- 1Faculty of Sports Science, Ningbo University, Ningbo, China
- 2Doctoral School on Safety and Security Sciences, Óbuda University, Budapest, Hungary
- 3Science Laboratory, Innovation center of Xtep Co., Ltd., Xiamen, China
- 4Savaria Institute of Technology, Eötvös Loránd University, Budapest, Hungary
The study aimed to research the effects of innovative running shoes (a high heel-to-toe drop and special structure of midsole) on the biomechanics of the lower limbs and perceptual sensitivity in female runners. Fifteen healthy female runners were recruited to run through a 145-m runway with planted force plates at one peculiar speed (3.6 m/s ± 5%) with two kinds of shoe conditions (innovative running shoes vs. normal running shoes) while getting biomechanical data. The perception of shoe characteristics was assessed simultaneously through a 15-cm visual analog scale. The statistical parametric mapping technique calculated the time-series parameters. Regarding 0D parameters, the ankle dorsiflexion angle of innovative running shoes at touchdown was higher, and the peak dorsiflexion angle, range of motion, peak dorsiflexion velocity, and plantarflexion moment on the metatarsophalangeal joint of innovative running shoes during running were significantly smaller than those of normal running shoes (all p < 0.001). In addition, the braking phase and the time of peak vertical force 1 of innovative running shoes were found to be longer than those of normal running shoes (both p < 0.05). Meanwhile, the average vertical loading rate 1, peak vertical loading rate 1, peak braking force, and peak vertical force 1 in the innovative running shoes were lower than those of the normal running shoes during running (both p < 0.01). The statistical parametric mapping analysis exhibited a higher ankle dorsiflexion angle (0–4%, p < 0.05), a smaller knee internal rotation angle (0–6%, p < 0.05) (63–72%, p < 0.05), a decreased vertical ground reaction force (11–17%, p = 0.009), and braking anteroposterior ground reaction force (22–27%, p = 0.043) for innovative running shoes than normal running shoes. Runners were able to perceive the cushioning of innovative running shoes was better than that of normal running shoes. These findings suggested combining the high offset and structure of the midsole would benefit the industrial utilization of shoe producers in light of reducing the risk of running injuries for female runners.
Introduction
Participants in the half marathon have grown rapidly. The number of participants has increased from 300,000 in 1990 to nearly 2 million in 2013, and over 60% were female runners (Wilder, 2014). It had been recorded that the number of traditionally shod runners who landed with a rearfoot strike (RFS) was more than 80% (Hasegawa et al., 2007; Larson et al., 2011). Commonly running-related injuries such as tibial stress fractures, patellofemoral pain, and plantar fasciitis were linked to the high loading rates and impact transients during rearfoot striking (Milner et al., 2006; Pohl et al., 2009; Davis et al., 2010).
It was related to the fact that male and female runners have significantly different physiological characteristics and sports running posture. A greater active hip internal rotation, vertical ground reaction force (GRF), accessible vertical torque, peak hip flexion angle, and negative work were displayed in females than in males (Ferber et al., 2003). Women had a greater ratio of hip-width to femur length, which resulted in greater hip internal rotation. In addition, women exhibiting higher Q angles increase lateral quadriceps pull on the patella. It would exacerbate patellar tenderness or recurrent lateral patellar subluxation conditions, which induced a higher incidence of patellofemoral joint pain (Horton and Hall, 1989). Tendon stiffness might be associated with the regular use of high-heeled shoes in some females, which led to higher hypertrophy and shortening of the Achilles tendon, a higher pre-activation amplitude of the peroneal muscle, and greater gluteus maximus muscle activation (Baur et al., 2010; Csapo et al., 2010; Napier et al., 2018). Also, 76% of knee pain was found in women. In addition, it was stated that female runners would be twice compared to males as likely to sustain certain running injuries like the aforementioned sports injuries (Taunton et al., 2002). Therefore, it was essential to design running shoes according to female athletes’ biomechanics and body structural characteristics.
Running shoe manufacturers have focused on cushioning, stability, and motion control while making shoes for female runners to diminish running injuries (Malisoux et al., 2016). It was previously proved that reducing the impact forces by wearing a more cushioned shoe may release stress on the musculoskeletal tissue (James et al., 1978; Lieberman et al., 2010). Footwears such as the thickness of the midsole and heel-toe drop (HTD) have been considered in studies of young athletes that could influence a runner’s performance, particularly in cushioning (Hasegawa et al., 2007; Sinclair et al., 2012; Chambon et al., 2015; Nigg et al., 2015). Increasing the midsole thickness could protonate the runner’s effective leg length, such as the Nike Vaporfly 4%, which has a 31-mm heel height (Allen and Kurihara, 1982). It could decrease energy loss for the runner by increasing an effective leg length of 8 mm (Pontzer, 2007; Hoogkamer et al., 2018). In addition, some researchers also figured out that the effect of midsole thickness was about 1% for running economy (Tung et al., 2014).
The HTD would increase with the increased thickness of the heeling material. Recently, the HTD as a critical feature in the shoe design has been linked to running injury risk (Malisoux et al., 2016). Several authors from the biomechanics view had researched the effect of different HTDs. It was reported that a 4-mm HTD induced a higher vertical loading rate than 8 and 12 mm HTD, and the lower limb biomechanics performance of a 4-mm HTD was not similar to barefoot running (Richert et al., 2019). During the investigation, there was no specific adaptation in spatiotemporal variables and kinematics between the three kinds of shoes (0 mm HTD, 6 mm HTD, and 10 mm HTD) (Malisoux et al., 2017). It also found that heel-toe drops (4 and 12 mm) did not directly affect the spatiotemporal parameters of the running cycle in female runners (Gijon-Nogueron et al., 2019). It was still being debated that the thickness of the rearfoot over 45 mm was associated with some gait troubles such as postural disorders and changing spatiotemporal parameters because it would modify the muscle balance up to muscle overuse and strain injuries (Kim et al., 2011; Barkema et al., 2012; Mika et al., 2012; Silva et al., 2013). This was reported that it was essential to affect the center of pressure that the height of the rearfoot had to be more than 2.5 cm (Chien et al., 2013). The vertical loading rate and the associated transient peak reduced as the shoe drops grew. Above all, few shoe manufacturers have made running shoes with an over 12-mm HTD or according to female runners’ characteristics. It was worth researching whether increasing the HTD by more than 12 mm can significantly reduce the impact force or not. Samir et al. (Hessas et al., 2017) asked the volunteer to test barefoot or equipped with three types of stiffness with the same lift height of 20 mm. They found no significant influence of material stiffness on the anterior-posterior displacement of the center of pressure and metatarsal pressures, but it obviously affected the calcaneus’s peak pressure. Furthermore, it was insufficient to investigate the effect of different HTDs on lower limb biomechanics and perceptual sensitivity.
Thus, this study was targeted to investigate how the biomechanics of the lower limb changed when wearing a pair of innovative running shoes (IRS) with a 16-mm HTD which has three layers of the midsole (upper and lower layers were for cushioning, and the middle layer was for support) and to investigate the biomechanics difference between IRS and normal running shoes (NRS). According to the previous literature, it was hypothesized that 1) greater ankle dorsiflexion at touchdown and a lower vertical loading rate would be found in the IRS model than in NRS. 2) The IRS would induce increasing the joints’ moment in the sagittal plane in comparison to the NRS.
Methods
Participants
Prior to the study, the sample size of the current study was calculated via G*Power 3.1.9.7 (effect size = 0.8, αvalue = 0.05, and power value = 0.8) (Kang, 2021). Also, fifteen female runners [mean (SD) age: 39.00 (10.09) years, height: 1.58 (0.37) m, weight: 50.34 (3.24) kg, and BMI: 20.14 (1.28) kg/m2] joined in this research. All participants were recruited from the Xiamen running club and identified themselves as rearfoot strike runners. Participants were uninjured in the lower extremities for at least 6 months before data collection, had a minimum weekly running mileage of 30 km, and could run 10 km in less than 70 min. Participants’ average running experience was 3.53 (0.15) years, their current running exposure was 35.67 (11.48) km/week, and they had an average 10-km time of 72.26 (2.19) minutes. All participants had been confirmed in foot size (EU 37 ± 0.5) by the using Brannock Device (The Brannock Device Co., Syracuse, NY, USA.) before the official test.
Experimental Footwear
There were two kinds of experimental footwear (innovative running shoes: IRS and normal running shoes: NRS) which differed in their offset, mechanical midsole hardness, rearfoot impact, and forefoot flexion properties used in this research (Figure 1).
The mechanical impact measurement considered the final five impacts from 30 repetitive impacts by using an impact tester (Brentwood, NH, USA) on the experimental shoes with a drop height of 5.0 mm and a drop mass of 8.5 kg (ASTM F19976-13, 2022).
The shoe longitudinal bending stiffness (LBS) and energy return were measured by fixing the forefoot area in the location of 70% foot length (heel to toe) and then bending at 45° by applying a dynamic shoe flexor device (Brentwood, NH, USA) (ASTM F911-85, 1994; Isherwood et al., 2020). All shoe conditions featured an XTEP SoftPad Lite HD foam insole with a forefoot and rearfoot thickness of 5.0 mm. All characteristics of shoes are shown in Table1.
Experiment Protocol and Procedures
Biomechanical Data Collection and Processing Process
The data collection methodology was carried out as in the previous research (Isherwood et al., 2020). Participants performed eight valid right foot rearfoot strike running trials per shoe condition on a 145-m concrete indoor running loop. A valid trial was one within the specified velocity range (3.6 m/s ± 5%) and made up of the whole right foot contacting the force plate area. Previously, data collection participants warmed up for about 5 min and were acquainted with the target speed and shoe conditions by running two laps in each shoe condition. Upon failing to match the required speed in the first two laps, further familiarization laps were performed as necessary.
In GRF and 3D kinematic measurements, participants ran a set of three consecutive laps and flushed into the floor force plates (combined dimensions 270 × 60 cm, 1,000 Hz (AMTI, Watertown, MA, USA)) in each shoe condition. The test sequence of shoes was randomized for each participant. The two-timing gates 8 m far from the middle force plate were used to record the running speed (Smart speed, Burbank, CA, USA) set 8 m apart, centering the middle force plate. Right leg kinematics were collected at 250 Hz and were ordered using a 10-camera motion analysis system in a capture volume of 4.0 × 1.0 × 1.5 m (Vantage 5, Vicon, Metrics Ltd., Oxford, UK). The marker set was according to the calibrated anatomical systems technique (Cappozzo et al., 1995). The right thigh, the right shank, and the right foot (forefoot and rearfoot) were defined as segments by attaching retro-reflective markers of 14 mm in diameter on the skin of the right and left anterior superior iliac spine (ASIS), the right and the left posterior superior iliac spine (PSIS), the right greater trochanter, the medial and lateral epicondyle of the femur, and the medial and lateral malleolus, as well as attached to the shoe, representing the first and fifth metatarsal heads and second toe. Four marker tracking clusters were attached to the lateral side of the thigh and the lateral side of the lower leg (Manal et al., 2000). The extra-reflective markers were added to the distal, proximal heel, and lateral rearfoot, respectively, and were defined as shoe-mounted tracking markers (Heiderscheit et al., 2002). Before data collection, a static trial was conducted; all study procedures about biomechanical data collection were performed in the XTEP science laboratory. In the trial, valid data could be used when the first impact peak and shoe ground angle more than zero appeared. We used the Vicon Nexus 2.7 and Visual3D systems (C-Motion, Germantown, MD, USA) to process the collected experimental data. A fourth-order low pass Butterworth filter was used with a cut-off frequency of 100 Hz (kinetic) and 10 Hz (kinematic) (Isherwood et al., 2020). The XYZ Cardan sequence was used to calculate lower limbs’ kinematic and kinetic data, in which X represents flexion-extension, Y represents abduction–adduction, and Z represents internal-external rotation (Hennig et al., 1993). The angle, the angular velocity, the ground reaction force, and the work of the hip, the knee, the ankle, and the MTP joints of the right lower limb were measured during the stance phase using Visual3D (C-Motion, Germantown, MD, USA). The stride length (SL) was calculated as the anterior-posterior displacement of the right heel marker during two consecutive heel-strike events. The loading rate was calculated as the slope of the vertical GRF between 20 and 80% of the period from heel strike to impact force. All vertical GRF variables were calculated based on the recommendations by Ueda et al. (2016).
Subjective Perception
Testing took place simultaneously with biomechanical data collection, with participants filling in on the questionnaire immediately after completing the eight successful trials required for the respective shoe condition. Runners assessed six perception variables (shoe weight, fit, arch support, cushioning, stability, and over preference) on a questionnaire that had been repeatedly highlighted in some articles (Sterzing et al., 2015; Tay et al., 2017; Bishop et al., 2020). Also, a 15-cm visual analog scale (VAS) was carried out; these have been previously applied for running footwear assessment (Mündermann et al., 2002; Mills et al., 2010; Kong et al., 2015; Sterzing et al., 2015). Participants were shown and explained both variables before each shoe condition during the initial familiarization and data collection.
Statistical Analysis
For 0D parameters, such as spatiotemporal parameters, average loading rate 1, peak loading rate 1, peak vertical force 1, joint moment, and some kinematics parameters, Shapiro–Wilk tests were adopted for normality distribution. Permutation non-parametric tests were chosen with MATLAB (The MathWorks, Naticks, MA) when the null hypothesis of the normality test was rejected. Paired t-tests were applied when appropriate. Statistics 0D parametric tests were processed by SPSS (24, IBM. Corp, Armonk, NY, USA). Effect sizes (Cohen’s d) were displayed for all statistical tests (0.2 < Cohen’s d < 0.5 = small effect, 0.5 < Cohen’s d < 0.8 = medium effect, and Cohen’s d > 0.8 = large effect).
A statistical parametric mapping (SPM) technique was used to assess the time series parameters such as one-dimensional (1D) kinematic and force trajectories (Pataky et al., 2015; Besson et al., 2019). SPM paired t-tests were performed on shoe effects for every 1D parameter (Nichols and Holmes, 2002). SPM tests were calculated with SPM1D v0.4 for MATLAB (www.spm1d.org, (Pataky et al., 2015)). The statistical significance alpha levels were set to < 0.05 for all statistical tests.
Results
The running speed was 3.59 ± 0.27 m/s and 3.61 ± 0.31 m/s for IRS and NRS (p = 0.673). Shapiro–Wilk tests revealed that 100% of biomechanical variables and 100% of perception variables were normally distributed (both p > 0.05).
Kinematics Variables
Concerning joint angles at touchdown, the ankle of IRS was at a more dorsiflexed position (p = 0.023) (Table 2), with no significant changes of the knee, hip flexion, and ankle inversion at the beginning of the contact ground being reported than that of the NRS.
As for running during stance time, the peak MTPJ dorsiflexion angle and peak MTPJ dorsiflexion velocity of IRS during running were significantly lower than those of NRS (all p < 0.001) (Table 2). In the frontal plane, there was no significant difference between IRS and NRS regarding the peak ankle eversion angle and peak ankle eversion velocity. The MTPJ range of motion (ROM) of IRS in the sagittal plane was significantly smaller in comparison with that of NRS (p < 0.001) (Table 2); there were no effects of wearing experience on ROM on the ankle, knee, and hip joints in this plane. Ankle ROM (in-eversion) showed no apparent difference between IRS and NRS.
The SPM analysis showed a significantly higher ankle dorsiflexion angle for the IRS than for the NRS between 0 and 4% of the stance time (p < 0.05). There was a smaller knee internal rotation angle for the IRS from 0 to 6% and from 63 to 72% of stance time than that of NRS (both p < 0.05). No significant angle difference between shoe conditions was found around the hip joint (Figure 2).
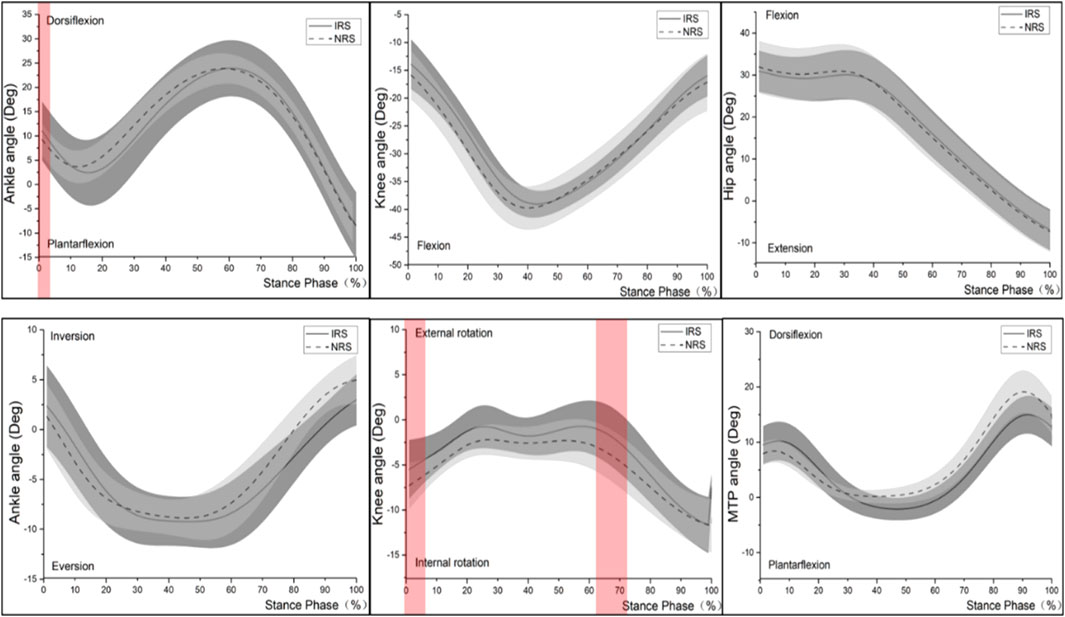
FIGURE 2. Lower limb joint angles time-normalized. Note: the red horizontal bars within the figure during corresponding periods represent significant shoe effects (SPMT-paired) between IRS and NRS.
Kinetics
There was no significant effect between IRS and NRS on the contact time and push-off phase, yet the braking phase and the time of peak vertical force 1 of IRS were found longer than those of NRS (p = 0.019; p = 0.001). It induced a certainly lower average vertical loading rate 1 (95%CI (IRS: 67.97–88.83; NRS: 87.55–112.25)) and peak vertical loading rate 1 (95%CI (IRS: 88.68–124.72; NRS: 153.10–185.70)) (both p < 0.001). Meanwhile, a lower peak braking force (95%CI (IRS: 0.38–0.46 BW; NRS: 0.42–0.50 BW)) and peak vertical force 1 (95%CI (IRS: 1.79– 2.07 BW; NRS: 1.95–2.26 BW)) were present in the IRS in comparison to the NRS during running (both p < 0.001). There was no shoe effect on the step frequency and step length (p = 0.410; p = 0.400). A lower peak MTPJ plantarflexion moment (95%CI (IRS: 1.42 to 1.70 Nm/kg; NRS: 1.61 to 1.99 Nm/kg)) was found for IRS than NRS (p = 0.047). Meanwhile, no significant difference was found in the joints’ work and peak moment (the ankle and knee) in the sagittal plane (Table 2).
The SPM test exhibited a significant effect of shoes on the vertical and anteroposterior components of the ground reaction force (both p < 0.001). The IRS decreased vertical GRF from 11 to 17% of the stance phase (p = 0.009) (Figure 3A) and decreased the braking anteroposterior GRF from 22 to 27% of the stance phase (p = 0.043) compared to the NRS (Figure 3B). No significant moment differences between shoe conditions were reported at the ankle, knee, and hip levels (all p > 0.05) (Figure 4).
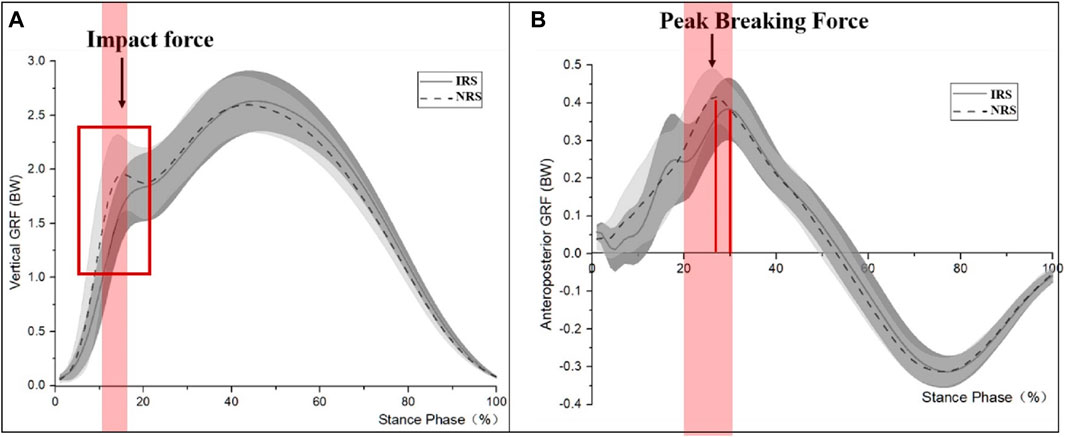
FIGURE 3. Mean vertical and anteroposterior ground reaction force-time and weight-normalized [(A) Vertical GRF, (B) anteroposterior GRF]. Positive and negative values are braking and propulsive forces. Standard deviations are presented by white and gray shaded areas. The red horizontal bars within the figure during corresponding periods represent significant shoe effects (SPMT-paired) between IRS and NRS.
Subjective Perception
Runners did not figure out the apparent difference between the two kinds of shoe conditions about the shoe weight, arch support, fit, stability, and over preference. Still, most of them thought that the cushioning of IRS was significantly better than that of NRS (Figure 5).
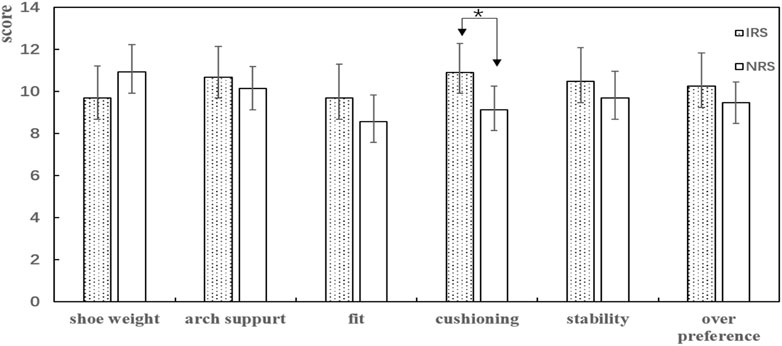
FIGURE 5. Mean and standard deviations for subjective perception were displayed (higher value and better performance). Note: * showed a significant effect between IRS and NRS.
Discussion
This study was targeted to clarify the consequence of IRS on female runners and compare the biomechanical difference between these kinds of running shoes during the stance phase. It also aimed to get the longitudinal bending stiffness (LBS) of the forefoot and peak acceleration of the rearfoot by mechanical testing and then on the biomechanical characteristics while running at 3.6 m/s.
According to the first hypothesis, the IRS would improve the shoe cushioning by increasing the HTD and height of the rearfoot during landing. In line with this behavior theory, the mechanical testing indicated a lower peak acceleration in the IRS (9.9 g) than the NRS (13.7 g), which induced significantly biomechanical changes, particularly during the braking phase, like a longer braking phase and the time to peak vertical force 1. Meanwhile, a certainly lower vertical force transient (average and peak) and peak vertical force 1 were present in the IRS in comparison to the NRS during running (all p < 0.001). The SPM test exhibited that the IRS decreased vertical GRF from 11 to 17% of the stance phase (p = 0.009) (Figure 3A) and decreased braking anteroposterior GRF from 22 to 27% of the stance phase (p = 0.043), as compared to the NRS (Figure 3B). All the results were consistent with previous studies (Chambon et al., 2015). It was reported that the vertical loading rate and the associated transient peak increased when the shoe drop decreased. In other words, increasing the shoe drop was the benefit of improving the cushioning of the running shoes.
As for the braking force, a reduced peak braking force was present in the IRS in comparison to the NRS during running. Some authors argued that the peak braking force (higher value) was associated with the risk of injury hazards such as iliotibial band syndrome and should be considered a target for gait retraining interventions (Napier et al., 2018). In other words, IRS in this study can effectively reduce the risk of lower extremity injuries than that of the NRS (Davis et al., 2010; Zadpoor and Nikooyan, 2011; van der Worp et al., 2016; Napier et al., 2018).
During the braking phase, the SPM analysis also showed a significantly higher ankle dorsiflexion angle for IRS, as compared to NRS between 0 and 4% of the stance time, which was consistent with previous reports (Paquette et al., 2013; Chambon et al., 2014; Breine et al., 2017). Horvais and Samozino (2013) confirmed a positive correlation between the shoe drop and shoe ground angle at touchdown in rearfoot runners. For example, the foot/ground angle at touchdown increased when the shoe drop increased.
It should be pointed out that the stability of the shoes is a particularly critical issue when increasing the HTD over 12 mm. In this study, there was no noticeable difference in the running posture at touchdown between the two kinds of shoes, except the ankle of IRS had a more dorsiflexed. In addition, there was no significant difference between IRS and NRS in some stability parameters such as the peak ankle eversion angle, peak ankle eversion velocity, and ankle ROM in the frontal plane in this study. This may be related to the ability of female runners to adapt to high heels and also induced by the special hardness composition of the midsole in IRS. From subjective perception perspectives, runners did not figure out the obvious difference between the two kinds of shoe conditions regarding stability, but most of them thought that the cushioning of IRS was better than that of NRS, which was consistent with our biomechanical results. In addition, there was a smaller knee internal rotation angle for IRS than for NRS from 0 to 6% and from 63 to 72% of stance time (both p < 0.05) (Figure 2). It was associated with the structure of the midsole with three layers (upper and lower layers are for cushioning, and the middle layer is for support) which played the role of motion control. Motion-control shoes were beneficial to reduce knee internal rotation in runners with over-pronation, no matter whether fresh or fatigued, which may assist pronated runners in maintaining their stability throughout a fatiguing running (Cheung and Ng, 2007; Lilley et al., 2013; Jafarnezhadgero et al., 2019). Referring to the second hypothesis, as previously shown, a higher net flexion moment on the knee joint or ankle with a higher heel-to-toe offset might increase the strain around the joint (Kerrigan et al., 2009). It was worth noting that a 16-mm shoe drop in this study did not cause significant changes in joints’ (knee and ankle) torque, which would be associated with the midsole’s three layers to modify the stress around the joints (ankle and knee). In addition, the effective limb length of the leg as a strut drives the scaling of locomotor cost for a runner, which was the leg length with the HTD when running with shoes (Allen and Kurihara, 1982). The HTD of the IRS was 9.5 mm more than that of NRS which could protonate the runner’s effective leg length, in other words, increased the force arm of the knee joint, offsetting the influence of the ground reaction force, which might be the reason why no significant difference was found in the knee joint moment (Allen and Kurihara, 1982; Pontzer, 2007; Hoogkamer et al., 2018). This study suggested that it was essential to combine the HTD and hardness of the midsole into account when designing a shoe to improve cushioning and reduce the risk of injuries.
From the perspective of running economy, mechanical testing proved a larger LBS in the IRS (0.307 ± 0.01 Nm/deg) than in the NRS (0.169 ± 0.01 Nm/deg), which induced significant modifications to the running biomechanics during running. Several authors figured out that increasing the stiffness of running shoes might cause a series of biomechanical changes, such as a lower MTPJ range of motion, peak MTPJ dorsiflexion angle, peak MTPJ dorsiflexion velocity, and peak MTPJ plantarflexion moment (Roy and Stefanyshyn, 2006; Willwacher et al., 2013; Smith et al., 2014; Oh and Park, 2017; Barnes and Kilding, 2019; Hunter et al., 2019). Those results were in line with this study. In other words, IRS could improve the running performance by increasing the LBS compared to that of the NRS. Studies had found that increasing LBS improved athletes’ running efficiency ranging between 1 and 2% (Fredericson and Misra, 2007).
Limitations and Conclusions
Referring to the limitations, the experimental shoes differed in the heel-to-toe offset height and shoe properties. Future investigations should only modify the shoe drop or hardness components of the midsole. In addition, motion-control shoes prevented exacerbated fatigue-related increases in pronated female runners (Dempster et al., 2021; Xiang et al., 2022). It is also extremely important to take the muscle activation of the lower limb and fatigue during running into account, when wearing a running shoe with the midsole’s three layers and 16-mm shoe drop.
Special innovative running shoes had two key points: the heel-to-toe drop of 16 mm and the second was the special hardness component of the midsole. It was the first time to explore the mechanism of the shoe drop reaching 16 mm on running biomechanics. This research added new insights into the mechanism of shoe drops on the female runner. Compared with normal running shoes, innovative running shoes in this study could effectively improve the cushioning and propulsion performance and play the role of motion control. In addition, the female running shoe with a 16 mm shoe drop did not cause significant joint (knee and ankle) torque changes in this study.
Data Availability Statement
The original contributions presented in the study are included in the article/Supplementary Material; further inquiries can be directed to the corresponding author.
Ethics Statement
The studies involving human participants were reviewed and approved by principles of the Declaration of Helsinki and approved by the Ethics Committee of the University of Ningbo. The patients/participants provided their written informed consent to participate in this study.
Author Contributions
Conceptualization: FF, LG, XT, QH, and YC; mechanical and biomechanical testing: FF, JW, YG, and ZX; writing—original draft preparation: FF; writing—review and editing: FF and YG. All authors have read and agreed to the published version of the manuscript.
Funding
This study was supported by the National Natural Science Foundation of China (81772423), the National Key R&D Program of China (2018YFF0300905), the NSFC-RSE Joint Research Programme (8181101592), the K.C. Wong Magna Fund in Ningbo University, and the János Bolyai Research Scholarship of the Hungarian Academy of Sciences (B.O./00047/21/6).
Conflict of Interest
FF, LG, XT, ZX, and YC were employed by the Company Science Laboratory, Innovation center of Xtep (China) Co., Ltd.
The remaining authors declare that the research was conducted in the absence of any commercial or financial relationships that could be construed as a potential conflict of interest.
Publisher’s Note
All claims expressed in this article are solely those of the authors and do not necessarily represent those of their affiliated organizations, or those of the publisher, the editors, and the reviewers. Any product that may be evaluated in this article, or claim that may be made by its manufacturer, is not guaranteed or endorsed by the publisher.
References
Allen, D. G., and Kurihara, S. (1982). The Effects of Muscle Length on Intracellular Calcium Transients in Mammalian Cardiac Muscle. J. Physiol. 327, 79–94. doi:10.1113/jphysiol.1982.sp014221
ASTM F19976-13 (2022). Standard Test Method for Impact Attenuation of Athletic Shoe Cushioning Systems and Materials. American Society of Testing Materials
ASTM F911-85 (1994). Standard Test Method for Flexibility of Running Shoes (Withdrawn 2002). American Society of Testing Materials
Barkema, D. D., Derrick, T. R., and Martin, P. E. (2012). Heel Height Affects Lower Extremity Frontal Plane Joint Moments during Walking. Gait Posture 35 (3), 483–488. doi:10.1016/j.gaitpost.2011.11.013
Barnes, K. R., and Kilding, A. E. (2019). A Randomized Crossover Study Investigating the Running Economy of Highly-Trained Male and Female Distance Runners in Marathon Racing Shoes versus Track Spikes. Sports Med. 49 (2), 331–342. doi:10.1007/s40279-018-1012-3
Baur, H., Hirschmüller, A., Cassel, M., Müller, S., and Mayer, F. (2010). Gender-Specific Neuromuscular Activity of the M. Peroneus Longus in Healthy Runners - A Descriptive Laboratory Study. Clin. Biomech. 25 (9), 938–943. doi:10.1016/j.clinbiomech.2010.06.009
Besson, T., Morio, C., Millet, G. Y., and Rossi, J. (2019). Influence of Shoe Drop on Running Kinematics and Kinetics in Female Runners. Eur. J. Sport Sci. 19, 1320–1327. doi:10.1080/17461391.2019.1603327
Bishop, C., Buckley, J. D., Esterman, A. E., and Arnold, J. B. (2020). The Running Shoe Comfort Assessment Tool (Run-cat): Development and Evaluation of a New Multi-Item Assessment Tool for Evaluating the Comfort of Running Footwear. J. Sports Sci. 38, 2100–2107. doi:10.1080/02640414.2020.1773613
Breine, B., Malcolm, P., Van Caekenberghe, I., Fiers, P., Frederick, E. C., and De Clercq, D. (2017). Initial Foot Contact and Related Kinematics Affect Impact Loading Rate in Running. J. Sports Sci. 35 (15), 1556–1564. doi:10.1080/02640414.2016.1225970
Cappozzo, A., Catani, F., Della Croce, U., and Leardini, A. (1995). Position and Orientation in Space of Bones during Movement: Anatomical Frame Definition and Determination. Clin. Biomech. 10 (4), 171–178. doi:10.1016/0268-0033(95)91394-T
Chambon, N., Delattre, N., Guéguen, N., Berton, E., and Rao, G. (2014). Shoe Drop Has Opposite Influence on Running Pattern when Running Overground or on a Treadmill. Eur. J. Appl. Physiol. 115 (5), 911–918. doi:10.1007/s00421-014-3072-x
Chambon, N., Delattre, N., Guéguen, N., Berton, E., and Rao, G. (2015). Shoe Drop Has Opposite Influence on Running Pattern when Running Overground or on a Treadmill. Eur. J. Appl. Physiol. 115 (5), 911–918. doi:10.1007/s00421-014-3072-x
Cheung, R. T. H., and Ng, G. Y. F. (2007). Efficacy of Motion Control Shoes for Reducing Excessive Rearfoot Motion in Fatigued Runners. Phys. Ther. Sport 8 (2), 75–81. doi:10.1016/j.ptsp.2006.12.002
Chien, H.-L., Lu, T.-W., and Liu, M.-W. (2013). Control of the Motion of the Body's Center of Mass in Relation to the Center of Pressure during High-Heeled Gait. Gait Posture 38, 391–396. doi:10.1016/j.gaitpost.2012.12.015
Csapo, R., Maganaris, C. N., Seynnes, O. R., and Narici, M. V. (2010). On Muscle, Tendon and High Heels. J. Exp. Biol. 213, 2582–2588. doi:10.1242/jeb.044271
Davis, I. S., Bowser, B. J., and Hamill, J. (2010). Vertical Impact Loading in Runners with a History of Patellofemoral Pain Syndrome. Med. Sci. Sports Exerc. 42, 682. doi:10.1249/01.mss.0000385903.65728.85
Dempster, J., Dutheil, F., and Ugbolue, U. C. (2021). The Prevalence of Lower Extremity Injuries in Running and Associated Risk Factors: A Systematic Review. Phys. Activity Health 5, 133–145. doi:10.5334/paah.109
Ferber, R., Davis, I. M., and Williams, D. S. (2003). Gender Differences in Lower Extremity Mechanics during Running. Clin. Biomech. (Bristol, Avon) 18 (4), 350–357. doi:10.1016/S0268-0033(03)00025-1
Fredericson, M., and Misra, A. K. (2007). Epidemiology and Aetiology of Marathon Running Injuries. Sports Med. 37, 437–439. doi:10.2165/00007256-200737040-00043
Gijon-Nogueron, G., Soler-Crespo, F. J., Sanchez-Rodriguez, R., Cabello-Marique, D., Lopezosa-Reca, E., and Ortega-Avila, A. B. (2019). Influence of Speed and Heel-To-Toe Drop in Running Shoes for Female Recreational Runners. Med. Baltim. 98 (19), e15649. doi:10.1097/MD.0000000000015649
Hasegawa, H., Yamauchi, T., and Kraemer, W. J. (2007). Foot Strike Patterns of Runners at the 15-km Point during an Elite-Level Half Marathon. J. Strength Cond. Res. 21, 888–893. doi:10.1519/R-22096.1
Heiderscheit, B. C., Hamill, J., and van Emmerik, R. E. A. (2002). Variability of Stride Characteristics and Joint Coordination Among Individuals with Unilateral Patellofemoral Pain. J. Appl. Biomech. 18 (2), 110–121. doi:10.1123/jab.18.2.110
Hennig, E. M., Milani, T. L., and Lafortune, M. A. (1993). Use of Ground Reaction Force Parameters in Predicting Peak Tibial Accelerations in Running. J. Appl. Biomech. 9 (4), 306–314. doi:10.1123/jab.9.4.306
Hessas, S., Behr, M., Rachedi, M., and Belaidi, I. (2017). Heel Lifts Stiffness of Sports Shoes Could Influence Posture and Gait Patterns. Sci. Sports 33 (2), e43–e50. doi:10.1016/j.scispo.2017.04.015
Hoogkamer, W., Kipp, S., Frank, J. H., Farina, E. M., Luo, G., and Kram, R. (2018). A Comparison of the Energetic Cost of Running in Marathon Racing Shoes. Sports Med. 48, 1009–1019. doi:10.1007/s40279-017-0811-2
Horton, M. G., and Hall, T. L. (1989). Quadriceps Femoris Muscle Angle: Normal Values and Relationships with Gender and Selected Skeletal Measures. Phys. Ther. 69, 897–901. doi:10.1093/ptj/69.11.897
Horvais, N., and Samozino, P. (2013). Effect of Midsole Geometry on Foot-Strike Pattern and Running Kinematics. Footwear Sci. 5 (2), 81–89. doi:10.1080/19424280.2013.767863
Hunter, I., Mcleod, A., Valentine, D., Low, T., Ward, J., and Hager, R. (2019). Running Economy, Mechanics, and Marathon Racing Shoes. J. Sports Sci. 37, 2367–2373. doi:10.1080/02640414.2019.1633837
Isherwood, J., Hughes, L., Qian, J. Y., and Sterzing, T. (2020). Biomechanical Effects of Continuous Loop Running in Comparison to Discontinuous Runway Running on Locomotion and Running Shoe Characterization. Footwear Sci. 12 (1), 39–54. doi:10.1080/19424280.2019.1696896
Jafarnezhadgero, A. A., Sorkhe, E., and Oliveira, A. S. (2019). Motion-Control Shoes Help Maintaining Low Loading Rate Levels during Fatiguing Running in Pronated Female Runners. Gait Posture 73, 65–70. doi:10.1016/j.gaitpost.2019.07.133
James, S. L., Bates, B. T., and Osternig, L. R. (1978). Injuries to Runners. Am. J. Sports Med. 6 (2), 40–50. doi:10.1177/036354657800600202
Kang, H. (2021). Sample Size Determination and Power Analysis Using the G*Power Software. J. Educ. Eval. Health Prof. 18, 17–12. doi:10.3352/jeehp.2021.18.17
Kerrigan, D. C., Franz, J. R., Keenan, G. S., Dicharry, J., Della Croce, U., and Wilder, R. P. (2009). The Effect of Running Shoes on Lower Extremity Joint Torques. PM R 1, 1058–1063. doi:10.1016/j.pmrj.2009.09.011
Kim, M. H., Yi, C. H., Yoo, W. G., and Choi, B. R. (2011). EMG and Kinematics Analysis of the Trunk and Lower Extremity during the Sit-To-Stand Task while Wearing Shoes with Different Heel Heights in Healthy Young Women. Hum. Mov. Sci. 30 (3), 596–605. doi:10.1016/j.humov.2010.09.003
Kong, P. W., Lim, C. Y., Ding, R., and Sterzing, T. (2015). Subjective Evaluation of Running Footwear Depends on Country and Assessment Method: A Bi-National Study. Ergonomics 58 (9), 1589–1604. doi:10.1080/00140139.2015.1018957
Larson, P., Higgins, E., Kaminski, J., Decker, T., Preble, J., Lyons, D., et al. (2011). Foot Strike Patterns of Recreational and Sub-Elite Runners in a Long-Distance Road Race. J. Sports Sci. 29, 1665–1673. doi:10.1080/02640414.2011.610347
Lieberman, D. E., Venkadesan, M., Werbel, W. A., Daoud, A. I., D’Andrea, S., Davis, I. S., et al. (2010). Foot Strike Patterns and Collision Forces in Habitually Barefoot Versus Shod Runners. Nature 463 (7280), 531–535. doi:10.1038/nature08723
Lilley, K., Stiles, V., and Dixon, S. (2013). The Influence of Motion Control Shoes on the Running Gait of Mature and Young Females. Gait Posture 37 (3), 331–335. doi:10.1016/j.gaitpost.2012.07.026
Malisoux, L., Chambon, N., Urhausen, A., and Theisen, D. (2016). Influence of the Heel-To-Toe Drop of Standard Cushioned Running Shoes on Injury Risk in Leisure-Time Runners. Am. J. Sports Med. 44 (11), 2933–2940. doi:10.1177/0363546516654690
Malisoux, L., Gette, P., Chambon, N., Urhausen, A., and Theisen, D. (2017). Adaptation of Running Pattern to the Drop of Standard Cushioned Shoes: A Randomised Controlled Trial with a 6-Month Follow-Up. J. Sci. Med. Sport 20 (8), 734–739. doi:10.1016/j.jsams.2017.01.238
Manal, K., Mcclay, I., Stanhope, S., Richards, J., and Galinat, B. (2000). Comparison of Surface Mounted Markers and Attachment Methods in Estimating Tibial Rotations during Walking: An In Vivo Study. Gait Posture 11 (4), 38–45. doi:10.1016/S0966-6362(99)00042-9
Mika, A., Oleksy, Ł., Mika, P., Marchewka, A., and Clark, B. C. (2012). The Influence of Heel Height on Lower Extremity Kinematics and Leg Muscle Activity during Gait in Young and Middle-Aged Women. Gait Posture 35 (4), 677–680. doi:10.1016/j.gaitpost.2011.12.001
Mills, K., Blanch, P., and Vicenzino, B. (2010). Identifying Clinically Meaningful Tools for Measuring Comfort Perception of Footwear. Med. Sci. Sports Exerc. 42, 1966–1971. doi:10.1249/MSS.0b013e3181dbacc8
Milner, C. E., Ferber, R., Pollard, C. D., Hamill, J., and Davis, I. S. (2006). Biomechanical Factors Associated with Tibial Stress Fracture in Female Runners. Med. Sci. Sports Exerc 38 (2), 323–328. doi:10.1249/01.mss.0000183477.75808.92
Mündermann, A., Nigg, B. M., Stefanyshyn, D. J., and Humble, R. N. (2002). Development of a Reliable Method to Assess Footwear Comfort during Running. Gait Posture 16 (1), 38–45. doi:10.1016/s0966-6362(01)00197-7
Napier, C., Maclean, C. L., Maurer, J., Taunton, J. E., and Hunt, M. A. (2018). Kinetic Risk Factors of Running-Related Injuries in Female Recreational Runners. Scand. J. Med. Sci. Sports 28 (10), 2164–2172. doi:10.1111/sms.13228
Nichols, T. E., and Holmes, A. P. (2002). Nonparametric Permutation Tests for Functional Neuroimaging: A Primer with Examples. Hum. Brain Mapp. 15 (1), 1–25. doi:10.1002/hbm.1058
Nigg, B., Baltich, J., Hoerzer, S., and Enders, H. (2015). Running Shoes and Running Injuries: Mythbusting and a Proposal for Two New Paradigms: 'preferred Movement Path' and 'comfort Filter'. Br. J. Sports Med. 49, 1290–1294. doi:10.1136/bjsports-2015-095054
Oh, K., and Park, S. (2017). The Bending Stiffness of Shoes is Beneficial to Running Energetics if it Does Not Disturb the Natural MTP Joint Flexion. J. Biomechanics 53, 127–135. doi:10.1016/j.jbiomech.2017.01.014
Paquette, M. R., Zhang, S., and Baumgartner, L. D. (2013). Acute Effects of Barefoot, Minimal Shoes and Running Shoes on Lower Limb Mechanics in Rear and Forefoot Strike Runners. Footwear Sci. 5 (1), 9–18. doi:10.1080/19424280.2012.692724
Pataky, T. C., Vanrenterghem, J., and Robinson, M. A. (2015). Zero- vs. One-Dimensional, Parametric vs. Non-parametric, and Confidence Interval vs. Hypothesis Testing Procedures in One-Dimensional Biomechanical Trajectory Analysis. J. Biomechanics 48, 1277–1285. doi:10.1016/j.jbiomech.2015.02.051
Pohl, M. B., Hamill, J., and Davis, I. S. (2009). Biomechanical and Anatomic Factors Associated with a History of Plantar Fasciitis in Female Runners. Clin. J. Sports Med. Off. J. Can. Acad. Sport Med. 19, 372–376. doi:10.1097/JSM.0b013e3181b8c270
Pontzer, H. (2007). Effective Limb Length and the Scaling of Locomotor Cost in Terrestrial Animals. J. Exp. Biol. 210, 1752–1761. doi:10.1242/jeb.002246
Richert, F. C., Stein, T., Ringhof, S., and Stetter, B. J. (2019). The Effect of the Heel-To-Toe Drop of Standard Running Shoes on Lower Limb Biomechanics. Footwear Sci. 11 (3), 161–170. doi:10.1080/19424280.2019.1630016
Roy, J.-P. R., and Stefanyshyn, D. J. (2006). Shoe Midsole Longitudinal Bending Stiffness and Running Economy, Joint Energy, and EMG. Med. Sci. Sports Exerc 38 (3), 562–569. doi:10.1249/01.mss.0000193562.22001.e8
Silva, A. M., Siqueira, G. R. D., and Silva, G. A. P. D. (2013). Implications of High Heeled Shoes on Body Posture of Adolescents. Rev. Paul. Pediatr. 31 (2), 265–271. doi:10.1590/S0103-05822013000200020
Sinclair, J., Greenhalgh, A., Brooks, D., Edmundson, C. J., and Hobbs, S. J. (2012). The Influence of Barefoot and Barefoot-Inspired Footwear on the Kinetics and Kinematics of Running in Comparison to Conventional Running Shoes. Footwear Sci. 5, 45–53. doi:10.1080/19424280.2012.693543
Smith, G., Lake, M., and Lees, A. (2014). Metatarsophalangeal Joint Function during Sprinting: A Comparison of Barefoot and Sprint Spike Shod Foot Conditions. J. Appl. Biomech. 30, 206–212. doi:10.1123/jab.2013-0072
Sterzing, T., Custoza, G., Ding, R., and Cheung, J. T.-M. (2015). Segmented Midsole Hardness in the Midfoot to Forefoot Region of Running Shoes Alters Subjective Perception and Biomechanics during Heel-Toe Running Revealing Potential to Enhance Footwear. Footwear Sci. 7 (2), 63–79. doi:10.1080/19424280.2015.1008589
Taunton, J. E., Ryan, M. B., Clement, D. B., McKenzie, D. C., LloydSmith, D. R., and Zumbo, B. D. (2002). A Retrospective Case-Control Analysis of 2002 Running Injuries. Br. J. Sports Med. 36, 95–101. doi:10.1136/bjsm.36.2.95
Tay, C. S., Sterzing, T., Lim, C. Y., Ding, R., and Kong, P. W. (2017). Overall Preference of Running Shoes Can Be Predicted by Suitable Perception Factors Using a Multiple Regression Model. Hum. Factors 59 (3), 432–441. doi:10.1177/0018720816681147
Tung, K. D., Franz, J. R., and Kram, R. (2014). A Test of the Metabolic Cost of Cushioning Hypothesis during Unshod and Shod Running. Sci. Sports Exerc. 46, 324–329. doi:10.1249/MSS.0b013e3182a63b81
Ueda, T., Hobara, H., Kobayashi, Y., Heldoorn, T., Mochimaru, M., and Mizoguchi, H. (2016). Comparison of 3 Methods for Computing Loading Rate during Running. Int. J. Sports Med. 37, 1087–1090. doi:10.1055/s-0042-107248
van der Worp, H., Vrielink, J. W., and Bredeweg, S. W. (2016). Do runners Who Suffer Injuries Have Higher Vertical Ground Reaction Forces Than Those Who Remain Injury-free? A Systematic Review and Meta-Analysis. Br. J. Sports Med. 50 (8), 450–457. doi:10.1136/bjsports-2015-094924
Wilder, B. (2014). On the Run: A Physician's Perspective. J. Orthop. Sports Phys. Ther. 44, 731–732. doi:10.2519/jospt.2014.0116
Willwacher, S., König, M., Potthast, W., and Brüggemann, G.-P. (2013). Does Specific Footwear Facilitate Energy Storage and Return at the Metatarsophalangeal Joint in Running? J. Appl. Biomech. 29, 583–592. doi:10.1016/j.scispo.2013.02.00310.1123/jab.29.5.583
Xiang, L., Mei, Q., Wang, A., Shim, V., Fernandez, J., and Gu, Y. (2022). Evaluating Function in the Hallux Valgus Foot Following a 12-Week Minimalist Footwear Intervention: A Pilot Computational Analysis. J. Biomechanics 132, 110941. doi:10.1016/j.jbiomech.2022.110941
Keywords: innovation shoes, shoe drop, running, biomechanics, women
Citation: Fu F, Guo L, Tang X, Wang J, Xie Z, Fekete G, Cai Y, Hu Q and Gu Y (2022) Effect of the Innovative Running Shoes With the Special Midsole Structure on the Female Runners’ Lower Limb Biomechanics. Front. Bioeng. Biotechnol. 10:866321. doi: 10.3389/fbioe.2022.866321
Received: 31 January 2022; Accepted: 27 April 2022;
Published: 06 June 2022.
Edited by:
Veronica Cimolin, Politecnico di Milano, ItalyReviewed by:
AmirAli Jafarnezhadgero, University of Mohaghegh Ardabili, IranCheng-Feng Lin, National Cheng Kung University, Taiwan
Copyright © 2022 Fu, Guo, Tang, Wang, Xie, Fekete, Cai, Hu and Gu. This is an open-access article distributed under the terms of the Creative Commons Attribution License (CC BY). The use, distribution or reproduction in other forums is permitted, provided the original author(s) and the copyright owner(s) are credited and that the original publication in this journal is cited, in accordance with accepted academic practice. No use, distribution or reproduction is permitted which does not comply with these terms.
*Correspondence: Yaodong Gu, guyaodong@nbu.edu.cn