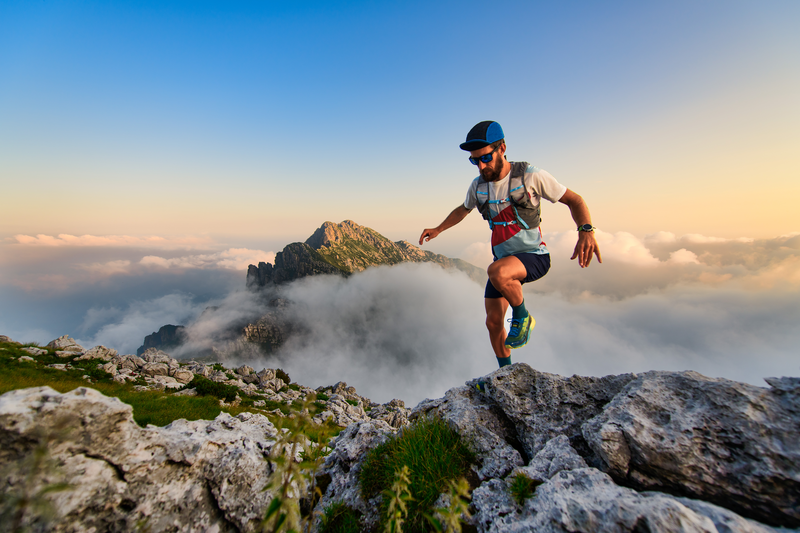
94% of researchers rate our articles as excellent or good
Learn more about the work of our research integrity team to safeguard the quality of each article we publish.
Find out more
ORIGINAL RESEARCH article
Front. Bioeng. Biotechnol. , 22 March 2022
Sec. Biomechanics
Volume 10 - 2022 | https://doi.org/10.3389/fbioe.2022.831545
Background: Injury to the medial collateral ligament (MCL) of the elbow has become increasingly common in sports, and the elbow is prone to contracture and ossification in trauma. Effective reconstruction of the MCL with reduction of irritation to the elbow has rarely been reported. This study introduces a minimally invasive elbow MCL reconstruction technique and evaluates the valgus stability.
Methods: Eight fresh-frozen elbow specimens underwent reconstruction of the anterior bundle of the MCL (AMCL) first with the flexor carpi ulnaris fascia patches, followed by reconstruction of the posterior bundle of the MCL (PMCL) with the triceps tendon patches. The valgus angles of each specimen were examined in three stages as follows: intact MCL, reconstruction of the AMCL alone, and reconstruction of the MCL (including AMCL and PMCL). Finally, specimens were loaded to failure, and failure modes were recorded.
Results: AMCL reconstruction alone had similar valgus stability at all testing angles (p = 0.080, 30° flexion; p = 0.064, 60° flexion; p = 0.151, 90° flexion; p = 0.283, 120° flexion) compared with the intact MCL, as did MCL reconstruction (p = 0.951, 30° flexion; p = 0.739, 60° flexion; p = 0.841, 90° flexion; p = 0.538, 120° flexion). More importantly, a significant difference existed between the MCL reconstruction and the AMCL reconstruction alone at 30° flexion (p = 0.043) and 60° flexion (p = 0.013) but not at the 90° flexion (p = 0.369) and 120° flexion (p = 0.879). The mean maximum failure torque of MCL reconstruction was 24.02 Nm at 90° elbow flexion.
Conclusion: Both AMCL reconstruction alone and MCL reconstruction provided valgus stability comparable with the native MCL, and importantly, MCL reconstruction provided more valgus stability than AMCL reconstruction alone at 30° flexion and 60° flexion of the elbow. Therefore, the new MCL reconstruction technique might be a useful guide for the treatment of elbow MCL injuries or deficiencies.
In sports injuries, damage to the medial collateral ligament (MCL) of the elbow has become increasingly common (Petty et al., 2004). In elbow trauma, the elbow soft tissue develops posttraumatic contracture in 50% of patients (Anakwe et al., 2011) who experience elbow dislocation or fracture; furthermore, the elbow is prone to heterotopic ossification (HO) in 37% of patients who undergo open reduction and internal fixation (Foruria et al., 2013). The elbow is sensitive to surgical trauma, which is a risk factor for stiffness progression (Qian et al., 2020). The tendon grafts used in reconstruction techniques were confirmed to have undergone ossification (Wear et al., 2011; Kodde et al., 2012; Andrachuk et al., 2016) and occurred in the early stages of ligament reconstruction (Park et al., 2018). Considering that the elbow is sensitive to trauma, it is a severe challenge to reduce irritation to the elbow and effectively reconstruct the MCL.
Previous studies demonstrated that the anterior bundle of the MCL (AMCL) was the primary restraint to valgus stress (Morrey and An, 1983; Hassan et al., 2015; Frangiamore et al., 2017), the posterior bundle of the MCL (PMCL) could resist motion of the humero-ulnar joint (Shukla et al., 2016), and the transverse bundle of the MCL had little effect on maintaining medial stability (Morrey and An, 1983). Based on the importance of AMCL, many alternative reconstruction techniques for the role of AMCL had been proposed (Rohrbough et al., 2002; Paletta and Wright, 2006; Schwartz et al., 2008; Cain et al., 2010). The reconstruction techniques, using tendon grafts and bulky bone tunnels, showed good results in stabilizing the medial elbow (Rohrbough et al., 2002; Paletta and Wright, 2006; Schwartz et al., 2008; Cain et al., 2010), but it exposed the elbow to further trauma, causing a 5% odds of HO after MCL reconstruction (Park et al., 2018). Simultaneously, traditional reconstruction techniques demanded additional incisions for obtaining tendon grafts that might cause dysfunction in the tendon supply area (Vitale and Ahmad, 2008; Cain et al., 2010). Therefore, a less invasive ligament reconstruction technique is urgently needed for those patients who are prone to contracture or ossification.
With advancements in research on AMCL, some authors reported that the proximal and distal parts of the insertion of the AMCL played different roles in maintaining medial elbow stability (Hassan et al., 2015; Frangiamore et al., 2017). In addition, some reports claimed that the PMCL played a vital part in maintaining the posteromedial stability of the elbow and suggested a reconstruction of the PMCL after injury (Shukla et al., 2016; Shukla et al., 2018).
Considering the complication rate of elbow surgery and the role of AMCL and PMCL, we performed the fan shape reconstruction technique, which was minimally invasive to the bone. In this study, we introduce the new MCL reconstruction technique and evaluate the valgus stability with biomechanical analyses. We hypothesized that this technique could provide the same valgus stability as intact ligaments.
Eight fresh-frozen human cadaveric upper extremities (three women and five men; donated for medical research to a tissue bank and purchased by our institution) were used for this study. The mean age of the cadavers was 61.1 years (age range: 52–67 years). The specimens were stored at −20°C and thawed at room temperature for 24 h. We preserved the hand, forearm, and distal half of the upper arm, including the skin and muscles.
Using a medial incision, we carefully split the flexor-pronator muscle mass without damaging the flexor carpi ulnaris fascia patch and exposed the origins and insertions of the MCL (Supplementary Video S1). The flexor carpi ulnaris fascia patch was released from three sides of the muscle surface, whereas the proximal end remained intact 2 cm distal to the joint line (Figure 1A). The size of the fascia patch was approximately 6 cm long, 1 cm wide, and 2 mm thick (Figure 1A). The triceps tendon patch was released on three sides with the distal end at the insertion (Figure 1E). The size of the tendon patch was approximately 3 cm long, 0.5 cm wide, and 2 mm thick (Figure 1E). To prevent the patches from being further torn, the patches were sutured to their terminals by absorbable sutures.
FIGURE 1. Illustration of technical procedures. (A) Preparation of fascia patch; (B,C) course of fascia patch; (D) option of reconstruction location, a: origin point of AMCL, b: center of ulnar attachments of AMCL on ulnar ridge; (E) creation of bone tunnels and preparation of triceps tendon patch; (F) course of triceps tendon patch; (G) fixation of fascia and tendon patches; (H) suture of remaining fascia patch and common flexor tendon, anterior transposition of ulnar nerve.
The MCL reconstruction was performed at 90° of elbow flexion. At the stage of reconstruction of the AMCL alone, the prepared fascia patch was placed beneath the flexor carpi ulnaris muscle, close to the posteromedial part of the sublime tubercle, and extended proximally to the medial epicondyle (Figure 1B). The fascial patch was tensioned toward the medial epicondyle to determine the appropriate length and then fixed at the origin of the AMCL using a suture anchor (Twinfix; Smith & Nephew, Andover, MA, USA). Then, the patch was reversed and tensioned toward the ulnar ridge (Figure 1C). After obtaining the proper length, the fascia patch was fixed on the ulnar ridge with a suture anchor, distal to the sublime tubercle, and approximately 7 mm distal to the joint line, at the center of ulnar attachments of the AMCL (Figure 1D) (Frangiamore et al., 2018). A 2.5-mm K-wire was used to create suture anchor tunnels in the sublime tubercle and the medial epicondyle (Figure 1E). These drill holes did not broach the distal bone cortex. At the stage of reconstruction of the PMCL (Figure 1F), the triceps tendon patch was tensioned toward the medial epicondyle and fixed at the same point with the fascial patch on the medial epicondyle after determining the appropriate length of the tendon patch. The MCL reconstruction with strips A, B, and C resembles a fan shape (Figure 1G), named the Fan technique. After MCL reconstruction, the ulnar nerve was transposed subcutaneously and anteriorly, and then, the remaining flexor carpi ulnaris fascia patch and the common flexor tendon were sutured separately with interrupted sutures (Figure 1H).
The specimens were tested on the Instron 5569 Materials Testing System (Instron Corp, Canton, MA, USA). The proximal end of the specimen was potted in a custom fixture (Figure 2, Supplementary Figures S1, S2) using denture base acrylic resins. The distal end was fixed in the slider, which could not rotate to ensure that the forearm was in a neutral position and could only slide horizontally. One end of the 0.2-mm flexible steel wire rope was placed on the ulna, 20 cm from the elbow joint activity center, wherein loading this location created an elbow valgus moment. The other end was fixed in a small module, which could be clamped by the MTS clamps. By adjusting the pulley position, the wire rope could be first passed horizontally through the bottom of the pulley and then vertically upward linked to the MTS system. A preload of 2 Nm was applied, followed by 10 cycles, and held a final 2 Nm load for 60 s (Dugas et al., 2016). Kinematics was defined as the joint coordinate system of ulnar motion relative to the humerus, as recommended by the International Society of Biomechanics (Wu et al., 2005). The valgus angle was measured by the three-dimensional motion-capture system (Optotrak Certus motion-capture system; Northern Digital Inc., Waterloo, ON, Canada) at a torque of 2 Nm. One smart marker set was attached to the forearm and the other to the humerus. One smart marker set contained three markers, which consisted of infrared diodes. The marker set acted as a single “rigid body,” allowing it to specify its position in space. The markers allowed determining the relative displacement of the two rigid bodies (the forearm and humerus). The three-dimensional motion-capture system could calculate the coordinates of the markers, the centroid coordinates, and orientation angles. The MATLAB software (version R2016; Math Works, Natick, MA) was used to analyze the elbow valgus angle from the motion trajectory.
FIGURE 2. Schematic illustration of testing system. This image shows test under valgus stress with 2 Nm of torque, whereas elbow is in 90° of flexion, and forearm is in neutral.
Each specimen was operated on in three different stages (Table 1). After stage A, the AMCL and PMCL were transected, then stages B and C were performed. After all testing, the specimen was positioned at 90° of flexion and loaded to failure at a rate of 0.2 mm/s. Failure was defined as a sudden decrease in tension with increasing elbow valgus. The maximum tension and the failure mode of MCL reconstruction were recorded.
Statistical data were analyzed using SPSS (IBM Corporation Ltd., Armonk, NY, USA). Each variable was reported as the actual measured value or the mean ± standard deviation (95% confidence intervals). After verification of the normal distribution, Levene's test was used to assess the equality of variance. Values were compared by use of one-way analysis of variance. Last, Tukey's post-hoc test was used to adjust for multiple comparisons with a significance criterion of p < 0.05.
A significant difference existed among the intact MCL, AMCL reconstruction alone, and the MCL reconstruction at 30° (p = 0.033) and 60° flexion (p = 0.013) but not at 90° (p = 0.160) and 120° flexion (p = 0.298) (Table 2). Intergroup comparison showed that reconstructing AMCL alone had a similar contribution to elbow medial stability at all testing angles (p = 0.080, 30° flexion; p = 0.064, 60° flexion; p = 0.151, 90° flexion; p = 0.283, 120° flexion) as the intact MCL. Reconstructing MCL had the ability to maintain elbow medial stability at all angles of testing (p = 0.951, 30° flexion; p = 0.739, 60° flexion; p = 0.841, 90° flexion; p = 0.538, 120° flexion) similar to intact MCL. More importantly, a significant difference existed between the MCL reconstruction and reconstruction of the AMCL alone at 30° flexion (p = 0.043) and 60° flexion (p = 0.013), although not at 90° flexion (p = 0.369) and 120° flexion (p = 0.879). In the line chart (Figure 3), at 30° and 60° flexion, the MCL reconstruction had an advantage over the AMCL alone with respect to resisting valgus stress, implying that reconstructed MCL contributed significantly more to maintaining medial elbow stability than reconstructed AMCL alone.
FIGURE 3. Line graph showing valgus angle applied 2 Nm torque at different elbow flexion angles for intact MCL, AMCL reconstruction alone, MCL reconstruction. Error bars indicate standard deviation. *p < 0.05.
The mean maximum failure torque of MCL (including AMCL and PMCL) reconstruction at 90° flexion was 24.02 Nm (Table 3). The modes of failure were strip A tear (3/8) and strip B tear (5/8) (Table 3).
Our AMCL reconstruction alone and MCL reconstruction techniques were sufficient to maintain elbow stability. Both AMCL reconstruction alone and MCL reconstruction provided valgus stability comparable with the native MCL at 30°, 60°, 90°, and 120° of elbow flexion. Moreover, MCL reconstruction was better than AMCL reconstruction alone in maintaining valgus stability at 30° flexion and 60° flexion. The good results of the maximum failure test for the MCL reconstruction further demonstrated the excellent ability of the new MCL reconstruction technique to maintain stability.
In previous anatomical studies of AMCL, the insertion of AMCL was confirmed on the sublime tubercle of the ulnar ridge (Frangiamore et al., 2018; Farrow et al., 2011). Frangiamore et al. (2017) found that the proximal part of the insertion contributed more to stability at a higher elbow flexion angle, whereas the distal part of the insertion played a greater role at lower flexion angles. Hassan et al. (2015) suggested that the proximal half of the insertion footprint had a primary role in maintaining posteromedial stability of the elbow, whereas the distal half of the insertion tear had no significance in the biomechanical study. Considering the importance of the proximal ligament insertion, we proposed the reconstruction of AMCL with strips A and B to simulate the function of native AMCL (Figure 1G). Strip A was proximal to the distal half of the insertion and could mimic the role of the proximal half of the AMCL insertion (Figure 4). Strip B was proximal to the proximal half of the insertion, strengthening the role of the proximal half of the AMCL insertion. The Jobe technique (Mullen et al., 2002; Armstrong et al., 2005; Paletta et al., 2006) and the Docking technique (Armstrong et al., 2005; Paletta et al., 2006; Ciccotti et al., 2009) used double-strand reconstruction such that the two strands of the palmaris graft converged into a single-strand at the epicondyle. The direction of strip A was similar to the lateral strand of the two strands in the Jobe and Docking techniques. The direction of strip B was similar to but posterior to the medial strand. This posterior location was close to the functional area of AMCL. Our new AMCL reconstruction technique had the characteristics of the reconstructed ligaments in the traditional technique and was closer to the functional area than the traditional technique.
FIGURE 4. Schematic diagram shows position of reconstructed ligaments in relation to original ligaments.
Based on the role of PMCL in resisting motion of the humero-ulnar joint (Shukla et al., 2016), the new technique was also used to perform PMCL reconstruction. Baumfeld et al. (2010) found that the central and medial portions of the triceps tendon were significantly thicker and stiffer than the lateral portion. Therefore, we used the medial portion of the triceps tendon to reconstruct the PMCL. In the reconstruction of PMCL, the triceps tendon patch was posterior to native PMCL, which was 1.6 mm distal, 9.8 mm anterior to the olecranon tip, and 4.4 mm posterior to the joint line (Ciccotti et al., 2009). The position of the reconstructed PMCL was closer to the proximal end of the ulna than the original PMCL. Thus, the reconstructed PMCL might play a role in maintaining posteromedial stability.
In previous biomechanical studies (Mullen et al., 2002), the Jobe technique was effective at 30°, 60°, and 90° flexion and invalid at 120° flexion. Moreover, at 120° flexion, the strength of the reconstructed ligament was only 89% of the strength of the original ligaments. Paletta et al. (2006) discovered that the Jobe and Docking techniques did not reproduce the biomechanical profile of the native MCL. Bodendorfer et al. (2018) discovered that the Docking technique provided valgus stability to the medial elbow comparable with the native ligament only at 90° flexion. These reconstruction techniques could not truly achieve the ability of the original ligament to maintain elbow stability. Our results showed that both reconstructions of the AMCL alone and MCL achieved similar elbow stability as intact MCL. Reconstructing the MCL could provide more stability than reconstruction of the AMCL alone at 30° flexion and 60° flexion, but not at 90° flexion and 120° flexion, because in the latter two flexion angles, the gathering of muscles and docking of bony structures might limit the elbow valgus and cover up the ability of the strips. This result implied that reconstruction of MCL was an ideal technique when facing a valgus torque of more than 2.0 Nm at 30° flexion and 60° flexion.
The maximum failure torque of MCL reconstruction was 24.02 Nm at 90° flexion, where the valgus torque reached a peak in the throwing elbow (Ciccotti et al., 2009). The maximum torque of the natural ligament varied in different biomechanical studies. The maximum torque of the intact cadaver MCL was 18.8 Nm in the Palette study (Paletta et al., 2006), 22.7 Nm in the Hechtman study (Hechtman et al., 1998), 21.8 Nm in the Armstrong study (Armstrong et al., 2005), and 34 Nm in the Ahmad study (Ahmad et al., 2003). In our study, we did not test the maximum valgus failure of the native MCL. By comparison, it could be seen that our new reconstructed MCL was better than the intact ligament in several studies (Hechtman et al., 1998; Armstrong et al., 2005; Paletta et al., 2006) but worse than the intact ligament in one study (Ahmad et al., 2003). In the maximum failure experiment, Ahmad used young (mean age, 43 years) male cadavers, which might account for the large values of intact ligaments (Ahmad et al., 2003). The maximum failure torque of some previous reconstruction techniques did not reach the maximum failure level of intact ligaments. The maximum failure torque for the Jobe technique was 13.2 Nm (range, 8.0–22.7 Nm), for the Docking technique was 11.3 Nm (range, 8.2–14.3 Nm), and for the interference screw technique was 21.98 Nm (range, 13.4–30.55 Nm) (Watson et al., 2014). Many factors might contribute to differences in observations, such as the age of cadavers, bone quality, and surgical skills. In general, our new MCL reconstruction technique could achieve similar results as intact MCL; moreover, it seems better than other techniques in maximum failure experiments.
In previous techniques (Mullen et al., 2002; Armstrong et al., 2005; Paletta et al., 2006), a 3.5-mm K-wire was used to create a bone tunnel on the ulnar and medial epicondyle, and the distal bone cortex was disrupted. The bone loss led to tunnel fracture (Rohrbough et al., 2002; Paletta et al., 2006; Paletta and Wright, 2006; Schwartz et al., 2008; Cain et al., 2010) and HO (Wear et al., 2011; Kodde et al., 2012; Watson et al., 2014; Andrachuk et al., 2016; Park et al., 2018). The bone cortex in the narrow ligament function area was destroyed more in the traditional technique than in the new technique. The bone cortex in the narrow ligament function area was less destroyed in the new technique, which used a 2.5-mm K-wire without disrupting the distal bone cortex. Compared with previous techniques using tendon palmaris longus or hamstring tendons, this fan shape reconstruction technique using the fascia and tendon patches around the elbow had the potential to reduce damage and complications in the tendon supply area.
Our study has some limitations. The advanced age of the cadavers and their decreased bone density may have had a deleterious effect on the strength of anchor-bone fixation. Furthermore, the specimens' advanced age and frozen preservation could have affected the toughness and strength of the flexor carpi ulnaris fascia and triceps tendon, which may not accurately reflect the situation in young patients and achieve actual clinical outcomes. A limited sample may increase variability and lack statistical significance between the intact and reconstructed groups. Another limitation was that we did not test the tension and strength of the fascia and tendon. In addition, failure testing of MCL reconstruction cannot be repeated, which may increase variability. In future work, prospective studies are needed to analyze further the clinical value of this novel, minimally invasive reconstruction technique.
This cadaveric study proved that both AMCL reconstruction alone and MCL reconstruction provided valgus stability comparable with the native MCL, and more importantly, MCL reconstruction provided more valgus stability than AMCL reconstruction alone at 30° flexion and 60° flexion of the elbow. Therefore, the new MCL reconstruction technique might be useful to guide the treatment of elbow MCL injuries or deficiencies.
The original contributions presented in the study are included in the article/Supplementary Material, further inquiries can be directed to the corresponding authors.
The studies involving human participants were reviewed and approved by Shanghai Sixth People's Hospital East Campus. Written informed consent for participation was not required for this study in accordance with the national legislation and the institutional requirements.
CF and WL conceived the initial idea and study design. HX and SC participated in data extraction, WL, JZ, and WW participated in statistical analysis. WL and HX drafted the manuscript. WL, YQ, and CF revised the manuscript. All authors approved the final manuscript.
This work was supported by the Key Project of National Natural Science Foundation of China grant number 81830076 and the Youth Program of National Natural Science Foundation of China grant number 81802157, and clinical project of Shanghai Jiao Tong University Affiliated Sixth People's Hospital grant number (ynlc201821).
The authors declare that the research was conducted in the absence of any commercial or financial relationships that could be construed as a potential conflict of interest.
All claims expressed in this article are solely those of the authors and do not necessarily represent those of their affiliated organizations or those of the publisher, the editors, and the reviewers. Any product that may be evaluated in this article, or claim that may be made by its manufacturer, is not guaranteed or endorsed by the publisher.
The authors thank Hai Hu (Shanghai Jiao Tong University, Shanghai, China) for the contribution to guide the use of the test equipment.
The Supplementary Material for this article can be found online at: https://www.frontiersin.org/articles/10.3389/fbioe.2022.831545/full#supplementary-material
Ahmad, C. S., Lee, T. Q., and ElAttrache, N. S. (2003). Biomechanical Evaluation of a New Ulnar Collateral Ligament Reconstruction Technique with Interference Screw Fixation. Am. J. Sports Med. 31, 332–337. doi:10.1177/03635465030310030201
Anakwe, R. E., Middleton, S. D., Jenkins, P. J., McQueen, M. M., and Court-Brown, C. M. (2011). Patient-reported Outcomes after Simple Dislocation of the Elbow. J. Bone Jt. Surg Am. 93, 1220–1226. doi:10.2106/jbjs.j.00860
Andrachuk, J. S., Scillia, A. J., Aune, K. T., Andrews, J. R., Dugas, J. R., and Cain, E. L. (2016). Symptomatic Heterotopic Ossification after Ulnar Collateral Ligament Reconstruction: Clinical Significance and Treatment Outcome. Am. J. Sports Med. 44, 1324–1328. doi:10.1177/0363546515626185
Armstrong, A. D., Dunning, C. E., Ferreira, L. M., Faber, K. J., Johnson, J. A., and King, G. J. W. (2005). A Biomechanical Comparison of Four Reconstruction Techniques for the Medial Collateral Ligament-Deficient Elbow. J. Shoulder Elbow Surg. 14, 207–215. doi:10.1016/j.jse.2004.06.006
Baumfeld, J. A., van Riet, R. P., Zobitz, M. E., Eygendaal, D., An, K.-N., and Steinmann, S. P. (2010). Triceps Tendon Properties and its Potential as an Autograft. J. Shoulder Elbow Surg. 19, 697–699. doi:10.1016/j.jse.2009.12.001
Bodendorfer, B. M., Looney, A. M., Lipkin, S. L., Nolton, E. C., Li, J., Najarian, R. G., et al. (2018). Biomechanical Comparison of Ulnar Collateral Ligament Reconstruction with the Docking Technique versus Repair with Internal Bracing. Am. J. Sports Med. 46, 3495–3501. doi:10.1177/0363546518803771
Cain, E. L., Andrews, J. R., Dugas, J. R., Wilk, K. E., McMichael, C. S., Walter, J. C., et al. (2010). Outcome of Ulnar Collateral Ligament Reconstruction of the Elbow in 1281 Athletes. Am. J. Sports Med. 38, 2426–2434. doi:10.1177/0363546510378100
Ciccotti, M. G., Siegler, S., Kuri, J. A., Thinnes, J. H., and Murphy, D. J. (2009). Comparison of the Biomechanical Profile of the Intact Ulnar Collateral Ligament with the Modified Jobe and the Docking Reconstructed Elbow: an In Vitro Study. Am. J. Sports Med. 37, 974–981. doi:10.1177/0363546508331136
Dugas, J. R., Walters, B. L., Beason, D. P., Fleisig, G. S., and Chronister, J. E. (2016). Biomechanical Comparison of Ulnar Collateral Ligament Repair with Internal Bracing versus Modified Jobe Reconstruction. Am. J. Sports Med. 44, 735–741. doi:10.1177/0363546515620390
Farrow, L. D., Mahoney, A. J., Stefancin, J. J., Taljanovic, M. S., Sheppard, J. E., and Schickendantz, M. S. (2011). Quantitative Analysis of the Medial Ulnar Collateral Ligament Ulnar Footprint and its Relationship to the Ulnar Sublime Tubercle. Am. J. Sports Med. 39, 1936–1941. doi:10.1177/0363546511406220
Foruria, A. M., Augustin, S., Morrey, B. F., and Sánchez-Sotelo, J. (2013). Heterotopic Ossification after Surgery for Fractures and Fracture-Dislocations Involving the Proximal Aspect of the Radius or Ulna. J. Bone Jt. Surgery-Am. 95, e66. doi:10.2106/jbjs.k.01533
Frangiamore, S. J., Lynch, T. S., Vaughn, M. D., Soloff, L., Forney, M., Styron, J. F., et al. (2017). Magnetic Resonance Imaging Predictors of Failure in the Nonoperative Management of Ulnar Collateral Ligament Injuries in Professional Baseball Pitchers. Am. J. Sports Med. 45, 1783–1789. doi:10.1177/0363546517699832
Frangiamore, S. J., Moatshe, G., Kruckeberg, B. M., Civitarese, D. M., Muckenhirn, K. J., Chahla, J., et al. (2018). Qualitative and Quantitative Analyses of the Dynamic and Static Stabilizers of the Medial Elbow: An Anatomic Study. Am. J. Sports Med. 46, 687–694. doi:10.1177/0363546517743749
Hassan, S. E., Parks, B. G., Douoguih, W. A., and Osbahr, D. C. (2015). Effect of Distal Ulnar Collateral Ligament Tear Pattern on Contact Forces and Valgus Stability in the Posteromedial Compartment of the Elbow. Am. J. Sports Med. 43, 447–452. doi:10.1177/0363546514557239
Hechtman, K. S., Tjin-A-Tsoi, E. W., Zvijac, J. E., Uribe, J. W., and Latta, L. L. (1998). Biomechanics of a Less Invasive Procedure for Reconstruction of the Ulnar Collateral Ligament of the Elbow. Am. J. Sports Med. 26, 620–624. doi:10.1177/03635465980260050401
Kodde, I. F., Rahusen, F. T. G., and Eygendaal, D. (2012). Long-term Results after Ulnar Collateral Ligament Reconstruction of the Elbow in European Athletes with Interference Screw Technique and Triceps Fascia Autograft. J. Shoulder Elbow Surg. 21, 1656–1663. doi:10.1016/j.jse.2012.07.010
Morrey, B. F., and An, K.-N. (1983). Articular and Ligamentous Contributions to the Stability of the Elbow Joint. Am. J. Sports Med. 11, 315–319. doi:10.1177/036354658301100506
Mullen, D. J., Goradia, V. K., Parks, B. G., and Matthews, L. S. (2002). A Biomechanical Study of Stability of the Elbow to Valgus Stress before and after Reconstruction of the Medial Collateral Ligament. J. Shoulder Elbow Surg. 11, 259–264. doi:10.1067/mse.2002.122622
Paletta, G. A., and Wright, R. W. (2006). The Modified Docking Procedure for Elbow Ulnar Collateral Ligament Reconstruction. Am. J. Sports Med. 34, 1594–1598. doi:10.1177/0363546506289884
Paletta, G. A., Klepps, S. J., Difelice, G. S., Allen, T., Brodt, M. D., Burns, M. E., et al. (2006). Biomechanical Evaluation of 2 Techniques for Ulnar Collateral Ligament Reconstruction of the Elbow. Am. J. Sports Med. 34, 1599–1603. doi:10.1177/0363546506289340
Park, J.-Y., Seo, B. H., Hong, K.-H., Lee, J.-H., Oh, K.-S., Chung, S. W., et al. (2018). Prevalence and Clinical Outcomes of Heterotopic Ossification after Ulnar Collateral Ligament Reconstruction. J. Shoulder Elbow Surg. 27, 427–434. doi:10.1016/j.jse.2017.11.031
Petty, D. H., Andrews, J. R., Fleisig, G. S., and Cain, E. L. (2004). Ulnar Collateral Ligament Reconstruction in High School Baseball Players. Am. J. Sports Med. 32, 1158–1164. doi:10.1177/0363546503262166
Qian, Y., Yu, S., Shi, Y., Huang, H., and Fan, C. (2020). Risk Factors for the Occurrence and Progression of Posttraumatic Elbow Stiffness: A Case-Control Study of 688 Cases. Front. Med. 7, 604056. doi:10.3389/fmed.2020.604056
Rohrbough, J. T., Altchek, D. W., Hyman, J., Williams, R. J., and Botts, J. D. (2002). Medial Collateral Ligament Reconstruction of the Elbow Using the Docking Technique. Am. J. Sports Med. 30, 541–548. doi:10.1177/03635465020300041401
Schwartz, M. L., Thornton, D. D., Larrison, M. C., Cain, E. L., Aaron, D. G., Wilk, K. E., et al. (2008). Avulsion of the Medial Epicondyle after Ulnar Collateral Ligament Reconstruction: Imaging of a Rare Throwing Injury. Am. J. Roentgenol. 190, 595–598. doi:10.2214/AJR.07.3027
Shukla, D. R., Golan, E., Nasser, P., Culbertson, M., and Hausman, M. (2016). Importance of the Posterior Bundle of the Medial Ulnar Collateral Ligament. J. Shoulder Elbow Surg. 25, 1868–1873. doi:10.1016/j.jse.2016.04.008
Shukla, D. R., Golan, E., Weiser, M. C., Nasser, P., Choueka, J., and Hausman, M. (2018). The Posterior Bundle's Effect on Posteromedial Elbow Instability after a Transverse Coronoid Fracture: A Biomechanical Study. J. Hand Surg. 43, 381.e1–381.e8. doi:10.1016/j.jhsa.2017.09.018-
Vitale, M. A., and Ahmad, C. S. (2008). The Outcome of Elbow Ulnar Collateral Ligament Reconstruction in Overhead Athletes: A Systematic Review. Am. J. Sports Med. 36, 1193–1205. doi:10.1177/0363546508319053
Watson, J. N., McQueen, P., and Hutchinson, M. R. (2014). A Systematic Review of Ulnar Collateral Ligament Reconstruction Techniques. Am. J. Sports Med. 42, 2510–2516. doi:10.1177/0363546513509051
Wear, S. A., Thornton, D. D., Schwartz, M. L., Weissmann, R. C., Cain, E. L., and Andrews, J. R. (2011). MRI of the Reconstructed Ulnar Collateral Ligament. Am. J. Roentgenol. 197, 1198–1204. doi:10.2214/AJR.11.6461
Wu, G., van der Helm, F. C. T., Veeger, H. E. J., Makhsous, M., Van Roy, P., Anglin, C., et al. (2005). ISB Recommendation on Definitions of Joint Coordinate Systems of Various Joints for the Reporting of Human Joint Motion-Part II: Shoulder, Elbow, Wrist and Hand. J. Biomech. 38, 981–992. doi:10.1016/j.jbiomech.2004.05.042
Keywords: elbow, MCL reconstruction, biomechanics, elbow valgus stability, fascia patch, tendon patch
Citation: Liu W, Xiong H, Chen S, Zhang J, Wang W, Qian Y and Fan C (2022) Biomechanical Evaluation of a Low-Invasive Elbow Medial Collateral Ligament Reconstruction Technique With Fascia and Tendon Patches. Front. Bioeng. Biotechnol. 10:831545. doi: 10.3389/fbioe.2022.831545
Received: 21 December 2021; Accepted: 18 February 2022;
Published: 22 March 2022.
Edited by:
Cristina Bignardi, Polytechnic University of Turin, ItalyReviewed by:
Laura Gastaldi, Politecnico di Torino, ItalyCopyright © 2022 Liu, Xiong, Chen, Zhang, Wang, Qian and Fan. This is an open-access article distributed under the terms of the Creative Commons Attribution License (CC BY). The use, distribution or reproduction in other forums is permitted, provided the original author(s) and the copyright owner(s) are credited and that the original publication in this journal is cited, in accordance with accepted academic practice. No use, distribution or reproduction is permitted which does not comply with these terms.
*Correspondence: Wei Wang, d2FoYWExOTg3QDE2My5jb20=; Yun Qian, bG9sbGlwb3BjbG91ZGxhbmRAZm94bWFpbC5jb20=; Cunyi Fan, Y3lmYW5Ac2p0dS5lZHUuY24=
†These authors have contributed equally to this work
Disclaimer: All claims expressed in this article are solely those of the authors and do not necessarily represent those of their affiliated organizations, or those of the publisher, the editors and the reviewers. Any product that may be evaluated in this article or claim that may be made by its manufacturer is not guaranteed or endorsed by the publisher.
Research integrity at Frontiers
Learn more about the work of our research integrity team to safeguard the quality of each article we publish.