- 1Department of Orthopaedics, Emory University School of Medicine, Atlanta, GA, United States
- 2Atlanta VA Medical Center, Department of Veterans Affairs, Decatur, GA, United States
Introduction
The menisci are semi-lunar wedge-shaped discs that are vital to load distribution, stability, and lubrication of the knee (Fox et al., 2012). Due to the variety of stresses placed on these tissues, they are often injured, both through trauma and degeneration. Due to the relative avascularity in the tissue (Henning et al., 1987), it mostly lacks the capacity to self-heal, necessitating surgical intervention, with nearly 500,000 arthroscopic meniscal procedures annually in the US alone (Kim et al., 2011). Meniscectomy, or removal of the torn tissue, remains a leading treatment modality (Abrams et al., 2013; DeFroda et al., 2020), as it provides symptomatic relief from catching and locking; however, it predisposes the joint to long-term degeneration due to increased stresses placed on the articular surfaces (McDermott and Amis, 2006; Wang et al., 2015). Meniscus replacement options, such as allografts and scaffolds (Rodeo, 2001; Steadman and Rodkey, 2005; Efe et al., 2012; Lee et al., 2012), certainly exist, but they are currently limited in their long-term efficacy due to lacking formation and/or maintenance of functional meniscus tissue. For this reason, meniscal suture repair to preserve the native tissue has become increasingly popular (Beaufils and Pujol, 2017; Momaya, 2019), yet these procedures are only performed at a fraction of the rate (10–15%) of meniscectomy.
The decision to perform meniscectomy versus suture repair is often predicated on the region, geometry, and severity of the tear (Figure 1A). Furthermore, there are often many patient-level and joint-level factors that influence a clinician’s decision-making process. For example, degraded meniscal tissue in older patients may be treated more conservatively to provide symptomatic relief as opposed to a younger patient with more acute tears, where the goal would be to preserve the meniscus and its function. Other factors such as comorbidities (e.g., cartilage wear, ligament status, alignment) may also factor into this decision. Even in a relatively healthy patient, there are many complex and challenging tear types, such as posterior root tears, that cannot simply be repaired. Here, we focus on acute tears within the body of the meniscus, where a surgeon often decides between meniscectomy and suture repair. In this subset, tears in the outer half of the meniscus, which is relatively more vascular (Henning et al., 1987), are mostly repaired, since the vascular supply is thought to provide enough nutrients to naturally bridge the tissue gap following suturing. However, since tears in the inner half (almost devoid of vascularity) lack access to this blood supply, the torn tissue is typically removed to alleviate symptoms (Henning et al., 1987; Mordecai, 2014), since suturing the torn edges may not result in eventual tissue bridging. This inner vs. outer dogma of meniscus repair has long governed mode of injury management; however, findings from the musculoskeletal research field may challenge this philosophy as the sole player. The purpose of this opinion article is to extend the impediments of meniscal repair beyond the traditional inner vs. outer paradigm, suggesting the role of other factors: disruption of the circumferential network, dense matrix as an obstruction to tissue joining, and other joint pathologies that may influence repair quality.
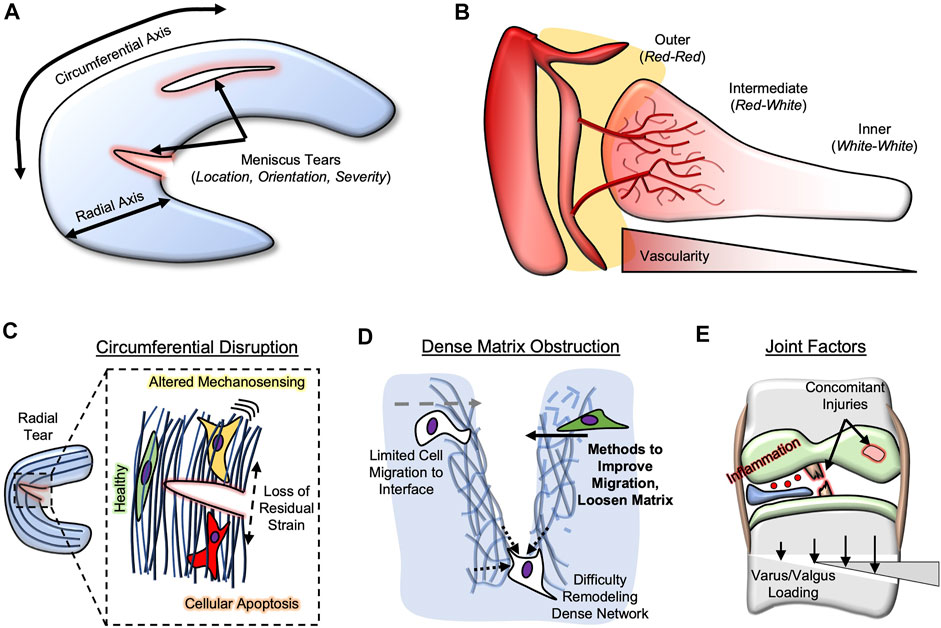
FIGURE 1. Meniscus Repair Impediments. (A) Meniscus tears are often classified based on location, orientation, and severity. Location is usually classified by radial axis (inner, intermediate, outer) and circumferential axis (anterior, body, posterior). (B) Meniscus vascularity penetrates only partially into the meniscal body. (C) Circumferential network disruption (radial tears) lead to loss of residual strain, resulting in altered mechano-sensing and potentially cellular apoptosis. (D) Dense extracellular matrix can obstruct repair by limiting cell migration and matrix remodeling. Methods (e.g., growth factors) can improve migration and loosen matrix. (E) Joint factors that influence meniscal repair include concomitant injuries (e.g., ACl, cartilage), inflammation, and varus/valgus loading.
The Inner Versus Outer Paradigm
The meniscus is often divided into zones along the radial axis, perpendicular to the circumferential network (Figure 1B). Often, the outer meniscus is deemed the red zone, as it contains vascular structures that penetrate, from the meniscosynovial junction, into the tissue. These vessels terminate in the middle third of the meniscus, deemed the red-white zone, leaving the inner third to half of the meniscus devoid of blood supply (termed the white zone). Studies comparing inner to outer meniscus healing rates are few in number (Cinque et al., 2019), perhaps since inner meniscus tears have not historically been repaired. Thus, the recent push to “save the meniscus” is complicated by the majority of tears occurring in either the red-white or white zones (Terzidis et al., 2006), limiting repair potential with suturing. Further exacerbating this issue is that the posterior horn of the meniscus appears to be most injured (Mansori et al., 2018; Jackson et al., 2019), yet exhibits the lowest vascular penetration (Crawford et al., 2020).
The role of vascularity in outer meniscal healing seems to rely on a wound healing response from blood supply, as well as the availability of stimulating growth factors, such as hypoxia inducible factor-1 (HIF-1a) and vascular endothelial growth factor (VEGF) (Lu et al., 2017). For this reason, a plethora of basic science researchers have attempted to augment inner meniscal repair using these vascular-derived factors. Meniscal “perforations”, or surgical holes punctured from the inner-zone meniscus tear outwards towards the periphery, have been attempted preclinically and clinically (Zhongnan et al., 1988; Cook and Fox, 2007), albeit with inconsistent improvement in outcomes. Platelet-rich plasma and bone marrow aspirate have both been widely utilized in conjunction with avascular meniscal tears (Griffin et al., 2015; Muckenhirn et al., 2017; Kaminski et al., 2018), further highlighting the propensity towards “recreating vascularity” to enhance healing of inner meniscal tears. Certainly, vascularity is a player in meniscal healing, but is it the only one? Findings from the musculoskeletal field suggest that there may be others involved, and these may need to be considered to advance the meniscal repair field.
Disruption of Circumferential Collagen Network
As mentioned previously, the menisci are semi-lunar fibrocartilage tissues, and perhaps most important to their function is their array of circumferentially-aligned collagen fibers (Bullough et al., 1970; Fithian et al., 1990). This organization enables the tissue to distribute loads in the knee by generating circumferential hoop stresses (Lee et al., 2006). Meniscus tears occur in a variety of orientations relative to this network; vertical and horizontal tears are parallel to the circumferential arrangement, while radial tears are perpendicular. Thus, radial tears disrupt the aligned collagen network; disruption of similar networks in other aligned tissues, such as tendon and annulus fibrosus, have been shown to be especially problematic. For example, annulus fibrosus cells exhibit aberrant behavior, including fibrotic phenotypic changes (alpha-smooth muscle actin expression) and even apoptosis, following removal of residual strains (Bonnevie et al., 2019). Similarly, transection of tendons (e.g., rotator cuff (Osti et al., 2017)) perpendicular to the aligned axis leads to similar fibrotic and apoptotic behavior (Egerbacher et al., 2008; Maeda et al., 2011; Lundgreen et al., 2013; T. et al., 2020). Thus, it is expected that radial meniscus tears may cause similar cellular changes (Figure 1C), especially near the lesion site, altering their capacity for healing. Of interest is that there seems to be a trend towards more radial tears in the lateral meniscus (Terzidis et al., 2006), which also exhibits a greater number of white-zone tears than the medial meniscus, potentially implicating circumferential network disruption, and not avascularity alone, as a player in lower healing capacity of the inner meniscus. Differences in the medial vs. lateral meniscus could also be influential in a surgeon’s management; the lateral meniscus displaces more during loading (Bylski-Austrow et al., 1994) and less force is typically transmitted through the lateral compartment (Zhao et al., 2007), meaning that circumferential disruption could present a greater issue in the medial meniscus. Clinical systematic reviews, and perhaps preclinical animal studies (Bansal et al., 2020), that investigate the healing rates of inner vs. outer zone radial tears, and inner vertical vs. inner radial tears, would help to test this hypothesis.
Dense Extracellular Matrix as an Obstructor to Healing
One of the greatest obstacles in the field of musculoskeletal repair is the re-integration of wound edges. Frequently thought of in the context of tissue engineering [scaffold-to-tissue integration; (Moffat et al., 2009)], healing of meniscal tears requires two edges of the meniscus to join back together. Suturing holds these edges together initially, but long-term bridging of this gap will require a combination of tissue deposition and remodeling along the interface. A plethora of researchers have attempted to improve meniscal repair healing with scaffolds [e.g., fibrin, collagen, electrospun polymers (Scotti et al., 2009; González-Fernández et al., 2016; Baek et al., 2019)] that are often supplemented with cells (meniscal fibrochondrocytes, marrow stromal cells) and factors (transforming growth factor-beta 3, connective tissue growth factor) (He et al., 2011; Cucchiarini et al., 2015; Sasaki et al., 2018), yet the overall shear strengths of this repair interface are typically orders of magnitude lower than the native meniscus, leaving it susceptible to re-tear. A potential obstructor in this repair interface is the dense nature of native meniscal tissue; the dense matrix limits cell migration to, and eventual healing at, the tear site, and it may lack the capacity to undergo active remodeling to integrate the two edges (Figure 1D).
Many techniques have been employed to improve meniscus cell migration to improve integrative repair. This is especially important in older patients, as both cell motility and proliferation decrease with age (Bartling et al., 2009; Qu et al., 2019), affecting repair potential and efficacy. For example, resident meniscus cells can be “activated” with electrical stimulation (Gunja et al., 2012; Yuan et al., 2014), growth factor delivery (e.g., platelet derived growth factor; (Qu et al., 2017)), or perhaps the addition of exogenous cells/biologics [e.g., endothelial cells (Yuan et al., 2015), platelets (Wong et al., 2017), hyaluronic acid (Murakami et al., 2019)]. A portion of the field is also studying a subpopulation of meniscal progenitor cells that further aid in the process of regeneration (Muhammad et al., 2014; Seol et al., 2017); thus, their migration and recruitment to the site of injury using these techniques would be greatly beneficial. Since the stiffest part of these cells is their nucleus, nuclear softening is also a promising approach to improve migration through the dense connective networks of the meniscus (Heo et al., 2020). Rather than improving cell recruitment by altering the cells, the matrix around the cell could also be loosened via local digestion (Qu et al., 2013, 2015). Similar techniques have been employed in cartilage repair (van de Breevaart Bravenboer et al., 2004; Seol et al., 2014; Liebesny et al., 2019), showing that loosening the network can not only enhance migration, but also improve the ability of the two torn edges to merge back together. This latter concept may be most important, as the dense meniscal network experiences little to no remodeling (Våben et al., 2020), whereas slightly degraded matrix can be remodeled more readily to integrate the two edges.
Other Joint Factors That Influence Healing
Perhaps the most obvious environmental factor that has been implicated in meniscal healing potential is inflammation. The release of pro-inflammatory cytokines (e.g., interleukin-1, tumor necrosis factor-alpha) following soft tissue injury in the joint is well-documented (Irie et al., 2003; Haslauer et al., 2014; Ogura et al., 2016), both by the synovial/synovium cells and the injured tissue itself. In the meniscus specifically, integrative repair of the meniscus, both in vitro and in vivo, is reduced under inflamed environments (Hennerbichler et al., 2007; Riera et al., 2011), perhaps due to reduced proliferation and migration, and reduced capacity for meniscus specific matrix deposition and remodeling. Thus, intra-articular augmentation with, and perhaps even localized delivery of, anti-inflammatory agents may present promising improvements in repair success. Novel methods to deliver these drugs include capsules, carriers, spheres, both at the micro-scale and nano-scale to enhance retention, duration, and activity of both anti-inflammatory and pro-regenerative cues (Patel et al., 2019). Since both inflammation post-injury and the reparative process occur on the order of weeks to months, these prolonging attributes are especially helpful. Delaying meniscal repair procedures after injury, similar to what is done with anterior cruciate ligament reconstruction (Inoue et al., 2016), may help to delay repair until inflammation has subsided, improving the integrative nature, and thus long-term stability, of the repair.
Beyond the biological milieu within the joint, there are a variety of biomechanical joint factors at play. First and foremost, concomitant injuries, especially anterior cruciate ligament injuries and reconstruction, place a large mechanical burden on the meniscus (Dargel et al., 2007; Chen et al., 2017), and restoration of these tissues and their function are paramount to meniscal function and its ability to be repaired. This may also include an open-wedge osteotomy to correct varus or valgus malalignment (Jing et al., 2019; Rocha de Faria et al., 2021), to alleviate loads that are placed on one compartment of the knee. Furthermore, along the same lines, the rehabilitation timeline needs to be precisely controlled (Cavanaugh and Killian, 2012; Spang Iii et al., 2018); early overloading may cause re-tear before adequate tissue has been deposited to bridge the tear. Alternatively, since mechanical loading is beneficial to meniscal cell activity and matrix deposition (McNulty et al., 2010; Puetzer et al., 2012), a protocol that is too conservative can prohibit the increased regenerative capacity provided by loading. Timing prior to the procedure is also an important biomechanical consideration. While waiting can calm inflammation to enhance repair potential, it must be balanced with the increased risk of other injuries that can occur in this timeframe (Fok and Yau, 2013; Kolin et al., 2021; Prodromidis et al., 2021).
Conclusion
The meniscal repair field has long cited the location along the radial axis, indicative of vascular content, as the sole determinant of treatment modality. I believe that other factors (circumferential disruption, dense matrix obstruction, and joint factors) may be just as influential to repair potential. Thus, the field would greatly benefit from additional clinical studies and reviews to better track outcomes with regards to these variables, which is fully possible with the increasing performance of inner zone repairs. Additional preclinical work, both in vitro and in vivo, will help to elucidate the healing potential of various tear configurations, especially as they relate to the alignment of collagen bundles, the relative density of the matrix, and the environmental inflammatory state. The recent shift to “save the meniscus” with repair techniques would greatly benefit from consideration of these alternative impediments to healing.
Author Contributions
The author confirms being the sole contributor of this work and has approved it for publication.
Conflict of Interest
The author declares that the research was conducted in the absence of any commercial or financial relationships that could be construed as a potential conflict of interest.
Publisher’s Note
All claims expressed in this article are solely those of the authors and do not necessarily represent those of their affiliated organizations, or those of the publisher, the editors and the reviewers. Any product that may be evaluated in this article, or claim that may be made by its manufacturer, is not guaranteed or endorsed by the publisher.
References
Abrams, G. D., Frank, R. M., Gupta, A. K., Harris, J. D., McCormick, F. M., and Cole, B. J. (2013). Trends in Meniscus Repair and Meniscectomy in the United States, 2005-2011. Am. J. Sports Med. 41, 2333–2339. doi:10.1177/0363546513495641
Baek, J., Lotz, M. K., and D'Lima, D. D. (2019). Core-Shell Nanofibrous Scaffolds for Repair of Meniscus Tears. Tissue Eng. A 25, 1577–1590. doi:10.1089/ten.tea.2018.0319
Bansal, S., Miller, L. M., Patel, J. M., Meadows, K. D., Eby, M. R., Saleh, K. S., et al. (2020). Transection of the Medial Meniscus Anterior Horn Results in Cartilage Degeneration and Meniscus Remodeling in a Large Animal Model. J. Orthop. Res. 38, 2696–2708. doi:10.1002/jor.24694
Bartling, B., Desole, M., Rohrbach, S., Silber, R. E., and Simm, A. (2009). Age‐associated Changes of Extracellular Matrix Collagen Impair Lung Cancer Cell Migration. FASEB J. 23, 1510–1520. doi:10.1096/fj.08-122648
Beaufils, P., and Pujol, N. (2017). Management of Traumatic Meniscal Tear and Degenerative Meniscal Lesions. Save the Meniscus. Orthopaedics Traumatol. Surg. Res. 103, S237–S244. doi:10.1016/j.otsr.2017.08.003
Bonnevie, E. D., Gullbrand, S. E., Ashinsky, B. G., Tsinman, T. K., Elliott, D. M., Chao, P., et al. (2019). Aberrant Mechanosensing in Injured Intervertebral Discs as a Result of Boundary-Constraint Disruption and Residual-Strain Loss. Nat. Biomed. Eng. 3, 998–1008. doi:10.1038/s41551-019-0458-4
Bullough, P. G., Munuera, L., Murphy, J., and Weinstein, A. M. (1970). The Strength of the Menisci of the Knee as it Relates to Their fine Structure. The J. Bone Jt. Surg. Br. volume 52-B, 564–570. doi:10.1302/0301-620x.52b3.564
Bylski-Austrow, D. I., Ciarelli, M. J., Kayner, D. C., Matthews, L. S., and Goldstein, S. A. (1994). Displacements of the Menisci under Joint Load: An In Vitro Study in Human Knees. J. Biomech. 27, 421–431. doi:10.1016/0021-9290(94)90018-3
Cavanaugh, J. T., and Killian, S. E. (2012). Rehabilitation Following Meniscal Repair. Curr. Rev. Musculoskelet. Med. 5, 46–58. doi:10.1007/s12178-011-9110-y
Chen, T., Wang, H., Warren, R., and Maher, S. (2017). Loss of ACL Function Leads to Alterations in Tibial Plateau Common Dynamic Contact Stress Profiles. J. Biomech. 61, 275–279. doi:10.1016/j.jbiomech.2017.07.024
Cinque, M. E., DePhillipo, N. N., Moatshe, G., Chahla, J., Kennedy, M. I., Dornan, G. J., et al. (2019). Clinical Outcomes of Inside-Out Meniscal Repair According to Anatomic Zone of the Meniscal Tear. Orthop. J. Sport Med. 7 (7), 2325967119860806. doi:10.1177/2325967119860806
Cook, J. L., and Fox, D. B. (2007). A Novel Bioabsorbable Conduit Augments Healing of Avascular Meniscal Tears in a Dog Model. Am. J. Sports Med. 35 (11), 1877–1887. doi:10.1177/0363546507304330
Crawford, M. D., Hellwinkel, J. E., Aman, Z., Akamefula, R., Singleton, J. T., Bahney, C., et al. (2020). Microvascular Anatomy and Intrinsic Gene Expression of Menisci from Young Adults. Am. J. Sports Med. 48, 3147–3153. doi:10.1177/0363546520961555
Cucchiarini, M., Schmidt, K., Frisch, J., Kohn, D., and Madry, H. (2015). Overexpression of TGF-β via rAAV-Mediated Gene Transfer Promotes the Healing of Human Meniscal Lesions Ex Vivo on Explanted Menisci. Am. J. Sports Med. 43 (5), 1197–1205. doi:10.1177/0363546514567063
DeFroda, S. F., Yang, D. S., Donnelly, J. C., Bokshan, S. L., Owens, B. D., and Daniels, A. H. (2020). Trends in the Surgical Treatment of Meniscal Tears in Patients with and without Concurrent Anterior Cruciate Ligament Tears. Phys. Sportsmed 48 (2), 229–235. doi:10.1080/00913847.2019.1685363
Efe, T., Getgood, A., Schofer, M. D., Fuchs-Winkelmann, S., Mann, D., Paletta, J. R. J., et al. (2012). The Safety and Short-Term Efficacy of a Novel Polyurethane Meniscal Scaffold for the Treatment of Segmental Medial Meniscus Deficiency. Knee Surg. Sports Traumatol. Arthrosc. 20, 1822–1830. doi:10.1007/s00167-011-1779-3
Egerbacher, M., Arnoczky, S. P., Caballero, O., Lavagnino, M., and Gardner, K. L. (2008). Loss of Homeostatic Tension Induces Apoptosis in Tendon Cells: an In Vitro Study. Clin. Orthop. Relat. Res. 466, 1562–1568. doi:10.1007/s11999-008-0274-8
Fithian, D. C., Kelly, M. A., and Mow, V. C. (1990). Material Properties and Structure-Function Relationships in the Menisci. Clin. Orthopaedics Relat. Res. 252, 19–31. doi:10.1097/00003086-199003000-00004
Fok, A. W. M., and Yau, W. P. (2013). Delay in ACL Reconstruction Is Associated with More Severe and Painful Meniscal and Chondral Injuries. Knee Surg. Sports Traumatol. Arthrosc. 21, 928–933. doi:10.1007/s00167-012-2027-1
Fox, A. J. S., Bedi, A., and Rodeo, S. A. (2012). The Basic Science of Human Knee Menisci. Sports Health 4, 340–351. doi:10.1177/1941738111429419
González-Fernández, M. L., Pérez-Castrillo, S., Sánchez-Lázaro, J. A., Prieto-Fernández, J. G., López-González, M. E., Lobato-Pérez, S., et al. (2016). Assessment of Regeneration in Meniscal Lesions by Use of Mesenchymal Stem Cells Derived from Equine Bone Marrow and Adipose Tissue. Am. J. Vet. Res. 77 (7), 779–788. doi:10.2460/ajvr.77.7.779
Griffin, J. W., Hadeed, M. M., Werner, B. C., Diduch, D. R., Carson, E. W., and Miller, M. D. (2015). Platelet-rich Plasma in Meniscal Repair: Does Augmentation Improve Surgical Outcomes? Clin. Orthop. Relat. Res. 473 (5), 1665–1672. doi:10.1007/s11999-015-4170-8
Gunja, N. J., Dujari, D., Chen, A., Luengo, A., Fong, J. V., and Hung, C. T. (2012). Migration Responses of Outer and Inner Meniscus Cells to Applied Direct Current Electric fields. J. Orthop. Res. 30, 103–111. doi:10.1002/jor.21489
Haslauer, C. M., Proffen, B. L., Johnson, V. M., Hill, A., and Murray, M. M. (2014). Gene Expression of Catabolic Inflammatory Cytokines Peak before Anabolic Inflammatory Cytokines after ACL Injury in a Preclinical Model. J. Inflamm. 11, 34. doi:10.1186/s12950-014-0034-3
He, W., Liu, Y. J., Wang, Z. G., Guo, Z. K., Wang, M. X., and Wang, N. (2011). Enhancement of Meniscal Repair in the Avascular Zone Using Connective Tissue Growth Factor in a Rabbit Model. Chin. Med. J. (Engl) 124, 3968–3975. doi:10.3760/cma.j.issn.2011.23.023
Hennerbichler, A., Moutos, F. T., Hennerbichler, D., Weinberg, J. B., and Guilak, F. (2007). Interleukin-1 and Tumor Necrosis Factor Alpha Inhibit Repair of the Porcine Meniscus In Vitro. Osteoarthritis and Cartilage 15, 1053–1060. doi:10.1016/j.joca.2007.03.003
Henning, C. E., Lynch, M. A., and Clark, J. R. (1987). Vascularity for Healing of Meniscus Repairs. Arthrosc. J. Arthroscopic Relat. Surg. 3, 13–18. doi:10.1016/S0749-8063(87)80004-X
Heo, S.-J., Song, K. H., Thakur, S., Miller, L. M., Cao, X., Peredo, A. P., et al. (2020). Nuclear Softening Expedites Interstitial Cell Migration in Fibrous Networks and Dense Connective Tissues. Sci. Adv. 6 (25), eaax5083. doi:10.1126/sciadv.aax5083
Inoue, M., Muneta, T., Ojima, M., Nakamura, K., Koga, H., Sekiya, I., et al. (2016). Inflammatory Cytokine Levels in Synovial Fluid 3, 4 Days Postoperatively and its Correlation with Early-phase Functional Recovery after Anterior Cruciate Ligament Reconstruction: a Cohort Study. J. Exp. Ortop 3, 30. doi:10.1186/s40634-016-0067-z
Irie, K., Uchiyama, E., and Iwaso, H. (2003). Intraarticular Inflammatory Cytokines in Acute Anterior Cruciate Ligament Injured Knee. The Knee 10, 93–96. doi:10.1016/S0968-0160(02)00083-2
Jackson, T., Fabricant, P. D., Beck, N., Storey, E., Patel, N. M., and Ganley, T. J. (2019). Epidemiology, Injury Patterns, and Treatment of Meniscal Tears in Pediatric Patients: A 16-Year Experience of a Single Center. Orthop. J. Sport Med. 7 (12), 2325967119890325. doi:10.1177/2325967119890325
Jing, L., Liu, K., Wang, X., Wang, X., Li, Z., Zhang, X., et al. (2019). Second-look Arthroscopic Findings after Medial Open-Wedge High Tibial Osteotomy Combined with All-Inside Repair of Medial Meniscus Posterior Root Tears. J. Orthop. Surg. (Hong Kong) 28, 230949901988883. doi:10.1177/2309499019888836
Kaminski, R., Kulinski, K., Kozar-Kaminska, K., Wielgus, M., Langner, M., Wasko, M. K., et al. (2018). A Prospective, Randomized, Double-Blind, Parallel-Group, Placebo-Controlled Study Evaluating Meniscal Healing, Clinical Outcomes, and Safety in Patients Undergoing Meniscal Repair of Unstable, Complete Vertical Meniscal Tears (Bucket Handle) Augmented Wit. Biomed. Res. Int. 2018, 9315815. doi:10.1155/2018/9315815
Kim, S., Bosque, J., Meehan, J. P., Jamali, A., and Marder, R. (2011). Increase in Outpatient Knee Arthroscopy in the United States: A Comparison of National Surveys of Ambulatory Surgery, 1996 and 2006. J. Bone Jt. Surg Am 93 (11), 994–1000. Available at:doi:10.2106/JBJS.I.01618https://journals.lww.com/jbjsjournal/Fulltext/2011/06010/Increase_in_Outpatient_Knee_Arthroscopy_in_the.2.aspx
Kolin, D. A., Dawkins, B., Park, J., Fabricant, P. D., Gilmore, A., Seeley, M., et al. (2021). ACL Reconstruction Delay in Pediatric and Adolescent Patients Is Associated with a Progressive Increased Risk of Medial Meniscal Tears. J. Bone Jt. Surg Am. 103 (15), 1368–1373. Available at:doi:10.2106/jbjs.20.01459https://journals.lww.com/jbjsjournal/Fulltext/2021/08040/ACL_Reconstruction_Delay_in_Pediatric_and.2.aspx
Lee, S. J., Aadalen, K. J., Malaviya, P., Lorenz, E. P., Hayden, J. K., Farr, J., et al. (2006). Tibiofemoral Contact Mechanics after Serial Medial Meniscectomies in the Human Cadaveric Knee. Am. J. Sports Med. 34, 1334–1344. doi:10.1177/0363546506286786
Lee, S. R., Kim, J. G., and Nam, S. W. (2012). The Tips and Pitfalls of Meniscus Allograft Transplantation. Knee Surg. Relat. Res. 24, 137–145. doi:10.5792/ksrr.2012.24.3.137
Liebesny, P. H., Mroszczyk, K., Zlotnick, H., Hung, H.-H., Frank, E., Kurz, B., et al. (2019). Enzyme Pretreatment Plus Locally Delivered HB-IGF-1 Stimulate Integrative Cartilage Repair In Vitro. Tissue Eng. Part A 25, 1191–1201. doi:10.1089/ten.tea.2019.0013
Lu, Z., Furumatsu, T., Fujii, M., Maehara, A., and Ozaki, T. (2017). The Distribution of Vascular Endothelial Growth Factor in Human Meniscus and a Meniscal Injury Model. J. Orthopaedic Sci. 22, 715–721. doi:10.1016/j.jos.2017.02.006
Lundgreen, K., Lian, Ø., Scott, A., and Engebretsen, L. (2013). Increased Levels of Apoptosis and P53 in Partial-Thickness Supraspinatus Tendon Tears. Br. J. Sports Med. 47, 46. e2 LP-e2. doi:10.1136/bjsports-2013-092459.50
Mader, K., Pennig, D., Dargel, J., Gotter, M., Koebke, J., and Schmidt-Wiethoff, R. (2007). Biomechanics of the Anterior Cruciate Ligament and Implications for Surgical Reconstruction. Strateg. Trauma Limb Reconstr. 2, 1–12. doi:10.1007/s11751-007-0016-6
Maeda, T., Sakabe, T., Sunaga, A., Sakai, K., Rivera, A. L., Keene, D. R., et al. (2011). Conversion of Mechanical Force into TGF-β-Mediated Biochemical Signals. Curr. Biol. 21, 933–941. doi:10.1016/j.cub.2011.04.007
Mansori, A. E., Lording, T., Schneider, A., Dumas, R., Servien, E., and Lustig, S. (2018). Incidence and Patterns of Meniscal Tears Accompanying the Anterior Cruciate Ligament Injury: Possible Local and Generalized Risk Factors. Int. Orthop. 42 (9), 2113–2121. doi:10.1007/s00264-018-3992-x
McDermott, I. D., and Amis, A. A. (2006). The Consequences of Meniscectomy. J. Bone Jt. Surg. Br. volume 88-B, 1549–1556. doi:10.1302/0301-620X.88B12.18140
McNulty, A. L., Estes, B. T., Wilusz, R. E., Weinberg, J. B., and Guilak, F. (2010). Dynamic Loading Enhances Integrative Meniscal Repair in the Presence of Interleukin-1. Osteoarthritis and Cartilage 18, 830–838. doi:10.1016/j.joca.2010.02.009
Moffat, K. L., Wang, I.-N. E., Rodeo, S. A., and Rodeo, H. H. (2009). Orthopedic Interface Tissue Engineering for the Biological Fixation of Soft Tissue Grafts. Clin. Sports Med. 28, 157–176. doi:10.1016/j.csm.2008.08.006
Momaya, A. (2019). Editorial Commentary: Save the Meniscus? Show Me the Money! Arthrosc. J. Arthroscopic Relat. Surg. 35, 3287–3288. doi:10.1016/j.arthro.2019.08.008
Mordecai, S. C. (2014). Treatment of Meniscal Tears: An Evidence Based Approach. Wjo 5, 233. doi:10.5312/wjo.v5.i3.233
Muckenhirn, K. J., Kruckeberg, B. M., Cinque, M. E., Chahla, J., DePhillipo, N. N., Godin, J. A., et al. (2017). Arthroscopic Inside-Out Repair of a Meniscus Bucket-Handle Tear Augmented with Bone Marrow Aspirate Concentrate. Arthrosc. Tech. 6 (4), e1221–e1227. doi:10.1016/j.eats.2017.04.014
Muhammad, H., Schminke, B., Bode, C., Roth, M., Albert, J., von der Heyde, S., et al. (2014). Human Migratory Meniscus Progenitor Cells Are Controlled via the TGF-β Pathway. Stem Cel. Rep. 3, 789–803. doi:10.1016/j.stemcr.2014.08.010
Murakami, T., Otsuki, S., Okamoto, Y., Nakagawa, K., Wakama, H., Okuno, N., et al. (2019). Hyaluronic Acid Promotes Proliferation and Migration of Human Meniscus Cells via a CD44-dependent Mechanism. Connect. Tissue Res. 60, 117–127. doi:10.1080/03008207.2018.1465053
Ogura, T., Suzuki, M., Sakuma, Y., Yamauchi, K., Orita, S., Miyagi, M., et al. (2016). Differences in Levels of Inflammatory Mediators in Meniscal and Synovial Tissue of Patients with Meniscal Lesions. J. Exp. Ortop 3, 7. doi:10.1186/s40634-016-0041-9
Osti, L., Buda, M., Del Buono, A., Osti, R., Massari, L., and Maffulli, N. (2017). Apoptosis and Rotator Cuff Tears: Scientific Evidence from Basic Science to Clinical Findings. Br. Med. Bull. 122, 123–133. doi:10.1093/bmb/ldx008
Patel, J. M., Saleh, K. S., Burdick, J. A., and Mauck, R. L. (2019). Bioactive Factors for Cartilage Repair and Regeneration: Improving Delivery, Retention, and Activity. Acta Biomater. 93, 222–238. doi:10.1016/j.actbio.2019.01.061
Prodromidis, A. D., Drosatou, C., Mourikis, A., Sutton, P. M., and Charalambous, C. P. (2021). Relationship between Timing of Anterior Cruciate Ligament Reconstruction and Chondral Injuries: A Systematic Review and Meta-Analysis. Am. J. Sports Med., 036354652110361. doi:10.1177/03635465211036141
Puetzer, J. L., Ballyns, J. J., and Bonassar, L. J. (2012). The Effect of the Duration of Mechanical Stimulation and Post-Stimulation Culture on the Structure and Properties of Dynamically Compressed Tissue-Engineered Menisci. Tissue Eng. Part A 18, 1365–1375. doi:10.1089/ten.tea.2011.0589
Qu, F., Guilak, F., and Mauck, R. L. (2019). Cell Migration: Implications for Repair and Regeneration in Joint Disease. Nat. Rev. Rheumatol. 15, 167–179. doi:10.1038/s41584-018-0151-0
Qu, F., Holloway, J. L., Esterhai, J. L., Burdick, J. A., and Mauck, R. L. (2017). Programmed Biomolecule Delivery to Enable and Direct Cell Migration for Connective Tissue Repair. Nat. Commun. 8, 1780. doi:10.1038/s41467-017-01955-w
Qu, F., Lin, J.-M. G., Esterhai, J. L., Fisher, M. B., and Mauck, R. L. (2013). Biomaterial-mediated Delivery of Degradative Enzymes to Improve Meniscus Integration and Repair. Acta Biomater. 9, 6393–6402. doi:10.1016/j.actbio.2013.01.016
Qu, F., Pintauro, M. P., Haughan, J. E., Henning, E. A., Esterhai, J. L., Schaer, T. P., et al. (2015). Repair of Dense Connective Tissues via Biomaterial-Mediated Matrix Reprogramming of the Wound Interface. Biomaterials 39, 85–94. doi:10.1016/j.biomaterials.2014.10.067
Riera, K. M., Rothfusz, N. E., Wilusz, R. E., Weinberg, J. B., Guilak, F., and McNulty, A. L. (2011). Interleukin-1, Tumor Necrosis Factor-Alpha, and Transforming Growth Factor-Beta 1 and Integrative Meniscal Repair: Influences on Meniscal Cell Proliferation and Migration. Arthritis Res. Ther.. 13(6):R187. doi:10.1186/ar3515
Rocha de Faria, J. L., Pavão, D. M., Moreirão, M. d. C., Titonelli, V. E., de Sousa, E. B., Minamoto, S. T. N., et al. (2021). Posterior Root Repair of Medial Meniscus Combined with Valgus Opening Wedge Tibial Osteotomy. Arthrosc. Tech. 10, e1373–e1382. doi:10.1016/j.eats.2021.01.042
Rodeo, S. A. (2001). Meniscal Allografts-Where Do We Stand? Am. J. Sports Med. 29, 246–261. doi:10.1177/03635465010290022401
Sasaki, H., Rothrauff, B. B., Alexander, P. G., Lin, H., Gottardi, R., Fu, F. H., et al. (2018). In Vitro Repair of Meniscal Radial Tear with Hydrogels Seeded with Adipose Stem Cells and TGF-Β3. Am. J. Sports Med. 46 (10), 2402–2413. doi:10.1177/0363546518782973
Scotti, C., Pozzi, A., Mangiavini, L., Vitari, F., Boschetti, F., Domeneghini, C., et al. (2009). Healing of Meniscal Tissue by Cellular Fibrin Glue: An In Vivo Study. Knee Surgery, Sport Traumatol. Arthrosc. 17 (6), 645–651. doi:10.1007/s00167-009-0745-9
Seol, D., Yu, Y., Choe, H., Jang, K., Brouillette, M. J., Zheng, H., et al. (2014). Effect of Short-Term Enzymatic Treatment on Cell Migration and Cartilage Regeneration: In Vitro Organ Culture of Bovine Articular Cartilage. Tissue Eng. Part A 20, 1807–1814. doi:10.1089/ten.tea.2013.0444
Seol, D., Zhou, C., Brouillette, M. J., Song, I., Yu, Y., Choe, H. H., et al. (2017). Characteristics of Meniscus Progenitor Cells Migrated from Injured Meniscus. J. Orthop. Res. 35, 1966–1972. doi:10.1002/jor.23472
Spang Iii, R. C., Mohamadi, A., DeAngelis, J. P., Nazarian, A., Ramappa, A. J., and Ramappa, A. J. (2018). Rehabilitation Following Meniscal Repair: a Systematic Review. BMJ Open Sport Exerc. Med. 4, e000212. doi:10.1136/bmjsem-2016-000212
Steadman, J. R., and Rodkey, W. G. (2005). Tissue-engineered Collagen Meniscus Implants: 5- to 6-year Feasibility Study Results. Arthrosc. J. Arthroscopic Relat. Surg. 21, 515–525. doi:10.1016/j.arthro.2005.01.006
Terzidis, I. P., Christodoulou, A., Ploumis, A., Givissis, P., Natsis, K., and Koimtzis, M. (2006). Meniscal Tear Characteristics in Young Athletes with a Stable Knee: Arthroscopic Evaluation. Am. J. Sports Med. 34 (7), 1170–1175. doi:10.1177/0363546506287939
Våben, C., Heinemeier, K. M., Schjerling, P., Olsen, J., Petersen, M. M., Kjaer, M., et al. (2020). No Detectable Remodelling in Adult Human Menisci: an Analysis Based on the C14 Bomb Pulse. Br. J. Sports Med. 54, 1433–1437. doi:10.1136/bjsports-2019-101360
van de Breevaart Bravenboer, J., In der Maur, C. D., Bos, P. K., Feenstra, L., Verhaar, J. A., Weinans, H., et al. (2004). Improved Cartilage Integration and Interfacial Strength after Enzymatic Treatment in a Cartilage Transplantation Model. Arthritis Res. Ther. 6, R469. doi:10.1186/ar1216
Wang, H., Chen, T., Gee, A. O., Hutchinson, I. D., Stoner, K., Warren, R. F., et al. (2015). Altered Regional Loading Patterns on Articular Cartilage Following Meniscectomy Are Not Fully Restored by Autograft Meniscal Transplantation. Osteoarthritis and Cartilage 23, 462–468. doi:10.1016/j.joca.2014.12.003
Wong, C. C., Kuo, T. F., Yang, T. L., Tsuang, Y. H., Lin, M. F., Chang, C. H., et al. (2017). Platelet-rich Fibrin Facilitates Rabbit Meniscal Repair by Promoting Meniscocytes Proliferation, Migration, and Extracellular Matrix Synthesis. Int. J. Mol. Sci. 18 (8), 1722. doi:10.3390/ijms18081722
Yuan, X., Arkonac, D. E., Chao, P.-h. G., and Vunjak-Novakovic, G. (2014). Electrical Stimulation Enhances Cell Migration and Integrative Repair in the Meniscus. Sci. Rep. 4, 3674. doi:10.1038/srep03674
Yuan, X., Eng, G. M., Arkonac, D. E., Chao, P. H. G., and Vunjak-Novakovic, G. (2015). Endothelial Cells Enhance the Migration of Bovine Meniscus Cells. Arthritis Rheumatol. 67 (1), 182–192. doi:10.1002/art.38889
Zhao, D., Banks, S. A., D'Lima, D. D., Colwell, C. W., and Fregly, B. J. (2007). In Vivo medial and Lateral Tibial Loads during Dynamic and High Flexion Activities. J. Orthop. Res. 25, 593–602. doi:10.1002/jor.20362
Keywords: meniscus, meniscus repair, mechanobiolgy, tissue engineering, vascularity
Citation: Patel JM (2022) Impediments to Meniscal Repair: Factors at Play Beyond Vascularity. Front. Bioeng. Biotechnol. 10:843166. doi: 10.3389/fbioe.2022.843166
Received: 24 December 2021; Accepted: 04 February 2022;
Published: 01 March 2022.
Edited by:
Mustafa Zakkar, University of Leicester, United KingdomReviewed by:
Eran Linder-Ganz, Active Implants, United StatesFrancesco Travascio, University of Miami, United States
Copyright © 2022 Patel. This is an open-access article distributed under the terms of the Creative Commons Attribution License (CC BY). The use, distribution or reproduction in other forums is permitted, provided the original author(s) and the copyright owner(s) are credited and that the original publication in this journal is cited, in accordance with accepted academic practice. No use, distribution or reproduction is permitted which does not comply with these terms.
*Correspondence: Jay M. Patel, amF5Lm1pbGFuLnBhdGVsQGVtb3J5LmVkdQ==