Satapathy, M., Mohapatra, S., and Kumar, A. (2017). Fabrication of durable and regenerable superhydrophobic coatings with excellent self-cleaning and anti-fogging properties for aluminium surfaces. J. Alloys Compd. 702, 161–170. doi:10.1016/j.jallcom.2017.01.243
Lu, L., Zou, S., and Fang, B. (2021). The critical impacts of ligands on heterogeneous nanocatalysis: A review. ACS Catal. 11 (10), 6020–6058. doi:10.1021/acscatal.1c00903
Lu, Y., Yu, L., Zhang, Z., Wu, S., Li, G., Wu, P., et al. (2017). Biomimetic surfaces with anisotropic sliding wetting by energy-modulation femtosecond laser irradiation for enhanced water collection. RSC Adv. 7 (18), 11170–11179. doi:10.1039/c6ra28174e
Lv, Y., Song, C., Hou, Y., Shi, M., Li, Q., and Zhang, T. (2020). Bioinspired like lotus leaf hierarchical micropapillae structure for efficient oil-water separation and antibacterial performance. J. Dispersion Sci. Technol. 41 (11), 1690–1702. doi:10.1080/01932691.2019.1634588
Ma, L., Wang, L., Li, C., Guo, J., Shrotriya, P., Deng, C., et al. (2019). Hybrid nanosecond laser processing and heat treatment for rapid preparation of super-hydrophobic copper surface. Metals 9 (6), 668. doi:10.3390/met9060668
Manoharan, K., Anwar, M., and Bhattacharya, S. (2021). Development of hydrophobic paper substrates using silane and sol–gel based processes and deriving the best coating technique using machine learning strategies. Sci. Rep. 11 (1), 11352. doi:10.1038/s41598-021-90855-7
Michael, N., and Bhushan, B. (2007). Hierarchical roughness makes superhydrophobic states stable. Microelectron. Eng. 84 (3), 382–386. doi:10.1016/j.mee.2006.10.054
Milošev, I., Bakarič, T., Zanna, S., Seyeux, A., Rodic, P., Poberznik, M., et al. (2019). Electrochemical, surface-analytical, and computational DFT study of alkaline etched aluminum modified by carboxylic acids for corrosion protection and hydrophobicity. J. Electrochem. Soc. 166 (11), C3131–C3146. doi:10.1149/2.0181911jes
Ming, W., Wu, D., van Benthem, R., and de With, G. (2005). Superhydrophobic films from raspberry-like particles. Nano Lett. 5 (11), 2298–2301. doi:10.1021/nl0517363
Moghaddasi, Z., and Mohammadizadeh, M. R. (2022). Synthesis and effectiveness of Cu-infused TiO2-SiO2 based self-cleaning and antibacterial thin-films coating on ceramic tiles. J. Sol-Gel Sci. Technol. 1–9. doi:10.1007/s10971-022-05853-6
Mor, G. K., Carvalho, M. A., Varghese, O. K., Pishko, M. V., and Grimes, C. A. (2004). A room-temperature TiO2-nanotube hydrogen sensor able to self-clean photoactively from environmental contamination. J. Mat. Res. 19 (2), 628–634. doi:10.1557/jmr.2004.19.2.628
Nageswaran, G., Jothi, L., and Jagannathan, S. (2019). Plasma assisted polymer modifications Non-thermal plasma technology for polymeric materials. Amsterdam, Netherlands: Elsevier, 95–127.
Nanda, D., Sahoo, A., Kumar, A., and Bhushan, B. (2019). Facile approach to develop durable and reusable superhydrophobic/superoleophilic coatings for steel mesh surfaces. J. Colloid Interface Sci. 535, 50–57. doi:10.1016/j.jcis.2018.09.088
Nguyen, V. H., Nguyen, B. D., Pham, H. T., Lam, S. S., Vo, D. V. N., Shokouhimehr, M., et al. (2021). Anti-icing performance on aluminum surfaces and proposed model for freezing time calculation. Sci. Rep. 11 (1), 3641. doi:10.1038/s41598-020-80886-x
Nguyen-Tri, P., Altiparmak, F., Nguyen, N., Tuduri, L., Ouellet-Plamondon, C. M., and Prud’homme, R. E. (2019). Robust superhydrophobic cotton fibers prepared by simple dip-coating approach using chemical and plasma-etching pretreatments. Acs Omega 4 (4), 7829–7837. doi:10.1021/acsomega.9b00688
Nguyen-Tri, P., Tran, H. N., Plamondon, C. O., Tuduri, L., Vo, D. V. N., Nanda, S., et al. (2019). Recent progress in the preparation, properties and applications of superhydrophobic nano-based coatings and surfaces: A review. Prog. Org. Coatings 132, 235–256. doi:10.1016/j.porgcoat.2019.03.042
Nosonovsky, M., and Bhushan, B. (2008). Energy transitions in superhydrophobicity: Low adhesion, easy flow and bouncing. J. Phys. Condens. Matter 20 (39), 395005. doi:10.1088/0953-8984/20/39/395005
Ou, J., Zhu, W., Xie, C., and Xue, M. (2019). Mechanically robust and repairable superhydrophobic zinc coating via a fast and facile method for corrosion resisting. Materials 12 (11), 1779. doi:10.3390/ma12111779
Pan, Q., Cao, Y., Xue, W., Zhu, D., and Liu, W. (2019). Picosecond laser-textured stainless steel superhydrophobic surface with an antibacterial adhesion property. Langmuir 35 (35), 11414–11421. doi:10.1021/acs.langmuir.9b01333
Panda, B. (2021). Corrosion resistant superhydrophobic aluminum alloy: A review. IOP Conf. Ser. Mat. Sci. Eng. 1017 (1), 012008. doi:10.1088/1757-899x/1017/1/012008
Parin, R., Martucci, A., Sturaro, M., Bortolin, S., Bersani, M., Carraro, F., et al. (2018). Nano-structured aluminum surfaces for dropwise condensation. Surf. Coatings Technol. 348, 1–12. doi:10.1016/j.surfcoat.2018.05.018
Parkin, I. P., and Palgrave, R. G. (2005). Self-cleaning coatings. J. Mat. Chem. 15 (17), 1689. doi:10.1039/b412803f
Peng, F., Zhang, D., Liu, X., and Zhang, Y. (2021). Recent progress in superhydrophobic coating on Mg alloys: A general review. J. Magnesium Alloys 9 (5), 1471–1486. doi:10.1016/j.jma.2020.08.024
Peng, H., Luo, Z., Li, L., Xia, Z., Du, J., and Zheng, B. (2019). Facile fabrication of superhydrophobic aluminum surfaces by chemical etching and its anti-icing/self-cleaning performances. Mat. Res. Express 6 (9), 096586. doi:10.1088/2053-1591/ab3173
Peng, S., and Deng, W. (2015). A simple method to prepare superamphiphobic aluminum surface with excellent stability. Colloids Surfaces A Physicochem. Eng. Aspects 481, 143–150. doi:10.1016/j.colsurfa.2015.04.037
Qian, C., Guang-hua, C., Yan, F., and Lu-quan, R. (1900). Super-hydrophobic characteristics of butterfly wing surface. J. Bionic Eng. 1 (4), 249–255.
Qu, J., Yu, C., Cui, R., Qin, J., Wang, H., and Cao, Z. (2018). Preparation of super-hydrophobic and corrosion resistant colored films on chemically etched 304 stainless steel substrate. Surf. Coatings Technol. 354, 236–245. doi:10.1016/j.surfcoat.2018.09.022
Qu, M., Zhang, B., Song, S., Chen, L., Zhang, J., and Cao, X. (2007). Fabrication of superhydrophobic surfaces on engineering materials by a solution-immersion process. Adv. Funct. Mat. 17 (4), 593–596. doi:10.1002/adfm.200600472
Qu, R., Zhang, W., Li, X., Liu, Y., Wei, Y., Feng, L., et al. (2020). Peanut leaf-inspired hybrid metal–organic framework with humidity-responsive wettability: Toward controllable separation of diverse emulsions. ACS Appl. Mat. Interfaces 12 (5), 6309–6318. doi:10.1021/acsami.9b21118
Ran, M., Zheng, W., and Wang, H. (2019). Fabrication of superhydrophobic surfaces for corrosion protection: A review. Mater. Sci. Technol. 35 (3), 313–326. doi:10.1080/02670836.2018.1560985
Rasouli, S., Rezaei, N., Hamedi, H., Zendehboudi, S., and Duan, X. (2021). Superhydrophobic and superoleophilic membranes for oil-water separation application: A comprehensive review. Mater. Des. 204, 109599. doi:10.1016/j.matdes.2021.109599
Richard, D., Clanet, C., and Quéré, D. (2002). Contact time of a bouncing drop. Nature 417 (6891), 811. doi:10.1038/417811a
Rius-Ayra, O., Castellote-Alvarez, R., Escobar, A. M., and Llorca-Isern, N. (2018). Superhydrophobic coating bioinspired on rice leaf: A first attempt to enhance erosion resistance properties at environmental conditions with ceramic particles[C]//Materials science forum. Trans. Tech. Publ. Ltd. 941, 1874–1879. doi:10.4028/www.scientific.net/MSF.941.1874
Rodič, P., Kapun, B., and Milošev, I. (2022). Superhydrophobic aluminium surface to enhance corrosion resistance and obtain self-cleaning and anti-icing ability. Molecules 27 (3), 1099. doi:10.3390/molecules27031099
Rodič, P., Kapun, B., Panjan, M., and Milosev, I. (2020). Easy and fast fabrication of self-cleaning and anti-icing perfluoroalkyl silane film on aluminium. Coatings (Basel). 10 (3), 234. doi:10.3390/coatings10030234
Rodič, P., and Milošev, I. (2019). One-step ultrasound fabrication of corrosion resistant, self-cleaning and anti-icing coatings on aluminium. Surf. Coatings Technol. 369, 175–185. doi:10.1016/j.surfcoat.2019.03.082
Román‐Kustas, J., Hoffman, J. B., Reed, J. H., Gonsalves, A. E., Oh, J., Li, L., et al. (2020). Molecular and topographical organization: Influence on cicada wing wettability and bactericidal properties. Adv. Mat. Interfaces 7 (10), 2000112. doi:10.1002/admi.202000112
Rong, W., Zhang, H., Mao, Z., Chen, L., and Liu, X. (2021). Stable drag reduction of anisotropic superhydrophobic/hydrophilic surfaces containing bioinspired micro/nanostructured arrays by laser ablation. Colloids Surfaces A Physicochem. Eng. Aspects 622, 126712. doi:10.1016/j.colsurfa.2021.126712
Rus, A. Z. M., Mohid, S. R., Nurulsaidatulsyida, S., and Marsi, N. (2013). Biopolymer doped with titanium dioxide superhydrophobic photocatalysis as self-clean coating for lightweight composite. Adv. Mater. Sci. Eng., 2013, 486253. doi:10.1155/2013/486253
Sajjadi, M., Ahmadpoor, F., Nasrollahzadeh, M., and Ghafuri, H. (2021). Lignin-derived (nano)materials for environmental pollution remediation: Current challenges and future perspectives. Int. J. Biol. Macromol. 178, 394–423. doi:10.1016/j.ijbiomac.2021.02.165
Saleema, N., Sarkar, D. K., Paynter, R. W., and Chen, X. G. (2010). Superhydrophobic aluminum alloy surfaces by a novel one-step process. ACS Appl. Mat. Interfaces 2 (9), 2500–2502. doi:10.1021/am100563u
Saleh, T. A., Baig, N., Alghunaimi, F. I., and Aljuryyed, N. W. (2020). A flexible biomimetic superhydrophobic and superoleophilic 3D macroporous polymer-based robust network for the efficient separation of oil-contaminated water. RSC Adv. 10 (9), 5088–5097. doi:10.1039/c9ra06579b
Saleh, T. A., and Baig, N. (2019). Efficient chemical etching procedure for the generation of superhydrophobic surfaces for separation of oil from water. Prog. Org. Coatings 133, 27–32. doi:10.1016/j.porgcoat.2019.03.049
Sam, E. K., Sam, D. K., Lv, X., Padmanabhan, D., Wesley, R. J., Obadiah, A., et al. (2019). Recent development in the fabrication of self-healing superhydrophobic surfaces. Chem. Eng. J. 373, 531–546. doi:10.1016/j.cej.2019.05.077
Scalisi, F. (2017). Nano-materials for architecture. J. Civ. Eng. Archit. 11, 1061. doi:10.17265/1934-7359/2017.12.001
Sethi, S. K., Kadian, S., Chauhan, R. P., Goel, R, and Manik, G. (2020). Fabrication and analysis of ZnO quantum dots based easy clean coating: A combined theoretical and experimental investigation. ChemistrySelect 5 (29), 8942–8950. doi:10.1002/slct.202001092
Sethi, S. K., Kadian, S., and Manik, G. (2022). A review of recent progress in molecular dynamics and coarse-grain simulations assisted understanding of wettability. Archives Comput. Methods Eng. 29, 3059–3085. doi:10.1007/s11831-021-09689-1
Sethi, S. K., and Manik, G. (2018). Recent progress in super hydrophobic/hydrophilic self-cleaning surfaces for various industrial applications: A review. Polymer-Plastics Technol. Eng. 57 (18), 1932–1952. doi:10.1080/03602559.2018.1447128
Sethi, S. K., Shankar, U., and Manik, G. (2019). Fabrication and characterization of non-fluoro based transparent easy-clean coating formulations optimized from molecular dynamics simulation. Prog. Org. Coatings 136, 105306. doi:10.1016/j.porgcoat.2019.105306
Sethi, S. K., Soni, L., Shankar, U., Chauhan, R. P., and Manik, G. (2020). A molecular dynamics simulation study to investigate poly(vinyl acetate)-poly(dimethyl siloxane) based easy-clean coating: An insight into the surface behavior and substrate interaction. J. Mol. Struct. 1202, 127342. doi:10.1016/j.molstruc.2019.127342
Shaikh, M. O., Yang, J. Y., and Chuang, C. H. (2021). Thermally stable and durable superhydrophobic surfaces on stainless steel sheets with microholes via acid pretreatment and modification with self-assembled monolayers. Microfluid. Nanofluidics 25 (11), 1–9. doi:10.1007/s10404-021-02499-8
Shao, C., Chi, J., Chen, Z., Cai, L., and Zhao, Y. (2019). Superwettable colloidal crystal micropatterns on butterfly wing surface for ultrasensitive detection. J. colloid interface Sci. 546, 122–129. doi:10.1016/j.jcis.2019.03.064
Sharma, K., Hooda, A., Goyat, M. S., Rai, R., and Mittal, A. (2021). A review on challenges, recent progress and applications of silica nanoparticles based superhydrophobic coatings. Ceram. Int. 48, 5922–5938. doi:10.1016/j.ceramint.2021.11.239
Siddiqui, M. T. H., Nizamuddin, S., Baloch, H. A., Mubarak, N., Al-Ali, M., Mazari, S. A., et al. (2019). Fabrication of advance magnetic carbon nano-materials and their potential applications: A review. J. Environ. Chem. Eng. 7 (1), 102812. doi:10.1016/j.jece.2018.102812
Song, J., Xu, W., Liu, X., Lu, Y., Wei, Z., and Wu, L. (2012). Ultrafast fabrication of rough structures required by superhydrophobic surfaces on Al substrates using an immersion method. Chem. Eng. J. 211, 143–152. doi:10.1016/j.cej.2012.09.094
Song, R., Zhang, N., Dong, H., Wang, P., Ding, H., Wang, J., et al. (2022). Three-dimensional biomimetic superhydrophobic nickel sponge without chemical modifications for efficient oil/water separation. Sep. Purif. Technol. 289, 120723. doi:10.1016/j.seppur.2022.120723
Stoddart, P. R., Cadusch, P. J., Boyce, T. M., Erasmus, R. M., and Comins, J. D. (2006). Optical properties of chitin: Surface-enhanced Raman scattering substrates based on antireflection structures on cicada wings. Nanotechnology 17 (3), 680–686. doi:10.1088/0957-4484/17/3/011
Stratakis, E., Bonse, J., Heitz, J., Siegel, J., Tsibidis, G., Skoulas, E., et al. (2020). Laser engineering of biomimetic surfaces. Mater. Sci. Eng. R Rep. 141, 100562. doi:10.1016/j.mser.2020.100562
Stratakis, E., Ranella, A., and Fotakis, C. (2011). Biomimetic micro/nanostructured functional surfaces for microfluidic and tissue engineering applications. Biomicrofluidics 5 (1), 013411. doi:10.1063/1.3553235
Sun, W., Wang, L., Yang, Z., Li, S., Wu, T., and Liu, G. (2017). Fabrication of polydimethylsiloxane-derived superhydrophobic surface on aluminium via chemical vapour deposition technique for corrosion protection. Corros. Sci. 128, 176–185. doi:10.1016/j.corsci.2017.09.005
Sun, Y., and Guo, Z. (2019). Recent advances of bioinspired functional materials with specific wettability: From nature and beyond nature. Nanoscale Horiz. 4 (1), 52–76. doi:10.1039/c8nh00223a
Sun, Y., Sui, X., Wang, Y., Liang, W., and Wang, F. (2020). Passive anti-icing and active electrothermal deicing system based on an ultraflexible carbon nanowire (CNW)/PDMS biomimetic nanocomposite with a superhydrophobic microcolumn surface. Langmuir 36 (48), 14483–14494. doi:10.1021/acs.langmuir.0c01745
Suresh Kumar, N., Padma Suvarna, R., Chandra Babu Naidu, K., Ratnamala, A., and Manjunatha, H. (2020). A review on biological and biomimetic materials and their applications. Appl. Phys. A 126 (6), 1–18. doi:10.1007/s00339-020-03633-z
Tadanaga, K., Morinaga, J., Matsuda, A., and Minami, T. (2000). Superhydrophobic−Superhydrophilic micropatterning on flowerlike alumina coating film by the Sol−Gel method. Chem. Mat. 12 (3), 590–592. doi:10.1021/cm990643h
Tavana, H., Amirfazli, A., and Neumann, A. W. (2006). Fabrication of superhydrophobic surfaces of n-hexatriacontane. Langmuir 22 (13), 5556–5559. doi:10.1021/la0607757
Tian, G., Xing, Z., Xie, Y., Deng, C., Wu, X., and Sun, X. (2017). “Aluminum and stainless steel base bionic super-hydrophobic surface preparation technology using in space instruments,” in 2017 Chinese Automation Congress (CAC), Jinan, China, 20-22 October 2017 (IEEE), 5690–5694.
Tudu, B. K., Kumar, A., and Bhushan, B. (2019). Facile approach to develop anti-corrosive superhydrophobic aluminium with high mechanical, chemical and thermal durability. Phil. Trans. R. Soc. A 377 (2138), 20180272. doi:10.1098/rsta.2018.0272
Tuo, Y., Zhang, H., Rong, W., Jiang, S., Chen, W., and Liu, X. (2019). Drag reduction of anisotropic superhydrophobic surfaces prepared by laser etching. Langmuir 35 (34), 11016–11022. doi:10.1021/acs.langmuir.9b01040
Varshney, P., Mohapatra, S. S., and Kumar, A. (2016). Superhydrophobic coatings for aluminium surfaces synthesized by chemical etching process. Int. J. Smart Nano Mater. 7 (4), 248–264. doi:10.1080/19475411.2016.1272502
Waked, A. M. (2011). Nano materials applications for conservation of cultural heritage. WIT Trans. Built Environ. 118, 577–588. doi:10.2495/STR110481
Wan, Y., Chen, M., Liu, W., Shen, X., Min, Y., and Xu, Q. (2018). The research on preparation of superhydrophobic surfaces of pure copper by hydrothermal method and its corrosion resistance. Electrochimica Acta 270, 310–318. doi:10.1016/j.electacta.2018.03.060
Wan, Y., Wang, X., Zhang, M., Xu, J., and Yu, H. (2022). Biomimetic bamboo leaf double-sided microstructure composite surface and its delayed icing performance. Surf. Topogr. Metrology Prop. doi:10.1088/2051-672X/ac6c3e
Wan, Y., Zhang, C., Zhang, M., and Xu, J. (2021). Anti-condensation behavior of bamboo leaf surface (backside) and its bionic preparation. Mat. Res. Express 8 (5), 055002. doi:10.1088/2053-1591/abfc01
Wang, B., Liang, W., Guo, Z., and Liu, W. (2015). Biomimetic super-lyophobic and super-lyophilic materials applied for oil/water separation: A new strategy beyond nature. Chem. Soc. Rev. 44 (1), 336–361. doi:10.1039/c4cs00220b
Wang, D., Zhao, A., Jiang, R., Li, D., Zhang, M., Gan, Z., et al. (2012). Surface properties of bionic micro-pillar arrays with various shapes of tips. Appl. Surf. Sci. 259, 93–98. doi:10.1016/j.apsusc.2012.06.106
Wang, G., Zeng, Z., Wang, H., Zhang, L., Sun, X., He, Y., et al. (2015). Low drag porous ship with superhydrophobic and superoleophilic surface for oil spills cleanup. ACS Appl. Mat. Interfaces 7 (47), 26184–26194. doi:10.1021/acsami.5b08185
Wang, H., Wei, Y., Liang, M., Hou, L., Li, Y., and Guo, C. (2016). Fabrication of stable and corrosion-resisted super-hydrophobic film on Mg alloy. Colloids Surfaces A Physicochem. Eng. Aspects 509, 351–358. doi:10.1016/j.colsurfa.2016.09.027
Wang, S., and Jiang, L. (2007). Definition of superhydrophobic states. Adv. Mat. 19 (21), 3423–3424. doi:10.1002/adma.200700934
Wang, S., Yang, Z., Gong, G., Wang, J., Wu, J., Yang, S., et al. (2016). Icephobicity of penguins Spheniscus humboldti and an artificial replica of penguin feather with air-infused hierarchical rough structures. J. Phys. Chem. C 120 (29), 15923–15929. doi:10.1021/acs.jpcc.5b12298
Wang, X., Ding, H., Sun, S., Zhang, H., Zhou, R., Li, Y., et al. (2021). Preparation of a temperature-sensitive superhydrophobic self-cleaning SiO2-TiO2@ PDMS coating with photocatalytic activity. Surf. Coatings Technol. 408, 126853. doi:10.1016/j.surfcoat.2021.126853
Wang, X., Yu, H., Li, P., Zhang, Y., Wen, Y., Qiu, Y., et al. (2021). Femtosecond laser-based processing methods and their applications in optical device manufacturing: A review. Opt. Laser Technol. 135, 106687. doi:10.1016/j.optlastec.2020.106687
Watson, G. S., Green, D. W., Schwarzkopf, L., Li, X., Cribb, B. W., Myhra, S., et al. (2015). A gecko skin micro/nano structure – a low adhesion, superhydrophobic, anti-wetting, self-cleaning, biocompatible, antibacterial surface. Acta biomater. 21, 109–122. doi:10.1016/j.actbio.2015.03.007
Watson, G. S., and Watson, J. A. (2004). Natural nano-structures on insects—Possible functions of ordered arrays characterized by atomic force microscopy. Appl. Surf. Sci. 235 (1-2), 139–144. doi:10.1016/j.apsusc.2004.05.129
Wei, D., Wang, J., Li, S., Liu, Y., and Wang, H. (2021). Novel corrosion-resistant behavior and mechanism of a biomimetic surface with switchable wettability on Mg alloy. Chem. Eng. J. 425, 130450. doi:10.1016/j.cej.2021.130450
Wei, D., Wang, J., Liu, Y., Li, S., and Wang, H. (2021). Controllable superhydrophobic surfaces with tunable adhesion on Mg alloys by a simple etching method and its corrosion inhibition performance. Chem. Eng. J. 404, 126444. doi:10.1016/j.cej.2020.126444
Wenzel, R. N. (1936). Resistance of solid surfaces to wetting by water. Ind. Eng. Chem. 28 (8), 988–994. doi:10.1021/ie50320a024
Wong, T. S., Kang, S. H., Tang, S. K. Y., Smythe, E. J., Hatton, B. D., Grinthal, A., et al. (2011). Bioinspired self-repairing slippery surfaces with pressure-stable omniphobicity. Nature 477 (7365), 443–447. doi:10.1038/nature10447
Wu, D., Wang, J. N., Wu, S. Z., Chen, Q. D., Zhao, S., Zhang, H., et al. (2011). Three-level biomimetic rice-leaf surfaces with controllable anisotropic sliding. Adv. Funct. Mat. 21 (15), 2927–2932. doi:10.1002/adfm.201002733
Wu, R., Liang, S., Pan, A., Yuan, Z., Tang, Y., Tan, X., et al. (2012). Fabrication of nano-structured super-hydrophobic film on aluminum by controllable immersing method. Appl. Surf. Sci. 258 (16), 5933–5937. doi:10.1016/j.apsusc.2011.10.029
Wu, Y., Du, J., Liu, G., Chen, W.-Q., Chen, S., and Du, T. (2021). A review of self-cleaning technology to reduce dust and ice accumulation in photovoltaic power generation using superhydrophobic coating. Renew. Energy 185, 1034–1061. doi:10.1016/j.renene.2021.12.123
Xia, A., He, L., Qie, S., Zhang, J., Li, H., He, N., et al. (2022). Fabrication of an anti-icing aluminum alloy surface by combining wet etching and laser machining. Appl. Sci. 12 (4), 2119. doi:10.3390/app12042119
Xie, H., Huang, H. X., and Peng, Y. J. (2017). Rapid fabrication of bio-inspired nanostructure with hydrophobicity and antireflectivity on polystyrene surface replicating from cicada wings. Nanoscale 9 (33), 11951–11958. doi:10.1039/c7nr04176d
Xing, L., Zhou, Q., Chen, G., Sun, G., and Xing, T. (2022). Recent developments in preparation, properties, and applications of superhydrophobic textiles. Text. Res. J. doi:10.0040/5175221097716
Xu, M., Feng, Y., Li, Z., Wang, X., Li, C., Jiang, H., et al. (2019). A novel, efficient and cost-effective synthesis technique for the development of superhydrophobic glass surface. J. Alloys Compd. 781, 1175–1181. doi:10.1016/j.jallcom.2018.12.084
Xu, Q. F., Mondal, B., and Lyons, A. M. (2011). Fabricating superhydrophobic polymer surfaces with excellent abrasion resistance by a simple lamination templating method. ACS Appl. Mat. Interfaces 3 (9), 3508–3514. doi:10.1021/am200741f
Yang, H., Dou, X., Fang, Y., and Jiang, P. (2013). Self-assembled biomimetic superhydrophobic hierarchical arrays. J. Colloid Interface Sci. 405, 51–57. doi:10.1016/j.jcis.2013.05.040
Yang, H., Gao, Y., Qin, W., Sun, J., Huang, Z., Li, Y., et al. (2022). A robust superhydrophobic surface on AA3003 aluminum alloy with intermetallic phases in-situ pinning effect for corrosion protection. J. Alloys Compd. 898, 163038. doi:10.1016/j.jallcom.2021.163038
Yang, L., Shen, X., Yang, Q., Liu, J., Wu, W., Li, D., et al. (2021). Fabrication of biomimetic anisotropic super-hydrophobic surface with rice leaf-like structures by femtosecond laser. Opt. Mater. 112, 110740. doi:10.1016/j.optmat.2020.110740
Yang, S., Ju, J., Qiu, Y., He, Y., Wang, X., Dou, S., et al. (2014). Superhydrophobic materials: Peanut leaf inspired multifunctional surfaces (small 2/2014). Small 10 (2), 214. doi:10.1002/smll.201470010
Yang, Y., Li, X., Zheng, X., Chen, Z., Zhou, Q., and Chen, Y. (2018). 3D‐Printed biomimetic super‐hydrophobic structure for microdroplet manipulation and oil/water separation. Adv. Mat. 30 (9), 1704912. doi:10.1002/adma.201704912
Yang, Z., Liu, X., and Tian, Y. (2019). Hybrid laser ablation and chemical modification for fast fabrication of bio-inspired super-hydrophobic surface with excellent self-cleaning, stability and corrosion resistance. J. Bionic Eng. 16 (1), 13–26. doi:10.1007/s42235-019-0002-y
Yao, M., Zhang, P., Nie, J., and He, Y. (2021). The superhydrophobic fluorine‐containing material prepared through biomimetic UV lithography for oil–water separation and anti‐bioadhesion. Macromol. Chem. Phys. 222 (17), 2100149. doi:10.1002/macp.202100149
Yeganeh, M., and Mohammadi, N. (2018). Superhydrophobic surface of Mg alloys: A review. J. Magnesium Alloys 6 (1), 59–70. doi:10.1016/j.jma.2018.02.001
Yilgor, I., Bilgin, S., Isik, M., and Yilgor, E. (2012). Facile preparation of superhydrophobic polymer surfaces. Polymer 53 (6), 1180–1188. doi:10.1016/j.polymer.2012.01.053
Yong, J., Yang, Q., Chen, F., Zhang, D., Farooq, U., Du, G., et al. (2014). A simple way to achieve superhydrophobicity, controllable water adhesion, anisotropic sliding, and anisotropic wetting based on femtosecond-laser-induced line-patterned surfaces. J. Mat. Chem. A 2 (15), 5499–5507. doi:10.1039/c3ta14711h
Young, T. (1805). An essay on the cohesion of fluids. Philosophical Trans. R. Soc. Lond. 95, 65–87. doi:10.1098/rstl.1805.0005
Yuan, Z., Bin, J., Wang, X., Peng, C., Wang, M., Xing, S., et al. (2014). Fabrication of superhydrophobic surface with hierarchical multi-scale structure on copper foil. Surf. Coatings Technol. 254, 151–156. doi:10.1016/j.surfcoat.2014.06.004
Zhan, Y., Yu, S., Amirfazli, A., Rahim Siddiqui, A., and Li, W. (2022). Recent advances in antibacterial superhydrophobic coatings. Adv. Eng. Mat. 24 (4), 2101053. doi:10.1002/adem.202101053
Zhang, B., Xu, W., Zhu, Q., Guan, F., and Zhang, Y. (2022). Nepenthes pitcher-inspired lubricant-infused slippery surface with superior anti-corrosion durability, hot water repellency and scratch resistance. J. Industrial Eng. Chem. 107, 259–267. doi:10.1016/j.jiec.2021.11.052
Zhang, G., Zhang, J., Xie, G., Liu, Z., and Shao, H. (2006). Cicada wings: A stamp from nature for nanoimprint lithography. Small 2 (12), 1440–1443. doi:10.1002/smll.200600255
Zhang, H., Yang, J., Chen, B., Liu, C., Zhang, M., and Li, C. (2015). Fabrication of superhydrophobic textured steel surface for anti-corrosion and tribological properties. Appl. Surf. Sci. 359, 905–910. doi:10.1016/j.apsusc.2015.10.191
Zhang, X., Zhao, J., Mo, J., Sun, R., Li, Z., and Guo, Z. (2019). Fabrication of superhydrophobic aluminum surface by droplet etching and chemical modification. Colloids Surfaces A Physicochem. Eng. Aspects 567, 205–212. doi:10.1016/j.colsurfa.2019.01.046
Zhang, Y., Liu, Z., Chen, A., Wang, Q., Zhang, J., Zhao, C., et al. (2020). Fabrication of micro-/submicro-/nanostructured polypropylene/graphene superhydrophobic surfaces with extreme dynamic pressure resistance assisted by single hierarchically porous anodic aluminum oxide template. J. Phys. Chem. C 124 (11), 6197–6205. doi:10.1021/acs.jpcc.9b12038
Zhang, Z., Zhao, J., Lei, Y., Wang, Y., Zhou, G., Xu, C., et al. (2020). Preparation of intricate nanostructures on 304 stainless steel surface by SiO2-assisted HF etching for high superhydrophobicity. Colloids Surfaces A Physicochem. Eng. Aspects 586, 124287. doi:10.1016/j.colsurfa.2019.124287
Zhao, H., Sun, Q., Deng, X., and Cui, J. (2018). Earthworm-Inspired rough polymer coatings with self-replenishing lubrication for adaptive friction-reduction and antifouling surfaces. Adv. Mat. 30 (29), 1802141. doi:10.1002/adma.201802141
Zheng, H., Chang, S., Ma, G., and Wang, S. (2020). Anti-icing performance of superhydrophobic surface fabricated by femtosecond laser composited dual-layers coating. Energy Build. 223, 110175. doi:10.1016/j.enbuild.2020.110175
Zheng, S., Li, C., Zhang, Y., Xiang, T., Cao, Y., Li, Q., et al. (2021). A general strategy towards superhydrophobic self-cleaning and anti-corrosion metallic surfaces: An example with aluminum alloy. Coatings (Basel). 11 (7), 788. doi:10.3390/coatings11070788
Zheng, Y., Bai, H., Huang, Z., Tian, X., Nie, F. Q., Zhao, Y., et al. (2010). Directional water collection on wetted spider silk. Nature 463 (7281), 640–643. doi:10.1038/nature08729
Zheng, Y., Gao, X., and Jiang, L. (2007). Directional adhesion of superhydrophobic butterfly wings. Soft Matter 3 (2), 178–182. doi:10.1039/b612667g
Zheng, Y., Zhang, C., Wang, J., Liu, Y., Shen, C., and Yang, J. (2019). Robust adhesion of droplets via heterogeneous dynamic petal effects. J. colloid interface Sci. 557, 737–745. doi:10.1016/j.jcis.2019.09.070
Zhu, Z., Zhang, Y., and Sun, D. W. (2021). Biomimetic modification of freezing facility surfaces to prevent icing and frosting during freezing for the food industry. Trends Food Sci. Technol. 111, 581–594. doi:10.1016/j.tifs.2021.02.034
Keywords: bionic modification, superhydrophobic surface, micro/nanostructure, etching modification method, chemical etching method, laser-etching method
Citation: Ge-Zhang S, Yang H, Ni H, Mu H and Zhang M (2022) Biomimetic superhydrophobic metal/nonmetal surface manufactured by etching methods: A mini review. Front. Bioeng. Biotechnol. 10:958095. doi: 10.3389/fbioe.2022.958095
Received: 31 May 2022; Accepted: 04 July 2022;
Published: 05 August 2022.
Edited by:
Xin Li, DWI – Leibniz-Institut für Interaktive Materialien, GermanyReviewed by:
Longgang Wang, Yanshan University, ChinaCopyright © 2022 Ge-Zhang, Yang, Ni, Mu and Zhang. This is an open-access article distributed under the terms of the Creative Commons Attribution License (CC BY). The use, distribution or reproduction in other forums is permitted, provided the original author(s) and the copyright owner(s) are credited and that the original publication in this journal is cited, in accordance with accepted academic practice. No use, distribution or reproduction is permitted which does not comply with these terms.
*Correspondence: Hongbo Mu, bWhiNTA2QG5lZnUuZWR1LmNu; Mingming Zhang, emhhbmdtaW5nbWluZ0BocmJtdS5lZHUuY24=
†These authors have contributed equally to this work
Disclaimer: All claims expressed in this article are solely those of the authors and do not necessarily represent those of their affiliated organizations, or those of the publisher, the editors and the reviewers. Any product that may be evaluated in this article or claim that may be made by its manufacturer is not guaranteed or endorsed by the publisher.
Research integrity at Frontiers
Learn more about the work of our research integrity team to safeguard the quality of each article we publish.