- 1Department of Chemical Engineering, National Chung Hsing University, Taichung, Taiwan
- 2Innovation and Development Center of Sustainable Agriculture, National Chung Hsing University, Taichung, Taiwan
An antibiotic- and inducer-free culture condition was proposed for polyhydroxybutyrate (PHB) production in recombinant Escherichia coli. First, antibiotic-free vectors were constructed by installing the plasmid maintenance system, alp7, hok/sok, and the hok/sok and alp7 combination into the pUC19 vector. The plasmid stability test showed that pVEC02, the pUC19 vector containing the hok/sok system, was the most effective in achieving antibiotic-free cultivation in the E. coli B strain but not in the K strain. Second, the putative phaCAB operon derived from Caldimonas manganoxidans was inserted into pVEC02 to yield pPHB01 for PHB production in E. coli BL21 (DE3). The putative phaCAB operon was first shown function properly for PHB production and thus, inducer-free conditions were achieved. However, the maintenance of pPHB01 in E. coli requires antibiotics supplementation. Finally, an efficient E. coli ρ factor-independent terminator, thrLABC (ECK120033737), was inserted between the phaCAB operon and the hok/sok system to avoid possible transcriptional carry-over. The newly constructed plasmid pPHB01-1 facilitates an antibiotic- and inducer-free culture condition and induces the production of PHB with a concentration of 3.0 on0.2 g/L, yield of 0.26 /L0.07 g/g-glucose, and content of 44 /g3%. The PHB production using E. coli BL21 (DE3)/pPHB01-1 has been shown to last 84 and 96 h in the liquid and solid cultures.
Introduction
Plasmid-based genetic manipulation is a common approach in metabolic engineering or whole-cell bio-catalysis. One advantage of employing plasmid-based genetic manipulation is the permit of in vitro genetic manuscription. The maintenance of recombinant plasmids in bacterial cells usually depends on the use of selective conditions, such as antibiotics supplementations. However, the scaling up of antibiotics use is expensive.
Strategies for the maintenance of plasmids during cell growth under antibiotic-free conditions have been extensively studied (Zielenkiewicz and Cegłowski, 2001; Kroll et al., 2010; Akiyama et al., 2011; Laguna et al., 2015; Singha et al., 2017). Among such strategies, post-segregational killing (PSK) and active partition systems have been proven to increase plasmid stability (Nordström and Austin, 1989; Sayeed et al., 2000). The hok/sok (formerly parB) locus, following the PSK mechanism, is a toxin-antidote system that simultaneously produces a toxin (Hok) and a short-lived antidote (Sok), such that in the event of plasmid loss, the cell will be killed by the long-lived toxin (Wu and Wood, 1994; Pecota et al., 2003; Friehs, 2004; Danino et al., 2015). In other words, only the cell that harbors the hok/sok locus can propagate because of Sok production.
The alp7 system, found in Bacillus subtilis, is an active partition system consisting of two acting proteins (Alp7A and Alp7R; actin-like proteins) and a centromere-like cis-acting site (alp7C). Alp7A protein is an ATPase which interact with the Alp7R protein. The Alp7R protein recognizes and binds to the alp7C site, and the formation of the Alp7CR complex with the help of Alp7A pushes plasmids to the cellular poles during plasmid replication, ensuring equal segregation during cell division (Friehs, 2004; Derman et al., 2009; Derman et al., 2012; Million-Weaver et al., 2014).
Wu and Wood showed that Escherichia coli BK6 harboring a hok/sok-containing system maintained the plasmid for over 300 h without antibiotics (reaching a 90% proportion of antibiotic-resistant cells) (Wu and Wood, 1994). A recombinant plasmid has been shown to be maintained in E. coli Nissle 1917 over 72 h with the help of a hok/sok and alp7 combination so that the recombinant E. coli Nissle 1917 can serve as a programmable probiotic for cancer detection (Danino et al., 2015). A recombinant plasmid harboring the alp7 system can be maintained in B. subtilis over 14 generations without the supplementation of antibiotics (Derman et al., 2009; Derman et al., 2012). A trpA-containing plasmid can be maintained in E. coli M72 (K-strain) over 100 generations by integrating the hok/sok locus in the plasmid backbone (Kim and Kang, 1996).
Poly-3-hydroxybutyrate (PHB) has attracted much attention owing its biodegradability and biocompatibility, particularly because of the increase in global environmental concerns in recent years. The common method for PHB biosynthesis from acetyl-CoA in microorganisms comprises three steps. First, two molecules of acetyl-CoA are condensed by β-ketothiolase, encoded by phaA gene, to form acetoacetyl-CoA. Second, acetoacetyl-CoA reductase (encoded by phaB) converts acetoacetyl-CoA to 3-hydroxybutyryl-CoA using NADPH. Finally, the enzyme PHA synthase (encoded by phaC) polymerizes 3-hydroxybutyryl-CoA monomers to PHB, liberating CoA (Bernd, 2003; Stubbe et al., 2005; Peña et al., 2014; Boontip et al., 2021). In our previous study, we expressed the PHB biosynthesis genes, phaCAB, from the thermophilic Caldimonas manganoxidans in E. coli and achieved PHB production with a concentration of 16.8 ± 0.6 g/L, content of 74%, and yield of 0.28 g/g glucose (Lin et al., 2017). The expression of the three genes was achieved by inserting phaA and phaB into the commercial vector, pCDF-Duet1, and inserting phaC into a vector, T-BAD, which is ampicillin resistant.
In this study, we constructed three pUC19-based vectors, pVEC01, pVEC02, and pVEC03, which contain alp7, hok/sok, and the alp7-hok/sok combination, respectively. The stability of pVEC01-03 without the ampicillin supplementation was tested in various E. coli strains. The most stable vector among the three was chosen for application in PHB production. The putative phaCAB operon of C. manganoxidans was first amplified from the C. manganoxidans genome, where the amplicon (3,919 bp in total) contained the phaCAB gene cluster and a 194-bp fragment upstream of phaC. In this manner, the expression of phaCAB genes will be under the control of the promoter and ribosome binding site (RBS) from C. manganoxidans. This is practically important because any possible inducer, such as IPTG, can be avoided for the economics purpose, where IPTG was calculated to cost 10% of total cost of recombinant β-glucosidase production (Ferreira et al., 2018). While the phaCAB operon has been shown to be functionalized in E. coli in this study, the function of the recombinant plasmid containing the putative phaCAB operon in E. coli can only be shown with ampicillin supplementation in the first trial. The latter part of the study proposes a solution to take advantage of the hok/sok for antibiotic-free PHB production.
Materials and Methods
Bacteria Strains and Plasmids
All strains and plasmids used in this study are listed in Table 1.
DNA Manipulation and Transformation
Plasmids were constructed by sequence- and ligation-independent cloning (Li and Elledge, 2007; Jeong et al., 2012; Islam et al., 2017) and schematic diagrams of plasmid construction are shown in Figure 1 The primers used in this study are listed in Table 2. To construct the recombinant plasmid pVEC01, alp7 was amplified from pTKW106alp7 (Addgene, Watertown, United States) by polymerase chain reaction (PCR), and pUC19 was digested with XbaI and BamHI. To construct the recombinant plasmids pVEC02 and pVEC03, the hok/sok locus was amplified from pTKW106alp7 by PCR, and the vectors pUC19 and pVEC01 were digested with SalI. To construct the recombinant plasmid pPHB01, the phaCAB operon was amplified from the genomic DNA of C. manganoxidans and pVEC02 was digested with SphI. After the preparation of inserts and vectors, the DNA fragments were purified using the Gene-Spin™ 1-4-3 DNA Extraction Kit (PROTECH, Taipei, Taiwan). The insert and corresponding vector were mixed at an appropriate ratio and incubated at 37°C for 1 min with T4 DNA polymerase to generate 5′-overhangs. Thereafter, the reaction mixture was placed on ice for 20 min for single-strand annealing and was introduced into competent E. coli DH5α cells for transformation.
To construct the recombinant plasmid pPHB01-1, the Q5® site-directed mutagenesis kit (NEB, Ipswich, United States) was used to insert a 57-bp E. coli ρ factor-independent terminator, thrLABC (ECK120033737) between the phaCAB operon and the hok/sok locus in pPHB01. The primers used for constructing pPHB01-1 were In/del-F- pPHB01-1 and In/del-R-pPHB01-1 (Table 2). After PCR, the amplified DNA was treated with a Kinase-Ligase-DpnI enzyme mix at room temperature for 5 min for circularization and template removal. Mutant selection was enriched by simultaneous ligation and DpnI treatment. Next, 5 μL of the KLD mix was directly introduced into competent E. coli DH5α cells. Colony PCR was used for pPHB01-1 screening with the primers CK-F-pPHB01-1 (in-del) and CK-R-pPHB01-1 (in-del) (Table 2). Finally, pPHB01-1 was confirmed by DNA sequencing. All recombinant plasmids constructed in this study were verified by colony PCR, restriction enzyme digestion, and DNA sequencing.
Plasmid Stability Test
Escherichia coli strains DH5α, MG1655, BL21 (DE3), and MZLF (Yang et al., 2016) were used as hosts to test plasmid stability. Cells for the plasmid stability test were grown from -80°C stocks by streaking on an LB plate with ampicillin. After 16–24 h, a single colony was picked and used to inoculate LB containing antibiotics for 12 h. Then, 10 μL of the cell suspensions was inoculated into 3 ml LB without antibiotics to start the stability experiment. The inoculum was diluted and plated on LB plates supplemented with or without antibiotics.
After 12 h, 10 μL of the cell suspensions was transferred to a new test tube. Every 12 h, 10 μL of the sample was transferred to a new flask, and the cells were diluted and plated on LB plates supplemented with or without antibiotics. Plasmid stability (segregation) was estimated by the equation:
Characterization of the Putative Promoter of the phCAB Operon From C. Manganoxidans
The putative promoter of the phaCAB operon was predicted by BPROM (http://www.softberry.com/berry.phtml) and putative ribosome binding sites (RBSs) were reported in a previous study (Lin et al., 2017).
Culture Conditions for PHB Production
The strains used for shake flask experiments were grown aerobically at 200 rpm and 37°C in fresh 25-ml LB medium supplemented with 20 g/L glucose, 0.6 g/L MgSO4 7H2O, 0.07 g/L CaCl2 2H2O, 0.04 g/L KH2PO4, 0.04 g/L K2HPO4, 0.4 g/L NaHCO3 and 100 μg/ml ampicillin. In the shake flask experiments, the initial OD600 was adjusted to 0.05. Samples were taken and stored every 12 h until 48 h for the subsequent analysis.
Analytical Methods
Cell density was monitored by measuring the optical density at 600 nm (GENESYS 10S, Thermo Scientific, United States). For the measurement of biomass concentration, 3 ml of the cultured cells were centrifuged for 1 min at 6,791 rcf. The cells were frozen at −20°C and lyophilized. After freeze-drying, the weight of the sample was recorded to calculate the biomass concentration. The PHB produced by the recombinant E. coli cells was quantified by gas chromatography (GC), and the samples were treated as described before (Hsiao et al., 2016). Briefly, an appropriate amount of biomass was transferred to a clean spiral test tube and 1 ml of chloroform, 0.85 ml of methanol, and 0.15 ml of sulfuric acid were added. The tube was incubated in a water bath for 140 min at 80°C and cooled down to room temperature. Then 1 ml of DI H2O was mixed well by vortexing. After standing and layering, the organic phase was removed and filtered through a 0.2-μm PVDF filter and then analyzed by GC. The temperatures of the injector and detector were 230 and 275°C, respectively. The temperature of the column was set at 100°C and increased to 200°C at a rate of 10°C/min and maintained at 200°C for 2 min. The nitrogen was used as the carrier gas at 3 ml/min. The split mode with the split ratio of 1:10 was used (Chen S.-K. et al., 2013).
The metabolites were determined using a Thermo Scientific™ Dionex™ Ulitmate 3000 LC system equipped with an ORH-801 column. Sulfuric acid (5 mM) was used as the mobile phase, and the flow rate was maintained at 0.6 ml/min. Samples for quantification were collected from the culture media after the removal of the cells by centrifugation for 1 min at 6,791 rcf. The supernatant was passed through a 0.2-μm PVDF filter, and the sample injection (10 μL) was performed using an auto-sampler.
Sodium Dodecyl Sulphate-Polyacrylamide Gel Electrophoresis
The bacterial culture solutions were centrifuged at 4°C and 9,072 × g for 10 min and resuspended in lysis buffer (50 mM Tris, 0.1 M NaCl, 1 mM EDTA, pH 7.8) to obtain a final OD of 20. The concentrated bacterial solutions were subjected to ultrasonication (10 s break and 5 s intermittent, total 60 cycle) (QSonica Q700, United States). The cell lysates were then diluted with 4x Laemmli sample buffer (Bio-Rad Laboratories, Inc., United States) and heated in a dry batch for 10 min. Samples (10 µL) were loaded into the wells for SDS-PAGE analysis (12% acrylamide).
Nile Red Stain Assay
To confirm in vivo PHB production, the recombinant E. coli BL21 (DE3)/pPHB01 was grown on LB plates containing 10 g/L glucose and 0.8 mg/ml nile red. After 24 h of cultivation at 37°C, the plates were exposed to monochromatic light at 470 nm for observation.
Results
Stability of pVEC01, pVEC02, and pVEC03 in E. coli
The recombinant plasmids pVEC01, pVEC02, and pVEC03 were tested for plasmid stability in different E. coli hosts. Compared to the parental plasmid pUC19, pVEC02 showed good stability in E. coli DH5α E. coli BL21 (DE3) and with time required to reach 50% ampicillin-resistant cells of 26 and 336 h, respectively (while pUC19 had times of 25 and 54 h, respectively, Figures 2A,B). The hok/sok system increased the segregational stability in BL21 (DE3) by 6 fold, but not in E. coli K strain. In fact, pVEC01, pVEC02, and pVEC03 were not stable in E. coli K strains DH5α. pVEC01 was slightly more stable than pUC19 in BL21 (DE3) cells and pVEC03 showed instability. Because of the stability of pVEC02 in this test, it was chosen as the new vector for the subsequent induction of PHB production.
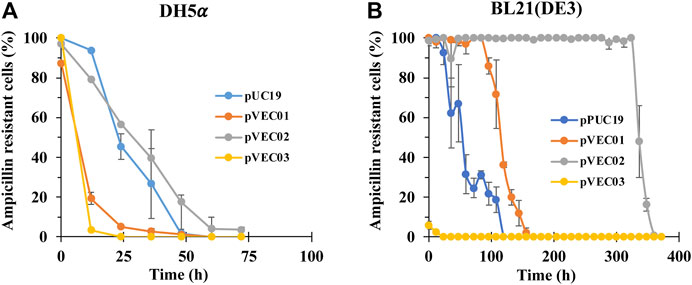
FIGURE 2. The plasmid stability test of pUC19, pVEC01, pVEC02, and pVEC03 in E. coli strains (A) DH5α and (B) BL21 (DE3). Errors represent standard deviation with n = 3.
PHB Production by E. Coli BL21(DE3)/pPHB01 in Shake Flasks
We tested PHB production by conducting shake flask experiments at 37°C under the control of the native promoter of phaCAB operon from C. manganoxidans. Figure 3A shows that E. coli BL21 (DE3)/pPHB01 consumed 18 DE1 and 13 a1 g/L glucose with and without ampicillin, respectively. In the presence of ampicillin, E. coli BL21 (DE3) harboring pPHB01 was able to effectively produce PHB and achieved a PHB concentration of 3.1 DE0.6 g/L (Figure 3B), yield of 0.16 , 0.03 g/g-glucose, and content of 36 /g4% (Figure 3D). In contrast, in the absence of ampicillin, E. coli BL21 (DE3) harboring pPHB01 produced PHB with a decreased concentration of 0.5 w0.1 g/L, as shown in Figures 3B–D. The effective PHB production in E. coli BL21 (DE3)/pPHB01 justifies the high glucose consumption in the presence of ampicillin.
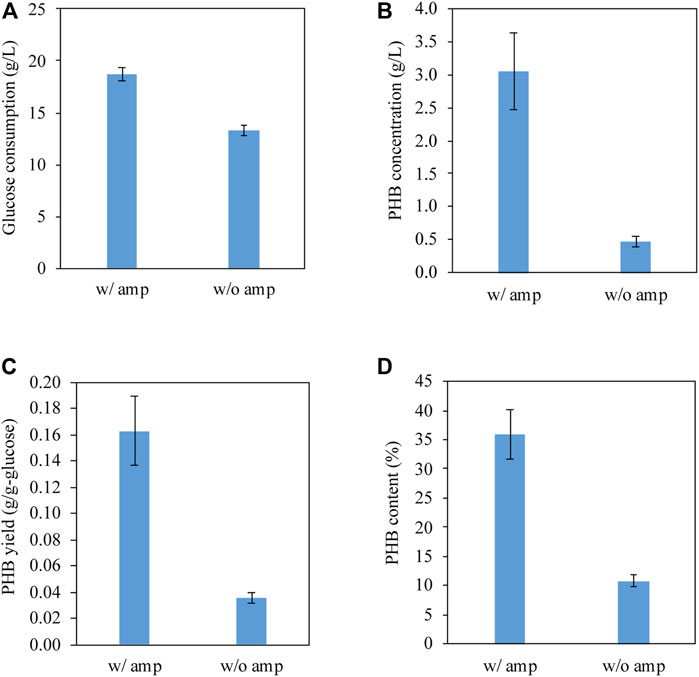
FIGURE 3. (A) Glucose consumption, (B) PHB concentration, (C) PHB yield, and (D) PHB content of E. coli BL21 (DE3)/pPHB01 with and without ampicillin supplementation. The initial glucose concentration for conditions of with and without amp were 19.6 BL0.4 and 18.7 .40.5 g/L, respectively. Errors represent standard deviation with n = 3.
The capability of E. coli BL21 (DE3)/pPHB01-1 to produce PHB without the inducer and antibiotics was confirmed by the Nile red assay, as shown in Figure 4.
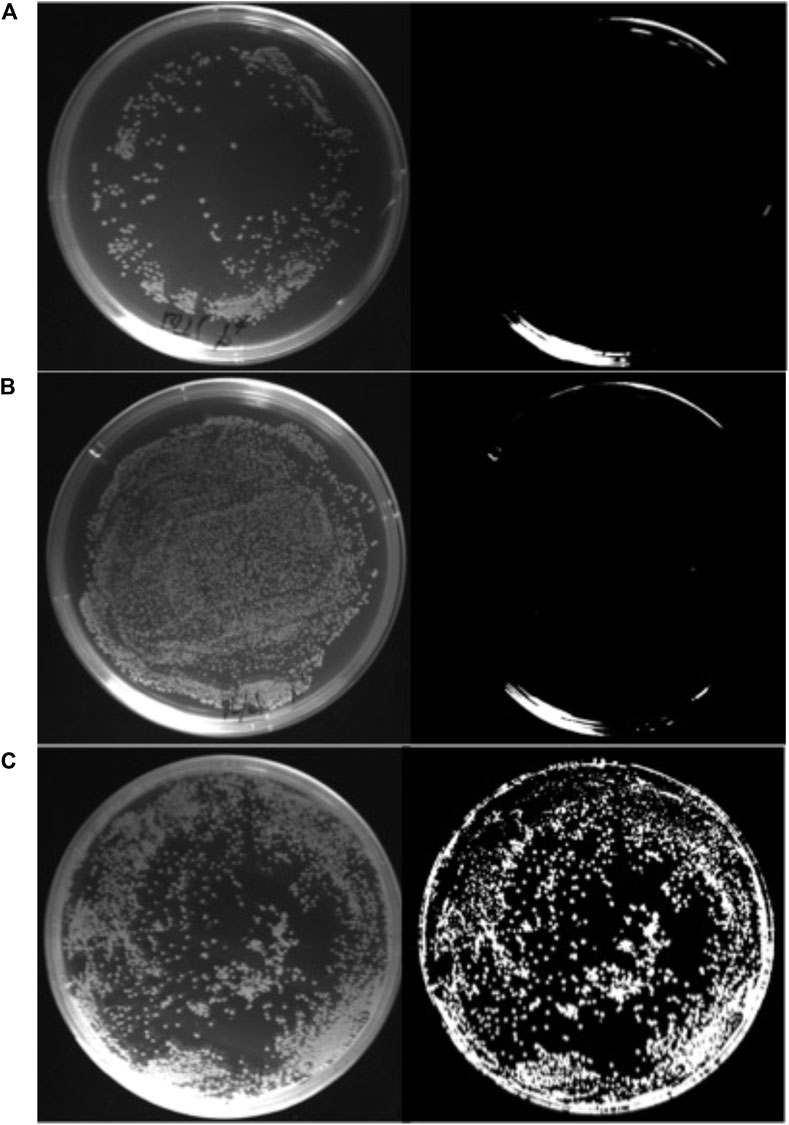
FIGURE 4. Nile red stain assay of (A) E. coli BL21 (DE3), (B) E. coli BL21 (DE3)/pPHB01 (C) E. coli BL21 (DE3)/pPHB01-1. The LB agar plate contained 10 g/L glucose and 0.8 mg/ml nile red without ampicillin. The plate on the left and right in each panel was taken under white light and monochromatic light at 470 nm, respectively.
Characterization of the phaCAB Operon From C. manganoxidans
The putative promoter of the phaCAB operon was predicted by BPROM, and the sequences of the putative promoter, -35 box and -10 box sequences were TTCGATTTCGCAAGGCGGCCGGTGTAAAAA, TTCGAT, and GTGTAAAAA, respectively (Figure 5). Furthermore, RBSs of the phaCAB operon were labeled according to a previous study (Figure 5) (Lin et al., 2017).
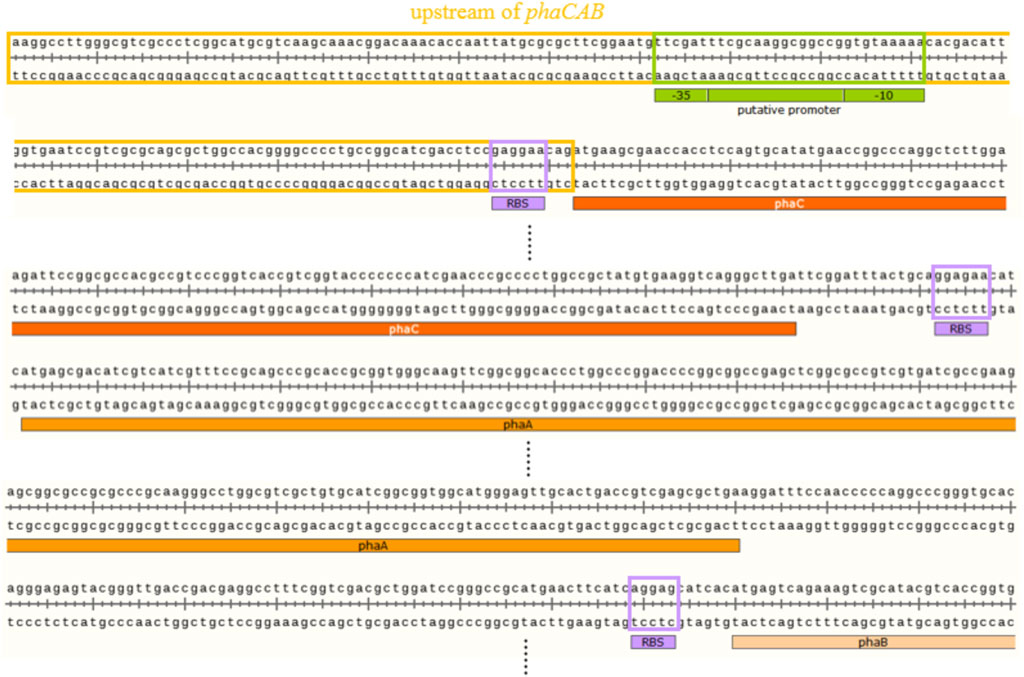
FIGURE 5. The putative promoter of phaCAB operon and the putative ribosome binding sites (RBSs) of phaCAB genes.
Isolation of the phaCAB Operon and Hok/Sok Genetic Units by Inserting a Terminator
While pVEC02 (hok/sok) was shown to be a stable vector in E. coli without ampicillin supplementation, the low PHB production by E. coli BL21 (DE3)/pPHB01 in the absence of ampicillin indicates the instability of pPHB01 in E. coli BL21 (DE3). It is speculated that the insertion of the phaCAB operon in pVEC02 disrupted the balance of hok/sok transcripts. In other words, the promoter of the phaCAB operon may carry an additional transcript of hok and make pPHB01 toxic to E. coli (Figure 6). To verify this speculation, an efficient E. coli ρ factor-independent terminator, thrLABC (ECK120033737) (Chen Y.-J. et al., 2013), was inserted between the phaCAB operon and the hok/sok locus to isolate the two genetic units (Figure 6). The newly constructed plasmid was named pPHB01-1.
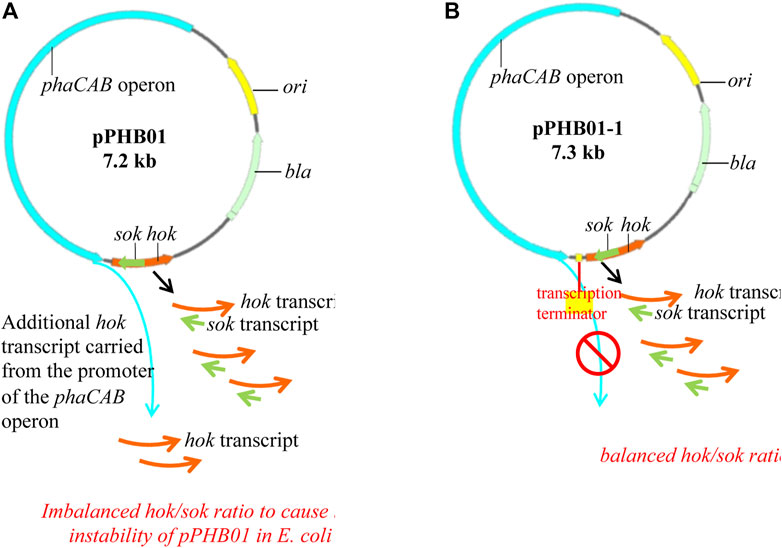
FIGURE 6. Maps of (A) pPHB01 and (B) pPHB01-1, where pPHB01-1 was constructed by inserting a 57-bp E. coli ρ factor-independent terminator (thrLABC, ECK120033737) into the pPHB01 to isolate the phaCAB operon and the hok/sok system.
E. coli BL21 (DE3) harboring the newly constructed plasmid pPHB01-1 in was tested for the PHB production with and without ampicillin supplementation (Figure 7). In the presence of ampicillin, E. coli BL21 (DE3)/pPHB01-1 was able to produce PHB effectively. All the fed glucose of 13 DE3 g/L was completely consumed in 48 h (Figure 7A), and the PHB concentration, yield, and content were 3.1 , 0.3 g/L, 0.26 /L0.08 g/g-glucose, and 45 /g4%, respectively (Figures 7B–D). In the absence of ampicillin supplementation, E. coli BL21 (DE3)/pPHB01-1 was still able to provide a comparable PHB performance, where the PHB concentration, yield, and content was 3.0 PH0.2 g/L, 0.26 /L0.07 g/g-glucose, and 44 /g3%, respectively (Figures 7B–D).
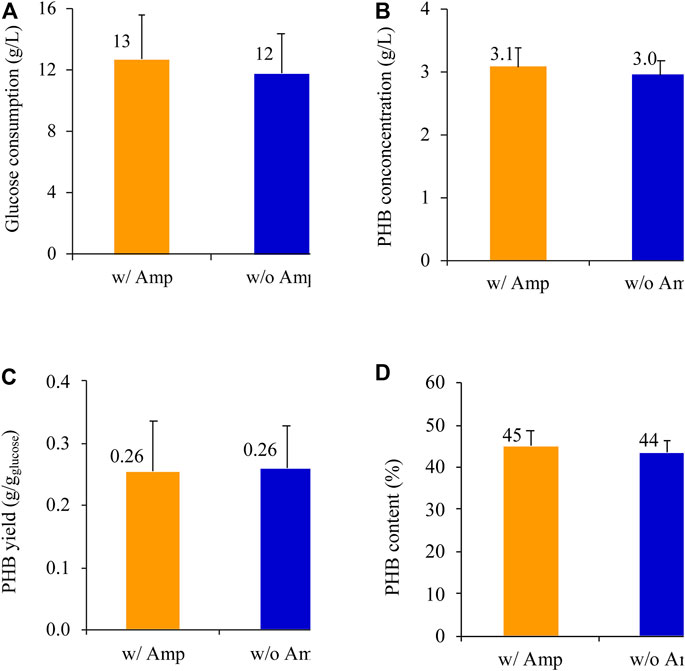
FIGURE 7. (A) Glucose consumption, (B) PHB conconcentration, (C) PHB yield, and (D) PHB content of E. coli BL21 (DE3)/pPHB01-1 with out and with 100 μg/ml ampicillin. The initial glucose concentration for conditions of with and without amp were 13 am3 and 12 a3 g/L, respectively. Errors represent standard deviation with n = 3.
To characterize the long-term stability of PHB production in E. coli BL21 (DE3)/pPHB01-1, E. coli BL21 (DE3)/pPHB01-1 was first cultivated in the liquid culture without the ampicillin (the medium composition was described in 2.5) and transferred to a fresh medium every 12 h. During the transfer, the grown-culture solution was spread on the agar plate without the ampicillin but 0.8 mg/ml nile red. After the formation of CFU, the total CFU as well as the fluorescent CFU were counted to quantify the percentage of CFU that can produce PHB. It can be seen in Figure 8A that the OD600, which is positively correlated to the PHB production, can be maintained in the range of 15 and 18 in the first 84 h and gradually dropped to 5.1 a0.1 at 132 h. Figure 8B shows that the E. coli BL21 (DE3)/pPHB01-1 maintained its ability to produce PHB in the first 96 h and less than 20% of cell population can produce PHB afterwards.
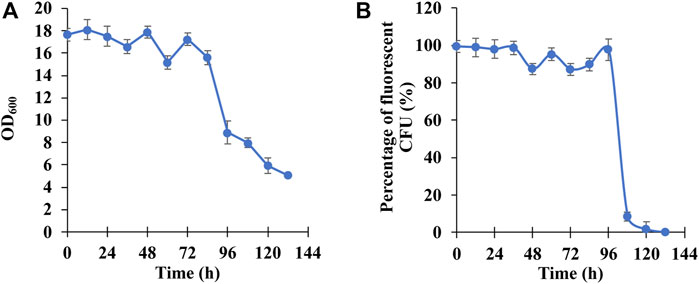
FIGURE 8. (A) OD600 and (B) percentage of fluorescent colony forming unit (CFU) of E. coli BL21 (DE3)/pPHB01-1 for the long-term PHB production. Errors represent standard deviation with n = 3.
SDS-PAGE of E. Coli BL21(DE3)/pPHB01 and E. Coli BL21(DE3)/pPHB01-1
SDS-PAGE was used to confirm the expression of the phaCAB operon derived from C. manganoxidans in E. coli. It can be clearly seen in Figure 9 (lanes 7–12) that three overexpressed signals were found in both E. coli BL21 (DE3)/pPHB01 and E. coli BL21 (DE3)/pPHB01-1 samples, which correspond to PhaC (61 kDa), PhaA (43 kDa), and PhaB (27 kDa). PhaA and PhaB in E. coli BL21 (DE3)/pPHB01 and E. coli BL21 (DE3)/pPHB01-1 were mainly found in the soluble fractions (lanes 8 and 11 of Figure 9), while PhaC was mainly found in the insoluble fraction (lanes 9 and 12 of Figure 9).
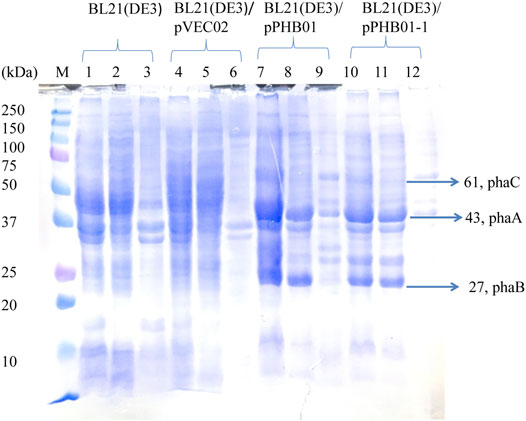
FIGURE 9. SDS-PAGE of E. coli BL21 (DE3)/pPHB01 and BL21 (DE3)/pPHB01-1. M: marker; lane 1- lane 3: BL21 (DE3); lane 4 - lane 6: BL21 (DE3)/pVEC02; lane 7- lane 9: BL21 (DE3)/pPHB01; lane 10 - lane 12: BL21 (DE3)/pPHB0-1. Lane 1, 4, 7, 10: whole cells; lane 2, 5, 8, 11: soluble protein; lane 3, 6, 9, 12: insoluble fractions.
Discussion
Synthetic biology has gradually become the keystone of our current technology-based society (Tseng et al., 2018; Voigt, 2020; Pelletier et al., 2021). The management of synthetic biology relies on the characterization of genetic units and their interactions. Plasmid system is a general platform to carry genetic information and therefore plasmid instability becomes a concern to maintain the newly installed traits. In previous studies, hok/sok was found to be a potential system to increase the plasmid stability in E. coli strains (Gerdes et al., 1986; Schweder et al., 1992; Lee et al., 1994). Multiple copies of the hok/sok locus in the target plasmid can further improve the stability of the plasmid in E. coli (Gerdes et al., 1986; Lee et al., 1994; Kim and Kang, 1996; Pecota et al., 1997). However, it is common to see the expression of recombinant proteins cause the imbalance of the hok/sok system, where transcription and translation are simultaneously involved in the plasmid maintenance (Lee et al., 1994; Wu and Wood, 1994). Therefore, the combination of plasmid maintenance systems as a hybrid one was proposed to increase the plasmid stability and the time to reach 50% plasmid-bearing cells was extended to ∼ 72 h (Pecota et al., 2003).
In this study, a high-copy number backbone, pUC19, was chosen since it may benefit recombinant protein production and metabolic engineering. PSK and active partition systems are usually found in a low-copy number plasmid. It will be interesting to see how well PSK and active partition systems maintain the high-copy pUC19 in E. coli. This study clearly demonstrated that alp7 system was incompatibility with the high-copy backbone. This may because the active partition of the plasmid consumes ATP during DNA replication (Baxter and Funnell, 2015). Energy consumption is critical for the function of alp7, and the installation of alp7 in a high-copy number backbone may consume too much ATP, making alp7/pUC19 incompatible with E. coli. In contrast, the genetic unit hok/sok in pVEC02 was found to be an efficient antibiotic-free selection marker in E. coli B strains, including E. coli BL21 (DE3) and MZLF. The time to reach 50% ampicillin-resistant cells can go up to 360 h, which is competitive among literatures. Perplexedly, this study presented a result that the hok/sok system was not compatible with E. coli K strains, including MG1655 and DH5α. E. coli K and B strains bear 5 and 6 hok/sok loci in the chromosome, respectively (Schneider et al., 2002), and all of them are considered inactive yet may be induced by unknown signal (Pedersen and Gerdes, 1999). More study can be conducted in detail in the future to discriminate the compatibility of the hok/sok system among E. coli strains.
The application of the antibiotic-free vector pVEC02 was further investigated in metabolic engineering for PHB production by constructing the recombinant pPHB01. E. coli BL21 (DE3)/pPHB01 can effectively produce PHB; however, only in the presence of antibiotics. The ineffective function of the hok/sok system in pPHB01 demonstrates that the hok/sok system in the engineering perspective needs more detailed investigation. We focused on the interaction among genetic units to determine the antibiotic demand of pPHB01. It is speculated that the transcription activity of the phaCAB operon passes downstream of the hok/sok gene unit. The transcriptional carry-over increases hok transcription; therefore, the hok/sok balance is interrupted. This carry-over of the transcription activity can be especially severe when the downstream hok is a short transcript. To prevent transcriptional carry-over, an efficient E. coli ρ factor-independent terminator, thrLABC (ECK120033737) (Chen Y.-J. et al., 2013), was inserted between the phaCAB operon and the hok/sok gene unit (Figure 7). The isolation of the two genetic units was shown in this study to be effective in finding an application for pVEC02 in metabolic engineering. In addition, a putative promoter region of the phaCAB operon from C. manganoxidans was first shown properly while effectively function in E. coli. To our knowledge, this is the first study to demonstrate that the phaCAB operon from C. manganoxidans can be used for effective PHB production in E. coli. A previous study showed that the expression level of PhaC was important for the phaCAB-mediated pathway (Hiroe et al., 2012). As shown in Figure 9 that PhaC expression level was not strong, the PhaC expression level of the phaCAB operon in E. coli can be further optimized in the future. In summary, the inducer-free PHB production can be achieved by adopting heterologous promoter from C. manganoxidans. The antibiotic-free PHB production involves the interplay among bacteria chassis, antibiotic-free genetic markers, and the genetic operon for PHB production. This study presents a right combination to achieve the inducer- and antibiotic-free system for PHB production.
Data Availability Statement
The original contributions presented in the study are included in the article/Supplementary Material, further inquiries can be directed to the corresponding author.
Author Contributions
Y-CL and BS conducted experiments and wrote the draft of the manuscript. J-WC and X-ZZ conducted experiments. Y-CL, BS, J-WC, X-ZZ, and S-YL analyzed the data. S-YL conceived and supervised the study along with editing the manuscript.
Funding
This work was funded by the Ministry of Science and Technology Taiwan MOST-108-2628-E-005-002-MY3, MOST-110-2622-E-005-011-CC2, and the Ministry of Education, Taiwan, R.O.C. under the Higher Education Sprout Project.
Conflict of Interest
The authors declare that the research was conducted in the absence of any commercial or financial relationships that could be construed as a potential conflict of interest.
Publisher’s Note
All claims expressed in this article are solely those of the authors and do not necessarily represent those of their affiliated organizations, or those of the publisher, the editors and the reviewers. Any product that may be evaluated in this article, or claim that may be made by its manufacturer, is not guaranteed or endorsed by the publisher.
References
Akiyama, H., Okuhata, H., Onizuka, T., Kanai, S., Hirano, M., Tanaka, S., et al. (2011). Antibiotics-free Stable Polyhydroxyalkanoate (PHA) Production from Carbon Dioxide by Recombinant Cyanobacteria. Bioresour. Technol. 102, 11039–11042. doi:10.1016/j.biortech.2011.09.058
Baxter, J. C., and Funnell, B. E. (2015). “Plasmid Partition Mechanisms,” in Plasmids: Biology and Impact in Biotechnology and Discovery American Society for Microbiology ASM, 135–155. doi:10.1128/9781555818982.ch8
Boontip, T., Waditee-Sirisattha, R., Honda, K., and Napathorn, S. C. (2021). Strategies for Poly(3-Hydroxybutyrate) Production Using a Cold-Shock Promoter in Escherichia coli. Front. Bioeng. Biotechnol. 9, 666036. doi:10.3389/fbioe.2021.666036
Chen, S.-K., Chin, W.-C., Tsuge, K., Huang, C.-C., and Li, S.-Y. (2013a). Fermentation Approach for Enhancing 1-butanol Production Using Engineered Butanologenic Escherichia coli. Bioresour. Technol. 145, 204–209. doi:10.1016/j.biortech.2013.01.115
Chen, Y.-J., Liu, P., Nielsen, A. A. K., Brophy, J. A. N., Clancy, K., Peterson, T., et al. (2013b). Characterization of 582 Natural and Synthetic Terminators and Quantification of Their Design Constraints. Nat. Methods 10, 659–664. doi:10.1038/nmeth.2515
Danino, T., Prindle, A., Kwong, G. A., Skalak, M., Li, H., Allen, K., et al. (2015). Programmable Probiotics for Detection of Cancer in Urine. Sci. Transl Med. 7, 289ra84. doi:10.1126/scitranslmed.aaa3519
Derman, A. I., Becker, E. C., Truong, B. D., Fujioka, A., Tucey, T. M., Erb, M. L., et al. (2009). Phylogenetic Analysis Identifies many Uncharacterized Actin-like Proteins (Alps) in Bacteria: Regulated Polymerization, Dynamic Instability and Treadmilling in Alp7A. Mol. Microbiol. 73, 534–552. doi:10.1111/j.1365-2958.2009.06771.x
Derman, A. I., Nonejuie, P., Michel, B. C., Truong, B. D., Fujioka, A., Erb, M. L., et al. (2012). Alp7R Regulates Expression of the Actin-like Protein Alp7A in Bacillus Subtilis. J. Bacteriol. 194, 2715–2724. doi:10.1128/jb.06550-11
Ferreira, R. d. G., Azzoni, A. R., and Freitas, S. (2018). Techno-economic Analysis of the Industrial Production of a Low-Cost Enzyme Using E. coli: the Case of Recombinant β-glucosidase. Biotechnol. Biofuels 11, 81. doi:10.1186/s13068-018-1077-0
Friehs, K. (2004). “Plasmid Copy Number and Plasmid Stability,” in New Trends and Developments in Biochemical Engineering (Springer), 47–82. doi:10.1007/b12440
Gerdes, K., Rasmussen, P. B., and Molin, S. (1986). Unique Type of Plasmid Maintenance Function: Postsegregational Killing of Plasmid-free Cells. Proc. Natl. Acad. Sci. U.S.A. 83, 3116–3120. doi:10.1073/pnas.83.10.3116
Hiroe, A., Tsuge, K., Nomura, C. T., Itaya, M., and Tsuge, T. (2012). Rearrangement of Gene Order in the phaCAB Operon Leads to Effective Production of Ultrahigh-Molecular-Weight Poly[( R )-3-Hydroxybutyrate] in Genetically Engineered Escherichia coli. Appl. Environ. Microbiol. 78, 3177–3184. doi:10.1128/aem.07715-11
Hsiao, L.-J., Lin, J.-H., Sankatumvong, P., Wu, T.-M., and Li, S.-Y. (2016). The Feasibility of Thermophilic Caldimonas Manganoxidans as a Platform for Efficient PHB Production. Appl. Biochem. Biotechnol. 180, 852–871. doi:10.1007/s12010-016-2138-0
Islam, M. N., Lee, K. W., Yim, H.-S., Lee, S. H., Jung, H. C., Lee, J.-H., et al. (2017). Optimizing T4 DNA Polymerase Conditions Enhances the Efficiency of One-step Sequence- and Ligation-independent Cloning. BioTechniques 63, 125–130. doi:10.2144/000114588
Jeong, J.-Y., Yim, H.-S., Ryu, J.-Y., Lee, H. S., Lee, J.-H., Seen, D.-S., et al. (2012). One-Step Sequence- and Ligation-independent Cloning as a Rapid and Versatile Cloning Method for Functional Genomics Studies. Appl. Environ. Microbiol. 78, 5440–5443. doi:10.1128/aem.00844-12
Kim, J.-Y., and Kang, H. A. (1996). Comparison of Effects of the Par Locus and the parB Locus on Plasmid Stability and Host Cell Growth. J. Ferment. Bioeng. 82, 495–497. doi:10.1016/s0922-338x(97)86990-5
Kroll, J., Klinter, S., Schneider, C., Voss, I., and Steinbüchel, A. (2010). Plasmid Addiction Systems: Perspectives and Applications in Biotechnology. Microb. Biotechnol. 3, 634–657. doi:10.1111/j.1751-7915.2010.00170.x
Laguna, R., Young, S. J., Chen, C.-C., Ruiz, N., Yang, S.-T., and Tabita, F. R. (2015). Development of a Plasmid Addicted System that Is Independent of Co-inducers, Antibiotics and Specific Carbon Source Additions for Bioproduct (1-butanol) Synthesis in Escherichia coli. Metab. Eng. Commun. 2, 6–12. doi:10.1016/j.meteno.2014.12.001
Lee, S. Y., Yim, K. S., Chang, H. N., and Chang, Y. K. (1994). Construction of Plasmids, Estimation of Plasmid Stability, and Use of Stable Plasmids for the Production of Poly(3-Hydroxybutyric Acid) by Recombinant Escherichia coli. J. Biotechnol. 32, 203–211. doi:10.1016/0168-1656(94)90183-x
Li, M. Z., and Elledge, S. J. (2007). Harnessing Homologous Recombination In Vitro to Generate Recombinant DNA via SLIC. Nat. Methods 4, 251–256. doi:10.1038/nmeth1010
Lin, J.-H., Lee, M.-C., Sue, Y.-S., Liu, Y.-C., and Li, S.-Y. (2017). Cloning of phaCAB Genes from Thermophilic Caldimonas Manganoxidans in Escherichia coli for Poly(3-Hydroxybutyrate) (PHB) Production. Appl. Microbiol. Biotechnol. 101, 6419–6430. doi:10.1007/s00253-017-8386-2
Million-Weaver, S., Camps, M., and Camps, M. (2014). Mechanisms of Plasmid Segregation: Have Multicopy Plasmids Been Overlooked? Plasmid 75, 27–36. doi:10.1016/j.plasmid.2014.07.002
Nordström, K., and Austin, S. J. (1989). Mechanisms that Contribute to the Stable Segregation of Plasmids. Annu. Rev. Genet. 23, 37–69.
Pecota, D. C., Kim, C. S., Wu, K., Gerdes, K., and Wood, T. K. (1997). Combining the Hok/sok, parDE, and Pnd Postsegregational Killer Loci to Enhance Plasmid Stability. Appl. Environ. Microbiol. 63, 1917–1924. doi:10.1128/aem.63.5.1917-1924.1997
Pecota, D., Osapay, G., Selsted, M., and Wood, T. (2003). Antimicrobial Properties of the R1 Plasmid Host Killing Peptide. J. Biotechnol. 100, 1–12. doi:10.1016/s0168-1656(02)00240-7
Pedersen, K., and Gerdes, K. (1999). Multiple Hok Genes on the Chromosome of Escherichia coli. Mol. Microbiol. 32, 1090–1102. doi:10.1046/j.1365-2958.1999.01431.x
Pelletier, J. F., Sun, L., Wise, K. S., Assad-Garcia, N., Karas, B. J., Deerinck, T. J., et al. (2021). Genetic Requirements for Cell Division in a Genomically Minimal Cell. Cell 184, 2430–2440. e2416. doi:10.1016/j.cell.2021.03.008
Peña, C., Castillo, T., García, A., Millán, M., and Segura, D. (2014). Biotechnological Strategies to Improve Production of Microbial Poly‐(3‐hydroxybutyrate): a Review of Recent Research Work. Microb. Biotechnol. 7, 278–293.
Sayeed, S., Reaves, L., Radnedge, L., and Austin, S. (2000). The Stability Region of the Large Virulence Plasmid of Shigella Flexneri Encodes an Efficient Postsegregational Killing System. J. Bacteriol. 182, 2416–2421. doi:10.1128/jb.182.9.2416-2421.2000
Schneider, D., Duperchy, E., Depeyrot, J., Coursange, E., Lenski, R. E., and Blot, M. (2002). Genomic Comparisons Among Escherichia coli Strains B, K-12, and O157:H7 Using IS Elements as Molecular Markers. BMC Microbiol. 2, 18. doi:10.1186/1471-2180-2-18
Schweder, T., Schmidt, I., Herrmann, H., Neubauer, P., Hecker, M., and Hofmann, K. (1992). An Expression Vector System Providing Plasmid Stability and Conditional Suicide of Plasmid-Containing Cells. Appl. Microbiol. Biotechnol. 38, 91–93. doi:10.1007/BF00169425
Singha, T. K., Gulati, P., Mohanty, A., Khasa, Y. P., Kapoor, R. K., and Kumar, S. (2017). Efficient Genetic Approaches for Improvement of Plasmid Based Expression of Recombinant Protein in Escherichia coli : A Review. Process Biochem. 55, 17–31. doi:10.1016/j.procbio.2017.01.026
Stubbe, J., Tian, J., He, A., Sinskey, A. J., Lawrence, A. G., and Liu, P. (2005). Nontemplate-dependent Polymerization Processes: Polyhydroxyalkanoate Synthases as a Paradigm. Annu. Rev. Biochem. 74, 433–480. doi:10.1146/annurev.biochem.74.082803.133013
Tseng, I.-T., Chen, Y.-L., Chen, C.-H., Shen, Z.-X., Yang, C.-H., and Li, S.-Y. (2018). Exceeding the Theoretical Fermentation Yield in Mixotrophic Rubisco-Based Engineered Escherichia coli. Metab. Eng. 47, 445–452. doi:10.1016/j.ymben.2018.04.018
Voigt, C. A. (2020). Synthetic Biology 2020-2030: Six Commercially-Available Products that Are Changing Our World. Nat. Commun. 11, 6379. doi:10.1038/s41467-020-20122-2
Wu, K., and Wood, T. K. (1994). Evaluation of Thehok/sok Killer Locus for Enhanced Plasmid Stability. Biotechnol. Bioeng. 44, 912–921. doi:10.1002/bit.260440807
Yang, C. H., Liu, E. J., Chen, Y. L., Ou-Yang, F. Y., and Li, S. Y. (2016). The Comprehensive Profile of Fermentation Products during In Situ CO2 Recycling by Rubisco-Based Engineered Escherichia coli. Microb. Cel Fact 15, 133–210. doi:10.1186/s12934-016-0530-7
Keywords: antibiotic-free vector, hok/sok, Alp7A, inducer-free fermentation, polyhydroxybutyrate
Citation: Liao Y-C, Saengsawang B, Chen J-W, Zhuo X-Z and Li S-Y (2022) Construction of an Antibiotic-Free Vector and its Application in the Metabolic Engineering of Escherichia Coli for Polyhydroxybutyrate Production. Front. Bioeng. Biotechnol. 10:837944. doi: 10.3389/fbioe.2022.837944
Received: 17 December 2021; Accepted: 11 March 2022;
Published: 03 June 2022.
Edited by:
Shota Atsumi, University of California, Davis, United StatesReviewed by:
Jian Wang, University of Georgia, United StatesMin-Kyu Oh, Korea University, South Korea
Copyright © 2022 Liao, Saengsawang, Chen, Zhuo and Li. This is an open-access article distributed under the terms of the Creative Commons Attribution License (CC BY). The use, distribution or reproduction in other forums is permitted, provided the original author(s) and the copyright owner(s) are credited and that the original publication in this journal is cited, in accordance with accepted academic practice. No use, distribution or reproduction is permitted which does not comply with these terms.
*Correspondence: Si-Yu Li, c3lsaUBkcmFnb24ubmNodS5lZHUudHc=