- 1Department of Medical Biology, Faculty of Medicine, Balıkesir University, Balıkesir, Turkey
- 2Department of Medical Biochemistry, Faculty of Medicine, Near East University, Nicosia, Mersin, Turkey
Although ongoing medical research is working to find a cure for a variety of cancers, it continues to be one of the major causes of death worldwide. Chemotherapy and immunotherapy, as well as surgical intervention and radiation therapy, are critical components of cancer treatment. Most anti-cancer drugs are given systemically and distribute not just to tumor tissues but also to normal tissues, where they may cause side effects. Furthermore, because anti-cancer drugs have a low delivery efficiency, some tumors do not respond to them. As a result, tumor-targeted drug delivery is critical for improving the safety and efficacy of anti-cancer treatment. Exosomes are microscopic extracellular vesicles that cells produce to communicate with one another. MicroRNA (miRNA), long non-coding RNA (lncRNA), small interfering RNA (siRNA), DNA, protein, and lipids are among the therapeutic cargos found in exosomes. Recently, several studies have focused on miRNAs as a potential therapeutic element for the treatment of cancer. Mesenchymal stem cells (MSC) have been known to have angiogenic, anti-apoptotic, anti-inflammatory and immunomodulatory effects. Exosomes derived from MSCs are gaining popularity as a non-cellular alternative to MSC-based therapy, as this method avoids unwanted lineage differentiation. Therefore more research have focused on transferring miRNAs to mesenchymal stem cells (MSC) and targeting miRNA-loaded exosomes to cancer cells. Here, we initially gave an overview of the characteristics and potentials of MSC as well as the use of MSC-derived exosomes in cancer therapy. Finally, we emphasized the utilization of MSC-derived exosomes for miRNA delivery in the treatment of cancer.
Introduction
The human body comprises a variety of cell types that make up tissues and organs with distinct functions that contribute to long-term survival. Long ago, it was discovered that differentiated cells in several tissues, such as the skin, intestinal epithelium, and blood, have a short lifecycle and are unable to self-renew (Watt and Driskell, 2010). Stem cells may self-renew and have the ability to differentiate into a variety of cell types in an organism. This discovery gave rise to the concept of stem cells, which are small unspecialized cells in the human body that lack a variety of phenotypic features observed in adult tissues and are used to maintain static and temporary cell types (Alvarez et al., 2012). Embryonic and non-embryonic stem cells (somatic stem cells) are the two basic types of stem cells. Embryonic stem cells are pluripotent, but somatic stem cells, mesenchymal stem cells (MSCs), for example, are multipotent stem cells (Singh et al., 2016). Because of their unique properties, such as self-renewal and the ability to differentiate into a variety of cell types, MSCs are among the most studied stem cells (Pittenger et al., 2019).
Friedenstein and colleagues were the first to isolate and define MSCs from bone marrow as adherent, highly replicative cells that can differentiate into mesodermal lineages such as osteoblasts, chondrocytes, adipocytes, and hematopoietic stroma (Friedenstein et al., 1966). In addition to bone marrow, MSCs can be isolated from variety of tissues (da Silva Meirelles et al., 2006). According to the International Society for Cellular Therapy (ISCT), MSCs must fulfill three minimal conditions; (1) adherence to plastic surface when cultured in vitro, (2) expression of the surface antigens CD73, CD90, and CD105, and absence of CD34, CD45, CD14 or CD11b, CD79α or CD19, and HLA-DR, (3) ability to form several mesodermal cell types, such as adipocytes, chondrocytes, and osteoblasts when cultured in vitro under appropriate conditions (Dominici et al., 2006).
MSCs are attractive therapeutic targets for a variety of disorders, including cancer treatment and tissue regeneration, because of their versatility and ability to self-renew. MSCs have undeniable medical potential; yet, their capacity to develop into tumor-associated fibroblasts (Mishra et al., 2008; Miyazaki et al., 2021), which promote tumor growth via their secretome (Liang W. et al., 2021), and resistance to apoptosis, makes them potentially dangerous (Bellagamba et al., 2016). MSCs have not been successfully used in anticancer therapy because of their contradictory involvement in cancer progression and regression. To effectively harness MSCs’ therapeutic potential, it is critical to understand their underlying molecular pathways.
Exosomes are extracellular vesicles (EVs) produced by eukaryotic cells that serve as carriers for the transfer of membrane and cytosolic proteins, lipids, and RNA between cells, making them a key component of intercellular communication (Raposo and Stoorvogel, 2013). These membrane–bounded vesicles can be divided into three subtypes, exosomes (50–150 nm), microvesicles (100–1,1000 nm), and apoptotic bodies (500–5,5000 nm) (Doyle and Wang, 2019). Exosomes and other EVs have been found in a variety of tissues and biological fluids, including urine, blood, and cerebrospinal fluid. MicroRNAs (miRNAs) and proteins are mostly found in exosomes, which are enclosed by a lipid bilayer membrane (Zhang et al., 2018). Exosomes also contain other RNA types such as nucleolar RNA, long noncoding RNA, and ribosomal RNA, as well as DNA fragments (Sato-Kuwabara et al., 2015). Studies have shown that released exosomes can be guided to other cells via proteins found on cell surfaces (Neviani and Fabbri, 2015).
Exosomes derived from MSCs have been shown to possess potential benefits for the management of several pathological conditions, including cancer. MSC-derived exosomes have almost all of the properties of the original cells, in terms of paracrine effects and immunomodulatory functions. Recently, loading MSC-derived exosomes with defined cargos such as miRNAs has been suggested to be a promising strategy for the treatment of different diseases. Even more, genetically engineered miRNAs can be used in correcting the pathways disrupted in cancer. In the present review we discuss the function of exosomal miRNAs derived from MSCs in different type of cancers.
Biological functions of mesenchymal stem cells
MSCs share many properties with other stem cells, including robust self-renewal and multidirectional differentiation capacity. In previous studies, MSCs have been shown to be capable of differentiating into cells of the mesodermal, ectodermal, and endodermal lineages (Dominici et al., 2006; Paunescu et al., 2007). MSCs can regulate the immune system by interacting with immune cells and also have paracrine effects. Furthermore, because MSCs have a low immunogenicity, allograft matching requirements are less stringent, and immunological rejection is less likely. MSCs can thus be used as ideal seed cells for repairing tissue and organ damage caused by aging and pathological changes, and they also have broad clinical applications in the treatment of autoimmune diseases, inflammation-related diseases, and cancer (Farini et al., 2014).
MSCs exert their immunomodulatory activity through interacting with immune cells in both the native and acquired immune systems. First, MSCs decrease natural killer (NK) cell proliferation, cytotoxicity, and cytokine secretion by secreting prostaglandin E2 (PGE2), indoleamine 2,3-dioxygenase (IDO), and soluble human leukocyte antigen G5 (sHLA-G5) (Galland et al., 2017). MSCs can also influence the development of dendritic cells (DCs) by suppressing monocyte differentiation into DCs (Jung et al., 2007). MSCs can limit the expression of tumour necrosis factor (TNF) (Yan et al., 2018) and enhance the expression of interleukin 10 (IL-10) (Selleri et al., 2013) by DCs, which is likewise regulated by PGE2. MSCs also decrease the ability of naïve T cells to induce Th1 differentiation (Consentius et al., 2015), ultimately leading to immunosuppression.
In 2008, Le Blanc and Davies reported success in the treatment of graft versus host disease (GVHD) with allogeneic, semicompatible, and mismatched bone marrow-derived MSC transplantation, indicating that a strict match was not necessary in the treatment of GVHD with MSCs (Le Blanc and Davies, 2015). The low immunogenicity of MSCs is crucial to the success of allogeneic MSC transplantation in preclinical and clinical settings. MSCs express major histocompatibility complex class I (MHC I) and lymphocyte function-associated antigen (LFA-3) on a constitutive manner, but only following stimulation with interferon-gamma they express MHC II and intercellular adhesion molecule (ICAM) (Tse, et al., 2003). Furthermore, MSCs do not stimulate the proliferation of peripheral blood mononuclear cells (PBMCs) showing low immunogenicity characteristics (Parys et al., 2017). Additionally, MSCs have the ability to significantly reduce the proliferation of activated T cells and interferon-gamma has a vital role in this process (Chinnadurai et al., 2014).
MSCs can migrate to the site of a lesion in a variety of illnesses, including inflammation, tissue damage, and tumors (Nitzsche et al., 2017). Several cell adhesion molecules and chemokine receptors expressed by MSCs influence their migration to the lesion site, and MSC-targeted migration to the lesion site is referred to as “homing” of MSCs (Naji et al., 2019) which is a multistep process that includes activation, adhesion, and migration (De Becker and Riet, 2016). First, inflammatory cytokines generated by inflamed or wounded tissues activate vascular cell adhesion molecule-1 (VCAM-1) on the surface of endothelial cells and α4β1 integrin (VLA-4) on the surface of MSCs, trapping MSCs on the endothelial cell surface (Uchibori et al., 2013). Following that, many growth factors generated by inflammation or damaged tissues might bind to MSC receptors and increase MSC adherence to endothelial cells (Lejmi et al., 2015). Finally, MSCs express matrix metalloproteinase 2 (MMP-2) and membrane type-1-MMP (MT-1-MMP), which activate proteasomes that breakdown the extracellular matrix and assist MSCs migrate across the basement membrane to the lesion site (Ries et al., 2007).
Mesenchymal stem cells in cancer therapy
Many mediators have been identified in the cross-talk between MSCs, the tumor microenvironment, and tumor cells. By triggering numerous signaling pathways, MSCs have different roles on the cells in the tumor microenvironment. MSCs can block Wnt signaling by regulating the Dickkopf-related protein 1 (DKK1) secreted by tumor cells, downregulating c-Myc and Cyclin D2 and upregulating the expression of P21CIP1 and P27KIP1, resulting to tumor cell suppression (Qiao et al., 2008; Zhu et al., 2009). By suppressing angiogenesis, naive MSCs can cause vascular endothelial cells to die (Otsu et al., 2009). On the contrary, MSCs have been shown to be linked to increased metastasis, tumorigenesis, and recurrence of tumors by producing cancer stem cells (CSCs) (Liu et al., 2011). MSCs also produce chemokines such as CXCR4 (Corcoran et al., 2008), CCL5 (Karnoub et al., 2007), ICAMs (Tsukamoto et al., 2012), and VCAMs (Hu et al., 2012). Breast cancer cells induce mesenchymal stem cells to secrete the chemokine CCL5, which subsequently acts in a paracrine manner on the cancer cells to promote motility, invasion, and metastasis (Karnoub et al., 2007). MSCs obtained from mouse lymphomas produce CCL2 and enhance cancer cell proliferation as well as the recruitment of immunosuppressive cells to lymphoid organs (Ren et al., 2012). MSCs originating from breast cancer tissues also produce some immunosuppressive mediators such as IL-4, TGF-β, and IL-10 (Razmkhah et al., 2011). Although the majority of studies aimed at using MSCs in cancer therapy have focused on their tumor-suppressing capabilities, these cells may potentially stimulate tumor progression by increasing metastasis, tumor angiogenesis, epithelial–mesenchymal transition, and disrupting immune surveillance (Hmadcha et al., 2020) (Figure 1). These unfavorable effects may appear depending on the number of MSCs injected, their source or origin, differentiation level, and tumor type, As a result, restrictions in MSC-based cancer therapy should be considered, and more research is needed to assess the safety and efficacy of such a therapeutic approach in the treatment of cancer.
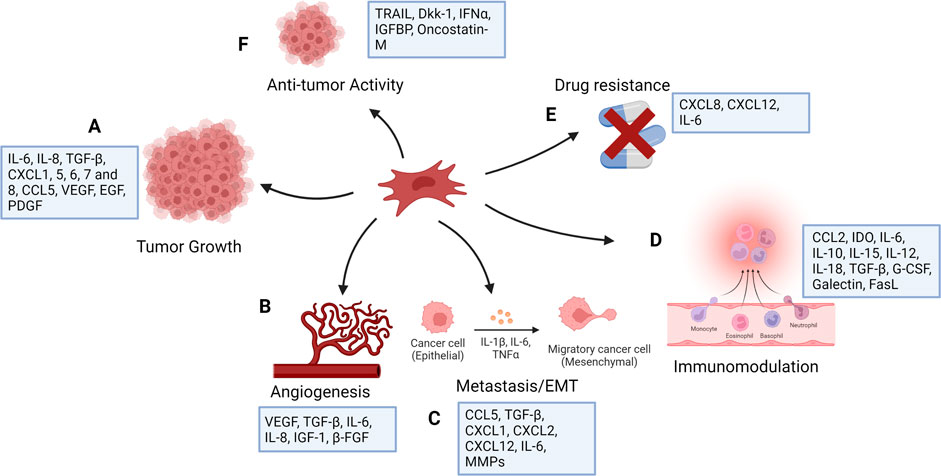
FIGURE 1. Functions of mesenchymal stem cells in cancer (created with BioRender). MSCs have number of effects on tumor cells, mostly increasing tumor growth as a result of their function in controlling inflammation and tissue repair. They affect tumor cell survival and stemness (A) and contribute to angiogenesis (B) by producing angiogenic factors. MSCs stimulate tumor cell motility, epithelial mesenchymal transition (EMT), and metastasis (C), and secrete chemokines, including CXCL1, CXCL2, and CXCL12, and cytokines, including IL-6 and several matrix metalloproteinases (MMPs), which degrade the extracellular matrix to facilitate tumor cell migration. They show immunomodulatory function (D) and can induce drug resistance (E). MSCs are generally pro-tumorigenic, however research has suggested that they may also have anti-tumor properties (F).
Exosomes as drug carriers
Exosomes are more commonly used as drug delivery vehicles because of their transport capabilities in delivering functional content to specific cells. Some natural exosomes can be used as therapeutic agents because they contain endogenous anti-tumor biomolecules. Furthermore, bioengineered exosomes with extra required payloads and targeting specificity offer more promise in cancer treatment. In contrast to other regularly used drug delivery vehicles (e.g., liposomes), bioengineered exosomes have intrinsic targeting capabilities, low immunogenicity, high modification flexibility, and biological barrier permeability (Walker et al., 2019).
Different methods are currently being employed for the purification of exosomes, such as differential ultracentrifugation, density gradient ultracentrifugation, size exclusion chromatography, etc. (Wang et al., 2021). For isolation, the International Society for Extracellular Vesicles (ISEV) has established detailed guidelines. However, none of the methods were able to accomplish absolute purification, or total separation of exosomes from other biological products. Each approach has advantages and limitations, and combining them for optimum exosome enrichment may be recommended (Thery et al., 2018). It is necessary to characterize exosomes thoroughly according to ISEV’s report for the validation of the isolation technique. Generally, Western Blot or ELISA are used for this purpose. The ISEV recommends identifying at least three positive and one negative protein markers. At least one transmembrane/lipid-bound protein (e.g., CD63, CD9, CD81) and one cytosolic protein (e.g., TSG101, ALIX) must be present as a positive protein marker. Single vesicle characterization requires imaging techniques (atomic force microscopy (AFM) and electron microscopy (EM)) and biophysical characterization (nanoparticle tracking analysis (NTA), tunable resistance pulse sensing (TRPS), dynamic light scattering (DLS), and flow cytometry (FC)) (Thery et al., 2018).
Bioengineered exosomes have greater therapeutic potential as delivery vehicles due their ability to transfer desired payloads and give better targeting specificity. To date, two key strategies for maximizing therapeutic efficacy of exosomes have been employed; (1) cargo engineering and, (2) surface engineering.
Cargo engineering
Different medicinal substances, such as drugs, proteins, and nucleic acids, can be encapsulated by exosomes. Pre-loading (before separation) and post-loading (after isolation) are the two main types of cargo loading techniques. In pre-loading, therapeutic molecules can be endogenously packed into exosomes during the biogenesis stage by modifying parental cells. This can be accomplished by manipulating the genetics of parental cells. Parental cells can overexpress therapeutic miRNAs, siRNAs, mRNAs, proteins, and peptides by transfection, which then be encapsulated into exosomes. Another method is to directly incubate drugs with parental cells, resulting in drug-containing exosomes (Herrmann et al., 2021). The post-loading occurs after exosomes are isolated. Exogenous payloads are passively or actively loaded into exosomes. After direct co-incubation, hydrophobic drugs can be mixed with the exosome lipid bilayer membrane and incorporated into the surface. The hydrophobic nature of the payloads and the concentration gradient of the molecules determine this passive loading method, which usually results in a poor loading capacity (Liang Y. et al., 2021). Different active loading strategies for hydrophilic molecules have been developed to temporarily permeabilize the hydrophobic lipid barrier, either physically or chemically, allowing the passage of the drug into exosomes. Electroporation, sonication, freeze-thaw cycles, and extrusion are examples of physical techniques that entail brief disruption of the exosome membrane by external forces (Walker et al., 2019). Electroporation is currently the most popular method, particularly for RNA encapsulation. Chemical techniques, on the other hand, use transfection reagents or permeabilizers like saponin to help payloads enter the exosomes without disrupting its lipid bilayer structure (Haney et al., 2019).
Surface engineering
Exosomes isolated from distinct cell origins have different surface molecules, indicating that they are selective for specific recipient cells. The biodistribution and tropism of exosomes can be influenced by changing their surface, particularly their protein composition. The major purpose of surface engineering is to give exosomes more targeting specificity, raising the local concentration of exosomes at desirable localizations while lowering unwanted systemic toxicity. Genetic engineering, chemical modification, and hybrid membrane engineering are the three types of surface engineering technologies (Liang W et al., 2021) (Figure 2).
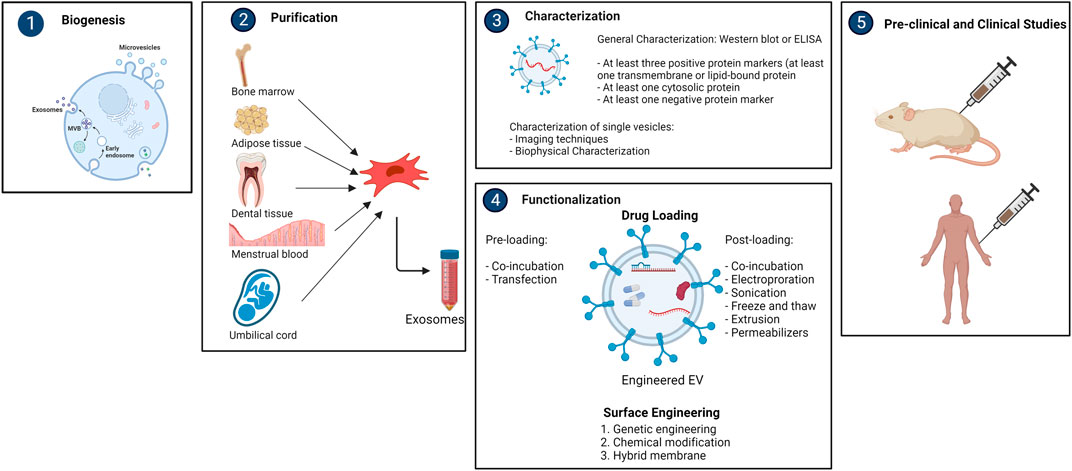
FIGURE 2. Overview of purification, characterization and functionalization of mesencyhmal stem cell-derived exosomes (created with BioRender).
Mesenchymal stem cell-derived exosomes in cancer
Exosomes can be isolated from cell cultures or body fluids. The most common cell sources are MSCs, immune cells, and cancer cells. MSCs are the most abundant producer when compared to other cell sources, and they have a large expansion capacity for economically feasible exosome production (Kim et al., 2021). Additionally, MSCs can also be isolated from a variety of human tissues without having an ethical concern (Zhou et al., 2021). Numerous in vivo and in vitro studies demonstrate the immunoregulatory, pro-angiogenic, and tissue-regeneration properties of MSC-derived exosomes. For instance, MSC-derived exosomes alleviate the severity of myocardial injury (Ma J. et al., 2017); promote tissue damage repair (Zhang B. et al., 2015); and regulate the immune system (Ti et al., 2015). Other benefits include the prevention of acute tubular injury (Bruno et al., 2009), nerve injury (Drommelschmidt et al., 2017), and lung injury (Lee et al., 2012). Preclinical data have proven the safety of exosome therapy and scalability of their isolation methods from MSCs for clinical application. However, due to the lack of established cell culture conditions, suitable protocols for production, isolation, and storage of exosomes, optimal therapeutic dose and administration schedule, and reliable potency assays to assess the efficacy of exosome therapy, the use of MSC-derived exosomes in clinical settings is limited (Börger et al., 2017).
Recent studies have shown that MSC-derived exosomes play an important role in angiogenesis, tumor development, and tumor invasion. It is still unclear whether natural MSC-derived exosomes have beneficial or detrimental effects on tumors. Several studies have reported that natural MSC-derived exosomes enhanced tumor development. However, some studies suggested that these exosomes can prevent tumor progression. According to a prior study, the dual effect may be influenced by the origin of the MSC-derived exosomes, the dose and timing of the MSC injection, the kind of malignancy, and other parameters (Shojaei et al., 2019). Zhu et al. (2012) showed that exosomes released by MSCs could stimulate tumor growth in vivo. In xenograft mouse models of stomach and colon malignancies, exosomes generated from human bone marrow mesenchymal stem cells (hBMSCs) promoted tumor growth. However, exosomes had no similar effects on tumor cells in vitro. Angiogenesis-related molecular signaling pathway activation was detected in vivo and in vitro with increased VEGF and CXCR4 mRNA levels, which corresponded to enhanced vascular density in tumor tissues in vivo. Finally, they showed that stimulation of the ERK1/2 and p38 MAPK pathways by hBMSC-derived exosomes increased VEGF and CXCR4 expression in tumor cells, resulting in increased angiogenesis and hence tumor growth in vivo (Zhu et al., 2012). In non-small cell lung cancer (NSCLC), MSC and MSC-derived exosomes promote malignancy by triggering epithelial mesenchymal transition, migration, autophagy, and also inhibiting apoptosis through the activation of the AMPK signaling pathway (Wang et al., 2022). In hepatocellular carcinoma (HCC) cells, MSC-derived exosomes increase proliferation, invasion, sphere formation ability and suppress apoptosis through TMBIM6. As a result of silencing TMBIM6, viability, sphere formation, invasion, epithelial mesenchymal transition and PI3K/AKT signaling pathway are suppressed, and apoptosis is triggered (Shang et al., 2022). Adipocyte-derived exosomes differentiated from MSC in breast cancer promote cell proliferation and migration, and also inhibit apoptosis via the Hippo signaling pathway. Suppression of the signaling pathway blocks the growth-promoting effect of adipocyte exosomes (Wang S. et al., 2019).
On the contrary, Wu et al. (2013) found that human umbilical cord Wharton’s jelly mesenchymal stem cells (hWJMSCs)-derived exosomes could induce apoptosis and cell cycle arrest in T24, a bladder cancer cell line, by increasing the expression of caspase-3 and decreasing the phosphorylation of Akt. According to a study by Kalimuthu et al. (2016) treatment with MSC-derived extracellular vesicles led lung cancer cells to undergo apoptosis.
Functions of miRNA loaded mesenchymal stem cell-derived exosomes in cancer
miRNAs are a family of short single-stranded non-coding RNAs that regulate gene expression in target cells. They range in length from 20 to 25 nucleotides (Leavitt et al., 2019). miRNAs act at the 3′UTR of mRNAs to downregulate their translation or cause their degradation as part of the RNA-induced silencing complex (RISC) (Gu et al., 2009). miRNA expression can be altered due to many reasons such as germline and somatic mutations in miRNA genes, amplification or deletion of miRNA genes, epigenetic regulation in miRNA locus, changes in miRNA biogenesis mechanisms, editing and chemical modifications of miRNAs. These dysregulations result in up- or downregulation of miRNAs and predispose to the formation of many diseases, including cancer (Urbanek-Trzeciak et al., 2020). Under specific circumstances, miRNAs can act as tumor suppressors or oncogenes. It has been demonstrated that dysregulated miRNAs have an impact on the characteristics of cancer, including maintaining proliferative signaling, avoiding growth inhibitors, resisting cell death, triggering invasion and metastasis, and promoting angiogenesis (Table 1). miRNAs have been identified as possible biomarkers for the diagnosis and prognosis of human cancers and therapeutic targets (Peng and Croce, 2016).
Two types of miRNA-based approaches can be used to change the expression levels of target genes for therapeutic purposes: (a) miRNA suppression therapy when the target gene is downregulated and (b) miRNA replacement therapy when the target gene is upregulated. Usually, the reticuloendothelial system and the ribonucleases present in the blood rapidly degrade naked RNA. The stability of oligonucleotides can be improved by chemical modifications for in vivo delivery. Antisense oligonucleotide (ASO) technology was developed for studying miRNA, and the ASOs that are used to silence miRNA are called anti-miRNA oligonucleotides (AMOs) (Zhang and Farwell, 2008).
miRNA suppression therapy can remove miRNA suppression on the target mRNA, thus increasing the mRNA expression level. AMOs bind to the miRNA sense strand, block interactions between miRISC and its target mRNA, prevent the degradation of the mRNA, and thus allow the mRNA to be translated. In miRNA replacement therapy, miRNA mimics, synthetic double-stranded miRNA-like RNA molecules, can stimulate endogenous miRNAs and bind to mRNA of the target gene, resulting in posttranscriptional suppression (Fu et al., 2019). Since cancer is related with the deregulation of multiple genes and miRNAs, it is commonly accepted that focusing on just one target is insufficient for an effective treatment. Therefore high target specificity has been replaced with multi-specificity. In that regard, miRNA-based therapies are an advantage since they affect the regulatory sequence, commonly functioning on an entire pathway or even several pathways rather than just one gene (Baumann and Winkler, 2014).
Because of their negative charge and hydrophilic nature, miRNAs are difficult to cross the cell membrane. Additionally, they are destroyed after entering the body. Therefore, exosomes can serve as excellent carriers for miRNAs (Zhang et al., 2022). There are two methods for miRNA enrichment/loading in exosomes. The first strategy involves creating a cell line that overexpresses the desired therapeutic miRNA. The cell line then displays a high level of miRNA in their cytoplasm, followed by exosome secretion containing therapeutic miRNA. The second strategy involves separating exosomes from the source (cell lines or body fluids) and then loading them with selected miRNA by using chemical or physical approaches. Since it is widely known that increasing the quantity of miRNA in the cytosol may increase their passive loading in exosomes, it is possible to transfect a designed miRNA into cells for exosomal therapy. Choosing the right cell type is one of the requirements for transfection. Although MSCs are the most commonly used “biofactories” for producing exosomes with loaded miRNA, there are some limitations in their utilization for therapeutic purposes. Initially, the cell system should be selected carefully according to the purpose of miRNA-loading. The disease being studied, the dynamics of communication between exosome-producing cells and the recipient cell, the rate of exosome secretion, and the capacity of exosomes to uptake exogenous therapeutic miRNAs should also be considered (Munir et al., 2020). Exosomes essentially have proteins on their surface, such as tetraspanins (CD-81, -82, -37, and CD-63), membrane trafficking proteins, cytoskeletal proteins, and two members of the Endosomal Sorting Complex Required for Transport (ESCRT) pathway, namely Alix and Tumor Susceptibility Gene 101 (TSG-101). The propensity of these proteins to target particular tissues is modest. Additionally, these proteins enable exosomes to accumulate in the liver, kidney, and spleen. They can be also eliminated through bile, renal filtration, and reticuloendothelial phagocytosis (Xitong and Xiaorong, 2016). Therefore, it is strongly advised to change the surface of exosomes in order to improve precise targeting and decrease the clearance rate. This can be accomplished by directly or genetically altering the exosome membrane proteins. Exosome surfaces can be directly altered using non-covalent or covalent techniques. In the non-covalent technique, exosomes and protein are combined. The covalent technique, on the other hand, involves the attachment of a peptide with covalent bonding. However, it remains to be unclear how effective these methods are for developing miRNA-enriched exosomes for targeted therapy. Both techniques have the potential for chemical contamination and have varying degrees of modification efficacy. Additionally, non-covalent attachment may dissociate under physiologic conditions (Hu et al., 2020). Genetic alteration involves producing a particular protein on the exosome surface which results in more homogenous population and sustained target specificity. It is more expensive than a direct approach. Additionally, it raises safety issues, which makes it unsuitable for clinical uses (Ohno et al., 2013).
The effect of miRNAs carried by MSC-derived exosomes in tumor treatment is contradictory, with some research claiming that they can stimulate tumor growth and others claiming that they can repress tumor growth. In osteosarcoma, miR-208a in MSC-derived exosomes increased tumor growth by downregulating programmed cell death and activating the ERK1/2 pathway (Qin et al., 2020). Furthermore, MSC-exosome-derived miR-142-3p and miR-146a have been shown to stimulate tumor growth via many mechanisms (De Veirman et al., 2016; Li and Li, 2018). Similarly, miR-146a can enhance the progression of multiple myeloma, validating this concept (De Veirman et al., 2016).
On the other hand, anti-tumor effects of miRNA carrying MSC-derived exosomes have been shown by different groups (Kang et al., 2015; Renjie and Haiqian, 2015; Gopalan et al., 2018). In prostate cancer, human bone marrow MSC-derived exosomal miR-143 has been shown to inhibit cell proliferation, invasion, metastasis, and tumor growth (Che et al., 2019). miR-23b in MSC-derived exosomes can prevent tumor development, keep tumors dormant, improve patient’s life quality, and lengthen survival time (Ono et al., 2014). In hepatocellular carcinoma, MSC-derived exosomes transfected with miR-122 can improve drug sensitivity (Lou et al., 2015). miR-34c in MSC has been proven to improve tumor sensitivity to radiotherapy in addition to enhancing chemical sensitivity (Wan et al., 2020). This shows that MSC-exosomes can be used in combination with conventional cancer treatments such as chemotherapy and radiotherapy.
miRNAs in MSC-derived exosomes have received a great deal of interest recently, and they are being studied largely for tumor inhibition. These studies differ from each other in terms of the cancer type of interest, selected MSC subtype, the way of miRNAs is transferred to MSCs, preferred miRNA and target genes according to the cancer type. The general approach in studies is to first detect and confirm miRNA and target genes that negatively regulate each other in bioinformatic studies or healthy/patient samples, and then detect alteration in the proliferation, apoptosis, migration and invasion capacities of cancer cells after administration in vitro and in vivo. The general conclusion reached is that miRNA transfer with MSC-derived exosomes has positive effects. However, it is emphasized that such studies are at a preclinical stage, the data on the mechanism of action are still insufficient, and therefore studies should continue in order to reveal the mechanisms. We summarized the studies on the use of MSC-derived exosomes as vehicles for the delivery and restoration of miRNAs in Table 2, with the goal of developing an effective therapeutic strategy for various malignancies.
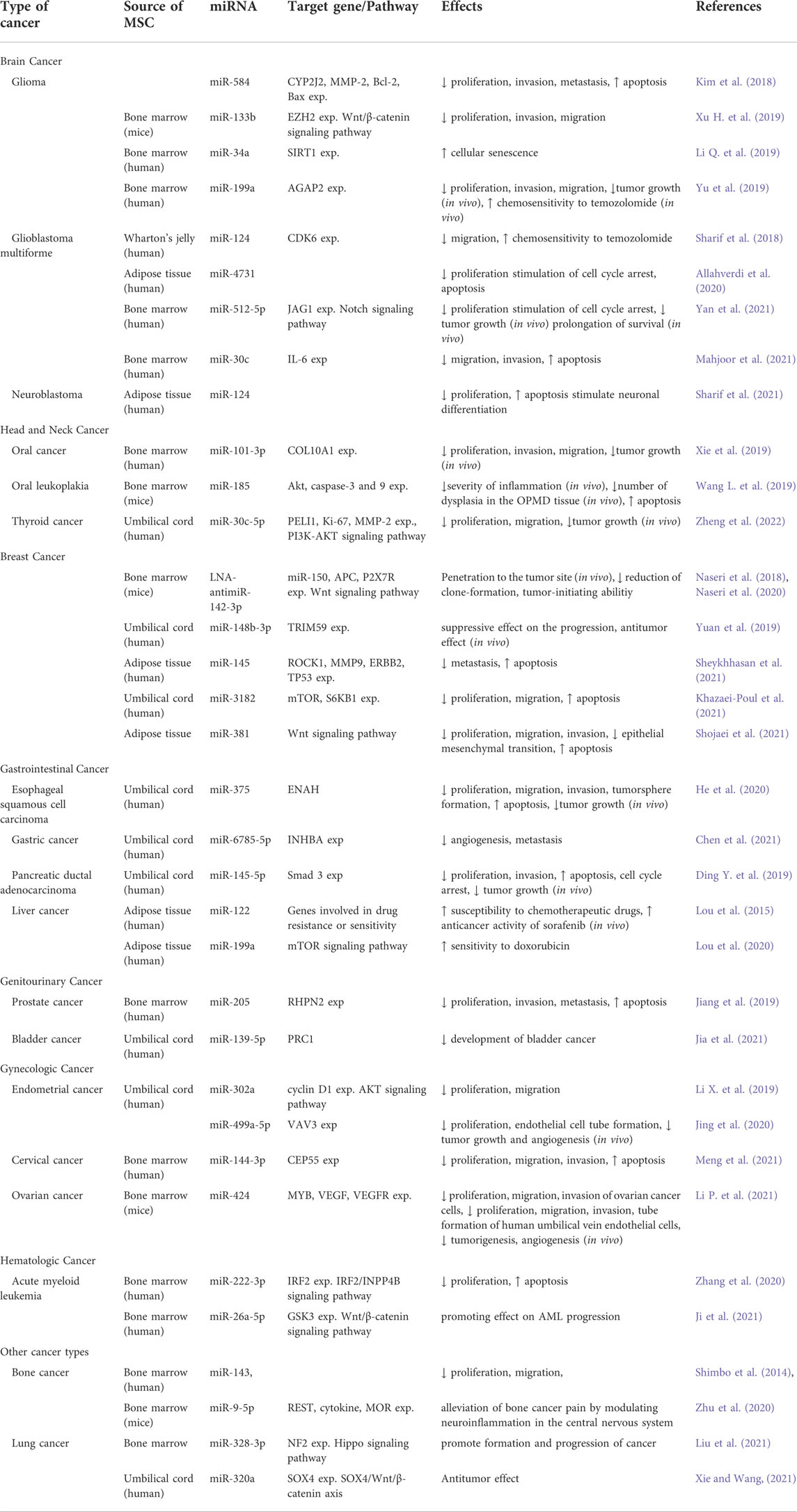
TABLE 2. Effects of miRNAs delivered by mesencymal stem cell-derived exosomes in different cancer types.
Conclusion
While significant progress has been made in the fight against cancer, it remains a leading cause of mortality in the twenty-first century, necessitating a greater understanding of the biology of cancer cells and their environment in order to create novel therapeutic options. Over the last three decades, researchers and clinicians have mostly concentrated on identifying cancer-specific targets and developing targeted medicines that can effectively destroy cancer cells while sparing their normal counterparts, decreasing undesired side effects. A variety of intriguing and very effective small compounds targeting cancer-specific mutations and/or altered signal transduction pathways that control cancer cell proliferation and survival have been developed as a result of this global endeavor.
MSC-derived exosomes have been identified as significant mediators in the therapeutic benefits of MSCs. MSC-derived exosomes can promote or inhibit tumor growth, but engineered MSC-derived exosomes are implicated in the suppression of cancer formation and progression by the delivery of numerous therapeutic compounds, including miRNAs. Dysregulation of miRNAs is thought to be involved in the initiation and progression of tumors. Furthermore, promising results show that restoring these regulatory miRNAs can be used as a therapeutic method in cancer treatment. Replacement of these molecules can contribute to the inhibition of cell proliferation, invasion, migration, and metastasis, along with increased sensitivity to chemotherapeutic drugs and activation of apoptosis through direct control of their target genes.
To summarize, recent findings have confirmed the capacity of MSC-derived exosomes to transport therapeutic miRNAs in a variety of malignancies, indicating that this approach is novel and extremely promising in the treatment of cancer. Despite the fact that MSCs have been shown to have anticancer properties, there have also been some conflicting claims about their roles in tumor growth. Hence, their potential in tumor progression should also be considered.
Author contributions
OD contributed to conception and design of the study. AD organized the literature. Both authors contributed to manuscript writing, revision, read, and approved the submitted version.
Conflict of interest
The authors declare that the research was conducted in the absence of any commercial or financial relationships that could be construed as a potential conflict of interest.
Publisher’s note
All claims expressed in this article are solely those of the authors and do not necessarily represent those of their affiliated organizations, or those of the publisher, the editors and the reviewers. Any product that may be evaluated in this article, or claim that may be made by its manufacturer, is not guaranteed or endorsed by the publisher.
References
Allahverdi, A., Arefian, E., Soleimani, M., Ai, J., Nahanmoghaddam, N., Yousefi-Ahmadipour, A., et al. (2020). MicroRNA-4731-5p delivered by AD-mesenchymal stem cells induces cell cycle arrest and apoptosis in glioblastoma. J. Cell. Physiol. 235 (11), 8167–8175. doi:10.1002/jcp.29472
Alvarez, C. V., Garcia-Lavandeira, M., Garcia-Rendueles, M. E., Diaz-Rodriguez, E., Garcia-Rendueles, A. R., Perez-Romero, S., et al. (2012). Defining stem cell types: Understanding the therapeutic potential of ESCs, ASCs, and iPS cells. J. Mol. Endocrinol. 49 (2), R89–R111. doi:10.1530/JME-12-0072
Amir, S., Ma, A. H., Shi, X. B., Xue, L., Kung, H. J., and Devere White, R. W. (2013). Oncomir miR-125b suppresses p14(ARF) to modulate p53-dependent and p53-independent apoptosis in prostate cancer. PloS One 8 (4), e61064. doi:10.1371/journal.pone.0061064
Ayala-Ortega, E., Arzate-Mejía, R., Pérez-Molina, R., González-Buendía, E., Meier, K., Guerrero, G., et al. (2016). Epigenetic silencing of miR-181c by DNA methylation in glioblastoma cell lines. BMC Cancer 16, 226. doi:10.1186/s12885-016-2273-6
Baumann, V., and Winkler, J. (2014). miRNA-based therapies: Strategies and delivery platforms for oligonucleotide and non-oligonucleotide agents. Future Med. Chem. 6 (17), 1967–1984. doi:10.4155/fmc.14.116
Bellagamba, B. C., Abreu, B. R., Grivicich, I., Markarian, C. F., Chem, E., Camassola, M., et al. (2016). Human mesenchymal stem cells are resistant to cytotoxic and genotoxic effects of cisplatin in vitro. Genet. Mol. Biol. 39 (1), 129–134. doi:10.1590/1678-4685-GMB-2015-0057
Börger, V., Bremer, M., Ferrer-Tur, R., Gockeln, L., Stambouli, O., Becic, A., et al. (2017). Mesenchymal stem/stromal cell-derived extracellular vesicles and their potential as novel immunomodulatory therapeutic agents. Int. J. Mol. Sci. 18 (7), 1450. doi:10.3390/ijms18071450
Bruno, S., Grange, C., Deregibus, M. C., Calogero, R. A., Saviozzi, S., Collino, F., et al. (2009). Mesenchymal stem cell-derived microvesicles protect against acute tubular injury. J. Am. Soc. Nephrol. 20 (5), 1053–1067. doi:10.1681/asn.2008070798
Che, Y., Shi, X., Shi, Y., Jiang, X., Ai, Q., Shi, Y., et al. (2019). Exosomes derived from miR-143-overexpressing MSCs inhibit cell migration and invasion in human prostate cancer by downregulating TFF3. Mol. Ther. - Nucleic Acids 18, 232–244. doi:10.1016/j.omtn.2019.08.010
Chen, J., Ouyang, H., An, X., and Liu, S. (2019). miR-125a is upregulated in cancer stem-like cells derived from TW01 and is responsible for maintaining stemness by inhibiting p53. Oncol. Lett. 17 (1), 87–94. doi:10.3892/ol.2018.9587
Chen, L., Jia, J., Zang, Y., Li, J., and Wan, B. (2019). MicroRNA-101 regulates autophagy, proliferation and apoptosis via targeting EZH2 in laryngeal squamous cell carcinoma. Neoplasma 66 (4), 507–515. doi:10.4149/neo_2018_180811N611
Chen, Q., Zeng, X., Huang, D., and Qiu, X. (2018). Identification of differentially expressed miRNAs in early-stage cervical cancer with lymph node metastasis across the cancer genome atlas datasets. Cancer Manag. Res. 10, 6489–6504. doi:10.2147/CMAR.S183488
Chen Y. N, Y. N., Ren, C. C., Yang, L., Nai, M. M., Xu, Y. M., Zhang, F., et al. (2019). MicroRNA let-7d-5p rescues ovarian cancer cell apoptosis and restores chemosensitivity by regulating the p53 signaling pathway via HMGA1. Int. J. Oncol. 54 (5), 1771–1784. doi:10.3892/ijo.2019.4731
Chen, Z., Xie, Y., Chen, W., Li, T., Chen, X., and Liu, B. (2021). microRNA-6785-5p-Loaded human umbilical cord mesenchymal stem cells-derived exosomes suppress angiogenesis and metastasis in gastric cancer via INHBA. Life Sci. 284, 119222. doi:10.1016/j.lfs.2021.119222
Cheng, Y., Geng, L., Zhao, L., Zuo, P., and Wang, J. (2017). Human papillomavirus E6-regulated microRNA-20b promotes invasion in cervical cancer by targeting tissue inhibitor of metalloproteinase 2. Mol. Med. Rep. 16 (4), 5464–5470. doi:10.3892/mmr.2017.7231
Chiang, C. H., Chu, P. Y., Hou, M. F., and Hung, W. C. (2016). MiR-182 promotes proliferation and invasion and elevates the HIF-1α-VEGF-A Axis in breast cancer cells by targeting FBXW7. Am. J. Cancer Res. 6 (8), 1785–1798.
Chinnadurai, R., Copland, I. B., Patel, S. R., and Galipeau, J. (2014). Ido-independent suppression of T cell effector function by IFN-γ-Licensed human mesenchymal stromal cells. J. I. 192 (4), 1491–1501. doi:10.4049/jimmunol.1301828
Chou, J., Wang, B., Zheng, T., Li, X., Zheng, L., Hu, J., et al. (2016). MALAT1 induced migration and invasion of human breast cancer cells by competitively binding miR-1 with cdc42. Biochem. Biophysical Res. Commun. 472 (1), 262–269. doi:10.1016/j.bbrc.2016.02.102
Consentius, C., Akyüz, L., Schmidt-Lucke, J. A., Tschöpe, C., Pinzur, L., Ofir, R., et al. (2015). Mesenchymal stromal cells prevent allostimulation in vivo and control checkpoints of Th1 priming: Migration of human DC to lymph nodes and NK cell activation. Stem Cells 33 (10), 3087–3099. doi:10.1002/stem.2104
Corcoran, K. E., Trzaska, K. A., Fernandes, H., Bryan, M., Taborga, M., Srinivas, V., et al. (2008). Mesenchymal stem cells in early entry of breast cancer into bone marrow. PloS One 3 (6), e2563. doi:10.1371/journal.pone.0002563
da Silva Meirelles, L., Chagastelles, P. C., and Nardi, N. B. (2006). Mesenchymal stem cells reside in virtually all post-natal organs and tissues. J. Cell Sci. 119 (11), 2204–2213. doi:10.1242/jcs.02932
Das, S. G., Romagnoli, M., Mineva, N. D., Barillé-Nion, S., Jézéquel, P., Campone, M., et al. (2016). miR-720 is a downstream target of an ADAM8-induced ERK signaling cascade that promotes the migratory and invasive phenotype of triple-negative breast cancer cells. Breast Cancer Res. 18 (1), 40. doi:10.1186/s13058-016-0699-z
De Becker, A., and Riet, I. V. (2016). Homing and migration of mesenchymal stromal cells: How to improve the efficacy of cell therapy? World J. Stem Cells 8 (3), 73–87. doi:10.4252/wjsc.v8.i3.73
De Veirman, K., Wang, J., Xu, S., Leleu, X., Himpe, E., Maes, K., et al. (2016). Induction of miR-146a by multiple myeloma cells in mesenchymal stromal cells stimulates their pro-tumoral activity. Cancer Lett. 377 (1), 17–24. doi:10.1016/j.canlet.2016.04.024
Ding, C., Yu, H., Shi, C., Shi, T., Qin, H., and Cui, Y. (2019). MiR-let-7e inhibits invasion and magration and regulates HMGB1 expression in papillary thyroid carcinoma. Biomed. Pharmacother. 110, 528–536. doi:10.1016/j.biopha.2018.11.057
Ding, Y., Cao, F., Sun, H., Wang, Y., Liu, S., Wu, Y., et al. (2019). Exosomes derived from human umbilical cord mesenchymal stromal cells deliver exogenous miR-145-5p to inhibit pancreatic ductal adenocarcinoma progression. Cancer Lett. 442, 351–361. doi:10.1016/j.canlet.2018.10.039
Dominici, M., Le Blanc, K., Mueller, I., Slaper-Cortenbach, I., Marini, F., Krause, D., et al. (2006). Minimal criteria for defining multipotent mesenchymal stromal cells. The international society for cellular therapy position statement. Cytotherapy 8 (4), 315–317. doi:10.1080/14653240600855905
Dou, L., Li, J., Zheng, D., Li, Y., Gao, X., Xu, C., et al. (2013). MicroRNA-142-3p inhibits cell proliferation in human acute lymphoblastic leukemia by targeting the MLL-AF4 oncogene. Mol. Biol. Rep. 40 (12), 6811–6819. doi:10.1007/s11033-013-2798-6
Doyle, L. M., and Wang, M. Z. (2019). Overview of extracellular vesicles, their origin, composition, purpose, and methods for exosome isolation and analysis. Cells 8 (7), 727. doi:10.3390/cells8070727
Drommelschmidt, K., Serdar, M., Bendix, I., Herz, J., Bertling, F., Prager, S., et al. (2017). Mesenchymal stem cell-derived extracellular vesicles ameliorate inflammation-induced preterm brain injury. Brain Behav. Immun. 60, 220–232. doi:10.1016/j.bbi.2016.11.011
Fahim, S. A., Abdullah, M. S., Espinoza-Sánchez, N. A., Hassan, H., Ibrahim, A. M., Ahmed, S. H., et al. (2020). Inflammatory breast carcinoma: Elevated microRNA miR-181b-5p and reduced miR-200b-3p, miR-200c-3p, and miR-203a-3p expression as potential biomarkers with diagnostic value. Biomolecules 10 (7), 1059. doi:10.3390/biom10071059
Farini, A., Sitzia, C., Erratico, S., Meregalli, M., and Torrente, Y. (2014). Clinical applications of mesenchymal stem cells in chronic diseases. Stem Cells Int. 2014, 1–11. doi:10.1155/2014/306573
Foekens, J. A., Sieuwerts, A. M., Smid, M., Look, M. P., de Weerd, V., Boersma, A. W., et al. (2008). Four miRNAs associated with aggressiveness of lymph node-negative, estrogen receptor-positive human breast cancer. Proc. Natl. Acad. Sci. U. S. A. 105 (35), 13021–13026. doi:10.1073/pnas.0803304105
Friedenstein, A. J., Piatetzky-Shapiro, I. I., and Petrakova, K. V. (1966). Osteogenesis in transplants of bone marrow cells. Development 16 (3), 381–390. doi:10.1242/dev.16.3.381
Fu, Y., Chen, J., and Huang, Z. (2019). Recent progress in microRNA-based delivery systems for the treatment of human disease. ExRNA 1, 24. doi:10.1186/s41544-019-0024-y
Galland, S., Vuille, J., Martin, P., Letovanec, I., Caignard, A., Fregni, G., et al. (2017). Tumor-derived mesenchymal stem cells use distinct mechanisms to block the activity of natural killer cell subsets. Cell Rep. 20 (12), 2891–2905. doi:10.1016/j.celrep.2017.08.089
Gao, S., Zhao, Z., Wu, R., Wu, L., Tian, X., and Zhang, Z. (2019). MiR-1 inhibits prostate cancer PC3 cells proliferation through the akt/mTOR signaling pathway by binding to c-met. Biomed. Pharmacother. 109, 1406–1410. doi:10.1016/j.biopha.2018.10.098
Gao, X., Zhu, X., Sun, Y., and Liu, J. (2017). MicroRNA-141 inhibits the self-renewal of glioblastoma stem cells via Jagged1. Mol. Med. Rep. 16 (1), 167–173. doi:10.3892/mmr.2017.6598
Gopalan, V., Ebrahimi, F., Islam, F., Vider, J., Qallandar, O. B., Pillai, S., et al. (2018). Tumour suppressor properties of miR-15a and its regulatory effects on BCL2 and SOX2 proteins in colorectal carcinomas. Exp. Cell Res. 370 (2), 245–253. doi:10.1016/j.yexcr.2018.06.025
Gu, S., Jin, L., Zhang, F., Sarnow, P., and Kay, M. A. (2009). Biological basis for restriction of microRNA targets to the 3' untranslated region in mammalian mRNAs. Nat. Struct. Mol. Biol. 16 (2), 144–150. doi:10.1038/nsmb.1552
Haney, M. J., Klyachko, N. L., Harrison, E. B., Zhao, Y., Kabanov, A. V., and Batrakova, E. V. (2019). TPP1 delivery to lysosomes with extracellular vesicles and their enhanced brain distribution in the animal model of batten disease. Adv. Healthc. Mat. 8 (11), e1801271. doi:10.1002/adhm.201801271
He, Z., Li, W., Zheng, T., Liu, D., and Zhao, S. (2020). Human umbilical cord mesenchymal stem cells-derived exosomes deliver microRNA-375 to downregulate ENAH and thus retard esophageal squamous cell carcinoma progression. J. Exp. Clin. Cancer Res. 39 (1), 140. doi:10.1186/s13046-020-01631-w
Herrmann, I. K., Wood, M., and Fuhrmann, G. (2021). Extracellular vesicles as a next-generation drug delivery platform. Nat. Nanotechnol. 16 (7), 748–759. doi:10.1038/s41565-021-00931-2
Hmadcha, A., Martin-Montalvo, A., Gauthier, B. R., Soria, B., and Capilla-Gonzalez, V. (2020). Therapeutic potential of mesenchymal stem cells for cancer therapy. Front. Bioeng. Biotechnol. 8, 43. doi:10.3389/fbioe.2020.00043
Hu, Q., Su, H., Li, J., Lyon, C., Tang, W., Wan, M., et al. (2020). Clinical applications of exosome membrane proteins. Precis. Clin. Med. 3 (1), 54–66. doi:10.1093/pcmedi/pbaa007
Hu, T., Chong, Y., Lu, S., Wang, R., Qin, H., Silva, J., et al. (2018). miR-339 promotes development of stem cell leukemia/lymphoma syndrome via downregulation of the BCL2L11 and BAX proapoptotic genes. Cancer Res. 78 (13), 3522–3531. doi:10.1158/0008-5472.CAN-17-4049
Hu, Y., Cheng, P., Xue, Y. X., and Liu, Y. H. (2012). Glioma cells promote the expression of vascular cell adhesion molecule-1 on bone marrow-derived mesenchymal stem cells: A possible mechanism for their tropism toward gliomas. J. Mol. Neurosci. 48 (1), 127–135. doi:10.1007/s12031-012-9784-7
Huang, Y., Li, J., Chen, Y., Jiang, P., Wang, L., and Hu, J. (2020). Identification of early recurrence factors in childhood and adolescent B-cell acute lymphoblastic leukemia based on integrated bioinformatics analysis. Front. Oncol. 10, 565455. doi:10.3389/fonc.2020.565455
Hunter, S., Nault, B., Ugwuagbo, K. C., Maiti, S., and Majumder, M. (2019). Mir526b and Mir655 promote tumour associated angiogenesis and lymphangiogenesis in breast cancer. Cancers 11 (7), 938. doi:10.3390/cancers11070938
Huo, L., Wang, Y., Gong, Y., Krishnamurthy, S., Wang, J., Diao, L., et al. (2016). MicroRNA expression profiling identifies decreased expression of miR-205 in inflammatory breast cancer. Mod. Pathol. 29 (4), 330–346. doi:10.1038/modpathol.2016.38
Ji, D., He, Y., Lu, W., Rong, Y., Li, F., Huang, X., et al. (2021). Small-sized extracellular vesicles (EVs) derived from acute myeloid leukemia bone marrow mesenchymal stem cells transfer miR-26a-5p to promote acute myeloid leukemia cell proliferation, migration, and invasion. Hum. Cell 34 (3), 965–976. doi:10.1007/s13577-021-00501-7
Jia, Y., Ding, X., Zhou, L., Zhang, L., and Yang, X. (2021). Mesenchymal stem cells-derived exosomal microRNA-139-5p restrains tumorigenesis in bladder cancer by targeting PRC1. Oncogene 40 (2), 246–261. doi:10.1038/s41388-020-01486-7
Jiang, S., Mo, C., Guo, S., Zhuang, J., Huang, B., and Mao, X. (2019). Human bone marrow mesenchymal stem cells-derived microRNA-205-containing exosomes impede the progression of prostate cancer through suppression of RHPN2. J. Exp. Clin. Cancer Res. 38 (1), 495. doi:10.1186/s13046-019-1488-1
Jin, C., Yan, B., Lu, Q., Lin, Y., and Ma, L. (2016). Reciprocal regulation of hsa-miR-1 and long noncoding RNA MALAT1 promotes triple-negative breast cancer development. Tumor Biol. 37 (6), 7383–7394. doi:10.1007/s13277-015-4605-6
Jing, L., Hua, X., Yuanna, D., Rukun, Z., and Junjun, M. (2020). Exosomal miR-499a-5p inhibits endometrial cancer growth and metastasis via targeting VAV3. Cancer Manag. Res. 12, 13541–13552. doi:10.2147/CMAR.S283747
Jung, Y. J., Ju, S. Y., Yoo, E. S., Cho, S., Cho, K. A., Woo, S. Y., et al. (2007). MSC-DC interactions: MSC inhibit maturation and migration of BM-derived DC. Cytotherapy 9 (5), 451–458. doi:10.1080/14653240701452057
Kalimuthu, S., Gangadaran, P., Li, X. J., Oh, J. M., Lee, H. W., Jeong, S. Y., et al. (2016). In vivo therapeutic potential of mesenchymal stem cell-derived extracellular vesicles with optical imaging reporter in tumor mice model. Sci. Rep. 6, 30418. doi:10.1038/srep30418
Kang, W., Tong, J. H., Lung, R. W., Dong, Y., Zhao, J., Liang, Q., et al. (2015). Targeting of YAP1 by microRNA-15a and microRNA-16-1 exerts tumor suppressor function in gastric adenocarcinoma. Mol. Cancer 14, 52. doi:10.1186/s12943-015-0323-3
Karnoub, A. E., Dash, A. B., Vo, A. P., Sullivan, A., Brooks, M. W., Bell, G. W., et al. (2007). Mesenchymal stem cells within tumour stroma promote breast cancer metastasis. Nature 449 (7162), 557–563. doi:10.1038/nature06188
Khazaei-Poul, Y., Shojaei, S., Koochaki, A., Ghanbarian, H., and Mohammadi-Yeganeh, S. (2021). Evaluating the influence of human umbilical cord mesenchymal stem cells-derived exosomes loaded with miR-3182 on metastatic performance of triple negative breast cancer cells. Life Sci. 286, 120015. doi:10.1016/j.lfs.2021.120015
Kim, J. Y., Rhim, W. K., Yoo, Y. I., Kim, D. S., Ko, K. W., Heo, Y., et al. (2021). Defined MSC exosome with high yield and purity to improve regenerative activity. J. Tissue Eng. 12, 204173142110086. doi:10.1177/20417314211008626
Kim, R., Lee, S., Lee, J., Kim, M., Kim, W. J., Lee, H. W., et al. (2018). Exosomes derived from microRNA-584 transfected mesenchymal stem cells: Novel alternative therapeutic vehicles for cancer therapy. BMB Rep. 51 (8), 406–411. doi:10.5483/bmbrep.2018.51.8.105
Kong, W., He, L., Richards, E. J., Challa, S., Xu, C. X., Permuth-Wey, J., et al. (2014). Upregulation of miRNA-155 promotes tumour angiogenesis by targeting VHL and is associated with poor prognosis and triple-negative breast cancer. Oncogene 33 (6), 679–689. doi:10.1038/onc.2012.636
Labib, H. A., Elantouny, N. G., Ibrahim, N. F., and Alnagar, A. A. (2017). Upregulation of microRNA-21 is a poor prognostic marker in patients with childhood B cell acute lymphoblastic leukemia. Hematology 22 (7), 392–397. doi:10.1080/10245332.2017.1292204
Le Blanc, K., and Davies, L. C. (2015). Mesenchymal stromal cells and the innate immune response. Immunol. Lett. 168 (2), 140–146. doi:10.1016/j.imlet.2015.05.004
Leavitt, R. J., Limoli, C. L., and Baulch, J. E. (2019). miRNA-based therapeutic potential of stem cell-derived extracellular vesicles: A safe cell-free treatment to ameliorate radiation-induced brain injury. Int. J. Radiat. Biol. 95 (4), 427–435. doi:10.1080/09553002.2018.1522012
Lee, C., Mitsialis, S. A., Aslam, M., Vitali, S. H., Vergadi, E., Konstantinou, G., et al. (2012). Exosomes mediate the cytoprotective action of mesenchymal stromal cells on hypoxia-induced pulmonary hypertension. Circulation 126 (22), 2601–2611. doi:10.1161/circulationaha.112.114173
Lei, K., Du, W., Lin, S., Yang, L., Xu, Y., Gao, Y., et al. (2016). 3B, a novel photosensitizer, inhibits glycolysis and inflammation via miR-155-5p and breaks the JAK/STAT3/SOCS1 feedback loop in human breast cancer cells. Biomed. Pharmacother. 82, 141–150. doi:10.1016/j.biopha.2016.04.049
Lejmi, E., Perriraz, N., Clément, S., Morel, P., Baertschiger, R., Christofilopoulos, P., et al. (2015). Inflammatory chemokines MIP-1δ and MIP-3α are involved in the migration of multipotent mesenchymal stromal cells induced by hepatoma cells. Stem Cells Dev. 24 (10), 1223–1235. doi:10.1089/scd.2014.0176
Li, H., and Li, F. (2018). Exosomes from BM-MSCs increase the population of CSCs via transfer of miR-142-3p. Br. J. Cancer 119 (6), 744–755. doi:10.1038/s41416-018-0254-z
Li, H., Yu, L., Liu, J., Bian, X., Shi, C., Sun, C., et al. (2017). miR-320a functions as a suppressor for gliomas by targeting SND1 and β-catenin, and predicts the prognosis of patients. Oncotarget 8 (12), 19723–19737. doi:10.18632/oncotarget.14975
Li, M., Tian, L., Ren, H., Chen, X., Wang, Y., Ge, J., et al. (2015). MicroRNA-101 is a potential prognostic indicator of laryngeal squamous cell carcinoma and modulates CDK8. J. Transl. Med. 13, 271. doi:10.1186/s12967-015-0626-6
Li, P., Xin, H., and Lu, L. (2021). Extracellular vesicle-encapsulated microRNA-424 exerts inhibitory function in ovarian cancer by targeting MYB. J. Transl. Med. 19 (1), 4. doi:10.1186/s12967-020-02652-x
Li, Q., Wang, C., Cai, L., Lu, J., Zhu, Z., Wang, C., et al. (2019). miR-34a derived from mesenchymal stem cells stimulates senescence in glioma cells by inducing DNA damage. Mol. Med. Rep. 19 (3), 1849–1857. doi:10.3892/mmr.2018.9800
Li, X., Liu, L. L., Yao, J. L., Wang, K., and Ai, H. (2019). Human umbilical cord mesenchymal stem cell-derived extracellular vesicles inhibit endometrial cancer cell proliferation and migration through delivery of exogenous miR-302a. Stem Cells Int. 2019, 1–11. doi:10.1155/2019/8108576
Li, Z., Fan, H., Chen, W., Xiao, J., Ma, X., Ni, P., et al. (2021). MicroRNA-653-5p promotes gastric cancer proliferation and metastasis by targeting the SOCS6-STAT3 pathway. Front. Mol. Biosci. 8, 655580. doi:10.3389/fmolb.2021.655580
Liang, W., Chen, X., Zhang, S., Fang, J., Chen, M., Xu, Y., et al. (2021). Mesenchymal stem cells as a double-edged sword in tumor growth: Focusing on MSC-derived cytokines. Cell. Mol. Biol. Lett. 26, 3. doi:10.1186/s11658-020-00246-5
Liang, Y., Duan, L., Lu, J., and Xia, J. (2021). Engineering exosomes for targeted drug delivery. Theranostics 11 (7), 3183–3195. doi:10.7150/thno.52570
Liao, W., and Zhang, Y. (2020). MicroRNA-381 facilitates autophagy and apoptosis in prostate cancer cells via inhibiting the RELN-mediated PI3K/AKT/mTOR signaling pathway. Life Sci. 254, 117672. doi:10.1016/j.lfs.2020.117672
Lin, J., Liu, Z., Liao, S., Li, E., Wu, X., and Zeng, W. (2020). Elevated microRNA-7 inhibits proliferation and tumor angiogenesis and promotes apoptosis of gastric cancer cells via repression of raf-1. Cell Cycle 19 (19), 2496–2508. doi:10.1080/15384101.2020.1807670
Lin, Z., Chen, Y., Lin, Y., Lin, H., Li, H., Su, X., et al. (2020). Potential miRNA biomarkers for the diagnosis and prognosis of esophageal cancer detected by a novel absolute quantitative RT-qPCR method. Sci. Rep. 10 (1). doi:10.1038/s41598-020-77119-6
Liu, C. J., Shen, W. G., Peng, S. Y., Cheng, H. W., Kao, S. Y., Lin, S. C., et al. (2014). miR-134 induces oncogenicity and metastasis in head and neck carcinoma through targeting WWOX gene. Int. J. Cancer 134 (4), 811–821. doi:10.1002/ijc.28358
Liu, F., Yin, R., Chen, X., Chen, W., Qian, Y., Zhao, Y., et al. (2019). Over-expression of miR-206 decreases the euthyrox-resistance by targeting MAP4K3 in papillary thyroid carcinoma. Biomed. Pharmacother. 114, 108605. doi:10.1016/j.biopha.2019.108605
Liu, J., Shen, J. X., He, D., and Zhang, G. J. (2018). Bioluminescence imaging for monitoring miR-200c expression in breast cancer cells and its effects on epithelial-mesenchymal transition progress in living animals. Mol. Imaging Biol. 20 (5), 761–770. doi:10.1007/s11307-018-1180-4
Liu, J., Xu, J., Li, H., Sun, C., Yu, L., Li, Y., et al. (2015). miR-146b-5p functions as a tumor suppressor by targeting TRAF6 and predicts the prognosis of human gliomas. Oncotarget 6 (30), 29129–29142. doi:10.18632/oncotarget.4895
Liu, N., Zhang, L., Wang, Z., Cheng, Y., Zhang, P., Wang, X., et al. (2017). MicroRNA-101 inhibits proliferation, migration and invasion of human glioblastoma by targeting SOX9. Oncotarget 8 (12), 19244–19254. doi:10.18632/oncotarget.13706
Liu, R., Liu, C., Zhang, D., Liu, B., Chen, X., Rycaj, K., et al. (2016). miR-199a-3p targets stemness-related and mitogenic signaling pathways to suppress the expansion and tumorigenic capabilities of prostate cancer stem cells. Oncotarget 7 (35), 56628–56642. doi:10.18632/oncotarget.10652
Liu, S., Ginestier, C., Ou, S. J., Clouthier, S. G., Patel, S. H., Monville, F., et al. (2011). Breast cancer stem cells are regulated by mesenchymal stem cells through cytokine networks. Cancer Res. 71 (2), 614–624. doi:10.1158/0008-5472.CAN-10-0538
Liu, X., Jiang, F., Wang, Z., Tang, L., Zou, B., Xu, P., et al. (2021). Hypoxic bone marrow mesenchymal cell-extracellular vesicles containing miR-328-3p promote lung cancer progression via the NF2-mediated Hippo Axis. J. Cell. Mol. Med. 25 (1), 96–109. doi:10.1111/jcmm.15865
Liu, Z., Jiang, Z., Huang, J., Huang, S., Li, Y., Yu, S., et al. (2014). miR-7 inhibits glioblastoma growth by simultaneously interfering with the PI3K/ATK and raf/MEK/ERK pathways. Int. J. Oncol. 44 (5), 1571–1580. doi:10.3892/ijo.2014.2322
Lou, G., Chen, L., Xia, C., Wang, W., Qi, J., Li, A., et al. (2020). MiR-199a-Modified exosomes from adipose tissue-derived mesenchymal stem cells improve hepatocellular carcinoma chemosensitivity through mTOR pathway. J. Exp. Clin. Cancer Res. 39 (1), 4. doi:10.1186/s13046-019-1512-5
Lou, G., Song, X., Yang, F., Wu, S., Wang, J., Chen, Z., et al. (2015). Exosomes derived from miR-122-modified adipose tissue-derived MSCs increase chemosensitivity of hepatocellular carcinoma. J. Hematol. Oncol. 8, 122. doi:10.1186/s13045-015-0220-7
Lou, Y., Liu, L., Zhan, L., Wang, X., and Fan, H. (2016). miR-187-5p regulates cell growth and apoptosis in acute lymphoblastic leukemia via DKK2. Oncol. Res. 24 (2), 89–97. doi:10.3727/096504016X14597766487753
Lu, Q., Chen, Y., Sun, D., Wang, S., Ding, K., Liu, M., et al. (2019). MicroRNA-181a functions as an oncogene in gastric cancer by targeting caprin-1. Front. Pharmacol. 9, 1565. doi:10.3389/fphar.2018.01565
Luo A, A., Zhou, X., Shi, X., Zhao, Y., Men, Y., Chang, X., et al. (2019). Exosome-derived miR-339-5p mediates radiosensitivity by targeting Cdc25A in locally advanced esophageal squamous cell carcinoma. Oncogene 38 (25), 4990–5006. doi:10.1038/s41388-019-0771-0
Luo, D., Fan, H., Ma, X., Yang, C., He, Y., Ge, Y., et al. (2021). miR-1301-3p promotes cell proliferation and facilitates cell cycle progression via targeting SIRT1 in gastric cancer. Front. Oncol. 11, 664242. doi:10.3389/fonc.2021.664242
Luo, M., Sun, G., and Sun, J. W. (2019). MiR-196b affects the progression and prognosis of human LSCC through targeting PCDH-17. Auris, Nasus, Larynx 46 (4), 583–592. doi:10.1016/j.anl.2018.10.020
Ma, C., Wei, F., Xia, H., Liu, H., Dong, X., Zhang, Y., et al. (2017). MicroRNA-10b mediates TGF-β1-regulated glioblastoma proliferation, migration and epithelial-mesenchymal transition. Int. J. Oncol. 50 (5), 1739–1748. doi:10.3892/ijo.2017.3947
Ma, J., Zhao, Y., Sun, L., Sun, X., Zhao, X., Sun, X., et al. (2017). Exosomes derived from akt-modified human umbilical cord mesenchymal stem cells improve cardiac regeneration and promote angiogenesis via activating platelet-derived growth factor D. Stem Cells Transl. Med. 6 (1), 51–59. doi:10.5966/sctm.2016-0038
Mahjoor, M., Afkhami, H., Mollaei, M., Nasr, A., Shahriary, S., and Khorrami, S. (2021). MicroRNA-30c delivered by bone marrow-mesenchymal stem cells induced apoptosis and diminished cell invasion in U-251 glioblastoma cell line. Life Sci. 279, 119643. doi:10.1016/j.lfs.2021.119643
Matos, B., Bostjancic, E., Matjasic, A., Popovic, M., and Glavac, D. (2018). Dynamic expression of 11 miRNAs in 83 consecutive primary and corresponding recurrent glioblastoma: Correlation to treatment, time to recurrence, overall survival and MGMT methylation status. Radiol. Oncol. 52 (4), 422–432. doi:10.2478/raon-2018-0043
Meng, Q., Zhang, B., Zhang, Y., Wang, S., and Zhu, X. (2021). Human bone marrow mesenchymal stem cell-derived extracellular vesicles impede the progression of cervical cancer via the miR-144-3p/CEP55 pathway. J. Cell. Mol. Med. 25 (4), 1867–1883. doi:10.1111/jcmm.15573
Meng, Y., Hu, X., Li, S., Zeng, X., Qiu, L., Wei, M., et al. (2020). miR-203 inhibits cell proliferation and ERK pathway in prostate cancer by targeting IRS-1. BMC Cancer 20 (1), 1028. doi:10.1186/s12885-020-07472-2
Mishra, P. J., Mishra, P. J., Humeniuk, R., Medina, D. J., Alexe, G., Mesirov, J. P., et al. (2008). Carcinoma-associated fibroblast-like differentiation of human mesenchymal stem cells. Cancer Res. 68 (11), 4331–4339. doi:10.1158/0008-5472.CAN-08-0943
Miyazaki, Y., Oda, T., Inagaki, Y., Kushige, H., Saito, Y., Mori, N., et al. (2021). Adipose-derived mesenchymal stem cells differentiate into heterogeneous cancer-associated fibroblasts in a stroma-rich xenograft model. Sci. Rep. 11 (1), 4690. doi:10.1038/s41598-021-84058-3
Mou, Z., Xu, X., Dong, M., and Xu, J. (2016). MicroRNA-148b acts as a tumor suppressor in cervical cancer by inducing G1/S-phase cell cycle arrest and apoptosis in a caspase-3-dependent manner. Med. Sci. Monit. 22, 2809–2815. doi:10.12659/msm.896862
Munir, J., Yoon, J. K., and Ryu, S. (2020). Therapeutic miRNA-enriched extracellular vesicles: Current approaches and future prospects. Cells 9 (10), 2271. doi:10.3390/cells9102271
Nabhan, M., Louka, M. L., Khairy, E., Tash, F., Ali-Labib, R., and El-Habashy, S. (2017). MicroRNA-181a and its target smad 7 as potential biomarkers for tracking child acute lymphoblastic leukemia. Gene 628, 253–258. doi:10.1016/j.gene.2017.07.052
Naji, A., Eitoku, M., Favier, B., Deschaseaux, F., Rouas-Freiss, N., and Suganuma, N. (2019). Biological functions of mesenchymal stem cells and clinical implications. Cell. Mol. Life Sci. 76 (17), 3323–3348. doi:10.1007/s00018-019-03125-1
Naseri, Z., Oskuee, R. K., Forouzandeh-Moghadam, M., and Jaafari, M. R. (2020). Delivery of LNA-antimiR-142-3p by mesenchymal stem cells-derived exosomes to breast cancer stem cells reduces tumorigenicity. Stem Cell Rev. Rep. 16 (3), 541–556. doi:10.1007/s12015-019-09944-w
Naseri, Z., Oskuee, R. K., Jaafari, M. R., and Forouzandeh Moghadam, M. (2018). Exosome-mediated delivery of functionally active miRNA-142-3p inhibitor reduces tumorigenicity of breast cancer in vitro and in vivo. Int. J. Nanomedicine 13, 7727–7747. doi:10.2147/IJN.S182384
Neviani, P., and Fabbri, M. (2015). Exosomic microRNAs in the tumor microenvironment. Front. Med. 2, 47. doi:10.3389/fmed.2015.00047
Nip, H., Dar, A. A., Saini, S., Colden, M., Varahram, S., Chowdhary, H., et al. (2016). Oncogenic microRNA-4534 regulates PTEN pathway in prostate cancer. Oncotarget 7 (42), 68371–68384. doi:10.18632/oncotarget.12031
Nitzsche, F., Müller, C., Lukomska, B., Jolkkonen, J., Deten, A., and Boltze, J. (2017). Concise review: MSC adhesion cascade—insights into homing and transendothelial migration. Stem Cells 35 (6), 1446–1460. doi:10.1002/stem.2614
Nonaka, R., Miyake, Y., Hata, T., Kagawa, Y., Kato, T., Osawa, H., et al. (2015). Circulating miR-103 and miR-720 as novel serum biomarkers for patients with colorectal cancer. Int. J. Oncol. 47 (3), 1097–1102. doi:10.3892/ijo.2015.3064
Nonaka, R., Nishimura, J., Kagawa, Y., Osawa, H., Hasegawa, J., Murata, K., et al. (2014). Circulating miR-199a-3p as a novel serum biomarker for colorectal cancer. Oncol. Rep. 32 (6), 2354–2358. doi:10.3892/or.2014.3515
Ohno, S., Takanashi, M., Sudo, K., Ueda, S., Ishikawa, A., Matsuyama, N., et al. (2013). Systemically injected exosomes targeted to EGFR deliver antitumor microRNA to breast cancer cells. Mol. Ther. 21 (1), 185–191. doi:10.1038/mt.2012.180
Ono, M., Kosaka, N., Tominaga, N., Yoshioka, Y., Takeshita, F., Takahashi, R. U., et al. (2014). Exosomes from bone marrow mesenchymal stem cells contain a microRNA that promotes dormancy in metastatic breast cancer cells. Sci. Signal. 7 (332), ra63. doi:10.1126/scisignal.2005231
Otsu, K., Das, S., Houser, S. D., Quadri, S. K., Bhattacharya, S., and Bhattacharya, J. (2009). Concentration-dependent inhibition of angiogenesis by mesenchymal stem cells. Blood 113 (18), 4197–4205. doi:10.1182/blood-2008-09-176198
Ou, L., Wang, D., Zhang, H., Yu, Q., and Hua, F. (2018). Decreased expression of miR-138-5p by lncRNA H19 in cervical cancer promotes tumor proliferation. Oncol. Res. 26 (3), 401–410. doi:10.3727/096504017X15017209042610
Park, Y., and Kim, J. (2019). Regulation of IL-6 Signaling by miR-125a and let-7e in Endothelial Cells Controls Vasculogenic Mimicry Formation of Breast Cancer Cells. BMB Rep. 52 (3), 214–219. doi:10.5483/BMBRep.2019.52.3.308
Parys, M., Kruger, J. M., and Yuzbasiyan-Gurkan, V. (2017). Evaluation of immunomodulatory properties of feline mesenchymal stem cells. Stem Cells Dev. 26 (10), 776–785. doi:10.1089/scd.2016.0041
Păunescu, V., Deak, E., Herman, D., Siska, I. R., Tănasie, G., Bunu, C., et al. (2007). In vitro differentiation of human mesenchymal stem cells to epithelial lineage. J. Cell. Mol. Med. 11 (3), 502–508. doi:10.1111/j.1582-4934.2007.00041.x
Peng, C. Y., Wang, T. Y., Lee, S. S., Hsieh, P. L., Liao, Y. W., Tsai, L. L., et al. (2018). Let-7c restores radiosensitivity and chemosensitivity and impairs stemness in oral cancer cells through inhibiting interleukin-8. J. Oral Pathol. Med. 47 (6), 590–597. doi:10.1111/jop.12711
Peng S. Y, S. Y., Tu, H. F., Yang, C. C., Wu, C. H., Liu, C. J., Chang, K. W., et al. (2018). miR-134 targets PDCD7 to reduce E-cadherin expression and enhance oral cancer progression. Int. J. Cancer 143 (11), 2892–2904. doi:10.1002/ijc.31638
Peng, Y., and Croce, C. M. (2016). The role of MicroRNAs in human cancer. Signal Transduct. Target. Ther. 1, 15004. doi:10.1038/sigtrans.2015.4
Pittenger, M. F., Discher, D. E., Péault, B. M., Phinney, D. G., Hare, J. M., and Caplan, A. I. (2019). Mesenchymal stem cell perspective: Cell biology to clinical progress. NPJ Regen. Med. 4, 22. doi:10.1038/s41536-019-0083-6
Qiao, L., Xu, Z. L., Zhao, T. J., Ye, L. H., and Zhang, X. D. (2008). Dkk-1 secreted by mesenchymal stem cells inhibits growth of breast cancer cells via depression of Wnt signalling. Cancer Lett. 269 (1), 67–77. doi:10.1016/j.canlet.2008.04.032
Qin, F., Tang, H., Zhang, Y., Zhang, Z., Huang, P., and Zhu, J. (2020). Bone marrow-derived mesenchymal stem cell-derived exosomal microRNA-208a promotes osteosarcoma cell proliferation, migration, and invasion. J. Cell. Physiol. 235 (5), 4734–4745. doi:10.1002/jcp.29351
Qiu, Y., Yuan, Y., and Luo, P. (2022). MiR-1299 functions as a tumor suppressor to inhibit the proliferation and metastasis of gastric cancer by targeting ARF6. Genes Genomics 44 (2), 237–245. doi:10.1007/s13258-021-01124-w
Raposo, G., and Stoorvogel, W. (2013). Extracellular vesicles: Exosomes, microvesicles, and friends. J. Cell Biol. 200 (4), 373–383. doi:10.1083/jcb.201211138
Razmkhah, M., Jaberipour, M., Erfani, N., Habibagahi, M., Talei, A. R., and Ghaderi, A. (2011). Adipose derived stem cells (ASCs) isolated from breast cancer tissue express IL-4, IL-10 and TGF-β1 and upregulate expression of regulatory molecules on T cells: Do they protect breast cancer cells from the immune response? Cell. Immunol. 266 (2), 116–122. doi:10.1016/j.cellimm.2010.09.005
Ren, G., Zhao, X., Wang, Y., Zhang, X., Chen, X., Xu, C., et al. (2012). CCR2-Dependent recruitment of macrophages by tumor-educated mesenchymal stromal cells promotes tumor development and is mimicked by TNFα. Cell Stem Cell 11 (6), 812–824. doi:10.1016/j.stem.2012.08.013
Renjie, W., and Haiqian, L. (2015). MiR-132, miR-15a and miR-16 synergistically inhibit pituitary tumor cell proliferation, invasion and migration by targeting Sox5. Cancer Lett. 356 (2), 568–578. doi:10.1016/j.canlet.2014.10.003
Ries, C., Egea, V., Karow, M., Kolb, H., Jochum, M., and Neth, P. (2007). MMP-2, MT1-MMP, and TIMP-2 are essential for the invasive capacity of human mesenchymal stem cells: Differential regulation by inflammatory cytokines. Blood 109 (9), 4055–4063. doi:10.1182/blood-2006-10-051060
Rogers, T. J., Christenson, J. L., Greene, L. I., O'Neill, K. I., Williams, M. M., Gordon, M. A., et al. (2019). Reversal of triple-negative breast cancer EMT by miR-200c decreases tryptophan catabolism and a program of immunosuppression. Mol. Cancer Res. 17 (1), 30–41. doi:10.1158/1541-7786.MCR-18-0246
Ru, P., Steele, R., Hsueh, E. C., and Ray, R. B. (2011). Anti-miR-203 upregulates SOCS3 expression in breast cancer cells and enhances cisplatin chemosensitivity. Genes & Cancer 2 (7), 720–727. doi:10.1177/1947601911425832
Salinas-Vera, Y. M., Marchat, L. A., García-Vázquez, R., González de la Rosa, C. H., Castañeda-Saucedo, E., Tito, N. N., et al. (2018). Cooperative multi-targeting of signaling networks by angiomiR-204 inhibits vasculogenic mimicry in breast cancer cells. Cancer Lett. 432, 17–27. doi:10.1016/j.canlet.2018.06.003
Sato-Kuwabara, Y., Melo, S. A., Soares, F. A., and Calin, G. A. (2015). The fusion of two worlds: Non-coding RNAs and extracellular vesicles--diagnostic and therapeutic implications (review). Int. J. Oncol. 46 (1), 17–27. doi:10.3892/ijo.2014.2712
Selleri, S., Dieng, M. M., Nicoletti, S., Louis, I., Beausejour, C., Le Deist, F., et al. (2013). Cord-blood-derived mesenchymal stromal cells downmodulate CD4+ T-cell activation by inducing IL-10-producing Th1 cells. Stem Cells Dev. 22 (7), 1063–1075. doi:10.1089/scd.2012.0315
Shabani, N., Razaviyan, J., Paryan, M., Tavangar, S. M., Azizi, F., Mohammadi-Yeganeh, S., et al. (2018). Evaluation of miRNAs expression in medullary thyroid carcinoma tissue samples: miR-34a and miR-144 as promising overexpressed markers in MTC. Hum. Pathol. 79, 212–221. doi:10.1016/j.humpath.2018.05.019
Shang, C., Ke, M., Liu, L., Wang, C., Liu, Y., and Zheng, X. (2022). Exosomes from cancer-associated mesenchymal stem cells transmit TMBIM6 to promote the malignant behavior of hepatocellular carcinoma via activating PI3K/AKT pathway. Front. Oncol. 12, 868726. doi:10.3389/fonc.2022.868726
Sharif, S., Ghahremani, M. H., and Soleimani, M. (2018). Delivery of exogenous miR-124 to glioblastoma multiform cells by wharton's jelly mesenchymal stem cells decreases cell proliferation and migration, and confers chemosensitivity. Stem Cell Rev. Rep. 14 (2), 236–246. doi:10.1007/s12015-017-9788-3
Sharif, S., Ghahremani, M. H., and Soleimani, M. (2021). Differentiation induction and proliferation inhibition by a cell-free approach for delivery of exogenous miRNAs to neuroblastoma cells using mesenchymal stem cells. Cell J. 22 (4), 556–564. doi:10.22074/cellj.2021.6928
Shen, W., Xie, X. Y., Liu, M. R., and Wang, L. L. (2019). MicroRNA-101-5p inhibits the growth and metastasis of cervical cancer cell by inhibiting CXCL6. Eur. Rev. Med. Pharmacol. Sci. 23 (5), 1957–1968. doi:10.26355/eurrev_201903_17234
Sheykhhasan, M., Kalhor, N., Sheikholeslami, A., Dolati, M., Amini, E., and Fazaeli, H. (2021). Exosomes of mesenchymal stem cells as a proper vehicle for transfecting miR-145 into the breast cancer cell line and its effect on metastasis. Biomed. Res. Int. 2021, 1–15. doi:10.1155/2021/5516078
Shi, C., Ren, L., Sun, C., Yu, L., Bian, X., Zhou, X., et al. (2017). miR-29a/b/c function as invasion suppressors for gliomas by targeting CDC42 and predict the prognosis of patients. Br. J. Cancer 117 (7), 1036–1047. doi:10.1038/bjc.2017.255
Shiah, S. G., Hsiao, J. R., Chang, H. J., Hsu, Y. M., Wu, G. H., Peng, H. Y., et al. (2020). MiR-30a and miR-379 modulate retinoic acid pathway by targeting DNA methyltransferase 3B in oral cancer. J. Biomed. Sci. 27 (1), 46. doi:10.1186/s12929-020-00644-z
Shimbo, K., Miyaki, S., Ishitobi, H., Kato, Y., Kubo, T., Shimose, S., et al. (2014). Exosome-formed synthetic microRNA-143 is transferred to osteosarcoma cells and inhibits their migration. Biochem. Biophys. Res. Commun. 445 (2), 381–387. doi:10.1016/j.bbrc.2014.02.007
Shojaei, S., Hashemi, S. M., Ghanbarian, H., Salehi, M., and Mohammadi-Yeganeh, S. (2019). Effect of mesenchymal stem cells-derived exosomes on tumor microenvironment: Tumor progression versus tumor suppression. J. Cell. Physiol. 234 (4), 3394–3409. doi:10.1002/jcp.27326
Shojaei, S., Hashemi, S. M., Ghanbarian, H., Sharifi, K., Salehi, M., and Mohammadi-Yeganeh, S. (2021). Delivery of miR-381-3p mimic by mesenchymal stem cell-derived exosomes inhibits triple negative breast cancer aggressiveness; an in vitro study. Stem Cell Rev. Rep. 17 (3), 1027–1038. doi:10.1007/s12015-020-10089-4
Singh, V. K., Saini, A., Kalsan, M., Kumar, N., and Chandra, R. (2016). Describing the stem cell potency: The various methods of functional assessment and in silico diagnostics. Front. Cell Dev. Biol. 4, 134. doi:10.3389/fcell.2016.00134
Stroese, A. J., Ullerich, H., Koehler, G., Raetzel, V., Senninger, N., and Dhayat, S. A. (2018). Circulating microRNA-99 family as liquid biopsy marker in pancreatic adenocarcinoma. J. Cancer Res. Clin. Oncol. 144 (12), 2377–2390. doi:10.1007/s00432-018-2749-7
Sun, B., Zhao, X., Ming, J., Liu, X., Liu, D., and Jiang, C. (2019). Stepwise detection and evaluation reveal miR-10b and miR-222 as a remarkable prognostic pair for glioblastoma. Oncogene 38 (33), 6142–6157. doi:10.1038/s41388-019-0867-6
Théry, C., Witwer, K. W., Aikawa, E., Alcaraz, M. J., Anderson, J. D., Andriantsitohaina, R., et al. (2018). Minimal information for studies of extracellular vesicles 2018 (MISEV2018): A position statement of the international society for extracellular vesicles and update of the MISEV2014 guidelines. J. Extracell. Vesicles 7 (1), 1535750. doi:10.1080/20013078.2018.1535750
Ti, D., Hao, H., Tong, C., Liu, J., Dong, L., Zheng, J., et al. (2015). LPS-preconditioned mesenchymal stromal cells modify macrophage polarization for resolution of chronic inflammation via exosome-shuttled let-7b. J. Transl. Med. 13, 308. doi:10.1186/s12967-015-0642-6
Tian, J., Zhang, H., Mu, L., Wang, M., Li, X., Zhang, X., et al. (2020). The miR-218/GAB2 Axis regulates proliferation, invasion and EMT via the PI3K/AKT/GSK-3β pathway in prostate cancer. Exp. Cell Res. 394 (1), 112128. doi:10.1016/j.yexcr.2020.112128
Tian, L., Cao, J., Ji, Q., Zhang, C., Qian, T., Song, X., et al. (2017). The downregulation of miR-3173 in B-cell acute lymphoblastic leukaemia promotes cell invasion via PTK2. Biochem. Biophysical Res. Commun. 494 (3-4), 569–574. doi:10.1016/j.bbrc.2017.10.013
Tse, W. T., Pendleton, J. D., Beyer, W. M., Egalka, M. C., and Guinan, E. C. (2003). Suppression of allogeneic T-cell proliferation by human marrow stromal cells: Implications in transplantation. Transplantation, 75, 389–397. doi:10.1097/01.TP.0000045055.63901
Tsukamoto, S., Honoki, K., Fujii, H., Tohma, Y., Kido, A., Mori, T., et al. (2012). Mesenchymal stem cells promote tumor engraftment and metastatic colonization in rat osteosarcoma model. Int. J. Oncol. 40 (1), 163–169. doi:10.3892/ijo.2011.1220
Uchibori, R., Tsukahara, T., Mizuguchi, H., Saga, Y., Urabe, M., Mizukami, H., et al. (2013). NF-κB activity regulates mesenchymal stem cell accumulation at tumor sites. Cancer Res. 73 (1), 364–372. doi:10.1158/0008-5472.CAN-12-0088
Urbanek-Trzeciak, M. O., Galka-Marciniak, P., Nawrocka, P. M., Kowal, E., Szwec, S., Giefing, M., et al. (2020). Pan-cancer analysis of somatic mutations in miRNA genes. EBioMedicine 61, 103051. doi:10.1016/j.ebiom.2020.103051
Vafadar, A., Mokaram, P., Erfani, M., Yousefi, Z., Farhadi, A., Elham Shirazi, T., et al. (2019). The effect of decitabine on the expression and methylation of the PPP1CA, BTG2, and PTEN in association with changes in miR-125b, miR-17, and miR-181b in NALM6 cell line. J. Cell. Biochem. 120 (8), 13156–13167. doi:10.1002/jcb.28590
Walker, S., Busatto, S., Pham, A., Tian, M., Suh, A., Carson, K., et al. (2019). Extracellular vesicle-based drug delivery systems for cancer treatment. Theranostics 9 (26), 8001–8017. doi:10.7150/thno.37097
Wan, F. Z., Chen, K. H., Sun, Y. C., Chen, X. C., Liang, R. B., Chen, L., et al. (2020). Exosomes overexpressing miR-34c inhibit malignant behavior and reverse the radioresistance of nasopharyngeal carcinoma. J. Transl. Med. 18 (1), 12. doi:10.1186/s12967-019-02203-z
Wang, H., Tao, T., Yan, W., Feng, Y., Wang, Y., Cai, J., et al. (2015). Upregulation of miR-181s reverses mesenchymal transition by targeting KPNA4 in glioblastoma. Sci. Rep. 5, 13072. doi:10.1038/srep13072
Wang, J., Chen, D., and Ho, E. A. (2021). Challenges in the development and establishment of exosome-based drug delivery systems. J. Control. Release 329, 894–906. doi:10.1016/j.jconrel.2020.10.020
Wang L, L., Yin, P., Wang, J., Wang, Y., Sun, Z., Zhou, Y., et al. (2019). Delivery of mesenchymal stem cells-derived extracellular vesicles with enriched miR-185 inhibits progression of OPMD. Artif. Cells Nanomed. Biotechnol. 47 (1), 2481–2491. doi:10.1080/21691401.2019.1623232
Wang, L., Ouyang, M., Xing, S., Zhao, S., Liu, S., Sun, L., et al. (2022). Mesenchymal stem cells and their derived exosomes promote malignant phenotype of polyploid non-small-cell lung cancer cells through AMPK signaling pathway. Anal. Cell. Pathol. Amst. 2022, 1–15. doi:10.1155/2022/8708202
Wang S, S., Su, X., Xu, M., Xiao, X., Li, X., Li, H., et al. (2019). Exosomes secreted by mesenchymal stromal/stem cell-derived adipocytes promote breast cancer cell growth via activation of Hippo signaling pathway. Stem Cell Res. Ther. 10 (1), 117. doi:10.1186/s13287-019-1220-2
Wang, S., Huang, J., Lyu, H., Lee, C. K., Tan, J., Wang, J., et al. (2013). Functional cooperation of miR-125a, miR-125b, and miR-205 in entinostat-induced downregulation of erbB2/erbB3 and apoptosis in breast cancer cells. Cell Death Dis. 4 (3), e556. doi:10.1038/cddis.2013.79
Watt, F. M., and Driskell, R. R. (2010). The therapeutic potential of stem cells. Phil. Trans. R. Soc. B 365 (1537), 155–163. doi:10.1098/rstb.2009.0149
Wong, S. T., Zhang, X. Q., Zhuang, J. T., Chan, H. L., Li, C. H., and Leung, G. K. (2012). MicroRNA-21 inhibition enhances in vitro chemosensitivity of temozolomide-resistant glioblastoma cells. Anticancer Res. 32 (7), 2835–2841.
Wu, S., Ju, G. Q., Du, T., Zhu, Y. J., and Liu, G. H. (2013). Microvesicles derived from human umbilical cord wharton's jelly mesenchymal stem cells attenuate bladder tumor cell growth in vitro and in vivo. PloS One 8 (4), e61366. doi:10.1371/journal.pone.0061366
Xiao, F., Cheng, Z., Wang, P., Gong, B., Huang, H., Xing, Y., et al. (2018). MicroRNA-28-5p inhibits the migration and invasion of gastric cancer cells by suppressing AKT phosphorylation. Oncol. Lett. 15 (6), 9777–9785. doi:10.3892/ol.2018.8603
Xie, C., Du, L. Y., Guo, F., Li, X., and Cheng, B. (2019). Exosomes derived from microRNA-101-3p-overexpressing human bone marrow mesenchymal stem cells suppress oral cancer cell proliferation, invasion, and migration. Mol. Cell. Biochem. 458 (1-2), 11–26. doi:10.1007/s11010-019-03526-7
Xie, H., and Wang, J. (2021). MicroRNA-320a-Containing exosomes from human umbilical cord mesenchymal stem cells curtail proliferation and metastasis in lung cancer by binding to SOX4. J. Recept. Signal Transduct. 7, 268–278. doi:10.1080/10799893.2021.1918166
Xitong, D., and Xiaorong, Z. (2016). Targeted therapeutic delivery using engineered exosomes and its applications in cardiovascular diseases. Gene 575 (2), 377–384. doi:10.1016/j.gene.2015.08.067
Xu, C. H., Liu, Y., Xiao, L. M., Chen, L. K., Zheng, S. Y., Zeng, E. M., et al. (2019). Silencing microRNA-221/222 cluster suppresses glioblastoma angiogenesis by suppressor of cytokine signaling-3-dependent JAK/STAT pathway. J. Cell. Physiol. 234 (12), 22272–22284. doi:10.1002/jcp.28794
Xu, H., Zhao, G., Zhang, Y., Jiang, H., Wang, W., Zhao, D., et al. (2019). Mesenchymal stem cell-derived exosomal microRNA-133b suppresses glioma progression via wnt/β-catenin signaling pathway by targeting EZH2. Stem Cell Res. Ther. 10 (1), 381. doi:10.1186/s13287-019-1446-z
Xu, S., Zhao, N., Hui, L., Song, M., Miao, Z. W., and Jiang, X. J. (2016). MicroRNA-124-3p inhibits the growth and metastasis of nasopharyngeal carcinoma cells by targeting STAT3. Oncol. Rep. 35 (3), 1385–1394. doi:10.3892/or.2015.4524
Yan, L., Zheng, D., and Xu, R. H. (2018). Critical role of tumor necrosis factor signaling in mesenchymal stem cell-based therapy for autoimmune and inflammatory diseases. Front. Immunol. 9, 1658. doi:10.3389/fimmu.2018.01658
Yan, T., Wu, M., Lv, S., Hu, Q., Xu, W., Zeng, A., et al. (2021). Exosomes derived from microRNA-512-5p-transfected bone mesenchymal stem cells inhibit glioblastoma progression by targeting JAG1. Aging 13 (7), 9911–9926. doi:10.18632/aging.202747
Yang, Y., Ji, C., Guo, S., Su, X., Zhao, X., Zhang, S., et al. (2017). The miR-486-5p plays a causative role in prostate cancer through negative regulation of multiple tumor suppressor pathways. Oncotarget 8 (42), 72835–72846. doi:10.18632/oncotarget.20427
Yao, J., Deng, B., Zheng, L., Dou, L., Guo, Y., and Guo, K. (2016). miR-27b is upregulated in cervical carcinogenesis and promotes cell growth and invasion by regulating CDH11 and epithelial-mesenchymal transition. Oncol. Rep. 35 (3), 1645–1651. doi:10.3892/or.2015.4500
Ye, T., Yang, M., Huang, D., Wang, X., Xue, B., Tian, N., et al. (2019). MicroRNA-7 as a potential therapeutic target for aberrant NF-κB-Driven distant metastasis of gastric cancer. J. Exp. Clin. Cancer Res. 38 (1), 55. doi:10.1186/s13046-019-1074-6
Yi, Y., Liu, Y., Wu, W., Wu, K., and Zhang, W. (2018). The role of miR-106p-5p in cervical cancer: From expression to molecular mechanism. Cell Death Discov. 4, 94. doi:10.1038/s41420-018-0096-8
Yu, L., Gui, S., Liu, Y., Qiu, X., Zhang, G., Zhang, X., et al. (2019). Exosomes derived from microRNA-199a-overexpressing mesenchymal stem cells inhibit glioma progression by down-regulating AGAP2. Aging 11 (15), 5300–5318. doi:10.18632/aging.102092
Yuan, L., Liu, Y., Qu, Y., Liu, L., and Li, H. (2019). Exosomes derived from microRNA-148b-3p-overexpressing human umbilical cord mesenchymal stem cells restrain breast cancer progression. Front. Oncol. 9, 1076. doi:10.3389/fonc.2019.01076
Zhang, B., and Farwell, M. A. (2008). Translational medicine: microRNAs: A new emerging class of players for disease diagnostics and gene therapy. J. Cell. Mol. Med. 12 (1), 3–21. doi:10.1111/j.1582-4934.2007.00196.x
Zhang, B., Wang, M., Gong, A., Zhang, X., Wu, X., Zhu, Y., et al. (2015). HucMSC-exosome mediated-wnt4 signaling is required for cutaneous wound healing. Stem Cells 33 (7), 2158–2168. doi:10.1002/stem.1771
Zhang, F., Lu, Y., Wang, M., Zhu, J., Li, J., Zhang, P., et al. (2020). Exosomes derived from human bone marrow mesenchymal stem cells transfer miR-222-3p to suppress acute myeloid leukemia cell proliferation by targeting IRF2/INPP4B. Mol. Cell. Probes 51, 101513. doi:10.1016/j.mcp.2020.101513
Zhang, H., and Fan, Q. (2015). MicroRNA-205 inhibits the proliferation and invasion of breast cancer by regulating AMOT expression. Oncol. Rep. 34 (4), 2163–2170. doi:10.3892/or.2015.4148
Zhang, L., Hao, C., Yao, S., Tang, R., Guo, W., Cong, H., et al. (2018). Exosomal miRNA profiling to identify nanoparticle phagocytic mechanisms. Small 14 (15), e1704008. doi:10.1002/smll.201704008
Zhang, Y., Liu, Q., Zhang, X., Huang, H., Tang, S., Chai, Y., et al. (2022). Recent advances in exosome-mediated nucleic acid delivery for cancer therapy. J. Nanobiotechnology 20 (1), 279. doi:10.1186/s12951-022-01472-z
Zhao, J. L., Zhang, L., Guo, X., Wang, J. H., Zhou, W., Liu, M., et al. (2015). miR-212/132 downregulates SMAD2 expression to suppress the G1/S phase transition of the cell cycle and the epithelial to mesenchymal transition in cervical cancer cells. IUBMB Life 67 (5), 380–394. doi:10.1002/iub.1381
Zhao, J., Xu, H., Duan, Z., Chen, X., Ao, Z., Chen, Y., et al. (2020). miR-31-5p regulates 14-3-3 ɛ to inhibit prostate cancer 22RV1 cell survival and proliferation via PI3K/AKT/Bcl-2 signaling pathway. Cancer Manag. Res. 12, 6679–6694. doi:10.2147/CMAR.S247780
Zhao, S., Yao, D., Chen, J., Ding, N., and Ren, F. (2015). MiR-20a promotes cervical cancer proliferation and metastasis in vitro and in vivo. PloS One 10 (3), e0120905. doi:10.1371/journal.pone.0120905
Zheng, T., Zhou, Y., Xu, X., Qi, X., Liu, J., Pu, Y., et al. (2022). MiR-30c-5p loss-induced PELI1 accumulation regulates cell proliferation and migration via activating PI3K/AKT pathway in papillary thyroid carcinoma. J. Transl. Med. 20 (1), 20. doi:10.1186/s12967-021-03226-1
Zhou, T., Yuan, Z., Weng, J., Pei, D., Du, X., He, C., et al. (2021). Challenges and advances in clinical applications of mesenchymal stromal cells. J. Hematol. Oncol. 14 (1), 24. doi:10.1186/s13045-021-01037-x
Zhou, W., Shi, G., Zhang, Q., Wu, Q., Li, B., and Zhang, Z. (2014). MicroRNA-20b promotes cell growth of breast cancer cells partly via targeting phosphatase and tensin homologue (PTEN). Cell Biosci. 4 (1), 62. doi:10.1186/2045-3701-4-62
Zhou, X., Ren, Y., Moore, L., Mei, M., You, Y., Xu, P., et al. (2010). Downregulation of miR-21 inhibits EGFR pathway and suppresses the growth of human glioblastoma cells independent of PTEN status. Lab. Invest. 90 (2), 144–155. doi:10.1038/labinvest.2009.126
Zhou, X., Wen, W., Zhu, J., Huang, Z., Zhang, L., Zhang, H., et al. (2017). A six-microRNA signature in plasma was identified as a potential biomarker in diagnosis of esophageal squamous cell carcinoma. Oncotarget 8 (21), 34468–34480. doi:10.18632/oncotarget.16519
Zhu, C., Wang, K., Chen, Z., Han, Y., Chen, H., Li, Q., et al. (2020). Antinociceptive effect of intrathecal injection of miR-9-5p modified mouse bone marrow mesenchymal stem cells on a mouse model of bone cancer pain. J. Neuroinflammation 17 (1), 85. doi:10.1186/s12974-020-01765-w
Zhu, N., Zhang, D., Xie, H., Zhou, Z., Chen, H., Hu, T., et al. (2011). Endothelial-specific intron-derived miR-126 is down-regulated in human breast cancer and targets both VEGFA and PIK3R2. Mol. Cell. Biochem. 351 (1-2), 157–164. doi:10.1007/s11010-011-0723-7
Zhu, W., Huang, L., Li, Y., Zhang, X., Gu, J., Yan, Y., et al. (2012). Exosomes derived from human bone marrow mesenchymal stem cells promote tumor growth in vivo. Cancer Lett. 315 (1), 28–37. doi:10.1016/j.canlet.2011.10.002
Zhu, Y., Sun, Z., Han, Q., Liao, L., Wang, J., Bian, C., et al. (2009). Human mesenchymal stem cells inhibit cancer cell proliferation by secreting DKK-1. Leukemia 23 (5), 925–933. doi:10.1038/leu.2008.384
Zou, G., Wang, R., and Wang, M. (2019). Clinical response and prognostic significance of serum miR-497 expression in colorectal cancer. Cancer Biomarkers 25 (1), 11–18. doi:10.3233/CBM-181902
Zou, X., Wei, J., Huang, Z., Zhou, X., Lu, Z., Zhu, W., et al. (2019). Identification of a six-miRNA panel in serum benefiting pancreatic cancer diagnosis. Cancer Med. 8 (6), 2810–2822. doi:10.1002/cam4.2145
Keywords: mesenchymal stem cells, exosomes, micro RNA, cancer therapy, cell free therapy
Citation: Dalmizrak A and Dalmizrak O (2022) Mesenchymal stem cell-derived exosomes as new tools for delivery of miRNAs in the treatment of cancer. Front. Bioeng. Biotechnol. 10:956563. doi: 10.3389/fbioe.2022.956563
Received: 30 May 2022; Accepted: 01 September 2022;
Published: 26 September 2022.
Edited by:
Abdelkrim Hmadcha, Universidad Pablo de Olavide, SpainReviewed by:
Suchi Gupta, All India Institute of Medical Sciences, IndiaYujie Liang, Shenzhen KangNing Hospital, China
Peipei Wu, Jiangsu University, China
Copyright © 2022 Dalmizrak and Dalmizrak. This is an open-access article distributed under the terms of the Creative Commons Attribution License (CC BY). The use, distribution or reproduction in other forums is permitted, provided the original author(s) and the copyright owner(s) are credited and that the original publication in this journal is cited, in accordance with accepted academic practice. No use, distribution or reproduction is permitted which does not comply with these terms.
*Correspondence: Ozlem Dalmizrak, b3psZW0uZGFsbWl6cmFrQG5ldS5lZHUudHI=
†ORCID: Aysegul Dalmizrak, orcid.org/0000-0003-3331-6768; Ozlem Dalmizrak, orcid.org/0000-0002-5239-5871