- 1Key Laboratory of Brain Science Research and Transformation in Tropical Environment of Hainan Province, Department of Neurology, The First Affiliated Hospital, Hainan Medical University, Haikou, China
- 2Department of Human Anatomy and Department of Neurology of the First Affiliated Hospital, Hainan Medical University, Haikou, China
Our general purpose was to provide a theoretical and practical foundation for the use of exosomes (EXOs) that have high levels of CD47 as stable and efficient drug carriers. Thus, we prepared EXOs from adipose tissue-derived mesenchymal stromal cells (ADMSCs) that had high levels of CD47 (EXOsCD47) and control EXOs (without CD47), and then compared their immune escape in vivo and their resistance to phagocytosis in vitro. Nanoflow cytometry was used to determine the CD47 level in these EXOs, and the amount of EXOsCD47 that remained in rat plasma at 3 h after intraperitoneal injection. Phagocytosis of the EXOs was also determined using in vitro rat macrophage bone marrow (RMA-BM) experiments. Our in vitro results showed that macrophages ingested significantly more control EXOs than EXOsCD47 (p < 0.01), with confirmation by ultra-high-definition laser confocal microscopy. Consistently, our in vivo results showed that rats had 1.377-fold better retention of EXOsCD47 than control EXOs (p < 0.01). These results confirmed that these engineered EXOsCD47 had improved immune escape. Our results therefore verified that EXOsCD47 had increased immune evasion relative to control EXOs, and have potential for use as drug carriers.
Introduction
Pan and Johnstone first described the formation and release of small extracellular vesicles by sheep reticulocytes in 1983 (Pan and Johnstone, 1983), and Johnstone et al. (1987) first used the word “exosome” to refer to these extracellular vesicles in 1987. Exosomes (EXOs) are extracellular vesicles with diameters of 30–200 nm that are secreted by diverse living cells. Many different types of eukaryotic and prokaryotic cells produce EXOs (Trams et al., 1981; Kowal et al., 2014). For example, mesenchymal stem cells (MSCs), immune cells, cancer cells, and neurons all secrete EXOs (Fauré et al., 2006; Krämer-Albers et al., 2007; Guescini et al., 2010; Lachenal et al., 2011; Lai et al., 2015). An EXO can function as a carrier of genetic material and affect many physiological and pathological functions of cells, including signal transduction pathways. In particular, EXOs can contain mRNAs, microRNAs, and proteins, and transfer these molecules to nearby or distant cells, thereby regulating the function of recipient cells (Valadi et al., 2007). Many studies showed that EXOs play important roles in nerve development, nerve regeneration, and the protection of nerve synaptic activity (Antonucci et al., 2012; Frühbeis et al., 2012; Frühbeis et al., 2013; Morel et al., 2013; Yang et al., 2015). EXOs from different sources have different proteins, but some proteins are present in all known EXOs (CD9, CD82, and ALEX), and these can be used as marker proteins to identify EXOs (Zhang and Grizzle, 2014; Chen J. et al., 2021; Xu et al., 2022). The EXOs secreted by different cells have different functions. For example, EXOs derived from MSCs can promote peripheral nerve regeneration (Mead and Tomarev, 2017), and EXOs derived from neuronal stem cells may protect the heart from ischemia/reperfusion injury by altering signaling via the JAK1/2 and Gp130 pathways (Katsur et al., 2021). MSCs typically produce abundant EXOs (Madrigal et al., 2014).
Because EXOs are naturally produced nano-scale carriers, they can be loaded with drugs or other substances to facilitate intercellular transport. Moreover, the lipid bimolecular membrane structure of EXOs means they can easily penetrate cell membranes, and can even pass through the blood-brain barrier. The advantages of EXOs as drug delivery carriers is that they can encapsulate a drug and other substances, such as RNase and proteases, and then deliver these substances to target cells in complete form (Alvarez-Erviti et al., 2011; Kooijmans et al., 2012). Alvarez-Erviti et al. (2011) added drugs to EXOs using electroporation and other methods to engineer EXOs for treatment of diseases. Other studies reported that EXOs can be used as drug carriers in tissues, and that EXOs are useful for damage repair and treatment of chronic diseases (Wahlgren et al., 2012; Lamichhane et al., 2015). These novel scientific and technological developments potentially have major impacts on human health and life (Chen L. et al., 2021; Wang et al., 2021), and use of EXOs as drug carriers are gradually changing the treatments for certain diseases.
Many recent studies showed that EXOs play a key role in the repair of nerve injuries (Dong et al., 2019; Salarpour et al., 2022). EXOs act on neurons, Schwann cells, microglia, and other cells of the nervous system by release of active substances that promote nerve regeneration. In 2018, Li et al. (2021) (Yin et al., 2018) described the effect of adipose-derived mesenchymal stem cell-EXOs (ADMSC-EXOs) using the sciatic nerve injury model. They reported reduced damage within the nerve bundle, a more intact and functional perineurium, reduced Schwann cell apoptosis and autophagy, and increased repair of sciatic nerve injury. In 2019, Ren Z. W. et al. (2019) developed miRNA-133b-modified ADMSC-EXOs and found that they significantly promoted the recovery of nerve function in animals with spinal cord injury due to their effects on signal pathways related to axon regeneration and the expression of NF, GAP-43, GFAP, and MBP. Engineered EXOs derived from ADMSCs can therefore be used to reduce or repair nerve damage.
Initial research of our group found that EXOs can carry miRNA-21-5p, which then acts on dorsal root ganglion neurons by downregulating the SPRY gene and protein. CD47 promotes the growth of neurites, and also down-regulates the expression of TIMP3 and reduces neuronal apoptosis. Therefore, EXOs have potential use as carriers of drugs and active substances that function in tissue repair, such as nerve regeneration. Moreover, our laboratory is currently developing certain innovations in EXO extraction technologies. In particular, we added an ultra-filtration process to the extraction of EXOs by using an “ultra-isolation” method to increase the concentration and purity of EXOs, so that they have a wider application as drug carriers.
CD47, also known as integrin-associated protein (IAP), is a transmembrane glycoprotein that is present in a variety of cells (Ren Z. W. et al., 2019). This protein has an immunoglobulin variable N-terminal domain, five transmembrane domains, and a C-terminal intracellular domain that has four different spliced isoforms (Duperrier et al., 2002). CD47 functions as the ligand of SIRPα, and their binding can inhibit phagocytosis (Chao et al., 2012). CD47 inhibits the phagocytosis and stimulates transmembrane migration of a variety of immune cells (Stefanidakis et al., 2008), and also participates in a variety of immunophysiological processes, such as inducing the apoptosis of lymphocytes (Pettersen et al., 1999) and inhibiting the release of cytokine IL-from monocytes (Avice et al., 2000). Many studies (Willingham et al., 2012) reported that tumor cells had increased expression of CD47, and this provided them with successful immune escape. Kamerkar et al. (2017) injected mice with EXOs that had a high level of CD47 or with CD47 knock-out. Their EXOsCD47 activated the CD47-SIRPα pathway, accumulated in the circulation, and could be used to target the oncogenic Kras protein This study inspired us to construct EXOs with the CD47 phenotype to block their phagocytosis, so they can be used to escape immune responses and function as carriers for various types of drugs. However, the EXOsCD47 used in previous research were derived from tumor cells, and because these EXOs could possibly induce carcinogenesis, they are not suitable for use as drug carriers. We therefore examined the development of EXOs that have potential clinical applications as drug carriers (Chundawat et al., 2021).
We previously constructed CD63-GFP lentiviral vectors, transfected them into MSCs, and extracted fluorescently labeled EXOs from the serum-free culture supernatant (Ren et al., 2017). This research also showed successful use of PKH67 to label EXOs for in vivo tracing, allowing evaluation of the immune escape of EXOsCD47. Current research on EXOs derived from MSCs is still in the exploratory stage, but animal models have demonstrated their therapeutic potential. Compared with the transplantation of stem cells, the use of appropriate EXOs is likely to have fewer adverse effects and be more suitable for tissue regeneration therapy (Caseiro et al., 2015). In the present study, we used EXOs derived from ADMSCs to construct EXOsCD47 as a basis for subsequent examination of their effects on neural repair. Immune escape may occur due to antigenic changes, persistent infections, or immunosuppression; the EXOsCD47 studied here were designed to achieve immune escape by immunosuppression.
We used ADMSCs because they are readily available, easy to grow in vitro, and can secrete a variety of cytokines (Heo et al., 2019; Prakoeswa et al., 2020). Recent studies showed that ADMSC-EXOs contain 148 miRNAs (Zhang et al., 2017) and 1,688 proteins, and function in a variety of cells (Ni et al., 2018). This makes ADMSC-EXOs highly suitable as carriers of therapeutic drugs.
Our general goal was to assess the feasibility of using EXOsCD47 for clinical applications, especially for the treatment of nerve injuries. The specific purposes of this study were to construct a CD47 overexpression lentivirus, transfect ADMSCs to obtain CD47-ADMSCs, extract and purify the EXOs secreted by these cells (EXOsCD47), measure the levels of CD47 in these EXOs using flow cytometry and qPCR, and examine the immune escape of these EXOsCD47.
Materials and Methods
Cell Culture
HEK293T and rat ADMSC cells were maintained in Dulbecco’s modified Eagle medium (DMEM, Hyclone, United States) that was supplemented with 10% fetal bovine serum (HyClone, United States) and 1% penicillin/streptomycin (HyClone, United States). Rat macrophage bone marrow (RMA-BM) cells were maintained in DMEM supplemented with 10% fetal bovine serum (HyClone). All cells were cultured at 37°C with 5% CO2 (Raessi et al., 2021).
Plasmid Construction
CD47 was amplified using PCR with the primer sequences listed in Supplementary Table S1. Then, the amplicon was inserted into the GV492 vector (Jikai Gene) between BamHI and AgeI to create the GV492-CD47 plasmid. The final product was confirmed by sequencing, and the clone was stored at −80°C before use. GV492-CD47 was used to transfect HEK293T cells, and qPCR was used to measure the level of CD47.
Lentivirus Package
The pHelper 1.0 vector plasmid (15 µg), pHelper 2.0 vector plasmid (10 µg), Jikai Transfection Reagent (1 ml, all from Jikai Gene), and GV492-CD47 vector plasmid (20 µg) were mixed and allowed to stand at room temperature for 15 min. The solution was dripped into the culture medium with HEK293T cells, and the culture medium was discarded after 6 h. After washing with PBS, 20 ml of complete medium was added, and the cells were grown for 72 h. After centrifugation (4,200 g, 4°C, 10 min), the supernatant was collected and passed through a 0.45-μm filter. The filtrate was transferred to an “ultra-isolation” tube (40 ml, Beckman-Coulter) and centrifuged (54,000 g, 4°C, 2 h). Then, the supernatant was removed, DMEM was added, and the sample was mixed by pipetting. After full dissolution, the sample was centrifuged again (7,800 g, 5 min), and the supernatant was aspirated. The HEK293T cells that were transfected with the CD47 lentiviral vector were examined using fluorescence microscopy and the virus titer was calculated.
Transfection
To produce EXOsCD47, ADMSCs were transfected with lentiviral vectors. When the cell density was 60%–70% in 100 mm Petri dishes, the medium was supplemented with 2 ml of puromycin (6 μg/ml) as a selection medium. Then, 10 μg/ml of CD47 overexpression lentivirus (50 MOI) was added, and cells were cultivated for 1 day. The original medium was then discarded and replaced with complete medium, and the cells were cultivated to the fifth passage prior to observation of fluorescence.
qPCR of CD47-ADMSCs
Total RNA was extracted from cells using Trizol (Invitrogen). A Nanodrop-2000 spectrophotometer (Thermo Scientific, United States) was used to measure RNA concentration. To analyze mRNA expression, 2 μg of total RNA was reverse-transcribed using the Hifair II 1st strand cDNA Synthesis SuperMix (11119ES60, Yeasen, China), and qPCR was performed using Hieff qPCR SYBR Green Master Mix (11200ES60, Yeasen, China) with the primer sequences listed in Supplementary Table S2. Each kit was used in accordance with the manufacturer’s instructions.
Preparation of Exosomes
The detailed procedures used to prepare exosomes were described by Ren R. et al. (2019). Briefly, EXOs were extracted from the culture supernatant of ADMSCs. In this procedure, ADMSCs were washed twice with PBS, serum-free medium was added, cells were cultured at 37°C and 5% CO2 for 72 h, and the supernatant was then collected after centrifugation. The EXOs were then separated by ultracentrifugation. First, the sample was centrifuged (3,000 g, 15 min) to remove cell debris. Then, the supernatant was passed through a 0.22-μm filter and ultra-centrifuged at 100,000 g for 70 min. The pellet was resuspended, and the ultra-centrifugation was repeated. The resulting pellet consisted of EXOs, and was diluted in PBS and stored at −80°C until use.
Characterization of ExosomesCD47
The size distribution of extracted EXOs was determined using ZetaView (Colloid Metrix, Germany), and their morphology was examined using transmission electron microscopy (JEM-2000EX TEM, Japan). The level of CD47 was determined using a nanoflow cytometer. EXOs derived from ADMSCs that were not transfected were used as a control.
Fluorescence Tracer Quantification of Plasma Exosomes
All animal experiments were pre-approved by the local ethics committee. These experiments used healthy male Sprague-Dawley (SD) rats that were 6–8 weeks old that were provided by Changsha Tianqin Company. The two groups of rats received intraperitoneal injections with 1×106 EXOs derived from control ADMSCs or transfected cells (EXOsCD47), and rat plasma was extracted 3 h later. The plasma sample was diluted in PBS (11 ml) and passed through a 0.2-μm filter. Nanoflow cytometry was used to analyze the positivity of PKH67-EXOs in these samples, and comparison of the two groups was used to determine the extent of immune escape.
Western Blotting of Rat Peritoneal Lavage Fluid
At 30 min after intraperitoneal injection of EXOs, the peritoneal lavage fluid of the two groups of rats was drawn, lysed with RIPA lysis buffer at 4°C for 30 min, and the protein concentration was then measured using the Pierce BCA kit (Thermo, United States). The protein samples were concentrated on a 5% SDS-PAGE stacking gel, and then separated on a 12% SDS-PAGE resolving gel. The proteins were then transferred to a PVDF membrane. After blocking with 5% BSA, this membrane was incubated with the primary antibody at 4°C overnight, and then with the corresponding HRP-conjugated secondary antibody at room temperature for 1 h. The band was observed using an automated digital gel image developer (TANON 5200Multi, CN). The primary antibodies were rabbit anti-CD14 antibody (1:1,000, Invitrogen, PA5-95334) and rabbit anti-CD68 antibody (1:1,000, Invitrogen, PA5-78996). The secondary antibody was an HRP-conjugated goat anti-rabbit antibody (1:2000, Cell Signaling Technology, 7074P2).
Phagocytosis of Isolated Macrophages
Immunofluorescence was used to identify RMA-BMs based on the presence of CD68. These cells were cultured in a confocal laser culture dish, and different groups of EXOs (∼1×108) were added to each well, followed by incubation for 3 h. The controls consisted of PKH67-labeled non-transfected ADMSC-derived EXOs. Ultra-high-definition laser confocal microscopy (WHB Scientific, WHB-35-20-1) was used for observations at 1, 2, and 3 h after the addition of EXOs.
Statistical Analysis
All data were expressed as means ± SEMs. Student’s t-test was used for comparisons of two groups, and one-way ANOVA was used for comparisons of three or more groups. A difference was considered statistically significant when the p value was below 0.05.
Results
Lentiviral Plasmid With CD47 Overexpression
We constructed a GV492-CD47-GFP recombinant plasmid as the first step in engineering EXOs that overexpress CD47 from Rattus norvegicus (Figure 1A). Agarose gel electrophoresis confirmed that CD47 had a size of about 950 bp (Figure 1B). We then transfected HEK293T cells with these CD47 overexpression plasmids. The results showed green fluorescence, indicating successful overexpression of CD47 (Figure 1C), and significantly greater expression of CD47 in transfected cells than control cells (Figure 1D). To ensure the CD47 overexpression plasmid was stably expressed during the passage process, we used lentivirus to wrap the CD47 overexpression plasmid, and then transfected the HEK293T cells again. The results indicated green fluorescence (Figure 1E), and measurements of transduction units (TU) indicated about 1 × 109 TU/ml.
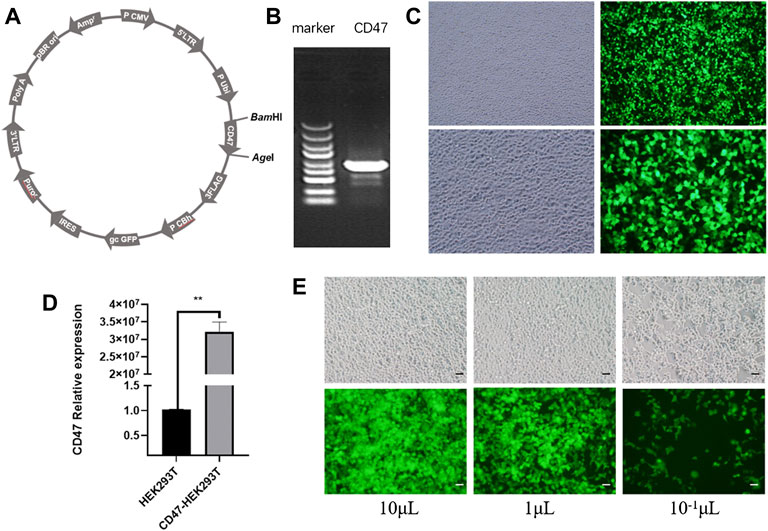
FIGURE 1. (A) Vector plasmid with CD47 overexpression. (B) Agarose gel electrophoresis of the target gene (CD47). (C) Phase contrast microscopy (left) and fluorescence microscopy (right) of HEK293T cells that were transfected with the CD47 overexpression plasmid. (D) Relative expression of CD47 (qPCR) in HEK293T cells before and after plasmid transfection. N = 3, **p < 0.01. (E) Phase contrast microscopy (top) and fluorescence microscopy (bottom) of HEK293T cells with the CD47 overexpression lentiviral vector after transfection. Scale bar: 500 μm.
Development and Characterization of ExosomesCD47
To obtain EXOs with high levels of CD47 (EXOsCD47), we transfected ADMSCs with a lentiviral vector containing a CD47 overexpression plasmid, conducted screening using puromycin, and then examined cells from the fifth generation (Figure 2A). The results indicated higher expression of CD47 in fifth-generation CD47-ADMSCs than in control fifth-generation ADMSCs (Figure 2B). We then extracted EXOs from these cells using ultrafiltration and “ultra-isolation” (Figure 2C). Transmission electron microscopy showed that the vesicles secreted by the transfected ADMSCs had morphological characteristics that were typical of EXOs (Figure 2D). Nanoparticle analysis showed that the particle size distribution of these EXOs was within the normal range for EXOs (Figure 2E). We then used nanoflow cytometry to determine the CD47 level in the EXOs secreted by CD47-ADMSCs relative to fifth-generation controls. The results indicated the level of CD47 in EXOsCD47 was about 1.7-times greater than in ADMSC-EXOs (Figure 2F).
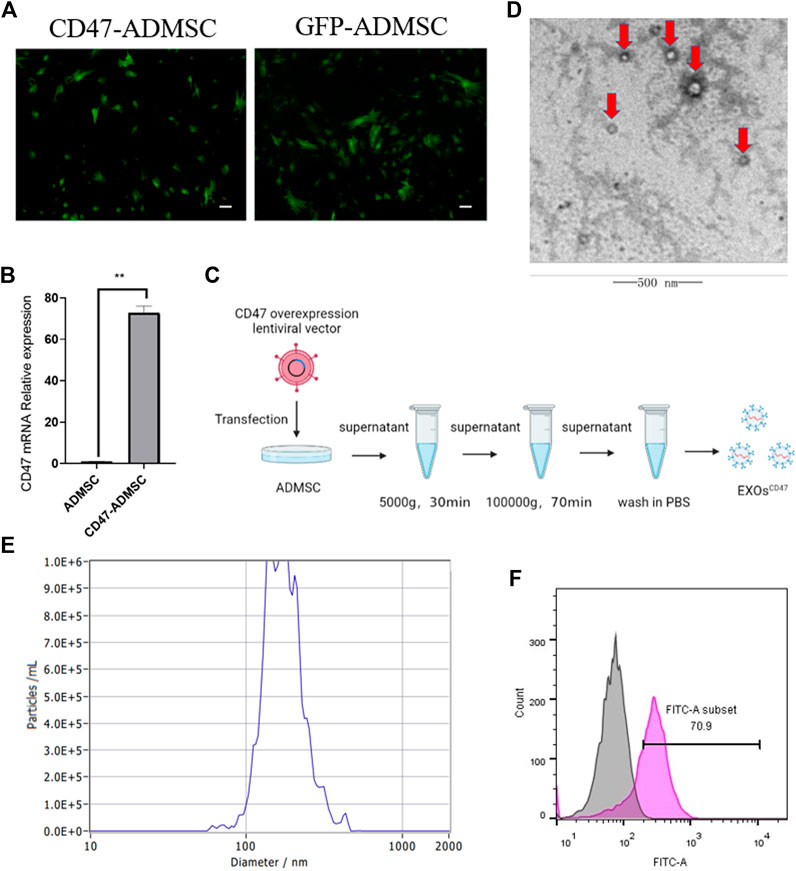
FIGURE 2. (A) Fluorescence microscopy of ADMSCs with the CD47 overexpression lentiviral vector at the fifth passage after transfection and GFP-ADMSCs (positive control). Scale bar: 500 μm. (B) Relative expression (qPCR) of CD47 in ADMSCs and CD47-ADMSCs at the fifth passage after transfection. N = 3, **p<0.01. (C) Experimental procedure used to extract EXOs. (D) Electron microscopy of EXOs produced by ADMSCs (red arrows). Scale bar: 500 nm (E) Size distribution of EXOs produced by ADMSCs. (F) Nanoflow cytometry of CD47 expression by EXOsCD47. Pink: EXOsCD47; Grey: ADMSC-EXOs.
Immune Escape of ExosomesCD47 in Rats
To test the immune escape of EXOsCD47 in vivo, we prepared PKH67-labeled EXOsCD47 and ADMSC-EXOs (control) and administered them by intraperitoneal injection into rats (1 ml containing 1×106 EXOs, six rats per group, Figure 3A). After 3 h, rat plasma was extracted, and total plasma EXOs were extracted by ultrafiltration and “ultra-isolation”. Nanoflow cytometry showed greater plasma retention of EXOsCD47 than ADMSC-EXOs (Figure 3B), suggesting increased immune escape by the EXOsCD47.
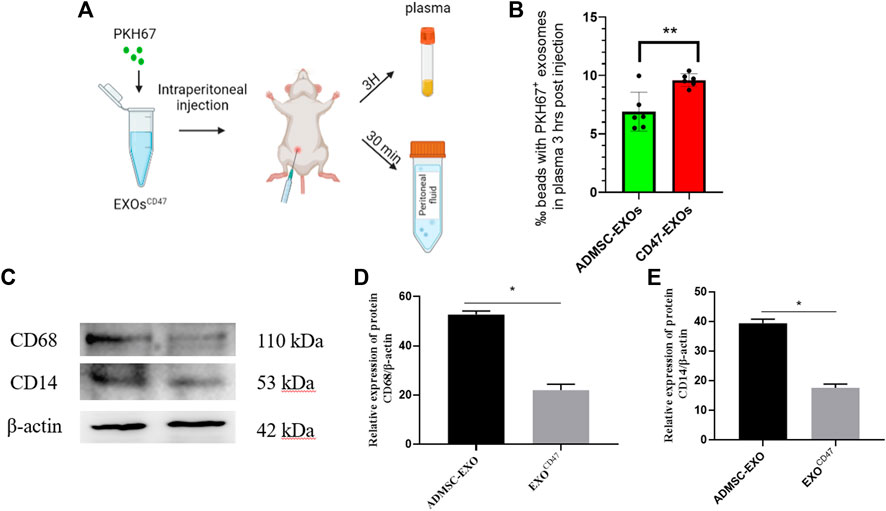
FIGURE 3. (A) Experimental procedure used to examine the in vivo stability of EXOs. (B) Retention of EXOs at 3 h after intraperitoneal injection of ADMSC-EXOs (control) and EXOsCD47. n = 6, **p<0.01. (C–E) Western blotting of lavage fluid for CD68 and CD14 (macrophage markers) in the ADMSC-EXOs (control) and EXOsCD47 groups. N = 3, *p < 0.05.
To determine whether the PKH67-labeled exosomes triggered the phagocytosis of macrophages in the abdominal cavity, we collected peritoneal lavage fluid from rats at 30 min after injection of the PKH67-labeled exosomes. Western blotting showed that two macrophage markers (CD14 and CD68) were significantly down-regulated in the EXOsCD47 group (Figure 3C). These results suggest that EXOsCD47 are less likely to induce macrophage phagocytosis in the abdominal cavity (Figure 3C).
Immune Escape of ExosomesCD47 in vitro
We performed immunofluorescence microscopy of RMA-BM to confirm they had the general characteristics of macrophages (Figure 4A). To assess immune escape of EXOsCD47 in vitro, we added PKH67-labeled EXOsCD47 or control EXOs into RMA-BM cell culture medium, and then analyzed these cells using ultra-high-definition laser confocal microscopy after 1, 2, and 3 h. The results indicated significantly greater engulfment of ADMSC-EXOs than EXOsCD47 (Figures 4B,C).
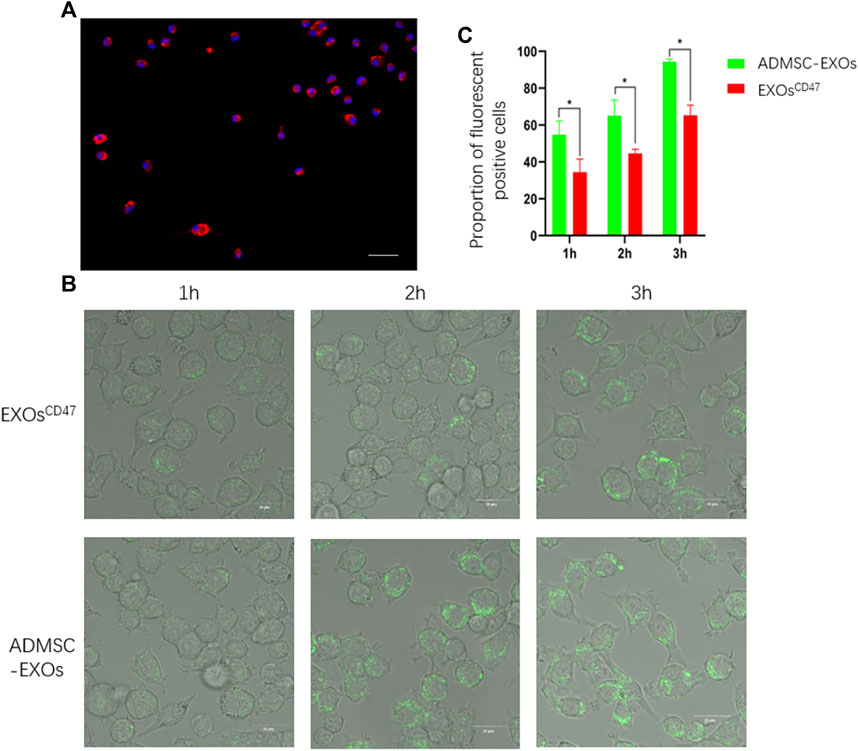
FIGURE 4. (A) Immunofluorescence analysis of isolated macrophages. Blue: DAPI, Red: CD68, scale bar: 100 μm. (B) Representative ultra-high-definition laser confocal microscopy of the phagocytosis of EXOs from the ADMSC-EXOs (control) and EXOsCD47 groups after 1, 2, and 3 h scale bar: 20 μm. (C). Quantitation of the results in Figure 4B. *p < 0.05, n = 3.
Discussion
Our in vivo and in vitro experiments indicated successful construction of functionalized EXOs derived from ADMSCs that had high levels of CD47 and were able to escape phagocytosis by macrophages. Many recent studies have used nanoparticles in diverse medical applications, most notably for experimental treatment of cancer (Roostaee and Sheikhshoaie, 2020; Chan et al., 2022; Roostaee et al., 2022). These EXOsCD47 thus have potential for use in subsequent research as drug carriers.
Previous studies found that CD47, a transmembrane glycoprotein, is present in a variety of cells, that its expression is significantly increased in tumor cells (Hu et al., 2021), and there is also some increased expression in normal ADMSCs. Other studies showed that the EXOs secreted by some tumor cells have roles in the onset and development of tumors (Whiteside, 2016). Although tumor cells produce EXOs with high levels of CD47, they are obviously not suitable for use as drug carriers in clinical practice. Instead, we chose ADMSCs as the source of EXOsCD47 because previous studies of the EXOs secreted by these cells reported no adverse reactions (Kibria et al., 2016).
A recent study reported that the CD47 level in EXOs from the plasma of breast cancer patients was higher than in healthy controls (Hu et al., 2021). Another study reported that the retention of EXOs derived from fibroblasts of mice with CD47 knockout was reduced in vivo (Kamerkar et al., 2017). This led us to hypothesize that a cell line with overexpression of CD47 can produce EXOsCD47 that have greater immune escape function. Our design of the CD47 overexpression plasmid was based a previous study (Ye et al., 2014). Thus, we added a lentivirus to the plasmid so that CD47 was stably expressed during cell passage. We then identified EXOsCD47 using nano-flow cytometry, which has certain advantages over western blotting for CD47, as employed by Du et al. (2021).
In this study, we performed intraperitoneal injection of EXOs into rats, as described by Kamerkar et al. (2017). We used this method because peritoneal washing fluid can be easily collected and analyzed to determine the chemotaxis and aggregation of macrophages. Administration of EXOs by other methods does not have this advantage. Kamerkar et al. (2017) extracted plasma from orbital blood to determine the retention of plasma EXOs, and then used a nanoparticle analyzer to measure the concentration and number of retained EXOs. We collected blood by perfusion, extracted plasma, and then performed nanoflow cytometry to detect the retention of EXOs.
To further verify the anti-macrophage effect of EXOsCD47, we designed an in vitro macrophage phagocytosis experiment to examine the relationship between EXOs and macrophages. The results showed that macrophages ingested significantly more control EXOs than EXOsCD47 at 1, 2, and 3 h. These results indicate that the EXOsCD47 described here had resistance to ingestion by macrophages, although they were ultimately phagocytized. This result supports our in vivo results regarding the prolonged residence time of EXOsCD47 in the blood. We used high-resolution confocal laser microscopy in these experiments to observe the phagocytosis of macrophages, and the results provided more definitive evidence of the anti-phagocytosis activity of EXOsCD47.
Conclusion
Many previous studies showed that EXOs have potential for use as agents that deliver therapeutic drugs, especially in the nervous system because EXOs may allow specific drugs to pass through the blood-brain barrier (Tian et al., 2018). However, the therapeutic potential of EXOs is limited due to their phagocytosis by macrophages (Yong et al., 2017). Therefore, we used ADMSCs to construct EXOsCD47. These EXOsCD47 have tissue repair function and increased immune escape, increasing their potential to function as drug carriers. In the future, we plan to examine the effects of loading these EXOsCD47 with drugs that repair nerve damage. We also plan to examine the possibility of mass production of EXOsCD47 to provide a theoretical and practical foundation for their use in commercial and clinical applications.
Data Availability Statement
The original contributions presented in the study are included in the article/Supplementary Material, further inquiries can be directed to the corresponding author.
Ethics Statement
The animal study was reviewed and approved by the All animal experiments were pre-approved by the local ethics committee.
Author Contributions
Conceptualization, X-YB, X-NY, Q-FL, and Y-QL; cell culture, X-YB and Y-RW; exosome isolation, H-HZ, and H-YZ; data analysis, X-YB, R-JF, and RR; Animal experiment, D-XL, P-LN; writing, review and editing, all authors. All authors have read and agreed to the published version of the manuscript.
Funding
This study was funded by Social Development Project of Hainan Province of China (ZDYF2020145 and ZDYF2022SHFZ109), the National Natural Science Foundation of China (81460196), supported by Hainan Provincial Natural Science Foundation of China (822QN312), Postgraduate Innovative Research Projects of Hainan Province (HYYB2021A10, HYYS2021A3), and the Finance Science and Technology Project of Hainan Province of China, Hainan Province Clinical Medical Center.
Conflict of Interest
The authors declare that the research was conducted in the absence of any commercial or financial relationships that could be construed as a potential conflict of interest.
Publisher’s Note
All claims expressed in this article are solely those of the authors and do not necessarily represent those of their affiliated organizations, or those of the publisher, the editors and the reviewers. Any product that may be evaluated in this article, or claim that may be made by its manufacturer, is not guaranteed or endorsed by the publisher.
Supplementary Material
The Supplementary Material for this article can be found online at: https://www.frontiersin.org/articles/10.3389/fbioe.2022.936951/full#supplementary-material
References
Alvarez-Erviti, L., Seow, Y., Yin, H., Betts, C., Lakhal, S., and Wood, M. J. A. (2011). Delivery of siRNA to the Mouse Brain by Systemic Injection of Targeted Exosomes. Nat. Biotechnol. 29 (4), 341–345. doi:10.1038/nbt.1807
Antonucci, F., Turola, E., Riganti, L., Caleo, M., Gabrielli, M., Perrotta, C., et al. (2012). Microvesicles Released from Microglia Stimulate Synaptic Activity via Enhanced Sphingolipid Metabolism. Embo J. 31 (5), 1231–1240. doi:10.1038/emboj.2011.489
Avice, M.-N., Rubio, M., Sergerie, M., Delespesse, G., and Sarfati, M. (2000). CD47 Ligation Selectively Inhibits the Development of Human Naive T Cells into Th1 Effectors. J. Immunol. 165 (8), 4624–4631. doi:10.4049/jimmunol.165.8.4624
Caseiro, A. R., Pereira, T., Ribeiro, J., Amorim, I., Faria, F., Bártolo, P. J., et al. (2015). Neuro-muscular Regeneration Using Scaffolds with Mesenchymal Stem Cells (MSCs) Isolated from Human Umbilical Cord Wharton's Jelly: Functional and Morphological Analysis Using Rat Sciatic Nerve Neurotmesis Injury Model. Procedia Eng. 110, 106–113. doi:10.1016/j.proeng.2015.07.016
Chan, M.-H., Chang, Z.-X., Huang, C.-Y. F., Lee, L. J., Liu, R.-S., and Hsiao, M. (2022). Integrated Therapy Platform of Exosomal System: Hybrid Inorganic/organic Nanoparticles with Exosomes for Cancer Treatment. Nanoscale Horiz. 7 (4), 352–367. doi:10.1039/d1nh00637a
Chao, M. P., Weissman, I. L., and Majeti, R. (2012). The CD47-Sirpα Pathway in Cancer Immune Evasion and Potential Therapeutic Implications. Curr. Opin. Immunol. 24 (2), 225–232. doi:10.1016/j.coi.2012.01.010
Chen, J., Li, P., Zhang, T., Xu, Z., Huang, X., Wang, R., et al. (2021). Review on Strategies and Technologies for Exosome Isolation and Purification. Front. Bioeng. Biotechnol. 9, 811971. doi:10.3389/fbioe.2021.811971
Chen, L., Huang, Y., Yu, X., Lu, J., Jia, W., Song, J., et al. (2021). Corynoxine Protects Dopaminergic Neurons through Inducing Autophagy and Diminishing Neuroinflammation in Rotenone-Induced Animal Models of Parkinson's Disease. Front. Pharmacol. 12, 642900. doi:10.3389/fphar.2021.642900
Chundawat, N. S., Jadoun, S., Zarrintaj, P., and Chauhan, N. P. S. (2021). Lanthanide Complexes as Anticancer Agents: A Review. Polyhedron 207, 115387. doi:10.1016/j.poly.2021.115387
Dong, R., Liu, Y., Yang, Y., Wang, H., Xu, Y., and Zhang, Z. (2019). MSC-Derived Exosomes-Based Therapy for Peripheral Nerve Injury: A Novel Therapeutic Strategy. BioMed Res. Int. 2019, 6458237. doi:10.1155/2019/6458237
Du, J., Wan, Z., Wang, C., Lu, F., Wei, M., Wang, D., et al. (2021). Designer Exosomes for Targeted and Efficient Ferroptosis Induction in Cancer via Chemo-Photodynamic Therapy. Theranostics 11 (17), 8185–8196. doi:10.7150/thno.59121
Duperrier, K., Farre, A., Bienvenu, J., Bleyzac, N., Bernaud, J., Gebuhrer, L., et al. (2002). Cyclosporin A Inhibits Dendritic Cell Maturation Promoted by TNF-Alpha or LPS but Not by Double-Stranded RNA or CD40L. J. Leukoc. Biol. 72 (5), 953–961.
Fauré, J., Lachenal, G., Court, M., Hirrlinger, J., Chatellard-Causse, C., Blot, B., et al. (2006). Exosomes Are Released by Cultured Cortical Neurones. Mol. Cell. Neurosci. 31 (4), 642–648. doi:10.1016/j.mcn.2005.12.003
Frühbeis, C., Fröhlich, D., and Krämer-Albers, E.-M. (2012). Emerging Roles of Exosomes in Neuron-Glia Communication. Front. Physio. 3, 119. doi:10.3389/fphys.2012.00119
Frühbeis, C., Fröhlich, D., Kuo, W. P., Amphornrat, J., Thilemann, S., Saab, A. S., et al. (2013). Neurotransmitter-triggered Transfer of Exosomes Mediates Oligodendrocyte-Neuron Communication. PLoS Biol. 11 (7), e1001604. doi:10.1371/journal.pbio.1001604
Guescini, M., Genedani, S., Stocchi, V., and Agnati, L. F. (2010). Astrocytes and Glioblastoma Cells Release Exosomes Carrying mtDNA. J. Neural Transm. 117 (1), 1–4. doi:10.1007/s00702-009-0288-8
Heo, J. S., Choi, Y., and Kim, H. O. (2019). Adipose-Derived Mesenchymal Stem Cells Promote M2 Macrophage Phenotype through Exosomes. Stem Cells Int. 2019, 7921760. doi:10.1155/2019/7921760
Hu, L., Si, L., Dai, X., Dong, H., Ma, Z., Sun, Z., et al. (2021). Exosomal miR-409-3p Secreted from Activated Mast Cells Promotes Microglial Migration, Activation and Neuroinflammation by Targeting Nr4a2 to Activate the NF-Κb Pathway. J. Neuroinflammation 18 (1), 68. doi:10.1186/s12974-021-02110-5
Johnstone, R. M., Adam, M., Hammond, J. R., Orr, L., and Turbide, C. (1987). Vesicle Formation during Reticulocyte Maturation. Association of Plasma Membrane Activities with Released Vesicles (Exosomes). J. Biol. Chem. 262 (19), 9412–9420. doi:10.1016/s0021-9258(18)48095-7
Kamerkar, S., LeBleu, V. S., Sugimoto, H., Yang, S., Ruivo, C. F., Melo, S. A., et al. (2017). Exosomes Facilitate Therapeutic Targeting of Oncogenic KRAS in Pancreatic Cancer. Nature 546 (7659), 498–503. doi:10.1038/nature22341
Katsur, M., He, Z., Vinokur, V., Corteling, R., Yellon, D. M., and Davidson, S. M. (2021). Exosomes from Neuronal Stem Cells May Protect the Heart from Ischaemia/reperfusion Injury via JAK1/2 and Gp130. J. Cell. Mol. Med. 25 (9), 4455–4465. doi:10.1111/jcmm.16515
Kibria, G., Ramos, E. K., Lee, K. E., Bedoyan, S., Huang, S., Samaeekia, R., et al. (2016). A Rapid, Automated Surface Protein Profiling of Single Circulating Exosomes in Human Blood. Sci. Rep. 6, 36502. doi:10.1038/srep36502
Schiffelers, R., Kooijmans, S., Vader, S. M., van Dommelen, W. W., and Van Solinge, R. M. (2012). Exosome Mimetics: a Novel Class of Drug Delivery Systems. Int. J. Nanomedicine 7, 1525–1541. doi:10.2147/ijn.s29661
Kowal, J., Tkach, M., and Théry, C. (2014). Biogenesis and Secretion of Exosomes. Curr. Opin. Cell. Biol. 29, 116–125. doi:10.1016/j.ceb.2014.05.004
Krämer-Albers, E.-M., Bretz, N., Tenzer, S., Winterstein, C., Möbius, W., Berger, H., et al. (2007). Oligodendrocytes Secrete Exosomes Containing Major Myelin and Stress-Protective Proteins: Trophic Support for Axons? Prot. Clin. Appl. 1 (11), 1446–1461. doi:10.1002/prca.200700522
Lachenal, G., Pernet-Gallay, K., Chivet, M., Hemming, F. J., Belly, A., Bodon, G., et al. (2011). Release of Exosomes from Differentiated Neurons and its Regulation by Synaptic Glutamatergic Activity. Mol. Cell. Neurosci. 46 (2), 409–418. doi:10.1016/j.mcn.2010.11.004
Lai, R. C., Yeo, R. W. Y., and Lim, S. K. (2015). Mesenchymal Stem Cell Exosomes. Seminars Cell. & Dev. Biol. 40, 82–88. doi:10.1016/j.semcdb.2015.03.001
Lamichhane, T. N., Raiker, R. S., and Jay, S. M. (2015). Exogenous DNA Loading into Extracellular Vesicles via Electroporation Is Size-Dependent and Enables Limited Gene Delivery. Mol. Pharm. 12 (10), 3650–3657. doi:10.1021/acs.molpharmaceut.5b00364
Li, W.-Y., Zhu, Q.-B., Jin, L.-Y., Yang, Y., and Xu, X.-Y. (2021). Exosomes Derived from Human Induced Pluripotent Stem Cell-Derived Neural Progenitor Cells Protect Neuronal Function under Ischemic Conditions. Neural Regen. Res. 16 (10), 2064–2070. doi:10.4103/1673-5374.308665
Madrigal, M., Rao, K. S., and Riordan, N. H. (2014). A Review of Therapeutic Effects of Mesenchymal Stem Cell Secretions and Induction of Secretory Modification by Different Culture Methods. J. Transl. Med. 12, 260. doi:10.1186/s12967-014-0260-8
Mead, B., and Tomarev, S. (2017). Bone Marrow-Derived Mesenchymal Stem Cells-Derived Exosomes Promote Survival of Retinal Ganglion Cells through miRNA-Dependent Mechanisms. Stem Cells Transl. Med. 6 (4), 1273–1285. doi:10.1002/sctm.16-0428
Morel, L., Regan, M., Higashimori, H., Ng, S. K., Esau, C., Vidensky, S., et al. (2013). Neuronal Exosomal miRNA-dependent Translational Regulation of Astroglial Glutamate Transporter GLT1. J. Biol. Chem. 288 (10), 7105–7116. doi:10.1074/jbc.M112.410944
Ni, J., Li, H., Zhou, Y., Gu, B., Xu, Y., Fu, Q., et al. (2018). Therapeutic Potential of Human Adipose-Derived Stem Cell Exosomes in Stress Urinary Incontinence - an In Vitro and In Vivo Study. Cell. Physiol. Biochem. 48 (4), 1710–1722. doi:10.1159/000492298
Pan, B.-T., and Johnstone, R. M. (1983). Fate of the Transferrin Receptor during Maturation of Sheep Reticulocytes In Vitro: Selective Externalization of the Receptor. Cell. 33 (3), 967–978. doi:10.1016/0092-8674(83)90040-5
Pettersen, R. D., Hestdal, K., Olafsen, M. K., Lie, S. O., and Lindberg, F. P. (1999). CD47 Signals T Cell Death. J. Immunol. 162 (12), 7031–7040.
Prakoeswa, C. R. S., Rindiastuti, Y., Wirohadidjojo, Y. W., Komaratih, E., NurwasisDinaryati, A., Dinaryati, A., et al. (2020). Resveratrol Promotes Secretion of Wound Healing Related Growth Factors of Mesenchymal Stem Cells Originated from Adult and Fetal Tissues. Artif. Cells, Nanomedicine, Biotechnol. 48 (1), 1159–1166. doi:10.1080/21691401.2020.1817057
Raessi, M., Alijani, H. Q., Nematollahi, F. F., Baty, R. S., El-Saber Batiha, G., Khan, A. U., et al. (2021). Barium Carbonate Nanostructures: Biosynthesis and Their Biomedical Applications. Ceram. Int. 47 (15), 21045–21050. doi:10.1016/j.ceramint.2021.04.106
Ren, R., Tan, X., Lu, Y., Liu, H., Zhang, X., Fu, Y., et al. (2017). Establishment and Identification of the Green Fluorescence Labeled Exosomes in Rat Bone Marrow Mesenchymal Stem Cells. Chin. J. Neuroanat. 33 (02), 167–171. doi:10.16557/j.cnki.1000-7547.2017.02.0010
Ren, R., Tan, X.-H., Zhao, J.-H., Zhang, Q.-P., Zhang, X.-F., Ma, Z.-J., et al. (2019). Bone Marrow Mesenchymal Stem Cell-Derived Exosome Uptake and Retrograde Transport Can Occur at Peripheral Nerve Endings. Artif. Cells, Nanomedicine, Biotechnol. 47 (1), 2918–2929. doi:10.1080/21691401.2019.1640713
Ren, Z. W., Zhou, J. G., Xiong, Z. K., Zhu, F. Z., and Guo, X. D. (2019). Effect of Exosomes Derived from MiR-133b-Modified ADSCs on the Recovery of Neurological Function after SCI. Eur. Rev. Med. Pharmacol. Sci. 23 (1), 52–60. doi:10.26355/eurrev_201901_16747
Roostaee, M., and Sheikhshoaie, I. (2020). Magnetic Nanoparticles; Synthesis, Properties and Electrochemical Application: A Review. Curr. Biochem. Eng. 06 (2). doi:10.2174/2212711906666200316163207
Roostaee, M., Sheikhshoaei, I., and Karimi-Maleh, H. (2022). Fe3O4@Au-rGO Nanocomposite/Ionic Liquid Modified Sensor for Ultrasensitive and Selective Sensing of Doxorubicin. Top. Catal. 65 (1), 1–10. doi:10.1007/s11244-021-01504-2
Salarpour, S., Barani, M., Pardakhty, A., Khatami, M., and Chauhan, N. P. S. (2022). The Application of Exosomes and Exosome-Nanoparticle in Treating Brain Disorders. J. Mol. Liq. 350, 118549. doi:10.1016/j.molliq.2022.118549
Stefanidakis, M., Newton, G., Lee, W. Y., Parkos, C. A., and Luscinskas, F. W. (2008). Endothelial CD47 Interaction with SIRPγ Is Required for Human T-Cell Transendothelial Migration under Shear Flow Conditions In Vitro. Blood 112 (4), 1280–1289. doi:10.1182/blood-2008-01-134429
Tian, T., Zhang, H.-X., He, C.-P., Fan, S., Zhu, Y.-L., Qi, C., et al. (2018). Surface Functionalized Exosomes as Targeted Drug Delivery Vehicles for Cerebral Ischemia Therapy. Biomaterials 150, 137–149. doi:10.1016/j.biomaterials.2017.10.012
Trams, E. G., Lauter, C. J., Norman Salem, Jr., and Heine, U. (1981). Exfoliation of Membrane Ecto-Enzymes in the Form of Micro-vesicles. Biochimica Biophysica Acta (BBA) - Biomembr. 645 (1), 63–70. doi:10.1016/0005-2736(81)90512-5
Valadi, H., Ekström, K., Bossios, A., Sjöstrand, M., Lee, J. J., and Lötvall, J. O. (2007). Exosome-mediated Transfer of mRNAs and microRNAs Is a Novel Mechanism of Genetic Exchange between Cells. Nat. Cell. Biol. 9 (6), 654–659. doi:10.1038/ncb1596
Wahlgren, J., Karlson, T. D. L., Brisslert, M., Vaziri Sani, F., Telemo, E., Sunnerhagen, P., et al. (2012). Plasma Exosomes Can Deliver Exogenous Short Interfering RNA to Monocytes and Lymphocytes. Nucleic Acids Res. 40 (17), e130. doi:10.1093/nar/gks463
Wang, F., Ning, S., Yu, B., and Wang, Y. (2021). USP14: Structure, Function, and Target Inhibition. Front. Pharmacol. 12, 801328. doi:10.3389/fphar.2021.801328
Whiteside, T. L. (2016). Tumor-Derived Exosomes and Their Role in Cancer Progression. Adv. Clin. Chem. 74, 103–141. doi:10.1016/bs.acc.2015.12.005
Willingham, S. B., Volkmer, J. P., Gentles, A. J., Sahoo, D., Dalerba, P., Mitra, S. S., et al. (2012). The CD47-Signal Regulatory Protein Alpha (SIRPa) Interaction Is a Therapeutic Target for Human Solid Tumors. Proc. Natl. Acad. Sci. U. S. A. 109 (17), 6662–6667. doi:10.1073/pnas.1121623109
Xu, K., Jin, Y., Li, Y., Huang, Y., and Zhao, R. (2022). Recent Progress of Exosome Isolation and Peptide Recognition-Guided Strategies for Exosome Research. Front. Chem. 10, 844124. doi:10.3389/fchem.2022.844124
Yang, T., Martin, P., Fogarty, B., Brown, A., Schurman, K., Phipps, R., et al. (2015). Exosome Delivered Anticancer Drugs across the Blood-Brain Barrier for Brain Cancer Therapy in Danio rerio. Pharm. Res. 32 (6), 2003–2014. doi:10.1007/s11095-014-1593-y
Ye, L., Deng, W., Chen, Q., Ke, D., and Li, G. (2014). Construction of CD47 Eukaryotic Expression Vector and Expression of it in Rat Bone Marrow Mesenchymal Stem Cells. Chin. J. Immunol. 30 (09), 1213.
Yin, G., Liu, C., Lin, Y., Xie, Z., Hou, C., and Lin, H. (2018). Effect of Exosomes from Adipose-Derived Stem Cells on Peripheral Nerve Regeneration. Zhongguo Xiu Fu Chong Jian Wai Ke Za Zhi 32 (12), 1592–1596. doi:10.7507/1002-1892.201707051
Yong, S.-B., Song, Y., Kim, H. J., Ain, Q. U., and Kim, Y.-H. (2017). Mononuclear Phagocytes as a Target, Not a Barrier, for Drug Delivery. J. Control. Release 259, 53–61. doi:10.1016/j.jconrel.2017.01.024
Zhang, H.-G., and Grizzle, W. E. (2014). Exosomes: Novel Pathway of Local and Distant Intercellular Communication That Facilitates The Growth And Metastasis of Neoplastic Lesions. Am. J. Pathology 184 (1), 28–41. doi:10.1016/j.ajpath.2013.09.027
Keywords: exosomes, cd47, immune escape, adipose stem cells, phagocytosis, macrophage
Citation: Ben X-Y, Wang Y-R, Zheng H-H, Li D-X, Ren R, Ni P-L, Zhang H-Y, Feng R-J, Li Y-Q, Li Q-F and Yi X-N (2022) Construction of Exosomes that Overexpress CD47 and Evaluation of Their Immune Escape. Front. Bioeng. Biotechnol. 10:936951. doi: 10.3389/fbioe.2022.936951
Received: 05 May 2022; Accepted: 13 June 2022;
Published: 30 June 2022.
Edited by:
Mahmood Barani, Kerman University of Medical Sciences, IranReviewed by:
Narendra Pal Singh Chauhan, Bhupal Nobles University, IndiaMaryam Roostaee, Shahid Bahonar University of Kerman, Iran
Copyright © 2022 Ben, Wang, Zheng, Li, Ren, Ni, Zhang, Feng, Li, Li and Yi. This is an open-access article distributed under the terms of the Creative Commons Attribution License (CC BY). The use, distribution or reproduction in other forums is permitted, provided the original author(s) and the copyright owner(s) are credited and that the original publication in this journal is cited, in accordance with accepted academic practice. No use, distribution or reproduction is permitted which does not comply with these terms.
*Correspondence: Xi-Nan Yi, YiXN001@163.com; Qi-Fu Li, lee-chief@163.com
†These authors have contributed equally to this work