- 1Department of Postgraduates, Shanghai University of Engineering Science, Shanghai, China
- 2School of Mathematics and Information Science, Henan Polytechnic University, Jiaozuo, China
- 3National Engineering Laboratory for Modern Silk, College of Textile and Clothing Engineering, Soochow University, Suzhou, China
- 4School of Textile and Garment, Anhui Polytechnic University, Wuhu, China
Polar bears can live in an extremely cold environment due to their hairs which possess some remarkable properties. The hollow structure of the hair enables the bear to absorb energy from water, and the white and transparent hairs possess amazing optical properties. However, the surface morphology function of bear hairs has been little-studied. Herein, we demonstrate that the micro-structured scales distributed periodically along the hair can absorb maximal radiative flux from the Sun. This polar bear hair effect has the ability for the hair surface not to reflect radiation with a wavelength of about 500 nm. Mimicking the polar bears’ solar performance in the fabrication of nanofibers will certainly stimulate intelligent nanomaterials for efficient solar energy absorption. Therefore, a new technology is discussed in this work for the fabrication of periodic unsmooth nanofibers toward solar energy harvesting.
Introduction
Recently, various devices have been developed for energy harvesting, such as the nanofluids (He and Elazem, 2022), the spring-pendulum systems (Wu et al., 2018; He et al., 2022a), and the low-frequency vibration systems (Zhang and Cai., 2012; He C.-H. et al., 2021; He et al., 2022b). In addition to the abovementioned methods, the nanotechnology for solar energy harvesting (Satharasinghe et al., 2020) is totally new and is quite promising. Though solar energy harvesting has attracted much attention due to the inexhaustible green energy, its efficiency is relatively low. Interestingly, some natural animals have a special ability to absorb solar energy with extremely high efficiency, benefiting from the amazing surface morphology of their hairs, for example, the wolverine (gulo-gulo) hair (Liu et al., 2018).
The polar bear (Ursus maritimus) is the largest predator in the Arctic region. As a kind of marine mammal, the animal is born on land but spends most of its time in the sea to absorb energy from water through its hairs (He et al., 2011; Jia et al., 2017). In order to survive in a harsh environment as low as −50°C in the Arctic, this huge animal has an extensive fat layer of up to 10 cm and bulky furs, which help protect against the cold surrounding. Polar bear fur consists of a layer of dense underfur and an outer layer of guard hairs, which are transparent and white in color (Bechshøft et al., 2012; Dietz et al., 2013). The white hairs contribute to camouflaging the bear in the snow and ice-covered environment (Ferguson et al., 1998; Stegmaier et al., 2009). As a protein fiber, the polar bear hair is not much different in appearance from other protein fibers such as the wool fiber (Fan et al., 2019) and down fiber (Yang et al., 2011). Much attention has been paid to the optical properties (Lavigne and Øritsland, 1974bib_Lavigne_and_Øritsland_1974; Grojean et al., 1980 and 1981; Koon, 1998) and chemical properties of polar bear hairs, and many biomimetic designs were proposed, including thermally insulating fabrics (Cui et al., 2018), textile solar light collectors (Bahners et al., 2008), and polar bear hair–based solar sensors (Tributsch et al., 1990). Many researchers have studied hair cortisol concentration (Mislan et al., 2016), which is considered a biomarker. Furthermore, the morphology and structure, especially the hollow structure of polar bear hairs, have also been studied extensively (Zhang et al., 2009). The fractal theory is a useful tool to reveal its biomechanism (Wang et al., 2015; Wang et al., 2018). However, energy absorption with regards to the scale distribution on polar bear hairs has not been studied yet, and this study intends to state its energy absorption based on the hair’s morphology. Many research studies have revealed that the graphene distribution in a composite affected its properties greatly (Zuo and Liu, 2021; Zuo, 2021). Geometry is always the main factor affecting materials’ properties (He, et al., 2021b) so that the energy absorption property of the polar bear hair.
Materials and Methods
Nano-scale surface morphology greatly affects a surface’s chemistry property (Marmur, 2004; Li X.-X. et al., 2021), friction property (Cao et al., 2021; Bains et al., 2020), and reflection property (Selkowitz 2021). According to the geometric potential theory (Peng and He, 2020; Han and He, 2021), a nano-scale surface can produce high geometric potential. It was reported that Fangzhu’s nano-scale surface can collect water molecules from the air (He and El-Dib, 2021; Wu et al., 2021). Gecko adhesion and the mimicking smart adhesion can also be explained by its nano-scale spatulas (Wang et al., 2019; Li et al., 2020). Here, an experiment is designed to study the nano-scale surface morphology of polar bear hairs.
Experimental Materials
The hair was obtained from a 2-year-old male polar bear in the Laohutan Pole Aquarium in Dalian, China. Polyvinyl alcohol (PVA, 1750 ± 50) was purchased from Sinopharm Chemical Reagent Co., Ltd. (Shanghai, China).
Experimental Instruments
The JEOL JSM-5600LV scanning electron microscope (SEM) with a magnification of 18-300,000 (Japan Electronics Co. Ltd.) and the S-4800 field emission scanning electron microscope (FE-SEM) (Hitachi Ltd., Japan) with resolutions of 1.0 nm (15 kV), 2.0 nm (1 kV), and 1.4 nm (1 kV, Deceleration mode) were used in our experiment. The scale density (scale/mm) and scale thickness on the hair surface were measured through the SEM images.
Experimental Process
First, we rinsed the samples with distilled water to remove debris from their surface. Second, the samples were washed with 0.1 M phosphate buffer three times (15 min per time). Third, the samples were fixed on the sample stage and sprayed in the ion sputter instrument. Finally, the samples were evaluated under the SEM and FE-SEM. In the electrospinning process, the PVA was dissolved in an aqueous solution (8 wt%) at 98°C for 4 h. The spinning parameters were as follows: the voltage was 20 kV, and the collector distance was 25 cm. After the spinning process, the resulting membrane was stretched with a draw ratio of 1.5 times. The draw process was carried out using a universal testing machine (Instron 3,365, Instron, Norwood, MA) (gauge length: 20 mm and cross-head speed: 0.2 mm/s) at 25 ± 0.5°C and 60 ± 5% relative humidity.
Nanoscale Surface Morphology
Before studying the polar bear hair effect, we give a brief introduction to the moth-eye, which can absorb all night light (Wilson and Hutley, 1982). This property is important for the nocturnal insect to escape from predators.
Figure 1 is the schematic diagram of the moth-eye effect. The height of protuberances is about h = 220 nm, and the diameter of the microtrichia is about d = 200 nm.
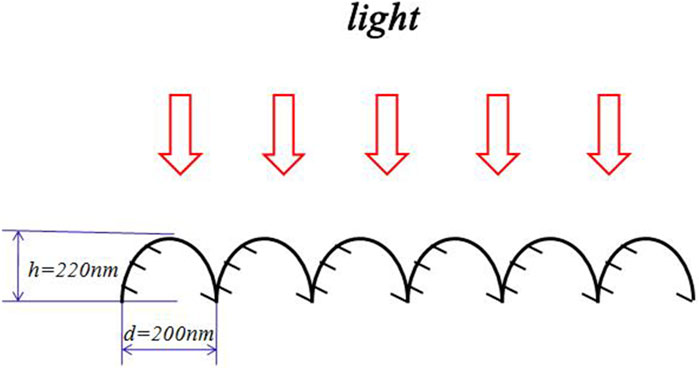
FIGURE 1. Schematic diagram of the moth-eye effect (Nosonovsky and Bhushan,2013).
It was reported that the reflectance is very low for wavelengths
The surface geometry of the moth eye is periodic, and it was used for the biomimetic design of an optically transparent microwave absorber with a periodic array of properly shaped glass caps (Kwon et al., 2021). A similar phenomenon occurs in the polar bear hair, and we carried out an experiment to study the morphology of the hair surface.
Results and Discussion
Figure 2 shows the morphology of the polar bear hair surface structure. Figures 2A–C are SEM images of the same hair at the magnifications × 1,000, × 2,000 and × 5,000, respectively. Figure 3 shows the FE-SEM of the polar bear hair surface structure. As we can see from Figures 2 and 3, the surface of polar bear hair fibers is not smooth, and there is a scale structure similar to the surface of wool fibers. Meanwhile, these figures also show that the polar bear hair fibers were covered with periodic scales in regular shapes. Scale density was relatively small, 70–90 scales/mm, and the scale edges seemed to be wavy or serrated. The scales are thicker at the top, and the scale thickness is about 0.5 µm.
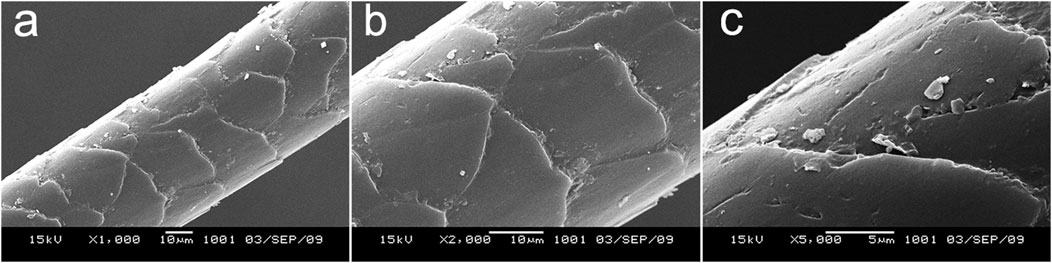
FIGURE 2. Polar bear hair surface structure by SEM with different magnifications (A) ×1,000, (B) ×2,000, and (C) ×5,000.
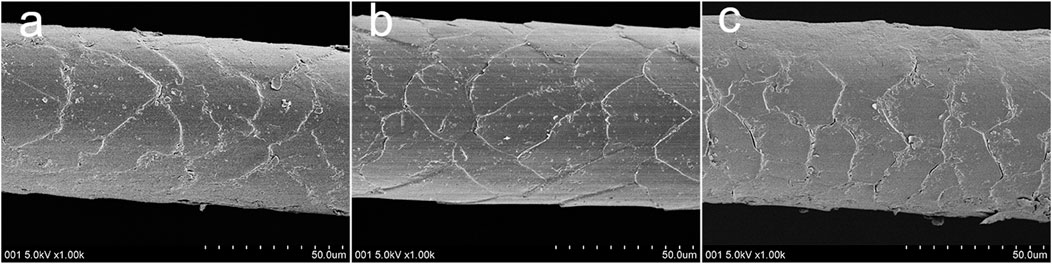
FIGURE 3. Polar bear hair surface structure by FE-SEM (A-C) different locations on the hair surface.
Similar to the moth effect (Nosonovsky and Bhushan, 2013), polar bear hair enables the animal to absorb as much energy as possible from natural light. According to Figures 2 and 3, polar bear hair can be geometrically illustrated, as shown in Figure 4.
The periodicity of the surface morphology of polar bear hair is similar to that of the moth eye and also shows a similar optical property to absorb light energy. Polar bear hairs are white and transparent to convert light energy to its body. The hair surface morphology (Figure 4) can increase transmission and reduce reflection. If the thickness of the scales is almost equal to the light wavelength (Nosonovsky and Bhushan, 2013), the light will not be reflected. Our experimental data reveal that the scale thickness is about 500 nm, corresponding to the spectrum of 500 nm wavelength. According to the laws of radiative heat transfer, the radiative flux from the Sun maximizes at a wavelength of about 0.5 μm (Scamarcio et al., 1997; Thuillier et al., 2003) (Figure 5). The polar bear hair effect is the ability of a micro-structured optical surface not to reflect light with the highest energy.
The general approaches to fabricating smooth nanofibers are electrospinning (Gao et al., 2021; Liu et al., 2021) and bubble electrospinning (He and Qian, 2022; Qian and He, 2022). However, Lin, et al., 2021 suggested a general strategy for the fabrication of unsmooth nanofibers. Yao and He (2020) used the geometric potential theory to control the surface morphology of nanofibers. These references suggested that the unsmooth nanofibers can be fabricated by the electrospinning method. Here, inspired by the natural polar bear, a new technology is discussed for the fabrication of periodic unsmooth nanofibers for solar energy harvesting (Liu et al., 2015). As shown in Figure 6, the resulting PVA nanofibers were endowed with peculiar morphology with a periodic unsmooth surface after being stretched (Figure 6A). The bulges with a diameter of about 80 nm were successfully constructed and periodically spread along the nanofiber axis (Figure 6A), exhibiting a similar appearance to natural bamboo (Figure 6B). The mutational surface morphology from a smooth surface to a bamboo-like unsmooth surface by the stretching method suggests a promising strategy to mimic the polar bear’s solar performance in the fabrication of intelligent nanomaterials for efficient solar energy–absorption.
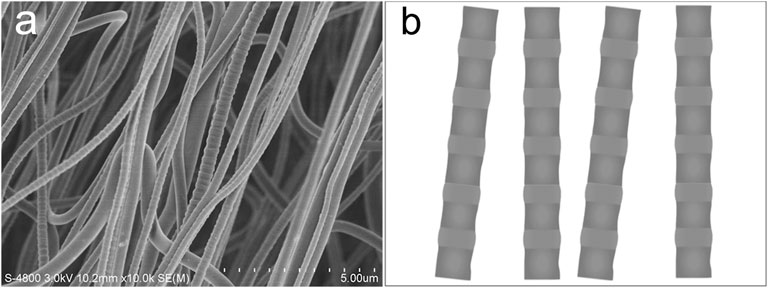
FIGURE 6. (A) Morphology of the periodic unsmooth PVA nanofiber. (B) Schematic diagram of the periodic bamboo-like unsmooth PVA nanofiber.
The nanofibers with a periodic unsmooth surface are potentially of great technological interest for the development of solar energy absorption, and their other applications include invisibility devices, electronic sensors, applied surface science, photonics, physics, microelectronics, nanomaterials, advanced textile, photothermo-promoted nanocatalysis, photothermal semiconduction, photoactivatable cancer immunotherapy, and environmental science (Li et al., 2019; Li X. et al., 2021; Li J. et al., 2021; Yang et al., 2021). We anticipate that this article will be a starting point for more sophisticated studies of intelligent nanomaterials for solar energy harvesting for solar cells (Pavlovic et al., 2021; Alshikhi and Kayfeci 2022) or solar collector systems (Al-Rabeeah et al., 2022). The periodic structure of nanomaterials via bioinspiration for energy gives many promises and great challenges (Gong et al., 2019).
Conclusion
Similar to the moth-eye effect, polar bear hair characteristics along the longitudinal direction were studied by means of SEM and FE-SEM, respectively. The result shows that the micro-structured scales distributed periodically along the hair can absorb maximal radiative flux from the Sun. Mimicking the polar bear’s solar energy harvesting property, we fabricated nanofibers with a periodic unsmooth surface, which exhibits the potential of stimulating intelligent nanomaterials for efficient solar energy absorption. The bio-mimic design of solar energy materials by bubble electrospinning can be used in the infrared stealth technology. Polar bear hair–inspired biomaterials with selective light absorption will attract much attention in the future.
Data Availability Statement
The original contributions presented in the study are included in the article/Supplementary Material; further inquiries can be directed to the corresponding authors.
Author Contributions
J-HH conceptualized the study. QW performed the experiments. J-HH, QW, and ZL analyzed and processed the data. QW and ZL wrote and reviewed the manuscript.
Conflict of Interest
The authors declare that the research was conducted in the absence of any commercial or financial relationships that could be construed as a potential conflict of interest.
Publisher’s Note
All claims expressed in this article are solely those of the authors and do not necessarily represent those of their affiliated organizations, or those of the publisher, the editors, and the reviewers. Any product that may be evaluated in this article, or claim that may be made by its manufacturer, is not guaranteed or endorsed by the publisher.
References
Al-Rabeeah, A. Y., Seres, I., and Farkas, I. (2022). Recent Improvements of the Optical and Thermal Performance of the Parabolic Trough Solar Collector Systems. FU Mech. Eng. 20 (1), 073–094. doi:10.22190/FUME201106030A
Alshikhi, O., and Kayfeci, M. (2022). Experimental Investigation of Using Graphene Nanoplatelets and Hybrid Nanofluid as Coolant in Photovoltaic Thermal Systems. Therm. Sci. 26 (1), 195–208. doi:10.2298/TSCI200524348A
Bahners, T., Schlosser, U., Gutmann, R., and Schollmeyer, E. (2008). Textile Solar Light Collectors Based on Models for Polar Bear Hair. Sol. Energy Mater. Sol. Cells 92 (12), 1661–1667. doi:10.1016/j.solmat.2008.07.023
Bains, P. S., Grewal, J. S., Sidhu, S. S., Kaur, S., and Singh, G. (2020). Surface Modification of Ring-Traveler of Textile Spinning Machine for Substantiality. FU Mech. Eng. 18 (1), 031–042. doi:10.22190/FUME190623005B
Bechshøft, T. Ø., Rigét, F. F., Sonne, C., Letcher, R. J., Muir, D. C. G., Novak, M. A., et al. (2012). Measuring Environmental Stress in East Greenland Polar Bears, 1892-1927 and 1988-2009: What Does Hair Cortisol Tell Us? Environ. Int. 45, 15–21. doi:10.1016/j.envint.2012.04.005
Cao, H., Tian, Y., and Meng, Y. (2021). A Fracture-Induced Adhesive Wear Criterion and its Application to the Simulation of Wear Process of the Point Contacts Under Mixed Lubrication Condition. FU Mech. Eng. 19 (1), 023–038. doi:10.22190/FUME210108021C
Cui, Y., Gong, H., Wang, Y., Li, D., and Bai, H. (2018). A Thermally Insulating Textile Inspired by Polar Bear Hair. Adv. Mat. 30 (14), 1706807. doi:10.1002/adma.201706807
Dietz, R., Rigét, F. F., Sonne, C., Born, E. W., Bechshøft, T., McKinney, M. A., et al. (2013). Three Decades (1983-2010) of Contaminant Trends in East Greenland Polar Bears (Ursus maritimus). Part 1: Legacy Organochlorine Contaminants. Environ. Int. 59, 485–493. doi:10.1016/j.envint.2012.09.004
Fan, J., Yang, X., and Liu, Y. (2019). Fractal Calculus for Analysis of Wool Fiber: Mathematical Insight of its Biomechanism. J. Eng. Fibers Fabr. 14. 1–4. doi:10.1177/1558925019872200
Ferguson, S. H., Taylor, M. K., Born, E. W., and Messier, F. (1998). Fractals, Sea-Ice Landscape and Spatial Patterns of Polar Bears. J. Biogeogr. 25, 1081–1092.
Gao, L., Wang, Y., and Xu, L. (2021). Mechanism Study of Highly Ordered Jets in an Improved Electrospinning Process. Therm. Sci. 25 (3B), 2327–2334. doi:10.2298/TSCI200124122G
Gong, C., Sun, S., Zhang, Y., Sun, L., Su, Z., Wu, A., et al. (2019). Hierarchical Nanomaterials via Biomolecular Self-Assembly and Bioinspiration for Energy and Environmental Applications. Nanoscale 11 (10), 4147–4182. doi:10.1039/C9NR00218A
Grojean, R. E., Sousa, J. A., and Henry, M. C. (1980). Utilization of Solar Radiation by Polar Animals: An Optical Model for Pelts. Appl. Opt. 19 (3), 339–346. doi:10.1364/AO.19.000339
Grojean, R. E., Sousa, J. A., and Henry, M. C. (1981). Utilization of Solar Radiation by Polar Animals: An Optical Model for Pelts; Authors' Reply to an Alternative Explanation. Appl. Opt. 20 (11), 1896–1897. doi:10.1364/AO.20.001896
Han, C., and He, J.-H. (2021). Effect of Fabric Surface's Cleanliness on its Moisture/Air Permeability. Therm. Sci. 25 (2B), 1517–1521. doi:10.2298/TSCI2102517H
He, J. H., and Elazem, N. Y. A. (2022). The Carbon Nanotube-Embedded Boundary Layer Theory for Energy Harvesting. Facta Univ-Ser Mech. 1. 1. doi:10.22190/FUME220221011H
He, J.-H., and El-Dib, Y. O. (2021). Homotopy Perturbation Method with Three Expansions for Helmholtz-Fangzhu Oscillator. Int. J. Mod. Phys. B 35 (24), 2150244. doi:10.1142/S0217979221502441
He, J.-H., Qian, M.-Y., and Li, Y. (2022). The Maximal Wrinkle Angle During the Bubble Collapse and its Application to the Bubble Electrospinning. Front. Mat. 8, 800567. doi:10.3389/fmats.2021.800567
He, J.-H., Wang, Q.-L., and Sun, J. (2011). Can Polar Bear Hairs Absorb Environmental Energy? Therm. Sci. 15 (3), 911–913. doi:10.2298/TSCI1103911H
He, C.-H., Liu, C., He, J.-H., and Gepreel, K. A. (2021a). Low Frequency Property of a Fractal Vibration Model for a Concrete Beam. Fractals 29 (05), 2150117. doi:10.1142/S0218348X21501176
He, C.-H., Liu, S.-H., Liu, C., and Mohammad-Sedighi, H. (2022b). A Novel Bond Stress-Slip Model for 3-D Printed Concretes. Discret Cont Dyn-S 15, 1669. doi:10.3934/dcdss.2021161
He, C.-H., Amer, T. S., Tian, D., Abolila, A. F., and Galal, A. A. (2022a). Controlling the Kinematics of a Spring-Pendulum System Using an Energy Harvesting Device. J. Low Freq. Noise, Vib. Act. Control. 1. 1. doi:10.1177/14613484221077474
He, J.-H., Moatimid, G. M., and Zekry, M. H. (2022b). Forced Nonlinear Oscillator in a Fractal Space. FU Mech. Eng. 20 (1), 001–020. doi:10.22190/FUME220118004H
Jia, H., Guo, J., and Zhu, J. (2017). Comparison of the Photo-Thermal Energy Conversion Behavior of Polar Bear Hair and Wool of Sheep. J. Bionic Eng. 14 (4), 616–621. doi:10.1016/S1672-6529(16)60427-4
Koon, D. W. (1998). Is Polar Bear Hair Fiber Optic? Appl. Opt. 37 (15), 3198–3200. doi:10.1364/AO.37.003198
Kwon, H., D’Aguanno, G., and Alú, A. (2021). Optically Transparent Microwave Absorber Based on Water-Based Moth-Eye Structures. Opt. Express 29 (6), 9190–9198. doi:10.1364/oe.418220
Lavigne, D. M., and Øritsland, N. A. (1974). Black Polar Bears. Nature 251 (5472), 218–219. doi:10.1038/251218b0
Li, J., Cui, D., Huang, J., He, S., Yang, Z., Zhang, Y., et al. (2019). Organic Semiconducting Pro‐Nanostimulants for Near‐Infrared Photoactivatable Cancer Immunotherapy. Angew. Chem. Int. Ed. 58, 12680–12687. doi:10.1002/ange.20190628810.1002/anie.201906288
Li, X., Li, Y., Li, Y., and He, J. (2020). Gecko-Like Adhesion in the Electrospinning Process. Results Phys. 16, 102899. doi:10.1016/j.rinp.2019.102899
Li, X.-X., Tian, D., and He, J.-H. (2021a). High Energy Surface as a Receptor in Electrospinning: A Good Switch for Hydrophobicity to Hydrophilicity. Therm. Sci. 25 (3), 2205–2212. doi:10.2298/TSCI191120107L
Li, X., Sun, H., Li, H., Hu, C., Luo, Y., Shi, X., et al. (2021b). Multi‐Responsive Biodegradable Cationic Nanogels for Highly Efficient Treatment of Tumors. Adv. Funct. Mater. 31 (26), 2100227. doi:10.1002/adfm.202100227
Li, J., Yu, X., Jiang, Y., He, S., Zhang, Y., Luo, Y., et al. (2021c). Second Near‐Infrared Photothermal Semiconducting Polymer Nanoadjuvant for Enhanced Cancer Immunotherapy. Adv. Mat. 33 (4), 2003458. doi:10.1002/adma.202003458
Lin, L., Liu, Y.-Q., Li, Y.-Y., Shen, Y., and He, J.-H. (2021). Fabrication of PVDF/PES Nanofibers with Unsmooth Fractal Surfaces by Electrospinning: A General Strategy and Formation Mechanism. Therm. Sci. 25, 1287–1294. doi:10.2298/TSCI191201024L
Liu, Z., Sun, Q.-L., and He, J.-H. (2015). Fabrication of Unsmooth Bamboo-Like Nanofibers. Therm. Sci. 19 (4), 1450–1451. doi:10.2298/TSCI1504450L
Liu, H., Bahi, A., and Ko, F. K. (2018). A One Dimensional Heat Transfer Model for Wolverine (Gulo-Gulo) Hair. Ijcst 30, 548–558. doi:10.1108/IJCST-08-2017-0108
Liu, L., Liu, Y.-Q., Li, Y.-Y., Shen, Y., and He, J.-H. (2021). Dropping in Electrospinning Process: A General Strategy for Fabrication of Microspheres. Therm. Sci. 25, 1295–1303. doi:10.2298/TSCI191228025L
Marmur, A. (2004). The Lotus Effect: Superhydrophobicity and Metastability. Langmuir 20 (9), 3517–3519. doi:10.1021/la036369u
Mislan, P., Derocher, A. E., St. Louis, V. L., Richardson, E., Lunn, N. J., and Janz, D. M. (2016). Assessing Stress in Western Hudson Bay Polar Bears Using Hair Cortisol Concentration as a Biomarker. Ecol. Indic. 71, 47–54. doi:10.1016/j.ecolind.2016.06.034
Nosonovsky, M., and Bhushan, B. (2013). Multiscale Dissipative Mechanisms and Hierarchical Surfaces: Friction, Superhydrophobicity, and Biomimetics. Beijing, China: Peking University Press.
Pavlovic, A., Fragassa, C., Bertoldi, M., and Mikhnych, V. (2021). Thermal Behavior of Monocrystalline Silicon Solar Cells: A Numerical and Experimental Investigation on the ModuleEncapsulation Materials. J. Appl. Comput. Mech. 7 (3), 1847–1855. doi:10.22055/JACM.2021.37852.3101
Peng, N.-b., and He, J.-h. (2020). Insight into the Wetting Property of a Nanofiber Membrane by the Geometrical Potential. Nanotec. 14 (1), 64–70. doi:10.2174/1872210513666191120104149
Qian, M.-Y., and He, J.-H. (2022). Collection of Polymer Bubble as a Nanoscale Membrane. Surfaces Interfaces 28, 101665. doi:10.1016/j.surfin.2021.101665
Satharasinghe, A., Hughes-Riley, T., and Dias, T. (2020). A Review of Solar Energy Harvesting Electronic Textiles. Sensors 20 (20), 5938. doi:10.3390/s20205938
Scamarcio, G., Capasso, F., Sirtori, C., Faist, J., Hutchinson, A. L., Sivco, D. L., et al. (1997). High-Power Infrared (8-Micrometer Wavelength) Superlattice Lasers. Science 276 (5313), 773–776. doi:10.1126/science.276.5313.773
Selkowitz, S. (2021). Reflections on Surface Morphology. Nat. Energy 6 (5), 456–457. doi:10.1038/s41560-021-00835-4
Stegmaier, T., Linke, M., and Planck, H. (2009). Bionics in Textiles: Flexible and Translucent Thermal Insulations for Solar Thermal Applications. Phil. Trans. R. Soc. A 367, 1749–1758. doi:10.1098/rsta.2009.0019
Thuillier, G., Hersé, M., Foujols, T., Peetermans, W., Gillotay, D., Simon, P. C., et al. (2003). The Solar Spectral Irradiance from 200 to 2400 Nm as Measured by the SOLSPEC Spectrometer from the ATLAS and EURECA Missions. Sol. Phys. 214 (1), 1–22. doi:10.1023/A:1024048429145
Tributsch, H., Goslowsky, H., Küppers, U., and Wetzel, H. (1990). Light Collection and Solar Sensing Through the Polar Bear Pelt. Sol. Energy Mat. 21 (2-3), 219–236. doi:10.1016/0165-1633(90)90056-7
Wang, Q.-L., Li, Z.-B., Kong, H.-Y., and He, J.-H. (2015). Fractal Analysis of Polar Bear Hairs. Therm. Sci. 19, 143–144. doi:10.2298/TSCI15S1S43W
Wang, Q., Shi, X., He, J.-H., and Li, Z. B. (2018). Fractal Calculus and its Application to Explanation of Biomechanism of Polar Bear Hairs. Fractals 26 (06), 1850086. doi:10.1142/S0218348X1850086X
Wang, C., Xu, L., Liu, G., Ren, Y., Lv, J., Gao, D., et al. (2019). Smart Adhesion by Surface Treatment Experimental and Theoretical Insights. Therm. Sci. 23 (4), 2355–2363. doi:10.2298/TSCI1904355W
Wilson, S. J., and Hutley, M. C. (1982). The Optical Properties of ‘Moth Eye’ Antireflection Surfaces. Opt. Acta Int. J. Opt. 29 (7), 993–1009. doi:10.1080/713820946
Wu, Y., Qiu, J., Zhou, S., Ji, H., Chen, Y., and Li, S. (2018). A Piezoelectric Spring Pendulum Oscillator Used for Multi-Directional and Ultra-Low Frequency Vibration Energy Harvesting. Appl. Energy 231, 600–614. doi:10.1016/j.apenergy.2018.09.082
Wu, P., Ling, W., Li, X., He, X., and Xie, L. (2021). Dynamics Research of Fangzhu's Nanoscale Surface. J. Low Freq. Noise, Vib. Act. Control 41, 479–487. doi:10.1177/14613484211052753
Shu Yang, S., Weidong Yu, W., and Ning Pan, N. (2011). Fractal Phenomenon in Sound Absorbing Behavior of Down Fiber Assembly. Text. Res. J. 81 (11), 1139–1144. doi:10.1177/0040517511398949
Yang, Z., Luo, Y., Hu, Y., Liang, K., He, G., Chen, Q., et al. (2021). Photothermo‐Promoted Nanocatalysis Combined with H 2 S‐Mediated Respiration Inhibition for Efficient Cancer Therapy. Adv. Funct. Mat. 31 (8), 2007991. doi:10.1002/adfm.202007991
Yao, X., and He, J.-H. (2020). On Fabrication of Nanoscale Non-Smooth Fibers with High Geometric Potential and Nanoparticle's Non-Linear Vibration. Therm. Sci. 24 (4), 2491–2497. doi:10.2298/TSCI2004491Y
Zhang, Y., and Cai, C. S. (2012). A Retrofitted Energy Harvester for Low Frequency Vibrations. Smart Mat. Struct. 21 (7), 075007. doi:10.1088/0964-1726/21/7/075007
Zhang, H. J., Chen, Z., Zhu, Y. L., Du, Z. Q., and Yu, W. D. (2009). Characterization for Hair Morphology and Structure of Cold-Resistant Animals. J. Xi’an Polytech. Univ. 23 (2), 333–339. doi:10.13338/j.issn.1674-649x.2009.02.073
Zuo, Y.-T., and Liu, H.-J. (2021). Fractal Approach to Mechanical and Electrical Properties of Graphene/Sic Composites. FU Mech. Eng. 19 (2), 271–284. doi:10.22190/FUME201212003Z
Keywords: biomimetic, polar bear hair, energy absorption, selective light absorption, moth-eye effect, bubble electrospinning
Citation: Wang Q, He J-H and Liu Z (2022) Intelligent Nanomaterials for Solar Energy Harvesting: From Polar Bear Hairs to Unsmooth Nanofiber Fabrication. Front. Bioeng. Biotechnol. 10:926253. doi: 10.3389/fbioe.2022.926253
Received: 22 April 2022; Accepted: 16 May 2022;
Published: 25 July 2022.
Edited by:
Yu Luo, Shanghai University of Engineering Sciences, ChinaReviewed by:
Jinfa Ming, Qingdao University, ChinaJamal Zare, Shahrekord University, Iran
Mohammad Malikan, Gdansk University of Technology, Poland
Copyright © 2022 Wang, He and Liu. This is an open-access article distributed under the terms of the Creative Commons Attribution License (CC BY). The use, distribution or reproduction in other forums is permitted, provided the original author(s) and the copyright owner(s) are credited and that the original publication in this journal is cited, in accordance with accepted academic practice. No use, distribution or reproduction is permitted which does not comply with these terms.
*Correspondence: Ji-Huan He, aGVqaWh1YW5Ac3VkYS5lZHUuY24=; Zhi Liu, bGl1emhpQGFocHUuZWR1LmNu