- 1Department of Clinical Laboratory, Xiangya Hospital of Central South University, Changsha, China
- 2National Clinical Research Center for Geriatric Diseases, Xiangya Hospital of Central South University, Changsha, China
- 3Hunan Key Laboratory for Super Microstructure and Ultrafast Process, School of Physics and Electronics, Central South University, Changsha, China
- 4Department of Microbiology, School of Basic Medical Science, Central South University, Changsha, China
The infection of Staphylococcus aureus (S.aureus) and the spread of drug-resistant bacteria pose a serious threat to global public health. Therefore, timely, rapid and accurate detection of S. aureus is of great significance for food safety, environmental monitoring, clinical diagnosis and treatment, and prevention of drug-resistant bacteria dissemination. Traditional S. aureus detection methods such as culture identification, ELISA, PCR, MALDI-TOF-MS and sequencing, etc., have good sensitivity and specificity, but they are complex to operate, requiring professionals and expensive and complex machines. Therefore, it is still challenging to develop a fast, simple, low-cost, specific and sensitive S. aureus detection method. Recent studies have demonstrated that fast, specific, low-cost, low sample volume, automated, and portable aptasensors have been widely used for S. aureus detection and have been proposed as the most attractive alternatives to their traditional detection methods. In this review, recent advances of aptasensors based on different transducer (optical and electrochemical) for S. aureus detection have been discussed in details. Furthermore, the applications of aptasensors in point-of-care testing (POCT) have also been discussed. More and more aptasensors are combined with nanomaterials as efficient transducers and amplifiers, which appears to be the development trend in aptasensors. Finally, some significant challenges for the development and application of aptasensors are outlined.
Introduction
S.aureus is one of the most pathogenic pathogens in the world and it was first discovered and named by Dr. Alexander Ogston in 1880 (Ogston, 1882). S. aureus is a major cause of foodborne poisoning (Hulme, 2017), with more than 240,000 cases annually in the United States, and it is a major public health concern (Zhang et al., 2019). S. aureus is also the most common pathogen in purulent infections in humans, and can enter any organ or enter the bloodstream when the host immunity is weakened or the skin and mucosal barriers are disrupted. It can cause skin and soft tissue infections (impetigo, folliculitis and scalded skin syndrome), and severe systemic diseases such as bacteremia, endocarditis, osteomyelitis, hemolytic pneumonitis, and toxic shock syndrome (Tong et al., 2015). Mild skin and mucosal infections are usually self-limiting, while severe systemic infections are usually associated with high mortality (20∼50%), high recurrence rate (5∼10%), and persistent injury (more than three points out of the survivors) (Kern and Rieg, 2020). Methicillin-resistant S. aureus (MRSA) has been a serious threat to global public health since it was first described in 1961 (Jevons, 1961). About 40∼60% of nosocomial S. aureus infections are MRSA in developed countries such as the United States, Europe and Japan (Fluit et al., 2001), and the MRSA infection rate is higher in developing countries (>70%), probably due to the widespread and improper use of antibiotics, and the spread of drug-resistant bacteria (Chen and Huang, 2014).
The rapid and accurate detection of S. aureus is of great significance to food safety, environmental monitoring, clinical diagnosis and treatment, and prevention of the spread of drug-resistant bacteria. The gold standard for detecting S. aureus is still culture identification, the results are accurate and reliable, economical and simple, but it is very time-consuming, usually taking 1∼2 days to form visible colonies on agar plates, and then 1∼2 days for biochemical identification and serological typing. In recent years, several rapid and automated detection methods have been developed, such as enzyme-linked immunosorbent assay (ELISA) (Nouri et al., 2018), polymerase chain reaction (PCR) (Umesha and Manukumar, 2018), next-generation sequencing and matrix-assisted laser desorption/ionization time-of-flight mass spectrometry (MALDI-TOF-MS) (Gill et al., 2019; Rajapaksha et al., 2019), et al. But they are complex to operate, requiring professionals and expensive and complex machines. Therefore, it is still a hot issue to develop a fast, simple, low-cost, specific and sensitive S. aureus detection method.
In the past two decades, biosensors have been more and more widely used in pathogen detection due to fast, low cost, high sensitivity, low sample volume, automation and portability, and have been proposed as the most promising alternative to traditional pathogen detection methods (Furst and Francis, 2019; Cesewski and Johnson, 2020). The biosensor is mainly composed of three parts: bioreceptor, transducer and signal readout system (Yu et al., 2021). Biorecognition element refers to the “biological receptor” that can recognize the target with strong affinity and high specificity, and is the most critical part of the biosensor, which determines the specificity (selectivity) of the biosensor (Morales and Halpern, 2018). Antibodies, peptides and bacteriophages are the most commonly used biorecognition elements, among which antibodies are considered to be the gold standard recognition element in biosensors due to their high affinity and specificity between antibodies and antigens (Sharma et al., 2016). In the past few decades, antibody technology has developed rapidly and has made great contributions to the development of medicine and the entire life sciences. However, there are still many limitations in the application of antibodies such as the antibody development process is cumbersome and complicated and it is very challenging to generate antibodies that recognize small molecule targets (Zhou and Rossi, 2017; Liu et al., 2021a). Aptamers are another class of biorecognition elements that have attracted great interest in biosensing in recent years, and are known as “chemical antibodies” due to their ability to interact with their targets with strong affinity and high specificity similar to antigen-antibody interactions (Banerjee, 2010).
Aptamer is a specific oligonucleotide sequence (most commonly single-stranded DNA or RNA), usually ranging in length from 25 to 90 bases, and their binding to the target is based on the diversity of single-stranded nucleic acid structure and spatial conformation (Tok et al., 2000). In the presence of the target, aptamers pass through the pairing and electrostatic interactions between complementary bases in the chain, and self-adaptive folding into different types of secondary structures, including stems (Tok et al., 2000), inner loops, purine-rich bulges, hairpins, pseudoknots (Tuerk et al., 1992), kissing complexes (Boiziau et al., 1999), and G-quadruplex (Bock et al., 1992). Subsequently, these secondary structures assemble to form unique three-dimensional (3D) structures, resulting in strong affinity and high specificity for binding to target. Aptamers can efficiently and specifically attach to a wide range of targets, including small molecules, ions, peptides, proteins, viruses, bacteria and even cells (Shen et al., 2014). The affinity dissociation constants (Kd) of the aptamers with their cognate ligand are generally 1 pM∼1 mM, most of them are between 1 and 10 nM, which is much larger than that of the related non-cognate ligand, indicating that aptamers have good specificity (Alizadeh et al., 2017). RNA-type oligonucleotide aptamers were fist found by Tuerk and Ellington in 1990 by phylogenetic evolution of ligands with exponential enrichment (SELEX) (Ellington and Szostak, 1990; Tuerk and Gold, 1990). Conventional SELEX consists of multiple cycles, each cycle including 5 main steps as shown in Figure 1: synthesis, binding, isolation, elution and amplification (Hamula et al., 2011; Teng et al., 2016). Since conventional SELEX is a very time-consuming process, many methods have been introduced in the past few decades to generate higher efficiency and reliability aptamers, such as Cell-SELEX (Dwivedi et al., 2013; Amraee et al., 2017; Li et al., 2018; He et al., 2019), Genomic SELEX (Lorenz et al., 2006), IP-SELEX (Wang et al., 2013), Capture-SELEX (Reinemann et al., 2016), CE-SELEX (Mendonsa and Bowser, 2004; Berezovski et al., 2006a; Berezovski et al., 2006b), M-SELEX (Lou et al., 2009), AFM-SELEX (Miyachi et al., 2010), AEGIS-SELEX (Sefah et al., 2014), Animal-SELEX (Cheng et al., 2013a), etc.
Aptamer-based biosensors are widely used in the rapid detection of S. aureus due to their fast, specific, low cost, low sample volume, automated, and portable. The commonly used aptamer sequences against S. aureus by SELEX technique are shown in Table 1. Therefore, this paper focuses on the latest progress of aptasensors based on different transducer (optical and electrochemical) for S. aureus detection. The detection principle, linear range, sensitivity and detection time of aptasensors are presented in Table 2. Furthermore, the applications of aptasensors in POCT have also been discussed. This review also highlights that more and more nanomaterials have been used as immobilized recognition elements or amplified detection signals to improve the sensitivity of aptasensors. Finally, some significant challenges for the development and application of aptasensors are outlined.
Aptasensors Based on Optical Transduction
Optical biosensors have been widely used in the detection of S. aureus due to simple, fast, low cost, high sensitivity, real-time monitoring capability and label-free possibility. Optical biosensors can be classified according to transduction mechanisms such as absorption, scattering, diffraction, reflection, refraction and luminescence (photoluminescence, chemiluminescence, electrochemiluminescence or bioluminescence) (Paniel et al., 2013). Colorimetric, fluorescence, chemiluminescence, surface plasmon resonance (SPR) and surface-enhanced Raman scattering (SERS) are the most commonly used optical techniques (Rubab et al., 2018).
Colorimetric-Based Aptasensor
Colorimetric is the simplest and oldest biosensors. It can detect the target by detecting the color change of the solution with the naked eye or a simple instrument such as a UV-Vis spectrophotometer. It has been widely used to monitor various targets due to its simplicity, stability and low cost (Wang et al., 2018). The key technology of colorimetric is the selection of catalytic enzymes and corresponding chromogenic substrates. Traditional catalytic enzymes such as horseradish peroxidase (HRP) show high substrate specificity and catalytic efficiency, and its substrates are usually 3,3',5,5'-tetramethylbenzidine (TMB) and 2,2'-azino-bis (3-ethylbenzothiazoline-6-sulfonic acid) (ABTS). Yuan et al. constructed a colorimetric-based aptasensor combining aptamer, HRP and tyramide signal amplification technology for the detection of S. aureus (Yuan et al., 2014a). With the help of tyramide signal amplification technology, the limit of detection (LOD) can reach to 8 CFU/ml, and the linear range is 10∼107 CFU/ml (Yuan et al., 2014a). G-quadruplex (G4) is a DNase with a steric configuration, which has been widely used in biosensors due to its ability to combine with hemin to form a DNA-mimicking enzyme with catalase activity. Xu et al. established an ultrasensitive colorimetric-based aptasensor for S. aureus detection by using nickase amplification reaction (NEAR), rolling circle cycle amplification technology (RCA) and G4/hemin complex (Xu et al., 2018). The results showed that the established biosensor exhibited good discrimination between live and dead S. aureus in addition to good specificity, low detection limit (5 CFU/ml), and wide linear range (5∼104 CFU/ml) (Xu et al., 2018).
Although HRP and G4 show high substrate specificity and catalytic efficiency, they also have disadvantages such as difficulty in storage, poor stability, and catalytic activity is easily affected by external conditions (such as pH). Since Woo discovered that Fe3O4 nanoparticles exhibited peroxidase activity in 2013 (Woo et al., 2013), Fe3O4 nanoparticles have received great attention due to their outstanding peroxidase-like activity, physicochemical stability, low cost, non-toxicity, and magnetic properties that can be used for enrichment and isolation of targets. Coating metal catalysts on the surface of magnetic nanoparticles to form hybrid nanocomposites can not only avoid the oxidation of Fe3O4 nanoparticles, but also significantly improve their catalytic performance (Zhang et al., 2021a; Zhang et al., 2021b). Zhang et al. synthesized a gold-coated Fe3O4 nanocomposites to construct a colorimetric-based aptasensor for one-step detection of S. aureus in water, urine and milk samples. The detection process does not require enrichment, separation or washing steps, and the results can be obtained by naked eye in 12 min. Under the optimal reaction conditions, the LOD is 10 CFU/ml, and the linear range is 10∼106 CFU/ml (Zhang et al., 2021b). Besides Fe3O4 nanoparticles, a variety of metal nanoparticles have also been synthesized as peroxide analogs in recent years, such as CeO2 NPs, Cu-MOF NPs, Co3O4 NPs, ZnFe2O4NPs (Su et al., 2012; Wang et al., 2017a; Li et al., 2017b; Cheng et al., 2019).
There is also a class of enzyme-free colorimetric-based aptasensors, such as gold and silver metal nanoparticles, which are widely used as signal indicators due to their size- and distance-dependent optical properties (Mokhtarzadeh et al., 2015; Taghdisi et al., 2016). The color of AuNPs solution will change from red to blue or purple when the well-dispersed AuNPs aggregate under certain conditions, which was caused by the change of the surface resonance frequency of the AuNPs. Due to the high extinction coefficient of AuNPs, the sensitivity of colorimetric sensors based on AuNPs is higher than that of organic dyes (Ghosh and Pal, 2007). Chang et al. constructed an sensor based on aptamer and AuNPs for accurate identification of S. aureus from common pathogens (Chang et al., 2016). In the presence of S. aureus, the aptamer was removed by binding to S. aureus. Then the AuNPs aggregated when AuNPs and high salt were added, and the color of AuNPs solution will turned to blue or purple. In the absence of S. aureus, the color of the AuNPs solution was red because the unscavenged aptamers can adsorbed on the surface of AuNPs and protected AuNPs from ion-induced aggregation (Chang et al., 2016). Zhang et al. discovered that the dsDNA-SYBR Green I (SG I) complex has photocatalytic activity for the first time, which can utilize dissolved oxygen to catalyze the oxidation of the substrate TMB under light irradiation (Zhang et al., 2015b). Compared with HRP and G4, dsDNA-SG I complex has obvious advantages, such as simplicity, high sensitivity and label-free. Yu et al. constructed a colorimetric-based photoirradiation aptasensor for detection of S. aureus based on catalysis of dsDNA-SG I complex (Figure 2). The results shown that the biosensor could specifically, sensitively, rapidly and quantitatively detect S. aureus. In addition, this method can be used for high throughput analysis as it can detect 96 samples at once (Yu et al., 2020).
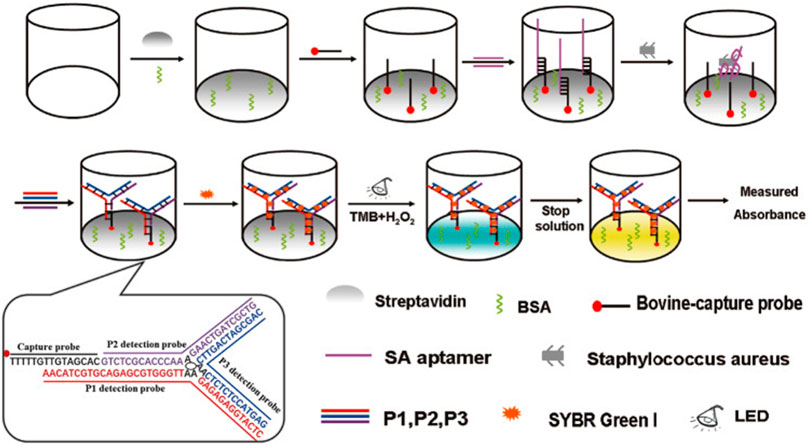
FIGURE 2. Schematic illustration of the principle for detection of S. aureus with aptamer-high throughput colorimetric biosensor based on photocatalytic activity of dsDNA-SG I complex. Reproduced with permission (Yu et al., 2020).
Fluorescence-Based Aptasensor
Fluorescence-based aptasensors mainly trigger or inactivate the fluorescence emission properties of fluorescent dyes or fluorescent nanomaterials through the interaction of aptamers with targets. Fluorescence-based aptasensors have become one of the most commonly used sensors for low-concentration analyte detection due to the advantages of the higher sensitivity, wider detection range and multiplex detection as compared with colorimetric-based aptasensors. It is relatively easy to construct distance-dependent fluorescence resonance energy transfer (FRET) aptasensors because aptamers can be easily modified with fluorophores or/and quenching dyes at the 3' or 5' end without affecting their binding affinity to the target. Traditional organic fluorescent dyes mainly include carboxyfluorescein (FAM), fluorescein isothiocyanate (FITC) and acridine orange, which have been widely used for a long time (Paniel et al., 2013). Lu et al. proposed an enzymatic cleavage aptasensor in which the aptamer act as biological recognition element for S. aureus in complex samples. The two ends of the molecular beacon were labeled with FAM (fluorophore) and BHQ1 (quencher) respectively, and can hybridize stably with aptamers. The aptamer can avoid being cleaved by exonuclease (Exonuclease I and III, Exo. I and III) when binging to S. aureus due to the enzymatic protection effect of aptamer-pathogen complex. The unscavenged aptamer then combined with the molecular beacon, which separated the fluorophore from the quencher, thereby restoring the fluorescence (Lu et al., 2020b). This detection system can be completed in the same tube without culture or separation, but its sensitivity (64 CFU/ml) need to be improved (Lu et al., 2020b).
DNA amplification systems such as rolling cycle amplification (RCA), strand displacement reaction (SDA) (Cai et al., 2020), hybridization Chain reaction (HCR) (Yang et al., 2021b) and hairpin DNA cascade hybridization reaction (HD-CHR) are often used to improve the sensitivity of aptasensor. The SDA is an isothermal amplification that does not require thermal cycling, which can exponentially expand the target sequence in less than 15 min (Wang et al., 2017b). Cai et al. designed an aptasensor for S. aureus detection based on functional chimera sequence, molecular beacon and SDA. The functional chimera sequence contains the S. aureus aptamer sequence and the molecular beacon complementary sequence, and it can form a hairpin structure. The molecular beacon was labeled with FAM (fluorophore) and DABCYL (quencher) in the end respectively. The distance between FAM and DABCYL in the hairpin structure of the molecular beacon is narrow, and the fluorescence signal of FAM is quenched by DABCYL. When the aptamer in the functional chimera sequence binds to S. aureus, the molecular beacon complementary sequence will be exposed and bound to the molecular beacon, keeping the FAM away from the DABCYL, and thereby emitting fluorescence (Figure 3A). The established aptasensor displays good detection limit (39 CFU/ml), wide linear range (8∼8 × 108 CFU/ml), satisfactory recovery and repeatability (Cai et al., 2019). However, the use of traditional organic fluorescent dyes in sensors is limited due to low fluorescence intensity and rapid photobleaching. Compared with traditional organic fluorescent dyes, fluorescent nanomaterials such as quantum dots (QDs) (Fu et al., 2020; Tao et al., 2021), upconversion nanoparticles (UCNPs) (Wu et al., 2014; Ouyang et al., 2021b) and carbon dots (Cui et al., 2019; Pebdeni et al., 2020) that have emerged in recent years have many advantages, such as wider absorption spectra, size-tunable narrower emission spectra and better stability (Yao et al., 2014).
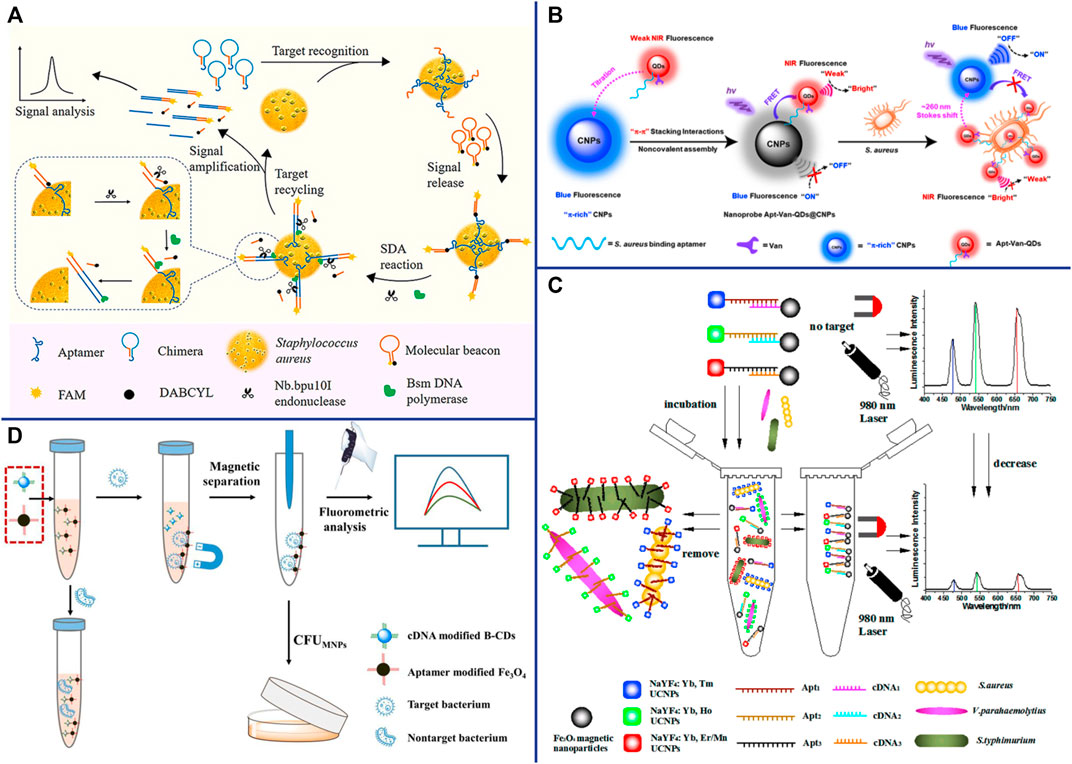
FIGURE 3. (A) Schematic representation of the fluorescent detection of S. aureus based on a finely designed functional chimera sequence, a molecular beacon (MB), and strand displacement target recycling. Reproduced with permission (Cai et al., 2019). (B) General design of the vancomycin and aptamer dual-recognition moieties-based ratiometric fluorescent nanoprobe with a remarkably large Stokes shift for ultrafast and accurate management of S. aureus at the single-cell level. Reproduced with permission (Shen et al., 2020). (C) Schematic illustration of the multiplexed luminescence bioassay based on aptamers-modified UCNPs for the simultaneous detection of various pathogenic bacteria. Reproduced with permission (Wu et al., 2014). (D) Schematic illustration of the fluorometric aptasensor for high-sensitivity S. aureus detection based on FRET of the self-assembled dimer, B-CD/Fe3O4. Reproduced with permission (Cui et al., 2019).
QDs are nanoscale semiconductors (1∼20 nm in diameter), which can be effectively excited by any wavelength shorter than the emission peak and emit a narrow and symmetrical characteristic spectrum that varies with the size of the QD. Since QDs of different sizes can be excited by the same wavelength and emit different emission peaks, Wang et al. achieved the simultaneous detection of Escherichia coli O157:H7, S. aureus and Vibrio parahaemolyticus by modifying different aptamers with QDs of different sizes (Wang et al., 2020). Shen et al. constructed a ratiometric FRET-based aptasensor which involves target-induced emission intensity changes at two or more different wavelengths to reduces interference from various target-independent factors (Shen et al., 2020). They employed novel π-electron-rich carbon nanoparticles (CNPs) with high photostability and blue fluorescence as energy donors, and bilayer-modified QDs with aptamers and vancomycin as energy acceptors (Apt-Van-QDs). Due to the π−π stacking interaction, the Apt-Van-QDs will bind to the surface of π-rich CNPs, thereby promoting FRET between QDs and CNPs, and resulting in strong blue fluorescence quenching at ∼465 nm for the CNPs and fluorescence enhancement at ∼725 nm for Apt-Van-QDs (Shen et al., 2020). The FRET between QDs and CNPs was disrupted and exhibited a large Stokes shift of ∼260 nm when Apt-Van-QDs binds to S. aureus and moves away from CNPs (Figure 3B). The dual-recognition ratiometric fluorescent nanosensor showed an ultrahigh specificity and can detect a single bacteria with 30 min (Shen et al., 2020). The use of a suitable donor-acceptor is crucial to improve the efficiency of FRET. AuNPs serve as excellent energy acceptors for ultrasensitive molecular detection due to easy synthesis, good optical and colloidal stability, and high extinction coefficient. Fu et al. established a dual-recognition FRET-based aptasensor for the detection of S. aureus by using aptamer-modified QDs as donors and teicoplanin-modified AuNPs as acceptors. This dual-recognition FRET-based aptasensor can detect a single S. aureus in cells, providing a simple, specific, sensitive and rapid diagnostic method for the detection of intracellular bacteria (Fu et al., 2020). QDs are typically extracted from a mixture of lead, cadmium and silicon that are often toxic and environmentally hazardous, which limiting their applications.
Lanthanide-doped NIR-to-visible UCNPs are able to emit intense visible light upon excitation by NIR (usually 980 nm), which is not absorbed by biological samples and does not cause autofluorescence and light scattering background (Wang et al., 2010; Cheng et al., 2013b). The optical properties of UCNPs can be tuned by changing their lanthanide dopants (including Er3+, Tm3+, and Ho3+) (Liu et al., 2013). Wu et al. constructed a sensitive and specific biosensor based on multicolor UCNPs as luminescent labels and aptamers as biological recognition elements for simultaneous detection of S. aureus, Vibrio parahaemolyticus and Salmonella typhimurium (Figure 3C). Under the optimal reaction conditions, the LOD of the sensor was 25 CFU/ml and the linear range was 50∼106 CFU/ml (Wu et al., 2014). AuNPs are considered as efficient fluorescence quenchers due to their large surface-to-volume ratio and strong surface plasmon absorption in the NIR and IR (Zhu et al., 2010). Taking advantage of the high fluorescence quenching effect of AuNPs, Ouyang et al. established a more sensitive FRET-based aptasensor for the detection of S. aureus. Aptamer-complementary sequence-modified UCNPs were used as the donor and aptamer-modified AuNPs were used as the acceptor. The fluorescence of the aptamer-complementary sequence-modified UCNPs were restored when it was replaced by S. aureus and released from the aptamer-modified AuNPs. The sensitivity of this detection system was 10.7 CFU/ml, and the linear range was 47∼4.7 × 107 CFU/ml (Ouyang et al., 2021b). UCNPs have been widely used in sensors due to their advantages of high quantum yield, narrow emission peak, good photostability, long fluorescence lifetime, large anti-Stokes shift, and resistance to photobleaching. However, there are still some limitations, for example, it is difficult for the naked eye to distinguish different target concentrations under excitation by a 980 nm laser beam.
CDs are spherical carbon particles (or graphite fragments) luminescent materials smaller than 10 nm with semiconductor quantum effect and up-conversion function. CDs have been widely used in FRET-based sensors due to their outstanding properties, such as easy synthesis, low cost, strong fluorescence emission, high water solubility, good biocompatibility, and resistance to photobleaching. Cui et al. synthesized multi-color fluorescent CDs with long fluorescence lifetime and high photostability for S. aureus detection. The aptamer complementary sequences modified CDs were used as energy donors, and their fluorescence signals could be quenched by the aptamer-modified Fe3O4 through FRET. The fluorescence of the aptamer complementary sequences modified CDs were restored when they were replaced by S. aureus and released from the aptamer-modified Fe3O4 (Figure 3D) (Cui et al., 2019). Their result shown that the sensor has fast detection speed (<30 min), good sensitivity (8 CFU/ml) and wide linear range (50∼107 CFU/ml). What’s more, CDs can be used as fluorescent probes for in vitro and in vivo bioimaging due to their excellent biocompatibility and low toxicity, providing a new platform for pathogen bioimaging detection (Cui et al., 2019). Yao et al. constructed an enzyme-free and label-free nano-metal surface energy transfer (NSET) aptasensor based on CDs (donor) and AuNPs (acceptor) for one-step detection of S. aureus (Yao et al., 2021). In this study, linker DNA was used to connect aptamer-modified CDs and aptamer-complementary sequence-modified AuNPs. Compared with the direct hybridization of CDs and AuNPs, the fluorescence of CDs was quenched by AuNPs as high as 63.5% in linker DNA based hybridization, which significantly improved the detection limit of S. aureus (Yao et al., 2021).
Nanomaterials are playing an increasingly important role in improving sensors for the detection of bacterial pathogens due to their unique optical properties, and are considered as one of the most promising candidates for accurate reporting of pathogens. Novel metal nanoclusters (NCs) are a new type of nanoparticles with diameters less than 2 nm, and are composed of less than 150 metal atoms. It has attracted more and more attention due to its outstanding advantages such as water solubility, high fluorescence and quantum yield, remarkable catalytic behavior, good biocompatibility and stability, etc (Munoz-Bustos et al., 2017; Yu et al., 2017; Pebdeni et al., 2021). Pebdeni et al. proposed a sensitive and specific S. aureus detection sensor based on vancomycin and aptamer dual receptor functionalized copper nanoclusters (CuNCs). CuNCs have photoluminescence properties and can induce fluorescence signal enhancement during the aggregation which was caused by S. aureus. Their result shown that the selectivity and sensitivity of this fluorescence sensor was enhanced by aptamer-CuNCs (Pebdeni et al., 2021). NCs not only possess unique fluorescent properties, but also have received extensive attention for their antibacterial properties (Javani et al., 2016). Yang et al. achieve a novel approach for visual detection and effective elimination of S. aureus by combing DNA-templated silver nanoclusters (DNA-AgNCs) and aptamers (Yang et al., 2021a). Compared with general fluorescent nanomaterials, polymer-based fluorescent nanomaterials exhibit excellent biocompatibility and biodegradability, and are easily prepared by self-polymerization (Zhang et al., 2015c). Polydopamine nanospheres (PDANS) have been used as excellent energy receptors for the construction of aptamer-based FRET biosensors because of its tunable diameters, broad absorption bands, and surface-conjugated rigid planar structures that facilitate PDANS binding interactions with aptamers via π-π stacking (Ye et al., 2020). Ye et al. designed a stimuli-responsive nanoprobe (PDANSs-FAM-Apt) for the detection of S. aureus at single-cell level, and it was able to destroy S. aureus and its biofilm on demand via NIR light-activated photothermal activity (Figure 4) (Ye et al., 2020). Porous materials with “molecular gates” have also been widely used in sensors for biomolecule detection. Nanoporous anodic aluminum (NAA) is widely used in aptasensors due to its stability, non-degradation in aqueous solution, multiple reuses after calcination, and support of nucleic acids (DNA, RNA, or aptamers) as molecular gates (Chen et al., 2015; Ribes and Aznar, 2019; Pla et al., 2020). Pla et al. installed the fluorescent indicator rhodamine B in the NAA well, and the entrance of the well was covered by DNA aptamer. The fluorescent indicator rhodamine B will be released when the orifice was opened by S. aureus binding to aptamer (Pla et al., 2020). This nanodevice can specific and sensitive (2 and 5 CFU in buffer and blood, respectively) detection of S. aureus in less than 1 h (Pla et al., 2020).
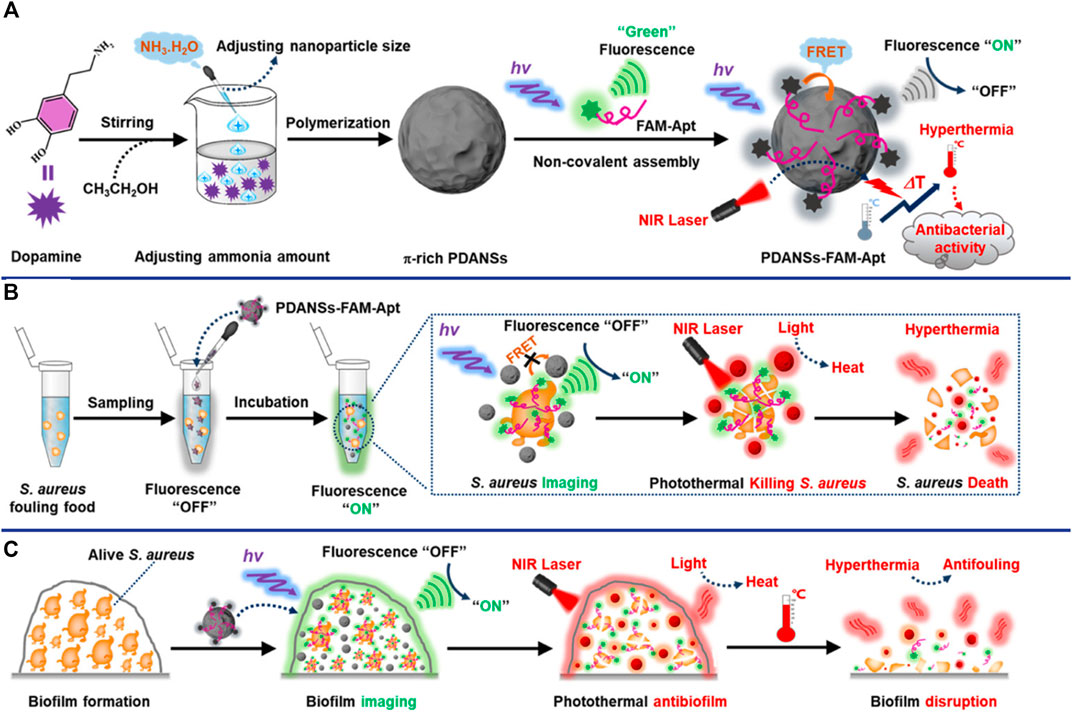
FIGURE 4. General design of the smart nanoprobe PDANSs-FAM-Apt for accurate fluorescence detection and imaging-guided precise photothermal antibacteria. (A) Illustration of the assembly procedure of the nanoprobe PDANSs-FAM-Apt. (B) Diagram of the FRET-based assay procedure for PDANSs-FAM-Apt responsive to living S. aureus and imaging-guided photothermal killing of S. aureus. (C) Schematic of imaging-guided photothermal antifouling of the nanoprobe PDANSs-FAM-Apt for the destruction of S. aureus biofilms. Reproduced with permission (Ye et al., 2020).
Surface Enhanced Raman Scattering-Based Aptasensor
“Raman scattering” means that the molecules in the substance absorb part of the energy from excitation light and vibrate in different ways and degrees (for example: the wobbling and twisting of atoms, the wobbling and vibration of chemical bonds), and then scatter lower frequency light. The frequency of Raman scattering is determined by the characteristics of the substance. The Raman scattering can be enhanced by several orders of magnitude when different molecules or ions are adsorbed on the surface of metal nanomaterials (Ag, Cu, Au and other metal nanomaterials), which is related to the submicroscopic surface roughness of metal nanomaterials. SERS-based aptasensors are widely used in pathogen detection due to their ultra-sensitive, simple and rapid, excellent photostability, label-free and non-destructive fingerprint recognition (Xu et al., 2019). Zhang et al. constructed a “capture probe-target-signal probe” sandwich sensor for S. aureus detection by using Raman molecules and aptamer-modified AuNPs as signal probes, and aptamer-modified Fe3O4 magnetic gold nanoparticles (MGNPs) as capture probes (Zhang et al., 2015a). Although this method is simple, rapid, wide linearity (102∼107 CFU/ml), high sensitivity (35 CFU/ml) and strong specificity, it is time-consuming (2∼3 h) (Zhang et al., 2015a). Gao et al. constructed a label-free SERS-based aptasensor with only 25 min for the detection of S. aureus (Gao et al., 2017). Using the aptamer that specifically recognizes and binds to S. aureus as a template, silver nanoparticles were synthesized in situ to directly obtain the SERS fingerprint spectrum (Gao et al., 2017). This strategy is simple, rapid and low-cost, creating an idea for in situ detection of pathogens on microarrays. SERS-based sensors are generally used in liquid systems, which greatly limit their application due to the large number of detection steps and high cost. Zhu et al. construct a reliable, rapid, sensitive and specific biosensor for S. aureus detection by using aptamer-AuNPs-polydimethylsiloxane (PDMS) as capture substrate, mercaptobenzoic acid (4-MBA) and aptamer-modified gold-silver Core-shell nanoflowers (Au@Ag NFs) as signal probes (Figure 5A) (Zhu et al., 2021a). The SERS spectra will change when the sandwich structure “capture substrate-S. aureus-signal probe” was formed in the presence of the S. aureus. The SERS aptasensor has high sensitivity (13 CFU/ml), and exhibits a good linear relationship in the range of 4.3 × 10 CFU/ml∼4.3 × 107 CFU/ml. Au and Ag are the most popular SERS substrates, but their applications are limited due to their easy aggregation, high chemical activity, and easy chemical reaction with external substances (Jiao et al., 2021). Core-shell structures have recently been widely used as SERS substrates due to thin shells composed of inert materials can successfully prevent noble metal cores from contacting the external environment. Silica is an ideal shell material because it has no absorbance in the visible wavelength band and is somewhat hydrophilic (Li et al., 2017a). Zhu et al. proposed a SERS sensor for the detection of S. aureus, which based on S. aureus can induce release of the Raman dye 4-aminothiophenol (4-ATP) from mesoporous silica nanoparticles (MSNs) by binding to the gating aptamer (Figure 5B) (Zhu et al., 2021b). The SERS sensor is low-cost, fast, high sensitivity (17 CFU/ml), wide linearity (4.7 × 10 CFU/ml-4.7 × 108 CFU/ml), and is more reliable for detection of foodborne pathogens (Zhu et al., 2021b). The nanodimer and nanotrimer are also widely used as SERS substrates and show a greater increase in SERS signal compared to monodisperse particles (Ma et al., 2021).
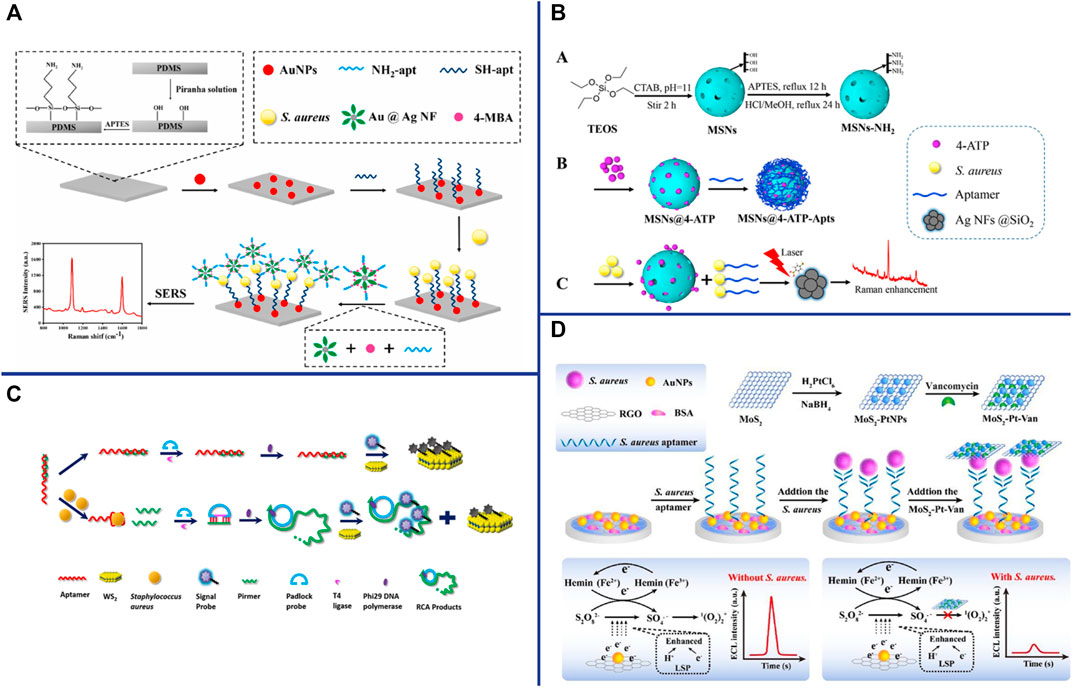
FIGURE 5. (A) Schematic illustration of the developed SERS biosensor based on aptamer functionalized PDMS film for the detection of S. aureus. Reproduced with permission (Zhu et al., 2021a). (B) Schematic illustration of S. aureus detection based on the target-responsive release of 4-ATP molecules from aptamer-gated MSNs. Reproduced with permission (Zhu et al., 2021b). (C) Schematic illustration of the CRET biosensor for the detection of S. aureus based on Co2+/ABEI-AuNFs and WS2 nanosheet. Reproduced with permission (Hao et al., 2017). (D) Schematic illustration of the enzyme-free ECL aptasensor for S. aureus detection based on AuNPs/hemin as the regenerable enhancers of S2O82−/O2 and the quenching effect of MoS2-PtNPs on S2O82−/O2. Reproduced with permission (Han et al., 2019).
Surface Plasmon Resonance-Based Aptasensor
Surface plasmon resonance (SPR) is a physical phenomenon that can cause resonance due to the light energy absorption of free electrons when incident light irradiates on the surface of plasmonic elements. The SPR was enhanced when light is irradiated onto plasmonic nanoparticles (eg, gold, silver, copper) or nanostructured and was called localized surface plasmon resonances (LSPRs) (Csáki et al., 2018). LSPR-based aptasensors have the advantages of ultrasensitive, real-time monitoring, label-free, miniaturization, and portability (Hoa et al., 2007). The LSPR frequency and strength primarily depends on the size, shape, geometry, interparticle spacing, and dielectric properties of the material. AuNPs have been widely used in LSPR-based aptasensors due to their easy preparation, high density, large dielectric constant, and good biocompatibility. Khateb et al. successfully constructed a simple, portable, and label-free LSPR-based aptasensor for S. aureus detection with short detection times of 120s (Khateb et al., 2020). Their results showed that the thickness of the sensing layer was critical, with a significantly greater responses for the thinner aptamer layer compared to antibody-based recognition elements (Khateb et al., 2020). They also measured the optical response experimentally through finite-difference time-domain simulations of differently sized metal nanostructures (100 and 200 nm disks) based sensor, and showed that the sensitivity of the 200 nm diameter disk structure was significantly higher compared to the 100 nm diameter disk structure, which is due to the increase in bulk refractive index sensitivity and the extent to which the local field extends from the metal surface (Khateb et al., 2020). The LSPR can also be simply tuned by changing the shape of the NPs, for example, only one LSPR has occurred in the 520 nm range (red color) for gold nanospheres (2∼50 nm), while two were detected in the range of 522 nm (transverse LSPR) and 698 nm (longitudinal LSPR) for gold nanorods (Sharifi et al., 2020).
Chemiluminescence-Based Aptasensor
Chemiluminescence (CL)-based biosensors are a promising detection method because their energy is generated by chemical reactions and the sample does not require photoexcitation, thus avoiding interference from light scattering, unstable light source, and high background (Dodeigne et al., 2000). Hao et al. constructed a Chemiluminescence resonance energy transfer-based aptasensor for S. aureus detection using Co2+-enhanced N-(aminobutyl) N-(ethylisoluminol) (ABEI)-modified AuNFs (Co2+/ABEI-AuNFs) as the donor and WS2 nanosheets as the acceptor (Figure 5C). The limits of detection of the electrochemiluminescence (ECL)-based aptasensor was 15 CFU/ml with the help of RCA amplification technology (Hao et al., 2017). Han et al. constructed an ECL aptasensor for S. aureus detection based on AuNPs/hemin as a regenerable enhancer for S2O82−/O2 and MoS2-PtNPs as a quencher for S2O82−/O2 (Figure 5D) (Han et al., 2019). RGO was coated on the GCE to increase the specific surface area and electronic conductivity. The thiol-modified aptamers were then immobilized on the AuNPs which were electrochemically deposited on the RGO surface. In the presence of S. aureus, the signal was turned off because aptamer-S. aureus -MoS2-PtNP-Van sandwich complex formed which act as quencher for S2O82−/O2. Conversely, in the absence of S. aureus, the signal was turned on (Han et al., 2019). The results shown that the ECL-based aptasensor has high specificity and sensitivity (28 CFU/ml), and can be used for the detection of S. aureus in urine samples (Han et al., 2019).
Aptasensors Based on Electrochemical Transduction
The electrochemical aptasensors refers to that when the aptamer immobilized on the electrode surface binds to the target, it will cause a change that can be converted into a measurable electrical signal (current, impedance, potential or conductance, etc.) (Li et al., 2019b). Electrochemical sensors are one of the preferred biosensors for their high sensitivity, low cost, multi-analyte analysis, reproducibility, miniaturization and portability (Maduraiveeran et al., 2018). Electrochemical sensors can be classified into potentiometric, amperometric/voltammetric, impedimetric and conductometric sensors according to the electrical signal measured (Paniel et al., 2013).
Potentiometric-Based Aptasensor
Potentiometric biosensor is one of the oldest electrochemical techniques and is widely used due to its low cost and simple operation. Recent studies have shown that an increasing number of electrochemical sensors employ nanomaterials as efficient transducers and amplifiers. Carbon nanotubes have been widely used as transducers in electrochemical sensors due to their strong ability to promote electron transfer between electroactive species and electrodes. Zelada-Guillén et al. construct a highly sensitive potentiometric sensor for real-time detection of S. aureus without labeling based on aptamers as biorecognition elements and single-walled carbon nanotubes (SWCNTs) as potential transducers (Zelada-Guillen et al., 2012). The results shown that the sensor could detect S. aureus in skin in real time with good stability and specificity (Zelada-Guillen et al., 2012). Graphene oxide (GO) and reduced graphene oxide (RGO) are the preferred material for fabricating highly sensitive sensing platforms due to their unique thermal, mechanical, and electrical properties. Hernández et al. constructed a potentiometric aptasensor based on GO and RGO for the detection of S. aureus. The potentiometric aptasensor has strong selectivity and high sensitivity and can detect single live S. aureus. However, when the potentiometric aptasensor was applied to real samples, it is necessary to filter out the electroactive substances in the sample that interfere with the detection (Hernandez et al., 2014).
Amperometric/Voltammetric-Based Aptasensor
Amperometric/voltammetric is the most common and successful electrochemical techniques for S. aureus detection. Abbaspour et al. constructed a voltammetric-based aptasensor for S. aureus detection, which based on aptamer-modified magnetic beads as capture probes and aptamer-modified silver nanoparticles (Apt-AgNPs) as signal probes. Apt/S. aureus/apt-AgNP sandwich complexes form in the presence of S. aureus, resulting in current changes (Abbaspour et al., 2015). This approach with good sensitivity (1.0 CFU/ml) and wild linear range (10∼106 CFU/ml) due to the combination of magnetic bead separation and signal amplification of AgNPs (Abbaspour et al., 2015). Besides metallic nanomaterials, other nanomaterials as efficient amplifiers have also become an integral part of electrochemical biosensor. For example, metal-organic framework (MOF) is a unique inorganic-organic hybrid nanomaterial, which has been widely used in many fields due to its flexibility, uniform structure, good chemical stability, and good biocompatibility (Han et al., 2020). Sun et al. electrodeposited Cu metal-organic framework (Cu-MOF) thin films on electrodes and in situ reduced AuNPs on their surfaces to enhance their electrical conductivity and form complexes (DNA/AuNPs/MOFs) with aptamers via Au-S bonds to construct electrochemical biosensors for dual detection of S. aureus (Figure 6A) (Sun et al., 2021). This aptasensor not only has high specificity and high sensitivity (1.9 CFU/ml), but also is easy to operate and requires no additional signal components (Sun et al., 2021). With the development of DNA nanotechnology, various nanomaterials constructed from DNA have also been widely used in electrochemical sensors. Cai et al. constructed a dual-signal amplifying electrochemical aptasensor based on DNA walker and DNA nanoflowers for high-sensitivity detection of S. aureus (Cai et al., 2021b). Two sets of double-stranded DNA were modified on the surface of the Au electrode. When the aptamer was bound to S. aureus, the DNA walker bound to the aptamer was released. With the help of exonuclease III (Exo III), the DNA walker moves along the electrode surface and continuously hydrolyzes the anchored short double-stranded DNA. The introduction of circular DNA and phi29 DNA polymerase initiates RCA and in turn forms nanoflowers, which provide binding sites for electroactive methylene blue (MB), resulting in a strong electrochemical signal (Figure 6B) (Cai et al., 2021b). The results shown that the electrochemical aptasensor has high sensitivity, high specificity and good anti-interference ability, which can distinguish S. aureus in mixed samples from other non-target bacteria (Cai et al., 2021b). In addition to nanomaterials, nucleic acid-based RCA, SDR, HCR, and HD-CHR amplification systems are also widely used as amplifiers in electrochemical sensors. Cai et al. constructed a multifunctional electrochemical sensor based on SDA and triple helix molecular switch for S. aureus detection (Cai et al., 2021a). In the presence of S. aureus, the aptamer binds to S. aureus and releases the complementary sequence (cDNA) into the solution, which initiates the SDA reaction under the action of primers and enzymes, and resulting in a large number of ssDNA probes. Subsequently, the interaction of the ssDNA probe with the triple helix molecular switch on the electrode leads to the release of the G-quadruplex-rich capture sequence, which reacts with hemin to form an electroactive G-quadruplex/heme complex and then turns on the electrochemical signal (Figure 6C) (Cai et al., 2021a). The results shown that this aptasensor has high sensitivity (8 CFU/ml), high specificity, and can detect S. aureus in lake water, tap water and honey samples (Cai et al., 2021a).
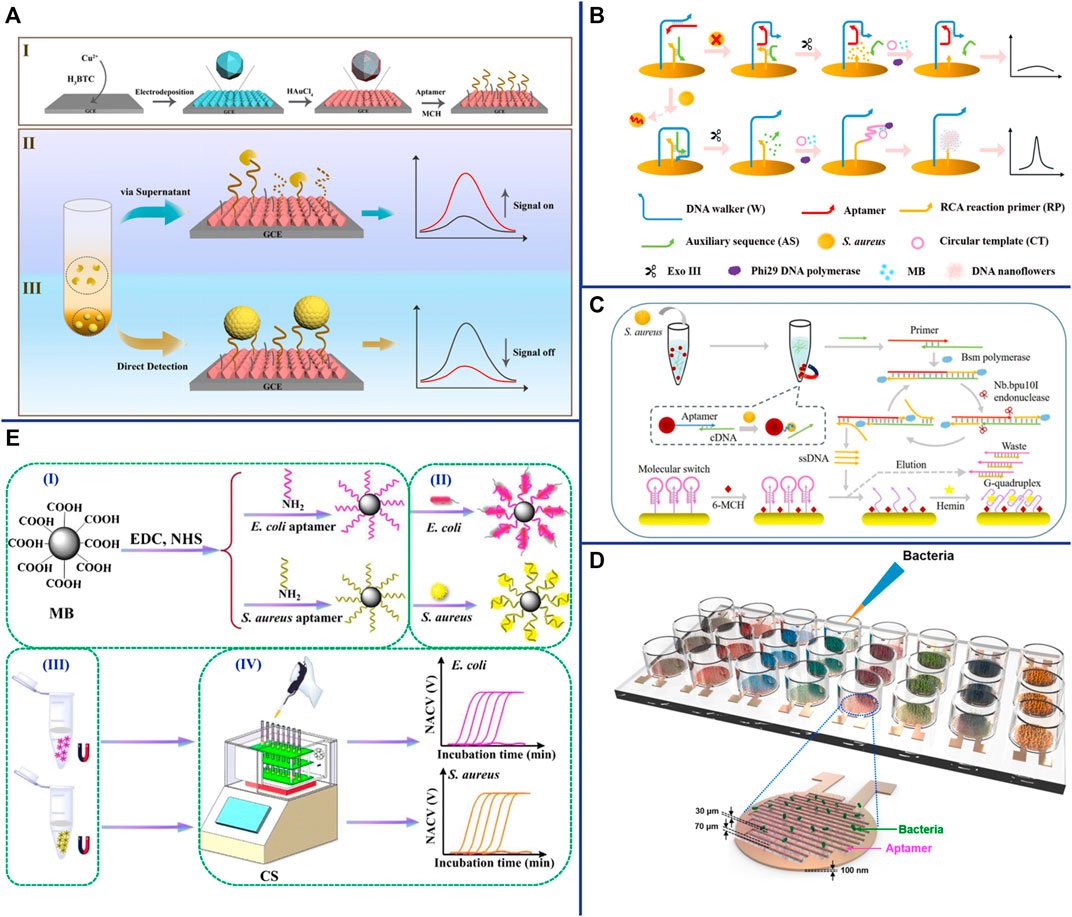
FIGURE 6. (A) (I) Fabrication of the DNA/AuNPs/MOFs-based sensing platform; (II) Schematic diagrams of the electrochemical biosensor for the detection of S. aureus via supernatants; and (III) pathogen cells. Reproduced with permission (Sun et al., 2021). (B) Schematic representation of the biosensor for S. aureus detection based on a DNA walker and DNA nanoflower. Reproduced with permission (Cai et al., 2021b). (C) Schematic representation of the versatile signal-on electrochemical biosensor for S. aureus detection based on triple-helix molecular switch. Reproduced with permission (Cai et al., 2021a). (D) Schematic of aptamer-functionalized capacitance sensor array for real-time monitoring of bacterial growth and antibiotic susceptibility. Reproduced with permission (Jo et al., 2018). (E) Schematic representation of the multichannel conductometric sensor for S. aureus detection based on magnetic analyte separation via aptamer. (I)the preparation of aptamer-functionalized magnetic beads, (II) selective capture and (III) separation of bacterial cells, and (IV) determination of viable bacteria by the conductometric sensor. Reproduced with permission (Zhang et al., 2020).
Impedimetric-Based Aptasensor
In recent years, impedimetric-based aptasensor has attracted interest for the detection of S. aureus due to its high sensitivity, no matrix interference, simple measurement, no labeling, and automation (Paniel et al., 2013). Jia et al. constructed an sensitive, specific and label-free impedimetric-based aptasensor for rapid and quantitative detection of S. aureus (Jia et al., 2014). This system adopts a novel nanocomposite material (rGO-ssDNA-AuNPs) that enhances electron transfer and electrochemical signals as an amplifier to improve the detection sensitivity (10 CFU/ml) (Jia et al., 2014). An effective strategy to improve the performance of biosensors is to increase the reaction surface area and thus capture the target more effectively. In recent years, cellulose nanofibers (CNFs) have been widely used in biosensing due to their large surface area, high aspect ratio, extensive chemical modification ability, good biocompatibility and adhesion (Golmohammadi et al., 2017). Ranjbar et al. using carbon nanoparticles (CNPs) and AuNPs to overcome the poor electrical conductivity of CNFs to construct nanocomposites (AuNPs/CNPs/CNFs) with large surface area, excellent electrical conductivity and good biocompatibility for constructing impedimetric aptasensor (Ranjbar and Shahrokhian, 2018). Studies have shown that the aptasensor has high sensitivity (1 CFU/ml), wide linear range (1.2 × 10∼1.2 × 108 CFU/ml), and can be applied to the accurate detection of S. aureus in human serum samples (Ranjbar and Shahrokhian, 2018). Electrochemical aptasensor not only used for S. aureus detection, but also be used for rapid antibiotic susceptibility testing (AST) of S. aureus. The aptamers were immobilized between the two finger electrodes of an interdigitated gold electrode (IDE). When S. aureus was captured by the aptamer, it can act as a dielectric particle connecting electrode, thereby increasing the capacitance (Figure 6D) (Jo et al., 2018). The result shown that this aptamer-functionalized capacitive sensor array can not only rapidly and specifically recognize S. aureus (1 h), but also monitor bacterial growth in real time with high sensitivity (10 CFU/ml), which can be used for AST of S. aureus (Jo et al., 2018).
Conductometric-Based Aptasensor
Conductometric is a measurement of monitoring the conductivity of solution using a low-amplitude alternating current potential, which relies on the change in conductivity that occurs in the sample through the production or consumption of charged species. The working electrode must be in contact with the solution in traditional electrochemical sensors, which inevitably causes electrode degradation and non-specific capture. To solve these problems, Zhang et al. developed a conductometric for real-time quantifying the number of live bacteria by using aptamer-modified magnetic beads to separate S. aureus and then a capacitively-coupled non-contact conductivity detector to measure and record conductivity changes. The bacteria captured by aptamer-modified magnetic beads can also be used for AST (Figure 6E) (Zhang et al., 2020).
Aptasensor For POCT
Point-of-care testing (POCT) refers to a miniature mobile detection system that is close to the test sample in a non-laboratory environment and reports the results quickly. Although POCT does not require tedious operating steps, professional operators, and expensive and sophisticated instruments, the development and popularization of highly sensitive and specific POCT technology is still a major challenge in the field of science and engineering. A major strategy of POCT is to infiltrate liquid reagents into filter paper and various water-absorbing materials, and fix them on rigid substrates after drying. Paper-based lateral flow strips (LFS), also known as test strips, have been widely used for POCT and are the most promising method for POCT in various fields. In addition to being sensitive and specific, LFS is simple to operate and does not require complicated and expensive instruments, and the detection results can be observed with the naked eye. Lu et al. constructed an aptamer- and AuNPs-based LFS for rapid detection of S. aureus, with a detection time of only 10 min and no cross-reaction with other common bacteria (Lu et al., 2020a). Raji et al. developed a novel aptamer-based swab sensor for qualitative and quantitative detection of MRSA on environmental surfaces (Raji et al., 2021). In the presence of MRSA on environmental surfaces, MRSA can be combined with aptamers immobilized on cotton swabs and aptamers modified by blue nanobeads to form an “aptamer-MRSA-aptamer” sandwich structure (Raji et al., 2021). The swab sensor is fast (5 min), sensitive (theoretical value 2 CFU/ml), specific, and can be read directly by the naked eye without the need for laboratory equipment (Raji et al., 2021). This novel aptasensor holds great promise because of its good detection performance, ease of use and relatively inexpensive to produce.
Another major strategy of POCT is to miniaturize the analytical instrument and simplify the operation method, making it a portable and palm-like device. Huang et al. have developed a microfluidic device based on volumetric bar-chart chip (V-Chip) technology for rapid and visually intuitive quantification of pathogenic bacteria in urine (Huang et al., 2019). Bacteria can combine with aptamer-modified magnetic beads and 2-(dodecyldimethylamino) acetate (BS-12) modified platinum nanoparticles (PtNPs-BS12) to form an “aptamer-bacteria-PtNPs-BS12” sandwich complex. Pt NPs with extremely high catalase-like activity can catalyze the decomposition of H2O2 to generate O2, thereby pushing the pink ink in the V-Chip into the S-shaped readout channel (Figure 7A) (Huang et al., 2019). Due to the fast detection speed (1.5 h) and high sensitivity (as low as 1 CFU/ml) of the device, and the detection results of clinical urine samples are consistent with the plate counting, the V-Chip can significantly improve the early diagnosis rate of bacterial infection (Huang et al., 2019). A pressure measuring instrument is an ideal POCT that is simple, portable and capable of quantitative detection. Li et al. constructed a pressure-based POCT for the detection of S. aureus using vancomycin-modified functionalized platinum nanoparticles (PtNPs@Van) and aptamer-modified magnetic CuFe2O4 nanoprobes. The Pt NPs catalyzed the decomposition of H2O2 to O2 in a sealed device, resulting in a significant increase in pressure that can be detected with a hand-held digital pressure gauge (Figure 7B) (Li et al., 2019a). Personal Glucose Meters (PGMs) also has been widely used as a readout means for highly sensitive non-glucose target identification and quantification due to its advantages of portability, speed, low cost, easy operation and reliable results. Yang et al. designed a novel aptasensor for S. aureus detection based on HCR and PGMs (Figure 7C). This aptasensor is specific, sensitive (2 CFU/ml) and accurate, providing a new perspective for the development of sensitive and portable pathogen detection solution in the future (Yang et al., 2021b). Wang et al. developed an aptamer-based micro-microfluidic chip for multi-pathogen detection, replacing the traditional single-pathogen detection (Figure 7D). The results shown that this new microfluidic chip has faster detection time (35 min) and smaller size (7.0 cm × 5.0 cm × 1.2 cm), higher specificity, and simultaneous detected multiple pathogens compared with traditional chips (Wang et al., 2019a). Therefore, it promises to be a powerful POCT for multibacterial diagnosis.
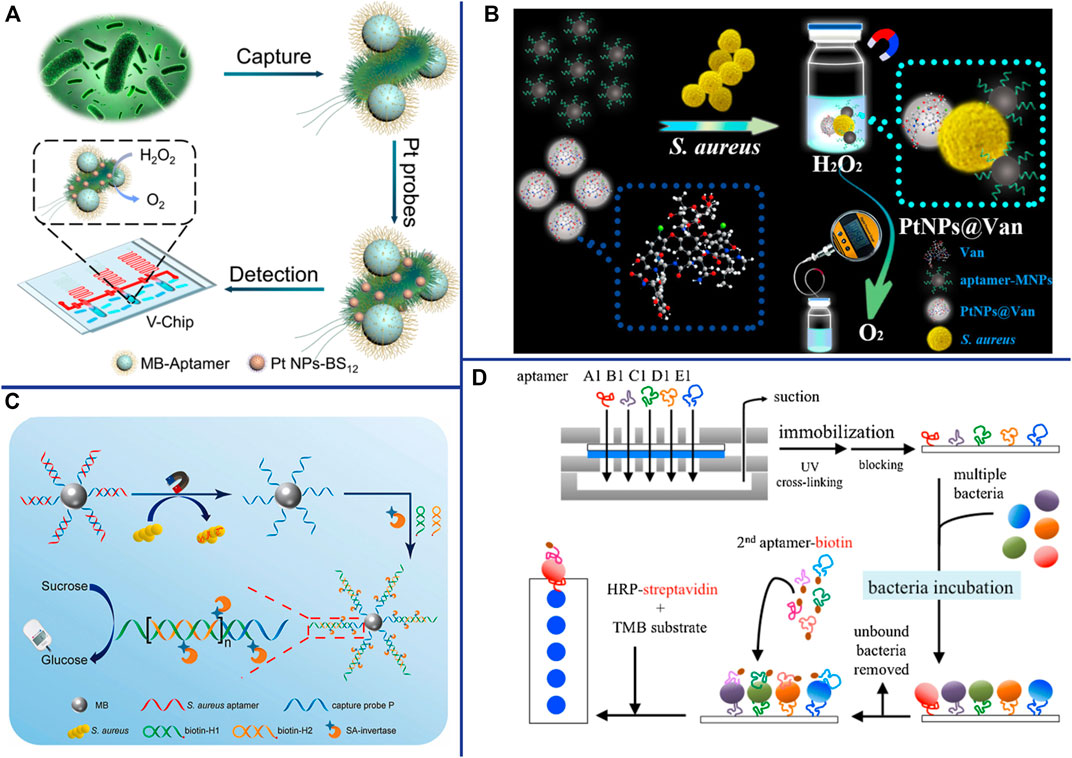
FIGURE 7. (A) Working principle for detection of bacteria using BV-Chip. Reproduced with permission (Huang et al., 2019). (B) Schematic illustration of gas pressure-based POC testing protocol for highly sensitive and specific detection of S. aureus. Reproduced with permission (Li J. et al., 2019a). (C) Schematic illustration of the principle for portable detection of S. aureus using PGM based on HCR strategy. Reproduced with permission (Yang et al., 2021b). (D) The experimental procedure for multi-bacterial detection via bacteria-specific aptamers immobilized on a nitrocellulose (NC) membrane. 2nd (secondary) aptamer-biotin conjugated with biotin. Reproduced with permission (Wang et al., 2019a).
Conclusion and Perspectives
This review presented an overview on the latest progress of aptasensors based on optical (colorimetric, fluorescence, SPR and SERS) and electrochemical (potentiometric, voltammetric, impedimetric and conductometric) for S. aureus detection. Overall, colorimetric-based aptasensors are simplicity, fast, low-cost, and the results can be quickly judged by the naked eye or simple instruments. However, its low sensitivity still needs to be improved when applying to practical detection. Fluorescence-based aptasensors have become one of the most commonly used sensors for low-concentration analyte detection due to their high sensitivity, but their results are susceptible to interference by autofluorescence or background fluorescence, and the fluorescent molecules used are unstable and prone to photobleaching. Novel optical transduction mechanisms such as SPR and SERS have also been used for S. aureus detection due to their advantages of ultra-sensitivity, real-time monitoring, and label-freeness, but their widespread application is limited by cumbersome sample preparation and the expensive equipment required. Compared with optical based aptasensors, electrochemical based aptasensors demonstrate the potential for the fabrication of POCT due to their fastness, simplicity, sensitivity, miniaturization and portability.
Although the development of aptasensors is the most active research topic in recent years, there are still some significant challenges in practical applications: First, since most of the existing aptamers are screened by time-consuming and inefficient traditional SELEX, it is necessary to introduce new technologies and new methods (such as Cell-SELEX, Genomic SELEX, IP-SELEX, Capture-SELEX, CE-SELX, M-SELEX, AFM-SELEX, AEGIS-SELEX, Animal-SELEX) to improve the screening efficiency and generate higher affinity and specificity aptamers. Second, aptamer density, aptamer orientation, surface charge and steric hindrance during the progress of aptamer immobilization should also be systematically studied to further improve aptamer properties and biostability. Although nanomaterials play an important role in increasing the immobilization density and orientation of aptamers, high-density immobilization will limit the formation of normal configurations of aptamers. Third, although most studies have shown that the aptasensors have good specificity and could distinguish other co-existing foodborne pathogens in artificially contaminated samples (recoveries ranged from 85.0 to 110.0%), the interference effects of various metal ions, anions and antioxidants that may be present in the real sample also require further studies to ensure the existing aptasensors compatibility in complex biological and clinical samples. Fourth, the stability and reproducibility of nanomaterials that play an increasingly important role in aptasensors still need to be improved. Fifth, how to achieve simultaneous detection of multiple pathogens is still a major challenge in a short period of time.
Overall, although there are still some challenges to be solved, aptasensors have great potential in the field of S. aureus detection. Rapid, sensitive, specific, high-throughput, simple operation, small sample size, low cost, reagent-safe, portable, and wearable aptamer based novel microfluidic chip will become the focus of future biosensor research, and will have a significant impact on human health and well-being.
Author Contributions
WC Designed the manuscript, conducted literature search, wrote the manuscript, revised and proofread manuscript. QTL performed literature search and helped write the manuscript. YKZ performed literature search, helped write the manuscript. ZCL provide the principal idea, designed the manuscript, helped revised and proofread manuscript.
Funding
This work was supported financially by the National Natural Science Foundation of China (Grant no. 61771493) and the Natural Science Foundation of Hunan province (Grant No. 2018JJ2532).
Conflict of Interest
The authors declare that the research was conducted in the absence of any commercial or financial relationships that could be construed as a potential conflict of interest.
Publisher’s Note
All claims expressed in this article are solely those of the authors and do not necessarily represent those of their affiliated organizations, or those of the publisher, the editors and the reviewers. Any product that may be evaluated in this article, or claim that may be made by its manufacturer, is not guaranteed or endorsed by the publisher.
References
Abbaspour, A., Norouz-Sarvestani, F., Noori, A., and Soltani, N. (2015). Aptamer-conjugated Silver Nanoparticles for Electrochemical Dual-Aptamer-Based Sandwich Detection of staphylococcus Aureus. Biosens. Bioelectron. 68, 149–155. doi:10.1016/j.bios.2014.12.040
Alizadeh, N., Memar, M. Y., Moaddab, S. R., and Kafil, H. S. (2017). Aptamer-assisted Novel Technologies for Detecting Bacterial Pathogens. Biomed. Pharmacother. 93, 737–745. doi:10.1016/j.biopha.2017.07.011
Amraee, M., Oloomi, M., Yavari, A., and Bouzari, S. (2017). DNA Aptamer Identification and Characterization for E. coli O157 Detection Using Cell Based SELEX Method. Anal. Biochem. 536, 36–44. doi:10.1016/j.ab.2017.08.005
Berezovski, M., Musheev, M., Drabovich, A., and Krylov, S. N. (2006a). Non-SELEX Selection of Aptamers. J. Am. Chem. Soc. 128, 1410–1411. doi:10.1021/ja056943j
Berezovski, M. V., Musheev, M. U., Drabovich, A. P., Jitkova, J. V., and Krylov, S. N. (2006b). Non-SELEX: Selection of Aptamers without Intermediate Amplification of Candidate Oligonucleotides. Nat. Protoc. 1, 1359–1369. doi:10.1038/nprot.2006.200
Bock, L. C., Griffin, L. C., Latham, J. A., Vermaas, E. H., and Toole, J. J. (1992). Selection of Single-Stranded DNA Molecules that Bind and Inhibit Human Thrombin. Nature 355, 564–566. doi:10.1038/355564a0
Boiziau, C., Dausse, E., Yurchenko, L., and Toulmé, J.-J. (1999). DNA Aptamers Selected against the HIV-1trans-Activation-Responsive RNA Element Form RNA-DNA Kissing Complexes. J. Biol. Chem. 274, 12730–12737. doi:10.1074/jbc.274.18.12730
Borsa, B. A., Tuna, B. G., Hernandez, F. J., Hernandez, L. I., Bayramoglu, G., Arica, M. Y., et al. (2016). Staphylococcus Aureus Detection in Blood Samples by Silica Nanoparticle-Oligonucleotides Conjugates. Biosens. Bioelectron. 86, 27–32.
Cai, R., Yin, F., Chen, H., Tian, Y., and Zhou, N. (2020). A Fluorescent Aptasensor for Staphylococcus aureus Based on Strand Displacement Amplification and Self-Assembled DNA Hexagonal Structure. Microchim. Acta 187, 304. doi:10.1007/s00604-020-04293-9
Cai, R., Yin, F., Zhang, Z., Tian, Y., and Zhou, N. (2019). Functional Chimera Aptamer and Molecular Beacon Based Fluorescent Detection of Staphylococcus aureus with Strand Displacement-Target Recycling Amplification. Anal. Chim. Acta 1075, 128–136. doi:10.1016/j.aca.2019.05.014
Cai, R., Zhang, S., Chen, L., Li, M., Zhang, Y., and Zhou, N. (2021b). Self-Assembled DNA Nanoflowers Triggered by a DNA Walker for Highly Sensitive Electrochemical Detection of Staphylococcus aureus. ACS Appl. Mat. Interfaces 13, 4905–4914. doi:10.1021/acsami.0c22062
Cai, R., Zhang, Z., Chen, H., Tian, Y., and Zhou, N. (2021a). A Versatile Signal-On Electrochemical Biosensor for Staphylococcus aureus Based on Triple-Helix Molecular Switch. Sensors And Actuators B-Chemical 326, 128842. doi:10.1016/j.snb.2020.128842
Cao, X., Li, S., Chen, L., Ding, H., Xu, H., Huang, Y., et al. (2009). Combining Use of a Panel of ssDNA Aptamers in the Detection of Staphylococcus aureus. Nucleic Acids Res. 37, 4621–4628. doi:10.1093/nar/gkp489
Catala, C., Mir-Simon, B., Feng, X., Cardozo, C., Pazos-Perez, N., Pazos, E., et al. (2016). Online SERS Quantification of Staphylococcus aureus and the Application to Diagnostics in Human Fluids. Adv. Mater. Technol. 1.
Cesewski, E., and Johnson, B. N. (2020). Electrochemical Biosensors for Pathogen Detection. Biosens. Bioelectron. 159, 112214. doi:10.1016/j.bios.2020.112214
Chang, T., Wang, L., Zhao, K., Ge, Y., He, M., and Li, G. (2016). Duplex Identification of Staphylococcus aureus by Aptamer and Gold Nanoparticles. J. Nanosci. Nanotechnol. 16, 5513–5519. doi:10.1166/jnn.2016.11656
Chang, Y. C., Yang, C. Y., Sun, R. L., Cheng, Y. F., Kao, W. C., and Yang, P. C. (2013). Rapid Single Cell Detection of Staphylococcus aureus by Aptamer-Conjugated Gold Nanoparticles. Sci. Rep. 3, 1863. doi:10.1038/srep01863
Chen, C.-J., and Huang, Y.-C. (2014). New Epidemiology of Staphylococcus aureus Infection in Asia. Clin. Microbiol. Infect. 20, 605–623. doi:10.1111/1469-0691.12705
Chen, Y., Santos, A., Wang, Y., Kumeria, T., Li, J., Wang, C., et al. (2015). Biomimetic Nanoporous Anodic Alumina Distributed Bragg Reflectors in the Form of Films and Microsized Particles for Sensing Applications. ACS Appl. Mat. Interfaces 7, 19816–19824. doi:10.1021/acsami.5b05904
Cheng, D., Yu, M., Fu, F., Han, W., Li, G., Xie, J., et al. (2016). Dual Recognition Strategy for Specific and Sensitive Detection of Bacteria Using Aptamer-Coated Magnetic Beads and Antibiotic-Capped Gold Nanoclusters. Anal. Chem. 88, 820–825.
Cheng, C., Chen, Y. H., Lennox, K. A., Behlke, M. A., and Davidson, B. L. (2013a). In Vivo SELEX for Identification of Brain-Penetrating Aptamers. Mol. Ther. - Nucleic Acids 2, e67. doi:10.1038/mtna.2012.59
Cheng, L., Wang, C., and Liu, Z. (2013b). Upconversion Nanoparticles and Their Composite Nanostructures for Biomedical Imaging and Cancer Therapy. Nanoscale 5, 23–37. doi:10.1039/c2nr32311g
Cheng, X., Huang, L., Yang, X., Elzatahry, A. A., Alghamdi, A., and Deng, Y. (2019). Rational Design of a Stable Peroxidase Mimic for Colorimetric Detection of H2O2 and Glucose: A Synergistic CeO2/Zeolite Y Nanocomposite. J. Colloid Interface Sci. 535, 425–435. doi:10.1016/j.jcis.2018.09.101
Csáki, A., Stranik, O., and Fritzsche, W. (2018). Localized Surface Plasmon Resonance Based Biosensing. Expert Rev. Mol. Diagnostics 18, 279–296. doi:10.1080/14737159.2018.1440208
Cui, F., Sun, J., de Dieu Habimana, J., Yang, X., Ji, J., Zhang, Y., et al. (2019). Ultrasensitive Fluorometric Angling Determination of Staphylococcus aureus In Vitro and Fluorescence Imaging In Vivo Using Carbon Dots with Full-Color Emission. Anal. Chem. 91, 14681–14690. doi:10.1021/acs.analchem.9b03916
Dodeigne, C., Thunus, L., and Lejeune, R. (2000). Chemiluminescence as Diagnostic Tool. A Review. Talanta 51, 415–439. doi:10.1016/s0039-9140(99)00294-5
Duan, N., Gong, W., Wang, Z., and Wu, S. (2016). An Aptasensor Based on Fluorescence Resonance Energy Transfer for Multiplexed Pathogenic Bacteria Determination. Anal. Methods 8, 1390–1395.
Duan, N., Wu, S., Zhu, C., Ma, X., Wang, Z., Yu, Y., et al. (2012). Dual-Color Upconversion Fluorescence and Aptamer-Functionalized Magnetic Nanoparticles-Based Bioassay for the Simultaneous Detection of Salmonella Typhimurium and Staphylococcus Aureus. Analytica. Chimica. Acta. 723, 1–6.
Dwivedi, H. P., Smiley, R. D., and Jaykus, L.-A. (2013). Selection of DNA Aptamers for Capture and Detection of Salmonella Typhimurium Using a Whole-Cell SELEX Approach in Conjunction with Cell Sorting. Appl. Microbiol. Biotechnol. 97, 3677–3686. doi:10.1007/s00253-013-4766-4
Ellington, A. D., and Szostak, J. W. (1990). In Vitro selection of RNA Molecules that Bind Specific Ligands. Nature 346, 818–822. doi:10.1038/346818a0
Fluit, A., Verhoef, J., Schmitz, F., and Sentry Participants, T. E. (2001). Frequency of Isolation and Antimicrobial Resistance of Gram-Negative and Gram-Positive Bacteria from Patients in Intensive Care Units of 25 European University Hospitals Participating in the European Arm of the SENTRY Antimicrobial Surveillance Program 1997-1998. Eur. J. Clin. Microbiol. Infect. Dis. 20, 617–625. doi:10.1007/s100960100564
Fu, F., Zhang, Y., Li, L., Wang, H., Li, Q., Tao, X., et al. (2020). Intracellular Pathogen Detection Based on Dual-Recognition Units Constructed Fluorescence Resonance Energy Transfer Nanoprobe. Anal. Chem. 92, 11462–11468. doi:10.1021/acs.analchem.0c02695
Furst, A. L., and Francis, M. B. (2019). Impedance-Based Detection of Bacteria. Chem. Rev. 119, 700–726. doi:10.1021/acs.chemrev.8b00381
Gao, W., Li, B., Yao, R., Li, Z., Wang, X., Dong, X., et al. (2017). Intuitive Label-free SERS Detection of Bacteria Using Aptamer-Based In Situ Silver Nanoparticles Synthesis. Anal. Chem. 89, 9836–9842. doi:10.1021/acs.analchem.7b01813
Ghosh, S. K., and Pal, T. (2007). Interparticle Coupling Effect on the Surface Plasmon Resonance of Gold Nanoparticles: from Theory to Applications. Chem. Rev. 107, 4797–4862. doi:10.1021/cr0680282
Gill, A. A. S., Singh, S., Thapliyal, N., and Karpoormath, R. (2019). Nanomaterial-based Optical and Electrochemical Techniques for Detection of Methicillin-Resistant Staphylococcus aureus: a Review. Microchim. Acta 186, 114. doi:10.1007/s00604-018-3186-7
Golmohammadi, H., Morales-Narváez, E., Naghdi, T., and Merkoçi, A. (2017). Nanocellulose in Sensing and Biosensing. Chem. Mat. 29, 5426–5446. doi:10.1021/acs.chemmater.7b01170
Hamula, C. L. A., Zhang, H., Li, F., Wang, Z., Chris Le, X., and Li, X.-F. (2011). Selection and Analytical Applications of Aptamers Binding Microbial Pathogens. TrAC Trends Anal. Chem. 30, 1587–1597. doi:10.1016/j.trac.2011.08.006
Han, D., Yan, Y., Wang, J., Zhao, M., Duan, X., Kong, L., et al. (2019). An Enzyme-free Electrochemiluminesce Aptasensor for the Rapid Detection of Staphylococcus aureus by the Quenching Effect of MoS2-PtNPs-Vancomycin to S2O82−/O2 System. Sensors Actuators B Chem. 288, 586–593. doi:10.1016/j.snb.2019.03.050
Han, X., Hong, Y., Ma, Y., Lu, W., Li, J., Lin, L., et al. (2020). Adsorption of Nitrogen Dioxide in a Redox-Active Vanadium Metal-Organic Framework Material. J. Am. Chem. Soc. 142, 15235–15239. doi:10.1021/jacs.0c06414
Hao, L., Gu, H., Duan, N., Wu, S., Ma, X., Xia, Y., et al. (2017). An Enhanced Chemiluminescence Resonance Energy Transfer Aptasensor Based on Rolling Circle Amplification and WS2 Nanosheet for Staphylococcus aureus Detection. Anal. Chim. Acta 959, 83–90. doi:10.1016/j.aca.2016.12.045
He, J., Wang, J., Zhang, N., Shen, L., Wang, L., Xiao, X., et al. (2019). In Vitro selection of DNA Aptamers Recognizing Drug-Resistant Ovarian Cancer by Cell-SELEX. Talanta 194, 437–445. doi:10.1016/j.talanta.2018.10.028
He, X., Li, Y., He, D., Wang, K., Shangguan, J., and Shi, H (2014). Aptamer-Fluorescent Silica Nanoparticles Bioconjugates Based Dual-Color Flow Cytometry for Specific Detection of Staphylococcus aureus. J. Biomed. Nanotechnol. 10, 1359–1368.
Hernández, R., Vallés, C., Benito, A. M., Maser, W. K., Xavier Rius, F., and Riu, J. (2014). Graphene-based Potentiometric Biosensor for the Immediate Detection of Living Bacteria. Biosens. Bioelectron. 54, 553–557. doi:10.1016/j.bios.2013.11.053
Hoa, X. D., Kirk, A. G., and Tabrizian, M. (2007). Towards Integrated and Sensitive Surface Plasmon Resonance Biosensors: a Review of Recent Progress. Biosens. Bioelectron. 23, 151–160. doi:10.1016/j.bios.2007.07.001
Huang, L., Sun, D-W., Wu, Z., Pu, H., and Wei, Q. (2021). Reproducible, Shelf-Stable, and Bioaffinity SERS Nanotags Inspired by Multivariate Polyphenolic Chemistry for Bacterial Identification. Analytica. Chimica. Acta. 1167.
Huang, T., Yang, J., Zhou, W., Liu, X., Pan, Y., and Song, Y. (2019). Rapid Identification of Urinary Tract Infections Based on Ultrasensitive Bacteria Detection Using Volumetric Bar-Chart Chip. Sensors Actuators B-Chemical 298. doi:10.1016/j.snb.2019.126885
Hulme, J. (2017). Recent Advances in the Detection of Methicillin Resistant Staphylococcus aureus (MRSA). BioChip J. 11, 89–100. doi:10.1007/s13206-016-1201-9
Javani, S., Lorca, R., Latorre, A., Flors, C., Cortajarena, A. L., and Somoza, Á. (2016). Antibacterial Activity of DNA-Stabilized Silver Nanoclusters Tuned by Oligonucleotide Sequence. ACS Appl. Mat. Interfaces 8, 10147–10154. doi:10.1021/acsami.6b00670
Jevons, M. P. (1961). "Celbenin" - Resistant Staphylococci. Bmj 1, 124–125. doi:10.1136/bmj.1.5219.124-a
Jia, F., Duan, N., Wu, S., Ma, X., Xia, Y., Wang, Z., et al. (2014). Impedimetric Aptasensor for Staphylococcus aureus Based on Nanocomposite Prepared from Reduced Graphene Oxide and Gold Nanoparticles. Microchim. Acta 181, 967–974. doi:10.1007/s00604-014-1195-8
Jiao, T., Mehedi Hassan, M., Zhu, J., Ali, S., Ahmad, W., Wang, J., et al. (2021). Quantification of Deltamethrin Residues in Wheat by Ag@ZnO NFs-Based Surface-Enhanced Raman Spectroscopy Coupling Chemometric Models. Food Chem. 337, 127652. doi:10.1016/j.foodchem.2020.127652
Jo, N., Kim, B., Lee, S.-M., Oh, J., Park, I. H., Jin Lim, K., et al. (2018). Aptamer-functionalized Capacitance Sensors for Real-Time Monitoring of Bacterial Growth and Antibiotic Susceptibility. Biosens. Bioelectron. 102, 164–170. doi:10.1016/j.bios.2017.11.010
Kern, W. V., and Rieg, S. (2020). Burden of Bacterial Bloodstream Infection-A Brief Update on Epidemiology and Significance of Multidrug-Resistant Pathogens. Clin. Microbiol. Infect. 26, 151–157. doi:10.1016/j.cmi.2019.10.031
Khateb, H., Klös, G., Meyer, R. L., and Sutherland, D. S. (2020). Development of a Label-free LSPR-Apta Sensor for Staphylococcus aureus Detection. ACS Appl. Bio Mat. 3, 3066–3077. doi:10.1021/acsabm.0c00110
Kurt, H., Yuce, M., Hussain, B., and Budak, H. (2016). Dual-Excitation Upconverting Nanoparticle and Quantum Dot Aptasensor for Multiplexed Food Pathogen Detection. Biosens. Bioelectron. 81, 280–286.
Lei, M., Xu, C., Shan, Y., Xia, C., Wang, R., Ran, H-H., et al. (2020). Plasmon-Coupled Microcavity Aptasensors for Visual and Ultra-Sensitive Simultaneous Detection of Staphylococcus aureus and Escherichia coli. Anal. Bioanal. Chem. 412, 8117–8126.
Li, H., Chen, Q., Mehedi Hassan, M., Chen, X., Ouyang, Q., Guo, Z., et al. (2017a). A Magnetite/PMAA Nanospheres-Targeting SERS Aptasensor for Tetracycline Sensing Using Mercapto Molecules Embedded Core/shell Nanoparticles for Signal Amplification. Biosens. Bioelectron. 92, 192–199. doi:10.1016/j.bios.2017.02.009
Li, J., Jiang, H., Rao, X., Liu, Z., Zhu, H., and Xu, Y. (2019a). Point-of-Care Testing of Pathogenic Bacteria at the Single-Colony Level via Gas Pressure Readout Using Aptamer-Coated Magnetic CuFe2O4 and Vancomycin-Capped Platinum Nanoparticles. Anal. Chem. 91, 1494–1500. doi:10.1021/acs.analchem.8b04584
Li, W.-M., Zhou, L.-L., Zheng, M., and Fang, J. (2018). Selection of Metastatic Breast Cancer Cell-specific Aptamers for the Capture of CTCs with a Metastatic Phenotype by Cell-SELEX. Mol. Ther. - Nucleic Acids 12, 707–717. doi:10.1016/j.omtn.2018.07.008
Li, Y.-Z., Li, T.-T., Chen, W., and Song, Y.-Y. (2017b). Co4N Nanowires: Noble-Metal-Free Peroxidase Mimetic with Excellent Salt- and Temperature-Resistant Abilities. ACS Appl. Mat. Interfaces 9, 29881–29888. doi:10.1021/acsami.7b09861
Li, Z., Mohamed, M. A., and Vinu Mohan, A. M. (2019b). Application of Electrochemical Aptasensors toward Clinical Diagnostics, Food, and Environmental Monitoring: Review. Sensors (Basel) 19, 5435. doi:10.3390/s19245435
Lian, Y., He, F., Wang, H., and Tong, F. (2015). A New Aptamer/Graphene Interdigitated Gold Electrode Piezoelectric Sensor for Rapid and Specific Detection of Staphylococcus aureus. Biosens. Bioelectron. 65, 314–319.
Lim, S. H., Ryu, Y. C., and Hwang, B. H. (2021). Aptamer-immobilized Gold Nanoparticles Enable Facile and On-site Detection of Staphylococcus aureus. Biotechnol. Bioprocess Eng. 26, 107–113.
Liu, L. S., Wang, F., Ge, Y., and Lo, P. K. (2021a). Recent Developments in Aptasensors for Diagnostic Applications. ACS Appl. Mat. Interfaces 13, 9329–9358. doi:10.1021/acsami.0c14788
Liu, S., Li, H., Hassan, M. M., Ali, S., and Chen, Q. (2021b). SERS Based Artificial Peroxidase Enzyme Regulated Multiple Signal Amplified System for Quantitative Detection of Foodborne Pathogens. Food Control 123.
Liu, Y., Tu, D., Zhu, H., and Chen, X. (2013). Lanthanide-doped Luminescent Nanoprobes: Controlled Synthesis, Optical Spectroscopy, and Bioapplications. Chem. Soc. Rev. 42, 6924–6958. doi:10.1039/c3cs60060b
Lorenz, C., von Pelchrzim, F., and Schroeder, R. (2006). Genomic Systematic Evolution of Ligands by Exponential Enrichment (Genomic SELEX) for the Identification of Protein-Binding RNAs Independent of Their Expression Levels. Nat. Protoc. 1, 2204–2212. doi:10.1038/nprot.2006.372
Lou, X., Qian, J., Xiao, Y., Viel, L., Gerdon, A. E., Lagally, E. T., et al. (2009). Micromagnetic Selection of Aptamers in Microfluidic Channels. Proc. Natl. Acad. Sci. U.S.A. 106, 2989–2994. doi:10.1073/pnas.0813135106
Lu, C., Gao, X., Chen, Y., Ren, J., and Liu, C. (2020a). Aptamer-Based Lateral Flow Test Strip for the Simultaneous Detection of Salmonella typhimurium, Escherichia coli O157:H7 and Staphylococcus aureus. Anal. Lett. 53, 646–659. doi:10.1080/00032719.2019.1663528
Lu, Y., Yuan, Z., Bai, J., Lin, Q., Deng, R., Luo, A., et al. (2020b). Directly Profiling Intact Staphylococcus aureus in Water and Foods via Enzymatic Cleavage Aptasensor. Anal. Chim. Acta 1132, 28–35. doi:10.1016/j.aca.2020.07.058
Ma, X., Lin, X., Xu, X., and Wang, Z. (2021). Fabrication of Gold/silver Nanodimer SERS Probes for the Simultaneous Detection of Salmonella typhimurium and Staphylococcus aureus. Microchim. Acta 188, 202. doi:10.1007/s00604-021-04791-4
Maduraiveeran, G., Sasidharan, M., and Ganesan, V. (2018). Electrochemical Sensor and Biosensor Platforms Based on Advanced Nanomaterials for Biological and Biomedical Applications. Biosens. Bioelectron. 103, 113–129. doi:10.1016/j.bios.2017.12.031
Maldonado, J., Estevez, M. C., Fernandez-Gavela, A., Jose Gonzalez-Lopez, J., Belen Gonzalez-Guerrero, A., and Lechuga, L. M. (2020). Label-free Detection of Nosocomial Bacteria Using a Nanophotonic Interferometric Biosensor. Analyst. 145, 497–506.
Mendonsa, S. D., and Bowser, M. T. (2004). In Vitro evolution of Functional DNA Using Capillary Electrophoresis. J. Am. Chem. Soc. 126, 20–21. doi:10.1021/ja037832s
Miyachi, Y., Shimizu, N., Ogino, C., and Kondo, A. (2010). Selection of DNA Aptamers Using Atomic Force Microscopy. Nucleic Acids Res. 38, e21. doi:10.1093/nar/gkp1101
Mokhtarzadeh, A., Ezzati Nazhad Dolatabadi, J., Abnous, K., de la Guardia, M., and Ramezani, M. (2015). Nanomaterial-based Cocaine Aptasensors. Biosens. Bioelectron. 68, 95–106. doi:10.1016/j.bios.2014.12.052
Moon, J., Kim, G., Park, S., Lim, J., and Mo, C. (2015). Comparison of Whole-Cell SELEX Methods for the Identification of Staphylococcus Aureus-specific DNA Aptamers. SENSORS 15, 8884–8897. doi:10.3390/s150408884
Morales, M. A., and Halpern, J. M. (2018). Guide to Selecting a Biorecognition Element for Biosensors. Bioconjugate Chem. 29, 3231–3239. doi:10.1021/acs.bioconjchem.8b00592
Muñoz-Bustos, C., Tirado-Guízar, A., Paraguay-Delgado, F., and Pina-Luis, G. (2017). Copper Nanoclusters-Coated BSA as a Novel Fluorescence Sensor for Sensitive and Selective Detection of Mangiferin. Sensors Actuators B Chem. 244, 922–927. doi:10.1016/j.snb.2017.01.071
Nguyen, T. T.-Q., Kim, E. R., and Gu, M. B. (2022). A New Cognate Aptamer Pair-Based Sandwich-type Electrochemical Biosensor for Sensitive Detection of Staphylococcus aureus. Biosens. Bioelectron. 198, 113835. doi:10.1016/j.bios.2021.113835
Nouri, A., Ahari, H., and Shahbazzadeh, D. (2018). Designing a Direct ELISA Kit for the Detection of Staphylococcus aureus Enterotoxin A in Raw Milk Samples. Int. J. Biol. Macromol. 107, 1732–1737. doi:10.1016/j.ijbiomac.2017.10.052
Ouyang, Q., Wang, L., Ahmad, W., Yang, Y., and Chen, Q. (2021a). Upconversion Nanoprobes Based on a Horseradish Peroxidase-Regulated Dual-Mode Strategy for the Ultrasensitive Detection of Staphylococcus aureus in Meat. J. Agric. Food Chem. 69, 9947–9956.
Ouyang, Q., Yang, Y., Ali, S., Wang, L., Li, H., and Chen, Q. (2021b). Upconversion Nanoparticles-Based FRET System for Sensitive Detection of Staphylococcus aureus. Spectrochimica Acta Part a-Molecular And Biomol. Spectrosc. 255, 119734. doi:10.1016/j.saa.2021.119734
Pang, Y., Wan, N., Shi, L., Wang, C., Sun, Z., Xiao, R., et al. (2019). Dual-Recognition Surface-Enhanced Raman Scattering(SERS) Biosensor for Pathogenic Bacteria Detection by Using Vancomycin-SERS Tags and Aptamer-Fe3O4@Au. Analytica. Chimica. Acta. 1077, 288–296.
Paniel, N., Baudart, J., Hayat, A., and Barthelmebs, L. (2013). Aptasensor and Genosensor Methods for Detection of Microbes in Real World Samples. Methods 64, 229–240. doi:10.1016/j.ymeth.2013.07.001
Pebdeni, A. B., Hosseini, M., and Ganjali, M. R. (2020). Fluorescent Turn-On Aptasensor of Staphylococcus aureus Based on the FRET between Green Carbon Quantum Dot and Gold Nanoparticle. Food Anal. Methods 13, 2070–2079. doi:10.1007/s12161-020-01821-4
Pebdeni, A. B., Mousavizadegan, M., and Hosseini, M. (2021). Sensitive Detection of S.Aureus Using Aptamer- and Vancomycin-Copper Nanoclusters as Dual Recognition Strategy. Food Chem. 361, 130137. doi:10.1016/j.foodchem.2021.130137
Pla, L., Santiago-Felipe, S., Angeles Tormo-Mas, M., Peman, J., Sancenon, F., Aznar, E., et al. (2020). Aptamer-Capped Nanoporous Anodic Alumina for Staphylococcus aureus Detection. Sensors Actuators B-Chemical 320, 128281. doi:10.1016/j.snb.2020.128281
Rahman, M. R. T., Lou, Z., Wang, H., and Ai, L. (2015). Aptamer Immobilized Magnetoelastic Sensor for the Determination of Staphylococcus aureus. Analytical Letters. 48, 2414–2422.
Rajapaksha, P., Elbourne, A., Gangadoo, S., Brown, R., Cozzolino, D., and Chapman, J. (2019). A Review of Methods for the Detection of Pathogenic Microorganisms. Analyst 144, 396–411. doi:10.1039/c8an01488d
Raji, M. A., Suaifan, G., Shibl, A., Weber, K., Cialla-May, D., Popp, J., et al. (2021). Aptasensor for the Detection of Methicillin Resistant Staphylococcus aureus on Contaminated Surfaces. Biosens. Bioelectron. 176, 112910. doi:10.1016/j.bios.2020.112910
Ramlal, S., Mondal, B., Lavu, P. S., N., B., and Kingston, J. (2018). Capture and Detection of Staphylococcus aureus with Dual Labeled Aptamers to Cell Surface Components. Int. J. Food Microbiol. 265, 74–83. doi:10.1016/j.ijfoodmicro.2017.11.002
Ranjbar, S., and Shahrokhian, S. (2018). Design and Fabrication of an Electrochemical Aptasensor Using Au Nanoparticles/carbon Nanoparticles/cellulose Nanofibers Nanocomposite for Rapid and Sensitive Detection of Staphylococcus aureus. Bioelectrochemistry 123, 70–76. doi:10.1016/j.bioelechem.2018.04.018
Reich, P., Stoltenburg, R., Strehlitz, B., Frense, D., and Beckmann, D. (2017). Development of An Impedimetric Aptasensor for the Detection of Staphylococcus aureus. Int. J. Mol. Sci. 18.
Reinemann, C., Freiin von Fritsch, U., Rudolph, S., and Strehlitz, B. (2016). Generation and Characterization of Quinolone-specific DNA Aptamers Suitable for Water Monitoring. Biosens. Bioelectron. 77, 1039–1047. doi:10.1016/j.bios.2015.10.069
Ribes, À., Aznar, E., Santiago-Felipe, S., Xifre-Perez, E., Tormo-Mas, M. Á., Pemán, J., et al. (2019). Selective and Sensitive Probe Based in Oligonucleotide-Capped Nanoporous Alumina for the Rapid Screening of Infection Produced by Candida Albicans. ACS Sens. 4, 1291–1298. doi:10.1021/acssensors.9b00169
Rubab, M., Shahbaz, H. M., Olaimat, A. N., and Oh, D.-H. (2018). Biosensors for Rapid and Sensitive Detection of Staphylococcus aureus in Food. Biosens. Bioelectron. 105, 49–57. doi:10.1016/j.bios.2018.01.023
Sefah, K., Yang, Z., Bradley, K. M., Hoshika, S., Jiménez, E., Zhang, L., et al. (2014). In Vitro selection with Artificial Expanded Genetic Information Systems. Proc. Natl. Acad. Sci. U.S.A. 111, 1449–1454. doi:10.1073/pnas.1311778111
Shangguan, J., Li, Y., He, D., He, X., Wang, K., Zou, Z., et al. (2015). A Combination of Positive Dielectrophoresis Driven on-Line Enrichment and Aptamer-Fluorescent Silica Nanoparticle Label for Rapid and Sensitive Detection of Staphylococcus aureus. Analyst. 140, 4489–4497.
Sharifi, M., Hosseinali, S. H., Hossein Alizadeh, R., Hasan, A., Attar, F., Salihi, A., et al. (2020). Plasmonic and Chiroplasmonic Nanobiosensors Based on Gold Nanoparticles. Talanta 212, 120782. doi:10.1016/j.talanta.2020.120782
Sharma, A., Catanante, G., Hayat, A., Istamboulie, G., Ben Rejeb, I., Bhand, S., et al. (2016). Development of Structure Switching Aptamer Assay for Detection of Aflatoxin M1 in Milk Sample. Talanta 158, 35–41. doi:10.1016/j.talanta.2016.05.043
Shen, J., Li, Y., Gu, H., Xia, F., and Zuo, X. (2014). Recent Development of Sandwich Assay Based on the Nanobiotechnologies for Proteins, Nucleic Acids, Small Molecules, and Ions. Chem. Rev. 114, 7631–7677. doi:10.1021/cr300248x
Shen, Y., Wu, T., Zhang, Y., Ling, N., Zheng, L., Zhang, S.-L., et al. (2020). Engineering of a Dual-Recognition Ratiometric Fluorescent Nanosensor with a Remarkably Large Stokes Shift for Accurate Tracking of Pathogenic Bacteria at the Single-Cell Level. Anal. Chem. 92, 13396–13404. doi:10.1021/acs.analchem.0c02762
Sheng, L., Lu, Y., Deng, S., Liao, X., Zhang, K., Ding, T., et al. (2019). A Transcription Aptasensor: Amplified, Label-Free and Culture-Independent Detection of Foodborne Pathogens via Light-up RNA Aptamers. Chem. Commun. 55, 10096–10099.
Shrivastava, S., Lee, W-I., and Lee, N-E. (2018). Culture-Free, Highly Sensitive, Quantitative Detection of Bacteria From Minimally Processed Samples Using Fluorescence Imaging by Smartphone. Biosens. Bioelectron. 109, 90–97.
Su, L., Feng, J., Zhou, X., Ren, C., Li, H., and Chen, X. (2012). Colorimetric Detection of Urine Glucose Based ZnFe2O4 Magnetic Nanoparticles. Anal. Chem. 84, 5753–5758. doi:10.1021/ac300939z
Sun, Z., Peng, Y., Wang, M., Lin, Y., Jalalah, M., Alsareii, S. A., et al. (2021). Electrochemical Deposition of Cu Metal-Organic Framework Films for the Dual Analysis of Pathogens. Anal. Chem. 93, 8994–9001. doi:10.1021/acs.analchem.1c01763
Taghdisi, S. M., Danesh, N. M., Beheshti, H. R., Ramezani, M., and Abnous, K. (2016). A Novel Fluorescent Aptasensor Based on Gold and Silica Nanoparticles for the Ultrasensitive Detection of Ochratoxin A. Nanoscale 8, 3439–3446. doi:10.1039/c5nr08234j
Tao, X., Liao, Z., Zhang, Y., Fu, F., Hao, M., Song, Y., et al. (2021). Aptamer-quantum Dots and Teicoplanin-Gold Nanoparticles Constructed FRET Sensor for Sensitive Detection of Staphylococcus aureus. Chin. Chem. Lett. 32, 791–795. doi:10.1016/j.cclet.2020.07.020
Teng, J., Yuan, F., Ye, Y., Zheng, L., Yao, L., Xue, F., et al. (2016). Aptamer-Based Technologies in Foodborne Pathogen Detection. Front. Microbiol. 7, 1426. doi:10.3389/fmicb.2016.01426
Tok, J. B.-H., Cho, J., and Rando, R. R. (2000). RNA Aptamers that Specifically Bind to a 16S Ribosomal RNA Decoding Region Construct. Nucleic Acids Res. 28, 2902–2910. doi:10.1093/nar/28.15.2902
Tong, S. Y. C., Davis, J. S., Eichenberger, E., Holland, T. L., and Fowler, V. G. (2015). Staphylococcus aureus Infections: Epidemiology, Pathophysiology, Clinical Manifestations, and Management. Clin. Microbiol. Rev. 28, 603–661. doi:10.1128/cmr.00134-14
Tuerk, C., and Gold, L. (1990). Systematic Evolution of Ligands by Exponential Enrichment: RNA Ligands to Bacteriophage T4 DNA Polymerase. Science 249, 505–510. doi:10.1126/science.2200121
Tuerk, C., MacDougal, S., and Gold, L. (1992). RNA Pseudoknots that Inhibit Human Immunodeficiency Virus Type 1 Reverse Transcriptase. Proc. Natl. Acad. Sci. U.S.A. 89, 6988–6992. doi:10.1073/pnas.89.15.6988
Umesha, S., and Manukumar, H. M. (2018). Advanced Molecular Diagnostic Techniques for Detection of Food-Borne Pathogens: Current Applications and Future Challenges. Crit. Rev. Food Sci. Nutr. 58, 84–104. doi:10.1080/10408398.2015.1126701
Wang, C.-H., Wu, J.-J., and Lee, G.-B. (2019a). Screening of Highly-specific Aptamers and Their Applications in Paper-Based Microfluidic Chips for Rapid Diagnosis of Multiple Bacteria. Sensors Actuators B Chem. 284, 395–402. doi:10.1016/j.snb.2018.12.112
Wang, C.-W., Chung, W.-H., Cheng, Y.-F., Ying, N.-W., Peck, K., Chen, Y.-T., et al. (2013). A New Nucleic Acid-Based Agent Inhibits Cytotoxic T Lymphocyte-Mediated Immune Disorders. J. Allergy Clin. Immunol. 132, 713–722. e711. doi:10.1016/j.jaci.2013.04.036
Wang, D., Lian, F., Yao, S., Liu, Y., Wang, J., Song, X., et al. (2020). Simultaneous Detection of Three Foodborne Pathogens Based on Immunomagnetic Nanoparticles and Fluorescent Quantum Dots. ACS Omega 5, 23070–23080. doi:10.1021/acsomega.0c02833
Wang, F., Banerjee, D., Liu, Y., Chen, X., and Liu, X. (2010). Upconversion Nanoparticles in Biological Labeling, Imaging, and Therapy. Analyst 135, 1839–1854. doi:10.1039/c0an00144a
Wang, J., Li, H., Li, T., and Ling, L. (2018). Determination of Bacterial DNA Based on Catalytic Oxidation of Cysteine by G-Quadruplex DNAzyme Generated from Asymmetric PCR: Application to the Colorimetric Detection of Staphylococcus aureus. Microchim. Acta 185, 410. doi:10.1007/s00604-018-2935-y
Wang, J., Wu, X., Wang, C., Shao, N., Dong, P., Xiao, R., et al. (2015). Magnetically Assisted Surface-Enhanced Raman Spectroscopy for the Detection of Staphylococcus aureus Based on Aptamer Recognition. ACS Appl. Mater. Interfaces 7, 20919–20929.
Wang, S., Deng, W., Yang, L., Tan, Y., Xie, Q., and Yao, S. (2017a). Copper-Based Metal-Organic Framework Nanoparticles with Peroxidase-like Activity for Sensitive Colorimetric Detection of Staphylococcus aureus. ACS Appl. Mat. Interfaces 9, 24440–24445. doi:10.1021/acsami.7b07307
Wang, W. W., Han, X., and Chu, L. Q. (2019b). Polyadenine-mediated Immobilization of Aptamers on a Gold Substrate for the Direct Detection of Bacterial Pathogens. Anal Sci. 35, 967–972.
Wang, X., Liu, W., Yin, B., Sang, Y., Liu, Z., Dai, Y., et al. (2017b). An Isothermal Strand Displacement Amplification Strategy for Nucleic Acids Using Junction Forming Probes and Colorimetric Detection. Microchim. Acta 184, 1603–1610. doi:10.1007/s00604-017-2158-7
Woo, M.-A., Kim, M., Jung, J., Park, K., Seo, T., and Park, H. (2013). A Novel Colorimetric Immunoassay Utilizing the Peroxidase Mimicking Activity of Magnetic Nanoparticles. Ijms 14, 9999–10014. doi:10.3390/ijms14059999
Wu, S., Duan, N., Shi, Z., Fang, C., and Wang, Z. (2014). Simultaneous Aptasensor for Multiplex Pathogenic Bacteria Detection Based on Multicolor Upconversion Nanoparticles Labels. Anal. Chem. 86, 3100–3107. doi:10.1021/ac404205c
Xu, J., Guo, J., Maina, S. W., Yang, Y., Hu, Y., Li, X., et al. (2018). An Aptasensor for staphylococcus Aureus Based on Nicking Enzyme Amplification Reaction and Rolling Circle Amplification. Anal. Biochem. 549, 136–142. doi:10.1016/j.ab.2018.03.013
Xu, K., Zhou, R., Takei, K., and Hong, M. (2019). Toward Flexible Surface‐Enhanced Raman Scattering (SERS) Sensors for Point‐of‐Care Diagnostics. Adv. Sci. 6, 1900925. doi:10.1002/advs.201900925
Yang, M., Chen, X., Zhu, L., Lin, S., Li, C., Li, X., et al. (2021a). Aptamer-Functionalized DNA-Silver Nanocluster Nanofilm for Visual Detection and Elimination of Bacteria. ACS Appl. Mat. Interfaces 13, 38647–38655. doi:10.1021/acsami.1c05751
Yang, Y., Wu, T., Xu, L. P., and Zhang, X. (2021b). Portable Detection of Staphylococcus aureus Using Personal Glucose Meter Based on Hybridization Chain Reaction Strategy. Talanta 226, 122132. doi:10.1016/j.talanta.2021.122132
Yao, J., Yang, M., and Duan, Y. (2014). Chemistry, Biology, and Medicine of Fluorescent Nanomaterials and Related Systems: New Insights into Biosensing, Bioimaging, Genomics, Diagnostics, and Therapy. Chem. Rev. 114, 6130–6178. doi:10.1021/cr200359p
Yao, S., Zhao, C., Shang, M., Li, J., and Wang, J. (2021). Enzyme-free and Label-free Detection of Staphylococcus aureus Based on Target-Inhibited Fluorescence Signal Recovery. Food Chem. Toxicol. 150, 112071. doi:10.1016/j.fct.2021.112071
Yao, S., Li, J., Pang, B., Wang, X., Shi, Y., Song, X., et al. (2020). Colorimetric Immunoassay for Rapid Detection of Staphylococcus aureus Based on Etching-Enhanced Peroxidase-like Catalytic Activity of Gold Nanoparticles. Microchimica Acta. 187.
Ye, Y., Zheng, L., Wu, T., Ding, X., Chen, F., Yuan, Y., et al. (2020). Size-Dependent Modulation of Polydopamine Nanospheres on Smart Nanoprobes for Detection of Pathogenic Bacteria at Single-Cell Level and Imaging-Guided Photothermal Bactericidal Activity. ACS Appl. Mat. Interfaces 12, 35626–35637. doi:10.1021/acsami.0c07784
Yu, H., Alkhamis, O., Canoura, J., Liu, Y., and Xiao, Y. (2021). Advances and Challenges in Small‐Molecule DNA Aptamer Isolation, Characterization, and Sensor Development. Angew. Chem. Int. Ed. 60, 16800–16823. doi:10.1002/anie.202008663
Yu, M., Wang, H., Fu, F., Li, L., Li, J., Li, G., et al. (2017). Dual-Recognition Förster Resonance Energy Transfer Based Platform for One-step Sensitive Detection of Pathogenic Bacteria Using Fluorescent Vancomycin-Gold Nanoclusters and Aptamer-Gold Nanoparticles. Anal. Chem. 89, 4085–4090. doi:10.1021/acs.analchem.6b04958
Yu, T., Xu, H., Zhao, Y., Han, Y., Zhang, Y., Zhang, J., et al. (2020). Aptamer Based High Throughput Colorimetric Biosensor for Detection of staphylococcus Aureus. Sci. Rep. 10, 9190. doi:10.1038/s41598-020-66105-7
Yuan, J., Yu, Y., Li, C., Ma, X., Xia, Y., Chen, J., et al. (2014a). Visual Detection and Microplate Assay for Staphylococcus aureus Based on Aptamer Recognition Coupled to Tyramine Signal Amplification. Microchim. Acta 181, 321–327. doi:10.1007/s00604-013-1120-6
Yuan, J., Wu, S., Duan, N., Ma, X., Xia, Y., Chen, J., et al. (2014b). A Sensitive Gold Nanoparticle-Based Colorimetric Aptasensor for Staphylococcus aureus. Talanta. 127, 163–168.
Zelada-Guillén, G. A., Sebastián-Avila, J. L., Blondeau, P., Riu, J., and Rius, F. X. (2012). Label-free Detection of Staphylococcus aureus in Skin Using Real-Time Potentiometric Biosensors Based on Carbon Nanotubes and Aptamers. Biosens. Bioelectron. 31, 226–232. doi:10.1016/j.bios.2011.10.021
Zhang, H., Liu, Y., Yao, S., Shang, M., Zhao, C., Li, J., et al. (2021a). A Multicolor Sensing System for Simultaneous Detection of Four Foodborne Pathogenic Bacteria Based on Fe3O4/MnO2 Nanocomposites and the Etching of Gold Nanorods. Food Chem. Toxicol. 149, 112035. doi:10.1016/j.fct.2021.112035
Zhang, H., Ma, X., Liu, Y., Duan, N., Wu, S., Wang, Z., et al. (2015a). Gold Nanoparticles Enhanced SERS Aptasensor for the Simultaneous Detection of Salmonella typhimurium and Staphylococcus aureus. Biosens. Bioelectron. 74, 872–877. doi:10.1016/j.bios.2015.07.033
Zhang, H., Yao, S., Song, X., Xu, K., Wang, J., Li, J., et al. (2021b). One-step Colorimetric Detection of Staphylococcus aureus Based on Target-Induced Shielding against the Peroxidase Mimicking Activity of Aptamer-Functionalized Gold-Coated Iron Oxide Nanocomposites. Talanta 232, 122448. doi:10.1016/j.talanta.2021.122448
Zhang, X., Wang, X., Yang, Q., Jiang, X., Li, Y., Zhao, J., et al. (2020). Conductometric Sensor for Viable Escherichia coli and Staphylococcus aureus Based on Magnetic Analyte Separation via Aptamer. Mikrochim. Acta 187, 43. doi:10.1007/s00604-019-3880-0
Zhang, X., Huang, C., Xu, S., Chen, J., Zeng, Y., Wu, P., et al. (2015b). Photocatalytic Oxidation of TMB with the Double Stranded DNA-SYBR Green I Complex for Label-free and Universal Colorimetric Bioassay. Chem. Commun. 51, 14465–14468. doi:10.1039/c5cc06105a
Zhang, X., Wang, K., Liu, M., Zhang, X., Tao, L., Chen, Y., et al. (2015c). Polymeric AIE-Based Nanoprobes for Biomedical Applications: Recent Advances and Perspectives. Nanoscale 7, 11486–11508. doi:10.1039/c5nr01444a
Zhang, Y., Tan, W., Zhang, Y., Mao, H., Shi, S., Duan, L., et al. (2019). Ultrasensitive and Selective Detection of Staphylococcus aureus Using a Novel IgY-Based Colorimetric Platform. Biosens. Bioelectron. 142, 111570. doi:10.1016/j.bios.2019.111570
Zhou, J., and Rossi, J. (2017). Aptamers as Targeted Therapeutics: Current Potential and Challenges. Nat. Rev. Drug Discov. 16, 181–202. doi:10.1038/nrd.2016.199
Zhu, A., Ali, S., Xu, Y., Ouyang, Q., and Chen, Q. (2021a). A SERS Aptasensor Based on AuNPs Functionalized PDMS Film for Selective and Sensitive Detection of Staphylococcus aureus. Biosens. Bioelectron. 172, 112806. doi:10.1016/j.bios.2020.112806
Zhu, A., Jiao, T., Ali, S., Xu, Y., Ouyang, Q., and Chen, Q. (2021b). SERS Sensors Based on Aptamer-Gated Mesoporous Silica Nanoparticles for Quantitative Detection of Staphylococcus aureus with Signal Molecular Release. Anal. Chem. 93, 9788–9796. doi:10.1021/acs.analchem.1c01280
Zhu, J., Li, J.-j., Wang, A.-q., Chen, Y., and Zhao, J.-w. (2010). Fluorescence Quenching of Alpha-Fetoprotein by Gold Nanoparticles: Effect of Dielectric Shell on Non-radiative Decay. Nanoscale Res. Lett. 5, 1496–1501. doi:10.1007/s11671-010-9668-0
Keywords: Staphylococcus aureus, aptasensor, optical biosensor, electrochemical biosensor, nanomaterials, POCT
Citation: Chen W, Lai Q, Zhang Y and Liu Z (2022) Recent Advances in Aptasensors For Rapid and Sensitive Detection of Staphylococcus Aureus. Front. Bioeng. Biotechnol. 10:889431. doi: 10.3389/fbioe.2022.889431
Received: 04 March 2022; Accepted: 12 April 2022;
Published: 23 May 2022.
Edited by:
Han-Sheng Chuang, National Cheng Kung University, TaiwanReviewed by:
Nien-Tsu Huang, National Taiwan University, TaiwanMegan Yi-Ping Ho, The Chinese University of Hong Kong, China
Copyright © 2022 Chen, Lai, Zhang and Liu. This is an open-access article distributed under the terms of the Creative Commons Attribution License (CC BY). The use, distribution or reproduction in other forums is permitted, provided the original author(s) and the copyright owner(s) are credited and that the original publication in this journal is cited, in accordance with accepted academic practice. No use, distribution or reproduction is permitted which does not comply with these terms.
*Correspondence: Wei Chen, 573570494@qq.com; Zhengchun Liu, liuzhengchunseu@126.com