- 1Department of Mechanical, Materials and Aerospace Engineering, School of Engineering, University of Liverpool, Liverpool, United Kingdom
- 2Institute of Life Course and Medical Sciences, University of Liverpool, Liverpool, United Kingdom
- 3Medical Research Council Versus Arthritis Centre for Integrated Research Into Musculoskeletal Ageing (CIMA), University of Liverpool, Liverpool, United Kingdom
- 4Institute of Medical and Biological Engineering, School of Mechanical Engineering, Faculty of Engineering, University of Leeds, Leeds, United Kingdom
- 5School of Dentistry, University of Liverpool, Liverpool, United Kingdom
- 6School of Veterinary Science, University of Liverpool, Liverpool, United Kingdom
Knee joint ligaments provide stability to the joint by preventing excessive movement. There has been no systematic effort to study the effect of OA and ageing on the mechanical properties of the four major human knee ligaments. This study aims to collate data on the material properties of the anterior (ACL) and posterior (PCL) cruciate ligaments, medial (MCL) and lateral (LCL) collateral ligaments. Bone-ligament-bone specimens from twelve cadaveric human knee joints were extracted for this study. The cadaveric knee joints were previously collected to study ageing and OA on bone and cartilage material properties; therefore, combining our previous bone and cartilage data with the new ligament data from this study will facilitate subject-specific whole-joint modelling studies. The bone-ligament-bone specimens were tested under tensile loading to failure, determining material parameters including yield and ultimate (failure) stress and strain, secant modulus, tangent modulus, and stiffness. There were significant negative correlations between age and ACL yield stress (p = 0.03), ACL failure stress (p = 0.02), PCL secant (p = 0.02) and tangent (p = 0.02) modulus, and LCL stiffness (p = 0.046). Significant negative correlations were also found between OA grades and ACL yield stress (p = 0.02) and strain (p = 0.03), and LCL failure stress (p = 0.048). However, changes in age or OA grade did not show a statistically significant correlation with the MCL tensile parameters. Due to the small sample size, the combined effect of age and the presence of OA could not be statistically derived. This research is the first to report tensile properties of the four major human knee ligaments from a diverse demographic. When combined with our previous findings on bone and cartilage for the same twelve knee cadavers, the current ligament study supports the conceptualisation of OA as a whole-joint disease that impairs the integrity of many peri-articular tissues within the knee. The subject-specific data pool consisting of the material properties of the four major knee ligaments, subchondral and trabecular bones and articular cartilage will advance knee joint finite element models.
Introduction
Tensile properties of the anterior cruciate ligament (ACL), posterior cruciate ligament (PCL), medial collateral ligament (MCL) and lateral collateral ligament (LCL) have been explored by numerous researchers (Noyes & Grood, 1976; Woo et al., 1991; Race & Amis, 1994; Robinson, Bull & Amis, 2005; Bonner et al., 2015; Smeets et al., 2017; Cho & Kwak, 2020; Patel et al., 2021), providing vital information on their structural and mechanical properties. There are data for all four ligaments from varying specimens in previous studies; however, there is marked variability in the reported values, likely due to variations in testing techniques and donor demographics, making it challenging to understand the whole-joint function (Peters et al., 2018a). To date, very few studies have explored all four ligaments from the same donor (donors were limited to healthy knee joints), with data suggesting higher stiffness and failure load in the cruciate ligaments compared to the collateral ligaments (Trent, Walker & Wolf, 1976; van Dommelen et al., 2005).
The lack of consistent healthy baseline measurements means our understanding of how tensile properties of all four ligaments within the same knee joint change with ageing or disease is presently unclear (Peters et al., 2018a). Structural and functional capabilities are known to decline with age in the ACL, in particular, a decrease in ultimate failure load from older donors (67–90 years) when compared to donors between 40 and 50 years old and younger donors (22–35 years) (Woo et al., 1991). This decline in properties is also reflected at a cellular level in ligaments such that ACL histological parameters showed an increase in tissue degeneration with age (Hasegawa et al., 2012). However, any differences in material properties in the PCL, MCL and LCL are yet to be systematically correlated with different age categories. Changes to integrity and tensile properties not only leave ligaments vulnerable to further injury but also affect the peri-articular tissues leading to muscle weakening through immobility and whole-joint disruption, including the development of osteoarthritis (OA) (Manninen et al., 1996; Rousseau & Garnero, 2012; Simon et al., 2015). In addition, our knowledge about the effect of OA on the tensile properties of the knee joint ligaments is limited, with current studies focusing primarily on histological analyses. There is evidence showing impaired integrity of the ACL and PCL during total knee replacements in the presence of OA and with age (Mullaji et al., 2008; Hasegawa et al., 2012).
Previously, we systematically investigated the effect of age and OA on the mechanical properties of bone and cartilage in human knee joints for the first time in the same donor (Peters et al., 2018b). Here, we have employed the same human cadavers to study the ligaments, which will allow 1) the first assessment of changes in the mechanical behaviour of ligaments due to ageing and OA, 2) ligament data to be combined with bone and cartilage trends from the same specimen to give a fuller picture of the multi-tissue joint (whole-joint) changes with age and OA, and 3) the subject-level data to be used in the future development of subject-specific OA knee joint computer models. Thus, this study aimed to obtain data on tissue-level material characteristics of cadaveric human knee joint ligaments with a wide span of age and OA grades and correlate these to age and OA grade as univariate parameters. The following objectives were performed to fulfil the aim of this study:
1 To harvest the four major knee joint ligaments (ACL, PCL, LCL and MCL) as bone-ligament-bone specimens and measure undeformed geometrical parameters of the ligaments.
2 To apply physiologically relevant tensile loads on the ligaments and determine their mechanical responses.
3 To analyse the ligaments’ tensile characteristics and tests their correlations with age and OA.
Materials and methods
Specimens
Fresh-frozen human cadaveric knee joints were sourced from Science Care (Phoenix, Arizona, United States) via Newcastle Surgical Training Centre (Newcastle upon Tyne, NE7 7DN, United Kingdom), and consents were obtained and held by Science Care. The knee cadavers were from humans aged 31–88 years (n = 12; four female and eight male) (Supplementary Table S2) as reported in our previous study (Peters et al., 2018b). Ethical permission for using the human cadaveric materials was sponsored by the University of Liverpool and granted by the National Research Ethics Service (15/NS/0053), who approved all protocols. All experiments were performed following relevant guidelines and regulations.
Cadaver limbs were initially frozen at -20°C and thawed at 3–5°C for 5 days before dissection. During dissection, cadavers were photographed and graded for OA using the International Cartilage Repair Society (ICRS) (Supplementary Table S1) as reported in our previous study (Peters et al., 2018b). Four bone-ligament-bone specimens were harvested from each cadaver using a low-speed oscillating saw (deSoutter Medical, Bucks, UK) (Figure 1A). Extracted specimens were stored at -20°C before thawed for 24 h at 3–5°C and submerged in phosphate-buffered saline (PBS). Overall the specimens underwent two freeze-thaw cycles, which have previously been shown not to affect ligament and tendon material properties (Woo et al., 1986; Moon et al., 2006; Huang et al., 2011; Jung et al., 2011; Peters et al., 2017). Specimen numbers are consistent with those in Peters et al. (2018b), allowing the matching of ligament properties presented here with previously reported cartilage and bone data from the same individuals (Supplementary Materials (Ligament Raw Data. xlsx)).
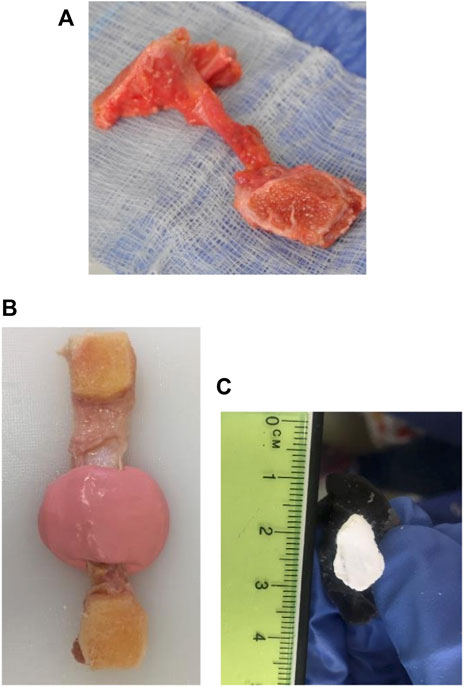
FIGURE 1. Bone-ligament-bone preparation and method for measuring the middle cross-sectional area of knee joint ligament specimens. (A) Bone-ligament-bone specimen. (B) Ligament encased in the impression material. (C) A polymethyl-methacrylate cast of a ligament photographed for cross-sectional area measurement.
Length and cross-sectional area measurements
Prior to mechanical tests, lengths of the ligaments were collected, and these were determined as the distance between the bone attachment areas using Vernier callipers (D00352, Duratool, Taiwan) (Readioff et al., 2020b).
The cross-sectional areas of all ligament specimens were obtained using a previously described method (Goodship & Birch, 2005; Readioff et al., 2020b). In brief, ligaments were encased in a fast-setting alginate impression paste (UnoDent, Essex, England) (Figure 1B). Once the impression material was set, a scalpel blade was used to slice the mould, then filled with polymethyl-methacrylate (PMMA) (Teknovit 6091, Heraeus Kulzer GmbH, Wehrheim, Germany) to create a replica of the ligament structure. Once the PMMA was set, the mould was sliced transversely, and the resulting ends were coloured with a permanent white marker pen (Figure 1C). The cement mould ends were then photographed and digitally measured using ImageJ (Schneider, Rasband & Eliceiri, 2012) to obtain the cross-sectional area of the ligament.
Specimen preparation
The femur and tibia bones around the attachment sites of each ligament were cut into a suitable shape, maintaining ligaments’ in vivo orientation, using a hand saw (Figure 1A). For example, the bone ends of the ACLs and PCLs were cut into a suitable shape to preserve the ligaments’ slight proximal-to-distal spiral during potting. The bone ends of the specimens were potted into custom-made stainless-steel holders and screwed in place. PMMA was then poured into the holder and left to cure for 4–5 min (Comerford et al., 2005; Bonner et al., 2015; Liu et al., 2020). Specimens were then attached to the load cell and encased into a watertight custom-made chamber. The chamber was filled with PBS to control specimen hydration during testing (Figure 2).
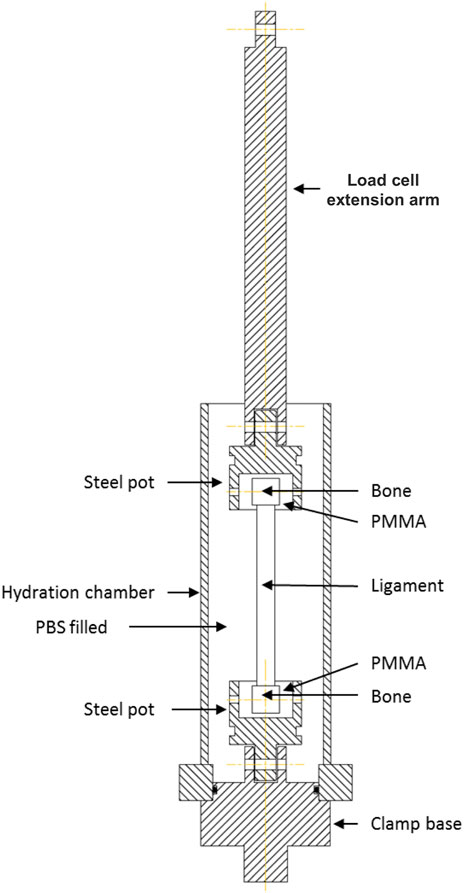
FIGURE 2. Schematic illustration of the custom-made rig for tensile testing of human knee joint ligaments. The bone ends of the ligament were secured by potting them into a polymethyl-methacrylate (PMMA) holder. Ligaments were encased into a watertight chamber filled with phosphate buffer saline (PBS) to maintain tissue hydration during mechanical tests.
Tensile testing protocol
A uniaxial tensile testing machine (Instron 3366, Buckinghamshire, UK) with a 5000 N load cell (Instron 2519) was used. A preload of 1 N was applied. All ligaments underwent ten preconditioning cycles at 10 mm/min with a load of 1–40 N, which provides a stable and repeatable viscoelastic response (Momersteeg et al., 1995). Loading was then set to zero, and ligaments were loaded to failure at 500 mm/min. A fast strain rate was chosen over slow stain rates to mimic the physiological loading (Noyes & Grood, 1976; Sharma et al., 2008; Bersini, Sansone & Frigo, 2016) and replicate a realistic injury environment (Robinson, Bull & Amis, 2005). In addition, faster strain rates improve the chances of the ligament rupturing mid-substance instead of a bony avulsion (Noyes & Grood, 1976).
Material properties
The bone-ligament-bone specimens were mechanically tested and analysed to collate multiple material property data. Parameters were obtained from the stress-strain curves, including yield and failure stresses and strains, tangent (the slope between yield and sub-yield) and secant moduli, and stiffness (Figure 3)
where
where
where
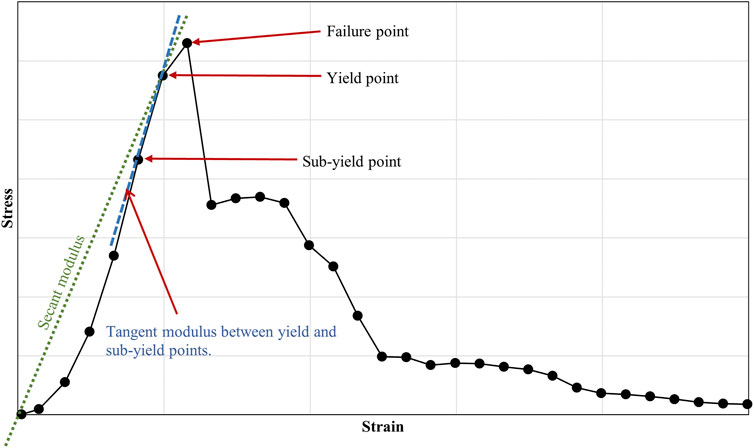
FIGURE 3. Example of a stress-strain curve, showing failure, yield and sub-yield points in a human knee joint ligament. The figure also highlights the secant and tangent moduli.
Statistical analysis
Kruskal–Wallis one-way ANOVA was conducted to compare mean differences of ligament material properties between young healthy (≤60 years old, ICRS grade 0), young OA (≤60 years old, ICRS grade 1–4) and old OA (>60, ICRS grade 1–4) cohorts. Ligament tensile properties were correlated with increasing age and grade of OA using Kendall’s Tau-b (
Results
Specimens
ACL (n = 12), PCL (n = 12), MCL (n = 12), and LCL (n = 12) specimens were obtained from twelve human cadavers. One MCL specimen from a young (37 years old) healthy donor was visually determined as severely abnormal, and data from an MCL specimen from ICRS grade 1 donor was unable to be retained (Supplementary Figure S1). Hence, they were excluded from statistical analyses.
The ICRS gradings for all 12 cadaveric knees were given and reported in Table 1, and they are the same as those reported in our previous work (Peters et al., 2018b). Three knees were given ICRS grade 0 (age: 31, 37 and 43 years old), another three knees with ICRS grade 1 (age: 49, 51 and 86 years old), two knees with ICRS grade 2 (age: 58 and 79 years old), three knees with ICRS grade 3 (age: two 72 and 88 years old) and one knee with ICRS grade 4 (age: 80 years old).
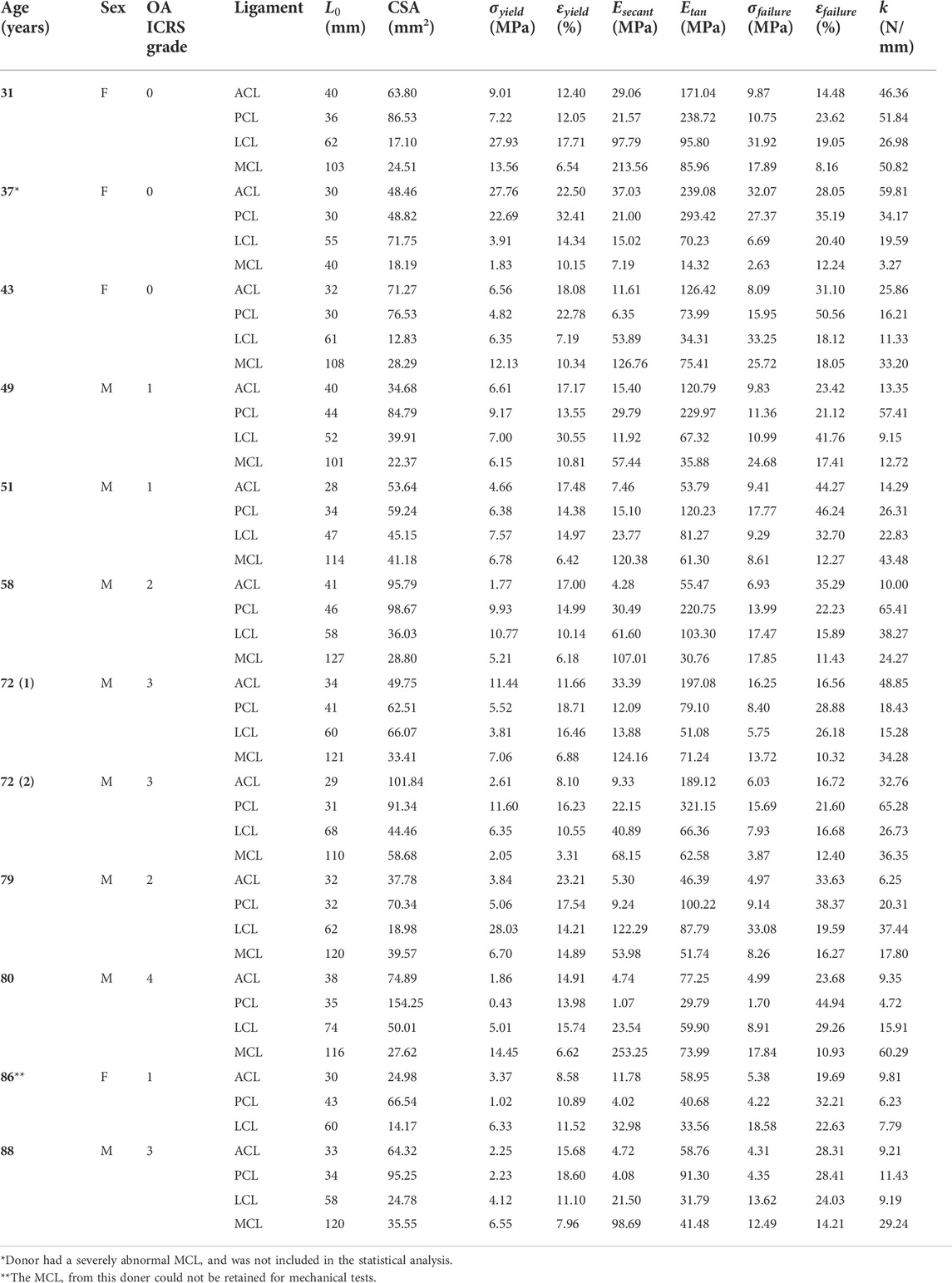
TABLE 1. Anterior (ACL) and posterior (PCL) cruciate ligaments, medial (MCL) and lateral (LCL) collateral ligaments material property data for all cadavers. ABBREVIATIONS: F, female; M, Male; OA ICRS, Osteoarthritis International Cartilage Repair Society;
Cross-sectional area and length measurements
Cross-sectional areas of the ACLs, PCLs, LCLs and MCLs were in the range of 25–102, 49 to 154, 13 to 72, and 18–59 mm2, respectively. Lengths of the ACLs, PCLs, LCLs and MCLs were in the range of 28–41, 30 to 46, 47 to 74, and 40–127 mm, respectively. The cross-sectional areas and lengths of individual ligaments for each donor are reported in Table 1 and illustrated in Figure 4.
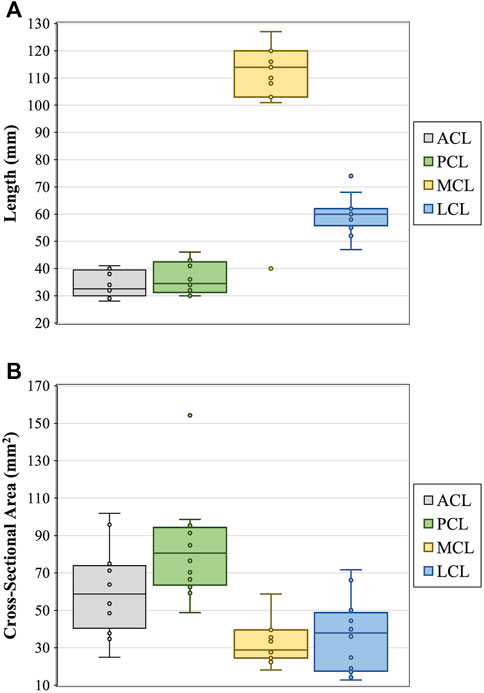
FIGURE 4. Measurements of ligament (A) length and (B) cross-sectional area for the anterior (ACL) and posterior (PCL) cruciate ligaments, and medial (MCL) and lateral (LCL) collateral ligaments.
Correlation with age
Increasing age resulted in statistically significant negative correlations with ACL yield force (
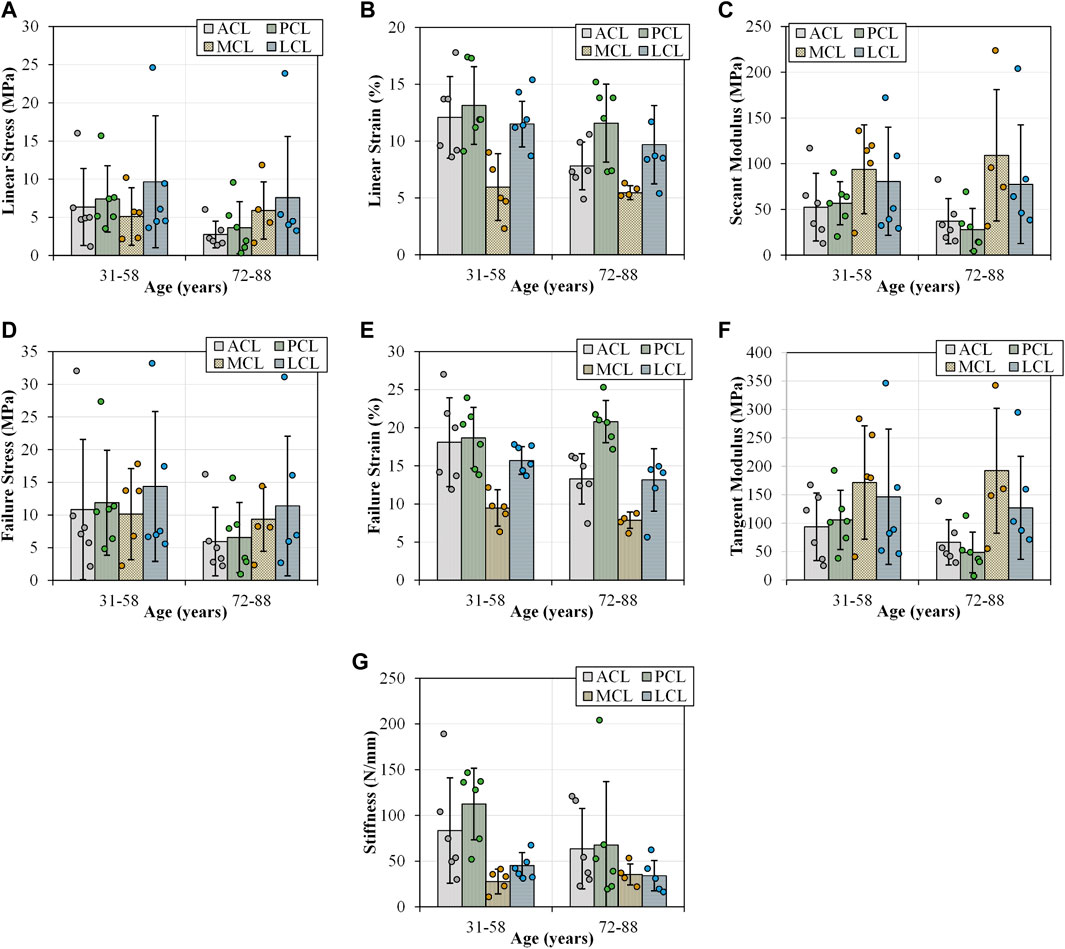
FIGURE 5. Tensile parameters for the anterior (ACL) and posterior (PCL) cruciate ligaments, and lateral (LCL) and medial (MCL) collateral ligaments across two age groups (31-58 and 72–88 years old). Error bars represent standard deviation. (A) Linear stress and (B) linear strain were utilised to determine (C) secant modulus. (D) and (E) demonstrates the maximum stresses and strains that resulted in ligament failures. (F) This sub-figure shows tangent modulus of the ligaments between the two age groups at the maximum linear region of load-extension curves. (G) This sub-figure documents the change in stiffness of the ligaments with age.
Increasing age showed statistically significant negative correlations with PCL secant modulus (
There were no statistically significant correlations between age and MCL tensile parameters (MCL_AllStat.xls). Only LCL stiffness showed a statistically significant negative correlation with age (
A detailed correlation of age with material properties of the four ligaments is reported in the (Supplementary Table S3, Supplementary Table S4 and Supplementary Figure S3).
Correlation with osteoarthritis
Increasing OA grade showed a statistically significant negative correlation with ACL yield force (
No statistically significant correlations existed between OA grade and PCL and MCL tensile parameters. Only LCL failure stress showed a statistically significant negative correlation between OA grade and LCL failure stress (
A detailed correlation of OA with material properties of the four ligaments is reported in the (Supplementary Table S3, Supplementary Table S4 and Supplementary Figure S3).
Discussion
This paper reports the first ex vivo study to quantify the effects of ageing and OA on the material properties of the four primary knee ligaments from the same cadaveric joints within a wide span of age (31–88 years old) and OA grade (ICRS 0–4). Our results showed statistically significant negative correlations with ACL yield and failure forces, stresses and extensions, PCL secant and tangent modulus and LCL stiffness (Supplementary Table S4). Similarly, increasing OA grade showed a statistically significant negative correlation with ACL yield forces, stresses, extensions, strains, and LCL failure stress (Supplementary Table S4). Changes in age or OA grade did not significantly correlate with the MCL material parameters (Supplementary Table S4). This data is vital for understanding joint mechanics, and it can provide an insight into the progression of OA as a whole-joint disease as well as the effects of ageing, notably because bone and cartilage mechanical properties for these specific human cadavers have already been reported in our previous study (Peters et al., 2018b).
Failure loads previously reported across any age category span two orders of magnitude between 495 and 2160 N in the ACL, 258–1620 N in the PCL, 194–534 N in the MCL and 376 N in the LCL (Noyes & Grood, 1976; Trent, Walker & Wolf, 1976; Woo et al., 1991; Race & Amis, 1994; Harner et al., 1995; Chandrashekar et al., 2006). Furthermore, previous studies also reported stiffness values which ranged between 124 and 308 N/mm in the ACL, 57–347 N/mm in the PCL, 70 N/mm in the MCL and 59 N/mm in the LCL, where values reported for failure load (Figure 5D) and stiffness (Figure 5G) in the current study fall within the previously reported range (Noyes & Grood, 1976; Trent, Walker & Wolf, 1976; Woo et al., 1991; Race & Amis, 1994; Harner et al., 1995; Chandrashekar et al., 2006). Previous research has indicated a decrease in the ACL failure load with increasing age, consistent with the current study (Figure 5D). Age-based differences show ACL failure loads of up to 2160 N amongst younger donors (22–35 years), 1503 N in middle-aged donors (40–50 years) and 658 N amongst older donors (60–97 years). However, Woo et al. (1991) did not indicate degeneration of joint integrity.
The current research showed a decrease in the failure strain of all four knee ligaments with the development of OA (Figure 6E and Supplementary Figure S3). The ACL in healthy knees showed higher yield and failure stresses (Figures 6A,D) and strains (Figures 6B,E), secant (Figure 6C) and tangent (Figure 6F) modulus and stiffness (Figure 6G) when compared to those with OA. The influence of OA has previously been investigated in animal models, and a reduction in tensile properties of the rat ACL was reported 10 weeks after collagen-induced arthritis. Ultimate failure load was reduced by 25.1% and stiffness by 38.0% compared to controls (Nawata et al., 2001). Despite a lack of knee joint material properties in the literature associated with OA in humans, previous research has found that between 39 and 78% of patients with OA have a degenerated ACL (Allain, Goutallier & Voisin, 2001; Cushner et al., 2003; Lee et al., 2005; Mullaji et al., 2008; Watanabe et al., 2011), and between 7 and 80% have a degenerated PCL (Nelissen & Hogendoorn, 2001; Stubbs et al., 2005; Mullaji et al., 2008). Such degeneration is consistent with the decrease in our current study’s tensile properties of the four knee joint ligaments.
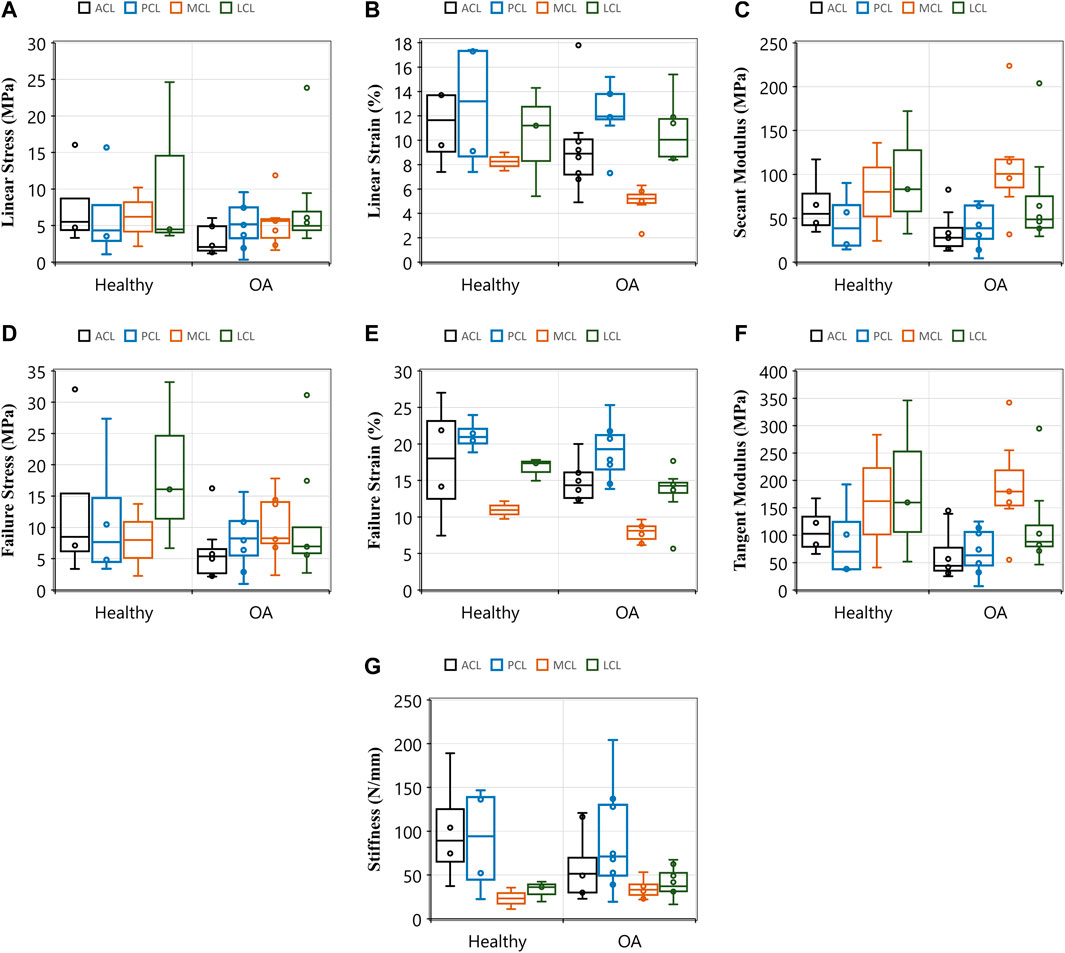
FIGURE 6. Comparisons of tensile properties of the anterior (ACL) and posterior (PCL) cruciate ligaments, and lateral (LCL) and medial (MCL) collateral ligaments between healthy and osteoarthritic (OA) groups. Healthy groups were defined by International Cartilage Repair Society (ICRS) grade 0 and osteoarthritic (OA) was defined by ICRS grade 1-4. (A) Linear stress and (B) linear strain were utilised to determine (C) secant modulus. (D) and (E) demonstrates the maximum stresses and strains that resulted in ligament failures. (F) This sub-figure shows tangent modulus of the ligaments between the healthy and OA groups at the maximum linear region of load-extension curves. (G) This sub-figure documents ligament stiffness values across ligaments and between the healthy and OA groups.
It is challenging to separate the effects of OA and ageing as they often happen concurrently. With only 12 cadavers and five groups of ICRS grades (0–4), it was challenging to statistically attribute changes in ligament tensile properties to both age and OA as related parameters, mainly when also accounting for sex (see further discussion below). However, trends were analysed from the data presented in Table 1 to understand the effect of age and OA as individual parameters. The trends suggest that the ACL and PCL material properties in younger donors were reduced in the OA knees compared to those in the healthy knees (Supplementary Figure S4). The findings imply that even mild OA in younger donors affects the material properties further exacerbated with advancing age and OA.
OA is believed to be a whole-joint disease impairing the integrity of associated tissues, including ligaments (Poole, 2012). In our previous study on the same human cadaveric knees, we found statistically significant correlations between changes in material properties of cartilage and subchondral bone with age and OA grade (Peters et al., 2018b). Similarly, the data in the current study for the same cadavers showed alterations in ligament tensile properties because of OA. Ligament degeneration or injury may occur in the first instance, leading to the initiation and progression of knee OA (Gianotti et al., 2009). Since the primary function of knee ligaments is to provide stability to the knee joint (Harner et al., 1995; Woo et al., 2006), any changes to the ligaments’ structure can alter the load distribution in the knee joint (Moore & Burris, 2015). The knee cadavers in this study showed that OA degeneration affected the medial more than the lateral compartments of the bones (Peters et al., 2018b). The difference in degeneration between the lateral and medial compartments of the knee joint could result from unbalanced load distribution caused by changes in the ligament material properties because of OA.
The reduction in the measured tensile parameters of the ACL during ageing and disease progression may be attributed to the relatively high forces experienced during walking. There is a consensus that peak force experienced by the ACL occurs at the contralateral toe-off during the stance phase of the gait cycle, up to 3.5 times body weight (Morrison, 1970; Collins & O’Connor, 1991; Shelburne et al., 2004). In particular, these high ACL kinematic forces may be consistent with the widely reported histological degeneration of the ACL in the presence of disease (Mullaji et al., 2008), suggesting high habitual forces could influence subsequent degeneration observed. The peak force of the PCL has also been reported to be 0.2 to 0.6 times body weight during walking (Morrison, 1970; Collins & O’Connor, 1991). Evidence shows that appropriate exercise training strengthens ligaments and knee joint mechanics (Tipton et al., 1975; Salem et al., 2000; Ng, 2002; Ferri et al., 2003). However, people exercise less as they age, increasing their risk of ligament degeneration (Daley & Spinks, 2000). Decreased capacity of the knee ligaments to resist motion due to reduced mechanical strength may alter joint contact forces, potentially causing increased loading on the medial femoral condyle and contributing to the preferential medial development of OA (Lohmander et al., 2007; Pelletier et al., 2007).
Further limitations of the current study, aside from a low sample number, include varying donor demographics, such as sex, which is known to affect tensile properties and the likelihood of knee ligament injury. It was found that ACLs in young human females had 22.49% lower Young’s modulus, and 8.3% and 14.3% lower failure strain and stress, respectively, compared to ACLs in young human males (Chandrashekar et al., 2006). These differences can be partially attributed to the physically smaller size of the female ACL, which can be linked to higher rates of ACL injuries in female athletes (Anderson et al., 2001; Chandrashekar, Slauterbeck & Hashemi, 2005). Human females are also at a greater risk of knee OA than their male counterparts (Hame & Alexander, 2013). Again, this study could not separate ligaments by sex for statistical analyses due to low sample numbers.
Finally, the current study may be limited by testing ligaments as whole bone-ligament-bone specimens along their loading axis. It has previously been acknowledged that ligaments may be best divided into fibre bundles to recruit fibres to their maximal potential and eliminate any slack due to orientation (Woo et al., 1991; Race & Amis, 1994). Significant differences have been reported between the anterior and posterior fibres of the ACL (Butler et al., 1992) and PCL (Race & Amis, 1994; Harner et al., 1995), suggesting that fibres play different roles in the stabilisation of the knee joint (Race & Amis, 1994); although ligaments naturally work as one functional unit. Such global approaches have been used to represent ligaments in finite element models as one functional unit (Readioff et al., 2020a). However, due to the lack of data on all four ligaments from the same donor (and in some instances, the same demographic or disease conditions of the donor) in the literature, material properties have often been applied globally in finite element models, where values for one ligament are replicated for all others (Blankevoort & Huiskes, 1991; Li, Lopez & Rubash, 2001; Kazemi & Li, 2014; Wang, Fan & Zhang, 2014). In some instances, tendon material properties have been used (Kazemi et al., 2011; Kazemi & Li, 2014; Wang, Fan & Zhang, 2014). Sensitivity analysis showed that varying intrinsic ligament material properties alter the internal and external rotation of the tibia-femoral joint, patella tilt and peak contact stress (Dhaher, Kwon & Barry, 2010). The data in this study, combined with cartilage and bone data in our previous study (Peters et al., 2018b), allows future research to apply a subject- or cohort-specific approach to computational modelling of the human knee joint to improve accuracy and predictive behaviour patterns of ligaments.
The knowledge of baseline material properties of all four ligaments from healthy donors can be used to replicate ligaments by developing more biologically representative synthetic materials for the repair and replacement following injury or degeneration (Yang, Rothrauff & Tuan, 2013; Ratcliffe et al., 2015). Future studies could investigate the effect of ageing and osteoarthritis on viscoelastic characteristics (creep and stress-relaxation) and biochemical composition (Kharaz et al., 2018) of these ligaments in the same knee. The data collected in this study provides insight into the healthy range for these parameters and how they change concurrently with surrounding ligaments during ageing and disease.
Conclusion
This research is the first to report material characteristics of the four major human knee ligaments from a diverse demographic such as healthy, aged, and osteoarthritic knees. We confirmed previous research findings that the ACL tensile properties decrease with age and OA. The results also showed that the PCL tangent and secant modulus decrease with increasing age. These data and our previously reported data on bone and cartilage material properties for the same cadavers support current research stating that OA is a whole-joint disease impairing many peri-articular tissues within the knee. The material properties of the four major knee ligaments in the twelve cadavers can be combined with their corresponding subchondral and trabecular bones and articular cartilage for future subject-specific applications, including the development of computational models.
Data availability statement
The original contributions presented in the study are included in the article/Supplementary Material, further inquiries can be directed to the corresponding authors.
Ethics statement
The studies involving human participants were reviewed and approved by the National Research Ethics Service (15/NS/0053). The patients/participants provided their written informed consent to participate in this study.
Author contributions
AP, KB, RA and EC Conceived and designed the experiments. AP Performed the experiments. RR, AP and BG analysed the data. RR, AP and BG prepared the figures and tables. All authors drafted the work or revised it critically for important content.
Funding
This project was funded by BBSRC (Research Grant: BB/J014516/1) and the School of Engineering, University of Liverpool.
Acknowledgments
The authors would like to thank Phil Jackson and the staff at the Newcastle Surgical Training Centre, who supported the obtainment of cadaveric specimens, and Lee Moore and the staff at the Veterinary Training Suite, Institute of Veterinary Science, University of Liverpool, for the use of surgical tools. We also thank Prof. Ahmed Elsheikh for providing access to the experimental facilities at the School of Engineering, University of Liverpool.
Conflict of interest
The authors declare that the research was conducted in the absence of any commercial or financial relationships that could be construed as a potential conflict of interest.
Publisher’s note
All claims expressed in this article are solely those of the authors and do not necessarily represent those of their affiliated organizations, or those of the publisher, the editors and the reviewers. Any product that may be evaluated in this article, or claim that may be made by its manufacturer, is not guaranteed or endorsed by the publisher.
Supplementary material
The Supplementary Material for this article can be found online at: https://www.frontiersin.org/articles/10.3389/fbioe.2022.954837/full#supplementary-material
References
Allain, J., Goutallier, D., and Voisin, M. C. (2001). Macroscopic and histological assessments of the cruciate ligaments in arthrosis of the knee. Acta Orthop. Scand. 72, 266–269. doi:10.1080/00016470152846592
Anderson, A. F., Dome, D. C., Gautam, S., Awh, M. H., and Rennirt, G. W. (2001). Correlation of anthropometric measurements, strength, anterior cruciate ligament size, and intercondylar notch characteristics to sex differences in anterior cruciate ligament tear rates. Am. J. Sports Med. 29, 58–66. doi:10.1177/03635465010290011501
Bersini, S., Sansone, V., and Frigo, C. A. (2016). A dynamic multibody model of the physiological knee to predict internal loads during movement in gravitational field. Comput. Methods Biomechanics Biomed. Eng. 19, 571–579. doi:10.1080/10255842.2015.1051972
Blankevoort, L., and Huiskes, R. (1991). Ligament-bone interaction in a three-dimensional model of the knee. J. Biomech. Eng. 113, 263–269. doi:10.1115/1.2894883
Bonner, T. J., Newell, N., Karunaratne, A., Pullen, A. D., Amis, A. A., and Masouros, S. D. (2015). Strain-rate sensitivity of the lateral collateral ligament of the knee. J. Mech. Behav. Biomed. Mater. 41, 261–270. doi:10.1016/j.jmbbm.2014.07.004
Butler, D. L., Guan, Y., Kay, M. D., Cummings, J. F., Feder, S. M., and Levy, M. S. (1992). Location-dependent variations in the material properties of the anterior cruciate ligament. J. Biomechanics 25, 511–518. doi:10.1016/0021-9290(92)90091-E
Chandrashekar, N., Mansouri, H., Slauterbeck, J., and Hashemi, J. (2006). Sex-based differences in the tensile properties of the human anterior cruciate ligament. J. Biomechanics 39, 2943–2950. doi:10.1016/j.jbiomech.2005.10.031
Chandrashekar, N., Slauterbeck, J., and Hashemi, J. (2005). Sex-based differences in the anthropometric characteristics of the anterior cruciate ligament and its relation to intercondylar notch geometry: A cadaveric study. Am. J. Sports Med. 33, 1492–1498. doi:10.1177/0363546504274149
Cho, H-J., and Kwak, D-S. (2020). Mechanical properties and characteristics of the anterolateral and collateral ligaments of the knee. Appl. Sci. 10, 6266. doi:10.3390/app10186266
Collins, J. J., and O’Connor, J. J. (1991). Muscle-ligament interactions at the knee during walking. Proc. Inst. Mech. Eng. H. 205, 11–18. doi:10.1243/PIME_PROC_1991_205_256_02
Comerford, E. J., Tarlton, J. F., Innes, J. F., Johnson, K. A., Amis, A. A., and Bailey, A. J. (2005). Metabolism and composition of the canine anterior cruciate ligament relate to differences in knee joint mechanics and predisposition to ligament rupture. J. Orthop. Res. 23, 61–66. doi:10.1016/j.orthres.2004.05.016
Cushner, F. D., La Rosa, D. F., Vigorita, V. J., Scuderi, G. R., Scott, W. N., and Insall, J. N. (2003). A quantitative histologic comparison: ACL degeneration in the osteoarthritic knee. J. Arthroplasty 18, 687–692. doi:10.1016/s0883-5403(03)00256-0
Daley, M. J., and Spinks, W. L. (2000). Exercise, mobility and aging. Sports Med. 29, 1–12. doi:10.2165/00007256-200029010-00001
Dhaher, Y. Y., Kwon, T-H., and Barry, M. (2010). The effect of connective tissue material uncertainties on knee joint mechanics under isolated loading conditions. J. Biomechanics 43, 3118–3125. doi:10.1016/j.jbiomech.2010.08.005
Ferri, A., Scaglioni, G., Pousson, M., Capodaglio, P., Van Hoecke, J., and Narici, M. V. (2003). Strength and power changes of the human plantar flexors and knee extensors in response to resistance training in old age. Acta Physiol. Scand. 177, 69–78. doi:10.1046/j.1365-201X.2003.01050.x
Gianotti, S. M., Marshall, S. W., Hume, P. A., and Bunt, L. (2009). Incidence of anterior cruciate ligament injury and other knee ligament injuries: A national population-based study. J. Sci. Med. Sport 12, 622–627. doi:10.1016/j.jsams.2008.07.005
Goodship, A. E., and Birch, H. L. (2005). Cross sectional area measurement of tendon and ligament in vitro: A simple, rapid, non-destructive technique. J. Biomechanics 38, 605–608. doi:10.1016/j.jbiomech.2004.05.003
Hame, S. L., and Alexander, R. A. (2013). Knee osteoarthritis in women. Curr. Rev. Musculoskelet. Med. 6, 182–187. doi:10.1007/s12178-013-9164-0
Harner, C. D., Xerogeanes, J. W., Livesay, G. A., Carlin, G. J., Smith, B. A., Kusayama, T., et al. (1995). The human posterior cruciate ligament complex: An interdisciplinary study. Ligament morphology and biomechanical evaluation. Am. J. Sports Med. 23, 736–745. doi:10.1177/036354659502300617
Hasegawa, A., Otsuki, S., Pauli, C., Miyaki, S., Patil, S., Steklov, N., et al. (2012). Anterior cruciate ligament changes in the human knee joint in aging and osteoarthritis. Arthritis Rheum. 64, 696–704. doi:10.1002/art.33417
Huang, H., Zhang, J., Sun, K., Zhang, X., and Tian, S. (2011). Effects of repetitive multiple freeze-thaw cycles on the biomechanical properties of human flexor digitorum superficialis and flexor pollicis longus tendons. Clin. Biomech. (Bristol, Avon) 26, 419–423. doi:10.1016/j.clinbiomech.2010.12.006
Jung, H-J., Vangipuram, G., Fisher, M. B., Yang, G., Hsu, S., Bianchi, J., et al. (2011). The effects of multiple freeze-thaw cycles on the biomechanical properties of the human bone-patellar tendon-bone allograft. J. Orthop. Res. 29, 1193–1198. doi:10.1002/jor.21373
Kazemi, M., and Li, L. P. (2014). A viscoelastic poromechanical model of the knee joint in large compression. Med. Eng. Phys. 36, 998–1006. doi:10.1016/j.medengphy.2014.04.004
Kazemi, M., Li, L. P., Savard, P., and Buschmann, M. D. (2011). Creep behavior of the intact and meniscectomy knee joints. J. Mech. Behav. Biomed. Mater. 4, 1351–1358. doi:10.1016/j.jmbbm.2011.05.004
Kharaz, Y. A., Canty‐Laird, E. G., Tew, S. R., and Comerford, E. J. (2018). Variations in internal structure, composition and protein distribution between intra- and extra-articular knee ligaments and tendons. J. Anat. 232, 943–955. doi:10.1111/joa.12802
Lee, G-C., Cushner, F. D., Vigoritta, V., Scuderi, G. R., Insall, J. N., and Scott, W. N. (2005). Evaluation of the anterior cruciate ligament integrity and degenerative arthritic patterns in patients undergoing total knee arthroplasty. J. Arthroplasty 20, 59–65. doi:10.1016/j.arth.2004.10.010
Li, G., Lopez, O., and Rubash, H. (2001). Variability of a three-dimensional finite element model constructed using magnetic resonance images of a knee for joint contact stress analysis. J. Biomech. Eng. 123, 341–346. doi:10.1115/1.1385841
Liu, A., Sanderson, W. J., Ingham, E., Fisher, J., and Jennings, L. M. (2020). Development of a specimen-specific in vitro pre-clinical simulation model of the human cadaveric knee with appropriate soft tissue constraints. PLOS ONE 15, e0238785. doi:10.1371/journal.pone.0238785
Lohmander, L. S., Englund, P. M., Dahl, L. L., and Roos, E. M. (2007). The long-term consequence of anterior cruciate ligament and meniscus injuries: Osteoarthritis. Am. J. Sports Med. 35, 1756–1769. doi:10.1177/0363546507307396
Manninen, P., Riihimäki, H., Heliövaara, M., and Mäkelä, P. (1996). Overweight, gender and knee osteoarthritis. Int. J. Obes. Relat. Metab. Disord. 20, 595–597.
Momersteeg, T. J., Blankevoort, L., Huiskes, R., Kooloos, J. G., Kauer, J. M., and Hendriks, J. C. (1995). The effect of variable relative insertion orientation of human knee bone-ligament-bone complexes on the tensile stiffness. J. Biomechanics 28, 745–752. doi:10.1016/0021-9290(94)00121-j
Moon, D. K., Woo, S. L. Y., Takakura, Y., Gabriel, M. T., and Abramowitch, S. D. (2006). The effects of refreezing on the viscoelastic and tensile properties of ligaments. J. Biomechanics 39, 1153–1157. doi:10.1016/j.jbiomech.2005.02.012
Moore, A., and Burris, D. L. (2015). Tribological and material properties for cartilage of and throughout the bovine stifle: Support for the altered joint kinematics hypothesis of osteoarthritis. Osteoarthr. Cartil. 23, 161–169. doi:10.1016/j.joca.2014.09.021
Morrison, J. B. (1970). The mechanics of the knee joint in relation to normal walking. J. Biomechanics 3, 51–61. doi:10.1016/0021-9290(70)90050-3
Mullaji, A. B., Marawar, S. V., Simha, M., and Jindal, G. (2008). Cruciate ligaments in arthritic knees: A histologic study with radiologic correlation. J. Arthroplasty 23, 567–572. doi:10.1016/j.arth.2007.05.024
Nawata, K., Enokida, M., Yamasaki, D., Minamizaki, T., Hagino, H., Morio, Y., et al. (2001). Tensile properties of rat anterior cruciate ligament in collagen induced arthritis. Ann. Rheum. Dis. 60, 395–398. doi:10.1136/ard.60.4.395
Nelissen, R. G., and Hogendoorn, P. C. (2001). Retain or sacrifice the posterior cruciate ligament in total knee arthroplasty? A histopathological study of the cruciate ligament in osteoarthritic and rheumatoid disease. J. Clin. Pathology 54, 381–384. doi:10.1136/jcp.54.5.381
Ng, G. Y. F. (2002). Ligament injury and repair: Current concepts. Hong Kong Physiother. J. 20, 22–29. doi:10.1016/S1013-7025(09)70028-4
Noyes, F. R., and Grood, E. S. (1976). The strength of the anterior cruciate ligament in humans and Rhesus monkeys. J. Bone Jt. Surg. 58, 1074–1082. doi:10.2106/00004623-197658080-00006
Patel, R. M., Castile, R. M., Jenkins, M. J., Lake, S. P., and Brophy, R. H. (2021). Microstructural and mechanical properties of the anterolateral ligament of the knee. Am. J. Sports Med. 49, 172–182. doi:10.1177/0363546520974381
Pelletier, J-P., Raynauld, J-P., Berthiaume, M-J., Abram, F., Choquette, D., Haraoui, B., et al. (2007). Risk factors associated with the loss of cartilage volume on weight-bearing areas in knee osteoarthritis patients assessed by quantitative magnetic resonance imaging: A longitudinal study. Arthritis Res. Ther. 9, R74. doi:10.1186/ar2272
Peters, A. E., Akhtar, R., Comerford, E. J., and Bates, K. T. (2018b). The effect of ageing and osteoarthritis on the mechanical properties of cartilage and bone in the human knee joint. Sci. Rep. 8, 5931. doi:10.1038/s41598-018-24258-6
Peters, A. E., Akhtar, R., Comerford, E. J., and Bates, K. T. (2018a). Tissue material properties and computational modelling of the human tibiofemoral joint: A critical review. PeerJ 6, e4298. doi:10.7717/peerj.4298
Peters, A. E., Comerford, E. J., Macaulay, S., Bates, K. T., and Akhtar, R. (2017). Micromechanical properties of canine femoral articular cartilage following multiple freeze-thaw cycles. J. Mech. Behav. Biomed. Mater. 71, 114–121. doi:10.1016/j.jmbbm.2017.03.006
Poole, A. R. (2012). Osteoarthritis as a whole joint disease. HSS Jrnl. 8, 4–6. doi:10.1007/s11420-011-9248-6
Race, A., and Amis, A. A. (1994). The mechanical properties of the two bundles of the human posterior cruciate ligament. J. Biomechanics 27, 13–24. doi:10.1016/0021-9290(94)90028-0
Ratcliffe, A., Butler, D. L., Dyment, N. A., Cagle, P. J., Proctor, C. S., Ratcliffe, S. S., et al. (2015). Scaffolds for tendon and ligament repair and regeneration. Ann. Biomed. Eng. 43, 819–831. doi:10.1007/s10439-015-1263-1
Readioff, R., Geraghty, B., Comerford, E., and Elsheikh, A. (2020a). A full-field 3D digital image correlation and modelling technique to characterise anterior cruciate ligament mechanics ex vivo. Acta Biomater. 113, 417–428. doi:10.1016/j.actbio.2020.07.003
Readioff, R., Geraghty, B., Elsheikh, A., and Comerford, E. (2020b). Viscoelastic characteristics of the canine cranial cruciate ligament complex at slow strain rates. PeerJ 8, e10635. doi:10.7717/peerj.10635
Robinson, J. R., Bull, A. M. J., and Amis, A. A. (2005). Structural properties of the medial collateral ligament complex of the human knee. J. Biomechanics 38, 1067–1074. doi:10.1016/j.jbiomech.2004.05.034
Rousseau, J. C., and Garnero, P. (2012). Biological markers in osteoarthritis. Bone 51, 265–277. doi:10.1016/j.bone.2012.04.001
Salem, G. J., Wang, M. Y., Young, J. T., Marion, M., and Greendale, G. A. (2000). Knee strength and lower- and higher-intensity functional performance in older adults. Med. Sci. Sports Exerc. 32, 1679–1684. doi:10.1097/00005768-200010000-00003
Schneider, C. A., Rasband, W. S., and Eliceiri, K. W. (2012). NIH image to Image J: 25 years of image analysis. Nat. Methods 9, 671–675. doi:10.1038/nmeth.2089
Sharma, A., Leszko, F., Komistek, R. D., Scuderi, G. R., Cates, H. E., and Liu, F. (2008). In vivo patellofemoral forces in high flexion total knee arthroplasty. J. Biomechanics 41, 642–648. doi:10.1016/j.jbiomech.2007.09.027
Shelburne, K. B., Pandy, M. G., Anderson, F. C., and Torry, M. R. (2004). Pattern of anterior cruciate ligament force in normal walking. J. Biomechanics 37, 797–805. doi:10.1016/j.jbiomech.2003.10.010
Simon, D., Mascarenhas, R., Saltzman, B. M., Rollins, M., Bach, B. R., and MacDonald, P. (2015). The relationship between anterior cruciate ligament injury and osteoarthritis of the knee. Adv. Orthop. 2015, e928301–11. doi:10.1155/2015/928301
Smeets, K., Slane, J., Scheys, L., Claes, S., and Bellemans, J. (2017). Mechanical analysis of extra-articular knee ligaments. Part One: Native knee ligaments. Knee 24, 949–956. doi:10.1016/j.knee.2017.07.013
Stubbs, G., Dahlstrom, J., Papantoniou, P., and Cherian, M. (2005). Correlation between macroscopic changes of arthrosis and the posterior cruciate ligament histology in the osteoarthritic knee. ANZ J. Surg. 75, 1036–1040. doi:10.1111/j.1445-2197.2005.03610.x
Tipton, C. M., Matthes, R. D., Maynard, J. A., and Carey, R. A. (1975). The influence of physical activity on ligaments and tendons. Med. Sci. Sports Exerc. 7, 165–175. doi:10.1249/00005768-197500730-00014
Trent, P. S., Walker, P. S., and Wolf, B. (1976). Ligament length patterns, strength, and rotational axes of the knee joint. Clin. Orthop. Relat. Res., 263–270. doi:10.1097/00003086-197606000-00034
van Dommelen, J. A. W., Ivarsson, B. J., Jolandan, M. M., Millington, S. A., Raut, M., Kerrigan, J. R., et al. (2005). Characterization of the rate-dependent mechanical properties and failure of human knee ligaments. SAE Trans. 114, 80–90.
Wang, Y., Fan, Y., and Zhang, M. (2014). Comparison of stress on knee cartilage during kneeling and standing using finite element models. Med. Eng. Phys. 36, 439–447. doi:10.1016/j.medengphy.2014.01.004
Watanabe, A., Kanamori, A., Ikeda, K., and Ochiai, N. (2011). Histological evaluation and comparison of the anteromedial and posterolateral bundle of the human anterior cruciate ligament of the osteoarthritic knee joint. Knee 18, 47–50. doi:10.1016/j.knee.2009.12.009
Woo, S. L-Y., Abramowitch, S. D., Kilger, R., and Liang, R. (2006). Biomechanics of knee ligaments: Injury, healing, and repair. J. Biomechanics 39, 1–20. doi:10.1016/j.jbiomech.2004.10.025
Woo, S. L. Y., Hollis, J. M., Adams, D. J., Lyon, R. M., and Takai, S. (1991). Tensile properties of the human femur-anterior cruciate ligament-tibia complex. The effects of specimen age and orientation. Am. J. Sports Med. 19, 217–225. doi:10.1177/036354659101900303
Woo, S. L. Y., Orlando, C. A., Camp, J. F., and Akeson, W. H. (1986). Effects of postmortem storage by freezing on ligament tensile behavior. J. Biomechanics 19, 399–404. doi:10.1016/0021-9290(86)90016-3
Keywords: human knee ligament, material characterisation, knee osteoarthitis, anterior cruciate ligament, posterior cruciate ligament, lateral collateral ligament, medial collateral ligament, ageing
Citation: Peters AE, Geraghty B, Bates KT, Akhtar R, Readioff R and Comerford E (2022) Ligament mechanics of ageing and osteoarthritic human knees. Front. Bioeng. Biotechnol. 10:954837. doi: 10.3389/fbioe.2022.954837
Received: 27 May 2022; Accepted: 25 July 2022;
Published: 23 August 2022.
Edited by:
Nicola Francesco Lopomo, University of Brescia, ItalyReviewed by:
Gregorio Marchiori, Rizzoli Orthopedic Institute (IRCCS), ItalyMatteo Berni, Rizzoli Orthopedic Institute (IRCCS), Italy
Copyright © 2022 Peters, Geraghty, Bates, Akhtar, Readioff and Comerford. This is an open-access article distributed under the terms of the Creative Commons Attribution License (CC BY). The use, distribution or reproduction in other forums is permitted, provided the original author(s) and the copyright owner(s) are credited and that the original publication in this journal is cited, in accordance with accepted academic practice. No use, distribution or reproduction is permitted which does not comply with these terms.
*Correspondence: Eithne Comerford, RWl0aG5lLkNvbWVyZm9yZEBsaXZlcnBvb2wuYWMudWs=; Rosti Readioff, ci5yZWFkaW9mZkBsZWVkcy5hYy51aw==