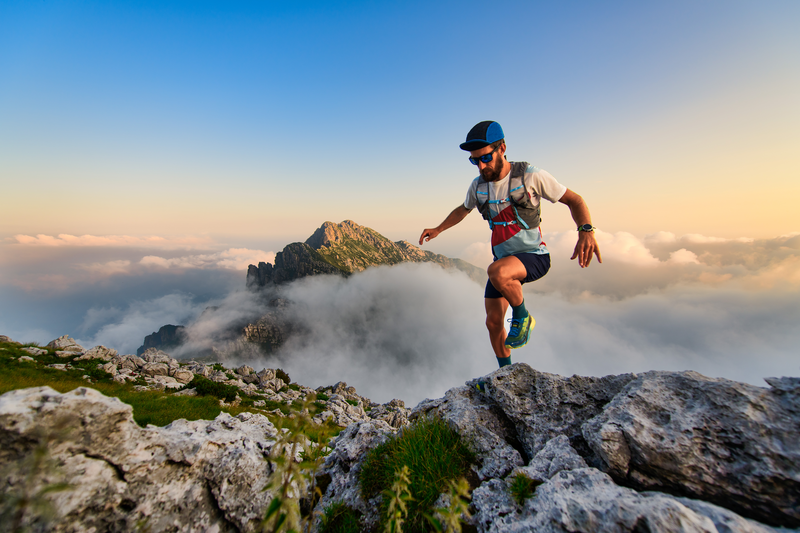
95% of researchers rate our articles as excellent or good
Learn more about the work of our research integrity team to safeguard the quality of each article we publish.
Find out more
PERSPECTIVE article
Front. Bioeng. Biotechnol. , 04 April 2022
Sec. Tissue Engineering and Regenerative Medicine
Volume 10 - 2022 | https://doi.org/10.3389/fbioe.2022.855095
This article is part of the Research Topic Insights in Tissue Engineering and Regenerative Medicine 2021: Novel Developments, Current Challenges, and Future Perspectives View all 14 articles
Mesenchymal stromal cells (MSC) represent a promising treatment option for tendon disorders and joint diseases, primarily osteoarthritis. Since MSC are highly context-sensitive to their microenvironment, their therapeutic efficacy is influenced by their tissue-specific pathologically altered targets. These include not only cellular components, such as resident cells and invading immunocompetent cells, but also components of the tissue-characteristic extracellular matrix. Although numerous in vitro models have already shown potential MSC-related mechanisms of action in tendon and joint diseases, only a limited number reflect the disease-specific microenvironment and allow conclusions about well-directed MSC-based therapies for injured tendon and joint-associated tissues. In both injured tissue types, inflammatory processes play a pivotal pathophysiological role. In this context, MSC-mediated macrophage modulation seems to be an important mode of action across these tissues. Additional target cells of MSC applied in tendon and joint disorders include tenocytes, synoviocytes as well as other invading and resident immune cells. It remains of critical importance whether the context-sensitive interplay between MSC and tissue- and disease-specific targets results in an overall promotion or inhibition of the desired therapeutic effects. This review presents the authors’ viewpoint on disease-related targets of MSC therapeutically applied in tendon and joint diseases, focusing on the equine patient as valid animal model.
The former idea behind the application of mesenchymal stromal cells (MSC) in injured tissue was to transplant a source of undifferentiated progenitor cells and thereby achieve local effects, which were deemed to be a direct integration of MSC, further leading to restoration and regeneration at the site of injury. In musculoskeletal conditions, this is supported by studies investigating the retention and fate of locally applied MSC and by the repeated proof of MSC differentiation potential into osteogenic, chondrogenic, adipogenic and myogenic cell lines (Forest et al., 2010; Sole et al., 2013; Vieira et al., 2014). However, this assumption is more and more supplemented by findings about the ability of MSC to communicate transcellularly by direct cell-to-cell contact and release of soluble factors and extracellular vesicles (Islam et al., 2012; Liu et al., 2018; Witwer et al., 2019). With the knowledge of these transcellular communication mechanisms, the idea of a locally acting MSC requires a broader explanation than the plausible cell replacement theory alone. Moreover, locally applied MSC seem to be adaptive and promote context-sensitive cell communication. To predict MSC-target interaction, the cell and matrix composition and immunological state of the target tissue are pivotal. However, due to the complex pathophysiological mechanisms in the course of inflammation, cell and matrix destruction as characteristics at the site of injury are inconsistent, depending on the type of tissue, the grade of cell destruction and the grade of local inflammatory reaction (Ackerman et al., 2021; Scanzello and Goldring, 2021). Furthermore, though not being addressed within the present work, tissue specific resident MSC have already been shown to react context-sensitive in the respective pathophysiological microenvironment and may also be relevant for target interaction of applied MSC (Costa-Almeida et al., 2019).
Animal models play a vital role in providing information about tissue-context-sensitivity of MSC. Particularly the clarification of the MSC mode of action in injured tendons and joints forced scientists to study artificially induced lesions in various species (Delling et al., 2015; Ahrberg et al., 2018; Kwon et al., 2018; Kim et al., 2019; Khan et al., 2020). Nevertheless, all artificial disease models remain an approximation and do not allow to investigate the naturally occurring pathophysiological circumstances. Therefore, we consider it crucial to include animal models based on naturally occurring tendon and joint diseases in basic research strategies, for which the horse is most suitable and repeatedly used (Becerra et al., 2013; Smith et al., 2013; Berner et al., 2016; Broeckx et al., 2019). However, the so far limited number of existing pre-clinical and clinical studies focus on clinically detectable effects and safety, thereby only indirect conclusions about target specific cellular mechanisms of action can be drawn.
Understanding the context-sensitive mode of action of MSC specifically in tendons and joints will form the basis for their future successful application. Here, we discuss selected therapeutic targets and mechanisms of MSC applications in tendon and joint diseases and illustrate their mutual interplay. Due to its high relevance with respect to investigating naturally occurring diseases, we focus on research in the equine model.
The desired targets of MSC in therapeutic applications can be deviated from the pathophysiological mechanisms driving the respective disease. However, it is important to note that the desired targets are not necessarily the targets that are actually addressed, and even if so, the effect on the target might not be as initially expected. In this line, it must be considered that MSC are highly sensitive to their environment, which will be further addressed below. Nevertheless, aiming at a well-directed cell therapy, the first step must be to identify pathophysiological key players and to observe the effects of MSC after transplantation.
Possible targets of MSC in tendon disease include cellular components on the one hand, such as the resident tenocytes, invading immune cells and endothelial cells, and the extracellular matrix (ECM) on the other hand. Regeneration of the highly specialized matrix must always be the central goal, as it is responsible for tendon function. However, a functional replacement of cells and ECM by the MSC alone is unlikely to be achieved. Rather, the MSC should support a milieu which allows the tendon to regenerate. In this line, the cellular targets could be in the foreground in phases of active inflammation and acute injury, whereas the scarred ECM may need to be focused in chronic tendon disease.
In acute tendon disease or injury, activated tenocytes and invading immune cells, namely macrophages, foster a milieu of inflammation and further matrix degradation. Indeed, in a canine model of acute tendon injury, macrophages could be identified as a target of MSC treatment, which promoted their M1/M2 phenotype switch (Shen et al., 2016; Gelberman et al., 2017). While the identification of the underlying molecular mechanisms and targets is more complex, this effect appeared to be mediated by interleukin (IL)-4 (Shen et al., 2016; Gelberman et al., 2017). The specific interplay of tenocytes and MSC in vivo has not been elucidated in much detail so far, yet besides direct tenocyte targeting, a protection of tenocytes via macrophage modulation and overall reduction of pro-inflammatory stimuli can be assumed (Manning et al., 2015). Furthermore, neovascularization plays a critical and often controversially discussed role in tendon healing, as it is crucial to successful healing but persistently increased vascularization negatively impacts long-term outcomes (Korntner et al., 2019; Liu et al., 2021). A transiently increased vascularization has repeatedly been observed as a response to MSC treatment of equine tendon lesions (Conze et al., 2014; Ahrberg et al., 2018). This suggests that vascular endothelial cells are targeted in a beneficial way, which likely is related to increased vascular endothelial growth factor (VEGF) levels (Okamoto et al., 2010; Yuksel et al., 2016).
In chronic tendon disease, failed ECM repair has led to scar tissue within the tendon. Its inferior biomechanical properties predispose to re-injury and its altered biophysical and biochemical properties fail to provide the appropriate guidance for regeneration to resident cells. Therefore, it appears advantageous to target the matrix remodeling process, with enzymatic degradation of scar matrix components and promotion of collagen I fibrillogenesis and cross-linking. So far, some insights into MSC-driven matrix remodeling in vivo can be deduced from studies dealing with acute equine tendon disease, but suitable models for chronic disease are lacking. Here, it could not only be shown that the tendon matrix has an improved architecture and composition after MSC injection (Schnabel et al., 2009; Crovace et al., 2010; Smith et al., 2013), but also that the treatment reduced collagenase [matrix metalloproteinase (MMP)13] activity (Smith et al., 2013) and upregulated stromelysin (MMP3) gene expression (Romero et al., 2017). This might indicate a selective and beneficial support of enzymatic tendon matrix remodeling, but data are still scarce and, importantly, these results were obtained after treatment of (sub)acute lesions but not after treatment of chronic disease.
Osteoarthritis (OA) was originally understood as a classical “wear and tear” disease, mainly related to degenerative changes in the articular cartilage. However, nowadays OA is referred to as a complex, multisystem disease affecting the whole joint and detailed pathophysiological mechanisms are still not completely understood. Nevertheless, it is beyond doubt that inflammatory processes in the synovial membrane play a crucial role in the initiation of OA and in promoting subsequent cartilage damage and pain at least in the inflammation-driven subset of patients (de Lange-Brokaar et al., 2012). Therefore, the previously suggested differentiation potential of MSC into chondrocytes aiding cartilage regeneration (Satué et al., 2019; Song et al., 2021) was pushed into the background against the ability of the MSC to target local immunocompetent cells.
OA-related synovitis is mainly driven by the innate immune system, which should therefore be targeted by MSC therapies, again with an outstanding role of macrophages. The macrophages potentially targeted by MSC include invading pro-inflammatory monocyte-derived macrophages within the synovial fluid and adjacent joint tissue as well as resident immunomodulatory synovial macrophages consisting of several subgroups of cells (van den Bosch, 2021). Besides their pivotal role in OA-related synovitis, synovial macrophages are considered critical for tissue homeostasis, thus being reasonably involved in the re-establishment of immune homeostasis within the injured joint. Recent studies in the mouse model indicate different roles of invading monocyte-derived and tissue resident synovial macrophages during homeostasis as well as disease. It was shown that locally renewing resident macrophages within the inner synovial lining form membrane-like structures as protective physical barrier between the intraarticular space and the synovial capillary network (Culemann et al., 2019). These resident synovial macrophages maintain their immune-regulatory function even within an inflammatory environment. However, the specific pathogenetic role of these cells has not yet been addressed in OA (Haubruck et al., 2021). Tracking ferumoxytol-labeled murine MSC in a model of induced OA, MSC-treated joints were reported to show a reduced number of pro-inflammatory macrophages in favor of an increased proportion of homeostatic polarized macrophages (Hamilton et al., 2019). In co-culture models, it has been shown that MSC reduce M1-like-activating factors such as IL-1β and tumor necrosis factor (TNF)-α and induce typical M2-like macrophage markers such as IL-10, cluster of differentiation (CD)163 and CD206, partially through the prostaglandin E2/cyclooxygenase two pathway. Since this was shown in contact as well as in trans-well cultures, the authors suggest different MSC-mediated phases of immunomodulation including firstly an interaction via soluble factors and secondly a direct adhesion to the synovium (Manferdini et al., 2017). Yet so far, it remains to be understood whether applied MSC rather support tissue-resident macrophages or regulate primarily the inflammatory phenotype of the invading, mainly pro-inflammatory macrophages. In vivo and ex vivo models, ideally based on naturally occurring OA, could shed more light on these MSC-macrophage interactions.
Polymorphonuclear cells could represent a further target for MSC applied during OA-related synovitis. In a murine model of induced OA of the knee, locally applied MSC attracted colocalizing polymorphonuclear cells within the synovium. This was likely due to an IL-1β-mediated increased chemokine release of the applied MSC and led to the up-regulation of the phagocytic activity as well as the down-regulation of the pro-inflammatory cytokine release of the locally clustered polymorphonuclear cells (Van Dalen et al., 2019). This upregulation of the phagocytic activity might contribute to the removal of cartilage fragments from the synovial fluid, thus breaking the vicious inflammatory circle in OA.
MSCare highly sensitive to their environment, which entails a mutual interplay between the transplanted cells and their pathologically altered targets. The latter represent a crucial part of the disease milieu that will influence the MSC once transplanted (Table 1). It remains a central question whether their adaptation to the disease milieu leads to a promotion or inhibition of the desired effects on the respective targets.
TABLE 1. Overview of microenvironmental factors and their effect on MSC potential mechanisms of action in tendon and joint disease.
Inflammatory conditions, which are often present in tendon as well as joint disease, are well-known to impact on MSC. MSC harvested from inflammatory environments show a decreased and variable fitness, which we have demonstrated for equine synovial fluid-derived MSC from osteoarthritic joints (Burk et al., 2017). In humans, the inflammatory state of synovial fluid in OA-affected knees modulates not only the proliferation of synovial fluid-derived MSC, but also induced a reduced differentiation potential, which is suggested to result in a lower ability to reverse OA (de Sousa et al., 2019). Additionally, MSC from discarded articular cartilage collected from OA patients during joint replacement therapy showed a rapid and strong mineralization upon chondrogenic induction, while markers of chondrocyte hypertrophy and stem cell osteogenesis were induced. These complex mechanisms demonstrate that MSC differentiation within the inflammatory environment might be coupled with undesired chondrocyte hypertrophy and osteogenesis (Hu et al., 2019).
Subjecting healthy MSC to inflammatory conditions impacts on their mode of action. This includes a decreased differentiation potential but can, up to a certain extent of inflammation, promote MSC immunomodulatory and protective mechanisms. With regard to tenogenic differentiation, we have shown that not only the presence of pro-inflammatory cytokines, namely IL-1β, but also the presence of leukocytes decreased the expression of the tendon transcription factor scleraxis in equine MSC (Brandt et al., 2018). Similarly, chondrogenic differentiation in pellet culture was decreased in the presence of the pro-inflammatory cytokines (Brandt et al., 2018), corresponding to findings in human MSC (Kondo et al., 2013; Liu et al., 2017). On the other hand, interestingly, chondrogenically differentiated equine MSC responded less to IL-1β stimulation than their naïve counterparts (Bundgaard et al., 2020). However, most likely more important than differentiation, pro-inflammatory stimulation has repeatedly been shown to increase MSC immunomodulatory potential. In a co-culture model using equine MSC and stimulated or non-stimulated leukocytes, we could show that the modulatory MSC mechanisms depended on the extent of inflammatory stimulation. Mild inflammatory conditions increased the percentage of MSC synthesizing the anti-inflammatory IL-10, while stronger inflammatory conditions promoted the regulatory effects of MSC on T cells, possibly via prostaglandin E2. However, not all effects observed in strong inflammatory conditions were strictly anti-inflammatory (Hillmann et al., 2019). With respect to joint disease, recent data has shown that synovial fluid collected from OA-affected joints influences the immunomodulatory properties of the MSC secretome and thereby promotes an anti-inflammatory subset of immune cells including an enhanced macrophage polarization into the M2-like phenotype (Cifù et al., 2020). Hypoxic conditions strongly influenced the migration and cytokine receptor expression of MSC cultured in synovial fluid collected from OA patients (Manferdini et al., 2020).
Extracellular matrices also have a strong impact on MSC properties and behavior (Li et al., 2021). However, the effects of pathologically altered ECM on MSC are still widely unknown, despite their relevance for treating chronic fibrotic conditions, attempting to specifically target the ECM. We observed that culturing equine MSC on decellularized tendon ECM failed to display synergistic effects with the tenogenic transforming growth factor (TGF)-β3 (Roth et al., 2018). This may be due to inhibitory effects of integrin/Rho/Rho-associated protein kinase (Rho/ROCK) axis activation by the extracellular matrix on canonical TGF-β3/smad2/3 signaling (Melzer et al., 2021), providing an example of cell-ECM interactions that could interfere with assumed mechanisms. Recently, we could also demonstrate that MSC undergo pathological adaptions upon exposure to scarred ECM. When culturing equine MSC on decellularized tendon matrices obtained from tendons with naturally occurred chronic disease, tenogenic differentiation was evident despite the ECM alterations in the tendon matrix, but the gene expression and activity of MMP was decreased (Doll et al., 2021). This effect was transient, but could hamper effective targeting of the scar tissue within the ECM.
Our growing understanding of pathophysiology and MSC behavior will promote the development of the next generation of MSC-based therapies. However, investigating target and therapeutic cells should go hand in hand and reflects naturally occurring disease. Deciphering the mutual interplay between MSC and their targets in relevant disease environments could provide the missing link for consistent therapeutic success. Recently, an interesting step in this direction was taken with the development of a bioassay, by which the effect of a patient’s OA joint microenvironment on the ability of MSC to support cartilage formation could be deduced. MSC-based cartilage formation was modified by the OA joint microenvironment, which could be useful to predict the therapeutic outcome (Neefjes et al., 2021). Such approaches may help to identify “non-responders” in advance and eventually lead to personalized OA treatments. Nevertheless, further strategies to deal with putative non-responders will still be required.
MSC priming, a promising strategy to enhance MSC potency and efficacy, directly results from the context-sensitive nature of the MSC, and aims to train the cells for their therapeutic task by subjecting them to pathophysiological stimuli. So far, this strategy has mainly been investigated with regard to inflammatory priming or “licensing” to enhance MSC immunomodulatory potential. For example, equine bone marrow-derived MSC primed with interferon (IFN)-γ were similarly activated as by co-culture with M1-polarized macrophages (Cassano et al., 2018). As the effects of IFN-γ were more consistent and also led to a chondroprotective secretome, the authors suggested that MSC priming before transplantation could be more successful than having the MSC activated by the pathological in vivo environment (Cassano et al., 2018), i.e., by their cellular targets, alone. In this line, in an equine model of chemically induced OA of the radio-carpal joint, inflammatory priming with TNF-α and IFN-γ led to an increased anti-inflammatory and regulatory effect of applied MSC. However, the repeated intraarticular application of inflammatory primed allogeneic MSC resulted in a slight transient inflammatory response, possibly indicative for an increased immunogenicity of primed MSC (Barrachina et al., 2018). This must be carefully considered, along with the risk of exacerbation upon excessive stimulation, which might affect therapeutic safety of primed MSC. So far, a therapy with non-primed MSC, which naturally adapt to the disease environment, appears to be sufficient to target the immune cells in most patients and represents the safer option until more knowledge is available. However, MSC priming may help non-responding patients and this concept in general appears highly valuable for future therapies.
Aiming to further improve MSC priming approaches, it will be interesting whether priming regimes can be tailored for specific targeting of certain cell types or ECM components. With respect to tendon and OA therapies, macrophages remain the most promising cell type to modulate or alter their functional phenotype, as key regulatory cells in tendon as well as OA-related inflammation. However, little is known about the conditions of the disease environment in which MSC target inflammation and decrease the pro-inflammatory state of macrophages at their best (van den Bosch, 2021). Therefore, pre-treatment options to improve MSC efficacy by targeted influencing of tendon and joint-associated macrophages should be set on the scientific agenda. This implies optimizing MSC and target macrophage communication applicable for different disease stages, including conditions with an unfavourable environment such as the exacerbated OA-related synovitis.
We conclude that the mode of action of locally applied MSC is influenced by the cellular and molecular microenvironment at the injured site and vice versa. In this context, MSC-mediated macrophage modulation represents a key tool to positively influence inflammation in injured tendons and joints. However, a broad range of additional target cells as well as the ECM also have to be addressed. The best possible outcome for any MSC recipient will be achieved when the target tissue is characterized and the applied MSC, including potential priming, are matched with each other as specifically as possible.
The original contributions presented in the study are included in the article/Supplementary Material, further inquiries can be directed to the corresponding author.
AT, JB, and SR constructed the manuscript. AT, JB, SR, and WB edited the manuscript. All authors contributed to the article and approved the submitted version.
This work was partially funded by the a grant from the Leipzig veterinary junior scientist support program financed by the “Freundeskreis Tiermedizin” and the Faculty of Veterinary Medicine, University of Leipzig.
The authors declare that the research was conducted in the absence of any commercial or financial relationships that could be construed as a potential conflict of interest.
All claims expressed in this article are solely those of the authors and do not necessarily represent those of their affiliated organizations, or those of the publisher, the editors and the reviewers. Any product that may be evaluated in this article, or claim that may be made by its manufacturer, is not guaranteed or endorsed by the publisher.
Ackerman, J. E., Best, K. T., Muscat, S. N., and Loiselle, A. E. (2021). Metabolic Regulation of Tendon Inflammation and Healing Following Injury. Curr. Rheumatol. Rep. 23, 15. doi:10.1007/s11926-021-00981-4
Ahrberg, A. B., Horstmeier, C., Berner, D., Brehm, W., Gittel, C., Hillmann, A., et al. (2018). Effects of Mesenchymal Stromal Cells versus Serum on Tendon Healing in a Controlled Experimental Trial in an Equine Model. BMC Musculoskelet. Disord. 19, 1–14. doi:10.1186/s12891-018-2163-y
Barrachina, L., Remacha, A. R., Romero, A., Vitoria, A., Albareda, J., Prades, M., et al. (2018). Assessment of Effectiveness and Safety of Repeat Administration of Proinflammatory Primed Allogeneic Mesenchymal Stem Cells in an Equine Model of Chemically Induced Osteoarthritis. BMC Vet. Res. 14, 1–17. doi:10.1186/s12917-018-1556-3
Becerra, P., Valdés Vázquez, M. A., Dudhia, J., Fiske‐Jackson, A. R., Neves, F., Hartman, N. G., et al. (2013). Distribution of Injected Technetium99m‐labeled Mesenchymal Stem Cells in Horses with Naturally Occurring Tendinopathy. J. Orthop. Res. 31, 1096–1102. doi:10.1002/jor.22338
Berner, D., Brehm, W., Gerlach, K., Gittel, C., Offhaus, J., Paebst, F., et al. (2016). Longitudinal Cell Tracking and Simultaneous Monitoring of Tissue Regeneration after Cell Treatment of Natural Tendon Disease by Low-Field Magnetic Resonance Imaging. Stem Cell Int. 2016, 1–13. doi:10.1155/2016/1207190
Brandt, L., Schubert, S., Scheibe, P., Brehm, W., Franzen, J., Gross, C., et al. (2018). Tenogenic Properties of Mesenchymal Progenitor Cells Are Compromised in an Inflammatory Environment. Ijms 19, 2549–2626. doi:10.3390/ijms19092549
Broeckx, S. Y., Seys, B., Suls, M., Vandenberghe, A., Mariën, T., Adriaensen, E., et al. (2019). Equine Allogeneic Chondrogenic Induced Mesenchymal Stem Cells Are an Effective Treatment for Degenerative Joint Disease in Horses. Stem Cell Dev. 28, 410–422. doi:10.1089/scd.2018.0061
Bundgaard, L., Stensballe, A., Elbæk, K. J., and Berg, L. C. (2020). Mass Spectrometric Analysis of the In Vitro Secretome from Equine Bone Marrow-Derived Mesenchymal Stromal Cells to Assess the Effect of Chondrogenic Differentiation on Response to Interleukin-1β Treatment. Stem Cel Res. Ther. 11, 1. doi:10.1186/s13287-020-01706-7
Burk, J., Glauche, S. M., Brehm, W., Crovace, A., Francioso, E., Hillmann, A., et al. (2017). Characterisation and Intracellular Labelling of Mesenchymal Stromal Cells Derived from Synovial Fluid of Horses and Sheep. Vet. J. 222, 1–8. doi:10.1016/j.tvjl.2017.02.006
Cassano, J. M., Schnabel, L. V., Goodale, M. B., and Fortier, L. A. (2018). Inflammatory Licensed Equine MSCs Are Chondroprotective and Exhibit Enhanced Immunomodulation in an Inflammatory Environment. Stem Cel Res. Ther. 9, 1–13. doi:10.1186/s13287-018-0840-2
Cifù, A., Domenis, R., Pozzi-Mucelli, M., Di Benedetto, P., Causero, A., Moretti, M., et al. (20202020). The Exposure to Osteoarthritic Synovial Fluid Enhances the Immunomodulatory Profile of Adipose Mesenchymal Stem Cell Secretome. Stem Cell Int. 2020, 1–13. doi:10.1155/2020/4058760
Conze, P., van Schie, H. T., Weeren, R. v., Staszyk, C., Conrad, S., Skutella, T., et al. (2014). Effect of Autologous Adipose Tissue-Derived Mesenchymal Stem Cells on Neovascularization of Artificial Equine Tendon Lesions. Regenerative Med. 9, 743–757. doi:10.2217/rme.14.55
Costa-Almeida, R., Calejo, I., and Gomes, M. E. (2019). Mesenchymal Stem Cells Empowering Tendon Regenerative Therapies. Ijms 20, 3002. doi:10.3390/ijms20123002
Crovace, A., Lacitignola, L., Rossi, G., and Francioso, E. (2010). Histological and Immunohistochemical Evaluation of Autologous Cultured Bone Marrow Mesenchymal Stem Cells and Bone Marrow Mononucleated Cells in Collagenase-Induced Tendinitis of Equine Superficial Digital Flexor Tendon. Vet. Med. Int. 2010, 1–10. doi:10.4061/2010/250978
Culemann, S., Grüneboom, A., Nicolás-Ávila, J. Á., Weidner, D., Lämmle, K. F., Rothe, T., et al. (2019). Locally Renewing Resident Synovial Macrophages Provide a Protective Barrier for the Joint. Nature 572, 670–675. doi:10.1038/s41586-019-1471-1
de Lange-Brokaar, B. J. E., Ioan-Facsinay, A., van Osch, G. J. V. M., Zuurmond, A.-M., Schoones, J., Toes, R. E. M., et al. (2012). Synovial Inflammation, Immune Cells and Their Cytokines in Osteoarthritis: A Review. Osteoarthritis and Cartilage 20, 1484–1499. doi:10.1016/j.joca.2012.08.027
de Sousa, E. B., dos Santos Junior, G. C., Aguiar, R. P., da Costa Sartore, R., de Oliveira, A. C. L., Almeida, F. C. L., et al. (2019). Osteoarthritic Synovial Fluid Modulates Cell Phenotype and Metabolic BehaviorIn Vitro. Stem Cell Int. 2019, 1–14. doi:10.1155/2019/8169172
Delling, U., Brehm, W., Ludewig, E., Winter, K., and Jülke, H. (2015). Longitudinal Evaluation of Effects of Intra-articular Mesenchymal Stromal Cell Administration for the Treatment of Osteoarthritis in an Ovine Model. Cel Transpl. 24, 2391–2407. doi:10.3727/096368915X686193
Doll, C. U., Niebert, S., and Burk, J. (2021). Mesenchymal Stromal Cells Adapt to Chronic Tendon Disease Environment with an Initial Reduction in Matrix Remodeling. Ijms 22, 12798. doi:10.3390/ijms222312798
Forest, V. F., Tirouvanziam, A. M., Perigaud, C., Fernandes, S., Fusellier, M. S., Desfontis, J.-C., et al. (2010). Cell Distribution after Intracoronary Bone Marrow Stem Cell Delivery in Damaged and Undamaged Myocardium: Implications for Clinical Trials. Stem Cel Res. Ther. 1, 1–11. doi:10.1186/scrt4
Gelberman, R. H., Linderman, S. W., Jayaram, R., Dikina, A. D., Sakiyama-Elbert, S., Alsberg, E., et al. (2017). Combined Administration of ASCs and BMP-12 Promotes an M2 Macrophage Phenotype and Enhances Tendon Healing. Clin. Orthop. Relat. Res. 475, 2318–2331. doi:10.1007/s11999-017-5369-7
Hamilton, A. M., Cheung, W.-Y., Gómez-Aristizábal, A., Sharma, A., Nakamura, S., Chaboureau, A., et al. (2019). Iron Nanoparticle-Labeled Murine Mesenchymal Stromal Cells in an Osteoarthritic Model Persists and Suggests Anti-inflammatory Mechanism of Action. PLoS One 14, e0214107–20. doi:10.1371/journal.pone.0214107
Haubruck, P., Pinto, M. M., Moradi, B., Little, C. B., and Gentek, R. (2021). Monocytes, Macrophages, and Their Potential Niches in Synovial Joints - Therapeutic Targets in Post-Traumatic Osteoarthritis? Front. Immunol. 12, 1–26. doi:10.3389/fimmu.2021.763702
Hillmann, A., Paebst, F., Brehm, W., Piehler, D., Schubert, S., Tárnok, A., et al. (2019). A Novel Direct Co-culture Assay Analyzed by Multicolor Flow Cytometry Reveals Context- and Cell Type-specific Immunomodulatory Effects of Equine Mesenchymal Stromal Cells. PLoS One 14, e0218949–21. doi:10.1371/journal.pone.0218949
Hu, N., Gao, Y., Jayasuriya, C. T., Liu, W., Du, H., Ding, J., et al. (2019). Chondrogenic Induction of Human Osteoarthritic Cartilage-Derived Mesenchymal Stem Cells Activates Mineralization and Hypertrophic and Osteogenic Gene Expression through a mechanomiR. Arthritis Res. Ther. 21, 1–14. doi:10.1186/s13075-019-1949-0
Islam, M. N., Das, S. R., Emin, M. T., Wei, M., Sun, L., Westphalen, K., et al. (2012). Mitochondrial Transfer from Bone-Marrow-Derived Stromal Cells to Pulmonary Alveoli Protects against Acute Lung Injury. Nat. Med. 18, 759–765. doi:10.1038/nm.2736
Khan, M. R., Smith, R. K., David, F., Lam, R., Hughes, G., Godoy, R., et al. (2020). Evaluation of the Effects of Synovial Multipotent Cells on Deep Digital Flexor Tendon Repair in a Large Animal Model of Intra‐Synovial Tendinopathy. J. Orthop. Res. 38, 128–138. doi:10.1002/jor.24423
Kim, H., Yang, G., Park, J., Choi, J., Kang, E., and Lee, B.-K. (2019). Therapeutic Effect of Mesenchymal Stem Cells Derived from Human Umbilical Cord in Rabbit Temporomandibular Joint Model of Osteoarthritis. Sci. Rep. 9, 1–14. doi:10.1038/s41598-019-50435-2
Kondo, M., Yamaoka, K., Sonomoto, K., Fukuyo, S., Oshita, K., Okada, Y., et al. (2013). IL-17 Inhibits Chondrogenic Differentiation of Human Mesenchymal Stem Cells. PLoS One 8, e79463. doi:10.1371/journal.pone.0079463
Korntner, S., Lehner, C., Gehwolf, R., Wagner, A., Grütz, M., Kunkel, N., et al. (2019). Limiting Angiogenesis to Modulate Scar Formation. Adv. Drug Deliv. Rev. 146, 170–189. doi:10.1016/j.addr.2018.02.010
Kwon, D. R., Park, G.-Y., and Lee, S. C. (2018). Treatment of Full-Thickness Rotator Cuff Tendon Tear Using Umbilical Cord Blood-Derived Mesenchymal Stem Cells and Polydeoxyribonucleotides in a Rabbit Model. Stem Cell Int. 2018, 1–11. doi:10.1155/2018/7146384
Li, J., Liu, Y., Zhang, Y., Yao, B., Enhejirigala, B., Li, Z., et al. (2021). Biophysical and Biochemical Cues of Biomaterials Guide Mesenchymal Stem Cell Behaviors. Front. Cel Dev. Biol. 9, 1–13. doi:10.3389/fcell.2021.640388
Liu, W., Sun, Y., He, Y., Zhang, H., Zheng, Y., Yao, Y., et al. (2017). IL-1β Impedes the Chondrogenic Differentiation of Synovial Fluid Mesenchymal Stem Cells in the Human Temporomandibular Joint. Int. J. Mol. Med. 39, 317–326. doi:10.3892/ijmm.2016.2832
Liu, X., Zhu, B., Li, Y., Liu, X., Guo, S., Wang, C., et al. (2021). The Role of Vascular Endothelial Growth Factor in Tendon Healing. Front. Physiol. 12, 1–10. doi:10.3389/fphys.2021.766080
Liu, Y., Lin, L., Zou, R., Wen, C., Wang, Z., and Lin, F. (2018). MSC-derived Exosomes Promote Proliferation and Inhibit Apoptosis of Chondrocytes via lncRNA-KLF3-AS1/miR-206/GIT1 axis in Osteoarthritis. Cell Cycle 17, 2411–2422. doi:10.1080/15384101.2018.1526603
Manferdini, C., Paolella, F., Gabusi, E., Cattini, L., Rojewski, M., Schrezenmeier, H., et al. (2020). Osteoarthritic Milieu Affects Adipose‐Derived Mesenchymal Stromal Cells. J. Orthop. Res. 38, 336–347. doi:10.1002/jor.24446
Manferdini, C., Paolella, F., Gabusi, E., Gambari, L., Piacentini, A., Filardo, G., et al. (2017). Adipose Stromal Cells Mediated Switching of the Pro-inflammatory Profile of M1-like Macrophages Is Facilitated by PGE2: In Vitro Evaluation. Osteoarthritis and Cartilage 25, 1161–1171. doi:10.1016/j.joca.2017.01.011
Manning, C. N., Martel, C., Sakiyama-Elbert, S. E., Silva, M. J., Shah, S., Gelberman, R. H., et al. (2015). Adipose-derived Mesenchymal Stromal Cells Modulate Tendon Fibroblast Responses to Macrophage-Induced Inflammation In Vitro. Stem Cel Res. Ther. 6, 1–14. doi:10.1186/s13287-015-0059-4
Melzer, M., Schubert, S., Müller, S. F., Geyer, J., Hagen, A., Niebert, S., et al. (2021). Rho/ROCK Inhibition Promotes TGF-Β3-Induced Tenogenic Differentiation in Mesenchymal Stromal Cells. Stem Cell Int. 2021, 1–11. doi:10.1155/2021/8284690
Neefjes, M., Housmans, B. A. C., van Beuningen, H. M., Vitters, E. L., van den Akker, G. G. H., van den Akker, G. G. H., et al. (2021). Prediction of the Effect of the Osteoarthritic Joint Microenvironment on Cartilage Repair. Tissue Eng. - Part. A. 16, 27. doi:10.1089/ten.TEA.2021.0051
Okamoto, N., Kushida, T., Oe, K., Umeda, M., Ikehara, S., and Iida, H. (2010). Treating Achilles Tendon Rupture in Rats with Bone-Marrow-Cell Transplantation Therapy. J. Bone Jt. Surg. - Ser. A. 92, 2776–2784. doi:10.2106/JBJS.I.01325
Romero, A., Barrachina, L., Ranera, B., Remacha, A. R., Moreno, B., de Blas, I., et al. (2017). Comparison of Autologous Bone Marrow and Adipose Tissue Derived Mesenchymal Stem Cells, and Platelet Rich Plasma, for Treating Surgically Induced Lesions of the Equine Superficial Digital Flexor Tendon. Vet. J. 224, 76–84. doi:10.1016/j.tvjl.2017.04.005
Roth, S. P., Schubert, S., Scheibe, P., Groß, C., Brehm, W., and Burk, J. (2018). Growth Factor-Mediated Tenogenic Induction of Multipotent Mesenchymal Stromal Cells Is Altered by the Microenvironment of Tendon Matrix. Cel Transpl. 27, 1434–1450. doi:10.1177/0963689718792203
Satué, M., Schüler, C., Ginner, N., and Erben, R. G. (2019). Intra-articularly Injected Mesenchymal Stem Cells Promote Cartilage Regeneration, but Do Not Permanently Engraft in Distant Organs. Sci. Rep. 9, 1–10. doi:10.1038/s41598-019-46554-5
Scanzello, C. R., and Goldring, S. R. (2021). The Role of Synovitis in Osteoarthritis Pathogenesis. Bone 51, 249–257. doi:10.1016/j.bone.2012.02.012
Schnabel, L. V., Lynch, M. E., van der Meulen, M. C. H., Yeager, A. E., Kornatowski, M. A., and Nixon, A. J. (2009). Mesenchymal Stem Cells and Insulin-like Growth Factor-I Gene-Enhanced Mesenchymal Stem Cells Improve Structural Aspects of Healing in Equine Flexor Digitorum Superficialis Tendons. J. Orthop. Res. 27, 1392–1398. doi:10.1002/jor.20887
Shen, H., Kormpakis, I., Havlioglu, N., Linderman, S. W., Sakiyama-Elbert, S. E., Erickson, I. E., et al. (2016). The Effect of Mesenchymal Stromal Cell Sheets on the Inflammatory Stage of Flexor Tendon Healing. Stem Cel Res. Ther. 7, 1–13. doi:10.1186/s13287-016-0406-0
Smith, R. K. W., Werling, N. J., Dakin, S. G., Alam, R., Goodship, A. E., and Dudhia, J. (2013). Beneficial Effects of Autologous Bone Marrow-Derived Mesenchymal Stem Cells in Naturally Occurring Tendinopathy. PLoS One 8, e75697. doi:10.1371/journal.pone.0075697
Sole, A., Spriet, M., Padgett, K. A., Vaughan, B., Galuppo, L. D., Borjesson, D. L., et al. (2013). Distribution and Persistence of Technetium-99 Hexamethyl Propylene Amine Oxime-Labelled Bone Marrow-Derived Mesenchymal Stem Cells in Experimentally Induced Tendon Lesions after Intratendinous Injection and Regional Perfusion of the Equine Distal Limb. Equine Vet. J. 45, 726–731. doi:10.1111/evj.12063
Song, H., Zhao, J., Cheng, J., Feng, Z., Wang, J., Momtazi-Borojeni, A. A., et al. (2021). Extracellular Vesicles in Chondrogenesis and Cartilage Regeneration. J. Cel. Mol. Med. 25, 4883–4892. doi:10.1111/jcmm.16290
Van Dalen, S. C. M., Blom, A. B., Walgreen, B., Slöetjes, A. W., Helsen, M. M. A., Geven, E. J. W., et al. (2019). IL-1β-mediated Activation of Adipose-Derived Mesenchymal Stromal Cells Results in PMN Reallocation and Enhanced Phagocytosis: A Possible Mechanism for the Reduction of Osteoarthritis Pathology. Front. Immunol. 10, 1–13. doi:10.3389/fimmu.2019.01075
van den Bosch, M. H. J. (2021). Osteoarthritis Year in Review 2020: Biology. Osteoarthr. Cartil. 29, 143–150. doi:10.1016/j.joca.2020.10.006
Vieira, M., Oliveira, R. J., Eça, L., Pereira, I., Hermeto, L. C., Matuo, R., et al. (2014). Therapeutic Potential of Mesenchymal Stem Cells to Treat Achilles Tendon Injuries. Genet. Mol. Res. 13, 10434–10449. doi:10.4238/2014.December.12.5
Witwer, K. W., Van Balkom, B. W. M., Bruno, S., Choo, A., Dominici, M., Gimona, M., et al. (2019). Defining Mesenchymal Stromal Cell (MSC)-derived Small Extracellular Vesicles for Therapeutic Applications. J. Extracell. Vesicles 8, 1609206. doi:10.1080/20013078.2019.1609206
Yuksel, S., Guleç, M. A., Gultekin, M. Z., Adanır, O., Caglar, A., Beytemur, O., et al. (2016). Comparison of the Early Period Effects of Bone Marrow-Derived Mesenchymal Stem Cells and Platelet-Rich Plasma on the Achilles Tendon Ruptures in Rats. Connect. Tissue Res. 57, 360–373. doi:10.1080/03008207.2016.1189909
Keywords: mesenchymal stromal cells (MSC), tendon, joint, osteoarthritis, context sensitivity, target, immunoregulation
Citation: Roth SP, Burk J, Brehm W and Troillet A (2022) MSC in Tendon and Joint Disease: The Context-Sensitive Link Between Targets and Therapeutic Mechanisms. Front. Bioeng. Biotechnol. 10:855095. doi: 10.3389/fbioe.2022.855095
Received: 14 January 2022; Accepted: 07 March 2022;
Published: 04 April 2022.
Edited by:
Martijn Van Griensven, Maastricht University, NetherlandsReviewed by:
Jehan J. El-Jawhari, Nottingham Trent University, United KingdomCopyright © 2022 Roth, Burk, Brehm and Troillet. This is an open-access article distributed under the terms of the Creative Commons Attribution License (CC BY). The use, distribution or reproduction in other forums is permitted, provided the original author(s) and the copyright owner(s) are credited and that the original publication in this journal is cited, in accordance with accepted academic practice. No use, distribution or reproduction is permitted which does not comply with these terms.
*Correspondence: Antonia Troillet, dHJvaWxsZXRAdmV0bWVkLnVuaS1sZWlwemlnLmRl
Disclaimer: All claims expressed in this article are solely those of the authors and do not necessarily represent those of their affiliated organizations, or those of the publisher, the editors and the reviewers. Any product that may be evaluated in this article or claim that may be made by its manufacturer is not guaranteed or endorsed by the publisher.
Research integrity at Frontiers
Learn more about the work of our research integrity team to safeguard the quality of each article we publish.