- 1Department of Mechanical Engineering, Faculty of Engineering, Islamic University of Madina, Madinah, Saudi Arabia
- 2Department of Mechanical Engineering, Faculty of Engineering Science and Technology, Hamdard University, Karachi, Pakistan
- 3Department of Engineering Sciences, PN Engineering College, National University of Sciences and Technology, Karachi, Pakistan
- 4Department of Chemical Engineering, Faculty of Engineering, Islamic University of Madinah, Madinah, Saudi Arabia
Nanotechnology is considered one of the most significant advancements in science and technology over the last few decades. However, the contemporary use of nanomaterials in bioenergy production is very deficient. This study evaluates the application of nanomaterials for biogas production from different kinds of waste. A state-of-the-art comprehensive review is carried out to elaborate on the deployment of different categories of nano-additives (metal oxides, zero-valent metals, various compounds, carbon-based nanomaterials, nano-composites, and nano-ash) in several kinds of biodegradable waste, including cattle manure, wastewater sludge, municipal solid waste, lake sediments, and sanitary landfills. This study discusses the pros and cons of nano-additives on biogas production from the anaerobic digestion process. Several all-inclusive tables are presented to appraise the literature on different nanomaterials used for biogas production from biomass. Future perspectives to increase biogas production via nano-additives are presented, and the conclusion is drawn on the productivity of biogas based on various nanomaterials. A qualitative review of relevant literature published in the last 50 years is conducted using the bibliometric technique for the first time in literature. About 14,000 research articles are included in this analysis, indexed on the Web of Science. The analysis revealed that the last decade (2010–20) was the golden era for biogas literature, as 84.4% of total publications were published in this timeline. Moreover, it was observed that nanomaterials had revolutionized the field of anaerobic digestion, methane production, and waste activated sludge; and are currently the central pivot of the research community. The toxicity of nanomaterials adversely affects anaerobic bacteria; therefore, using bioactive nanomaterials is emerging as the best alternative. Conducting optimization studies by varying substrate and nanomaterials’ size, concentration and shape is still a field. Furthermore, collecting and disposing nanomaterials at the end of the anaerobic process is a critical environmental challenge to technology implementation that needs to be addressed before the nanomaterials assisted anaerobic process could pave its path to the large-scale industrial sector.
Introduction
Exponential growth in the world population has raised the energy demand drastically (Hagos et al., 2017). Meeting the energy requirement has now become an area of prime importance for all nations. At present, the world is highly dependent on conventional energy sources, i.e., fossil fuels (Palaniappan, 2017). The available reserves for fossil fuels are diminishing rapidly; one study indicated that existing reserves would last till 2050 (Satyanarayana et al., 2011). Besides, these conventional fuels contribute much to environmental pollution and ecological destruction. Along with fluctuating fuel prices, these factors have led the fuel industry to move towards sustainable renewable resources to fulfill the energy demand (Malik and Sangwan, 2012). Currently, fossil fuels fulfill almost 90% of world energy demands, and it is expected to minimize it to 50% by 2040 via incorporating more sustainable renewable energy sources such as solar, wind, geothermal, tidal, and biomass (biofuels) (Hussein, 2015).
Biofuels can be produced by utilizing locally available organic feedstock. Various methods are available for organic matter to energy conversion, but AD (Anaerobic Digestion) is among the most preferable, specifically for biogas production (Hao et al., 2019; Feng et al., 2021). In this process, the absence of O2 provides a favorable environment for bacteria to decompose organic matter by breaking it into methane and other by-products (Seadi et al., 2008). AD finds its implications for waste treatment on a broad category of waste, including sludge, wastewater, and municipal waste (Vasco-Correa et al., 2018). It is also mentioned among widely considered methods for converting complex waste to biogas (Holm-Nielsen et al., 2009; Feng et al., 2014). Additionally, applications of AD in the treatment of animal manure (Bidart et al., 2014), energy crops (Lönnqvist et al., 2013), organic food waste (Zhang et al., 2016), microalgae (Park et al., 2009), and agricultural residues (Mushtaq et al., 2016) make it stand among other methods.
In the mentioned process of organic waste conversion to biogas, four main phases are usually included; (i) hydrolysis, (ii) acidogenesis, (iii) acetogenesis, (iv) methanogenesis (Christy et al., 2014), see Figure 1. These four phases highly dependent upon the extent of interactions between microorganisms during each phase. In the first phase, hydrolytic bacteria are in action. They transform complex organic matters such as proteins, fats, and carbohydrates into organic monomers. Most organic matters contain complex macromolecules that cannot be directly used by acidogenic microorganisms. Therefore, hydrolysis is needed to break complex structures into small molecules (monomers), which ultimately can be used in the second phase of anaerobic digestion. In the second stage, acidogenesis, thus formed monomers are transformed into Volatile Fatty Acids (VFAs) with the help of fermentative bacteria. In the third phase, acetic acid is formed accompanied by evolving hydrogen gas by the action of acetogenic bacteria. Among four phases of anaerobic digestion, acidogenesis is considered the fastest one. The last stage is methanogenesis, where products of the last phase are transformed into methane and carbon dioxide (Mao et al., 2015; Zaidi et al., 2021a). Thus, formed methane significantly varies in quality based on a few factors such as biomass composition, additives, selection of conversion process, and precursors. Typically, the composition of biogas is specified by methane and carbon dioxide contributing 50–75% and 25–45%, respectively. A minute amount of other gasses can be there, usually of calorific values of 21–24 MJ/m3 (Ganzoury and Allam, 2015).
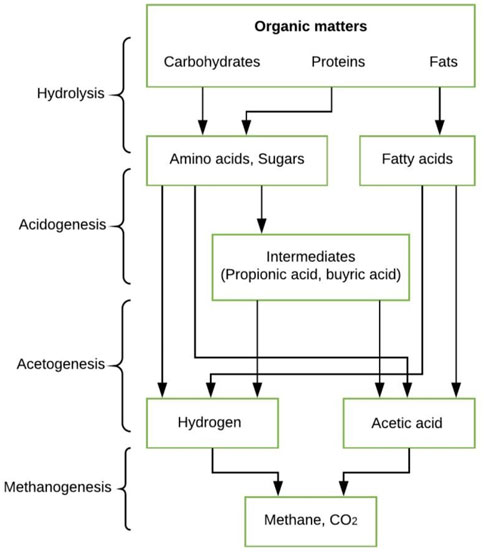
FIGURE 1. Common major sequential processes during anaerobic digestion (Feng et al., 2018).
Biogas, as a renewable energy source, is an emerging sector globally with consecutive increments in the production capacity over the years. Figure 2 represents the regional breakdown, not only reflecting the overall increment but also every region is showing growth over the years, which is a promising motivation for scientists and investors for the biogas augmentation utilizing all the available technologies to pursue state-of-the-art solutions for biogas production. Nanotechnology, which can be defined as interpolation of matter at very small dimensions (less than or equal to 100 nm), is in its emerging phase. At this small scale, material properties change (such as melting point and chemical changes) that has made this technology pivot to researchers (Antonio et al., 2017). Nanotechnology can be used in many fields such as materials engineering, life sciences, electronics, biotechnology, information technology, and cognitive sciences (Khan et al., 2009; Demetzos, 2016). The bioenergy field can be revolutionized by improving catalytic conversions and enhancing catalytic efficiency. Literature is evident from the recent implications of nanoparticles (NPs), nanomaterials (NMs), nanosheets, and others in bioenergy production (Rahman et al., 2016). Wu et al. (2021) recently conducted a literature review to highlight the importance of different operating parameters on biogas production and to understand the importance of different auxiliary technologies in optimizing these operational parameters. The study finds that the addition of NPs is a promising option, especially for mainstream biogas production plants, to enhance biogas production. However, some challenges (such as high investment cost, strict control of NPs concentration, energy demand, and disposal risks) need to be minimized before introducing NPs in the industrial sector (Zaidi et al., 2021b). In another review study (Jadhav et al., 2021), the authors studied the impact of metallic NPs on microbial direct interspecies electron transfer for biogas production enhancement. The use of metallic NPs was found to be cost-effective, efficient, and sustainable for biogas production. Hassanein studied the role of electro-conductive NPs. NPs were found to be promising for AD process stability and efficiency enhancement (Zaidi et al., 2019a; Kumar et al., 2021). Specifically, metallic NPs were highlighted as the most famous NPs for their potential to decrease lag time and improve the biogas production and process stability. Moreover, studying the role of size, type, and concentration of metallic NPs is still a challenge (Hassanein et al., 2021). After conducting a literature review, Ellacuriage stipulated that to increase volumetric efficiency and reduce initial capital cost, NPs augmentation is the most suitable approach (Ellacuriaga et al., 2021).
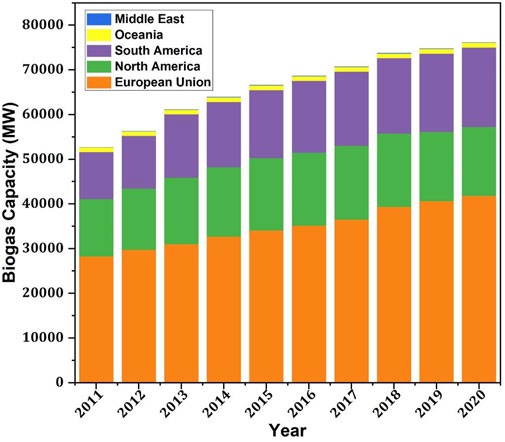
FIGURE 2. Regional breakdown of global biogas capacity (RENA, 2021).
The economic feasibility of large-scale AD has always been a prime concern for the research community. The application of NPs has contributed to the economic feasibility of AD by enhancing catalytic efficiency (Faisal et al., 2019). However, the disposal of these NPs after biogas production is still a significant environmental challenge. Therefore, there is a dire need to find environmentally friendly disposing methods for NPs being used in AD. Moreover, the main challenge in understanding nanomaterial’s augmentation with biogas is their kinetics. The root cause of lower biogas production in the absence of NPs is a cellular wall that restrains the interaction of catalysts with the substrate. Studying the impact of different NPs, through the lens of their positive and negative aspects could improve our understanding of biogas production.
This paper presents a comprehensive state-of-the-art review highlighting the direct influence of nano-additives and nano-nutrients on either biogas production enhancement or adverse effects during anaerobic digestion. Future perspectives to enhance biogas production via nano-additives are also presented. The focus has been placed on classifying available literature according to the type of nanomaterial employed during AD. The detailed discussion shows how nanomaterials can be effectively used for biogas augmentation to improve biomass utilization as a renewable and sustainable energy source. Furthermore, this study reports a bibliometric analysis of biogas literature published in the last 50 years. To the best of the authors’ knowledge, it is the first study based on a detailed quantitative literature review.
Nanomaterials role in chemical reactions
Nanomaterials (NMs) are materials having one or more dimensions smaller than 100 nm. This resulted in a much high surface area of the material just because of the size. A spherical NP of 1 nm diameter will have approximately 100% of its atoms on the surface. Whereas an NP having a diameter of 10 nm would have only 15% of its atoms on the surface. It would be expected from a particle having a higher surface area to be more reactive than the same mass of material consisting of larger particles, as chemical reactions typically take place at surfaces (Rao et al., 2001).
NMs can be classified into three categories contingent on a number of dimensions at the nanoscale as per the British Standards Institution (BSI, 2007). Table 1 depicts some NMs from each group. In the literature, nanoparticles are specified as 3D particles having at least one dimension of less than 100 nm. They could have various morphologies and shapes. As discussed earlier, the surface properties and high reactivity of the NPs are due to the increased surface area to volume ratio. This distinctive feature of NPs makes them popular in products and techniques where chemical reactions are important. In this text, nanomaterials and nanoparticles are used as interchangeable terms, both referring to the nano-scale materials in the context of the discussion.
There are numerous benefits of NMs for biogas production. NMs provide more exposed sites available for anaerobic bacteria (Rahman et al., 2016). It also helps in the solubilization of organic matter to release intercellular polymeric substances. The control over surface features aids in catalyzing animal fats, plant cell membranes, and cellular remains. They also help a chemical modification of organic matter (Nyberg et al., 2008). The application of NMs for biogas production can be one of the possible ways to sustain this renewable energy source for large-scale production. Several NMs are used as an additive to enhance biogas production.
Research trends in biogas studies: Past and contemporary
In order to find out a pattern, sequence, and significant research trends, quantitative analysis is performed using the web of science database, as shown in Figures 3,4. To conduct the analysis, 14,000 journal articles (research papers only) were explored from the web of science database, and content analysis was performed to determine the main keywords used by researchers. These keywords define the mainstream of research within a field. The colors depict different eras of research. The diameter of bubbles denotes the impact of that keyword, i.e., the occurrence of a keyword. These bubbles are interconnected using links. Link strength is evident in the relation between two keywords, i.e., co-occurrence in the same research article.
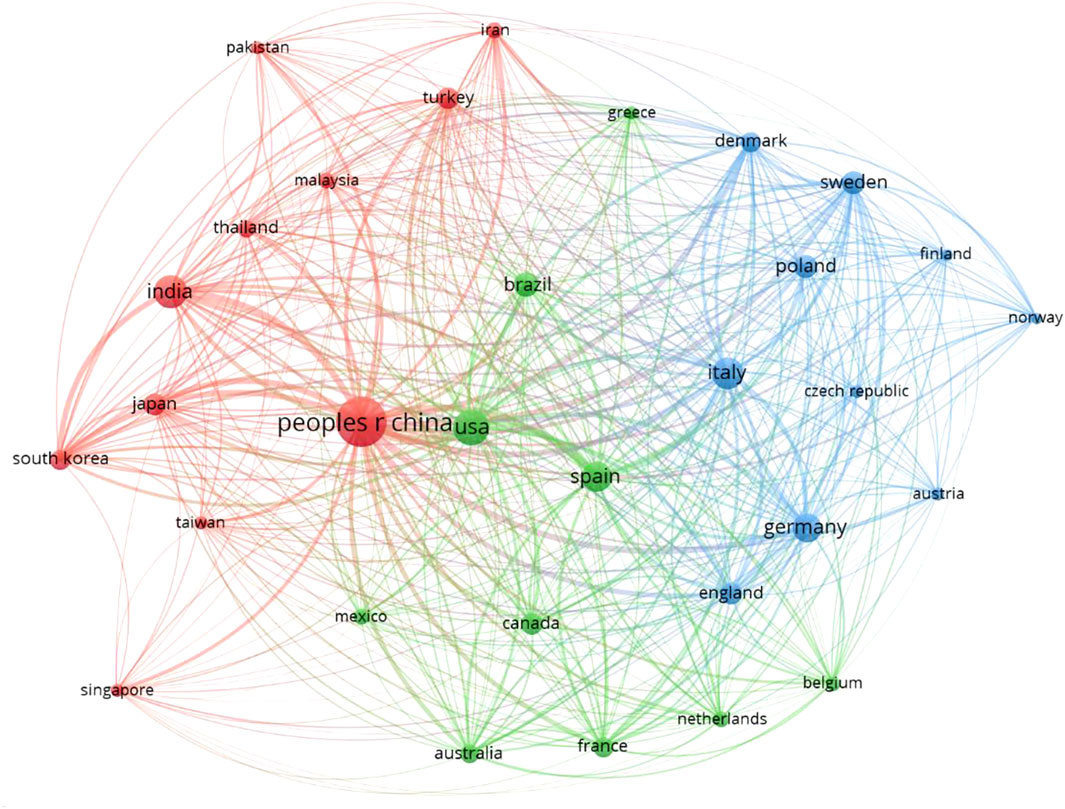
FIGURE 4. Currently active countries on nanotechnology-based biogas production on the basis of the number of citations.
The survey was divided into two eras for analysis purposes, the first 1970–2016 and the second 2017–2020. The purpose of this division was first to understand research evaluation within the field and second to determine the current topics of research to define future directions. Figure 3 revealed that anaerobic digestion and biogas production are among the most used keywords throughout the era 1970–2020. In addition, these keywords find their most implications in the last 5 years as denoted by red color. Therefore, it is concluded that anaerobic digestion and biogas production are among hot topics of research.
In order to further understand the main streams of research within anaerobic digestion and biogas production, data from the last 4 years were evaluated. It is pertinent to mention that 2010–2020 is observed as the main era of research rise in this field. A total of 84.4% of the publications have been published in the last 10 years. Out of this, 84.4%, 54.7% of publications belong to the last 4 years, 2017–2020. Therefore, 2017–2020 can be mentioned as a research-intensive period of biogas production. This high research interest is due to the emergence of new technologies and their implications for biogas production.
The analysis of research keywords used in the last 3 years depicts that the emergence of NP is the main technology that evolved in this era and got incredible attention from the research community. The yellow color of the keyword NP is evident to a sharp contrast and shift towards effective implementation of NP in producing biogas during 2019–2020. The strong link of NPs with anaerobic digestion, methane production, and waste activated sludge represents NPs’ reputation for mentioned technologies with in short duration. Owing to this reputation, NPs implications for biogas production can be regarded as the central pivot to the research community.
The most important aspect to note is the emergence of nanoparticles in the last decade and their strong connection with biogas production. Therefore, based on research trends, it can be concluded with confidence that nanoparticles and biogas production starting from sludge have gotten significant attention in recent years. In this regard, this review is conducted to update how nanomaterials have contributed to biogas production.
Application of nanomaterials for biogas production
This section presents a comprehensive review of the recently reported studies on biogas production based on the class of materials used for a different kind of feedstock. Nanomaterials are a vital candidate to enhance biogas production from different inorganic waste. Basically, at the nanoscale, the surface area of the material is high, making the reaction relatively fast (Zaidi et al., 2019a). In addition, these NPs interact with the cell membrane of sludge, leading to structural changes in the cells that finally make it bacteria permeable membranes. In this way, more bacteria find their way to attack sludge and hence increase overall biogas production (Faisal et al., 2019). Nevertheless, attention has been focused on the use, effects, and outcomes of various NMs for biogas production.
Trace metal nanomaterials for biogas enhancement
Trace metals are essential for methanogenic bacteria growth in an AD reactor (Qiang et al., 2013). Metals nutrients such as iron, cobalt, nickel, etc., are found to influence the AD process significantly (Kelly and Switzenbaum, 1984; Zaidi et al., 2018). Zero-valent iron has been widely employed to treat various kinds of waste. The literature showed that it releases electrons for methanogenesis during the AD process, resulting in biogas augmentation. Nanoscaled Zero-Valent Iron (NZVI) has a high surface-to-volume ratio; this characteristic increased the chemical reaction sites and positively influenced the AD. Su et al. (2015) investigated the influence of 0.05, 0.10, and 0.20 wt% NZVI (60–120 nm) on the AD of Waste Activated Sludge (WAS) for 20 days at the mesophilic temperature (32 ± 1°C). The results indicated that 0.05 wt% and 0.10 wt% NZVI increased the methane production by 9.8% and 4.6%, respectively. However, 0.20 wt% NZVI decreased methane production by 8.8%. The authors suggested that NZVI stimulates methanogenic populations and sulfate reducers. It also accelerates sludge stabilization in AD resulting in increased biogas and methane production. The metallic iron core caused a slow release of soluble Fe2+ acting as a donor and caused the formation of reactive oxygen species. The hydrogen sulfide reacted with NZVI oxide shell on the surface and resulted in the formation of FeS and FeS2, which was regarded as the main reason for decreasing H2S and an increase in methane. These findings agree with Carpenter et al. (2015), who reported that cytotoxicity of NZVI to the microorganism in the AD with varied particle size and reactivity could improve the degradation increase biogas production while decreasing CO2. The observed decrease in biogas production at a higher concentration of NZVI by Su et al. (2015) was confirmed by the study conducted by Suanon et al. (2016). According to the authors, improvement in biogas and methane production is dose-dependent, and a higher dose of NZVI could result in an inhibitory effect. Another study conducted by Suanon et al. (2017) investigated the effect of 0.1 wt% NZVI on methane yield from wastewater sludge at mesophilic conditions (37 ± 1°C) for 50 days. Results showed an increase of 25.2% in methane production.
The production efficiency of biogas and methane yield from Cattle Manure (CM) slurry were discussed under the influence of various concentrations of NZVI, ranging from 5 to 20 mg/L. Batch-wise, anaerobic fermentation of CM was conducted at 37 ± 0.3°C, 90 rpm of rotating speed, and 50 days of Hydraulic Retention Time (HRT). This study concludes that the addition of NZVI is favorable for biogas production. The addition of minute amount, amounting to only 5 mg/L, incremented biogas and methane production by 1.44 and 1.38 times, respectively. The best concentration was found to be 20 mg/L which increases biogas and methane volume by 1.45 times and methane production by 1.59 times. The authors mentioned that the addition of these NPs improves the startup of biogas production and hence reduces the lag phase in comparison with control. The optimal NZVI concentration found in this study was further experimented with by the same authors (Abdelsalam et al., 2016).
The influence of NZVI on the AD of WAS was studied by Wang et al. (2016) at concentrations of 1, 10, 100, and 500 mg/g Total Suspended Solids (TSS), respectively. Batch anaerobic digesters were used for the AD with working volume, operating temperature, and mixing rate of 1 L, 35 ± 1°C, and 120 rpm, respectively, for HRT of 30 days. The study indicated that 10 mg/g TSS increased methane production to 120% of the control, whereas other concentrations had no considerable effect, see Figure 5. This is also in agreement with results obtained by Su et al. (Su et al., 2015) and Suanon et al. (Suanon et al., 2016).
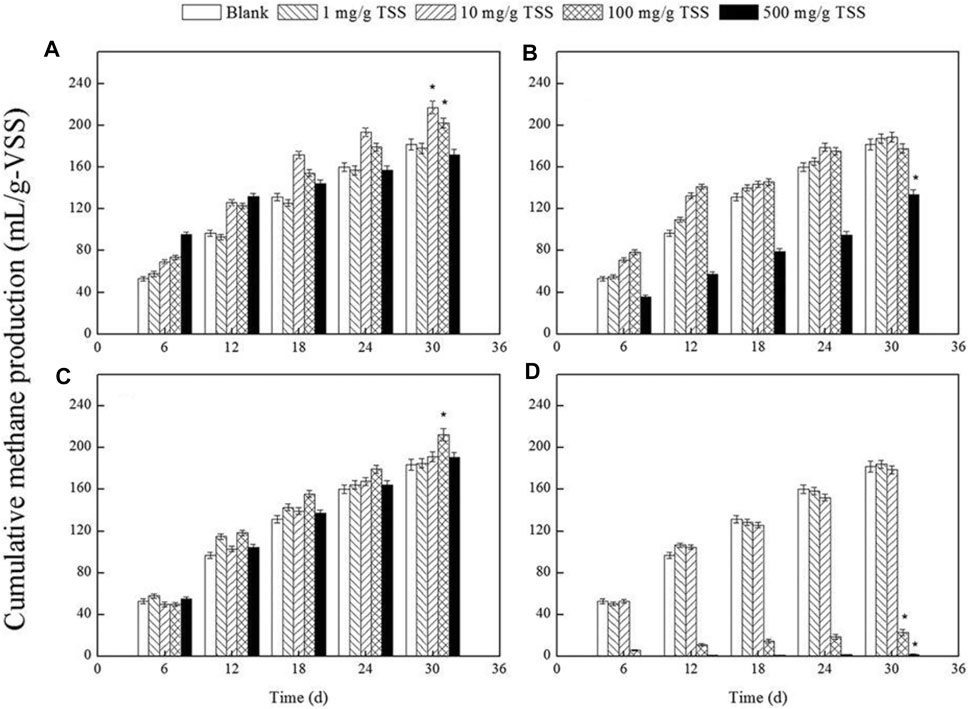
FIGURE 5. Influence of various concentrations of nZVI (A), Ag NPs (B), Fe2 O 3 NPs (C) and MgO NPs (D) on cumulative methane production during AD of WAS (Wang et al., 2016).
In contrast, Amen et al. (2017b) investigated different concentrations of NZVI (50, 100, and 250 mg/L) on anaerobic activated municipal sludge and showed 25% and 62% enhancement in biogas and methane, respectively, by 250 mg/L. In another study conducted by Amen et al. (2017), a novel method of coating NZVI on zeolite and mixing NZVI with zeolite is investigated for improving biochemical methane potential and the lag phase from the AD of anaerobic sludge at 37°C for 14 days of HRT. Zeolite is a mineral compound (a mixture of silica, aluminum, and oxygen). It is a non-cytotoxic mineral having a systematic structure containing channel and pore cavities. The authors worked on the idea that zeolite can trap NZVI inside channels and immobilize the NZVI particles on its surface. Using zeolite as an absorbent carrier for NZVI may be a suitable way to stimulate microorganisms and prevent cell membrane disruptions caused by NZVI. The authors used this method to examine the overall performance of the AD process. It can be observed that till day 8, ICZ caused a lag period, and then from day 9 to day 14, it caused significant biogas enhancement (Amen et al., 2017b). The lag phase is attributed to the time required by anaerobic sludge for the adaptation of ICZ. Results showed that 500 mg/L NZVI and 4 g/L zeolite mixture produced 130.87% increase in cumulative biogas production, whereas NZVI alone (45nm, 1000 mg/L) gave a 105.46% increase in cumulative biogas production. The NZVI coated zeolite (ICZ) with 500, and 1000 mg/L concentrations produced the highest amount of biogas in comparison with other additions and control. Cumulative biogas increase of 149.95% and 286.75% is observed for 500 and 1000 mg/L ICZ, respectively. The study concluded that the higher ICZ concentrations generated more biogas and positively affected the AD process.
The influence of NZVI on wastewater sludge AD was also studied by Jia et al. (2017). The impact of the different concentrations of NZVI (500, 1000, 1500, 2000 mg/L) on wastewater sludge at mesophilic conditions (35°C) for 35 days was investigated. The results showed that the group with 500 mg/L and 1000 mg/L NZVI increased cumulative biogas production by 7.30% and 18.11%, respectively, as shown in Figure 6. The higher concentrations of 1500 mg/L and 2000 mg/L NZVI decreased biogas production by 27.30% and 46.45%, respectively., The higher concentration of NZVI resulted in counter-productive, as observed in other studies (Su et al., 2013; Su et al., 2015; Wang et al., 2016). Therefore, in general, it is critical to find the optimal concentration of the NZVI with the specific waste to achieve the goal, i.e., enhancing biogas generation.
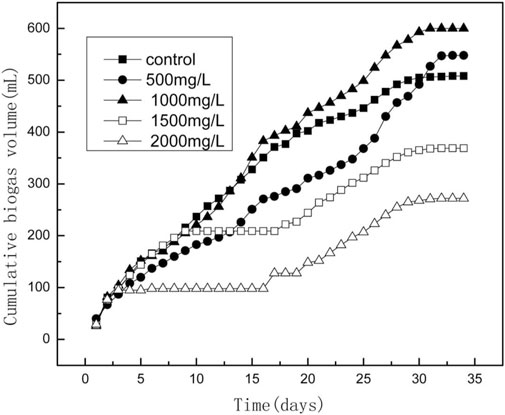
FIGURE 6. Cumulative biogas production by NZVI (Jia et al., 2017).
The long and short-term impact of Ag NPs on the AD of waste activated sludge (WAS) was investigated by Ünşar et al. (2016). During the short-term test, Ag NPs did not show any effect on biogas production. However, during the long-term test, high concentrations (150, 250, and 500 mg/g TS) of Ag NPs showed almost 5% inhibition in methane production, see Figure 7. Wang et al. (2016) studied the influence of Ag NPs on the AD of WAS at concentrations of 1, 10, 100, and 500 mg/g TSS, respectively. The study concluded that Ag NPs had no significant effect on biogas production. The 500 mg/g TSS concentration decreases methane production by 73.52%, as shown in Figure 5. Higher concentrations of Ag NPs decrease the biogas yield because they impede the microbes and activities of key enzymes for the AD process. Gitipour et al. (2016) studied the toxicity of cationic Ag NPs on bio-solids from the wastewater treatment plant to examine the antibacterial impacts of different Ag NPs on the AD process and compared to that of Ag+. Negatively charged citrate-coated Ag NPs (citrate-Ag NPs), minimally charged polyvinylpyrrolidone coated AgNPs (PVP-Ag NPs), and positively charged branched polyethyleneimine coated AgNPs (BPEI-Ag NPs) were investigated. BPEI-Ag NPs showed a significant increase (almost double the amount) in biogas production than control, as shown in Figure 7. Toxicity examination showed that at lower concentrations of Ag NPs, functional redundancy built within the microbial community resulted in low toxicity. However, at high doses, BPEI-Ag NPs resulted in eminent toxicity compared to PVP-Ag NPs and citrate-Ag NPs.
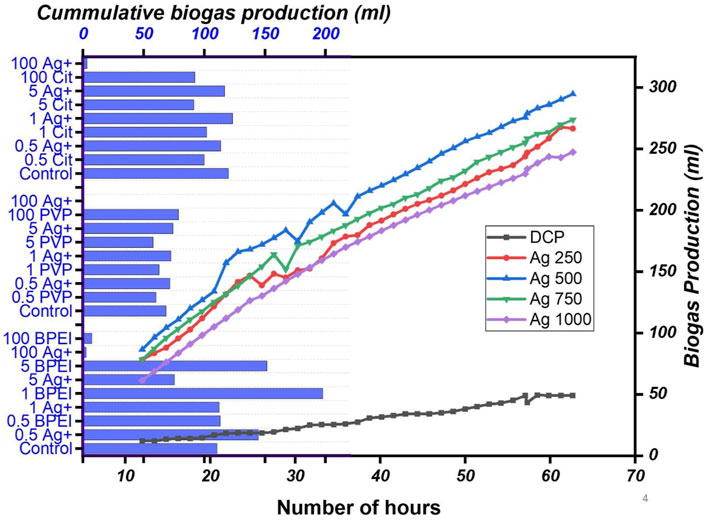
FIGURE 7. Biogas production for different concentrations of Ag NPs (Ünşar et al., 2016) and Cumulative biogas production (horizontal bar) resulted in different concentrations of Ag NPs or Ag+ (Gitipour et al., 2016).
Abdelsalam et al. (2017a) studied the effects of various concentrations (0.5, 1, and 2 mg/L) of Co. and Ni NPs on the production capability of methane and biogas from the conversion of CM (Abdelsalam et al., 2017a). AD of CM was carried out batch-wise at operating temperature and mixing rate of 37 ± 0.3°C and 90 rpm, respectively, for HRT of 50 days. The study indicated that adding 1 mg/L Co. NPs increases the biogas and methane volume by 1.64 and 1.86 times, respectively. The optimal concentration of Ni NPs was found to be 2 mg/L, which increases biogas and methane volume by 1.74 and 2.01 times, respectively. The authors mentioned that the addition of Ni and Co. NPs improved the startup of biogas production and reduced the lag phase compared to control. Co. and Ni NPs showed increased decomposition of organic matter as more decomposition of Total Solids (TS), and Volatile Solids (VS.) observed at the end of the experiment. Elreedy et al. (2017) also investigated the influence of Ni NPs (60 nm) at much higher concentrations compared to the work in (Abdelsalam et al., 2017a). The Ni NPs concentration in this study was 20, 30, 60, and 100 mg/L on the AD of industrial wastewater containing Mono-Ethylene Glycol (MEG). Results showed that 60 mg/L of Ni NPs produced an increase of 23% in hydrogen production. This result suggested that a higher dose of NPs is required for industrial waste to enhance biogas production. It would be interesting to see that similar waste has been tested for lower NPs concentration for industrial waste, but the authors of this review were unable to find it.
Our previous work (Zaidi et al., 2018) explored the influence of Ni and Co. NPs on biogas yield from the AD of green microalgae (Enteromorpha), which was the first study to discover the significance of NPs on microalgae. Results indicated that 1 mg/L of Ni and Co. NPs produced 26 and 9% cumulative increase in biogas production. It was observed that during the less effective domain (see Figure 8), NPs revealed no significant result to improve biogas production. However, approximately 60 h of the digestion process, NPs showed the cumulative effect on biogas production. The increase in biogas production was credited to the release of extracellular polymeric compounds (proteins, carbohydrates, and cellulose) after the dissolution of the microalgae cell wall. In order to understand the effectivity of NPs on the AD of microalgal biomass, measurement of soluble indexes such as Chemical Oxygen Demand (COD), reducing sugar, pH and VFA were measured. It was found that COD and VFA increased for the groups with NPs, whereas reducing sugar decreased as NPs stimulated bacteria to consume more sugar during the AD.
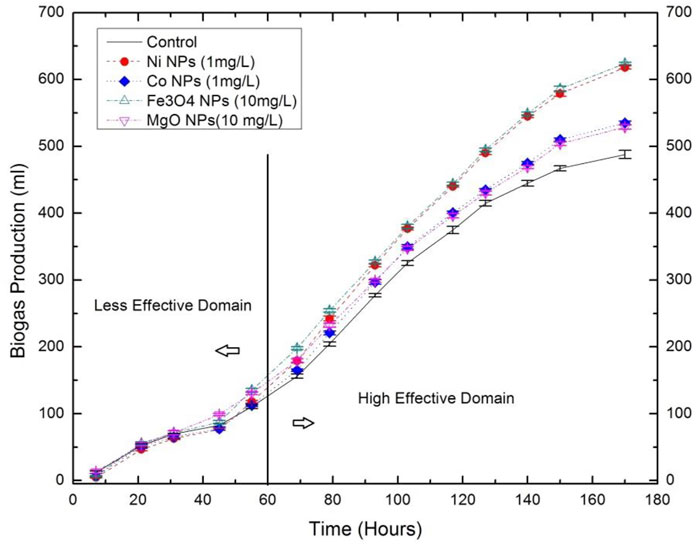
FIGURE 8. Biogas production influenced by nanoparticles (Zaidi et al., 2018).
An exhaustive list and summary of the reported metal NPs including size, concentration, type of feedstock used, anaerobic temperature, HRT, and their effect on biogas and methane production, is shown in Table 2.
Various metal NPs effect on biogas production from different feedstock is presented in this section. NZVI was the most reported one, along with Ni and Co. NPs, which showed an increase in biogas production. On the other hand, Ag, citrate-Ag, PVP-Ag, BPEI-Ag, Au, and Zn silica nanogel showed adverse effects on the biogas production rate, resulting in a dramatic decrease in the amount of biogas produced. This decrease was attributed to the toxicity of the materials.
Utilization of metal oxide nanoparticles for biogas production
The effect of ZnO and CeO2 NPs with different concentrations (10, 100, 500, and 1000 mg/L) on anaerobic sludge from an Up-flow Anaerobic Sludge Blanket (UASB) reactor was studied by Nguyen et al. (Nguyen et al., 2015) under mesophilic temperature (30°C) for 40 days. Results showed that all investigated concentrations of ZnO and CeO2 NPs produce biogas less than the control except 10 mg/L CeO2 NPs sample, which produced only an 11% increase in biogas, as shown in Figure 9. This study remotely suggested that the role of oxides may be limited to use for biogas production; fortunately, this is not the case. The authors performed a bacterial toxicity test to explore the biogas inhibition effect. They found that ZnO NPs are more highly toxic to Escherichia coli than CeO2 NPs and caused 99% cell death at 100 mg/L and so the same at higher concentrations. The authors attributed the positive effect of 10 mg/L CeO2 NPs on the bacterial viability of sludge digestion as their ability to act like free radicals.
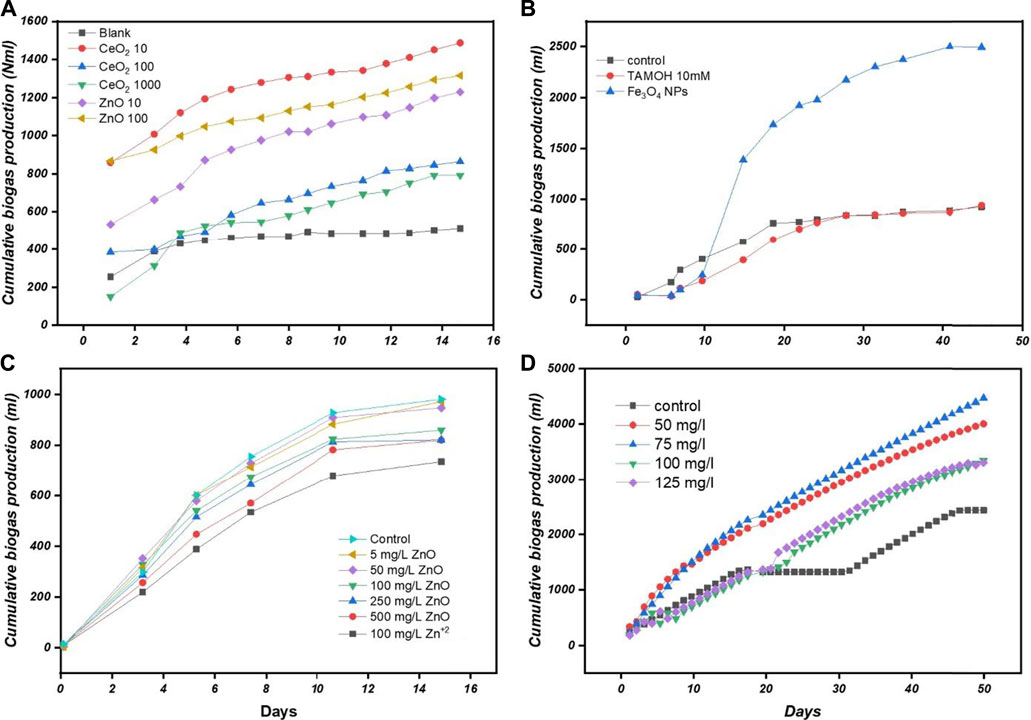
FIGURE 9. (A) Influence of Ce O 2 and ZnO NPs on biogas production (Nguyen et al., 2015) (B) Effect of 100ppm Fe3 O 4 on biogas production (scale bar is 20 nm) (Casals et al., 2014) (C) Effect of ZnO ENMs on production after 14 days (Zhang L. et al., 2017) (D) Cumulative methane production by Fe3 O 4 NPs (Ali et al., 2017).
The long and short-term inhibition impacts of CuO and CeO2 NPs was studied by Ünşar et al. (2016) on the AD of WAS. The AD inhibition effect was observed from 5.8% to 84% when CuO NPs concentration increased from 5 mg/g to 1000 mg/g TS. CeO2 NPs with dosages of 150, 250, and 500 mg/g TS enhanced the methane yield to 18.8%, 25.5%, and 9.2%, respectively (Ünşar et al., 2016). Fluorescence in situ hybridization (FISH) analysis exposed a decrease in archaea in CuO NPs samples, whereas the abundance of these bacteria was found in CeO2 NPs.
Casals et al. (2014) also performed an anaerobic experiment under mesophilic conditions by applying Fe3O4 NPs (100 ppm) to organic waste for about 2 months. It was concluded that this set of conditions promises an increment in the production of methane and biogas by 234% and 180%, respectively, as shown in Figure 9. In addition, Fe2+ was identified as the main contributing factor as it serves to disintegrate waste fabulously in anaerobic conditions. This is probably one of the highest increments of biogas and methane production one can find in the available literature.
In the AD process, metal distribution conversion is another important aspect, as discussed by Suanon et al. (Suanon et al., 2016). The effect was studied by employing Fe3O4 NPs in an anaerobic batch chamber with mesophilic conditions. The methane production was incremented by 1.5 gm per 500 ml of Fe3O4 NPs. It was concluded that the presence of Fe3O4 NPs is favorable for metal stabilization in the digestate as it ultimately results in an improvement in biogas production. However, it promotes the immobilization of phosphorus in digestate. The information mentioned in the paper was not conclusive to support the immobilization hypothesis, and the authors have acknowledged this to suggest further research.
Abdelsalam et al. (2017b) also contributed by studying the effect of Fe3O4 NPs on biogas production. By employing different concentrations on CM slurry, mixing temperature of 37 ± 0.3°C at an rpm of 90 and HRT of 50 days; biogas and methane production was incremented by 1.66 and 1.96 times, respectively, by adding just 20 mg/L Fe3O4 NPs as shown in Figure 9.
Wang et al. (2016) studied the influence of MgO and Fe2O3 NPs on the AD of WAS at concentrations of 1, 10, 100, and 500 mg/g TSS, respectively. The concentration of 100 mg/g TSS of iron oxide NPs gives 117% of the control, whereas other concentrations had no measurable effect on biogas; see Figure 5. MgO NPs had no significant effect on biogas production (Shi et al., 2020). The 500 mg/g TSS concentration inhibited methane production by 1.08%. Higher concentrations of MgO NPs decrease the biogas yield because they impede the microbes and activities of key enzymes for the AD process, see Figure 5.
Li et al. (2017) studied the fate and long-term exposure of CuO, TiO2, and ZnO NPs (50 mg/L) on the AD of Anaerobic Granular Sludge (AGS) for 90 days. The results showed that CuO NPs stopped the methane production on the 39th day. Long-term exposure resulted in inhibited methanogenesis strongly and quickly. The exposure of TiO2 NPs lowered the biogas and methane production by 30.70% and 14.01%, respectively. The study suggested that TiO2 NPs had an adverse effect on the acidogens and acetogens than methanogens. The effect of TiO2 NPs on anaerobic sludge from the UASB reactor was also investigated by Yadav et al. (Yadav et al., 2017). Outcomes of their study indicated a slight biogas inhibition in line with the results obtained by Li et al. (2017).
Syntrophic oxidation of butyrate (intermediates in the transformation of complex organics to methane) was studied by Zhang and Lu (2016) in two different lake sediments. The authors used conductive Fe3O4 NPs to accelerate the reaction kinetics. Results indicated that methane yield was substantially increased, and the lag phase reduced significantly under the presence of NPs. 25μmol CH4/liter was produced from 10 μmol of butyrate addition. The authors performed Direct Interspecies Electron Transfer (DIET) and found that cell-to-cell distance in enrichments amended with NPs was larger than control. They suggested that conductive NPs form cell-nanomaterial-cell networks and facilitate DIET, which contributed to an enhancement in methane.
The response of iron oxide NPs on AGS during AD of beet sugar industrial wastewater was investigated by Ambuchi et al. (2017). Three Plexiglas Expanded Granular Sludge Bed (EGSB) reactors were used under a mesophilic temperature of 36 ± 1°C for an incubation period of 74 days. More biogas was produced during the first 24 h than in the control reactor. The initial increase in biogas production was also observed in another study (Abdelsalam et al., 2017b). Results showed 1.25 times increase in biogas and 28.9% more ml/g-VSS CH4 gas. The authors stated that the employment of iron oxide NPs as conduits for electron transfer toward methanogens resulted in biogas enhancement.
A comparative study of Fe3O4, Co3O4, NiO, and MoO3 micronutrient and NPs with CM slurry in the single and bi-phasic AD at 37 ± 2°C for 20 days was carried out by Juntupally et al. (2017). During a single-phase AD, Fe3O4 NPs produced 0.16 L/(g VS. reduced) biogas. An increase in biogas production with enhanced methane (70–80%) is reported during single-phase, whereas in bi-phase, AD Fe2O3 and its corresponding NPs showed a 76% increase (Juntupally et al., 2017). NiO NPs yielded peak biogas of 0.3 L/(g VS. reduced) in the biphasic AD compared to Co3O4 and MoO3 NPs. During single-phasic AD, NiO and Co3O4 NPs provided the same biogas yield of 0.15 L/(g VS. reduced).
The effect of different concentrations of ZnO NPs (as shown in Figure 9) on VFAs and biogas production during AD of WAS investigated by Lingling Zhang et al. (2017). Results showed that VFA production is inversely correlated to ZnO NPs concentrations. ZnO NPs inhibited the waste sludge hydrolysis-acidification, mainly protein. ZnO NPs’ impact on protein hydrolysis slowed down the VFA accumulation during AD and decreased biogas production, as shown in Figure 9. This action also changed bacterial community structure and was identified to be the main reason for biogas reduction.
Temizel et al. (2017) investigated the influence of ZnO NPs on sanitary landfills for biogas production. They used landfill bioreactors operated at 35°C for 1 year. The results obtained indicated that reactors inoculated with ZnO NPs produced less biogas than the control reactor. The authors mentioned that the release of Zn2+ might adversely affect the methanogenic archaea activity, and hence inhibition in biogas yield occurred. Biogas from landfills is being recognized as one potential source for bioenergy production; the authors suggested that the presence of ZnO NPs in a waste matrix of landfills may become a hurdle to its application. The toxic effect of ZnO NPs indicated in this study agrees with Li et al. (2017), who also investigated the effect of ZnO NPs on the AD of AGS and found that methane and biogas yield was suppressed. They mentioned that long-term exposure resulted in inhibited methanogenesis vigorously and quickly.
The effect of bio-compatible Fe3O4 NPs (10–35 nm) at four different concentrations (50, 75, 100, and 125 mg/L) on the AD of Municipal Solid Waste (MSW) was investigated by Ali et al. (2017) at 37 ± 0.5°C for 60 days of HRT. Results indicated that concentration of 50 and 75 mg/L was found to be more effective in improving the methane production as compared to increased concentrations at 100 and 125 mg/L, see Figure 9. This is in contrast with the results obtained by Abdelsalam et al. (2017b).
In one of our previous studies, the experience of studying green microalgae’s anaerobic digestion (Enteromorpha) for biogas production by employing Fe3O4 and MgO NPs have been promising (Zaidi et al., 2018). A cumulative increase of 28% for 10 mg/L of Fe3O4 NPs and 8% for 10 mg/L of MgO NPs was noticed. As a controlled sample, an additional effect of NPs approaches zero in the less effective domain. Nevertheless, after observation of 60 h, a substantial effect incrementing biogas production was noticed. The increase in biogas production was credited to the release of extracellular polymeric compounds (proteins, carbohydrates, and cellulose) after the dissolution of the microalgae cell wall. Table 3 comprehensively summarizes the metal oxide NPs and their effect on biogas generation.
This section discussed the addition of different metal oxide NPs during the AD for biogas production. Fe2O3, Fe3O4, Co3O4, NiO, MoO3 showed an increase in biogas production. On the other hand, CeO2 showed mixed effects depending on their concentration in the reactor as well as the digestion time. The addition of nano-iron oxide (Fe3O4) enhanced methane production by 234% due to the presence of the non-toxic Fe3+ and Fe2+ ions. ZnO, CuO, TiO2, MgO, MnO2 showed a decrease or no change in biogas production rate (Mishra et al., 2018).
Nano-scaled Nb-based compounds in biogas
The functionality of Nb-based compounds (NbO2, Nb3.49N4.56O0.44, and NbN) with various concentrations (7.5, 15, 30, 60, and 120 mg/L) at mesophilic condition (36 ± 1°C) in the AD of dairy manure was investigated by Taihong Zhang et al. (2017). This is the first study discussing the application of these compounds for AD. The results showed that Nb-based compounds worked as efficient catalysts in the AD process. They improve the fermentation condition and stimulate the bacterial activity inside the digester. The cumulative biogas production by NbO2, Nb3.49N4.56O0.44, and NbN produced was 522.7, 437.1, and 455.7 ml/g VS., respectively (Zhang T. et al., 2017). Table 4 summarizes reported Nb-based compounds and their effect on biogas production.
Nano-scaled transition metal carbides for biogas enhancement
The effect of nano-scale transition metal carbides (HfC, SiC, TiC, and WC) at a concentration of 0.25 wt% on the AD of CM was investigated by Li et al. (2018) batch-wise under mesophilic temperature. The experiments were performed in triplicates and average data was presented. Results showed that all these four carbides worked as accelerants in the AD process. HfC, SiC, TiC and WC increased biogas production by 63.9, 69.7, 57.5 and 69%, respectively, as compared to control check (CK), see Figure 10. We found that this is the first and maybe the only report on using metal carbides to inoculate in AD digesters. Table 5 summarizes nano-scale transition metal carbides and their influence on biogas generation.
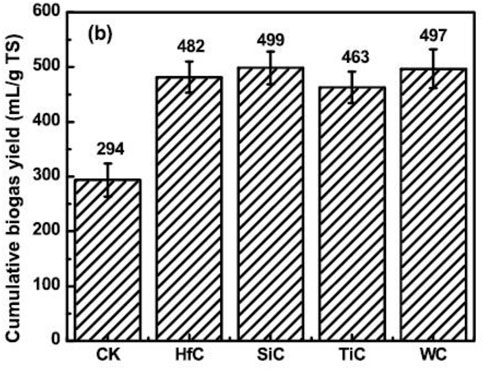
FIGURE 10. Cumulative biogas yield by nano-scale transition metal carbides (Li et al., 2018).
Utilization of carbon and carbon-based nanomaterials for biogas
The one and the only study discussing the influence of Single-Walled Carbon Nanotubes (SWCNTs) on AD of AGS, with average diameters of 1–2 nm and length of 5–20 nm at a concentration of 1000 mg/L, under mesophilic conditions (35°C) for 8 days was examined by Li et al. (2015). SWCNTs did not reflect any significant enhancement in biogas and methane generation, see Figure 11. In the presence of 1000 mg/L SWCNTs, the volume of generated CH4 was significantly larger (p < 0.05) than that in the control reactor for the initial 48 h. However, it slowly decreased and ended at almost the same or little lower cumulative production as control, showing no effect. The authors attributed this zero effect of SWCNTs as a decrement in cytotoxicity of sludge by nanotubes. The addition of SWCNTs in the AD system produced a more Extracellular Polymeric Substance (EPS) which prevented SWCNTs from reaching cells and hence resulted in limited to no effect on biogas yield.
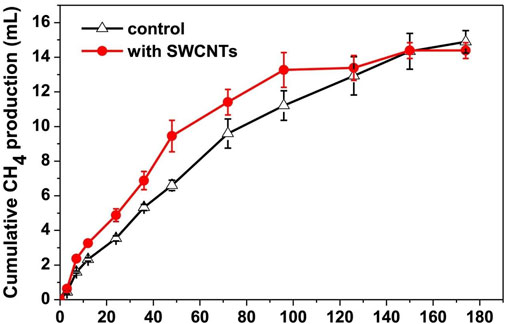
FIGURE 11. The influence of SWCNTs on methane production in Hours (Li et al., 2015).
Impact of Multi-Walled Carbon Nanotubes (MWCNTs) with the length of 1–10 μm, outer and inner diameters of 5–20 nm and 2–6 nm, respectively, were investigated on UASB microflora by Yadav et al. (Yadav et al., 2016). It was observed from SEM and fluorescent microscopy images that MWCNTs damaged acidogenic and acetogenic microbial cells, which caused an increase in EPS proteins, DNA, and carbohydrates. According to the authors, this microbial cell damage is the possible reason for low VFAs generation and biogas yield. The 1 mg/L and 100 mg/L concentration of MWCNTs caused 21% and 54% inhibition in biogas as compared to control.
In contrast, Zhang and Lu (2016) found an enhancement in biogas production with conductive MWCNTs (diameter: 10–20 nm, length: 10–30 mm) by syntrophic oxidation of butyrate in two different lake sediments. The CH4 production rate in the presence of MWCNTs was almost 50% greater than the control. The results showed that the electric conductivity of the added MWCNTs facilitated the syntrophic oxidation of butyrate and had a stimulatory effect on microorganisms. Microscopic observation showed that abundant aggregates formed in lake enrichments under the presence of MWCNTs. The microbial aggregates in control were in close physical proximity whereas, in MWCNTs samples, dark areas within aggregates filled with nanotubes. This showed that greater intercellular distances existed on average, which form cell-nanotube-cell networks and facilitate DIET, which contributed to an increase in methane yield.
In another study, Ambuchi et al. (2017) investigated the response of MWCNTs (10–20 nm outer diameter) on AGS during AD of beet sugar industrial wastewater. An increase in biogas (1.09 times than control) and methane production (12.6% more ml/g-VSS CH4 gas than control) was observed. Summarized results reported that carbon nanotubes influence on biogas generation is shown in Table 6.
Nanowires, nano composites and nano-ash augmentation for biogas
Nanowires
The Octahedral Molecular Sieve (OMS-2) is a form of manganese dioxide that holds distinctive features like mixed-valence of manganese, acidic sites and has wide applications. The effect of synthesized OMS-2 NPs (diameter of nanofibers of about 10–20 nm and lengths of about 100–500 nm) on Sludge from the sewage treatment plant at concentrations of 0.025, 0.25, and 2.50 g/L was investigated by Pan et al. (2015). The addition of 0.025 g/L OMS-2 NPs resulted in an 11% enhancement in biogas production. The investigation of microbial metabolism revealed an increase in microbial metabolic level and enhanced microbial diversity. OMS-2 NPs also increased the quantities of acetogenic bacteria and Archaea and promoted acetogenesis and methanogenesis.
Lupitskyy et al. (2018) studied the influence of zinc oxide nanowires at a concentration of 1 g/L on the AD of AGS. According to the author, the use of ZnO nanowires as inorganic reactive absorbents can help in reducing the sulfur-containing compounds in wastewater and improve biogas production. The experiment was carried out for three feeding cycles. Sulfates were added at the beginning of each feeding cycle. Results showed that nanowires reduced the sulfide toxicity during AD as no methanogenic activity and biogas inhibition were observed (Lupitskyy et al., 2018). The summary of the reported nanowire and its influence on biogas generation is shown in Table 7.
Nano-composites
The effect of Ni-graphene nano-composite (Ni-Gr-NC) as a supplement to an AD of industrial wastewater containing MEG to enhance biohydrogen production was studied by Elreedy et al. (2017). The authors used the unique properties of Ni-based NPs as Ni ion suppliers and graphene as support materials. This is the first study with Ni-Gr-NC addition to the AD process. The results showed that 60 mg/L dosages caused a 105% increase in hydrogen production from other concentrations. The maximum specific hydrogen production obtained by Ni-Gr-NC (60 mg/L dose) was 294.24 ± 12.06 ml/L, see Figure 12. The hydrogenase enzyme activity affected by Ni ions in the presence of graphene resulted in an enhanced hydrogen yield. The summary of the reported nano-composites and their influence on biogas generation is shown in Table 7.
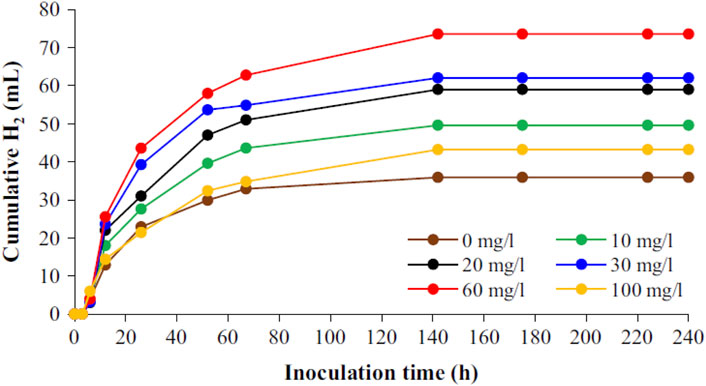
FIGURE 12. Cumulative hydrogen production at different concentrations of Ni-Gr NC (Elreedy et al., 2017).
Mansour et al. (2020) studied the effect of Ni-Co-Ferrite on biogas production and reported that these nano additives increase biogas production by about 30%. In another study, Hassaneen et al. (2020) proposed the use of a novel nanocomposite (based on metal enzyme cofactors, highly conductive carbon materials, and DIET activators) and tested different formulations for the enhancement of biogas production. Methane production was observed to boost by 185.3% using Zn ferrite.
Nano-ash
The influences of micro-nano fly and bottom ash attained from MSW incinerator on the AD of MSW were investigated by Lo et al. (2012) at mesophilic conditions (35°C) for 90 days. The concentrations used for micro-nano fly ash was 0.12, 3, 6, 18, and 30 g/g VS. whereas micro-nano bottom ash was used at the concentration of 0.6, 12, 36, 60, and 120 g/g VS Results indicated that micro-nano fly and bottom ash produced a significant enhancement in biogas generation. The inoculation of 36 g/g VS. bottom ash produced the highest amount of biogas production among all dosages, as shown in Figure 12. The authors mentioned that the presence of various compounds (Al2O3, ZnS, CaCO3, CaMg(CO3)2, Ca3SiO5, Ca(OH)2, PbO, SiO2, and Ca2SiO4) inside fly and bottom ash increased biogas production. The compounds present in the form of nano-substances supplied additional habitats for the microorganism. The summary of the reported nanoash and its influence on biogas generation is shown in Table 7.
Key challenges and way forward to nanomaterials augmentation in biogas production
Nanomaterials as additives to biomass were widely studied for biogas production enhancement, especially in the last decade. Unfortunately, their use may not always enhance biogas production, depending on many factors such as the size of nanomaterials, their concentration, and the type of substrate used. However, it is observed that nanomaterials used in the mixture tend to produce a much better effect on biogas production than separately used. Using different nanomaterials as a mixture and studying their interactions with different substrates could be a leading field research area in the years to come.
Furthermore, the environmental impact of NMs application with biomass for biogas production has not been discussed thoroughly, and climate concerns remain high for spent biomass with NMs. One of the significant challenges that need to be addressed urgently is that after utilizing NMs in AD, how to track them, and what would be the best methodology for dumping the waste and biomass that contains NMs? There is a possibility that spent biomass with a high concentration of NMs may prove beneficial for soil and help maintain a nutrient level in the soil. On the other hand, these nanomaterials can increase the toxicity of the area and can also mix with underground water. These aspects have to be answered in future studies. Moreover, multiple studies can be found on the feasibility and financial aspect of NMs application in biogas production throughout the literature. However, studies related to NMs in biomass applications’ environmental analysis and life cycle assessment are quite rare, which needs attention in future studies. The review and analysis of the available literature conducted in this study, the future direction, research area, and themes are depicted in Figure 3B. Currently, the most active countries working on nanotechnology-based biogas production as per citation record (minimum 100 documents and 100 citations) are presented in Figure 4. In addition, future guidelines may comprise the following:
1. In order to avoid the toxicity of the presently spent nanomaterials, causing an inhibitory effect on anaerobic bacteria, bioactive nanomaterials can be used for process improvement.
2. Recollecting spent nanomaterials at the end of the process remained a significant drawback for the environment and sustainability of their utilization in biogas or related applications. Avoiding the leak of nanomaterials in the natural resources and designing processes that limit this to happen should be the top priority for the implementation for large-scale production.
3. Optimization of nanomaterials for a wide range of sizes, doses, and shapes can be carried out to get the maximum advantage of nanotechnology for biogas and methane production.
4. Microalgae and lignocellulose biomass are potential feedstock for bioenergy production. However, the effect of NPs on these substrates can be carried out for improvement in biogas production.
5. Other commonly applied methods for biogas escalation, including pretreatment of substrate or inoculum and supplementation biological and inorganic additives, can be used in combination nanomaterials to get an overall energy gain.
Conclusion
By method of quantitative literature review, the impact of NMs on biogas production and methane yield is stated in this study. Several kinds of NMs have been investigated as additives in the AD process for biogas augmentation for various kinds of biodegradable wastes. For brevity, the eventual effect of nanomaterials and their positive or negative impacts on biogas generation are summarised in Table 8, which is concluded from the exhaustive literature review and presented from the materials’ point of view. Additionally, the following conclusions have been drawn from the reviewed literature.
• Metal NPs such as NZVI, Co., and Ni showed a positive effect on biogas yield. However, Ag NPs showed no inhibitory effect.
• Metal oxide NPs such as iron oxide (Fe2O3 and Fe3O4), Co3O4, NiO, MoO3 NPs showed an increase in biogas and methane production, whereas ZnO, TiO2, CeO2, and CuO NPs showed an inhibitory effect. In contrast, the literature showed MgO NPs showed a mixed effect.
• Nb-based compounds (NbO2, Nb3.49N4.56O0.44, and NbN) and nano-scale transition metal carbides (HfC, SiC, TiC, and WC) showed an enhancement in biogas yield.
• Carbon nanotubes showed a mixed effect. Single-walled CNTs showed no effect, whereas multiwall CNTs showed an increase in biogas production.
Author contributions
SK, AZ, and HA contributed to the conception and data collection of the study. SK and AZ wrote the first initial draft of the manuscript. MN performed a bibliometric technique for the data sets. All authors contributed to manuscript revision, read, and approved the submitted version.
Funding
The authors would like to express their appreciation for the support provided by the Scientific Research Deanship, Islamic University of Madinah, through the research program “Research Groups (first)” grant number 952.
Conflict of interest
The authors declare that the research was conducted in the absence of any commercial or financial relationships that could be construed as a potential conflict of interest.
Publisher’s note
All claims expressed in this article are solely those of the authors and do not necessarily represent those of their affiliated organizations, or those of the publisher, the editors and the reviewers. Any product that may be evaluated in this article, or claim that may be made by its manufacturer, is not guaranteed or endorsed by the publisher.
Abbreviations
AD, Anaerobic Digestion; AGS, Anaerobic Granular Sludge; CM, Cattle Manure; COD, Chemical Oxygen Demand; DIET, Direct Interspecies Electron Transfer; EGSB, Expanded Granular Sludge Bed; EPS, Extracellular Polymeric Substance; HRT, Hydraulic Retention Time; ICZ, NZVI coated zeolite; MEG, Mono-Ethylene Glycol; MSW, Municipal Solid Waste; MWCNT, Multi-Walled Carbon Nanotubes; NC, Nano-composite; NM, Nanomaterials; NP, Nanoparticles; NW, Nanowires; NZVI, Nanoscaled Zero-Valent Iron; OMS, Octahedral Molecular Sieve; SWCNT, Single-Walled Carbon Nanotubes; TS, Total Solids; TSS, Total Suspended Solids; UASB, Up-flow Anaerobic Sludge Blanket; VFA, Volatile Fatty Acids; VS, Volatile Solids; WAS, Waste Activated Sludge.
References
Abdallah, M. S., Hassaneen, F. Y., Faisal, Y., Mansour, M. S., Ibrahim, A. M., Abo-Elfadl, S., et al. (2019). Effect of Ni-Ferrite and Ni-Co-Ferrite nanostructures on biogas production from anaerobic digestion. Fuel 254, 115673. doi:10.1016/j.fuel.2019.115673
Abdelsalam, E., Samer, M., Attia, Y. A., Abdel-hadi, M. A., Hassan, H. E., and Badr, Y. (2016). Comparison of nanoparticles effects on biogas and methane production from anaerobic digestion of cattle dung slurry. Renew. Energy 87, 592–598. doi:10.1016/j.renene.2015.10.053
Abdelsalam, E., Samer, M., Attia, Y. A., Abdel-Hadi, M. A., Hassan, H. E., and Badr, Y. (2017a). Effects of Co and Ni nanoparticles on biogas and methane production from anaerobic digestion of slurry. Energy Convers. Manag. 141, 108–119. doi:10.1016/j.enconman.2016.05.051
Abdelsalam, E., Samer, M., Attia, Y. A., Abdel-Hadi, M. A., Hassan, H. E., and Badr, Y. (2017b). Influence of zero valent iron nanoparticles and magnetic iron oxide nanoparticles on biogas and methane production from anaerobic digestion of manure. Energy 120, 842–853. doi:10.1016/j.energy.2016.11.137
Ali, A., Mahar, R. B., Soomro, R. A., and Sherazi, S. T. H. (2017). Fe3O4 nanoparticles facilitated anaerobic digestion of organic fraction of municipal solid waste for enhancement of methane production. Energy Sources, Part A Recovery, Util. Environ. Eff. 39 (16), 1815–1822. doi:10.1080/15567036.2017.1384866
Alvarez, L. H., and Cervantes, F. J. (2012). Assessing the impact of alumina nanoparticles in an anaerobic consortium: Methanogenic and humus reducing activity. Appl. Microbiol. Biotechnol. 95 (5), 1323–1331. doi:10.1007/s00253-011-3759-4
Ambuchi, J. J., Zhang, Z., and Feng, Y. (2016). Biogas enhancement using iron oxide nanoparticles and multi-wall carbon nanotubes. Int. J. Chem. Mol. Nucl. Mater. Metallurgical Eng. 10, 1239–1246.
Ambuchi, J. J., Zhang, Z., Shan, L., Liang, D., Zhang, P., and Feng, Y. (2017). Response of anaerobic granular sludge to iron oxide nanoparticles and multi-wall carbon nanotubes during beet sugar industrial wastewater treatment. Water Res. 117, 87–94. doi:10.1016/j.watres.2017.03.050
Amen, T. W. M., Eljamal, O., Khalil, A. M. E., and Matsunaga, N. (2017b). Evaluation of nano zero valent iron effects on fermentation of municipal anaerobic sludge and inducing biogas production. IOP Conf. Ser. Earth Environ. Sci. 67, 012004. doi:10.1088/1755-1315/67/1/012004
Amen, T. W. M., Eljamal, O., Khalil, A. M. E., and Matsunaga, N. (2017a). Biochemical methane potential enhancement of domestic sludge digestion by adding pristine iron nanoparticles and iron nanoparticles coated zeolite compositions. J. Environ. Chem. Eng. 5 (5), 5002–5013. doi:10.1016/j.jece.2017.09.030
Antonio, F., Antunes, F., Gaikwad, S., and Ingle, A. P. (2017). “Nanotechnology for bioenergy and biofuel production,” in Green chemistry and sustainable technology (Springer International Publishing), 3–18. doi:10.1007/978-3-319-45459-7
Bidart, C., Fröhling, M., and Schultmann, F. (2014). Livestock manure and crop residue for energy generation: Macro-assessment at a national scale. Renew. Sustain. Energy Rev. 38, 537–550. doi:10.1016/j.rser.2014.06.005
Carpenter, A. W., Laughton, S. N., and Wiesner, M. R. (2015). Enhanced biogas production from nanoscale zero valent iron-amended anaerobic bioreactors. Environ. Eng. Sci. 32 (8), 647–655. doi:10.1089/ees.2014.0560
Casals, E., Barrena, R., Garcia, A., Gonzalez, E., Delgado, L., Busquets-Fite, M., et al. (2014). Programmed iron oxide nanoparticles disintegration in anaerobic digesters boosts biogas production. Small 10 (14), 2801–2808. doi:10.1002/smll.201303703
Cervantes-Avilés, P., Ida, J., Toda, T., and Cuevas-Rodríguez, G. (2018). Effects and fate of TiO2 nanoparticles in the anaerobic treatment of wastewater and waste sludge. J. Environ. Manag. 222, 227–233. doi:10.1016/j.jenvman.2018.05.074
Chen, J. L., Steele, T. W. J., and Stuckey, D. C. (2018). The effect of Fe2NiO4 and Fe4NiO4Zn magnetic nanoparticles on anaerobic digestion activity. Sci. Total Environ. 642, 276–284. doi:10.1016/j.scitotenv.2018.05.373
Chen, L., Hu, Q., Zhang, X., Cai, Z., and Wang, Y. (2019). Effects of ZnO nanoparticles on the performance of anaerobic membrane bioreactor: An attention to the characteristics of supernatant, effluent and biomass community. Environ. Pollut. 248, 743–755. doi:10.1016/j.envpol.2019.02.051
Chen, Y., Mu, H., and Zheng, X. (2014). Chronic response of waste activated sludge fermentation to titanium dioxide nanoparticles. Chin. J. Chem. Eng. 22 (10), 1162–1167. doi:10.1016/j.cjche.2014.09.007
Christy, P. M., Gopinath, L. R., and Divya, D. (2014). A review on anaerobic decomposition and enhancement of biogas production through enzymes and microorganisms. Renew. Sustain. Energy Rev. 34, 167–173. doi:10.1016/j.rser.2014.03.010
Demetzos, C. (2016). “Introduction to nanotechnology,” in Pharmaceutical nanotechnology -fundamentals and practical applications (Singapore: Adis Singapore), 3–16. doi:10.1007/978-981-10-0791-0
Eduok, S., Ferguson, R., Jefferson, B., Villa, R., and Coulon, F. (2017). Aged-engineered nanoparticles effect on sludge anaerobic digestion performance and associated microbial communities. Sci. Total Environ. 609, 232–241. doi:10.1016/j.scitotenv.2017.07.178
Ellacuriaga, M., Cascallana, J. G., González, R., and Gómez, X. (2021). High-solid anaerobic digestion: Reviewing strategies for increasing reactor performance. Environments 8 (8), 80. doi:10.3390/environments8080080
Elreedy, A., Fujii, M., Koyama, M., Nakasaki, K., and Tawfik, A. (2019). Enhanced fermentative hydrogen production from industrial wastewater using mixed culture bacteria incorporated with iron, nickel, and zinc-based nanoparticles. Water Res. 151, 349–361. doi:10.1016/j.watres.2018.12.043
Elreedy, A., Ibrahim, E., Hassan, N., El-Dissouky, A., Fujii, M., Yoshimura, C., et al. (2017). Nickel-graphene nanocomposite as a novel supplement for enhancement of biohydrogen production from industrial wastewater containing mono-ethylene glycol. Energy Convers. Manag. 140, 133–144. doi:10.1016/j.enconman.2017.02.080
Erdim, E., Yücesoy Özkan, Z., Kurt, H., and Alpaslan Kocamemi, B. (2019). Overcoming challenges in mainstream Anammox applications: Utilization of nanoscale zero valent iron (nZVI). Sci. Total Environ. 651, 3023–3033. doi:10.1016/j.scitotenv.2018.09.140
Faisal, S., Yusuf Hafeez, F., Zafar, Y., Majeed, S., Leng, X., Zhao, S., et al. (2019). A review on nanoparticles as boon for biogas producers—Nano fuels and biosensing monitoring. Appl. Sci. 9 (1), 59. doi:10.3390/app9010059
Farghali, M., Andriamanohiarisoamanana, F. J., Ahmed, M. M., Kotb, S., Yamashiro, T., Iwasaki, M., et al. (2019). Impacts of iron oxide and titanium dioxide nanoparticles on biogas production: Hydrogen sulfide mitigation, process stability, and prospective challenges. J. Environ. Manag. 240, 160–167. doi:10.1016/j.jenvman.2019.03.089
Feng, R., Li, Q., Zaidi, A. A., Peng, H., and Shi, Y. (2021). Effect of autoclave pretreatment on biogas production through anaerobic digestion of green algae. Period. Polytech. Chem. Eng. 65, 483–492. doi:10.3311/ppch.18064
Feng, R., Zaidi, A. A., Zhang, K., and Shi, Y. (2018). Optimisation of microwave pretreatment for biogas enhancement through anaerobic digestion of microalgal biomass. Period. Polytech. Chem. Eng. 63 (1), 65–72. doi:10.3311/PPch.12334
Feng, Y., Zhang, Y., Quan, X., and Chen, S. (2014). Enhanced anaerobic digestion of waste activated sludge digestion by the addition of zero valent iron. Water Res. 52, 242–250. doi:10.1016/j.watres.2013.10.072
Ganzoury, M. A., and Allam, N. K. (2015). Impact of nanotechnology on biogas production: A mini-review. Renew. Sustain. Energy Rev. 50, 1392–1404. doi:10.1016/j.rser.2015.05.073
García, A., Delgado, L., Torà, J. A., Casals, E., González, E., Puntes, V., et al. (2012). Effect of cerium dioxide, titanium dioxide, silver, and gold nanoparticles on the activity of microbial communities intended in wastewater treatment. J. Hazard. Mater. 199–200, 64–72. doi:10.1016/j.jhazmat.2011.10.057
Gitipour, A., Thiel, S. W., Scheckel, K. G., and Tolaymat, T. (2016). Anaerobic toxicity of cationic silver nanoparticles. Sci. Total Environ. 557–558, 363–368. doi:10.1016/j.scitotenv.2016.02.190
Gonzalez-Estrella, J., Sierra-Alvarez, R., and Field, J. A. (2013). Toxicity assessment of inorganic nanoparticles to acetoclastic and hydrogenotrophic methanogenic activity in anaerobic granular sludge. J. Hazard. Mater. 260, 278–285. doi:10.1016/j.jhazmat.2013.05.029
Hagos, K., Zong, J., Li, D., Liu, C., and Lu, X. (2017). Anaerobic co-digestion process for biogas production: Progress, challenges and perspectives. Renew. Sustain. Energy Rev. 76, 1485–1496. doi:10.1016/j.rser.2016.11.184
Hao, Y., Wang, Y., Ma, C., White, J. C., Zhao, Z., Duan, C., et al. (2019). Carbon nanomaterials induce residue degradation and increase methane production from livestock manure in an anaerobic digestion system. J. Clean. Prod. 240, 118257. doi:10.1016/j.jclepro.2019.118257
Hassaneen, F. Y., Abdallah, M. S., Ahmed, N., Taha, M. M., Abd ElAziz, S. M. M., El-Mokhtar, M. A., et al. (2020). Innovative nanocomposite formulations for enhancing biogas and biofertilizers production from anaerobic digestion of organic waste. Bioresour. Technol. 309, 123350. doi:10.1016/j.biortech.2020.123350
Hassanein, A., Kumar, A. N., and Lansing, S. (2021). Impact of electro-conductive nanoparticles additives on anaerobic digestion performance-a review. Bioresour. Technol. 342, 126023. doi:10.1016/j.biortech.2021.126023
Hassanein, A., Lansing, S., and Tikekar, R. (2019). Impact of metal nanoparticles on biogas production from poultry litter. Bioresour. Technol. 275, 200–206. doi:10.1016/j.biortech.2018.12.048
Holm-Nielsen, J. B., Al Seadi, T., and Oleskowicz-Popiel, P. (2009). The future of anaerobic digestion and biogas utilization. Bioresour. Technol. 100 (22), 5478–5484. doi:10.1016/j.biortech.2008.12.046
Hou, J., Yang, Y., Wang, P., Wang, C., Miao, L., Wang, X., et al. (2017). Effects of CeO2, CuO, and ZnO nanoparticles on physiological features of Microcystis aeruginosa and the production and composition of extracellular polymeric substances. Environ. Sci. Pollut. Res. 24 (1), 226–235. doi:10.1007/s11356-016-7387-5
Huang, D., Li, T., Xu, P., Zeng, G., Chen, M., Lai, C., et al. (2019). Deciphering the Fenton-reaction-aid lignocellulose degradation pattern by Phanerochaete chrysosporium with ferroferric oxide nanomaterials: Enzyme secretion, straw humification and structural alteration. Bioresour. Technol. 276, 335–342. doi:10.1016/j.biortech.2019.01.013
Huang, H., Zheng, X., Yang, S., and Chen, Y. (2019). More than sulfidation: Roles of biogenic sulfide in attenuating the impacts of CuO nanoparticle on antibiotic resistance genes during sludge anaerobic digestion. Water Res. 158, 1–10. doi:10.1016/j.watres.2019.04.019
Huang, J., Cao, C., Liu, J., Yan, C., and Xiao, J. (2019). The response of nitrogen removal and related bacteria within constructed wetlands after long-term treating wastewater containing environmental concentrations of silver nanoparticles. Sci. Total Environ. 667, 522–531. doi:10.1016/j.scitotenv.2019.02.396
Hussein, A. K. (2015). Applications of nanotechnology in renewable energies—a comprehensive overview and understanding. Renew. Sustain. Energy Rev. 42, 460–476. doi:10.1016/j.rser.2014.10.027
Jadhav, P., Nasrullah, M., Zularisam, A., Bhuyar, P., Krishnan, S., and Mishra, P. (2021). Direct interspecies electron transfer performance through nanoparticles (NPs) for biogas production in the anaerobic digestion process. Int. J. Environ. Sci. Technol. (Tehran)., 1–13. doi:10.1007/s13762-021-03664-w
Jia, T., Wang, Z., Shan, H., Liu, Y., and Gong, L. (2017). Effect of nanoscale zero-valent iron on sludge anaerobic digestion. Resour. Conservation Recycl. 127, 190–195. doi:10.1016/j.resconrec.2017.09.007
Juntupally, S., Begum, S., Allu, S. K., Nakkasunchi, S., Madugula, M., and Anupoju, G. R. (2017). Relative evaluation of micronutrients (MN) and its respective nanoparticles (NPs) as additives for the enhanced methane generation. Bioresour. Technol. 238, 290–295. doi:10.1016/j.biortech.2017.04.049
Kavitha, S., Schikaran, M., Yukesh Kannah, R., Gunasekaran, M., Kumar, G., and Rajesh Banu, J. (2019). Nanoparticle induced biological disintegration: A new phase separated pretreatment strategy on microalgal biomass for profitable biomethane recovery. Bioresour. Technol. 289, 121624. doi:10.1016/j.biortech.2019.121624
Kelly, C. R., and Switzenbaum, M. S. (1984). Anaerobic treatment: Temperature and nutrient effects. Agric. Wastes 10 (2), 135–154. doi:10.1016/0141-4607(84)90012-X
Khalid, M. J., Zeshan, , Waqas, A., and Nawaz, I. (2019). Synergistic effect of alkaline pretreatment and magnetite nanoparticle application on biogas production from rice straw. Bioresour. Technol. 275, 288–296. doi:10.1016/j.biortech.2018.12.051
Khan, S. Z., Yuan, Y., Abdolvand, A., Schmidt, M., Crouse, P., Li, L., et al. (2009). Generation and characterization of NiO nanoparticles by continuous wave fiber laser ablation in liquid. J. Nanopart. Res. 11 (6), 1421–1427. doi:10.1007/s11051-008-9530-9
Kökdemir Ünşar, E., and Perendeci, N. A. (2018). What kind of effects do Fe2O3 and Al2O3 nanoparticles have on anaerobic digestion, inhibition or enhancement? Chemosphere 211, 726–735. doi:10.1016/j.chemosphere.2018.08.014
Kong, X., Wei, Y., Xu, S., Liu, J., Li, H., Liu, Y., et al. (2016). Inhibiting excessive acidification using zero-valent iron in anaerobic digestion of food waste at high organic load rates. Bioresour. Technol. 211, 65–71. doi:10.1016/j.biortech.2016.03.078
Kumar, S. S., Ghosh, P., Kataria, N., Kumar, D., Thakur, S., Pathania, D., et al. (2021). The role of conductive nanoparticles in anaerobic digestion: Mechanism, current status and future perspectives. Chemosphere 280, 130601. doi:10.1016/j.chemosphere.2021.130601
Li, H., Cui, F., Liu, Z., and Li, D. (2017). Transport, fate, and long-term impacts of metal oxide nanoparticles on the stability of an anaerobic methanogenic system with anaerobic granular sludge. Bioresour. Technol. 234, 448–455. doi:10.1016/j.biortech.2017.03.027
Li, L. L., Tong, Z. H., Fang, C. Y., Chu, J., and Yu, H. Q. (2015). Response of anaerobic granular sludge to single-wall carbon nanotube exposure. Water Res. 70, 1–8. doi:10.1016/j.watres.2014.11.042
Li, X., Yun, S., Zhang, C., Fang, W., Huang, X., and Du, T. (2018). Application of nano-scale transition metal carbides as accelerants in anaerobic digestion. Int. J. Hydrogen Energy 43, 1926–1936. doi:10.1016/j.ijhydene.2017.11.092
Lin, R., Cheng, J., Zhang, J., Zhou, J., Cen, K., and Murphy, J. D. (2017). Boosting biomethane yield and production rate with graphene: The potential of direct interspecies electron transfer in anaerobic digestion. Bioresour. Technol. 239, 345–352. doi:10.1016/j.biortech.2017.05.017
Lizama, A. C., Figueiras, C. C., Gaviria, L. A., Pedreguera, A. Z., and Ruiz Espinoza, J. E. (2019a). Nanoferrosonication: A novel strategy for intensifying the methanogenic process in sewage sludge. Bioresour. Technol. 276, 318–324. doi:10.1016/j.biortech.2019.01.021
Lizama, A. C., Figueiras, C. C., Pedreguera, A. Z., and Ruiz Espinoza, J. E. (2019b). Enhancing the performance and stability of the anaerobic digestion of sewage sludge by zero valent iron nanoparticles dosage. Bioresour. Technol. 275, 352–359. doi:10.1016/j.biortech.2018.12.086
Lo, H. M., Chiu, H. Y., Lo, S. W., and Lo, F. C. (2012). Effects of micro-nano and non micro-nano MSWI ashes addition on MSW anaerobic digestion. Bioresour. Technol. 114, 90–94. doi:10.1016/j.biortech.2012.03.002
Lönnqvist, T., Silveira, S., and Sanches-Pereira, A. (2013). Swedish resource potential from residues and energy crops to enhance biogas generation. Renew. Sustain. Energy Rev. 21, 298–314. doi:10.1016/j.rser.2012.12.024
Luna-delRisco, M., Orupõld, K., and Dubourguier, H.-C. (2011). Particle-size effect of CuO and ZnO on biogas and methane production during anaerobic digestion. J. Hazard. Mater. 189 (1–2), 603–608. doi:10.1016/j.jhazmat.2011.02.085
Lupitskyy, R., Alvarez-Fonseca, D., Herde, Z. D., and Satyavolu, J. (2018). In-situ prevention of hydrogen sulfide formation during anaerobic digestion using zinc oxide nanowires. J. Environ. Chem. Eng. 6 (1), 110–118. doi:10.1016/j.jece.2017.11.048
Ma, B., Wang, S., Li, Z., Gao, M., Li, S., Guo, L., et al. (2017). Magnetic Fe 3 O 4 nanoparticles induced effects on performance and microbial community of activated sludge from a sequencing batch reactor under long-term exposure. Bioresour. Technol. 225, 377–385. doi:10.1016/j.biortech.2016.11.130
Ma, J., Quan, X., Si, X., and Wu, Y. (2013). Responses of anaerobic granule and flocculent sludge to ceria nanoparticles and toxic mechanisms. Bioresour. Technol. 149, 346–352. doi:10.1016/j.biortech.2013.09.080
Malik, P., and Sangwan, A. (2012). Nanotechnology: A tool for improving efficiency of bio-energy. J. Eng. Comput. Appl. Sci. 1 (1), 37–49.
Mansour, M. S., Abdallah, M. S., Allam, N. K., Ibrahim, A. M., Khedr, A. M., Al-Bulqini, H. M., et al. (2020). Biogas production enhancement using nanocomposites and its combustion characteristics in a concentric flow slot burner. Exp. Therm. Fluid Sci. 113, 110014. doi:10.1016/j.expthermflusci.2019.110014
Mao, C., Feng, Y., Wang, X., and Ren, G. (2015). Review on research achievements of biogas from anaerobic digestion. Renew. Sustain. Energy Rev. 45, 540–555. doi:10.1016/j.rser.2015.02.032
Marsalek, B., Jancula, D., Marsalkova, E., Mashlan, M., Safarova, K., Tucek, J., et al. (2012). Multimodal action and selective toxicity of zerovalent iron nanoparticles against cyanobacteria. Environ. Sci. Technol. 46 (4), 2316–2323. doi:10.1021/es2031483
Mishra, P., Singh, L., Amirul Islam, M., Nasrullah, M., Mimi Sakinah, A. M., and Wahid, Z. A. (2019). NiO and CoO nanoparticles mediated biological hydrogen production: Effect of Ni/Co oxide NPs-ratio. Bioresour. Technol. Rep. 5, 364–368. doi:10.1016/j.biteb.2018.02.004
Mishra, P., Thakur, S., Mahapatra, D. M., Ab Wahid, Z., Liu, H., and Singh, L. (2018). Impacts of nano-metal oxides on hydrogen production in anaerobic digestion of palm oil mill effluent–A novel approach. Int. J. Hydrogen Energy 43 (5), 2666–2676. doi:10.1016/j.ijhydene.2017.12.108
Mu, H., and Chen, Y. (2011). Long-term effect of ZnO nanoparticles on waste activated sludge anaerobic digestion. Water Res. 45 (17), 5612–5620. doi:10.1016/j.watres.2011.08.022
Mu, H., Chen, Y., and Xiao, N. (2011). Effects of metal oxide nanoparticles (TiO2, Al2O3, SiO2 and ZnO) on waste activated sludge anaerobic digestion. Bioresour. Technol. 102 (22), 10305–10311. doi:10.1016/j.biortech.2011.08.100
Mu, H., Zheng, X., Chen, Y., Chen, H., and Liu, K. (2012). Response of anaerobic granular sludge to a shock load of zinc oxide nanoparticles during biological wastewater treatment. Environ. Sci. Technol. 46 (11), 5997–6003. doi:10.1021/es300616a
Mushtaq, K., Zaidi, A. A., and Askari, S. J. (2016). Design and performance analysis of floating dome type portable biogas plant for domestic use in Pakistan. Sustain. Energy Technol. Assessments 14, 21–25. doi:10.1016/j.seta.2016.01.001
Nguyen, D., Visvanathan, C., Jacob, P., and Jegatheesan, V. (2015). Effects of nano cerium (IV) oxide and zinc oxide particles on biogas production. Int. Biodeterior. Biodegrad. 102, 165–171. doi:10.1016/j.ibiod.2015.02.014
Noonari, A. A., Mahar, R. B., Sahito, A. R., and Brohi, K. M. (2019). Anaerobic co-digestion of canola straw and banana plant wastes with buffalo dung: Effect of Fe3O4 nanoparticles on methane yield. Renew. Energy 133, 1046–1054. doi:10.1016/j.renene.2018.10.113
Nyberg, L., Turco, R. F., and Nies, L. (2008). Assessing the impact of nanomaterials on anaerobic microbial communities. Environ. Sci. Technol. 42 (6), 1938–1943. doi:10.1021/es072018g
Otero-González, L., Field, J. A., and Sierra-Alvarez, R. (2014a). Fate and long-term inhibitory impact of ZnO nanoparticles during high-rate anaerobic wastewater treatment. J. Environ. Manag. 135, 110–117. doi:10.1016/j.jenvman.2014.01.025
Otero-González, L., Field, J. A., and Sierra-Alvarez, R. (2014b). Inhibition of anaerobic wastewater treatment after long-term exposure to low levels of CuO nanoparticles. Water Res. 58, 160–168. doi:10.1016/j.watres.2014.03.067
Palaniappan, K. (2017). An overview of applications of nanotechnology in biofuel production. World Appl. Sci. J. 35 (8), 1305–1311. doi:10.5829/idosi.wasj.2017.1305.1311
Pan, F., Xu, A., Xia, D., Yu, Y., Chen, G., Meyer, M., et al. (2015). Effects of octahedral molecular sieve on treatment performance, microbial metabolism, and microbial community in expanded granular sludge bed reactor. Water Res. 87, 127–136. doi:10.1016/j.watres.2015.09.022
Park, J.-I., Lee, J., Sim, S. J., and Lee, J.-H. (2009). Production of hydrogen from marine macro-algae biomass using anaerobic sewage sludge microflora. Biotechnol. Bioprocess Eng. 14 (3), 307–315. doi:10.1007/s12257-008-0241-y
Qiang, H., Niu, Q., Chi, Y., and Li, Y. (2013). Trace metals requirements for continuous thermophilic methane fermentation of high-solid food waste. Chem. Eng. J. 222, 330–336. doi:10.1016/j.cej.2013.02.076
Rahman, K. M., Melville, L., Huq, S. M. I., and Khoda, S. K. (2016). Understanding bioenergy production and optimisation at the nanoscale – A review. J. Exp. Nanosci. 11 (10), 762–775. doi:10.1080/17458080.2016.1157905
Rao, C. N. R., Kulkarni, G. U., Thomas, P. J., and Edwards, P. P. (2001). Size-dependent chemistry: Properties of nanocrystals. Chem. Eur. J. 8 (1), 28–35. doi:10.1002/1521-3765(20020104)8:1<28::AID-CHEM28>3.0.CO;2-B
RENA (2021). Renewable energy statistics 2021. Abu Dhabi: The International Renewable Energy Agency.
Sarker, N. C., Rahman, S., Borhan, M. S., Rajasekaran, P., Santra, S., and Ozcan, A. (2019). Nanoparticles in mitigating gaseous emissions from liquid dairy manure stored under anaerobic condition. J. Environ. Sci. 76, 26–36. doi:10.1016/j.jes.2018.03.014
Satyanarayana, K. G., Mariano, A. B., and Vargas, J. V. C. (2011). A review on microalgae, a versatile source for sustainable energy and materials. Int. J. Energy Res. 35 (4), 291–311. doi:10.1002/er.1695
Seadi, T. A., Rutz, D., Prassl, H., Köttner, M., Finsterwalder, T., Volk, S., et al. (2008). Biogas handbook. In Igarss 2014. Sawston: Woodhead Publishing. doi:10.1533/9780857097415.1.85
Shi, Y., Huang, K., Feng, R., Wang, R., Liu, G., Zaidi, A. A., et al. (2020). “Combined MgO nanoparticle and microwave pre-treatment on biogas increase from Enteromorpha during anaerobic digestion,”. IOP Conference Series: Earth and Environmental Science, Volume 450 in 2nd International Conference on Air Pollution and Environmental Engineering, Xi'an, China, 15-16 December 2019, 012025.
Su, L., Shi, X., Guo, G., Zhao, A., and Zhao, Y. (2013). Stabilization of sewage sludge in the presence of nanoscale zero-valent iron (nZVI): Abatement of odor and improvement of biogas production. J. Mat. Cycles Waste Manag. 15 (4), 461–468. doi:10.1007/s10163-013-0150-9
Su, L., Zhen, G., Zhang, L., Zhao, Y., Niu, D., and Chai, X. (2015). The use of the core–shell structure of zero-valent iron nanoparticles (NZVI) for long-term removal of sulphide in sludge during anaerobic digestion. Environ. Sci. Process. Impacts 17 (12), 2013–2021. doi:10.1039/C5EM00470E
Suanon, F., Sun, Q., Li, M., Cai, X., Zhang, Y., Yan, Y., et al. (2017). Application of nanoscale zero valent iron and iron powder during sludge anaerobic digestion: Impact on methane yield and pharmaceutical and personal care products degradation. J. Hazard. Mater. 321, 47–53. doi:10.1016/j.jhazmat.2016.08.076
Suanon, F., Sun, Q., Mama, D., Li, J., Dimon, B., and Yu, C. P. (2016). Effect of nanoscale zero-valent iron and magnetite (Fe3O4) on the fate of metals during anaerobic digestion of sludge. Water Res. 88, 897–903. doi:10.1016/j.watres.2015.11.014
Temizel, İ., Emadian, S. M., Di Addario, M., Onay, T. T., Demirel, B., Copty, N. K., et al. (2017). Effect of nano-ZnO on biogas generation from simulated landfills. Waste Manag. 63, 18–26. doi:10.1016/j.wasman.2017.01.017
Tian, T., Qiao, S., Li, X., Zhang, M., and Zhou, J. (2017). Nano-graphene induced positive effects on methanogenesis in anaerobic digestion. Bioresour. Technol. 224, 41–47. doi:10.1016/j.biortech.2016.10.058
Tsapekos, P., Alvarado-Morales, M., Tong, J., and Angelidaki, I. (2018). Nickel spiking to improve the methane yield of sewage sludge. Bioresour. Technol. 270, 732–737. doi:10.1016/j.biortech.2018.09.136
Ünşar, E. K., Çığgın, A. S., Erdem, A., and Perendeci, N. A. (2016). Long and short term impacts of CuO, Ag and CeO 2 nanoparticles on anaerobic digestion of municipal waste activated sludge. Environ. Sci. Process. Impacts 18 (2), 277–288. doi:10.1039/C5EM00466G
Vasco-Correa, J., Khanal, S., Manandhar, A., and Shah, A. (2018). Anaerobic digestion for bioenergy production: Global status, environmental and techno-economic implications, and government policies. Bioresour. Technol. 247, 1015–1026. doi:10.1016/j.biortech.2017.09.004
Wang, T., Zhang, D., Dai, L., Chen, Y., and Dai, X. (2016). Effects of metal nanoparticles on methane production from waste-activated sludge and microorganism community shift in anaerobic granular sludge. Sci. Rep. 6 (1), 25857. doi:10.1038/srep25857
Wu, D., Peng, X., Li, L., Yang, P., Peng, Y., Liu, H., et al. (2021). Commercial biogas plants: Review on operational parameters and guide for performance optimization. Fuel 303, 121282. doi:10.1016/j.fuel.2021.121282
Xiang, Y., Yang, Z., Zhang, Y., Xu, R., Zheng, Y., Hu, J., et al. (2019). Influence of nanoscale zero-valent iron and magnetite nanoparticles on anaerobic digestion performance and macrolide, aminoglycoside, β-lactam resistance genes reduction. Bioresour. Technol. 294, 122139. doi:10.1016/j.biortech.2019.122139
Yadav, T., Mungray, A. A., and Mungray, A. K. (2016). Effect of multiwalled carbon nanotubes on UASB microbial consortium. Environ. Sci. Pollut. Res. 23 (5), 4063–4072. doi:10.1007/s11356-015-4385-y
Yadav, T., Mungray, A. A., and Mungray, A. K. (2017). Effect of TiO 2 nanoparticles on UASB biomass activity and dewatered sludge. Environ. Technol. 38 (4), 413–423. doi:10.1080/09593330.2016.1196738
Yan, W., Shen, N., Xiao, Y., Chen, Y., Sun, F., Kumar Tyagi, V., et al. (2017). The role of conductive materials in the start-up period of thermophilic anaerobic system. Bioresour. Technol. 239, 336–344. doi:10.1016/j.biortech.2017.05.046
Yang, Y., Guo, J., and Hu, Z. (2013a). Impact of nano zero valent iron (NZVI) on methanogenic activity and population dynamics in anaerobic digestion. Water Res. 47 (17), 6790–6800. doi:10.1016/j.watres.2013.09.012
Yang, Y., Zhang, C., and Hu, Z. (2013b). Impact of metallic and metal oxide nanoparticles on wastewater treatment and anaerobic digestion. Environ. Sci. Process. Impacts 15 (1), 39–48. doi:10.1039/C2EM30655G
Yazdani, M., Ebrahimi-Nik, M., Heidari, A., and Abbaspour-Fard, M. H. (2019). Improvement of biogas production from slaughterhouse wastewater using biosynthesized iron nanoparticles from water treatment sludge. Renew. Energy 135, 496–501. doi:10.1016/j.renene.2018.12.019
Zaidi, A. A., Feng, R., Malik, A., Khan, S. Z., Shi, Y., Bhutta, A. J., et al. (2019a). Combining microwave pretreatment with iron oxide nanoparticles enhanced biogas and hydrogen yield from green algae. Processes 7, 24. doi:10.3390/pr7010024
Zaidi, A. A., Khan, S. Z., Almohamadi, H., Mahmoud, E. R., and Naseer, M. N. (2021a). Nanoparticles synergistic effect with various substrate pretreatment and their comparison on biogas production from algae waste. Bull. Chem. React. Eng. Catal. 16, 374–382. doi:10.9767/bcrec.16.2.10637.374-382
Zaidi, A. A., Khan, S. Z., and Shi, Y. (2021b). Optimization of nickel nanoparticles concentration for biogas enhancement from green algae anaerobic digestion. Mater. Today Proc. 39, 1025–1028. doi:10.1016/j.matpr.2020.04.762
Zaidi, A. A., Ruizhe, F., Malik, A., Khan, S. Z., Bhutta, A. J., Shi, Y., et al. (2019b). Conjoint effect of microwave irradiation and metal nanoparticles on biogas augmentation from anaerobic digestion of green algae. Int. J. Hydrogen Energy 44, 14661–14670. doi:10.1016/j.ijhydene.2019.02.245
Zaidi, A. A., RuiZhe, F., Shi, Y., Khan, S. Z., and Mushtaq, K. (2018). Nanoparticles augmentation on biogas yield from microalgal biomass anaerobic digestion. Int. J. Hydrogen Energy 43 (31), 14202–14213. doi:10.1016/j.ijhydene.2018.05.132
Zhang, J., and Lu, Y. (2016). Conductive Fe3O4 nanoparticles accelerate syntrophic methane production from butyrate oxidation in two different lake sediments. Front. Microbiol. 7 (AUG), 1316–1319. doi:10.3389/fmicb.2016.01316
Zhang, J., Wang, Z., Lu, T., Liu, J., Wang, Y., Shen, P., et al. (2019c). Response and mechanisms of the performance and fate of antibiotic resistance genes to nano-magnetite during anaerobic digestion of swine manure. J. Hazard. Mater. 366, 192–201. doi:10.1016/j.jhazmat.2018.11.106
Zhang, L., He, X., Zhang, Z., Cang, D., Nwe, K. A., Zheng, L., et al. (2017). Evaluating the influences of ZnO engineering nanomaterials on VFA accumulation in sludge anaerobic digestion. Biochem. Eng. J. 125, 206–211. doi:10.1016/j.bej.2017.05.008
Zhang, L., Zhang, Z., He, X., Zheng, L., Cheng, S., and Li, Z. (2019b). Diminished inhibitory impact of ZnO nanoparticles on anaerobic fermentation by the presence of TiO2 nanoparticles: Phenomenon and mechanism. Sci. Total Environ. 647, 313–322. doi:10.1016/j.scitotenv.2018.07.468
Zhang, T., Yun, S., Li, X., Huang, X., Hou, Y., Liu, Y., et al. (2017). Fabrication of niobium-based oxides/oxynitrides/nitrides and their applications in dye-sensitized solar cells and anaerobic digestion. J. Power Sources 340, 325–336. doi:10.1016/j.jpowsour.2016.11.082
Zhang, Y., Yang, Z., Xu, R., Xiang, Y., Jia, M., Hu, J., et al. (2019a). Enhanced mesophilic anaerobic digestion of waste sludge with the iron nanoparticles addition and kinetic analysis. Sci. Total Environ. 683, 124–133. doi:10.1016/j.scitotenv.2019.05.214
Zhang, Z., Gao, P., Cheng, J., Liu, G., Zhang, X., and Feng, Y. (2018). Enhancing anaerobic digestion and methane production of tetracycline wastewater in EGSB reactor with GAC/NZVI mediator. Water Res. 136, 54–63. doi:10.1016/j.watres.2018.02.025
Zhang, Z., O’Hara, I. M., Mundree, S., Gao, B., Ball, A. S., Zhu, N., et al. (2016). Biofuels from food processing wastes. Curr. Opin. Biotechnol. 38, 97–105. doi:10.1016/j.copbio.2016.01.010
Zhao, L., Ji, Y., Sun, P., Deng, J., Wang, H., and Yang, Y. (2019). Effects of individual and combined zinc oxide nanoparticle, norfloxacin, and sulfamethazine contamination on sludge anaerobic digestion. Bioresour. Technol. 273, 454–461. doi:10.1016/j.biortech.2018.11.049
Zhao, L., Ji, Y., Sun, P., Li, R., Xiang, F., Wang, H., et al. (2018). Effects of individual and complex ciprofloxacin, fullerene C60, and ZnO nanoparticles on sludge digestion: Methane production, metabolism, and microbial community. Bioresour. Technol. 267, 46–53. doi:10.1016/j.biortech.2018.07.024
Zhao, Z., Li, Y., Yu, Q., and Zhang, Y. (2018a). Ferroferric oxide triggered possible direct interspecies electron transfer between Syntrophomonas and Methanosaeta to enhance waste activated sludge anaerobic digestion. Bioresour. Technol. 250, 79–85. doi:10.1016/j.biortech.2017.11.003
Zhao, Z., Zhang, Y., Li, Y., Quan, X., and Zhao, Z. (2018b). Comparing the mechanisms of ZVI and Fe3O4 for promoting waste-activated sludge digestion. Water Res. 144, 126–133. doi:10.1016/j.watres.2018.07.028
Zheng, X., Wu, L., Chen, Y., Su, Y., Wan, R., Liu, K., et al. (2015). Effects of titanium dioxide and zinc oxide nanoparticles on methane production from anaerobic co-digestion of primary and excess sludge. J. Environ. Sci. Health. A Tox. Hazard. Subst. Environ. Eng. 50 (9), 913–921. doi:10.1080/10934529.2015.1030279
Keywords: anaerobic fermentation, biogas, nanotechnology, nanoparticles (NPS), waste, biomass, biohydrogen, nanomaterial
Citation: Khan SZ, Zaidi AA, Naseer MN and AlMohamadi H (2022) Nanomaterials for biogas augmentation towards renewable and sustainable energy production: A critical review. Front. Bioeng. Biotechnol. 10:868454. doi: 10.3389/fbioe.2022.868454
Received: 02 February 2022; Accepted: 26 July 2022;
Published: 02 September 2022.
Edited by:
Caixia Wan, University of Missouri, United StatesReviewed by:
Raffaella Villa, De Montfort University, United KingdomLakhveer Singh, SRM University, India
Copyright © 2022 Khan, Zaidi, Naseer and AlMohamadi. This is an open-access article distributed under the terms of the Creative Commons Attribution License (CC BY). The use, distribution or reproduction in other forums is permitted, provided the original author(s) and the copyright owner(s) are credited and that the original publication in this journal is cited, in accordance with accepted academic practice. No use, distribution or reproduction is permitted which does not comply with these terms.
*Correspondence: Sohaib Z. Khan, szkhan@iu.edu.sa