- 1Insect Pest Control Laboratory, Joint FAO/IAEA Centre of Nuclear Techniques in Food and Agriculture, International Atomic Energy Agency, Vienna, Austria
- 2Unité de Formation et de Recherche en Sciences et Technologies (UFR/ST), Université Norbert ZONGO (UNZ), Koudougou, Burkina Faso
- 3Institut de Recherche en Sciences de la Santé/Direction Régionale de l’Ouest (IRSS/DRO), Bobo-Dioulasso, Burkina Faso
- 4Institut de Recherche Agricole pour le Développement (IRAD), Yaoundé-Messa, Cameroun
The developmental stage of the mosquito is one of the main factors that affect its response to ionizing radiation. Irradiation of adults has been reported to have beneficial effects. However, the main challenge is to immobilize and compact a large number of adult male mosquitoes for homogenous irradiation with minimal deleterious effects on their quality. The present study investigates the use of nitrogen in the irradiation of adult Aedes albopictus and Ae. aegypti. Irradiation in nitrogen (N2) and in air after being treated with nitrogen (PreN2) were compared with irradiation in air at gamma radiation doses of 0, 55, 70, 90, 110, and 125 Gy. In both species, approximately 0% egg hatch rate was observed following doses above 55 Gy in air versus 70 Gy in PreN2 and 90 Gy in N2. Males irradiated at a high mosquito density showed similar egg hatch rates as those irradiated at a low density. Nitrogen treatments showed beneficial effects on the longevity of irradiated males for a given dose, revealing the radioprotective effect of anoxia. However, irradiation in N2 or PreN2 slightly reduced the male flight ability. Nitrogen treatment was found to be a reliable method for adult mosquito immobilization. Overall, our results demonstrated that nitrogen may be useful in adult Aedes mass irradiation. The best option seems to be PreN2 since it reduces the immobilization duration and requires a lower dose than that required in the N2 environment to achieve full sterility but with similar effects on male quality. However, further studies are necessary to develop standardized procedures including containers, time and pressure for flushing with nitrogen, immobilization duration considering mosquito species, age, and density.
1 Introduction
The sterile insect technique (SIT), a species-specific and environment-friendly method, is a promising technique for the area-wide integrated management of vector mosquitoes, which cause diseases such as dengue, yellow fever, and Zika (Dyck et al., 2021). Indeed, many field trials to demonstrate the effectiveness of the SIT against Aedes aegypti and Aedes albopictus have shown satisfactory results on a small scale (Bellini et al., 2013, 2021; Gato et al., 2021; Becker et al., 2022). Although significant advances have been made in its development (Culbert et al., 2018; Bimbilé Somda et al., 2019; Mamai et al., 2019; Mamai et al., 2020; Yamada et al., 2019; Bouyer et al., 2020; Maïga et al., 2020), there is considerable scope for further development, especially for large-scale implementation. The SIT package includes mosquito mass rearing, sex separation, male sterilization, transport, and release (Dyck et al., 2021). Sterilizing large numbers of males in a reliable manner while maintaining their quality remains one of the most challenging steps. Chemosterilization (Patterson et al., 1970), ionizing radiation (Patterson et al., 1975), and genetic manipulations (Catteruccia et al., 2009) are the main methods explored for mosquito sterilization. However, because of limited effectiveness or ethical, health, and environmental concerns, ionizing radiation, which does not release residues that could be harmful to human health or the environment (Helinski et al., 2009), is the most used method in current SIT programs. Gamma radiation from a 60Co or 137Cs source and X-ray radiation are commonly used because of their high energy and penetration (Helinski et al., 2009). However, the overall response of mosquitoes to radiation, as with all biological material, has been shown to be affected by many critical factors, including the radiation source, dose rate, dose amount, environment during irradiation, mosquito species, strain, life stage, and handling procedures (Helinski et al., 2009; Yamada et al., 2019; Yamada et al., 2020). For example, irradiation of eggs or larvae causes a high mortality rate even at low irradiation doses and cannot be considered for the SIT programs (Wakid et al., 1976). The pupal and adult stages were found to be more eligible for irradiation (Helinski et al., 2006; Helinski et al., 2009). However, optimal pupae irradiation has many requirements: synchronized pupal production is needed for collection in a small age range, and generally, older pupae (>36 h) should be used to avoid variability in induced sterility. Indeed, younger pupae are more radiosensitive than older pupae, and irradiating young pupae also results in increased somatic damage and mortality (Yamada et al., 2019). Larger numbers of pupae also need to either be submerged in water to allow compacting the pupae in a small container without crushing those at the bottom (note that the pupae would be subjected to hypoxia) or undergo relaxed compaction (single layers of pupae) to avoid crushing, but where pockets of hypoxia can form in which a subset of the pupae may be underdosed (Yamada et al., 2020). These conditions are not easy to achieve or reproduce, especially for mass irradiation. While efforts are still being devoted to overcome these difficulties, the focus is now shifting to irradiation during the adult stage. It has been reported that adult Aedes mosquitoes are similarly or slightly more radiosensitive than old pupae with a better quality after irradiation in some cases (Du et al., 2019; Ernawan et al., 2022). However, the main challenge is the immobilization and compaction of a large number of adult males for homogenous irradiation with minimal deleterious effects on their downstream quality. In general, cold temperatures are used to knock down the mosquitoes (Zhang et al., 2020; Ernawan et al., 2022). However, the quality of the sterile males can be compromised if the chilling temperatures and duration are not carefully controlled. Indeed, many studies on the effects of chilling on insect quality have reported negative effects (reviewed in Yamada et al., 2022). Aiming for a more reliable outcome regarding quality following adult mosquito irradiation, the role of nitrogen has been investigated (Helinski et al., 2009). Beneficial effects of the use of nitrogen in insect irradiation have been reported in fruit flies (Fisher, 1996) and tsetse flies (Vreysen, 1995; Mutika and Parker, 2006). However, its impact on mosquitoes is debated. Indeed, tests performed on Anopheles gambiae pupae and Culex quinquefasciatus pupae and adults showed no beneficial effect (Curtis, 1976; El-Gazzar et al., 1983). In contrast, the irradiation of adult male Ae. aegypti in nitrogen resulted in better competitiveness compared with irradiation in air, although higher doses were required to achieve full sterility (Hallinan and Rai, 1973). A recent study (Yamada et al., 2022) involving Ae. albopictus also showed a radioprotective effect of nitrogen at 45 Gy.
The present study aims to further investigate the possibility to use nitrogen in the mass irradiation of adult Ae. albopictus and Ae. aegypti mosquitoes in order to optimize the effectiveness of the SIT. Two nitrogen treatments were assessed in comparison to irradiation in air. The irradiation dose–response curves under these nitrogen treatments were determined considering gamma radiation doses of 0–125 Gy at low and high mosquito densities. The effects on male flight ability and longevity were further evaluated. A preliminary study was carried out to determine knock-down and wake-up times of adult male Aedes mosquitoes following their exposure to nitrogen.
2 Materials and methods
2.1 Mosquito strains
Experiments were carried out on Ae. aegypti and Ae. albopictus species. The strains used were maintained at the Insect Pest Control Laboratory (IPCL) of the joint FAO/IAEA Centre of Nuclear Techniques in Food and Agriculture, Seibersdorf, Austria, under rearing protocols developed at the IPCL (FAO/IAEA, 2020b).
2.2 Determination of the response of adult male Aedes albopictus following exposure to nitrogen
A preliminary study was carried out to determine the time to the first stand-up and the first flight of adult male Aedes mosquitoes following increasing durations of immobilization with di-nitrogen (N2) gas. Eight immobilization durations were tested: 1, 5, 10, 20, 30, 40, 50, and 60 min. The mosquitoes were placed in N2 in gas-tight headspace vials (20 ml) with screw tops with PTFE/silicone septa (Merck KGaA, Darmstadt, Germany). Five adult Ae. albopictus males, aged 1–2 days old, were transferred to each vial. After the mosquitoes were transferred, the vials were closed with the screw tops, and the tops were sealed with PTFE Thread Seal Tape (Sigma-Aldrich, USA) before flushing with N2, as described by Yamada et al. (2022). To flush N2 in the vial, two syringe needles were inserted from the top. One served to add the N2 and the other served as an outlet for the air. Following exposure to N2, the mosquitoes from each vial were released into an individual BugDorm cage (15 cm3 × 15 cm3 × 15 cm3) (MegaView Science Co. Ltd., Taichung 40762, Taiwan). The times between the release and the first mosquito to stand up as well as the first mosquito to fly were recorded. Three biological replicates with three technical replicates were performed for all exposure durations.
2.3 Irradiation source and dosimetry
Irradiation was performed in a 60Co gamma irradiator, Gammacell 220 (Nordion Ltd., Kanata, Ontario, Canada), which had a dose rate of 65 Gy/min during the experiments. Gafchromic HD-V2 film (Ashland Advanced Materials, Bridgewater NJ, United States) was used to verify the dose received by the mosquitoes (IAEA, 2004). Three pieces of HD film were individually packed in small (2 cm × 2 cm) paper envelopes and placed in the same position as the mosquito samples in the irradiation container. The films were read using an optical density reader (DoseReader 4, RadGen, H-1118 Budapest, Sasadi út 36, Hungary) after 24 h of development.
2.4 Irradiation doses and environments
Mosquitoes were exposed to six irradiation doses, 0, 55, 70, 90, 110, and 125 Gy, in three environments, air, nitrogen, and pretreated with nitrogen. For irradiation in air, the mosquitoes were kept in vials in the ambient atmosphere. For irradiation in nitrogen (N2), the air in the vial was replaced with N2 gas. The immobilization duration in the nitrogen was 15–20 min, including the irradiation exposure duration. The pretreated with nitrogen (Pre.Nitrogen or PreN2) treatment involved immobilizing the mosquitoes with nitrogen for 10–15 min and then replacing the nitrogen with air by keeping the vial open for 20–30 s prior to irradiation.
Two- to three-day-old virgin male Aedes were knocked down in a cold room (6°C) for 10–15 min and then transferred into gas-tight headspace vials (20 ml) with screw tops with PTFE/silicone septa (Merck KGaA, Darmstadt, Germany) for irradiation. These knockdown conditions are known to have no impact on male quality (Culbert et al., 2019). For irradiation in air, the vials were only covered with a piece of mosquito bed-net instead of the normal screw top. For both types of nitrogen treatments, the vials containing the mosquitoes were closed with screw tops and the tops were sealed with PTFE Thread Seal Tape (Sigma-Aldrich, USA) before filling with N2. N2 was flushed in the vial as described above.
2.5 Determination of the dose–response curves following irradiation of low-density adult Aedes under different nitrogen environments
The dose–response curves were determined for both Aedes species at low-density irradiation considering the six doses and three environments. Each vial contained 20 males. After irradiation, the mosquitoes from each vial were immediately transferred to a BugDorm cage (15 cm3 × 15 cm3 × 15 cm3) (MegaView Science Co. Ltd., Taichung 40762, Taiwan) containing 20 virgin females from the same male collection cohort. Two consecutive blood meals were offered to the females on days 3 and 4 following the irradiation day. Oviposition cups were placed inside the cages on day 5 and were collected on day 10. Eggs were allowed to mature and were stored following the IAEA guidelines (FAO/IAEA, 2020a). On day 10 following egg collection, eggs were allowed to hatch for over 48 h in boiled and cooled (deoxygenated) water with a pinch of larval food (for Ae. aegypti) or in a hatching solution containing nutrient broth and brewer’s yeast (for Ae. albopictus) (FAO/IAEA, 2020a). To determine their hatching status, eggs were checked under a binocular microscope and counted as either hatched or nonhatched. The egg hatch rate was determined as the percentage of hatched eggs based on the total number of eggs checked. Two biological replicates with two technical replicates each were performed for each treatment and each Aedes species.
2.6 Determination of the effects of irradiation of adult male Aedes at high density in different nitrogen environments on egg hatch rate and male longevity
Both Aedes species were irradiated at a high mosquito density considering the six radiation doses and three environments. The number of males in the vial was increased to 1000–1200.
2.6.1 Determination of the effect on egg hatch rate
From the batches of mosquitoes irradiated at a high density, 50 males were randomly sampled from each vial and were transferred to a BugDorm cage (15 cm3 × 15 cm3 × 15 cm3) containing 50 virgin females from the same cohort of pupal collection. Three replicates were performed for all the treatments. Two consecutive blood meals were offered to the females on days 3 and 4 following the day the males were added to the cages. Egg collection, storage, and hatching were performed as described in the experiment above. However, to determine the egg hatch rate, the hatching status of 100 eggs randomly selected from each replicate was checked under a binocular microscope.
2.6.2 Determination of the effect on male survival
Longevity was measured for males that were allowed to mate with females (50 males + 50 females) for the hatch rate determinations. Male mortality was recorded daily, except on weekends, from the day the male mosquitoes were added to the cages with females (irradiation day) until day 21 postemergence. Three replicates were performed for each treatment and each mosquito species.
2.6.3 Determination of the effect on male flight ability
After the high-density irradiation, males from each vial were transferred to a 30 cm3 × 30 cm3 × 30 cm3 BugDorm cage and were allowed to recover for 1 day prior to the flight test. A sugar solution (10%) was provided. Flight ability tests were performed following the methods developed by Culbert et al. (2018). Three replicates were performed for each treatment and each species. For each replicate, approximately 100 males were randomly sampled and introduced into the flight test device. After 2 h, the numbers of escapees and nonescapees were recorded. The flight ability for each replicate was determined as the percentage of escapees based on the total number of males introduced into the flight test device.
2.7 Statistical analysis
Statistical analyses were performed using R software (version 4.1.2) (Chambers, 2008). Data were analyzed by mosquito species. The irradiation environment and dose (considering dose, log10 (dose), or log10 (dose +1)) were considered as explanatory variables. The egg hatch rate and flight ability were analyzed using binomial generalized linear mixed models fit by maximum likelihood (Laplace approximation) (Bolker et al., 2009), with the proportion of hatched eggs and the proportion of escaped males as response variables and the replicates as random effects. Male longevity was analyzed using mixed-effects Cox regression models with irradiation dose and the environment as explanatory variables. The best models were selected based on the lowest value of Akaike information criterion (Hurvich and Tsai, 1995; Burnham and Anderson, 1998). The response variables, time to the first stand-up and first flight, were analyzed using linear mixed-effect models with immobilization duration as the explanatory variable and the replicate as random effects.
3 Results
3.1 Response of adult male Aedes albopictus following exposure to nitrogen
The knockdown of adult males occurred almost immediately after the replacement of air by N2 in the vial. The males were completely immobilized in the N2 atmosphere, and their legs were stretched out straight, as shown in Figure 1.
The time for the male mosquitoes to stand up (Figure 2A) or fly (Figure 2B) ranged between 1 and 84 min and significantly increased with the immobilization duration (Table 1). In addition, larger variances among replicates were observed with longer immobilization durations (Figure 2).
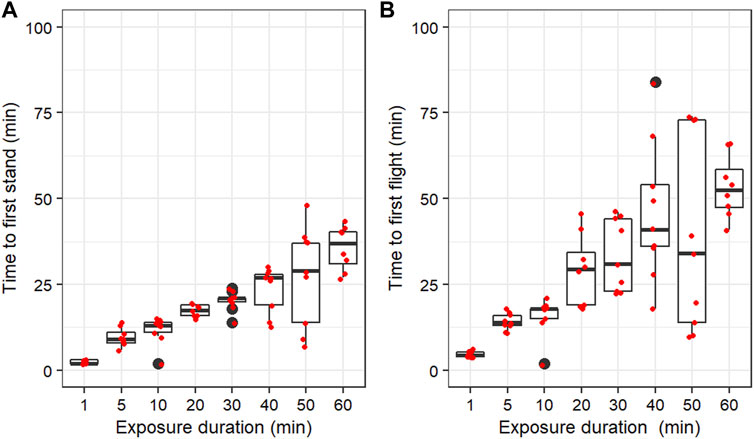
FIGURE 2. Time to first stand-up (A) and first flight (B) following exposure to nitrogen. Boxplots present the median values and quartiles, horizontal bars the 95% percentiles, black dots the minimal and maximal values, and red dots the replicate values.

TABLE 1. Fixed-effects coefficients of linear mixed-effect models of the impact of immobilization duration of male mosquitoes with nitrogen on the time to first stand-up and first flight in Aedes albopictus.
3.2 Dose–response curves of Aedes following irradiation of low-density adult males in different nitrogen environments
The dose–response curves of Ae. aegypti and Ae. albopictus are presented in Figures 3A and B, respectively. Overall, the irradiation dose and environment had significant effects on the egg hatch rate (Table 2). In the absence of irradiation, the egg hatch rates in the three environments (air, PreN2, N2) were above 98% in Ae. aegypti and above 85% in Ae. albopictus. The PreN2 and N2 treatments significantly decreased the radiosensitivity, with a higher effect observed with N2. An approximate of 0% egg hatch rate was reached with doses above 55 Gy in air, 70 Gy in PreN2, and 90 Gy in N2 in both species. However, full sterility was observed in all irradiation environments with doses of 110 and 120 Gy.
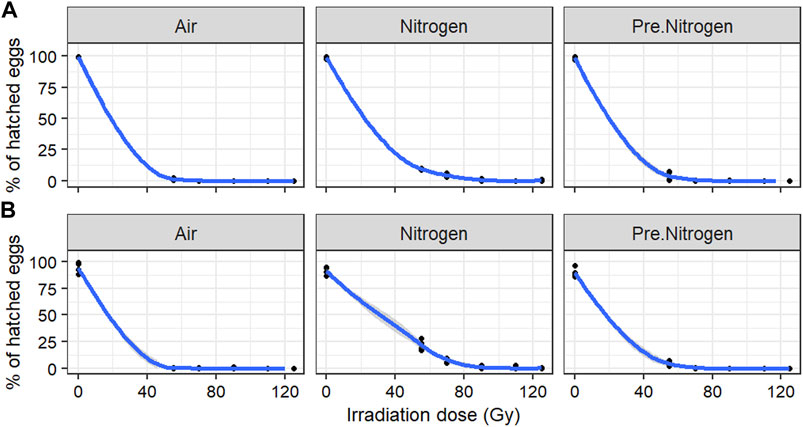
FIGURE 3. Egg hatch rate in Aedes aegypti (A) and Aedes albopictus (B) following irradiation of adult males at low density in different nitrogen environments.
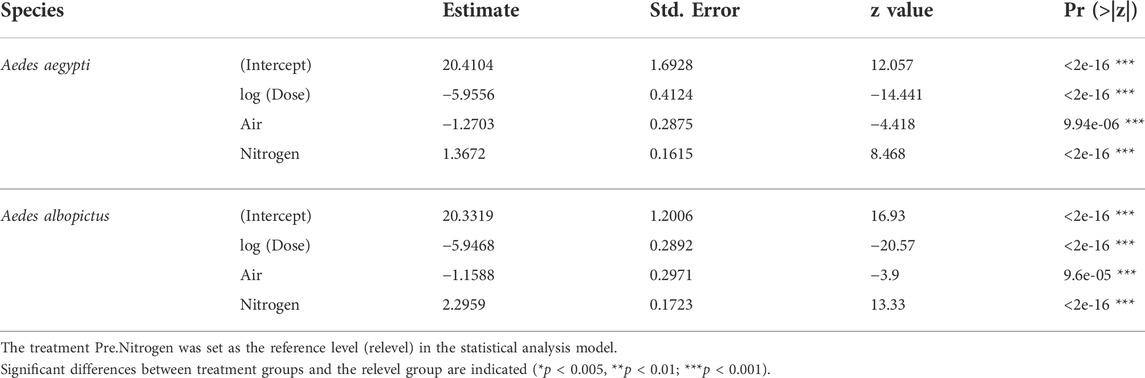
TABLE 2. Fixed-effects coefficients of binomial generalized linear mixed-effect models of the impact of irradiation dose and environment on the egg hatch rate in Aedes aegypti and Aedes albopictus following irradiation of adult males at low density.
3.3 Effect of irradiation of adult male Aedes at high density in different nitrogen environments on egg hatch rate and male survival
3.3.1 Effect on egg hatch rate
Figures 4A and B present the dose–response curves from high-density irradiation for Ae. aegypti and Ae. albopictus, respectively. The egg hatch rates showed the same trend as that observed in the low-density irradiation in each species, although a slight increase was recorded in the PreN2 and N2 treatments at doses of 55, 70, and 90 Gy (Figure 4; Table 3). In both species, approximately 0% egg hatch rate was reached with doses above 55 Gy in air, 70 Gy in PreN2, and 90 Gy in N2.
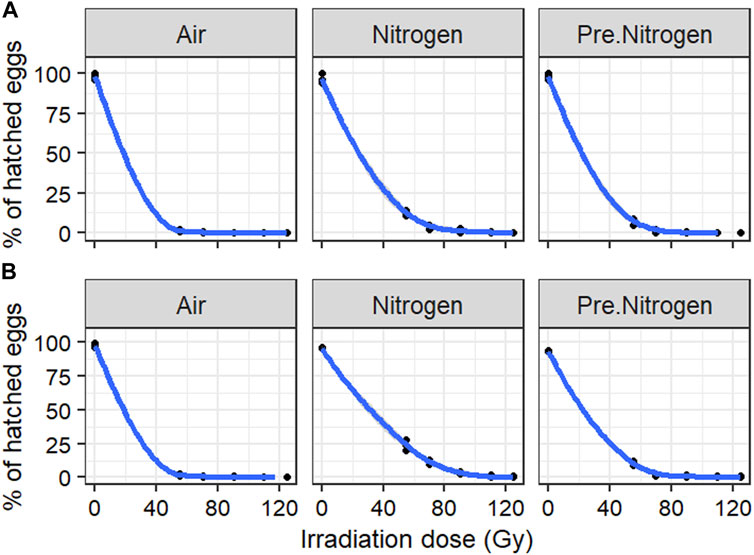
FIGURE 4. Egg hatch rate in Aedes aegypti (A) and Aedes albopictus (B) following irradiation of adult males at high density in different nitrogen environments.
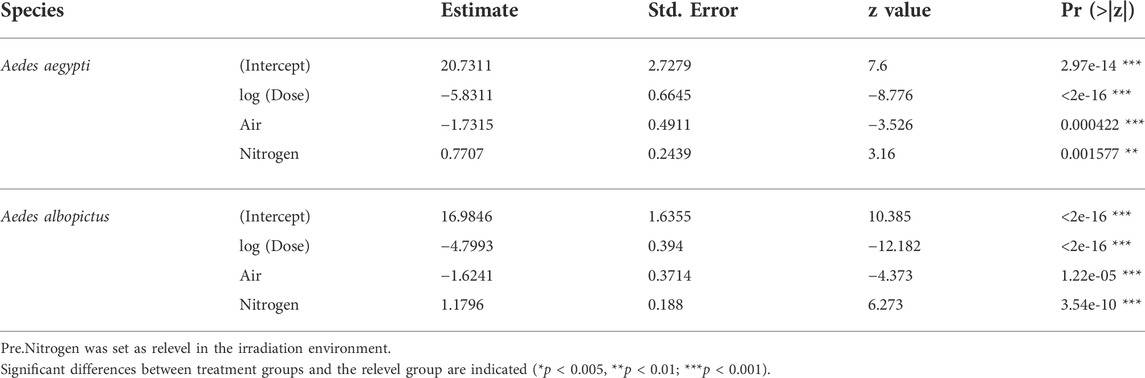
TABLE 3. Fixed-effects coefficients of binomial generalized linear mixed-effect models of the impact of irradiation dose and environment on egg hatch rate in Aedes aegypti and Aedes albopictus following irradiation of adult males at high density.
3.3.2 Effect on male flight ability
Overall, the male flight ability (percentage of escapees) ranged between 80% and 100% for Ae. aegypti (Figure 5A) and between 55% and 85% for Ae. albopictus (Figure 5B). In both species, irradiation significantly reduced the male flight ability independently from the irradiation environment. In addition, irradiation in air showed better male flight ability than the PreN2 and N2 treatments regardless of the dose. The two nitrogen treatments had similar effects (Table 4). However, the relative differences observed in the male flight ability between the doses inducing nearly full sterility in air (55%) and in PreN2 (70%) and N2 (90%) treatments were only approximately 10%.
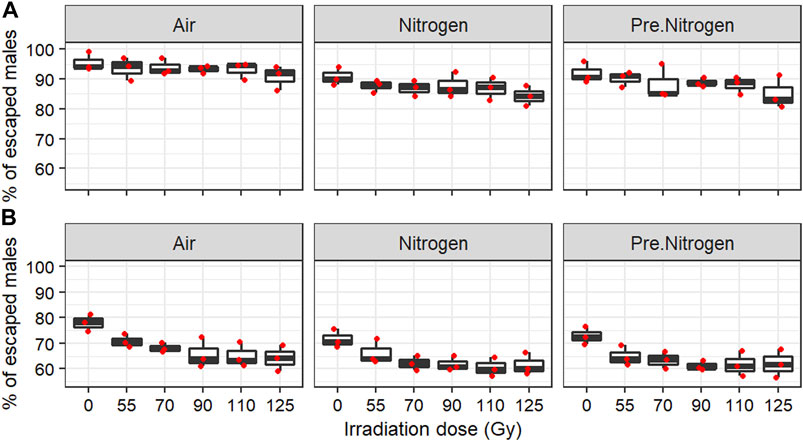
FIGURE 5. Flight ability of Aedes aegypti (A) and Aedes albopictus (B) following irradiation of adult males at high density in different nitrogen environments. Boxplots present the median values and quartiles, horizontal bars the 95% percentiles, black dots the minimal and maximal values, and red dots the replicate values.
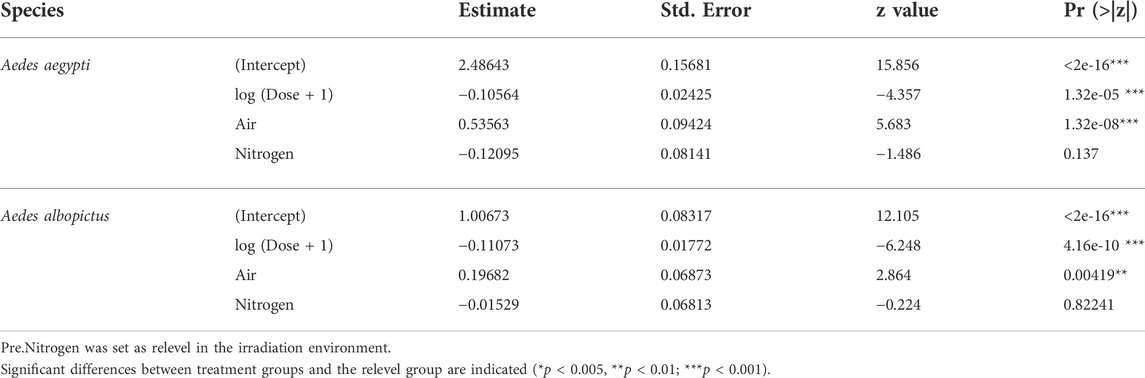
TABLE 4. Fixed-effects coefficients of binomial generalized linear mixed-effect models of the impact of irradiation dose and environment on male flight ability in Aedes aegypti and Aedes albopictus following irradiation of adult males at high density.
3.3.3 Effect on male survival
In both species, the survivorship was above 90% on day 10 and above 70% on day 15 in all treatments (Figures 6, 7). In addition, the PreN2 and N2 treatments had similar positive effects on survival compared with irradiation in air (Figures 6, 7; Table 5), except for the PreN2 treatment in Ae. aegypti, which did not have a significant impact on longevity.
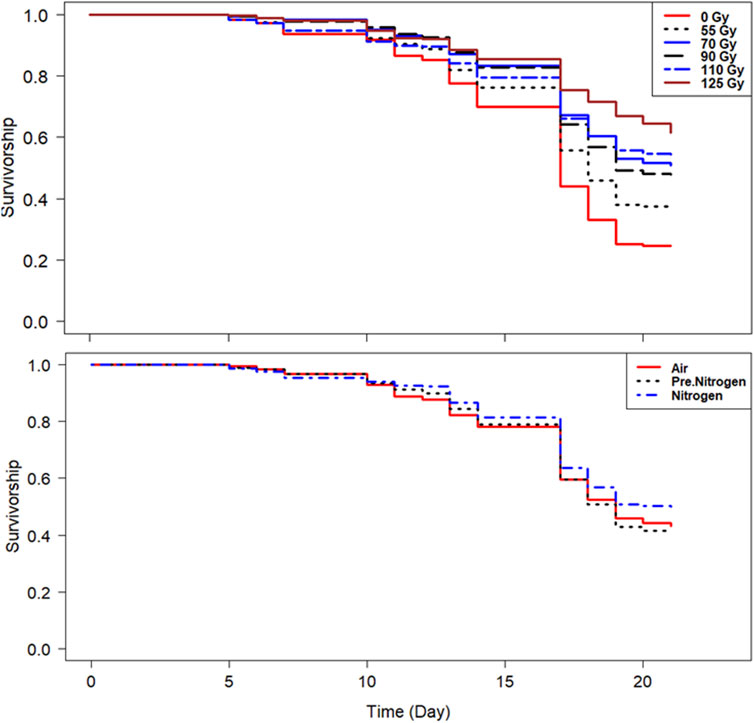
FIGURE 6. Survival of Aedes aegypti following irradiation of adult males at high density in different nitrogen environments.
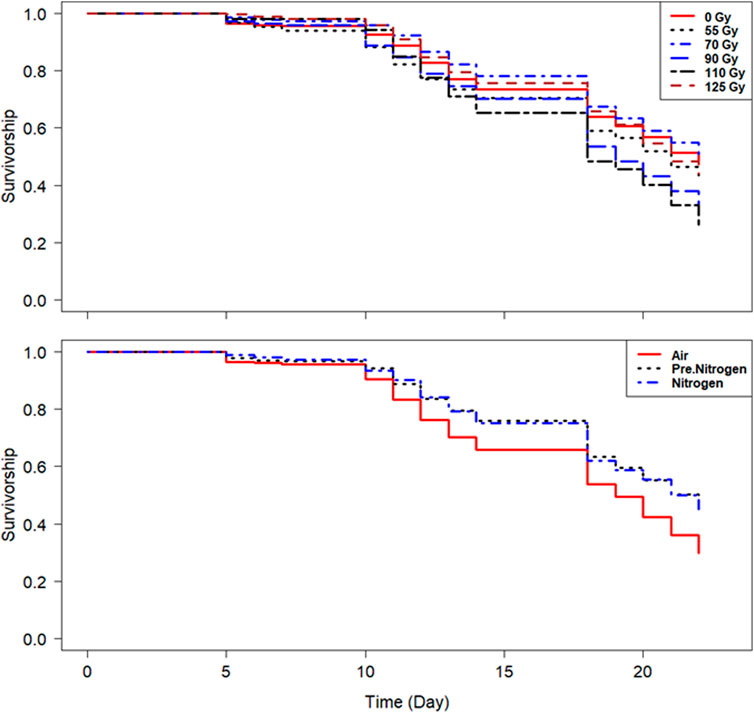
FIGURE 7. Survival of Aedes albopictus following irradiation of adult males at high density in different nitrogen environments.
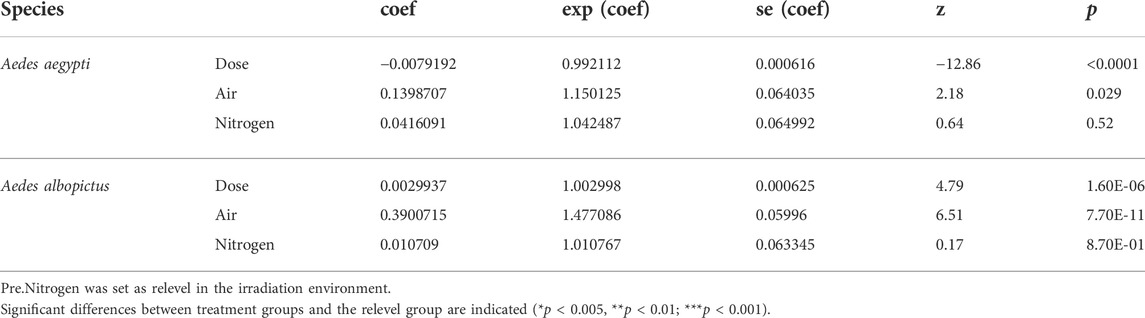
TABLE 5. Fixed-effects coefficients of mixed-effects Cox regression models of the impact of irradiation dose and environment on male longevity in Aedes aegypti and Aedes albopictus following irradiation of adult males at high density.
4 Discussion
Ionizing radiation is the method of choice for the sterilization of male mosquitoes in SIT programs (Helinski et al., 2009). Radiation-induced sterility is the result of dominant lethal mutations in the germ cells caused by radiation (LaChance, 1967). Although germ cells are more sensitive to radiation and are targets for mosquito sterilization, damage also occurs in somatic cells, especially in those undergoing mitotic division, leading to reduced quality traits such as longevity and competitiveness (Proverbs, 1969). For the SIT to be successful, it is important to minimize the negative effects of irradiation. Irradiation in low-oxygen environments (for example, in nitrogen) has been reported to have a radioprotective effect with a beneficial impact on the quality of sterile males in many insect species (Vreysen, 1995; Fisher, 1996; Mutika and Parker, 2006). However, its effect on mosquitoes was not clear (Hallinan and Rai, 1973; Curtis, 1976; El-Gazzar et al., 1983; Yamada et al., 2022) and needed to be investigated further.
The present study investigated the possibility to use nitrogen for adult immobilization in the mass irradiation of Ae. albopictus and Ae. aegypti without inducing adverse effects on their quality. Overall, significant differences were observed between the irradiation environments in the dose–response curves of the egg hatch rate within each species. The doses needed to achieve acceptable sterility were, by order of increase, higher in PreN2 and N2 compared with that for irradiation in air. These results are consistent with the results of previous studies that reported that higher radiation doses were required under nitrogen treatments to achieve adequate induced sterility in many insects, including fruit flies, tsetse flies, and mosquitoes (Hallinan and Rai, 1973; Curtis, 1976; El-Gazzar et al., 1983). Oxygen is known to be a radiosensitizer (Forshier, 2012). Therefore, the high sensitivity of insects to ionizing radiation in air is commonly attributed to the high level of oxygen. In contrast, the radioresistance under N2 is likely because of the absence or low level of oxygen in the cells, as reported previously (Condon et al., 2017; Sassù et al., 2019). The radioresistance observed in males pretreated with nitrogen is also likely because of low oxygen saturation in the tissues during irradiation, which is also the main mechanism responsible for paralysis, which can last for ∼15 min after removing the N2, and a lower metabolic rate. Immobilization of mosquitoes in nitrogen decreases the physiological and biochemical reactions that normally interact with radiation, resulting in radioresistance, since cells that are actively undergoing mitosis are known to be more sensitive to radiation (Proverbs, 1969). The hypothesis of anesthesia-induced radioresistance is supported by studies that assessed the effect of temperature during irradiation. Indeed, it has been reported that lower temperatures decreased the induced sterility of Ae. aegypti irradiated at the pupal or adult stages (Ernawan et al., 2022).
In our study, irradiation of high-density adult male Aedes either in air or in nitrogen treatments had similar extents of induced sterility as irradiation at low mosquito density. This result shows the potential of using nitrogen in adult mass irradiation. However, besides induced sterility, good quality of males is an important requirement to achieve the goal of the SIT.
Our results showed that the male flight ability was reduced by irradiation and that the effect was more pronounced under nitrogen treatments. These results corroborate those of previous studies that reported a negative effect of high ionizing radiation on sterile male quality, commonly attributed to the deleterious effect provoked in somatic cells (Forshier, 2012; Culbert et al., 2018). A recent study also found a reduction in the male flight ability of Ae. albopictus irradiated at 45 Gy in nitrogen compared with irradiation in normoxia as well as unirradiated males (Yamada et al., 2022). However, in our study, the relative differences observed in the male flight ability between the doses inducing nearly full sterility in air and nitrogen treatments were only approximately 10%. Therefore, it remains to be elucidated whether this extent of difference would affect the relative male competitiveness. Indeed, adult male Ae. aegypti irradiated at 100 Gy in nitrogen were found to be fully sterile and were as competitive as unirradiated males, while males irradiated in air at 35, 70, or 100 Gy were less competitive (Hallinan and Rai, 1973). A longer recovery time may enhance the flight ability of the males irradiated under nitrogen treatments and improve their competitiveness, as the flight tests were performed only 1 day after irradiation in the current study. This hypothesis is supported by the beneficial effect of irradiation in nitrogen treatments observed on male survival, as expected (Vreysen, 1995; Fisher, 1996). Overall, high survivorship (>90%) was recorded at day 10 postemergence (7–8 days after irradiation) regardless of the irradiation dose and environment. This suggests that the lifespan of males irradiated in our study conditions would be suitable for the SIT programs as male mosquitoes exhibit a higher mating ability within 10 days after emergence (Sawadogo et al., 2013; Damiens et al., 2016).
Furthermore, this study showed that PreN2 treatment could be a reliable method for immobilizing adult mosquitoes for irradiation. Indeed, in contrast to the control, where mobility was observed during the irradiation period, mosquitoes stayed immobilized in both types of nitrogen treatment. However, long-term immobilization could be detrimental to mosquitoes. The best option would thus be the PreN2 treatment as it reduces the immobilization duration, and it requires a lower dose than that required in N2 environments to achieve full sterility but with similar effects on male quality.
Nitrogen treatment requires additional methods such as a cooling system to knock down the mosquitoes for compaction before the nitrogen treatment as well as for handling and transport purposes after irradiation. It, however, reduces the duration of chilling and allows conduction of the irradiation process without reducing the temperature in the irradiator chamber. For the optimal use of nitrogen, further studies are necessary to develop standardized procedures including the type of container, time and pressure for filling nitrogen, and immobilization duration, considering mosquito species, age, and density.
Data availability statement
The raw data supporting the conclusion of this article will be made available by the authors, without undue reservation.
Author contributions
NB, HY and JB designed the study. NB performed the study, analysed the data, and drafted the manuscript, which was critically revised by HY, KC, WM, HM, and JB. HY, MW, HM, SK, TW, and CM participated in the experiments. JB supervised the study. All authors read and approved the manuscript.
Funding
The research presented in this study was partially funded by the United States under the grant to the IAEA entitled Surge expansion for the sterile insect technique to control mosquito populations that transmit the Zika virus and by FAO.
Conflict of interest
The authors declare that the research was conducted in the absence of any commercial or financial relationships that could be construed as a potential conflict of interest.
Publisher’s note
All claims expressed in this article are solely those of the authors and do not necessarily represent those of their affiliated organizations, or those of the publisher, the editors, and the reviewers. Any product that may be evaluated in this article, or claim that may be made by its manufacturer, is not guaranteed or endorsed by the publisher.
References
FAO/IAEA (2020a). “Guidelines for mark-release-recapture procedures of Aedes mosquitoes. Version 1.0, jérémy bouyer, fabrizio balestrino, nicole Culbert, hanano Yamada,” in Food and agriculture organization of the united. Editor Argilés Rafael (Austria: Nations International Atomic Energy Agency Vienna), 22.
Becker, N., Langentepe-Kong, S. M., Tokatlian Rodriguez, A., Oo, T. T., Reichle, D., Lühken, R., et al. (2022). Integrated control of Aedes albopictus in southwest Germany supported by the sterile insect technique. Parasites Vectors 15, 9. doi:10.1186/s13071-021-05112-7
Bellini, R., Carrieri, M., Balestrino, F., Puggioli, A., Malfacini, M., and Bouyer, J. (2021). Field competitiveness of Aedes albopictus (Diptera: Culicidae) irradiated males in pilot sterile insect technique trials in northern Italy. J. Med. Entomol. 58, 807–813. doi:10.1093/jme/tjaa235
Bellini, R., Medici, A., Puggioli, A., Balestrino, F., and Carrieri, M. (2013). Pilot field trials with Aedes albopictus irradiated sterile males in Italian urban areas. J. Med. Entomol. 50, 317–325. doi:10.1603/ME12048
Bimbilé Somda, N. S., Maïga, H., Mamai, W., Yamada, H., Ali, A., Konczal, A., et al. (2019). Insects to feed insects-feeding Aedes mosquitoes with flies for laboratory rearing. Sci. Rep. 9, 11403–11413. doi:10.1038/s41598-019-47817-x
Bolker, B. M., Brooks, M. E., Clark, C. J., Geange, S. W., Poulsen, J. R., Stevens, M. H. H., et al. (2009). Generalized linear mixed models: A practical guide for ecology and evolution. Trends Ecol. Evol. 24, 127–135. doi:10.1016/j.tree.2008.10.008
FAO/IAEA (2020b). “Guidelines for mass-rearing of Aedes mosquitoes. Version 1, hamidou maiga, wadaka Mamai, hanano Yamada, rafael argilés herrero and jeremy,” in Food and agriculture organization of the united nations international. Editor H Maiga, W Mamai, H Yamada, R Argilés Herrero, and J Bouyer (Austria: Atomic Energy Agency Vienna), 24.
Bouyer, J., Culbert, N. J., Dicko, A. H., Pacheco, M. G., Virginio, J., Pedrosa, M. C., et al. (2020). Field performance of sterile male mosquitoes released from an uncrewed aerial vehicle. Sci. Robot. 5, eaba6251. doi:10.1126/scirobotics.aba6251
Burnham, K. P., and Anderson, D. R. (1998). Model selection and multimodel inference. A practical information-theoretic approach. Available at: https://cir.nii.ac.jp/crid/1572543026243620608 (Accessed April 26, 2022).
Catteruccia, F., Crisanti, A., and Wimmer, E. A. (2009). Transgenic technologies to induce sterility. Malar. J. 8, S7. doi:10.1186/1475-2875-8-S2-S7
Condon, C. H., White, S., Meagher, R. L., Jeffers, L. A., Bailey, W. D., and Hahn, D. A. (2017). Effects of low-oxygen environments on the radiation tolerance of the cabbage looper moth (Lepidoptera: Noctuidae). J. Econ. Entomol. 110, 80–86. doi:10.1093/jee/tow273
Culbert, N. J., Balestrino, F., Dor, A., Herranz, G. S., Yamada, H., Wallner, T., et al. (2018). A rapid quality control test to foster the development of genetic control in mosquitoes. Sci. Rep. 8, 16179–9. doi:10.1038/s41598-018-34469-6
Culbert, N. J., Gilles, J. R. L., and Bouyer, J. (2019). Investigating the impact of chilling temperature on male Aedes aegypti and Aedes albopictus survival. PLOS ONE 14, e0221822. doi:10.1371/journal.pone.0221822
Curtis, C. F. (1976). Radiation sterilization. Report on mosquito research. Ross Inst. Trop. Hyg. 1, 76–31. doi:10.1186/1475-2875-5-41
Damiens, D., Tjeck, P. O., Lebon, C., Le Goff, G., and Gouagna, L. C. (2016). The effects of age at first mating and release ratios on the mating competitiveness of gamma-sterilised Aedes albopictus males under semi field conditions. Vector Biol. J. 1, 29–31. doi:10.4172/2473-4810.1000107
Du, W., Hu, C., Yu, C., Tong, J., Qiu, J., Zhang, S., et al. (2019). Comparison between pupal and adult X-ray radiation, designed for the sterile insect technique for Aedes albopictus control. Acta Trop. 199, 105110. doi:10.1016/j.actatropica.2019.105110
Dyck, V. A., Hendrichs, J., and Robinson, A. S. (2021). Sterile insect technique: Principles and practice in area-wide integrated pest management. Florida: CRC Press.
El-Gazzar, L. M., Dame, D. A., and Smittle, B. J. (1983). Fertility and competitiveness of Culex quinquefasciatus males irradiated in Nitrogen1. J. Econ. entomology 76, 821–823. doi:10.1093/jee/76.4.821
Ernawan, B., Anggraeni, T., Yusmalinar, S., and Ahmad, I. (2022). Investigation of developmental stage/age, gamma irradiation dose, and temperature in sterilization of male Aedes aegypti (Diptera: Culicidae) in a sterile insect technique program. J. Med. Entomol. 59, 320–327. doi:10.1093/jme/tjab166
Fisher, K. (1996). Queensland fruit fly (bactrocera tryoni): Eradication from western Australia. Fruit Fly Pests: CRC Press.
Gato, R., Menéndez, Z., Prieto, E., Argilés, R., Rodríguez, M., Baldoquín, W., et al. (2021). Sterile insect technique: Successful suppression of an Aedes aegypti field population in Cuba. Insects 12, 469. doi:10.3390/insects12050469
Hallinan, E., and Rai, K. S. (1973). Radiation sterilization of Aedes aegypti in nitrogen and implications for sterile male technique. Nature 244, 368–369. doi:10.1038/244368a0
Helinski, M. E., Parker, A. G., and Knols, B. G. (2009). Radiation biology of mosquitoes. Malar. J. 8, S6. doi:10.1186/1475-2875-8-S2-S6
Helinski, M. E., Parker, A. G., and Knols, B. G. (2006). Radiation-induced sterility for pupal and adult stages of the malaria mosquito Anopheles arabiensis. Malar. J. 5, 41. doi:10.1186/1475-2875-5-41
Hurvich, C. M., and Tsai, C.-L. (1995). Model selection for extended quasi-likelihood models in small samples. Biometrics 51, 1077–1084. doi:10.2307/2533006
IAEA (2004). Dosimetry system for SIT: Manual for Gafchromic® film. Vienna, Austria: IAEA. Available at: http://www-naweb.iaea.org/nafa/ipc-gafchromic-dosimetry-sit.html.
LaChance, L. E. (1967). The induction of dominant lethal mutations in insects by ionizing radiation and chemicals-as related to the sterile male technique of insect control. Genet. insect vectors Dis. 21, 617–650. doi:10.1111/j.1365-2915.1991.tb00538.x
Maïga, H., Mamai, W., Bimbilé Somda, N. S., Wallner, T., Poda, B. S., Salvador-Herranz, G., et al. (2020). Assessment of a novel adult mass-rearing cage for Aedes albopictus (skuse) and Anopheles arabiensis (patton). Insects 11, 801. doi:10.3390/insects11110801
Mamai, W., Bimbilé Somda, N. S., Maiga, H., Konczal, A., Wallner, T., Bakhoum, M. T., et al. (2019). Black soldier fly (Hermetia illucens) larvae powder as a larval diet ingredient for mass-rearing Aedes mosquitoes. Parasite 26, 57. doi:10.1051/parasite/2019059
Mamai, W., Maiga, H., Somda, N. S. B., Wallner, T., Konczal, A., Yamada, H., et al. (2020). Aedes aegypti larval development and pupal production in the FAO/IAEA mass-rearing rack and factors influencing sex sorting efficiency. Parasite 27, 43. doi:10.1051/parasite/2020041
Mutika, G. N., and Parker, A. G. (2006). Induced sterility of Glossina pallidipes Austen males after irradiation in a nitrogen atmosphere. Entomological Sci. 9, 47–53. doi:10.1111/j.1479-8298.2006.00153.x
Patterson, R. S., Sharma, V. P., Singh, K. R. P., LaBrecque, G. C., Seetheram, P. L., and Grover, K. K. (1975). Use of radiosterilized males to control indigenous populations of Culex pipiens quinquefasciatus say: Laboratory and field studies. Mosq. News 35, 1–7.
Patterson, R. S., Weidhaas, D. E., Ford, H. R., and Lofgren, C. S. (1970). Suppression and elimination of an island population of Culex pipiens quinquefasciatus with sterile males. Science 168, 1368–1370. doi:10.1126/science.168.3937.1368
Proverbs, M. D. (1969). Induced sterilization and control of insects. Annu. Rev. Entomol. 14, 81–102. doi:10.1146/annurev.en.14.010169.000501
Sassù, F., Nikolouli, K., Pereira, R., Vreysen, M. J., Stauffer, C., and Cáceres, C. (2019). Irradiation dose response under hypoxia for the application of the sterile insect technique in Drosophila suzukii. PloS one 14, e0226582. doi:10.1371/journal.pone.0226582
Sawadogo, S. P., Diabaté, A., Toé, H. K., Sanon, A., Lefevre, T., Baldet, T., et al. (2013). Effects of age and size on Anopheles gambiae ss male mosquito mating success. J. Med. Entomol. 50, 285–293. doi:10.1603/me12041
Vreysen, M. (1995). Radiation sterilization of Glossina tachnoides Westw. pupae. I. The effect of dose fractionation and nitrogen during irradiation in the mid-pupal phase. Revue d’Elevage de Med. Veterinaire Des Pays Tropicaux 48, 45–51. Available at: https://ci.nii.ac.jp/naid/10018122858/(Accessed February 18, 2022).
Wakid, A. M., Tantawy, A. O., Abdel-Malek, A. A., and El-Gazzar, L. M. (1976). Irradiation of the immature stages of the mosquito, Anopheles pharoensis Theob., with 60Co. Z. für Angew. Entomol. 80, 311–316. doi:10.1111/j.1439-0418.1976.tb03332.x
Yamada, H., Maiga, H., Bimbile-Somda, N. S., Carvalho, D. O., Mamai, W., Kraupa, C., et al. (2020). The role of oxygen depletion and subsequent radioprotective effects during irradiation of mosquito pupae in water. Parasit. Vectors 13, 198. doi:10.1186/s13071-020-04069-3
Yamada, H., Maiga, H., Juarez, J., Carvalho, D. D. O., Mamai, W., Ali, A., et al. (2019). Identification of critical factors that significantly affect the dose-response in mosquitoes irradiated as pupae. Parasit. Vectors 12, 435. doi:10.1186/s13071-019-3698-y
Yamada, H., Maiga, H., Kraupa, C., Mamai, W., Bimbilé Somda, N. S., Abrahim, A., et al. (2022). Effects of chilling and anoxia on the irradiation dose-response in adult Aedes mosquitoes. Front. Bioeng. Biotechnol. 10, 856780. doi:10.3389/fbioe.2022.856780
Zhang, D., Xi, Z., Li, Y., Wang, X., Yamada, H., Qiu, J., et al. (2020). Toward implementation of combined incompatible and sterile insect techniques for mosquito control: Optimized chilling conditions for handling Aedes albopictus male adults prior to release. PLoS Negl. Trop. Dis. 14, e0008561. doi:10.1371/journal.pntd.0008561
Keywords: sterile insect technique, irradiation, Aedes aegypti, Aedes albopictus, anoxia
Citation: Bimbilé Somda NS, Yamada H, Kraupa C, Mamai W, Maiga H, Kotla SS, Wallner T, Martina C and Bouyer J (2022) Response of male adult Aedes mosquitoes to gamma radiation in different nitrogen environments. Front. Bioeng. Biotechnol. 10:942654. doi: 10.3389/fbioe.2022.942654
Received: 12 May 2022; Accepted: 28 July 2022;
Published: 12 September 2022.
Edited by:
Mukesh Kumar Awasthi, Northwest A&F University, ChinaReviewed by:
Priyanka De Silva, University of Peradeniya, Sri LankaSampa Banerjee, University of Calcutta, India
Copyright © 2022 Bimbilé Somda, Yamada, Kraupa, Mamai, Maiga, Kotla, Wallner, Martina and Bouyer. This is an open-access article distributed under the terms of the Creative Commons Attribution License (CC BY). The use, distribution or reproduction in other forums is permitted, provided the original author(s) and the copyright owner(s) are credited and that the original publication in this journal is cited, in accordance with accepted academic practice. No use, distribution or reproduction is permitted which does not comply with these terms.
*Correspondence: Nanwintoum Séverin Bimbilé Somda, nansevbis@gmail.com