- 1Department of Dermatology, Changzheng Hospital, Naval Medical University, Shanghai, China
- 2Orthopaedic Oncology Center, Department of Orthopedics, Changzheng Hospital, Naval Medical University, Shanghai, China
Skin cancer has drawn attention for the increasing incident rates and high morbidity worldwide. Timely diagnosis and efficient treatment are of paramount importance for prompt and effective therapy. Thus, the development of novel skin cancer diagnosis and treatment strategies is of great significance for both fundamental research and clinical practice. Recently, the emerging field of nanotechnology has profoundly impact on early diagnosis and better treatment planning of skin cancer. In this review, we will discuss the current encouraging advances in functional nanomaterials for skin cancer theranostics. Challenges in the field and safety concerns of nanomaterials will also be discussed.
1 Introduction
Skin is a physical barrier made up of cells and intercellular matrix that is robust and long-lasting (Brogden et al., 2012). In humans, skin malignancies display a recurring malignant response in a significant number of cases, with over one million cases reported, with white individuals accounting for the majority of cases (D’Orazio et al., 2013). Ultraviolet (UV) radiation, a key contributing factor in the development of skin photoaging, causes uncontrolled cell growth and the death of keratinocytes (Lopes et al., 2021).
Skin cancers can be categorized into melanoma skin cancers (MSC) and non-melanoma skin cancers (NMSC) (Abi Karam et al., 2021). NMSC are then classified into basal cell carcinoma (BCC), squamous cell carcinoma (SCC), and Merkel cell carcinoma (MCC) (Armstrong and Kricker, 2001; Kaur and Kesharwani, 2021; Thai et al., 2021). NMSC are the most commonly occurring cancers worldwide, in which BCC account for 75% and SCC for 20%, respectively (Esteva et al., 2017). Meanwhile, MSC are responsible for a high amount of fatalities, putting a significant strain on medical services (Carlino et al., 2021).
According to the American Academy of Dermatology clinical practice guideline, diagnostic skin biopsy maintains the first line to identify MSC (Swetter et al., 2019). NMSC are generally diagnosed clinically, with histological confirmation after excision (Newlands et al., 2016). Nevertheless, there is the paucity of clinical practice to specifically and accurately diagnose tumor metastasis (Dinnes et al., 2018). Conventional treatment of the primary lesions involves surgical excision, cryotherapy, radiation therapy (RT), and topical agents (Swetter et al., 2019). Once metastasis occurs, chemotherapy, adjuvant immunotherapy, and targeted therapy are suggested (Majem et al., 2021). However, the potential accompanied disadvantages of chemotherapies, including normal cell damage, relatively low bioavailability, and tumor drug resistance, cannot be ignored (Kwon et al., 2021; Taeb et al., 2021; Tarik Alhamdany et al., 2021). As a result of these restrictions, skin cancer theranostics are unsatisfactory.
In recent decades, nanomaterials have given rise to a new discipline, which has gotten a lot of interest in the field of cancer theranostics (Song et al., 2010, 2016; Liu et al., 2018). Certain nanomaterials could concentrate in tumor primary site, lymph node metastases, and distant metastasis, which provides the ability for targeted imaging and efficient anti-cancer effect (Chen and Cai, 2014; Zhang P. et al., 2021; Gracia et al., 2021). In comparison to conventional nanomedicine, functionalized nanoparticles have several benefits, including increased therapeutic effectiveness and delivery, increased drug solubility, improved pharmacokinetic profile, and prolonged blood circulation time. Chemical and biofunctionalization are currently two strategies for the modification of nanoparticles. In addition, these advanced nanotechnologies assist the stabilization of anti-cancer drugs, which improve the bioavailability and controlled release (Chen and Stephen Inbaraj, 2019; Jokioja et al., 2021; Rashwan et al., 2021; Shen et al., 2022).
2 DILEMMA OF SKIN CANCER THERANOSTICS
2.1 Melanoma Skin Cancers
MSCare caused by abnormal melanogenic cells called melanocytes, which proliferate excessively and spread invasively (Molodtsov et al., 2021). Although most melanomas are pigmented, around 5–10% of cutaneous melanomas are amelanotic, which may cause delayed detection and worse prognosis (Chen and Sebaratnam, 2021; Chuchvara et al., 2021). From dermoscopy through reflectance confocal microscopy (RCM) to histology, this condition emphasizes difficulties in the diagnosis of amelanotic melanoma (Li et al., 2021; Rasmussen et al., 2021; Williams et al., 2021).
MSChave a predisposition for migrating to the brain and central nervous system, causing considerable morbidity and treatment resistance (Johnson and Young, 1996). Patients with active brain metastases were excluded from most of the clinical studies, despite the fact that anti-programmed cell death protein 1 (anti-PD1) and anti-cytotoxic T-lymphocyte antigen 4 (anti-CTLA4) immunotherapies and mitogen-activated protein kinase-targeted (MAPK-targeted) treatments have been extensively employed in the treatment of systemic metastases from melanoma (Eroglu et al., 2019).
The major molecular signaling pathways in MSC include MAPK signaling pathway (Falchook et al., 2012; Cancer Genome Atlas Network, 2015), phosphatase and tensin homolog (PTEN)/phosphoinositol-3-kinase (PI3K) signaling pathway (Damsky et al., 2015), Rac family small GTPase 1 (RAC1) signaling pathway (Krauthammer et al., 2012), and cyclin dependent kinase inhibitor 2A (CDKN2A) (Van Raamsdonk et al., 2009; Kwong et al., 2012; Horn et al., 2013; Mittal and Roberts, 2020). Accordingly, mitogen-activated protein kinase-kinase (MEK) inhibitors (B-Raf proto-oncogene, serine/threonine kinase) BRAF inhibitors, and immunomodulation are recommended for MSC that has progressed to stage IV (Hodi et al., 2010; Villanueva et al., 2010; Chapman et al., 2011; Larkin et al., 2014). Targeted therapy has a high response rate and can improve survival in most MSC cases, patients nearly always relapse and die from the illness (Ahronian et al., 2015; Samson et al., 2019; Foth and McMahon, 2021).
According to published studies, several drug-resistance mechanisms of target therapy have been found and may be categorized as follows: 1) reactivation of MAPK signaling pathways (Poulikakos et al., 2011; Shi et al., 2012, 2014; Johnson et al., 2018), 2) upregulation of p21-activated kinase (PAK) signaling pathway and growth factor receptors (Johannessen et al., 2013; Lu et al., 2017), and 3) low melanocyte inducing transcription factor (MITF) and dedifferentiated cell states (Hoek et al., 2006; Smith et al., 2016). To overcome the therapeutic resistance, inhibition of pathways mentioned above is attempted in clinical trials (Nathanson et al., 2013; Sullivan and Flaherty, 2015; Romano et al., 2018; Sullivan et al., 2018). Nevertheless, inhibition of cyclin dependent kinase4/6 (CDK4/6) and MEK is further limited by adverse effects that need a lower dose, leading to diminished effectiveness and susceptibility to resistance development (Kwong et al., 2012; Guo et al., 2021).
Immunotherapy has revolutionized the treatment of numerous malignancies, probably the most advanced melanoma (Sheng et al., 2021). In Eggermont et al.‘s study, 200 mg of pembrolizumab given every 3 weeks for up to a year as adjuvant therapy for high-risk stage III melanoma resulted in considerably longer recurrence-free survival than placebo, with no new adverse effects (Eggermont et al., 2018). Pembrolizumab adjuvant therapy produced a persistent and clinically substantial improvement in recurrence-free survival in resected high-risk stage III melanoma at a 3-years median follow-up (Eggermont et al., 2020). However, initial and acquired resistance to the therapy inevitably shorten survival and greatly compromised the quality of life in these patients. The underlying mechanisms associated with drug resistance include immune surveillance escape, interferon signaling deficiency, and MITFlow/dedifferentiated phenotype (Sosman et al., 2012; Sade-Feldman et al., 2017; Liu et al., 2019).
Because of the restrictive method including numerous medications, a novel technique for treating melanoma is desperately required (Bowman et al., 2021; Millán-Esteban et al., 2021; Teixido et al., 2021).
2.2 Non-Melanoma Skin Cancers
The majority of NMSC may be efficiently treated with surgery; however, less than 10% of cases are progressed and may need further therapy (Zaar et al., 2016). From an epidemiological viewpoint, SCC and BCC are known as keratinocyte carcinomas, owing to keratinocyte genesis. BCC seldom metastasizes, but commonly features with histological invasion of adjacent tissues, thereby causing significant morbidity (Cameron et al., 2019). The high mortality rate of SCC is mainly due to the complications of metastasis (Apalla et al., 2017). MCC, a rare cancer of the skin, is an aggressive neuroendocrine skin cancer (Kim et al., 2021). Despite the therapeutic efficacy, the increasing incidence of NMSC entails a large health and economic burden worldwide.
2.2.1 Basal Cell Carcinoma
The conventional surgical operation remains the first-line therapy of BCC, which is based on multidisciplinary collaboration. Knowing more about the molecular mechanisms contributing to BCC progression can help us develop more effective therapeutic regimens. Among the important molecular pathways in BCC development is the Hedgehog (HH) signaling pathway (Wong and Reiter, 2008). Based on the mechanisms activated along the HH pathway, several targeted therapies have been developed to apply for advanced BCC (Dessinioti et al., 2014). In the clinical trial of HH inhibitors, adverse events occur in roughly 30% of patients, which include fatigue, weight loss, dysgeusia, and so on (Sekulic et al., 2012; Sharpe et al., 2015).
2.2.2 Squamous Cell Carcinoma
The invasive form of SCC is the second most frequent kind of NMSC, accounting for 20% of all cutaneous malignancies (Rogers et al., 2010). Before metastasizing to distant locations, invasive SCC commonly spread to lymph nodes located in the vicinity. When distant metastasis occurs, patients usually suffer from a poor prognosis. As a result, it is critical to maintain SCC’s generally high odds of cure by carefully evaluating and managing all instances early on, and not to underestimate the tumor’s potential for aggressiveness.
When the tumor arises de novo or the early keratosis phase is lacking, SCC can present as an asymptomatic small plaque or nodule that enlarges over time. Tumor extension or infiltration may extend beyond the visible borders of the lesion, which may create difficulties for diagnosis.
The progression of SCC follows a multistage malignant transformation paradigm. Further mutational and cellular processes will result in invasive growth and, less often, metastasis. The most prevalent genetic abnormalities detected in SCC are mutations in the tumor suppressor gene p53 (Yan et al., 2021). A significant proportion of p53 mutations is localized opposite pyrimidine dimer sites (C–C) and likely derives from UV exposure (Boukamp, 2005). Aberrant activation of epidermal growth factor receptor (EGFR) and Fyn leads to downregulation of p53 mRNA and protein levels via a c-Jun dependent process, indicating another method for modulating p53 activity. (Zhao et al., 2009, 53).
Surgery is the gold standard treatment for SCC, although other treatments include laser dissection, intra-lesion medication injection, and electrodissection. In patients who were deemed inappropriate for surgery due to comorbidities, original tumor location, the danger of local infiltration, or quality of curative margins, other options were examined. External beam RT and brachytherapy are two of them.
The poor results of conventional chemotherapy in patients with advanced SCC, as well as the findings of original studies revealing a high number of genetic abnormalities and neo-antigen load, led to the development of clinical trials with immune checkpoint inhibitors (primarily PD-1) in patients who were not candidates for other treatments. Programmed cell death ligand 1 (PD-L1) levels did not correlate with clinical response to anti-PD1 mAbs in a manner comparable to melanoma). As a result, cemiplimab and pembrolizumab, immune checkpoint inhibitors, have been licensed in the United States for the treatment of locally progressed or metastatic SCC (Migden et al., 2018; Hernandez-Guerrero et al., 2019; Migden et al., 2020). Systemic chemotherapy is currently not licensed for SSC because of the low response rates and the high expense of major side effects, particularly in a vulnerable patient group.
2.2.3 Merkel Cell Carcinoma
Despite its rarity, MCC are becoming more common, owing to advances in detection as well as the worldwide population’s aging. The incidence of MCC rose from 0.5/100,000 people in 2000 to 0.7/100,000 people in 2013, of which the data are obtained from the Surveillance, Epidemiology, and End Results (SEER) database (Paulson et al., 2018).
MCC is difficult to diagnose clinically, requiring nearly exclusively on histological investigation. Immunohistochemistry seems to be effective in the differential diagnosis of MCC. Functional imaging is now regarded as the gold-standard approach for the clinical evaluation of MCC at diagnosis and follow-up because of the superior sensitivity of 18F-fluoro-2-deoxyglucose-positron emission tomography/computed tomography (18FDG-PET/CT) imaging compared to CT or magnetic resonance imaging (MRI) (Concannon et al., 2010).
The stage of the illness and the kind of lymph nodes affected to determine how MCC patients are treated. Sentinel lymph node biopsy (SLNB) is usually recommended in people who have no signs of lymph node involvement; in those who have lymphoma, a blood test may be necessary. In the first-line context, chemotherapeutic therapies such as platinum-based combos, etoposide, topotecan, taxanes, and anthracyclines were frequently utilized (Tai et al., 2000; Becker et al., 2017; Garcia-Carbonero et al., 2019). Chemotherapy’s immunosuppressive impact is now thought to be a plausible mechanism for the early development of resistance after cytotoxic treatment in the setting of a highly immunogenic malignancy (Pommier et al., 2004).
Understanding the pathophysiology of BCC, SCC, and MCC has enabled the development of innovative therapeutics, which have had a significant influence on patient survival and quality of life. The immune system plays an important role in SCC pathogenesis, and preclinical models have revealed important details regarding immune cell changes that govern skin cancer biology. Research on NMSC microenvironment abnormalities implies that malignant cells and those in charge of the innate or adaptive immune systems are in a constant state of interaction. The discovery of these events has gradually altered the landscape of metastatic SCC therapy, opening up new possibilities that are also being investigated in MCC and BCC. In this context, new approaches are being investigated in order to overcome current constraints, with the goal of tailoring therapy in response to the cancer cells’ ongoing phenotypic and antigenic changes.
3 FUNCTIONALIZED NANOMATERIALS FOR SKIN CANCER THERANOSTICS
Anticancer treatments are thought to fail owing to the negative effects of most anticancer medications, low drug concentrations at the tumor site, and the development of drug resistance. Because nanocarriers have the ability to selectively target afflicted organs and cells while sparing normal tissues, the application of functionalization methods, such as the production of nanocarriers for medication delivery or imaging agents, has earned a lot of interest (Marianecci et al., 2014). A number of functionalization techniques have been investigated to modify and functionalize the surface of nanoparticles for cancer theranostics applications (Ulbrich et al., 2016; Tomitaka et al., 2019). Surface modification of nanocarriers may possibly enhance the biological features of nanocarriers, building on the benefits of traditional nano-drug delivery methods (Frickenstein et al., 2021). Traditional nanoparticles are normally quickly opsonized and removed from the bloodstream by reticuloendothelial system (RES) macrophages, which are mostly found in the liver and spleen after intravenous administration (Neves et al., 2016). In this context, the functionalization of surfaces could enable nanocarriers to escape immune surveillance and break through the biological barriers. Chemical functionalization and biofunctionalization are the two main approaches of surface modification. Chemical functionalization has been achieved using a variety of chemical methods, including amide coupling (Giust et al., 2015), click reactions (Ulrich et al., 2021), thiol coupling (Fu et al., 2021), PEGylation (Park et al., 2021), etc. At present, bio-functionalization is confirmed to enhance the blood circulating duration, distribution of the drug, cellular uptake, and regulate immune response and intracellular trafficking (Tang et al., 2021).
3.1 Functionalized Liposomes
Liposomes are spherical vesicles with phospholipid bilayer membranes that are considered non-toxic and biodegradable carriers for the encapsulation and targeted administration of a variety of hydrophobic and hydrophilic medicinal substances (Samad et al., 2007; Chatzikleanthous et al., 2021). Their substantial utility as a replacement in delivering the therapeutic moiety to the targeted location has been utilized to broaden the therapeutic profile of anti-cancer medications while reducing the occurrence of adverse events (Jash et al., 2021). Liposomes are employed in a variety of applications, including biological imaging, fluorescent probes, and more (Erten et al., 2010).
In passive targeting, nanocarriers use the enhanced permeability and retention effect to accumulate within the tumor cells (Bazak et al., 2014). The US Food and Drug Administration (FDA) has authorized many passively targeted liposomal medicines for cancer therapy, which include daunorubicin, doxorubicin, paclitaxel and vincristine (Amreddy et al., 2018). Many studies have looked at the extent and technique of passively targeting liposomal-based medicinal substances for melanoma therapy. Despite the fact that liposomes are potential carriers for the delivery of medicinal substances, the development of liposomal drug delivery systems is currently hampered by a number of limitations. The rapid clearance of liposomes by the RES remains a major challenge. Liposomal absorption by the RES may be prevented by conjugating poly (ethylene glycol) (PEG) to the liposomal membrane. PEGylation, on the other hand, may result in the creation of anti-PEG IgM, causing the liposomes to lose their long-circulating properties and speeding up blood clearance. Immune responses or immunogenicity may also be elicited by PEGylated liposomes. For the purpose of clearance prevention, Fu et al. created a PEGylated PTX-loaded liposome (Fu et al., 2015). To overcome drug resistance and resistance-related metastases in melanoma, mitochondrial targeting topotecan-loaded liposomes have been produced (Yu et al., 2012). Liposome has also been utilized to deliver curcumin in tumors resistant to conventional therapy (Tomeh et al., 2019). Karewicz et al. found that chitosan-coated curcumin-loaded liposome exhibited more efficient anti-cancer effects than that of free curcumin (Karewicz et al., 2013). To address the constraints of photodynamic treatment (PDT), the nitrosyl ruthenium complex [Ru(NH.NHq) (tpy)NO]3+ (RuNO) was coencapsulated with ZnPc in ultradeformable liposomes (UDLs), which demonstrated better flexibility and skin penetration than traditional liposomes (de Lima et al., 2017).
Active targeting relies on the interaction of overexpressed receptors in tumor cells with ligands on surface-modified nanocarriers, such as antibodies, peptides, nucleic acids, and small molecules (Bi et al., 2016; Hu et al., 2021) (Figure 1). CD20+ melanoma stem cells are important for melanoma metastasis and initiation. As a result, targeted eradication of CD20+ melanoma stem cells is a viable strategy for melanoma eradication. Zeng et al. used a single-step nanoprecipitation approach to create salinomycin-loaded lipid-polymer nanoparticles with anti-CD20 aptamers (CD20-SA-NPs). Results indicated that CD20-SA-NPs (salinomycin 5 mg kg−1 d−1, iv, for 60 days) showed greater effectiveness in inhibiting melanoma development in mice carrying xenografts compared to SA-NPs and salinomycin (Zeng et al., 2018).
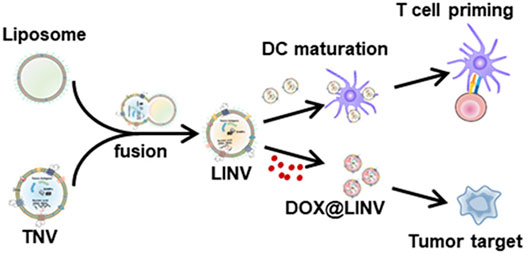
FIGURE 1. Illustration of the doxorubicin (DOX)-loaded biomimetic hybrid nanovesicles (DOX@LINV) via fusing artificial liposomes (LIPs) with tumor-derived nanovesicles (TNVs) for combinational immunochemotherapy. DOX@LINV with a homologous targeting ability could deliver DOX to tumor tissue and elicit an effective immunogenic cell death response to improve the immunogenicity of a tumor. Meanwhile, the preserved tumor antigens and endogenous danger signals in DOX@LINV activated dendritic cells and induced a subsequent antigen-specific T cell immune response (Hu et al., 2021).
3.2 Functionalized Metal Nanoparticles
Functionalized metal nanoparticles for skin cancer theranostics are widely investigated, of which gold and silver are explored in-depth (Lin et al., 2014; Mukherjee et al., 2016; Gaddam et al., 2017).
In skin cancer treatment, gold nanoparticles (Au NPs) were employed for targeted medication administration, tumor progression monitoring, and vaccination (Sau et al., 2014; Mukherjee et al., 2016; Kotcherlakota et al., 2019; Meka et al., 2019). (Brown et al., 2010; Yeh et al., 2012; Gao et al., 2021). Au NPs are now being studied for medicinal purposes due to their potential to increase anticancer activity while reducing undesirable side effects (Kodiha et al., 2015; Katoozi et al., 2021; Tomşa et al., 2021).
Additionally, numerous studies have also explored the application of silver nanoparticles (Ag NPs) for skin-cancer theranostics (Lin et al., 2014; Mukherjee et al., 2014). Lin et al. found that increasing autophagy using Ag NPs helped cells survive, whereas suppressing autophagy with ATG5 siRNA enhanced cancer cell death (Lin et al., 2014). Due to the appearance of hazardous after-effects and the high cost of processing techniques employed in the manufacturing of silver nanoparticles (Ag NPs), the focus has switched to the adaption of green alternatives as a means of overcoming the obstacles posed by them. The extra benefit of imparting biocompatible action while simultaneously lowering the costs associated with the manufacturing of such nanocarriers makes this a viable option for treating uncontrolled skin cancers. Horse chestnut leaves, according to Küp et al., have reduction potential as well as the ability to act as a capping agent in the production of well-defined nanoscale silver particles (Küp et al., 2020).
Because of its effectiveness as a cancer treatment technique, PDT is becoming more popular. Organic photosensitizers used in PDT have a number of drawbacks, including high toxicity, non-selectivity for tumors, and low light absorption. Low light penetration into tumor areas due to low absorption wavelength and long-term skin photosensitivity. As a result, non-toxic inorganic photosensitizers such as noble metal nanoparticles are receiving more attention these days. Nanomaterials are replacing organic dyes since they have photostability and non-toxicity. Among the metal nanoparticles, noble metals, especially gold and silver are attractive because of their size and shape-dependent unique optoelectronic properties. The noble metal is coated with inorganic/organic compounds, making the nanoparticles biocompatible and less poisonous. Furthermore, because of their distinct architectures, Ag- and Au-based inorganic/organic complex nanoparticles may provide a new potential (Dhanalekshmi et al., 2020). Meanwhile, the coating of inorganic/organic complex nanoparticles shields and stabilizes noble metals against chemical corrosion while also increasing reactive oxygen species generation.
Biopsy and radiography are not sensitive enough to identify melanoma in its early stages. In recent years, several attempts have been made to develop effective theranostics modalities that combine diagnostic and therapeutic roles to enhance cancer treatment (Andreiuk et al., 2022). Surface-enhanced Raman spectroscopy (SERS) is gaining popularity in the bioimaging and diagnostic fields. This approach uses surface plasmon resonance (SPR) to enhance sensitivity while inheriting crucial Raman fingerprint information (Kleinman et al., 2013). Au NPs are considered excellent for in vivo imaging applications because they are inert, biocompatible, and their localized surface plasmon resonances (LSPRs) may be adjusted toward the near-infrared regions (NIR) window - an optical window (700–950 nm) with low tissue absorption and autofluorescence (Smith et al., 2009). Recently, Au nanocages (Au NCs) have garnered considerable attention for their potential use in constructing theranostics nanoplatforms with adjustable size and shape and increased Raman active chemical content (Au et al., 2010; Pang et al., 2016; Wen et al., 2019) (Figure 2). Due to the self-aggregation property of unmodified Au NCs, however, the colloidal stability in the tumor microenvironment is decreased, lowering the repeatability of detection (Zeng et al., 2013). In this context, Farahavar et al. developed immuno-liposomal layer-coated p-Au NCs by conjugating anti-MUC18 scFv to liposomal layer coated p-Au NCs in order to build a theranostics system for selective SERS imaging and thermal ablation of MUC18-expressing melanoma malignant cells (A375). The findings suggested that the modified SERS nanoprobe was capable of actively identifying and diagnosing malignant melanoma cells expressing MUC18 (Farahavar et al., 2021).
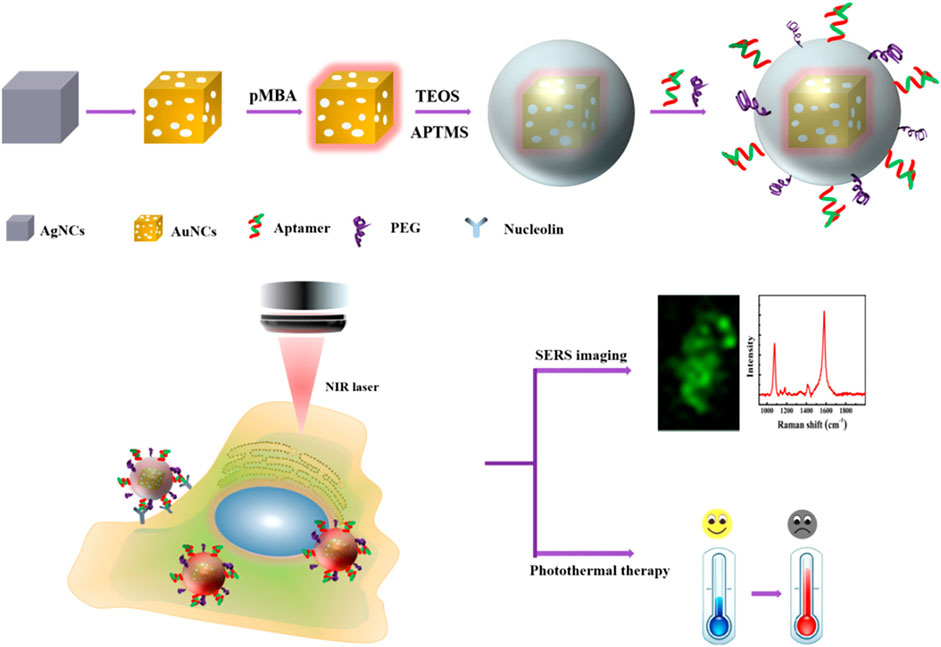
FIGURE 2. Schematic diagram of cellular SERS imaging and photothermal therapy (Wen et al., 2019)
3.3 Functionalized Polymeric Nanoparticles
The goal of developing polymeric nanoparticles was to reduce the loss and early degradation of the medicine contained inside them, which normally occurs after chemical and/or enzymatic deactivation. They’ve shown the capacity to improve medication bioavailability, lessen unpleasant side effects, and raise the proportion of drug stored in a specific part of the body (Narayanaswamy and Torchilin, 2021). Because the majority of melanoma anticancer medicines are lipophilic, their antitumor activity is restricted owing to their adverse pharmacokinetic and pharmacodynamic characteristics (Zheng et al., 2009). The use of amphiphilic polymers (which have both hydrophobic and hydrophilic sections) in anticancer medication formulations has effectively altered the release profile of free medicines (Zhang Y. et al., 2021) (Figure 3). Various forms of polymer nanoparticles, such as nanospheres and nanocapsules, polymer micelles, polymers, dendrimer-based micelles, and polymer drug conjugates, may be manufactured depending on the characteristics of the polymer and their uses (Pucek et al., 2020; Toro et al., 2021). Alves Batista et al. have suggested the usage of NPs made of poly (methyl methacrylate) (PMMA) (Alves Batista et al., 2020), with the ability to incorporate α-terpineol, a monoterpenoid known in the literature for exerting beneficial effects against leukemic cell lines (Nogueira et al., 2014). When α-terpineol is integrated into PMMA/-terpineol NPs and evaluated in melanoma-derived tumor cell lines, the findings imply that it boosts anticancer activity. Furthermore, no toxicity was seen in normal cells (human macrophages and MRC-5 human fibroblasts), suggesting that this formulation might be highly effective in reducing the adverse effects caused by many antineoplastic medications when given in their free forms.
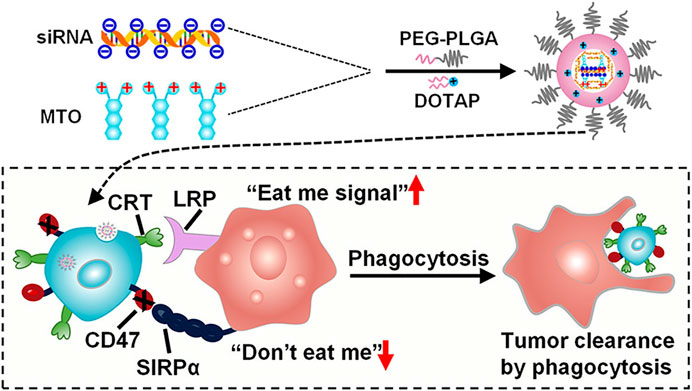
FIGURE 3. The diagram of PLGA-based nanoparticles for the simultaneous delivery of siRNA and mitoxantrone hydrochloride (MTO·2HCl) (Zhang Y. et al., 2021)
Given vitamin D and its analogs’ antiproliferative and differentiation-promoting properties, it appeared prudent to investigate their effectiveness as anticancer agents and their potential for favorable interactions with other anti-melanoma agents or treatment methods (Pettijohn et al., 2014). Scopel et al. created Lipid-polymer hybrid NPs made of a poly (lactic-co-glycolic) acid (PLGA) core and a lipid combination-hydrogenated soy phosphatidylcholine (HSPC), CHOL, and DSPE-PEG2000-as a shell. Vitamin D3 functionalized lipid-polymer hybrid nanoparticles were also created to target the vitamin D receptor (VDR) and promote cell internalization, in which Vitamin D3 was covalently linked to DSPE-PEG2000 (Scopel et al., 2022). HNP-VD was localized in the perinuclear area of B16 melanoma cells, presumably owing to the presence of the vitamin D ligand that targets nuclear receptor VDR. These findings indicate that HNP-VD is an excellent option for the establishment of tailored melanoma treatment regimens, as well as the delivery of encapsulated therapeutic molecules to other cells expressing nuclear vitamin D receptors.
3.4 Functionalized Carbon Nanotubes
Carbon nanotubes (CNTs) are molecular tubes made up of one or more graphene helical sheets (a single layer of carbon atoms). Single-walled carbon nanotubes (SWCNTs) and multi-walled carbon nanotubes (MWCNTs) are the two types (Beiu et al., 2020). Because of their biocompatibility and ability to carry vast cargos of medicines and biomolecules, CNTs have become more essential in recent years. (Degim et al., 2010).
SWCNTs are promising candidates for NIR photothermal agents due to their excellent absorption and photothermal conversion efficiency (Jeng et al., 2006). Modifications of SWCNTs are necessary for the creation of the SWCNT-based PTT materials in order to provide a stable dispersion for biocompatibility and to target the desired tumor while preserving their NIR absorbance (Li et al., 2019; Zhao et al., 2021). However, under physiological conditions, noncovalent or covalent functionalized SWCNTs may be quickly reversed or impair the NIR absorption capabilities (Zhou et al., 2008). To overcome these limitations, Nagai et al. have designed an antibody-conjugated gel-coated SWCNTs exhibiting both stable properties and a high NIR absorption signal (Nagai et al., 2021) (Figure 4). Using the PTT approach, the researchers proved that the antibody-conjugated gel-coated SWCNT was effective in targeting cancer cells and destroying them. This innovative approach for conjugating antibodies to SWCNTs will serve as the foundation for the development of an SWCNT-based platform for the development of NIR photothermal agents in the near-infrared.
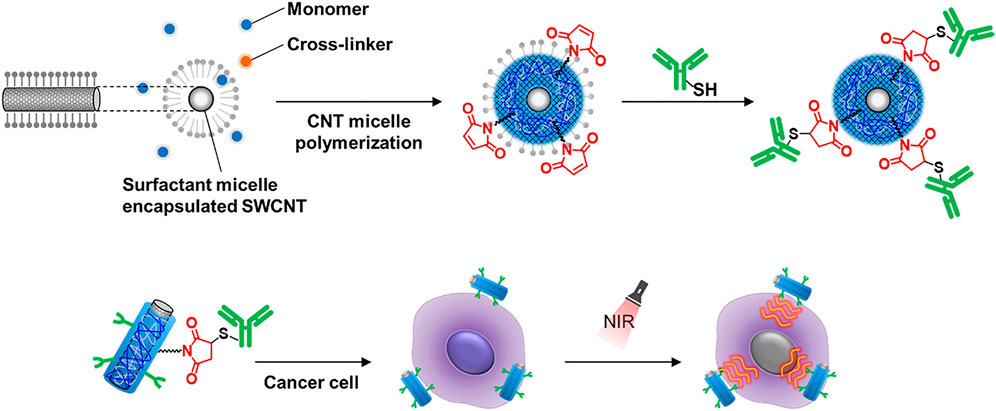
FIGURE 4. Illustration of the process used for the modification of single-walled carbon nanotubes (SWCNTs) with antibodies using the ene-thiol reaction and the use of the modified SWCNTs in the PTT-mediated killing of cancer cells (Nagai et al., 2021).
4 Conclusions and Outlooks
Nanotechnology has expanded the medical business by opening up new opportunities for treating a broad variety of ailments and illnesses. Emerging nanotechnological approaches are crucial in exhibiting robust anti-carcinogenic processes, with advantages such as tumor-specific medicine administration, increased treatment efficacy, fewer adverse event rates, and reduced tumor invasional dispersion. In comparison to standard therapy, careful selection of suitable nanocarriers for loading appropriate chemotherapeutic medicines has shown promising results in terms of dosage reduction. This area has the ability to detect proliferative episodes and improve the survival rate of skin cancer patients while also reducing the burden on medical facilities.
Author Contributions
CZ: Investigation and Writing. XZ: Writing and Revision. SH: Editing. WP: Writing, Review, Editing and Supervision. WL: Conceptualization, Project administration. All authors read and approved the final version of the manuscript.
Funding
This work was supported by the National Natural Science Foundation of China (82002124, and 81720108026).
Conflict of Interest
The authors declare that the research was conducted in the absence of any commercial or financial relationships that could be construed as a potential conflict of interest.
Publisher’s Note
All claims expressed in this article are solely those of the authors and do not necessarily represent those of their affiliated organizations, or those of the publisher, the editors and the reviewers. Any product that may be evaluated in this article, or claim that may be made by its manufacturer, is not guaranteed or endorsed by the publisher.
References
Abi Karam, M., Kourie, H. R., Jalkh, N., Mehawej, C., Kesrouani, C., Haddad, F. G., et al. (2021). Molecular Profiling of Basal Cell Carcinomas in Young Patients. BMC Med. Genomics 14, 187. doi:10.1186/s12920-021-01030-w
Ahronian, L. G., Sennott, E. M., Van Allen, E. M., Wagle, N., Kwak, E. L., Faris, J. E., et al. (2015). Clinical Acquired Resistance to RAF Inhibitor Combinations in BRAF-Mutant Colorectal Cancer through MAPK Pathway Alterations. Cancer Discov. 5, 358–367. doi:10.1158/2159-8290.CD-14-1518
Alves Batista, F., Brena Cunha Fontele, S., Beserra Santos, L. K., Alves Filgueiras, L., Quaresma Nascimento, S., de Castro e Sousa, J. M., et al. (2020). Synthesis, Characterization of α-terpineol-loaded PMMA Nanoparticles as Proposed of Therapy for Melanoma. Mater. Today Commun. 22, 100762. doi:10.1016/j.mtcomm.2019.100762
Amreddy, N., Babu, A., Muralidharan, R., Panneerselvam, J., Srivastava, A., Ahmed, R., et al. (2018). Recent Advances in Nanoparticle-Based Cancer Drug and Gene Delivery. Adv. Cancer Res. 137, 115–170. doi:10.1016/bs.acr.2017.11.003
Andreiuk, B., Nicolson, F., Clark, L. M., Panikkanvalappil, S. R., Kenry, null., Rashidian, M., et al. (2022). Design and Synthesis of Gold Nanostars-Based SERS Nanotags for Bioimaging Applications. Nanotheranostics 6, 10–30. doi:10.7150/ntno.61244
Apalla, Z., Nashan, D., Weller, R. B., and Castellsagué, X. (2017). Skin Cancer: Epidemiology, Disease Burden, Pathophysiology, Diagnosis, and Therapeutic Approaches. Dermatol. Ther. (Heidelb) 7, 5–19. doi:10.1007/s13555-016-0165-y
Armstrong, B. K., and Kricker, A. (2001). The Epidemiology of UV Induced Skin Cancer. J. Photochem. Photobiol. B: Biol. 63, 8–18. doi:10.1016/s1011-1344(01)00198-1
Au, L., Zhang, Q., Cobley, C. M., Gidding, M., Schwartz, A. G., Chen, J., et al. (2010). Quantifying the Cellular Uptake of Antibody-Conjugated Au Nanocages by Two-Photon Microscopy and Inductively Coupled Plasma Mass Spectrometry. ACS Nano 4, 35–42. doi:10.1021/nn901392m
Bazak, R., Houri, M., Achy, S. E., Hussein, W., and Refaat, T. (2014). Passive Targeting of Nanoparticles to Cancer: A Comprehensive Review of the Literature. Mol. Clin. Oncol. 2, 904–908. doi:10.3892/mco.2014.356
Becker, J. C., Lorenz, E., Ugurel, S., Eigentler, T. K., Kiecker, F., Pföhler, C., et al. (2017). Evaluation of Real-World Treatment Outcomes in Patients with Distant Metastatic Merkel Cell Carcinoma Following Second-Line Chemotherapy in Europe. Oncotarget 8, 79731–79741. doi:10.18632/oncotarget.19218
Beiu, C., Giurcaneanu, C., Grumezescu, A. M., Holban, A. M., Popa, L. G., and Mihai, M. M. (2020). Nanosystems for Improved Targeted Therapies in Melanoma. Jcm 9, 318. doi:10.3390/jcm9020318
Bi, Y., Hao, F., Yan, G., Teng, L., J. Lee, R., and Xie, J. (2016). Actively Targeted Nanoparticles for Drug Delivery to Tumor. Cdm 17, 763–782. doi:10.2174/1389200217666160619191853
Boukamp, P. (2005). Non-melanoma Skin Cancer: what Drives Tumor Development and Progression? Carcinogenesis 26, 1657–1667. doi:10.1093/carcin/bgi123
Bowman, R. L., Hennessey, R. C., Weiss, T. J., Tallman, D. A., Crawford, E. R., Murphy, B. M., et al. (2021). UVB Mutagenesis Differs in Nras- and Braf-Mutant Mouse Models of Melanoma. Life Sci. Alliance 4, e202101135. doi:10.26508/lsa.202101135
Brogden, N. K., Mehalick, L., Fischer, C. L., Wertz, P. W., and Brogden, K. A. (2012). The Emerging Role of Peptides and Lipids as Antimicrobial Epidermal Barriers and Modulators of Local Inflammation. Skin Pharmacol. Physiol. 25, 167–181. doi:10.1159/000337927
Brown, S. D., Nativo, P., Smith, J.-A., Stirling, D., Edwards, P. R., Venugopal, B., et al. (2010). Gold Nanoparticles for the Improved Anticancer Drug Delivery of the Active Component of Oxaliplatin. J. Am. Chem. Soc. 132, 4678–4684. doi:10.1021/ja908117a
Cameron, M. C., Lee, E., Hibler, B. P., Barker, C. A., Mori, S., Cordova, M., et al. (2019). Basal Cell Carcinoma. J. Am. Acad. Dermatol. 80, 303–317. doi:10.1016/j.jaad.2018.03.060
Cancer Genome Atlas Network (2015). Genomic Classification of Cutaneous Melanoma. Cell 161, 1681–1696. doi:10.1016/j.cell.2015.05.044
Carlino, M. S., Larkin, J., and Long, G. V. (2021). Immune Checkpoint Inhibitors in Melanoma. The Lancet 398, 1002–1014. doi:10.1016/S0140-6736(21)01206-X
Chapman, P. B., Hauschild, A., Robert, C., Haanen, J. B., Ascierto, P., Larkin, J., et al. (2011). Improved Survival with Vemurafenib in Melanoma with BRAF V600E Mutation. N. Engl. J. Med. 364, 2507–2516. doi:10.1056/NEJMoa1103782
Chatzikleanthous, D., O’Hagan, D. T., and Adamo, R. (2021). Lipid-Based Nanoparticles for Delivery of Vaccine Adjuvants and Antigens: Toward Multicomponent Vaccines. Mol. Pharmaceutics 18, 2867–2888. doi:10.1021/acs.molpharmaceut.1c00447
Chen, B.-H., and Stephen Inbaraj, B. (2019). Nanoemulsion and Nanoliposome Based Strategies for Improving Anthocyanin Stability and Bioavailability. Nutrients 11, 1052. doi:10.3390/nu11051052
Chen, F., and Cai, W. (2014). Tumor Vasculature Targeting: A Generally Applicable Approach for Functionalized Nanomaterials. Small 10, 1887–1893. doi:10.1002/smll.201303627
Chen, M. K., and Sebaratnam, D. F. (2021). A Non‐healing Ulcer: Amelanotic Melanoma. Med. J. Aust. 215, 405. doi:10.5694/mja2.51294
Chuchvara, N., Farabi, B., Milgraum, D., Lee, Y., Chamorro, P., Pappert, A., et al. (2021). Amelanotic Melanoma with Features of Keratinocytic Tumor on Reflectance Confocal Microscopy. J. Cutan. Pathol. 49, 317–320. doi:10.1111/cup.14186
Concannon, R., Larcos, G. S., and Veness, M. (2010). The Impact of 18F-FDG PET-CT Scanning for Staging and Management of Merkel Cell Carcinoma: Results from Westmead Hospital, Sydney, Australia. J. Am. Acad. Dermatol. 62, 76–84. doi:10.1016/j.jaad.2009.06.021
D'Orazio, J., Jarrett, S., Amaro-Ortiz, A., and Scott, T. (2013). UV Radiation and the Skin. Ijms 14, 12222–12248. doi:10.3390/ijms140612222
Damsky, W., Micevic, G., Meeth, K., Muthusamy, V., Curley, D. P., Santhanakrishnan, M., et al. (2015). mTORC1 Activation Blocks BrafV600E-Induced Growth Arrest but Is Insufficient for Melanoma Formation. Cancer Cell 27, 41–56. doi:10.1016/j.ccell.2014.11.014
de Lima, R. G., Tedesco, A. C., da Silva, R. S., and Lawrence, M. J. (2017). Ultradeformable Liposome Loaded with Zinc Phthalocyanine and [Ru(NH.NHq)(tpy)NO] 3+ for Photodynamic Therapy by Topical Application. Photodiagnosis Photodynamic Ther. 19, 184–193. doi:10.1016/j.pdpdt.2017.05.013
Degim, I. T., Burgess, D. J., and Papadimitrakopoulos, F. (2010). Carbon Nanotubes for Transdermal Drug Delivery. J. Microencapsulation 27, 669–681. doi:10.3109/02652048.2010.506581
Dessinioti, C., Plaka, M., and Stratigos, A. J. (2014). Vismodegib for the Treatment of Basal Cell Carcinoma: Results and Implications of the ERIVANCE BCC Trial. Future Oncol. 10, 927–936. doi:10.2217/fon.14.50
Dhanalekshmi, K. I., Sangeetha, K., Magesan, P., Johnson, J., Zhang, X., and Jayamoorthy, K. (2020). Photodynamic Cancer Therapy: Role of Ag- and Au-Based Hybrid Nano-Photosensitizers. J. Biomol. Struct. Dyn. 1, 1–8. doi:10.1080/07391102.2020.1858965
Dinnes, J., Deeks, J. J., Saleh, D., Chuchu, N., Bayliss, S. E., Patel, L., et al. (2018). Reflectance Confocal Microscopy for Diagnosing Cutaneous Melanoma in Adults. Cochrane Database Syst. Rev. 2018, CD013190. doi:10.1002/14651858.CD013190
Eggermont, A. M. M., Blank, C. U., Mandala, M., Long, G. V., Atkinson, V., Dalle, S., et al. (2018). Adjuvant Pembrolizumab versus Placebo in Resected Stage III Melanoma. N. Engl. J. Med. 378, 1789–1801. doi:10.1056/NEJMoa1802357
Eggermont, A. M. M., Blank, C. U., Mandala, M., Long, G. V., Atkinson, V. G., Dalle, S., et al. (2020). Longer Follow-Up Confirms Recurrence-free Survival Benefit of Adjuvant Pembrolizumab in High-Risk Stage III Melanoma: Updated Results from the EORTC 1325-MG/KEYNOTE-054 Trial. Jco 38, 3925–3936. doi:10.1200/JCO.20.02110
Eroglu, Z., Holmen, S. L., Chen, Q., Khushalani, N. I., Amaravadi, R., Thomas, R., et al. (2019). Melanoma central Nervous System Metastases: An Update to Approaches, Challenges, and Opportunities. Pigment Cel Melanoma Res 32, 458–469. doi:10.1111/pcmr.12771
Erten, A., Wrasidlo, W., Scadeng, M., Esener, S., Hoffman, R. M., Bouvet, M., et al. (2010). Magnetic Resonance and Fluorescence Imaging of Doxorubicin-Loaded Nanoparticles Using a Novel In Vivo Model. Nanomedicine: Nanotechnology, Biol. Med. 6, 797–807. doi:10.1016/j.nano.2010.06.005
Esteva, A., Kuprel, B., Novoa, R. A., Ko, J., Swetter, S. M., Blau, H. M., et al. (2017). Dermatologist-level Classification of Skin Cancer with Deep Neural Networks. Nature 542, 115–118. doi:10.1038/nature21056
Falchook, G. S., Lewis, K. D., Infante, J. R., Gordon, M. S., Vogelzang, N. J., DeMarini, D. J., et al. (2012). Activity of the Oral MEK Inhibitor Trametinib in Patients with Advanced Melanoma: a Phase 1 Dose-Escalation Trial. Lancet Oncol. 13, 782–789. doi:10.1016/S1470-2045(12)70269-3
Farahavar, G., Abolmaali, S. S., Nejatollahi, F., Safaie, A., Javanmardi, S., Khajeh Zadeh, H., et al. (2021). Single-chain Antibody-Decorated Au Nanocages@liposomal Layer Nanoprobes for Targeted SERS Imaging and Remote-Controlled Photothermal Therapy of Melanoma Cancer Cells. Mater. Sci. Eng. C 124, 112086. doi:10.1016/j.msec.2021.112086
Foth, M., and McMahon, M. (2021). Autophagy Inhibition in BRAF-Driven Cancers. Cancers 13, 3498. doi:10.3390/cancers13143498
Frickenstein, A. N., Hagood, J. M., Britten, C. N., Abbott, B. S., McNally, M. W., Vopat, C. A., et al. (2021). Mesoporous Silica Nanoparticles: Properties and Strategies for Enhancing Clinical Effect. Pharmaceutics 13, 570. doi:10.3390/pharmaceutics13040570
Fu, H., Shi, K., Hu, G., Yang, Y., Kuang, Q., Lu, L., et al. (2015). Tumor-targeted Paclitaxel Delivery and Enhanced Penetration Using TAT-Decorated Liposomes Comprising Redox-Responsive Poly(ethylene Glycol). J. Pharm. Sci. 104, 1160–1173. doi:10.1002/jps.24291
Fu, K., Zhang, G., Liu, Y., Tan, J., Zhang, H., and Zhang, Q. (2022). All‐Organic Superhydrophobic Coating Comprising Raspberry‐Like Particles and Fluorinated Polyurethane Prepared via Thiol‐Click Reaction. Macromol. Rapid Commun. 43, 2100599. doi:10.1002/marc.202100599
Gaddam, R. R., Mukherjee, S., Punugupati, N., Vasudevan, D., Patra, C. R., Narayan, R., et al. (2017). Facile Synthesis of Carbon Dot and Residual Carbon Nanobeads: Implications for Ion Sensing, Medicinal and Biological Applications. Mater. Sci. Eng. C 73, 643–652. doi:10.1016/j.msec.2016.12.095
Gao, Y., Zhang, S., Wu, C., Li, Q., Shen, Z., Lu, Y., et al. (2021). Self-Protected DNAzyme Walker with a Circular Bulging DNA Shield for Amplified Imaging of miRNAs in Living Cells and Mice. ACS Nano 15, 19211–19224. doi:10.1021/acsnano.1c04260
Garcia-Carbonero, R., Marquez-Rodas, I., de la Cruz-Merino, L., Martinez-Trufero, J., Cabrera, M. A., Piulats, J. M., et al. (2019). Recent Therapeutic Advances and Change in Treatment Paradigm of Patients with Merkel Cell Carcinoma. Oncologist 24, 1375–1383. doi:10.1634/theoncologist.2018-0718
Giust, S., La Sorella, G., Sperni, L., Strukul, G., and Scarso, A. (2015). Substrate Selective Amide Coupling Driven by Encapsulation of a Coupling Agent within a Self-Assembled Hexameric Capsule. Chem. Commun. 51, 1658–1661. doi:10.1039/c4cc08833f
Gracia, G., Cao, E., Kochappan, R., Porter, C. J. H., Johnston, A. P. R., and Trevaskis, N. L. (2022). Association of a Vaccine Adjuvant with Endogenous HDL Increases Lymph Uptake and Dendritic Cell Activation. Eur. J. Pharmaceutics Biopharmaceutics 172 (21), 240–252. doi:10.1016/j.ejpb.2021.09.004
Guo, W., Wang, H., and Li, C. (2021). Signal Pathways of Melanoma and Targeted Therapy. Sig Transduct Target. Ther. 6, 424. doi:10.1038/s41392-021-00827-6
Hernandez-Guerrero, T., Doger, B., and Moreno, V. (2019). Cemiplimab for the Treatment of Advanced Cutaneous Squamous Cell Carcinoma. Spain: Drugs of today, 55. doi:10.1358/dot.2019.55.8.3005176
Hodi, F. S., O'Day, S. J., McDermott, D. F., Weber, R. W., Sosman, J. A., Haanen, J. B., et al. (2010). Improved Survival with Ipilimumab in Patients with Metastatic Melanoma. N. Engl. J. Med. 363, 711–723. doi:10.1056/NEJMoa1003466
Hoek, K. S., Schlegel, N. C., Brafford, P., Sucker, A., Ugurel, S., Kumar, R., et al. (2006). Metastatic Potential of Melanomas Defined by Specific Gene Expression Profiles with No BRAF Signature. Pigment Cel Res. 19, 290–302. doi:10.1111/j.1600-0749.2006.00322.x
Horn, S., Figl, A., Rachakonda, P. S., Fischer, C., Sucker, A., Gast, A., et al. (2013). TERT Promoter Mutations in Familial and Sporadic Melanoma. Science 339, 959–961. doi:10.1126/science.1230062
Hu, M., Zhang, J., Kong, L., Yu, Y., Hu, Q., Yang, T., et al. (2021). Immunogenic Hybrid Nanovesicles of Liposomes and Tumor-Derived Nanovesicles for Cancer Immunochemotherapy. ACS Nano 15, 3123–3138. doi:10.1021/acsnano.0c09681
Jash, A., Ubeyitogullari, A., and Rizvi, S. S. H. (2021). Liposomes for Oral Delivery of Protein and Peptide-Based Therapeutics: Challenges, Formulation Strategies, and Advances. J. Mater. Chem. B 9, 4773–4792. doi:10.1039/d1tb00126d
Jeng, E. S., Moll, A. E., Roy, A. C., Gastala, J. B., and Strano, M. S. (2006). Detection of DNA Hybridization Using the Near-Infrared Band-gap Fluorescence of Single-Walled Carbon Nanotubes. Nano Lett. 6, 371–375. doi:10.1021/nl051829k
Johannessen, C. M., Johnson, L. A., Piccioni, F., Townes, A., Frederick, D. T., Donahue, M. K., et al. (2013). A Melanocyte Lineage Program Confers Resistance to MAP Kinase Pathway Inhibition. Nature 504, 138–142. doi:10.1038/nature12688
Johnson, D. B., Childress, M. A., Chalmers, Z. R., Frampton, G. M., Ali, S. M., Rubinstein, S. M., et al. (2018). BRAF Internal Deletions and Resistance to BRAF/MEK Inhibitor Therapy. Pigment Cel Melanoma Res. 31, 432–436. doi:10.1111/pcmr.12674
Johnson, J. D., and Young, B. (1996). Demographics of Brain Metastasis. Neurosurg. Clin. North America 7, 337–344. doi:10.1016/s1042-3680(18)30365-6
Jokioja, J., Yang, B., and Linderborg, K. M. (2021). Acylated Anthocyanins: A Review on Their Bioavailability and Effects on Postprandial Carbohydrate Metabolism and Inflammation. Compr. Rev. Food Sci. Food Saf. 20, 5570–5615. doi:10.1111/1541-4337.12836
Karewicz, A., Bielska, D., Loboda, A., Gzyl-Malcher, B., Bednar, J., Jozkowicz, A., et al. (2013). Curcumin-containing Liposomes Stabilized by Thin Layers of Chitosan Derivatives. Colloids Surf. B: Biointerfaces 109, 307–316. doi:10.1016/j.colsurfb.2013.03.059
Katoozi, D., Clayton, A. H. A., Moss, D. J., and Chon, J. W. M. (2021). Uptake Quantification of Gold Nanoparticles inside of Cancer Cells Using High Order Image Correlation Spectroscopy. Biomed. Opt. Express 12, 539–552. doi:10.1364/BOE.417321
Kaur, H., and Kesharwani, P. (2021). Advanced Nanomedicine Approaches Applied for Treatment of Skin Carcinoma. J. Controlled Release 337, 589–611. doi:10.1016/j.jconrel.2021.08.003
Kim, M. J., Ha, S. J., So, B. R., Kim, C.-K., Kim, K.-M., and Jung, S. K. (2021). NADPH Oxidase and Epidermal Growth Factor Receptor Are Promising Targets of Phytochemicals for Ultraviolet-Induced Skin Carcinogenesis. Antioxidants 10, 1909. doi:10.3390/antiox10121909
Kleinman, S. L., Frontiera, R. R., Henry, A.-I., Dieringer, J. A., and Van Duyne, R. P. (2013). Creating, Characterizing, and Controlling Chemistry with SERS Hot Spots. Phys. Chem. Chem. Phys. 15, 21–36. doi:10.1039/c2cp42598j
Kodiha, M., Wang, Y. M., Hutter, E., Maysinger, D., and Stochaj, U. (2015). Off to the Organelles - Killing Cancer Cells with Targeted Gold Nanoparticles. Theranostics 5, 357–370. doi:10.7150/thno.10657
Kotcherlakota, R., Nimushakavi, S., Roy, A., Yadavalli, H. C., Mukherjee, S., Haque, S., et al. (2019). Biosynthesized Gold Nanoparticles: In Vivo Study of Near-Infrared Fluorescence (NIR)-Based Bio-Imaging and Cell Labeling Applications. ACS Biomater. Sci. Eng. 5, 5439–5452. doi:10.1021/acsbiomaterials.9b00721
Krauthammer, M., Kong, Y., Ha, B. H., Evans, P., Bacchiocchi, A., McCusker, J. P., et al. (2012). Exome Sequencing Identifies Recurrent Somatic RAC1 Mutations in Melanoma. Nat. Genet. 44, 1006–1014. doi:10.1038/ng.2359
Küp, F. Ö., Çoşkunçay, S., and Duman, F. (2020). Biosynthesis of Silver Nanoparticles Using Leaf Extract of Aesculus Hippocastanum (Horse Chestnut): Evaluation of Their Antibacterial, Antioxidant and Drug Release System Activities. Mater. Sci. Eng. C 107, 110207. doi:10.1016/j.msec.2019.110207
Kwon, J.-H., Lee, N.-G., Kang, A.-R., Song, J.-Y., Hwang, S.-G., Um, H.-D., et al. (2021). Radiosensitizer Effect of β-Apopicropodophyllin against Colorectal Cancer via Induction of Reactive Oxygen Species and Apoptosis. Ijms 22, 13514. doi:10.3390/ijms222413514
Kwong, L. N., Costello, J. C., Liu, H., Jiang, S., Helms, T. L., Langsdorf, A. E., et al. (2012). Oncogenic NRAS Signaling Differentially Regulates Survival and Proliferation in Melanoma. Nat. Med. 18, 1503–1510. doi:10.1038/nm.2941
Larkin, J., Ascierto, P. A., Dréno, B., Atkinson, V., Liszkay, G., Maio, M., et al. (2014). Combined Vemurafenib and Cobimetinib in BRAF-Mutated Melanoma. N. Engl. J. Med. 371, 1867–1876. doi:10.1056/NEJMoa1408868
Li, D., Humayun, L., Vienneau, E., Vu, T., and Yao, J. (2021). Seeing through the Skin: Photoacoustic Tomography of Skin Vasculature and beyond. JID Innov. 1, 100039. doi:10.1016/j.xjidi.2021.100039
Li, Y., Li, X., Doughty, A., West, C., Wang, L., Zhou, F., et al. (2019). Phototherapy Using Immunologically Modified Carbon Nanotubes to Potentiate Checkpoint Blockade for Metastatic Breast Cancer. Nanomedicine: Nanotechnology, Biol. Med. 18, 44–53. doi:10.1016/j.nano.2019.02.009
Lin, J., Huang, Z., Wu, H., Zhou, W., Jin, P., Wei, P., et al. (2014). Inhibition of Autophagy Enhances the Anticancer Activity of Silver Nanoparticles. Autophagy 10, 2006–2020. doi:10.4161/auto.36293
Liu, D., Schilling, B., Liu, D., Sucker, A., Livingstone, E., Jerby-Arnon, L., et al. (2019). Integrative Molecular and Clinical Modeling of Clinical Outcomes to PD1 Blockade in Patients with Metastatic Melanoma. Nat. Med. 25, 1916–1927. doi:10.1038/s41591-019-0654-5
Liu, Y., Jiang, Y., Zhang, M., Tang, Z., He, M., and Bu, W. (2018). Modulating Hypoxia via Nanomaterials Chemistry for Efficient Treatment of Solid Tumors. Acc. Chem. Res. 51, 2502–2511. doi:10.1021/acs.accounts.8b00214
Lopes, F. C. P. S., Sleiman, M. G., Sebastian, K., Bogucka, R., Jacobs, E. A., and Adamson, A. S. (2021). UV Exposure and the Risk of Cutaneous Melanoma in Skin of Color. JAMA Dermatol. 157, 213–219. doi:10.1001/jamadermatol.2020.4616
Lu, H., Liu, S., Zhang, G., Zhu, Y., Frederick, D. T., Hu, Y., et al. (2017). PAK Signalling Drives Acquired Drug Resistance to MAPK Inhibitors in BRAF-Mutant Melanomas. Nature 550, 133–136. doi:10.1038/nature24040
Majem, M., Manzano, J. L., Marquez-Rodas, I., Mujika, K., Muñoz-Couselo, E., Pérez-Ruiz, E., et al. (2021). SEOM Clinical Guideline for the Management of Cutaneous Melanoma. Clin. translational Oncol. 23, 948. doi:10.1007/s12094-020-02539-9
Marianecci, C., Di Marzio, L., Rinaldi, F., Celia, C., Paolino, D., Alhaique, F., et al. (2014). Niosomes from 80s to Present: the State of the Art. Adv. Colloid Interf. Sci. 205, 187–206. doi:10.1016/j.cis.2013.11.018
Meka, R. R., Mukherjee, S., Patra, C. R., and Chaudhuri, A. (2019). Shikimoyl-ligand Decorated Gold Nanoparticles for Use in Ex Vivo Engineered Dendritic Cell Based DNA Vaccination. Nanoscale 11, 7931–7943. doi:10.1039/c8nr10293g
Migden, M. R., Khushalani, N. I., Chang, A. L. S., Lewis, K. D., Schmults, C. D., Hernandez-Aya, L., et al. (2020). Cemiplimab in Locally Advanced Cutaneous Squamous Cell Carcinoma: Results from an Open-Label, Phase 2, Single-Arm Trial. Lancet Oncol. 21, 294–305. doi:10.1016/S1470-2045(19)30728-4
Migden, M. R., Rischin, D., Schmults, C. D., Guminski, A., Hauschild, A., Lewis, K. D., et al. (2018). PD-1 Blockade with Cemiplimab in Advanced Cutaneous Squamous-Cell Carcinoma. N. Engl. J. Med. 379, 341–351. doi:10.1056/NEJMoa1805131
Millán-Esteban, D., Peña-Chilet, M., García-Casado, Z., Manrique-Silva, E., Requena, C., Bañuls, J., et al. (2021). Mutational Characterization of Cutaneous Melanoma Supports Divergent Pathways Model for Melanoma Development. Cancers 13, 5219. doi:10.3390/cancers13205219
Mittal, P., and Roberts, C. W. M. (2020). The SWI/SNF Complex in Cancer - Biology, Biomarkers and Therapy. Nat. Rev. Clin. Oncol. 17, 435–448. doi:10.1038/s41571-020-0357-3
Molodtsov, A. K., Khatwani, N., Vella, J. L., Lewis, K. A., Zhao, Y., Han, J., et al. (2021). Resident Memory CD8+ T Cells in Regional Lymph Nodes Mediate Immunity to Metastatic Melanoma. Immunity 54, 2117–2132. doi:10.1016/j.immuni.2021.08.019
Mukherjee, S., Chowdhury, D., Kotcherlakota, R., Patra, S., B, V., Bhadra, M. P., et al. (2014). Potential Theranostics Application of Bio-Synthesized Silver Nanoparticles (4-in-1 System). Theranostics 4, 316–335. doi:10.7150/thno.7819
Mukherjee, S., Sau, S., Madhuri, D., Bollu, V. S., Madhusudana, K., Sreedhar, B., et al. (2016). Green Synthesis and Characterization of Monodispersed Gold Nanoparticles: Toxicity Study, Delivery of Doxorubicin and its Bio-Distribution in Mouse Model. J. Biomed. Nanotechnol 12, 165–181. doi:10.1166/jbn.2016.2141
Nagai, Y., Nakamura, K., Ohno, J., Kawaguchi, M., and Fujigaya, T. (2021). Antibody-Conjugated Gel-Coated Single-Walled Carbon Nanotubes as Photothermal Agents. ACS Appl. Bio Mater. 4, 5049–5056. doi:10.1021/acsabm.1c00299
Narayanaswamy, R., and Torchilin, V. P. (2021). Targeted Delivery of Combination Therapeutics Using Monoclonal Antibody 2C5-Modified Immunoliposomes for Cancer Therapy. Pharm. Res. 38, 429–450. doi:10.1007/s11095-021-02986-1
Nathanson, K. L., Martin, A.-M., Wubbenhorst, B., Greshock, J., Letrero, R., D'Andrea, K., et al. (2013). Tumor Genetic Analyses of Patients with Metastatic Melanoma Treated with the BRAF Inhibitor Dabrafenib (GSK2118436). Clin. Cancer Res. 19, 4868–4878. doi:10.1158/1078-0432.CCR-13-0827
Neves, A. R., Queiroz, J. F., and Reis, S. (2016). Brain-targeted Delivery of Resveratrol Using Solid Lipid Nanoparticles Functionalized with Apolipoprotein E. J. Nanobiotechnol 14, 27. doi:10.1186/s12951-016-0177-x
Newlands, C., Currie, R., Memon, A., Whitaker, S., and Woolford, T. (2016). Non-melanoma Skin Cancer: United Kingdom National Multidisciplinary Guidelines. J. Laryngol. Otol. 130, S125–S132. doi:10.1017/S0022215116000554
Nogueira, M. N. M., Aquino, S. G., Rossa Junior, C., and Spolidorio, D. M. P. (2014). Terpinen-4-ol and Alpha-Terpineol (tea Tree Oil Components) Inhibit the Production of IL-1β, IL-6 and IL-10 on Human Macrophages. Inflamm. Res. 63, 769–778. doi:10.1007/s00011-014-0749-x
Pang, B., Yang, X., and Xia, Y. (2016). Putting Gold Nanocages to Work for Optical Imaging, Controlled Release and Cancer Theranostics. Nanomedicine 11, 1715–1728. doi:10.2217/nnm-2016-0109
Park, H., Otte, A., and Park, K. (2022). Evolution of Drug Delivery Systems: From 1950 to 2020 and beyond. J. Controlled Release 342, 53–65. doi:10.1016/j.jconrel.2021.12.030
Paulson, K. G., Park, S. Y., Vandeven, N. A., Lachance, K., Thomas, H., Chapuis, A. G., et al. (2018). Merkel Cell Carcinoma: Current US Incidence and Projected Increases Based on Changing Demographics. J. Am. Acad. Dermatol. 78, 457–463. doi:10.1016/j.jaad.2017.10.028
Pettijohn, E., Martone, B., Rademaker, A., and Kuzel, T. (2014). A Phase I Study of High-Dose Calcitriol in Combination with Temozolomide for Patients with Metastatic Melanoma. Jpm 4, 448–458. doi:10.3390/jpm4040448
Pommier, Y., Sordet, O., Antony, S., Hayward, R. L., and Kohn, K. W. (2004). Apoptosis Defects and Chemotherapy Resistance: Molecular Interaction Maps and Networks. Oncogene 23, 2934–2949. doi:10.1038/sj.onc.1207515
Poulikakos, P. I., Persaud, Y., Janakiraman, M., Kong, X., Ng, C., Moriceau, G., et al. (2011). RAF Inhibitor Resistance Is Mediated by Dimerization of Aberrantly Spliced BRAF(V600E). Nature 480, 387–390. doi:10.1038/nature10662
Pucek, A., Tokarek, B., Waglewska, E., and Bazylińska, U. (2020). Recent Advances in the Structural Design of Photosensitive Agent Formulations Using “Soft” Colloidal Nanocarriers. Pharmaceutics 12, 587. doi:10.3390/pharmaceutics12060587
Rashwan, A. K., Karim, N., Xu, Y., Xie, J., Cui, H., Mozafari, M. R., et al. (2021). Potential Micro-/nano-encapsulation Systems for Improving Stability and Bioavailability of Anthocyanins: An Updated Review. Crit. Rev. Food Sci. Nutr., 1–24. doi:10.1080/10408398.2021.1987858
Rasmussen, S. M., Nielsen, T., Hody, S., Hager, H., and Schousboe, L. P. (2021). Photoplethysmography for Demarcation of Cutaneous Squamous Cell Carcinoma. Sci. Rep. 11, 21467. doi:10.1038/s41598-021-00645-4
Rogers, H. W., Weinstock, M. A., Harris, A. R., Hinckley, M. R., Feldman, S. R., Fleischer, A. B., et al. (2010). Incidence Estimate of Nonmelanoma Skin Cancer in the United States, 2006. Arch. Dermatol. 146, 283–287. doi:10.1001/archdermatol.2010.19
Romano, G., Chen, P.-L., Song, P., McQuade, J. L., Liang, R. J., Liu, M., et al. (2018). A Preexisting Rare PIK3CAE545K Subpopulation Confers Clinical Resistance to MEK Plus CDK4/6 Inhibition in NRAS Melanoma and Is Dependent on S6K1 Signaling. Cancer Discov. 8, 556–567. doi:10.1158/2159-8290.CD-17-0745
Sade-Feldman, M., Jiao, Y. J., Chen, J. H., Rooney, M. S., Barzily-Rokni, M., Eliane, J.-P., et al. (2017). Resistance to Checkpoint Blockade Therapy through Inactivation of Antigen Presentation. Nat. Commun. 8, 1136. doi:10.1038/s41467-017-01062-w
Samad, A., Sultana, Y., and Aqil, M. (2007). Liposomal Drug Delivery Systems: an Update Review. Cdd 4, 297–305. doi:10.2174/156720107782151269
Samson, J. M., Ravindran Menon, D., Smith, D. E., Baird, E., Kitano, T., Gao, D., et al. (2019). Clinical Implications of ALDH1A1 and ALDH1A3 mRNA Expression in Melanoma Subtypes. Chemico-Biological Interactions 314, 108822. doi:10.1016/j.cbi.2019.108822
Sau, S., Agarwalla, P., Mukherjee, S., Bag, I., Sreedhar, B., Pal-Bhadra, M., et al. (2014). Cancer Cell-Selective Promoter Recognition Accompanies Antitumor Effect by Glucocorticoid Receptor-Targeted Gold Nanoparticle. Nanoscale 6, 6745–6754. doi:10.1039/c4nr00974f
Scopel, R., Falcão, M. A., Cappellari, A. R., Morrone, F. B., Guterres, S. S., Cassel, E., et al. (2022). Lipid-polymer Hybrid Nanoparticles as a Targeted Drug Delivery System for Melanoma Treatment. Int. J. Polymeric Mater. Polymeric Biomater. 71, 127–138. doi:10.1080/00914037.2020.1809406
Sekulic, A., Migden, M. R., Oro, A. E., Dirix, L., Lewis, K. D., Hainsworth, J. D., et al. (2012). Efficacy and Safety of Vismodegib in Advanced Basal-Cell Carcinoma. N. Engl. J. Med. 366, 2171–2179. doi:10.1056/NEJMoa1113713
Sharpe, H. J., Pau, G., Dijkgraaf, G. J., Basset-Seguin, N., Modrusan, Z., Januario, T., et al. (2015). Genomic Analysis of Smoothened Inhibitor Resistance in Basal Cell Carcinoma. Cancer Cell 27, 327–341. doi:10.1016/j.ccell.2015.02.001
Shen, Y., Zhang, N., Tian, J., Xin, G., Liu, L., Sun, X., et al. (2022). Advanced Approaches for Improving Bioavailability and Controlled Release of Anthocyanins. J. Controlled Release 341, 285–299. doi:10.1016/j.jconrel.2021.11.031
Sheng, X., Chen, H., Hu, B., Yao, X., Liu, Z., Yao, X., et al. (2021). Safety, Efficacy, and Biomarker Analysis of Toripalimab in Patients with Previously Treated Advanced Urothelial Carcinoma: Results from a Multicenter Phase II Trial POLARIS-03. Clin. Cancer Res. 28, 489–497. doi:10.1158/1078-0432.CCR-21-2210
Shi, H., Hugo, W., Kong, X., Hong, A., Koya, R. C., Moriceau, G., et al. (2014). Acquired Resistance and Clonal Evolution in Melanoma during BRAF Inhibitor Therapy. Cancer Discov. 4, 80–93. doi:10.1158/2159-8290.CD-13-0642
Shi, H., Moriceau, G., Kong, X., Lee, M.-K., Lee, H., Koya, R. C., et al. (2012). Melanoma Whole-Exome Sequencing Identifies V600EB-RAF Amplification-Mediated Acquired B-RAF Inhibitor Resistance. Nat. Commun. 3, 724. doi:10.1038/ncomms1727
Smith, A. M., Mancini, M. C., and Nie, S. (2009). Second Window for In Vivo Imaging. Nat. Nanotech 4, 710–711. doi:10.1038/nnano.2009.326
Smith, M. P., Brunton, H., Rowling, E. J., Ferguson, J., Arozarena, I., Miskolczi, Z., et al. (2016). Inhibiting Drivers of Non-mutational Drug Tolerance Is a Salvage Strategy for Targeted Melanoma Therapy. Cancer Cell 29, 270–284. doi:10.1016/j.ccell.2016.02.003
Song, H., He, R., Wang, K., Ruan, J., Bao, C., Li, N., et al. (2010). Anti-HIF-1α Antibody-Conjugated Pluronic Triblock Copolymers Encapsulated with Paclitaxel for Tumor Targeting Therapy. Biomaterials 31, 2302–2312. doi:10.1016/j.biomaterials.2009.11.067
Song, X., Feng, L., Liang, C., Yang, K., and Liu, Z. (2016). Ultrasound Triggered Tumor Oxygenation with Oxygen-Shuttle Nanoperfluorocarbon to Overcome Hypoxia-Associated Resistance in Cancer Therapies. Nano Lett. 16, 6145–6153. doi:10.1021/acs.nanolett.6b02365
Sosman, J. A., Kim, K. B., Schuchter, L., Gonzalez, R., Pavlick, A. C., Weber, J. S., et al. (2012). Survival in BRAF V600-Mutant Advanced Melanoma Treated with Vemurafenib. N. Engl. J. Med. 366, 707–714. doi:10.1056/NEJMoa1112302
Sullivan, R. J., and Flaherty, K. T. (2015). New Strategies in Melanoma: Entering the Era of Combinatorial Therapy. Clin. Cancer Res. 21, 2424–2435. doi:10.1158/1078-0432.CCR-14-1650
Sullivan, R. J., Infante, J. R., Janku, F., Wong, D. J. L., Sosman, J. A., Keedy, V., et al. (2018). First-in-Class ERK1/2 Inhibitor Ulixertinib (BVD-523) in Patients with MAPK Mutant Advanced Solid Tumors: Results of a Phase I Dose-Escalation and Expansion Study. Cancer Discov. 8, 184–195. doi:10.1158/2159-8290.CD-17-1119
Swetter, S. M., Tsao, H., Bichakjian, C. K., Curiel-Lewandrowski, C., Elder, D. E., Gershenwald, J. E., et al. (2019). Guidelines of Care for the Management of Primary Cutaneous Melanoma. J. Am. Acad. Dermatol. 80, 208. doi:10.1016/j.jaad.2018.08.055
Taeb, S., Ashrafizadeh, M., Zarrabi, A., Rezapoor, S., Musa, A. E., Farhood, B., et al. (2021). Role of Tumor Microenvironment in Cancer Stem Cells Resistance to Radiotherapy. Curr. Cancer Drug Targets 22, 18. doi:10.2174/1568009622666211224154952
Tai, P. T., Yu, E., Winquist, E., Hammond, A., Stitt, L., Tonita, J., et al. (2000). Chemotherapy in Neuroendocrine/Merkel Cell Carcinoma of the Skin: Case Series and Review of 204 Cases. J. Clin. Oncol. 18, 2493–2499. doi:10.1200/JCO.2000.18.12.2493
Tang, Z., Xiao, Y., Kong, N., Liu, C., Chen, W., Huang, X., et al. (2021). Nano-bio Interfaces Effect of Two-Dimensional Nanomaterials and Their Applications in Cancer Immunotherapy. Acta Pharm. Sin B 11, 3447–3464. doi:10.1016/j.apsb.2021.05.004
Tarik Alhamdany, A., Saeed, A. M. H., and Alaayedi, M. (2021). Nanoemulsion and Solid Nanoemulsion for Improving Oral Delivery of a Breast Cancer Drug: Formulation, Evaluation, and a Comparison Study. Saudi Pharm. J. 29, 1278–1288. doi:10.1016/j.jsps.2021.09.016
Teixido, C., Castillo, P., Martinez-Vila, C., Arance, A., and Alos, L. (2021). Molecular Markers and Targets in Melanoma. Cells 10, 2320. doi:10.3390/cells10092320
Thai, A. A., Lim, A. M., Solomon, B. J., and Rischin, D. (2021). Biology and Treatment Advances in Cutaneous Squamous Cell Carcinoma. Cancers (Basel) 13, 5645. doi:10.3390/cancers13225645
Tomeh, M. A., Hadianamrei, R., and Zhao, X. (2019). A Review of Curcumin and its Derivatives as Anticancer Agents. Int. J. Mol. Sci. 20, E1033. doi:10.3390/ijms20051033
Tomitaka, A., Kaushik, A., Kevadiya, B. D., Mukadam, I., Gendelman, H. E., Khalili, K., et al. (2019). Surface-engineered Multimodal Magnetic Nanoparticles to Manage CNS Diseases. Drug Discov. Today 24, 873–882. doi:10.1016/j.drudis.2019.01.006
Tomşa, A. M., Răchişan, A. L., Aldea, A. A., and Ciumărnean, L. (2021). Perspectives of Gold Nanoparticles and Their Applications in Pancreatic Cancer (Review). Exp. Ther. Med. 21, 258. doi:10.3892/etm.2021.9689
Toro, M. D., Gozzo, L., Tracia, L., Cicciù, M., Drago, F., Bucolo, C., et al. (2021). New Therapeutic Perspectives in the Treatment of Uveal Melanoma: A Systematic Review. Biomedicines 9, 1311. doi:10.3390/biomedicines9101311
Ulbrich, K., Holá, K., Šubr, V., Bakandritsos, A., Tuček, J., and Zbořil, R. (2016). Targeted Drug Delivery with Polymers and Magnetic Nanoparticles: Covalent and Noncovalent Approaches, Release Control, and Clinical Studies. Chem. Rev. 116, 5338–5431. doi:10.1021/acs.chemrev.5b00589
Ulrich, S., Wang, X., Rottmar, M., Rossi, R. M., Nelson, B. J., Bruns, N., et al. (2021). Nano-3D-Printed Photochromic Micro-objects. Small 17, e2101337. doi:10.1002/smll.202101337
Van Raamsdonk, C. D., Bezrookove, V., Green, G., Bauer, J., Gaugler, L., O’Brien, J. M., et al. (2009). Frequent Somatic Mutations of GNAQ in Uveal Melanoma and Blue Naevi. Nature 457, 599–602. doi:10.1038/nature07586
Villanueva, J., Vultur, A., Lee, J. T., Somasundaram, R., Fukunaga-Kalabis, M., Cipolla, A. K., et al. (2010). Acquired Resistance to BRAF Inhibitors Mediated by a RAF Kinase Switch in Melanoma Can Be Overcome by Cotargeting MEK and IGF-1R/PI3K. Cancer Cell 18, 683–695. doi:10.1016/j.ccr.2010.11.023
Wen, S., Miao, X., Fan, G.-C., Xu, T., Jiang, L.-P., Wu, P., et al. (2019). Aptamer-Conjugated Au Nanocage/SiO2 Core-Shell Bifunctional Nanoprobes with High Stability and Biocompatibility for Cellular SERS Imaging and Near-Infrared Photothermal Therapy. ACS Sens 4, 301–308. doi:10.1021/acssensors.8b00682
Williams, N. M., Rojas, K. D., Reynolds, J. M., Kwon, D., Shum-Tien, J., and Jaimes, N. (2021). Assessment of Diagnostic Accuracy of Dermoscopic Structures and Patterns Used in Melanoma Detection: A Systematic Review and Meta-Analysis. JAMA Dermatol. 157, 1078–1088. doi:10.1001/jamadermatol.2021.2845
Wong, S. Y., and Reiter, J. F. (2008). The Primary Cilium at the Crossroads of Mammalian Hedgehog Signaling. Curr. Top. Dev. Biol. 85, 225–260. doi:10.1016/S0070-2153(08)00809-0
Yan, G., Li, L., Zhu, S., Wu, Y., Liu, Y., Zhu, L., et al. (2021). Single-cell Transcriptomic Analysis Reveals the Critical Molecular Pattern of UV-Induced Cutaneous Squamous Cell Carcinoma. Cell Death Dis 13, 23. doi:10.1038/s41419-021-04477-y
Yeh, Y.-C., Creran, B., and Rotello, V. M. (2012). Gold Nanoparticles: Preparation, Properties, and Applications in Bionanotechnology. Nanoscale 4, 1871–1880. doi:10.1039/c1nr11188d
Yu, Y., Wang, Z.-H., Zhang, L., Yao, H.-J., Zhang, Y., Li, R.-J., et al. (2012). Mitochondrial Targeting Topotecan-Loaded Liposomes for Treating Drug-Resistant Breast Cancer and Inhibiting Invasive Metastases of Melanoma. Biomaterials 33, 1808–1820. doi:10.1016/j.biomaterials.2011.10.085
Zaar, O., Gillstedt, M., Lindelöf, B., Wennberg-Larkö, A.-M., and Paoli, J. (2016). Merkel Cell Carcinoma Incidence Is Increasing in Sweden. J. Eur. Acad. Dermatol. Venereol. 30, 1708–1713. doi:10.1111/jdv.13698
Zeng, J., Goldfeld, D., and Xia, Y. (2013). A Plasmon-Assisted Optofluidic (PAOF) System for Measuring the Photothermal Conversion Efficiencies of Gold Nanostructures and Controlling an Electrical Switch. Angew. Chem. Int. Ed. Engl. 52, 4169–4173. doi:10.1002/anie.201210359
Zeng, Y.-B., Yu, Z.-C., He, Y.-N., Zhang, T., Du, L.-B., Dong, Y.-M., et al. (2018). Salinomycin-loaded Lipid-Polymer Nanoparticles with Anti-CD20 Aptamers Selectively Suppress Human CD20+ Melanoma Stem Cells. Acta Pharmacol. Sin 39, 261–274. doi:10.1038/aps.2017.166
Zhang, P., Meng, J., Li, Y., Yang, C., Hou, Y., Tang, W., et al. (2021a). Nanotechnology-enhanced Immunotherapy for Metastatic Cancer. Innovation (N Y) 2, 100174. doi:10.1016/j.xinn.2021.100174
Zhang, Y., Zhang, Z., Li, S., Zhao, L., Li, D., Cao, Z., et al. (2021b). A siRNA-Assisted Assembly Strategy to Simultaneously Suppress “Self” and Upregulate “Eat-Me” Signals for Nanoenabled Chemo-Immunotherapy. ACS Nano 15, 16030–16042. doi:10.1021/acsnano.1c04458
Zhao, L., Li, W., Marshall, C., Griffin, T., Hanson, M., Hick, R., et al. (2009). Srcasm Inhibits Fyn-Induced Cutaneous Carcinogenesis with Modulation of Notch1 and P53. Cancer Res. 69, 9439–9447. doi:10.1158/0008-5472.CAN-09-2976
Zhao, Y., Zhao, T., Cao, Y., Sun, J., Zhou, Q., Chen, H., et al. (2021). Temperature-Sensitive Lipid-Coated Carbon Nanotubes for Synergistic Photothermal Therapy and Gene Therapy. ACS Nano 15, 6517–6529. doi:10.1021/acsnano.0c08790
Zheng, D., Li, X., Xu, H., Lu, X., Hu, Y., and Fan, W. (2009). Study on Docetaxel-Loaded Nanoparticles with High Antitumor Efficacy against Malignant Melanoma. Acta Biochim. Biophys. Sin (Shanghai) 41, 578–587. doi:10.1093/abbs/gmp045
Keywords: functionalization, nanomaterials, skin cancer, theranostics, advances
Citation: Zhang C, Zhu X, Hou S, Pan W and Liao W (2022) Functionalization of Nanomaterials for Skin Cancer Theranostics. Front. Bioeng. Biotechnol. 10:887548. doi: 10.3389/fbioe.2022.887548
Received: 01 March 2022; Accepted: 06 April 2022;
Published: 26 April 2022.
Edited by:
Mei Chen, Hunan University, ChinaCopyright © 2022 Zhang, Zhu, Hou, Pan and Liao. This is an open-access article distributed under the terms of the Creative Commons Attribution License (CC BY). The use, distribution or reproduction in other forums is permitted, provided the original author(s) and the copyright owner(s) are credited and that the original publication in this journal is cited, in accordance with accepted academic practice. No use, distribution or reproduction is permitted which does not comply with these terms.
*Correspondence: Wanqing Liao, liaowanqing@sohu.com; Weihua Pan, panweihua9@sina.com
†These authors have contributed equally to this work