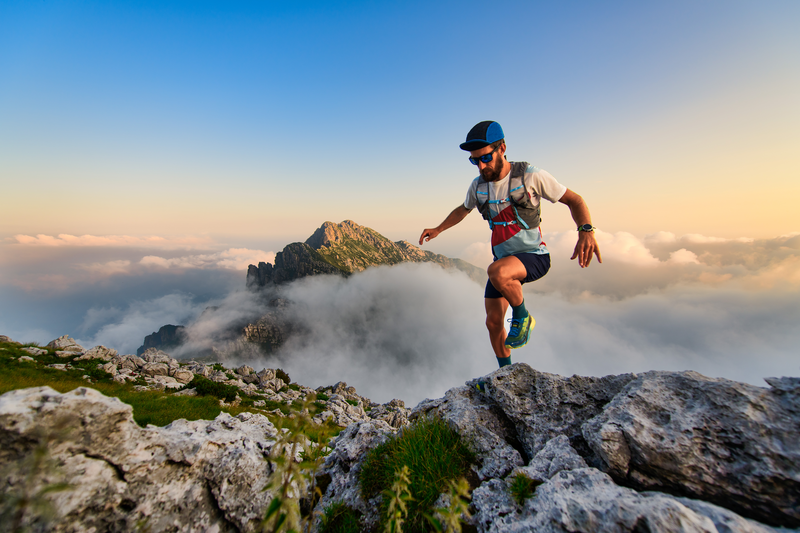
95% of researchers rate our articles as excellent or good
Learn more about the work of our research integrity team to safeguard the quality of each article we publish.
Find out more
ORIGINAL RESEARCH article
Front. Bioeng. Biotechnol. , 12 April 2022
Sec. Bioprocess Engineering
Volume 10 - 2022 | https://doi.org/10.3389/fbioe.2022.866419
This article is part of the Research Topic Value-Added Products from Agro-Industrial Residues by Biological Approaches View all 14 articles
Plant health is the fundamental of agricultural production, which is threatened by plant pathogens severely. The previous studies exhibited the effects of different pathogen control strategies (physical, chemical, and microbial methods), which resulted from bringing in exogenous additives, on microbial community structures and functions. Nevertheless, few studies focused on the potential inhibitory abilities of native microbial community in the soil, which could be activated or enhanced by different fertilization strategies. In this study, three plant diseases (TMV, TBS, and TBW) of tobacco, fungal community of tobacco rhizosphere soil, and the correlation between them were researched. The results showed that nitrogen-reducing fertilization strategies could significantly decrease the occurrence rate and the disease index of three tobacco diseases. The results of bioinformatics analyses revealed that the fungal communities of different treatments could differentiate the nitrogen-reducing fertilization group and the control group (CK). Furthermore, key genera which were responsible for the variation of fungal community were explored by LEfSe analysis. For instance, Tausonia and Trichocladium increased, while Naganishia and Fusicolla decreased under nitrogen-reducing fertilization conditions. Additionally, the correlation between tobacco diseases and key genera was verified using the Mantel test. Moreover, the causal relationship between key genera and tobacco diseases was deeply explored by PLS–PM analysis. These findings provide a theoretical basis for a nitrogen-reducing fertilization strategy against tobacco diseases without exogenous additives and make contributions to revealing the microbial mechanism of native-valued fungal key taxa against tobacco diseases, which could be stimulated by agricultural fertilization management.
Plant diseases can devastate agriculture by reducing crop yields and causing severe economic losses (Strange and Scott, 2005). Plant diseases caused by soilborne pathogens can diminish the yields of vegetables, fruits, and other economically important crops by up to 20% (Jaiswal et al., 2018). The perniciousness of plant diseases has risen significantly due to current agricultural practices including intensive cultivation, overuse of fertilizers, utilization of high-yield but pathogen-susceptible cultivars, and consecutive years of continuous cropping (Chakraborty and Newton, 2011; Ghorbanpour et al., 2018). Thus, disease management strategies targeting plant pathogens are of vital importance (Peng et al., 2017).
Much effort has been expended in exploring disease management strategies under various circumstances. Physical methods such as heating and solarizing the soil are laborious, and they can hardly eradicate pathogens (De Carvalho Pontes et al., 2019). Chemical methods such as pesticides are expensive, they can poison the environment, and they can result in pesticide-resistant bacterial populations (Morais et al., 2019) that may become ineffective in the face of the bacterial genetic variability (Kim et al., 2016). Crop rotation is a slow method for reducing pathogen density in soil, making it unsuitable when severe disease breakout (Chellemi et al., 2016). Biocontrol bacteria are receiving increasing attention as a potential alternative to traditional methods for controlling plant diseases (Choi et al., 2020; Ogunnaike et al., 2021; Ahmed et al., 2022), based on being harmless, effective, and sustainable (Xie et al., 2020). Previous studies have identified various biocontrol agents including Bacillus spp. (Cao et al., 2011), Paenibacillus polymyxa (Chunyu et al., 2017), Streptomyces spp. (Abo-Zaid et al., 2020), and Pseudomonas fluorescens (Huang et al., 2020). Additionally, a non-native arbuscular mycorrhizal fungal inoculant has been reported to improve the growth and enhance the resistance system of multiple plants (Pellegrino et al., 2012; Aliyu et al., 2019; Yang L et al., 2021).
Previous research corresponding to physical, chemical, and microbial strategies against plant diseases discovered that most of the strategies could convert the microbial community of the rhizosphere soil of plants into an enhanced status of pathogen resistance (Hu et al., 2020; Liu et al., 2020). According to the principle of low cost and environmental friendliness, strategies without exogenous additives received more and more attention. Evidence had demonstrated that nitrogen-reducing fertilization could change the microbial community of planting soils (Zhou et al., 2020; Yang T et al., 2021). As a no-additive strategy, the inhibitory effect of nitrogen-reducing fertilization against tobacco diseases was little researched.
In this study, a nitrogen-reducing fertilization strategy was used in the process of tobacco planting. The occurrence rate and disease index of tobacco diseases, including tobacco mosaic virus (TMV), tobacco black shank (TBS), and tobacco bacterial wilt (TBW), were investigated. The fungal community of tobacco rhizosphere soil differentiated the nitrogen-reducing treatments from the control group (CK). The key taxa which contributed to the variation of fungal community due to nitrogen-reducing fertilization were analyzed and identified. Moreover, the dominant role played by the key taxa to help inhibit tobacco diseases was verified using the Mantel test. This study contributed to reveal the conversion of the fungal community against tobacco pathogens, which provided a feasible, economic, and eco-friendly fertilization strategy in the tobacco-planting industry.
This experiment was conducted in Huangdao district, Qingdao, Shandong Province (36°00′44.86″ N, 119°51′35.30″ E), China, from April 10 to 15 October 2020. The average annual rainfall of the experimental field was 696.6 mm, the annual sunshine duration was 2,110.1 h, and the annual average temperature was 12.5 C. The previous crop in the experiment site was tobacco (Zhongyan 100 variety), which had been planted for four consecutive years. The flue-cured tobacco variety used in this experiment was Zhongyan 100. The fertilization procedure in this experiment was carried out according to the fertilization strategies (Supplementary Table S1). The tobacco was transplanted at May 5, and tobacco diseases were investigated on July 23, according to the grade and investigation method of tobacco diseases and insect pests (Tobacco Research Institute, 2008).
Rhizosphere soil is the soil that lies much closer to the plant roots. The samples of tobacco rhizosphere soil were collected using a sterilized brush and by gentle shaking (Pétriacq et al., 2017). Three replicates were prepared for each treatment. The soil samples were immediately stored in a −20°C refrigerator for subsequent extraction of soil DNA.
About 500 mg soil sample was collected from well-mixed rhizosphere soil from each replication. DNA was extracted from the soil using a DNA extraction kit (FastDNATM SPIN Kit for soil, MP Biomedicals, LLC, Solon, OH, United States) according to the manufacturer’s instructions. Subsequently, DNA was tested with 1% agarose gel, and the successfully extracted DNA was stored at −20°C immediately.
The PCR of the ITS1 region of the fungal ITS gene sequencing was conducted using the specific primers CTTGGTCATTTAGAGGAAGTAA and GCTGCGTTCTTCATCGATGC. The amplification products were tested for specificity in 1% agarose gel (Yuan et al., 2020). Then, the library was constructed using the library construction kit TruSeq® DNA PCR-Free Sample Preparation kit (Illumina, San Diego, CA, United States). After the library was successfully constructed, the quantitative process was implemented using the Qubit® 2.0 Fluorometer (Life Technologies, Carlsbad, CA, United States) and qPCR. After the quantitative test, subsequent sequencing was performed on the Illumina MiSeq platform.
The raw data after sequencing were assembled and attached by FLASH (V1.2.11, https://ccb.jhu.edu/software/FLASH/index.shtml) to obtain raw tags. Subsequently, QIIME software (V1.9.1, http://qiime.org/scripts/split_libraries_fastq.html) was used to filter raw tags. Then, the UCHIME algorithm (http://www.drive5.com/usearch/manual/uchime_algo.html) (Haas et al., 2011) was used to detect and remove chimeras against the Unite database (https://unite.ut.ee/) (Edgar et al., 2011) and get effective data (effective tags) (Callahan et al., 2016).
The valid data from all samples were clustered into the same operational taxonomic units (OTUs), and the OTUs with the highest frequency were selected as the representative of OTU sequences. The ITS database Unite (https://unite.ut.ee/) for species annotation analysis was used subsequently (Kõljalg et al., 2013). Finally, all OTUs were uniformized based on the smallest amount of sequencing data as the standard.
Afterward, the normalization process was followed by analyses performed based on the OTU statistics. Qiime software (version 1.9.1, http://qiime.org/install/index.html) was used to calculate Shannon, Simpson, Ace, Chao1, and PD whole tree indexes, and R software (version 3.6.0) was used to draw a rarefaction curve. Then, the Bray–Curtis distance was calculated by Qiime software (version 1.9.1), and the WGCNA, stats, and ggplot2 packages of R software (version 3.6.0) were used to draw PCA, PCoA, NMDS, and PLS-DA plots. The vegan package based on R software (version 3.6.0) was used to test the differences in the microbial community structure among different treatments through PERMANOVA. Subsequently, the significance P-value in the LEfSe analysis was conducted using the Wilcoxon rank-sum test. The LDA was set to 3.0. The correlation between fungal data and tobacco disease data was calculated, according to the Mantel test (Li Y. et al., 2021). Based on the analysis platform of Majorbio (Shanghai Majorbio Bio-pharm Technology Co., Ltd., Shanghai, China), the results of species annotations were used for further comparative analyses. The raw sequence data were uploaded to the Sequence Read Archive (accession number: PRJNA808161), which could be freely available on the NCBI (https://www.ncbi.nlm.nih.gov/bioproject/PRJNA808161).
The tobacco diseases data were analyzed by SPSS (version 25.0), and the single-factor ANOVA (Duncan’s multiple range test) in SPSS software was used to calculate the significance of the differences among nitrogen-reducing fertilization and CK. All figures were drawn through Origin (version 2018) (OriginLab Corporation, Northampton, MA, United States) and R software (version 3.6.0). All results were presented as mean ± standard deviation.
Regarded as the main plant diseases of tobacco, tobacco mosaic virus (TMV), tobacco black shank (TBS), and tobacco bacterial wilt (TBW) were investigated. The disease occurrence rates and disease indexes of the three diseases were calculated among all treatments (Table 1). The results demonstrated that nitrogen-reducing fertilization could decrease the occurrence rate and the disease index of tobacco diseases. In particular, all three nitrogen-reducing fertilization strategies (RNTe, RNTw, and RNTh) reduced the occurrence rate of TMV and TBS significantly (p < 0.05), compared to CK. Although the inhibitory effects of nitrogen-reducing fertilization strategies were weakened when they were faced by TBW, the difference of the disease occurrence rate between RNTe and CK reached the significant level (p < 0.05). Furthermore, the tendency of the disease index of these three tobacco diseases was consistent with the principle of their occurrence rates, except that there were no significant differences between RNTh and CK in the disease index of TBS.
To explore the microbial mechanism of the inhibitory effect of nitrogen-reducing fertilization strategies on tobacco diseases, the fungal community of different treatments was further researched. The composition of the fungal community was influenced by nitrogen-reducing fertilization (Figure 1 and Supplementary Figure S1). The lowest rarefaction curve occurred in RNTe, which represented that the richness of fungal community was smallest in RNTe, compared to other treatments (Figure 1A). The Venn diagram indicated that 754, 655, 752, and 737 OTUs were observed in CK, RNTe, RNTw, and RNTh, respectively. Among them, all treatments shared 414 common OTUs, whereas CK, RNTe, RNTw, and RNTh owned 90, 31, 63, and 50 unique OTUs, respectively (Figure 1B). According to the results of annotation, Ascomycota, Basidiomycota, and Mortierellomycota were the dominant fungi based on the phylum level. Due to nitrogen-reducing fertilization, the relative abundance of Ascomycota was decreased, whereas the relative abundance of Basidiomycota was improved. Additionally, the relative abundance of Mortierellomycota was up and down among different treatments, without an apparent discipline (Figure 1C). Corresponding to the genus level, the relative abundance of Tausonia was increased by nitrogen-reducing fertilization, while that of more genera (such as Fusarium and Aspergillus) was declined (Figure 1D).
FIGURE 1. Composition of fungal communities in different treatments, based on OTU level. (A) Rarefaction curves of different treatments. (B) Venn diagram of different treatments, based on the number of OTUs. (C) Main taxa of fungi in different treatments at the phylum level. (D) Main taxa of fungi in different treatments at the genus level.
Based on the heat map analysis, compared with the control, nitrogen reduction treatment increased the abundance of some genera and decreased the abundance of other genera (Figure 2A). In particular, nitrogen reduction treatment showed a strong trend of decreasing the relative abundance of genera for an increase in the relative abundance of certain bacterial genera. Based on the ternary analysis, the distribution of the main dominant bacterial genera (based on relative abundance) was relatively uniform among different nitrogen reduction treatments (Figure 2B). It demonstrated the common effect of different nitrogen reduction treatments on soil fungal communities. In addition, compared with RNTh, more species of the genera were enriched in RNTe and RNTw treatment, especially RNTw.
FIGURE 2. Composition of fungal communities in different treatments, based on the genus level. (A) Heat map of top 40 genera of different treatments. (B) Ternary analysis of different treatments, based on the genus level.
In different analyses, the fungal communities of the CK treatment were clustered into a unique area, revealing that the fungal community structure of the nitrogen-reducing treatment was significantly different from that of CK (Figure 3). In addition, the fungal communities of RNTw and RNTh clustered into a much closer location, which indicated that the community structure of the two was more similar.
FIGURE 3. Combined plots of PCA (A), PCoA (B), NMDS (C), and PLS-DA (D) of fungal communities in different treatments.
Furthermore, the LEfSe analysis of different treatments revealed that biomarkers of different treatments were identified as 4 phyla and 64 genera, among which CK, RNTe, RNTw, and RNTh had 17, 8, 16, and 23 genera, respectively (Figures 4A,B). Based on the relative abundance, the top 20 genera with significant differences among different treatments were further analyzed, revealing the key genera that caused the changes in the fungal community structure in the nitrogen reduction treatment, compared to CK (Figure 4C). Specifically, the relative abundance of Tausonia, Trichocladium, unclassified Didymellaceae, Gymnoascus, Humicola, and unclassified Sordariaceae was significantly (p < 0.05) enhanced by nitrogen-reducing fertilization, whereas the relative abundance of Chaetomium, Naganishia, Trichoderma, Fusicolla, Paraphaeosphaeria, Albifimbria, Cladophialophora, and unclassified GS13 was significantly declined by nitrogen-reducing fertilization (p < 0.05).
FIGURE 4. Combined plots of exploring key taxa among different treatments. (A) LDA effect size (LEfSe) analysis based on the genus among different treatments. (B) Cladogram of biomarkers among different treatments. (C) Bar plot of top 20 significant differential taxa based on the Kruskal–Wallis H test.
Furthermore, the fungal community functions of different treatments were predicted (Figure 5). In this study, different types of saprophytic functions are the dominant functions of fungal communities in different treatments. Compared with the control, nitrogen reduction treatment reduced the pathological nutritional functions of animals and plants and enhanced their saprophytic nutritional functions. In addition, nitrogen reduction treatments significantly enhanced unknown functions of the fungal community. The dominant effect of nitrogen reduction treatment in the process of tobacco growing is not only derived from its changes in common functions but also related to its activation of new and unknown functions.
To verify the microbial mechanism of the inhibitory effect against tobacco diseases caused by nitrogen-reducing fertilization, the Mantel test was implemented between key taxa and the results of tobacco diseases (Table 2). The results of the Mantel test indicated that the key taxa, whose relative abundances were significantly (p < 0.05) different from CK, had strong correlations to the occurrence rates and disease indexes of tobacco diseases among different treatments. Interestingly, the correlations between fungal key taxa were much stronger in inhibition against TMV and TBS with the R values (occurrence rate) of 0.7733 and 0.6927, and the R values (disease index) of 0.7194 and 0.7197, rather than in the inhibition against TBW.
To deeply pursue the causal relationship between key genera and those three tobacco diseases, partial least squares path modeling (PLS-PM) was employed to analyze the paths among all the modules (Figure 6). Five modules, the key genera whose relative abundances were significantly increased by nitrogen-reducing fertilization (InKG), the key genera whose relative abundances were significantly decreased by nitrogen-reducing fertilization (DeKG), TMV, TBS, and TBW, were set to establish an analysis model. The goodness of fit (Gof) was 0.7481, which meant the prediction power of the model was of 74.81%. The results of path coefficients showed that InKG had a negative causal relationship with TMV and TBS and had a positive causal relationship with TBW, whereas DeKG had a positive causal relationship with those three tobacco diseases. However, among these path coefficients, the causal relationships between InKG, TMV, and TBW were not significant (p < 0.05). Additionally, the causal relationship between DeKG and TBW did not reach a significant (p < 0.05) level neither. Furthermore, the R2 values of TMV, TBS, and TBW, which represented the amount of variance of the three diseases explained by this model, were 0.9158, 0.9101, and 0.7198, respectively.
FIGURE 6. PLS-PM diagram based on the path coefficients between different modules. Blue and red arrow lines represent the positive and negative causal relationship between each two modules, respectively. The lines composed of dash lines means the calculated result of the relationship between the two modules did not reach the significant (p < 0.05) level. InKG: the key genera whose relative abundances were significantly increased by nitrogen-reducing fertilization. DeKG: the key genera whose relative abundances were significantly decreased by nitrogen-reducing fertilization. Gof: goodness of fit. R2 indicated the amount of variance in the endogenous latent variable explained by its independent latent variables.
Plant health is the fundamental of agricultural production, which is threatened by plant pathogens severely. Regarded as one of the most popular commodities, merchandise corresponding to tobacco plays an important role in undertaking the national economic task. However, the tobacco-planting industry is hindered by plant diseases such as tobacco mosaic virus, tobacco black shrank, and tobacco bacterial wilt (Hu Q. et al., 2021; Hu Y. et al., 2021). Chemicals, chemical pesticide, soil fumigation, and microbial inoculants consist of the mainstream pathogen control strategies that have earned great achievements fighting against plant diseases (Alori and Babalola, 2018; Ren et al., 2018; Raymaekers et al., 2020; Long et al., 2021). The previous studies exhibited the effects of different pathogen control strategies, which resulted from exogenous additives, on microbial community structures and functions (Preininger et al., 2018; Li et al., 2020; Vassilev et al., 2020). Nevertheless, without additives, the soil microbial structure could be changed according to different amounts of fertilizers (Li B.-B. et al., 2021). It would be of great help to the agricultural management which could prevent cash crops from plant diseases using a fertilization strategy.
In this study, nitrogen-reducing fertilization strategies showed an obvious inhibitory effect against tobacco diseases, especially against TMV and TBS. Through the composition and structure of fungal communities between nitrogen-reducing fertilization and CK, the variation of fungal communities was explored to exhibit the effects of nitrogen-reducing fertilization on converting the microbial community into an inhibition-enhanced status. Previous studies had indicated that the appropriate reduction of chemical fertilizers, especially nitrogen fertilizer, could improve the yield of crops and impact the microbial community in rhizosphere soil of plants. Some research studies paid attention to the effects of the nitrogen-reducing strategy on fungal community of food crops such as wheat, rice, and maize (Wen et al., 2020; Liu et al., 2021; Wang et al., 2021). Wang et al. found that Humicola, Tausonia, and Codinaea could improve the chemical quality of tobacco (Wang et al., 2022). Nevertheless, few had focused on the fungal inhibitory effect of nitrogen-reducing fertilization on tobacco diseases.
Interestingly, the bioinformatics of the fungal community of all treatments were found to be closely correlated to the results of tobacco diseases. Based on LEfSe analysis and Kruskal–Wallis H test, the key taxa that dominated the variation of fungal communities between nitrogen-reducing fertilization and CK were excavated and summarized. Previous evidence verified that those abundance-improved genera, which were enhanced by nitrogen-reducing fertilization, were more likely to benefit the plants and their soil environment. For instance, Tausonia could help plants to tolerate low temperature and improve the fermentation process (Kim et al., 2020). Trichocladium was verified to be used as biofertilizers and biofungicides, which played an important role in declining the tobacco disease occurrence rate and index. Furthermore, some species of Trichocladium could produce cellulolytic enzymes, which provided microorganisms with carbon sources and provided tobacco with nutrients (Kubicek et al., 2019). Meanwhile, nitrogen-reducing fertilization not only enhanced the beneficial fungi in tobacco rhizosphere soil but also decreased the negative. For instance, Naganishia (Horváth et al., 2020), Trichoderma (Poveda et al., 2020), Fusicolla (Clocchiatti et al., 2021), Paraphaeosphaeria (Ding et al., 2020), and Albifimbria (Matić et al., 2019) were reported to be plant pathogens that destroyed the growth and yield of many crops (Masachis et al., 2016).
Afterward, the correlation between key taxa and tobacco diseases was analyzed, based on the Mantel test (Crabot et al., 2019). The results indicated that the key taxa, whose relative abundances were significantly (p < 0.05) different from CK, made great contributions to the present variation of occurrence rates and disease indexes of tobacco diseases among different treatments. It proved that the key taxa excavated in this study were the dominant reason why nitrogen-reducing fertilization could decrease the occurrence rate and disease index of tobacco diseases. Although the results of correlation between key taxa and tobacco diseases emphasized the importance of the key genera, the causal relationships between these genera and tobacco diseases were beyond understanding. Hence, the PLS-PM analysis was implemented to address this issue (Sanchez, 2013). Through the results, the operating mechanisms of key genera, which were integrated into two modules (InKG and DeKG), in the performance of inhibiting tobacco diseases were revealed. From the aspect of TMV, the inhibitory effect of nitrogen-reducing fertilization against TMV primarily owed to the decreased key genera which caused by this strategy. When it came to TBS, the increased key genera and decreased key genera both made contributions to the inhibitory effect of this strategy, whereas InKG and DeKG did not have significant path coefficients with TBW, which indicated that there was no obvious causal relationship between key genera and TBW. The reason why RNTe could decline the TBW should be further explored beyond the fungal community of rhizosphere soil. The endogenous fungus of tobacco was suggested to be researched.
Moreover, the inhibitory effect of nitrogen-reducing fertilization was highest in RNTe, while the efficiency declined from RNTe to RNTh. It demonstrated that the reducing amount of nitrogen fertilizer was limited according to the performance of this strategy. In the present research, ten percentage of nitrogen-reducing fertilization was appropriate for tobacco planting against diseases. Additionally, the inhibitory effect of nitrogen-reducing fertilization against TBW was much lower than that against TMV and TBS. Other methods should be cooperated with this fertilization strategy to better protect tobacco from TBW.
In this study, the inhibitory effect of nitrogen-reducing fertilization strategy against tobacco diseases was investigated to be significantly effective in tobacco-planting agriculture. The best performance of disease control occurred in 10% reduction of nitrogen fertilizer. The variation of the fungal community between nitrogen-reducing fertilization and CK was highly consistent with the circumstances of tobacco diseases. Furthermore, the positive genera such as Tausonia and Trichocladium and the negative genera such as Naganishia and Fusicolla were identified to be the key genera which dominated the fungal inhibition against tobacco diseases, due to the economic and eco-friendly fertilization strategy. This study provides a constructive strategy to inhibit tobacco diseases in continuous cropping areas of tobacco. It also provides a theoretical basis for developing the potential fungal inhibitory effects of native microorganisms stimulated by nitrogen-reducing fertilization.
The data presented in the study are deposited in the NCBI repository (https://www.ncbi.nlm.nih.gov/bioproject/PRJNA808161), accession number: PRJNA808161.
Conceptualization: X-ML and M-CS; experiment implementation: G-DB, Y-ZS, and M-CS; data processing: M-CS and G-DB; writing—original draft preparation: M-CS; writing—review and editing: M-CS, Y-ZS, and X-ML; and funding acquisition: X-ML. All authors have read and approved the final manuscript.
This research was supported by the Key Projects of Shandong Qingdao Tobacco Co., Ltd. (No. YCSKY202007030-C1) and the Technology Project of Hubei Tobacco Co., Ltd. (No. 027Y2021-011).
Author Y-ZS was employed by Cigar Institute of China Tobacco Hubei Industrial Co., Ltd.
The remaining authors declare that the research was conducted in the absence of any commercial or financial relationships that could be construed as a potential conflict of interest.
All claims expressed in this article are solely those of the authors and do not necessarily represent those of their affiliated organizations, or those of the publisher, the editors, and the reviewers. Any product that may be evaluated in this article, or claim that may be made by its manufacturer, is not guaranteed or endorsed by the publisher.
The Supplementary Material for this article can be found online at: https://www.frontiersin.org/articles/10.3389/fbioe.2022.866419/full#supplementary-material
Abo-Zaid, G. A., Matar, S. M., and Abdelkhalek, A. (2020). Induction of Plant Resistance against Tobacco Mosaic Virus Using the Biocontrol Agent Streptomyces Cellulosae Isolate Actino 48. Agronomy 10, 1620. doi:10.3390/agronomy10111620
Ahmed, W., Yang, J., Tan, Y., Munir, S., Liu, Q., Zhang, J., et al. (2022). Ralstonia Solanacearum, a Deadly Pathogen: Revisiting the Bacterial Wilt Biocontrol Practices in Tobacco and Other Solanaceae. Rhizosphere 21, 100479. doi:10.1016/j.rhisph.2022.100479
Aliyu, I. A., Yusuf, A. A., Uyovbisere, E. O., Masso, C., and Sanders, I. R. (2019). Effect of Co-application of Phosphorus Fertilizer and In Vitro-produced Mycorrhizal Fungal Inoculants on Yield and Leaf Nutrient Concentration of Cassava. PLoS One 14, e0218969. doi:10.1371/journal.pone.0218969
Alori, E. T., and Babalola, O. O. (2018). Microbial Inoculants for Improving Crop Quality and Human Health in Africa. Front. Microbiol. 9, 2213. doi:10.3389/fmicb.2018.02213
Callahan, B. J., Mcmurdie, P. J., Rosen, M. J., Han, A. W., Johnson, A. J. A., and Holmes, S. P. (2016). DADA2: High-Resolution Sample Inference from Illumina Amplicon Data. Nat. Methods 13, 581–583. doi:10.1038/nmeth.3869
Cao, Y., Zhang, Z., Ling, N., Yuan, Y., Zheng, X., Shen, B., et al. (2011). Bacillus Subtilis SQR 9 Can Control Fusarium Wilt in Cucumber by Colonizing Plant Roots. Biol. Fertil. Soils 47, 495–506. doi:10.1007/s00374-011-0556-2
Chakraborty, S., and Newton, A. C. (2011). Climate Change, Plant Diseases and Food Security: an Overview. Plant Pathol. 60, 2–14. doi:10.1111/j.1365-3059.2010.02411.x
Chellemi, D. O., Gamliel, A., Katan, J., and Subbarao, K. V. (2016). Development and Deployment of Systems-Based Approaches for the Management of Soilborne Plant Pathogens. Phytopathology 106, 216–225. doi:10.1094/phyto-09-15-0204-rvw
Choi, K., Choi, J., Lee, P. A., Roy, N., Khan, R., Lee, H. J., et al. (2020). Alteration of Bacterial Wilt Resistance in Tomato Plant by Microbiota Transplant. Front. Plant Sci. 11, 1186. doi:10.3389/fpls.2020.01186
Chunyu, L., Weicong, H., Bin, P., Yan, L., Saifei, Y., Yuanyuan, D., et al. (2017). Rhizobacterium Bacillus Amyloliquefaciens Strain SQRT3-Mediated Induced Systemic Resistance Controls Bacterial Wilt of Tomato. Pedosphere 27, 1135–1146.
Clocchiatti, A., Hannula, S. E., Van Den Berg, M., Hundscheid, M. P. J., and De Boer, W. (2021). Evaluation of Phenolic Root Exudates as Stimulants of Saptrophic Fungi in the Rhizosphere. Front. Microbiol. 12, 644046. doi:10.3389/fmicb.2021.644046
Crabot, J., Clappe, S., Dray, S., and Datry, T. (2019). Testing the Mantel Statistic with a Spatially‐constrained Permutation Procedure. Methods Ecol. Evol. 10, 532–540. doi:10.1111/2041-210x.13141
De Carvalho Pontes, N., Yamada, J. K., Fujinawa, M. F., Dhingra, O. D., and De Oliveira, J. R. (2019). Soil Fumigation with Mustard Essential Oil to Control Bacterial Wilt in Tomato. Eur. J. Plant Pathol. 155, 435–444. doi:10.1007/s10658-019-01777-0
Ding, T., Li, Z., Guo, L., and Li, J. (2020). First Report of Leaf Spots and Necrosis Caused by Paraphaeosphaeria Recurvifoliae on Yucca Gloriosa in China. Plant Dis. 104, 986. doi:10.1094/pdis-07-19-1383-pdn
Edgar, R. C., Haas, B. J., Clemente, J. C., Quince, C., and Knight, R. (2011). UCHIME Improves Sensitivity and Speed of Chimera Detection. Bioinformatics 27, 2194–2200. doi:10.1093/bioinformatics/btr381
Ghorbanpour, M., Omidvari, M., Abbaszadeh-Dahaji, P., Omidvar, R., and Kariman, K. (2018). Mechanisms Underlying the Protective Effects of Beneficial Fungi against Plant Diseases. Biol. Control. 117, 147–157. doi:10.1016/j.biocontrol.2017.11.006
Haas, B. J., Gevers, D., Earl, A. M., Feldgarden, M., Ward, D. V., Giannoukos, G., et al. (2011). Chimeric 16S rRNA Sequence Formation and Detection in Sanger and 454-pyrosequenced PCR Amplicons. Genome Res. 21, 494–504. doi:10.1101/gr.112730.110
Horváth, E., Sipiczki, M., Csoma, H., and Miklós, I. (2020). Assaying the Effect of Yeasts on Growth of Fungi Associated with Disease. BMC Microbiol. 20, 320. doi:10.1186/s12866-020-01942-0
Hu, D., Li, S., Li, Y., Peng, J., Wei, X., Ma, J., et al. (2020). Streptomyces Sp. Strain TOR3209: a Rhizosphere Bacterium Promoting Growth of Tomato by Affecting the Rhizosphere Microbial Community. Sci. Rep. 10, 20132–20215. doi:10.1038/s41598-020-76887-5
Hu, Q., Zhang, H., Zhang, L., Liu, Y., Huang, C., Yuan, C., et al. (2021a). Two Tobamovirus Multiplication 2A Homologs in Tobacco Control Asymptomatic Response to Tobacco Mosaic Virus. Plant Physiol. 187, 2674–2690. doi:10.1093/plphys/kiab448
Hu, Y., Zhao, W., Li, X., Feng, J., Li, C., Yang, X., et al. (2021b). Integrated Biocontrol of Tobacco Bacterial Wilt by Antagonistic Bacteria and Marigold. Sci. Rep. 11, 1–12. doi:10.1038/s41598-021-95741-w
Huang, K., Jiang, Q., Liu, L., Zhang, S., Liu, C., Chen, H., et al. (2020). Exploring the Key Microbial Changes in the Rhizosphere that Affect the Occurrence of Tobacco Root-Knot Nematodes. Amb Express 10, 72–11. doi:10.1186/s13568-020-01006-6
Jaiswal, A. K., Frenkel, O., Tsechansky, L., Elad, Y., and Graber, E. R. (2018). Immobilization and Deactivation of Pathogenic Enzymes and Toxic Metabolites by Biochar: a Possible Mechanism Involved in Soilborne Disease Suppression. Soil Biol. Biochem. 121, 59–66. doi:10.1016/j.soilbio.2018.03.001
Kim, M.-J., Lee, H.-W., Kim, J. Y., Kang, S. E., Roh, S. W., Hong, S. W., et al. (2020). Impact of Fermentation Conditions on the Diversity of white colony-forming Yeast and Analysis of Metabolite Changes by white colony-forming Yeast in Kimchi. Food Res. Int. 136, 109315. doi:10.1016/j.foodres.2020.109315
Kim, S. G., Hur, O.-S., Ro, N.-Y., Ko, H.-C., Rhee, J.-H., Sung, J. S., et al. (2016). Evaluation of Resistance to Ralstonia Solanacearum in Tomato Genetic Resources at Seedling Stage. Plant Pathol. J. 32, 58–64. doi:10.5423/ppj.nt.06.2015.0121
Kõljalg, U., Nilsson, R. H., Abarenkov, K., Tedersoo, L., Taylor, A. F., Bahram, M., et al. (2013). Towards a Unified Paradigm for Sequence‐based Identification of Fungi. Wiley Online Library.
Kubicek, C. P., Steindorff, A. S., Chenthamara, K., Manganiello, G., Henrissat, B., Zhang, J., et al. (2019). Evolution and Comparative Genomics of the Most Common Trichoderma Species. BMC Genomics 20, 485. doi:10.1186/s12864-019-5680-7
Li, B.-B., Roley, S. S., Duncan, D. S., Guo, J., Quensen, J. F., Yu, H.-Q., et al. (2021a). Long-term Excess Nitrogen Fertilizer Increases Sensitivity of Soil Microbial Community to Seasonal Change Revealed by Ecological Network and Metagenome Analyses. Soil Biol. Biochem. 160, 108349. doi:10.1016/j.soilbio.2021.108349
Li, H., Qiu, Y., Yao, T., Ma, Y., Zhang, H., and Yang, X. (2020). Effects of PGPR Microbial Inoculants on the Growth and Soil Properties of Avena Sativa, Medicago Sativa, and Cucumis Sativus Seedlings. Soil Tillage Res. 199, 104577. doi:10.1016/j.still.2020.104577
Li, Y., Chen, H., Song, L., Wu, J., Sun, W., and Teng, Y. (2021b). Effects on Microbiomes and Resistomes and the Source-specific Ecological Risks of Heavy Metals in the Sediments of an Urban River. J. Hazard. Mater. 409, 124472. doi:10.1016/j.jhazmat.2020.124472
Liu, H., Brettell, L. E., Qiu, Z., and Singh, B. K. (2020). Microbiome-mediated Stress Resistance in Plants. Trends Plant Sci. 25, 733–743. doi:10.1016/j.tplants.2020.03.014
Liu, J., Li, S., Yue, S., Tian, J., Chen, H., Jiang, H., et al. (2021). Soil Microbial Community and Network Changes after Long-Term Use of Plastic Mulch and Nitrogen Fertilization on Semiarid farmland. Geoderma 396, 115086. doi:10.1016/j.geoderma.2021.115086
Long, Z.-Q., Yang, L.-L., Zhang, J.-R., Liu, S.-T., Jiao Xie, J., Wang, P.-Y., et al. (2021). Fabrication of Versatile Pyrazole Hydrazide Derivatives Bearing a 1,3,4-Oxadiazole Core as Multipurpose Agricultural Chemicals against Plant Fungal, Oomycete, and Bacterial Diseases. J. Agric. Food Chem. 69, 8380–8393. doi:10.1021/acs.jafc.1c02460
Masachis, S., Segorbe, D., Turrà, D., Leon-Ruiz, M., Fürst, U., El Ghalid, M., et al. (2016). A Fungal Pathogen Secretes Plant Alkalinizing Peptides to Increase Infection. Nat. Microbiol. 1, 16043–16049. doi:10.1038/nmicrobiol.2016.43
Matić, S., Gilardi, G., Gullino, M. L., and Garibaldi, A. (2019). Emergence of Leaf Spot Disease on Leafy Vegetable and Ornamental Crops Caused by Paramyrothecium and Albifimbria Species. Phytopathology 109, 1053–1061. doi:10.1094/PHYTO-10-18-0396-R
Morais, T. P., Zaini, P. A., Chakraborty, S., Gouran, H., Carvalho, C. P., Almeida-Souza, H. O., et al. (2019). The Plant-Based Chimeric Antimicrobial Protein SlP14a-PPC20 Protects Tomato against Bacterial Wilt Disease Caused by Ralstonia Solanacearum. Plant Sci. 280, 197–205. doi:10.1016/j.plantsci.2018.11.017
Ogunnaike, B., Bogle, D., Banga, J. R., and Parker, R. (2021). Biological Control Systems and Disease Modeling. Front. Bioeng. Biotechnol. 9, 262. doi:10.3389/fbioe.2021.677976
Pellegrino, E., Turrini, A., Gamper, H. A., Cafà, G., Bonari, E., Young, J. P. W., et al. (2012). Establishment, Persistence and Effectiveness of Arbuscular Mycorrhizal Fungal Inoculants in the Field Revealed Using Molecular Genetic Tracing and Measurement of Yield Components. New Phytol. 194, 810–822. doi:10.1111/j.1469-8137.2012.04090.x
Peng, D., Luo, K., Jiang, H., Deng, Y., Bai, L., and Zhou, X. (2017). Combined Use ofBacillus Subtilisstrain B-001 and Bactericide for the Control of Tomato Bacterial Wilt. Pest Manag. Sci. 73, 1253–1257. doi:10.1002/ps.4453
Pétriacq, P., Williams, A., Cotton, A., Mcfarlane, A. E., Rolfe, S. A., and Ton, J. (2017). Metabolite Profiling of Non-sterile Rhizosphere Soil. Plant J. 92, 147–162. doi:10.1111/tpj.13639
Poveda, J., Eugui, D., and Abril-Urias, P. (2020). “Could Trichoderma Be a Plant Pathogen? Successful Root Colonization,” in Trichoderma (Springer), 35–59. doi:10.1007/978-981-15-3321-1_3
Preininger, C., Sauer, U., Bejarano, A., and Berninger, T. (2018). Concepts and Applications of Foliar spray for Microbial Inoculants. Appl. Microbiol. Biotechnol. 102, 7265–7282. doi:10.1007/s00253-018-9173-4
Raymaekers, K., Ponet, L., Holtappels, D., Berckmans, B., and Cammue, B. P. A. (2020). Screening for Novel Biocontrol Agents Applicable in Plant Disease Management - A Review. Biol. Control. 144, 104240. doi:10.1016/j.biocontrol.2020.104240
Ren, Z., Li, Y., Fang, W., Yan, D., Huang, B., Zhu, J., et al. (2018). Evaluation of Allyl Isothiocyanate as a Soil Fumigant against Soil-Borne Diseases in Commercial Tomato (Lycopersicon esculentumMill.) Production in China. Pest Manag. Sci. 74, 2146–2155. doi:10.1002/ps.4911
Strange, R. N., and Scott, P. R. (2005). Plant Disease: a Threat to Global Food Security. Annu. Rev. Phytopathol. 43, 83–116. doi:10.1146/annurev.phyto.43.113004.133839
Tobacco Research Institute (2008). Grade and Investigation Method of Tobacco Diseases and Insect Pests. Qingdao: General Administration of Quality Supervision, Inspection and Quarantine of the People's Republic of China; Standardization Administration of China.
Vassilev, N., Vassileva, M., Martos, V., Garcia Del Moral, L. F., Kowalska, J., Tylkowski, B., et al. (2020). Formulation of Microbial Inoculants by Encapsulation in Natural Polysaccharides: Focus on Beneficial Properties of Carrier Additives and Derivatives. Front. Plant Sci. 11, 270. doi:10.3389/fpls.2020.00270
Wang, J. L., Liu, K. L., Zhao, X. Q., Zhang, H. Q., Li, D., Li, J. J., et al. (2021). Balanced Fertilization over Four Decades Has Sustained Soil Microbial Communities and Improved Soil Fertility and rice Productivity in Red Paddy Soil. Sci. Total Environ. 793, 148664. doi:10.1016/j.scitotenv.2021.148664
Wang, M., Zhang, L., He, Y., Huang, L., Liu, L., Chen, D., et al. (2022). Soil Fungal Communities Affect the Chemical Quality of Flue-Cured Tobacco Leaves in Bijie, Southwest China. Sci. Rep. 12, 2815. doi:10.1038/s41598-022-06593-x
Wen, Y.-C., Li, H.-Y., Lin, Z.-A., Zhao, B.-Q., Sun, Z.-B., Yuan, L., et al. (2020). Long-term Fertilization Alters Soil Properties and Fungal Community Composition in Fluvo-Aquic Soil of the North China Plain. Sci. Rep. 10, 7198. doi:10.1038/s41598-020-64227-6
Xie, S., Vallet, M., Sun, C., Kunert, M., David, A., Zhang, X., et al. (2020). Biocontrol Potential of a Novel Endophytic Bacterium from mulberry (Morus) Tree. Front. Bioeng. Biotechnol. 7, 488. doi:10.3389/fbioe.2019.00488
Yang, L., Zou, Y.-N., Tian, Z.-H., Wu, Q.-S., and Kuča, K. (2021). Effects of Beneficial Endophytic Fungal Inoculants on Plant Growth and Nutrient Absorption of Trifoliate orange Seedlings. Scientia Horticulturae 277, 109815. doi:10.1016/j.scienta.2020.109815
Yang, T., Lupwayi, N., Marc, S.-A., Siddique, K. H. M., and Bainard, L. D. (2021). Anthropogenic Drivers of Soil Microbial Communities and Impacts on Soil Biological Functions in Agroecosystems. Glob. Ecol. Conservation 27, e01521. doi:10.1016/j.gecco.2021.e01521
Yuan, X.-L., Cao, M., Shen, G.-M., Zhang, H.-B., Du, Y.-M., Zhang, Z.-F., et al. (2020). Characterization of Nuclear and Mitochondrial Genomes of Two Tobacco Endophytic Fungi Leptosphaerulina Chartarum and Curvularia Trifolii and Their Contributions to Phylogenetic Implications in the Pleosporales. Ijms 21, 2461. doi:10.3390/ijms21072461
Zhou, S.-M., Zhang, M., Zhang, K.-K., Yang, X.-W., He, D.-X., Yin, J., et al. (2020). Effects of Reduced Nitrogen and Suitable Soil Moisture on Wheat (Triticum aestivum L.) Rhizosphere Soil Microbiological, Biochemical Properties and Yield in the Huanghuai Plain, China. J. Integr. Agric. 19, 234–250. doi:10.1016/s2095-3119(19)62697-3
Keywords: nitrogen-reducing fertilization, variation of fungal community, fungal inhibition, cash crop, sustainable agriculture
Citation: Shen M-C, Shi Y-Z, Bo G-D and Liu X-M (2022) Fungal Inhibition of Agricultural Soil Pathogen Stimulated by Nitrogen-Reducing Fertilization. Front. Bioeng. Biotechnol. 10:866419. doi: 10.3389/fbioe.2022.866419
Received: 31 January 2022; Accepted: 11 March 2022;
Published: 12 April 2022.
Edited by:
Zhipeng Wang, Qingdao Agricultural University, ChinaReviewed by:
Guodong Liu, Shandong University, ChinaCopyright © 2022 Shen, Shi, Bo and Liu. This is an open-access article distributed under the terms of the Creative Commons Attribution License (CC BY). The use, distribution or reproduction in other forums is permitted, provided the original author(s) and the copyright owner(s) are credited and that the original publication in this journal is cited, in accordance with accepted academic practice. No use, distribution or reproduction is permitted which does not comply with these terms.
*Correspondence: Xin-Min Liu, bGl1eGlubWluQGNhYXMuY24=
Disclaimer: All claims expressed in this article are solely those of the authors and do not necessarily represent those of their affiliated organizations, or those of the publisher, the editors and the reviewers. Any product that may be evaluated in this article or claim that may be made by its manufacturer is not guaranteed or endorsed by the publisher.
Research integrity at Frontiers
Learn more about the work of our research integrity team to safeguard the quality of each article we publish.