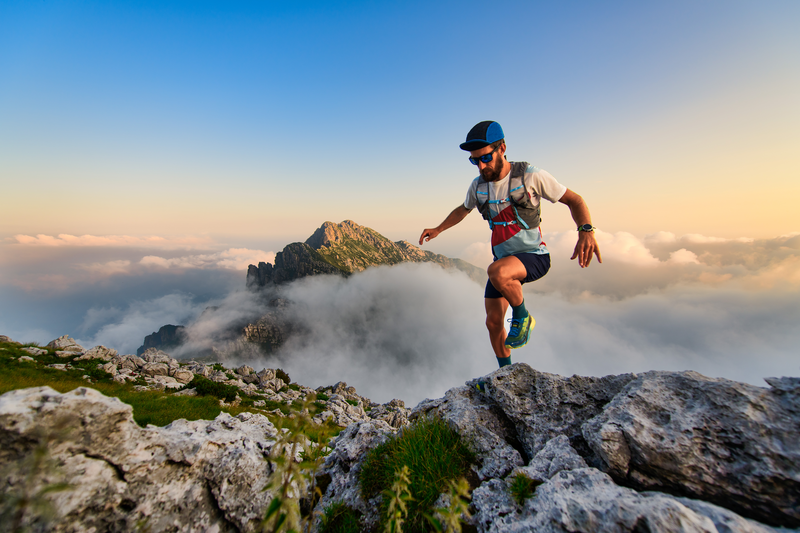
94% of researchers rate our articles as excellent or good
Learn more about the work of our research integrity team to safeguard the quality of each article we publish.
Find out more
REVIEW article
Front. Bioeng. Biotechnol. , 02 March 2022
Sec. Cell and Gene Therapy
Volume 10 - 2022 | https://doi.org/10.3389/fbioe.2022.823945
Intervertebral disc degeneration (IVDD) is the main cause of cervical and lumbar spondylosis. Over the past few years, the relevance between cellular senescence and IVDD has been widely studied, and the senescence-associated secretory phenotype (SASP) produced by senescent cells is found to remodel extracellular matrix (ECM) metabolism and destruct homeostasis. Elimination of senescent cells by senolytics and suppression of SASP production by senomorphics/senostatics are effective strategies to alleviate degenerative diseases including IVDD. Here, we review the involvement of senescence in the process of IVDD; we also discuss the potential of senolytics on eliminating senescent disc cells and alleviating IVDD; finally, we provide a table listing senolytic drugs and small molecules, aiming to propose potential drugs for IVDD therapy in the future.
Intervertebral disc degeneration (IVDD) refers to an age-related change that mainly occurs in the lumbar intervertebral disc and often precedes other age-related changes (Adams and Roughley, 2006; Vergroesen et al., 2015). During the process of IVDD, annulus fibrosis (AF), one of the important compositions of intervertebral disc, loses its original layer and toughness, and reticulated degeneration and hyalinization appear, while the percentage of water decreases in another component called nucleus pulposus (NP). As a result, the intervertebral disc loses its normal elasticity and tension (Roberts et al., 2006). Severe trauma or repeated inconspicuous damage may cause the annulus fibrosis to become weak or even rupture. There are two results depending on the severity of damage to AF. If the annulus fibrosus is partially teared, the nucleus pulposus will protrude from the weak or ruptured area, compressing the nerve root and producing signs of nerve root damage; however, if the annulus fibrosus is completely ruptured, the broken nucleus pulposus tissue is likely to prolapse from the disc and enter into the spinal canal, which will cause irreversible damage to spinal cord (Roberts et al., 2006; Carrino et al., 2009).
IVDD is considered to be one of the main causes of low back pain and lumbar herniated disc (Brinjikji et al., 2015). As the life expectancy of the global population rises slowly, the incidence of IVDD will become increasingly high, thus resulting in a high economic burden (Smith et al., 2011). Although the situation is severe, the molecular mechanism of IVDD has not yet been fully clarified yet. At present, there are two main treatment strategies for IVDD: surgery and drug therapy. However, the main effects of existing drugs are inhibition of inflammation and post-onset analgesia, which cannot effectively prevent intervertebral disc from degenerating in the early stage (Wu et al., 2020).
Aging is the primary risk factor for the development of IVDD, which causes the accumulation of senescent cells in the intervertebral disc (Wang et al., 2016). Researchers have found that senescent NP cells play an important role in the initiation of IVDD (Zhang Y. et al., 2020). The number of senescent NP cells increased significantly during IVDD (Sakai et al., 2012; Dudek et al., 2017), suggesting the deleterious effect of senescent NP cells on the pathogenesis of IVDD. Recent studies have shown that senescent cells could secrete metabolic factors such as pro-inflammatory cytokines, matrix-degrading proteases, growth factors, and chemokines, which caused changes of the extracellular matrix (ECM) (Gruber et al., 2011; Zhao et al., 2011; Bedore et al., 2014). In addition, senescent cells can affect adjacent cells through paracrine, thereby inducing the catabolism and inflammation in the microenvironment of intervertebral disc. The metabolic factors secreted by senescent cells are collectively named senescence-associated secretory phenotype (SASP) (Ngo et al., 2017). In recent years, investigations on new drugs that target the process of senescence have become a new therapeutic strategy for the early prevention and latter treatment for degenerative diseases. Evidence suggest that whether through genetically modified strategy (Baker et al., 2011; Liu et al., 2019) or chemotherapy (Zhu et al., 2015; Amor et al., 2020), the elimination of p16INK4a senescent cells has been shown to significantly extend the healthspan in murine. So, optimizing treatments to reduce senescence or eliminate senescent cells may exert positive effects on human health.
Senolytic represents a wide range of drugs or small molecules that can selectively eliminate senescent cells. Zhu et al. (2015) demonstrated a significant effect of senolytic on aging-related diseases in mice for the first time in 2015. Since then, senolytic has been investigated in age-related diseases extensively. Its characteristic of selectively inducing apoptosis of senescent cells has been validated in osteoporosis, primary biliary cholangitis, atherosclerosis, and chronic lung diseases, respectively (Childs et al., 2016; Farr et al., 2017; Barnes et al., 2019; Sasaki et al., 2020). Therefore, the application of senolytic drugs is a potential strategy for degenerative disease treatment, including IVDD.
Cellular senescence is usually defined as the process of gradual decline in cell proliferation and differentiation as well as physiological dysfunctions during life activities of cells (van Deursen, 2014). The dynamic balance of cell senescence, death, and cell proliferation is fundamental for normal metabolic activities (Calcinotto et al., 2019). Currently, it is widely believed that cellular senescence can be divided into two categories, replication senescence (RS) and stress-induced senescence (SIPS) (Muñoz-Espín and Serrano, 2014). The signaling pathways involved in these two types of senescence overlap with each other a lot, which makes it difficult to distinguish unless external predisposing factors are clarified (Herranz and Gil, 2018).
The mechanism of age-related replication senescence has been studied for decades, and a number of hypotheses have been proposed (Victorelli and Passos, 2017; Schmeer et al., 2019). Among them, the theory of aging circadian clock generally accepted by scientists claims that shortened chromosomal telomeres caused by DNA duplication during mitosis lead to senescence (Dudek et al., 2017; Hood and Amir, 2017; Sato et al., 2017). The reactive oxygen species (ROS) theory describes that the accumulation of ROS in cells will cause organelle dysfunction (Finkel and Holbrook, 2000; Victorelli and Passos, 2017; Wang R. et al., 2018). And, the theory of DNA damage accumulation is also used to explain the mechanism of RS (Lieber and Karanjawala, 2004; Chen et al., 2007). As for SIPS, which refers to cell senescence induced by external stimuli, it manifests as the senescence of local tissues or cells instead of the whole organism. Accordingly, SIPS is considered to be the main cause of age-related degenerative diseases (de Magalhães and Passos, 2018).
Non-random damage plays an important role in the initiation and development of senescence (Gladyshev, 2013). ROS, DNA lesions, and mitochondrial dysfunction caused by external stimulation have been shown to be the dominant mechanisms of SIPS (Passos et al., 2007). As one of the common causes leading to SIPS, DNA damage induced by external stressors mainly activates DNA damage response (DDR) signaling pathway-related kinase (ATM, ATR), which further activates the downstream p53-p21-retinoblastoma protein (Rb) pathway and thereby inhibits cell cycle (Gladyshev, 2013; Ou and Schumacher, 2018). Extensive mechanical tension represents a common external stressor, and the senescence of NP and AF cells causes a typical feature in degenerative intervertebral disc (Pang et al., 2017; Zhao et al., 2019). Additionally, due to the absence of blood vessels and nerves in the intervertebral disc, it is more susceptible to be damaged by oxidative stress, lower pH, hypertonic extracellular environment, and hypoglycemia (Huang et al., 2014; Che et al., 2020). Oxidative stress and hypertonic extracellular environment have been shown to promote NP cell senescence in vitro (Xu et al., 2019; Che et al., 2020).
When external stressors cause DNA damage, the DDR signaling pathway and p16Ink4a are both continuously activated (Rodier et al., 2009; Wang et al., 2013; Horikawa, 2020). Moreover, mitochondrial dysfunction and ROS production are also regulated through p38-MAPK pathway. They can then lead to cell cycle arrest, also known as cell senescence (Passos et al., 2007; Borodkina et al., 2014). Recent studies have shown that the inhibition of DDR-related kinase could get senescent cells back on the track of replication cycle instead of cycle arrest (Menolfi and Zha, 2020; Zhao et al., 2020). The significance of DDR is to restrict the damaged DNA to stimulated cell by inhibiting cell proliferation, which attenuates the accumulation of abnormal daughter cells with damaged DNA in the tissue (Lans et al., 2019).
Oxidative stress (OS) is a negative process with the production of ROS, which is a key factor leading to senescence and extensive cellular dysfunction (Junqueira et al., 2004; Hernandez et al., 2015). Hypoxia is the main cause of ROS production (Giordano, 2005). Increasing ROS in cells is closely related to DNA damage, and DDR-related p53-p21-Rb pathway is subsequently activated (Passos et al., 2007). The role of the p16-Rb pathway has also been validated in ROS-induced senescence (Rayesse et al., 2012).
Another important factor of SIPS is mitochondrial dysfunction-induced senescence, whose underlying mechanism is closely related to ROS (Bhatti et al., 2017). On the one hand, mitochondria, as the center of aerobic respiration, are manufacturers of ROS through the loss of the respiratory chain, the decrease of mitochondrial membrane potential, and electronic leak due to opening of mitochondrial permeability transition pores (Sas et al., 2018). On the other hand, mitochondria are the main targets of ROS in turn (Bhatti et al., 2017). When mitochondria are attacked by ROS, mutations of its DNA (mtDNA) increase and respiratory enzymes are damaged, subsequently leading to severe mitochondrial dysfunction (Bhatti et al., 2017; Stokman et al., 2017). Consequently, ROS and mitochondrial dysfunction form a vicious cycle of mutual deterioration (Passos et al., 2007). Furthermore, Wiley et al. (2016) have found that mitochondrial dysfunction-related senescence activated by AMPK and p53 axis produces a completely different SASP compared to that of the IL-1-dependent group, providing new evidence to explain the senescence induced by mitochondrial damage. This result elucidates that mitochondrial dysfunction has multiple effects on cell senescence, while the underlying mechanism needs to be further clarified. Besides hypoxia induced by DNA damage, ROS can also be generated by oxidative stress connected to inflammation and high glucose (McGarry et al., 2018; Fouda et al., 2020).
From the studies above, we conclude that the p53-p21-Rb pathway or/and the p16INK4a-Rb pathway play a key role in SIPS. Although these two pathways prevent cells from entering the S phase by inhibiting the phosphorylation of Rb through different cyclin-dependent kinases (CDKs), the cells finally manifest a similar senescence phenotype (Panossian et al., 2021). The difference between two pathways is that p21 is an inhibitor of CDK2 (Morisaki et al., 1999), while p16INK4a works as the inhibitor of CDK 4/6 (Tao et al., 2017). Moreover, the tumor suppressor p19Arf, which could activate p53, plays a role in replicating DNA damage instead of acute damage (Lim et al., 2021). Although p53 is essential to the initiation of senescence (Horikawa, 2020), studies have found that p16INK4a is the main factor maintaining senescence in some cells (Baker et al., 2011). Studies on intervertebral disc have only found increased expression of p16INK4a with increasing age, while the levels of p53 and p19Arf did not manifest a significant discrepancy, which emphasized the importance of p16INK4a as a key marker for maintaining age-dependent senescence in the intervertebral disc of mice (Novais et al., 2019).
In terms of transcriptome, miR-623 has been shown to prevent LPS-induced senescence of intervertebral disc cells through CXCL12 (Zhong et al., 2021).
Currently, SIPS is widely recognized as a typical stress response when suffering from external stimuli, which is involved in the repair of damaged tissue and inhibition of abnormal proliferation (Sikora et al., 2011; Dominic et al., 2020). With risk factors affecting the organization continuously, senescent cells will accumulate and bring about unexpected dysfunctions subsequently (de Magalhães and Passos, 2018). Studies have also found that senescent cells play an irreplaceable role in degenerative diseases such as IVDD through triggering extensive inflammatory responses by secreting SASP (Ngo et al., 2017).
As we have mentioned in the Introduction, aging is the most important factor that promotes the initiation and development of IVDD. Besides apoptosis, NP cells affect adjacent tissues in an aging-related way and aggravate the dysfunction of the intervertebral disc with our body becoming old generally (Wang et al., 2016). Among them, senescent cells are involved in the etiology of several age-related dysfunction, including Alzheimer’s disease (Lyons and Bartolomucci, 2020), obstructive pulmonary disease (Barnes et al., 2019), atherosclerosis (Stojanović et al., 2020), and osteoporosis (Khosla, 2013). Studies have found that the percentage of senescent cells experienced a significant increase in the nucleus pulposus during the process of IVDD (Zhang Y. et al., 2020). Senescent cells exhibit three main characteristics in vivo, including reduced replication, expression of SASP, and p16INK4a activation (Li et al., 2021). As the reduction of senescent cell replication has been elaborated in detail in the Cellular Senescence section, we will review the two remaining features in the following section (Figure 1).
FIGURE 1. Cellular senescence and IVDD. (A) Age-related senescence often leads to organ dysfunction and structure failure, while in the spine, it mainly manifested as IVDD. The senescence of NP cells and fibroblasts in the annulus fibrosus cells is the main cause of IVDD. Under the exposure of inappropriate mechanical stress, the degenerated intervertebral disc may lose its stability as a consequence of the annulus fibrosus enlargement or even rupture and the position changes of the nucleus pulposus, resulting in posterior disc protrusion and subsequent compressive myelopathy. (B) Senescence of NP cells is considered to be the main cause of IVDD. Various external and internal stressors can activate the aging-related signal cascade by changing the function of mitochondria, inducing ROS generation or DNA damage. These three initiation modes of senescence are interrelated to each other, forming a complex cellular senescence regulation network. In the downstream cascade, p16-CDK4/6-Rb and p53-p21-CDK2-Rb are recognized to block the G1/S phase of the cell cycle, while p53-p21-cdc2 blocks the S/G2 phase. (C) SASP secreted by senescent NP cells will result in structural degradation of the intervertebral disc. SASP in nucleus pulposus is mainly composed by two categories of elements, inflammatory factors (IL-1, IL-6, and IL-8) and ECM proteases (MMP-13, ADAMTS4, and ADAMTS5). For inflammatory factors, they participate in the local inflammatory response and induce the senescence phenotype of adjacent cells. As for ECM proteases, they mainly degrade the components of the extracellular matrix, aggrecan (ADAMT4 and ADAMT5), and collagen II (MMP13), to threaten the homeostasis of extracellular structure.
ECM degradation and the initiation of inflammation are the two major processes of IVDD (Yang S. et al., 2020). ECM, mostly composed of aggrecan and collagen type II (collagen II), provides a foster ground for the metabolism of intervertebral disc cells (Zhang Y. et al., 2020). However, with the body getting older, the synthesis of aggrecan and collagen II decreases year by year. These degenerative changes could alter the microenvironment of the NP cells, leading to changes of intervertebral disc structure and subsequently weakened load capacity (Smith et al., 2011). In addition, researchers have found that the ability of senescent NP cell to stabilize ECM declines with age (Vo et al., 2016). When subjected to the stimulation of external ROS, aggrecan and collagen Ⅱ are degraded as a consequence of increased expression of matrix metalloproteinase family (MMPs) and A Disintegrin And Metalloproteinase with Thrombo Spondin 1 repeats (ADAMTSs), which is considered to be the main microscopic pathological change of IVDD. (Bedore et al., 2014).
Inflammatory factors (IL-1, IL-6, IL-8, and TNF-α) are key cytokines involved in aging and age-related diseases, whose expression levels are related to the severity of degeneration to a certain extent (Krupkova et al., 2018; Zhang Y. et al., 2019; Kim et al., 2021). IL-1 has already been shown to be involved in the pathogenesis of intervertebral disc degeneration (Phillips et al., 2013). But, the research of Gorth et al. (2019) suggests that there is also an exception in IVDD. After knocking out gene IL-1 in mice, they found IVDD was remarkably exacerbated compared to the control group, which suggests the opposite effect of IL-1 in intervertebral disc. Therefore, it is worth noting that the function of inflammatory factors clarified in other degenerative diseases cannot be simply applied to IVDD. In the past few years, it is widely believed that senescent cells can play multiple roles, including but not limited to secreting metabolic factors, recruiting inflammatory cells, causing instability of extracellular microenvironment, and inducing the senescence of neighboring cells through paracrine. In this way, senescent cells could lead to the catabolism of the intervertebral disc matrix and activated inflammation, which are two main mechanisms of IVDD (McHugh, 2020; Yang S. et al., 2020).
The metabolic factors secreted by senescent cells are called SASP, which can be divided into proinflammatory factors (IL-6, IL-7, IL-8, and TNF-α), ECM proteases (MMPs and ADAMTS), growth factors, cytokines, and other biologically active substances (Faget et al., 2019; Basisty et al., 2020). ATM/ATR activated by DDR is considered to be an important target for initiating SASP synthesis (Victorelli and Passos, 2017; Menolfi and Zha, 2020), among which the silencing of ATM genes can remarkably inhibit the secretion of IL-6 (Rodier et al., 2009). The theory has also been validated in IVDD, and inhibition of ATM alleviated the symptom significantly with reducing the expression of IL-6 in SASP (Zhao et al., 2020). In addition, tumor suppressor genes have been confirmed to play a role in the initiation of cellular senescence, especially for the secretion of SASP. In astrocytes, p53 regulated the expression of a variety of SASP factors (IL-1β, IL-6, and IL-8) (Turnquist et al., 2016), while another tumor suppressor gene Rb promoted the activation of the SASP (IL-6) in the mouse osteosarcoma model (Li and Zhang, 2017). Moreover, mammalian rapamycin (mTOR) (Herranz et al., 2015), p38MAPK/MAPK-activated protein kinase 2 (MK2) (Freund et al., 2011), nuclear factor kappa-B (NF-κB) (Chien et al., 2011; Zhao et al., 2020), interferon gene cyclic GMP-AMP synthetase/stimulator (cGAS/STING), and CCAAT/enhancer binding protein β (C/EBPβ) have been confirmed to regulate the secretion of SASP (Huggins et al., 2013; Lim et al., 2020; Aguado et al., 2021).
The secretion of SASP causes a variety of extracellular reactions, in which inflammation is the most important one. Accordingly, the interaction between the immune system and cellular senescence provides a new direction for the diagnosis and treatment of age-related diseases. A contradiction is that SASP could specifically eliminate senescent tumor cells by recruiting immune cells (NK cells) (Chien et al., 2011), meanwhile SASP could also cause the “senescence cascade” as more cell senescence is induced by endocytosis of pro-inflammatory cytokines (Faget et al., 2019).
In IVDD, how SASP plays its role has attracted widespread attention currently. It has been determined that the expression level of multiple pro-inflammatory cytokines (IL-1, IL-6, and IL-8) and ECM proteases (MMP-13, ADAMTS4, and ADAMTS5) increased in senescent intervertebral disc (Ngo et al., 2017). When aging-related degeneration occurs, ECM is degraded by elevated ECM proteases (MMPs and ADAMTS), which is an important mechanism for the loss of structural stability of the intervertebral disc (Bedore et al., 2014).
At present, some drugs called senomorphics are found to improve the extracellular microenvironment by reducing the production of SASP without eliminating senescent cells (distinguish from senolytics) (Di Micco et al., 2021). In some literature, they are also called senostatics (Kang, 2019). Our previous result suggested that the senomorphic drug metformin and polydatin could alleviate IVDD by inhibiting the senescence and apoptosis of NP cells in intervertebral disc (Chen et al., 2016; Wang J. et al., 2018), and the inhibitory effect of metformin on SASP (IL-6, IL-1β, MMP3, and MMP13) was recently verified in the study of Han et al. (2019).
However, the composition of SASP varies from cell to cell (Basisty et al., 2020), and even for the same cell, the component of SASP it produces is partially different depending on the type of stimuli it receives and for which it enters senescence (Özcan et al., 2016). On this condition, components of SASP secreted by senescent NP cells are still not fully understood. On the other hand, as we mentioned before, SASP can recruit immune cells for self-elimination (Birch and Gil, 2020; Jin et al., 2021), while it is still unclear whether the mechanism exists in the intervertebral disc. So, further implementation of senomorphic drugs in IVDD is limited, although the deteriorating effect of SASP in IVDD has already been clarified (Zhao et al., 2011; Ngo et al., 2017). Analogically, the application of senolytic drugs that aim to remove senescent cells and reduce SASP secretion in intervertebral disc requires further research.
Since the accumulation of senescent cells is the main cause of age-related degenerative diseases, it is widely believed that removing senescent cells and reducing the production of SASP can not only alleviate the aging-related dysfunction but also improve age-related diseases or related phenotypes (Baker et al., 2011; Zhu et al., 2015). For example, removing senescent astrocytes and microglia has been shown to improve tau-dependent neurodegenerative diseases (Zhang P. et al., 2019), while eliminating senescent osteocytes can also delay osteoporosis caused by aging effectively (Farr et al., 2017). Similar effects have also been observed in chronic lung diseases (Barnes et al., 2019) and atherosclerosis (Childs et al., 2016) after senescent cells being eliminated. In the phase of pre-clinical research, selective elimination of transgenic marker p16INK4a and chemical pathways are two effective strategies to remove senescent cells (Baker et al., 2011; van Deursen, 2019).
p16INK4a, known as a cell cycle suppressor, can inhibit cyclin-dependent kinases 4 and 6 (CDK4 and CDK6), causing stagnation of senescent cells at G1 phase (Kim et al., 2021). The expression level of p16INK4a increases in senescent cells; thus, p16INK4a is always used as one of the commonly senescent cell markers (others: SA-β-gal, ki67) (Krupkova et al., 2018; Ogrodnik, 2021). In recent years, more and more studies have proved that p16INK4a is essential for inducing and maintaining senescence in intervertebral discs, and its conditional loss plays an important role in reducing cell apoptosis, restricting SASP, and changing matrix homeostasis of intervertebral disc cells (Liu et al., 2019; Novais et al., 2019; Patil et al., 2019; Che et al., 2020). After inserting a strain (tdTOM) into the p16 promoter, Liu et al. (2019) have found that p16tdTOM-positive cells accumulate accompanying aging and inflammation, among which mouse macrophages exhibited a senescent phenotype, and SASP-related expression was significantly elevated. Also, Che et al. (2020) detected the decrease of pro-inflammatory cytokine IL-1β and IL-6 after knocking out p16INK4a. Although a decreased expression in MMP13 was not detected, the inhibited degradation of collagen II in the intervertebral disc indicated that knocking out p16INK4a can prevent intervertebral disc from inflammatory response and matrix degradation through SASP. Interestingly, in the study by Novais et al. (2019), although the secretion of SASP was reduced and ECM homeostasis was improved in p16INK4a KO mice, there was no significant difference in the reduction of senescent cells in the intervertebral disc and the improvement of IVDD process compared with the control group. Instead, the increase of p19Arf and Rb expression suggests that perhaps some bypasses are activated when p16INK4a is silenced, maintaining the senescent phenotype. This result suggests that inhibiting the expression of p16INK4a merely cannot alleviate IVDD effectively.
Alternatively, the elimination of senescent cells has been proved to decrease the paracrine effect of SASP on neighboring cells (Ngo et al., 2017), which exhibits few obvious side effects simultaneously (Baker et al., 2011). Furthermore, after the elimination of transgenic p16INK4a (p16-3MR) senescent NP cells by target reagents, Patil et al. (2019) have detected the extensive reduction of MMP13 expression and ECM degradation in the intervertebral disc. Although the expression of IL-6 and ADAMTS4 exerts no distinction compared with negative control, which suggests that IL-6 and ADAMTS4 may not be the SASP components that cause the development of IVDD, the targeted elimination of senescent cells significantly improves the histological characteristics of the intervertebral disc compared with the knockout of p16INK4a. The results suggest that the elimination of senescent NP cells with a high expression of p16INK4a can delay the aging process and reduce the degeneration of intervertebral discs.
A senolytic is a kind of small molecules with the feature of clearing senescent cells selectively (van Deursen, 2019). After dasatinib and quercetin were first reported as a senolytic combination in 2015, it was discovered that the indiscriminate removal of senescent cells through senolytic can effectively inhibit the degradation of proteoglycans in the intervertebral disc (Zhu et al., 2015). Dozens of compounds with senolytic activity have been distinguished so far, which are mainly divided into natural extracts with their derivatives and synthetic chemical molecules (Partridge et al., 2020; Lagoumtzi and Chondrogianni, 2021).
As for natural extracts, compounds with senolytic features mainly include quercetin, fisetin, curcumin, and its derivative o-Vanillin (Di Micco et al., 2021). The latest study has found that the grape seed extract PCC1 also has senolytic properties, which may be stronger than existing compounds (Xu Q. et al., 2021). Among them, curcumin and its metabolite o-Vanillin have been proven to eliminate senescent cells in the intervertebral disc, reducing SASP secretion and alleviating IVDD (Cherif et al., 2019; Mannarino et al., 2021). Our previous study has elucidated that quercetin could significantly reduce the proportion of senescent cells in the intervertebral disc through the Nrf-2/NF-κB pathway and slow down the deterioration of IVDD (Shao et al., 2021). The antiaging effects of fisetin and PCC1 in the intervertebral disc reserve to be proven.
For synthetic chemical molecules, the effects of FDA-approved reagent RG7112 in eliminating senescent cells were validated in the intervertebral disc by targeting MDM2 (Cherif et al., 2020). From the preclinical studies above, we have seen the feasibility and great potential of senolytic therapy in IVDD. Compared with transgenic therapies, senolytic drugs have fewer side effects and clearer mechanisms. Also, some of them are clinical drugs that have been approved by the FDA, which have better prospects in application (Nogueira-Recalde et al., 2019; Sargiacomo et al., 2020). However, considering the beneficial effects of senescent cells in some diseases such as tissue repairing (Xu et al., 2018; Lagoumtzi and Chondrogianni, 2021), the systemic application of senolytic drugs needs a more in-depth evaluation.
In recent years, an increasing number of studies have shown that the application of transgenes or chemical reagents in eliminating senescent cells can protect organisms from aging and some age-related degenerative diseases and prolong the healthspan (Baker et al., 2011; van Deursen, 2019). As a type of aging treatment strategy, senolytics can selectively clear senescent cells by inducing apoptosis and reduce the production of SASP, contributing to the alleviation of aging-related diseases and improvement of metabolic function (Xu et al., 2018; Lagoumtzi and Chondrogianni, 2021). The naturally extracted senolytic has a complicated mechanism where multiple intracellular targets are involved (Ge et al., 2021). For synthetic senolytics, there are four types of potential targets that have been identified in senescent cells, including BCL family (Yosef et al., 2016), HSP90 inhibitor (Fuhrmann-Stroissnigg et al., 2017), PI3K/AKT (Wagner and Gil, 2020), and others (Hubackova et al., 2019; He et al., 2020a; Zhang et al., 2021). Here, we will discuss the underlying mechanisms of these senolytic target families on cell senescence as well as their role in the process of IVDD (Figure 2).
FIGURE 2. Potential intracellular targets of senolytics. According to the diverse intracellular targets of different senolytics, they are divided into certain groups. As one of the principal pathways targeted by senolytics, PI3K/Akt can be suppressed by dasatinib through receptor tyrosine kinase (RTK). The natural extracts quercetin and fisetin are proven to inhibit the phosphorylation of Akt and then induce senescent cell apoptosis and SASP-secret inhibition through FoxO4 and mTOR, respectively. Besides quercetin, curcumin with its derivative o-Vanillin and fisetin exert their senolytic effects by inhibiting NF-κB. HSP90 inhibitors have also been shown to induce apoptosis by indirectly inhibiting Akt. The FDA-approved cardiac glycoside drugs digoxin, digitoxin, and ouabain can mediate the elimination of senescent cells by inhibiting Na+/K+ ATPase and anti-apoptotic protein Bcl-2 simultaneously. In addition, senolytic properties of inhibitors on anti-apoptotic proteins (Bcl-2, Bcl-w, and Bcl-xL) of Bcl-2 family and transcription factor FoxO4 have also been demonstrated.
PI3K (phosphatidylinositol kinase) is a dimer that is composed of regulatory subunit p85 and catalytic subunit p110 (Cully et al., 2006). When binding to growth factors, cytokines, or hormone receptors, PI3K can change the structure of Akt and make it phosphorylated (p-AKT). p-AKT is the activated state of Akt that could regulate FoxO, mTOR, and other signal proteins in its downstream (Yang et al., 2019). PI3K/Akt is a classic pathway that plays an important regulatory role in cell proliferation, apoptosis, and autophagy (Koyasu, 2003; Cully et al., 2006; Greenhill, 2019; Zhou et al., 2020). Meanwhile, an increasing number of evidence show that the activation of the PI3K/AKT pathway is also involved in stabilizing senescent cells (Zhu et al., 2015; Mendez-Pertuz et al., 2017; Wagner and Gil, 2020).
Until now, two types of downstream targets have been figured out in inhibiting the apoptosis of senescent cells through PI3K/Akt.
The first is the pro-apoptotic signaling pathways mainly suppressing Bax, Bad, and FoxO (Stitt et al., 2004; She et al., 2005; Rahmani et al., 2018). Studies have found that after blocking the binding of FoxO4 and p53, FoxO4-DRI can induce the apoptosis of aging Leydig cells in testicles effectively (Zhang C. et al., 2020), while the role of Bax and Bad inhibitors in inducing apoptosis of senescent cells has not been reported yet.
mTOR, together with NF-κB signaling pathways, represents another kind of targets regulated by PI3K/Akt (Dutta Gupta and Pan, 2020). As an important regulatory protein in aging, autophagy, and apoptosis, mTOR plays a key role in cell senescence and autophagy (Nam et al., 2013; Kim and Guan, 2015). mTORC1 is widely involved in cell metabolisms whose suppression will lead to the surging autophagy and clearance of damaged proteins and organelles (such as mitochondria) (Weichhart, 2018), which could also reduce the accumulation of SASP and alleviate aging (Weichhart, 2018). The application of rapamycin, inhibitor of mTORC1, has been shown to extend the lifespan of model organisms, although the latest clinical trial results do not support its anti-aging effect in healthy older adults (Kraig et al., 2018). Accordingly, whether mTORC1 inhibitors can alleviate human aging needs further discussion. As in intervertebral disc, rapamycin can exert a variety of effects through mTORC1, including inhibiting the senescence of annulus fibrosus cell (Gao et al., 2018), promoting autophagy of end plate chondrocytes (Zhang C. et al., 2020), and protecting NP cell from apoptosis and senescence (Kang et al., 2019). Hence, the process of IVDD was delayed (Kakiuchi et al., 2019). Due to its properties in reducing SASP secretion, rapamycin is classified as senomorphic currently (Xu C. et al., 2021).
NF-κB is another important molecule regulated by PI3K/Akt (Hoesel and Schmid, 2013). We have validated the role of NF-κB in reducing the production of SASP and elimination of senescent NP cell under the treatment of quercetin (Shao et al., 2021). Furthermore, quercetin has also been shown to inhibit the upstream of PI3K/Akt pathway through certain mechanisms, suppressing the proliferation of tumor cells and inducing the apoptosis of senescent cells (Kim et al., 2019; Lan et al., 2019). In addition, another natural senolytic drug fisetin is also proven to promote apoptosis of tumor and senescent cell by inhibiting PI3K/Akt and mTOR (Yousefzadeh et al., 2018). Therefore, PI3K/Akt is considered to be an essential target of natural senolytic extracts as well as the potential signaling pathway for IVDD.
The B-cell lymphoma 2 (BCL-2) family is a special protein family that shares one or more BCL-2 homology (BH) domains. Most of BCL-2 are located on the outer mitochondrial membrane or transferred to outside of the mitochondria under certain stimuli (Adams and Cory, 1998). The family plays a decisive role in the intracellular apoptosis by regulating the permeability of the outer mitochondrial membrane (Korsmeyer, 1999).
At present, 25 proteins have already been recognized as Bcl-2 family homologue (Kale et al., 2018). According to different responses to apoptotic stimulus, they can be divided into two groups: Bcl-2, Bcl-xL, Bcl-w, MCL-1, and A1 who inhibit cell apoptosis and Bax, Bak, Bad, Noxa, etc. that promote cell apoptosis (Adams and Cory, 1998). Bcl-2 can prevent the release of cytochrome c from mitochondria to the cytoplasm, thereby inhibiting endogenous cell apoptosis (Chipuk et al., 2004; Sun et al., 2019). Besides, Bcl-2 also plays an important role in the survival of senescent cells (Chong et al., 2020). Studies have found that the expression of anti-apoptotic proteins Bcl-2, Bcl-xL, and Bcl-w increased, which enhances the cells’ ability of drug resistance through cell senescence by anti-apoptotic pathway and hence resisting external stimuli and maintaining tissue integrity (Yosef et al., 2016). This evidence indicates that anti-apoptotic proteins in the BCL-2 family have become potential targets for senolytic drugs.
Since inhibiting the expression of anti-apoptotic proteins such as BCL-2, BCL-xL, and BCL-w displays a positive effect on tumor cell apoptosis, a series of anti-cancer reagents targeting BCL-2 have been developed in recent years (Delbridge et al., 2016). For senescence, the senolytic properties of some BCL-2-targeting molecules [such as ABT263 (navitoclax), ABT737, and A-1331852] have been verified in a few systems (Chong et al., 2020; Sasaki et al., 2020; Yang H. et al., 2020). Another BCL-XL inhibitor A-1155463 has not been applied in the study of senescent cell clearance yet (Xu D. et al., 2021).
Interestingly, previous studies in IVDD have found that the apoptosis of normal functioning cells in the nucleus pulposus and endplate is an essential element to degeneration’s exacerbation (Chen et al., 2018; Xie et al., 2019), and researchers used to activate Bcl-2 by miRNA or chemical activators to inhibit the apoptosis and alleviate IVDD caused by apoptosis (Chen et al., 2015; Song et al., 2018). Given the contradiction, the challenge turns into how to guide Bcl-2 inhibitors to induce apoptosis in senescent cells exclusively instead of normal cells, which is the key to reducing the side effects of Bcl-2 inhibitors.
The latest study by Lim et al. (2021) elucidates that the BCl-2 inhibitor ABT-263 (navitoclax) did not display any interference to normal functioning cells while inducing the apoptosis of senescent NP cells in intervertebral disc. However, this study did not elucidate whether apoptosis of normal functioning cells occurred in the process of ABT-263 administration and whether the positive effect was dose related. In order to prevent ABT-263 (navitoclax) from acting on other tissues of the body, researchers loaded ABT-263 (navitoclax) in poly(lactic-co-glycolic acid) nanoparticles (PLGA-ABT), which can specifically release the reagent to the intervertebral disc. After the release of ABT-263, the secretion of SASP (IL-6 and MMP-13) in the intervertebral disc was significantly reduced, the degradation of ECM was weakened, and the structural integrity and stability of the intervertebral disc were restored as well (Lim et al., 2021). Besides ABT-263, several studies have also proven that some senolytic drugs could induce apoptosis of senescent disc cells in vitro and in vivo, providing inspirations for the application of BCL-2 inhibitor drugs in the treatment of IVDD (Wang et al., 2016; Xu et al., 2018; Wu et al., 2020). Although the study of Lim et al. has enlightened the application of senolytic in IVDD to a certain degree, how to accurately guide BCL-2 inhibitors to senescent cells in the intervertebral disc and adjust the dosage so that the positive effects of BCL-2 inhibitors by inducing the apoptosis of senescent cells far outweigh the negative effects caused by normal functioning cell apoptosis requires further research.
Heat shock protein (HSP) refers to a protein group produced under the stimulation by stressors, especially for high-temperature environment (Richter et al., 2010). The majority of HSP family are known as molecular chaperones, which can assist proteins to fold correctly. In light of molecular weight, HSPs are divided into five categories: HSP110, HSP90, HSP70, HSP60, and small heat shock proteins (sHSPs) (Saibil, 2013). HSP90 plays a vital role in stabilizing key signal proteins from heat stress whose inhibition will lead to apoptosis in tumor cells (Pungsrinont et al., 2020; Khaledian et al., 2021). As a consequence, HSP90 has been intensively studied as a target of cancer chemotherapy, and some anti-tumor compounds that inhibit HSP90 have been developed.
In recent years, the role of HSP90 in cell senescence has aroused a wide interest. Currently, in nasopharyngeal carcinoma, age-related cardiac disorders, age-related macular degeneration, and Alzheimer’s disease, the increase of HSP90 expression in senescent cells has been verified, and the senolytic activity of HSP90 inhibitors has also been recognized (Chan et al., 2013; Carnemolla et al., 2014; Wang et al., 2014; Fuhrmann-Stroissnigg et al., 2017; Chen et al., 2021; Criado-Marrero et al., 2021). Studies have found that HSP90 can activate Akt through phosphorylation and inhibit the apoptosis of senescent cells (Chen et al., 2017). In addition, according to what we have introduced before, DNA damage caused by radiation or external and internal factors is an important part of cell senescence and even apoptosis (Dote et al., 2006), and the expression of HSP90 increases during this process, which stabilizes the DDR reactive proteins in senescent cells (Solier et al., 2012; Orth et al., 2021).
Currently, the senolytic activity of HSP90 inhibitors has been gradually proven. For example, HSP90 inhibitor ganetespib has shown obvious senolytic effects in the treatment of prostate cancer (Pungsrinont et al., 2020). Besides anti-cancer effects, further studies on HSP90 inhibitors are suggested to evaluate its senolytic potential (Fuhrmann-Stroissnigg et al., 2017). In IVDD, inhibiting HSP90 in nucleoplasmic stem cells/progenitor cells (NPSCs) has been shown to alleviate the process, but its effect on the clearance of senescent cells in the intervertebral disc needs further exploration (Hu et al., 2020).
SirT1 is a deacetylase that can promote autophagy and suppress cell apoptosis and inflammation by inhibiting the p53-p21-Rb axis (Rubinsztein et al., 2011). SirT1-autophagy pathway’s activation can effectively slow down the senescence of AF and NP cells in the intervertebral disc (Zhou et al., 2016; Zhu et al., 2021). Except for NF-κB, SirT1 pathway has also been proven to be one of quercetin’s aging-resistant targets (Lazo-Gomez and Tapia, 2017; Liu et al., 2020). The study of Wang D. et al. (2020) has proven that quercetin can inhibit apoptosis and relieve IVDD by activating SirT1 in the intervertebral disc in vivo and in vitro. Resveratrol, a strong activator of SirT1, is also proven to alleviate IVDD (Li et al., 2019). However, its anti-apoptotic properties suggest that it should be classified as senomorphic senomorphic/senostatic, revealing that SirT1 may be a senormorphic’s target instead of senolytic’s.
In addition, some studies have also found that MDM2, HDAC, OXR1, and USP7 have the potential as senolytic targets (Samaraweera et al., 2017; Zhang et al., 2018; He et al., 2020a; Xu et al., 2020). Whether these targets can be inhibited by senolytics and subsequently decelerate the progression of IVDD needs to be further studied. Unambiguously, research on senolytic targets can provide a molecular basis for us to avoid side effects when applying such drugs in the future.
In this review, we first sort out the mechanism of cellular senescence, including RS and SIPS. SIPS triggered by external stimuli is considered to be one of the main reactions in IVDD, which includes DDR, oxidative stress, and mitochondrial dysfunction.
Secondly, we discussed the role and mechanism of senescent cells in the process of IVDD through SASP. The p16INK4a-Rb and the p53-p21-Rb pathways are considered to be two key signaling axes in regulating SIPS. The expression of p16INK4a in NP and AF cells increased in the intervertebral discs of young IVDD patients, with the telomeres shortened, which proved the intrinsic connection between cellular senescence and IVDD (Le Maitre et al., 2007). At present, it is generally believed that the mechanism of cell senescence affecting the intervertebral disc is as follows. Firstly, senescent NP and AF cells secrete SASP to promote ECM degradation and extracellular inflammation. Then, the intervertebral disc degenerates and loses its stability, causing low back pain and cervical and lumbar spondylosis.
Therefore, senotherapy, which eliminates senescent cells through senolytics or down-regulates the expression of related SASP by senomorphics/senostatics, has become a potential treatment for IVDD (Schmitt, 2017). Compared with surgical treatment and pain-relieving glucocorticoid therapy, the advantage of senotherapy is that it can intervene before the onset of IVDD symptoms. Unluckily, since the components of SASP produced in intervertebral discs are not yet clearly clarified, the application of senomorphics in IVDD remains to be further studied. However, senolytics that reduce the secretion of SASP by eliminating senescent cells have a greater chance of becoming a therapeutic drug for IVDD. Thus, we thirdly summarized several targets of senolytics, including PI3K/Akt, BCL-2 family, and HSP90.
Finally, although several senolytics have been proven to alleviate IVDD in murine, it is still unclear whether the clinical application of senolytics has an effect on the intervertebral disc. Hence, we summarized the intracellular targets of reagents with senolytic properties, reviewed their application in IVDD, and determined their clinical research status and whether they are approved by the FDA (Table 1).
TABLE 1. Identified senolytic agents, their targets, whether the role has been validated in IVDD, clinical trial’s status, and whether approved by the FDA.
Since some senolytics are clinically applied drugs, we introduce some early clinical research and application prospects for their senescent cell-eliminating property as follows.
Pre-clinical studies have proven that the application of senolytics to remove senescent cells could lead to tissue renewal and better physical performance. Moreover, several intracellular targets for senolytics have also been discovered, which provide directions for senolytic development and potential clinical applications in aging-related diseases. After discovering the senolytic effects of quercetin and dasatinib, Dr. Kirkland was also the first to put it into clinical trial. They recruited 14 patients with idiopathic pulmonary fibrosis caused by senescent cells and found that the exercise capacity of these patients continues to improve with the in-depth dasatinib + quercetin combination therapy, which is a miracle for patients with pulmonary fibrosis who were considered incurable before (Justice et al., 2019). The subsequent trial of Dr. Kirkland on patients with diabetic nephropathy manifested that senolytics can eliminate senescent cells in the human body as senescent cell markers and the level of SASP decreased significantly (Hickson et al., 2019).
Since senescent cells are also involved in beneficial processes such as wound healing, embryonic development, tissue regeneration, and cancer prevention, long-term use of senolytics may have potential side effects (He and Sharpless, 2017), and the usage plans of senolytics also need further discussion. Should senolytics be used systemically or locally and long-term or short-term? Accordingly, further studies are needed to enhance effectiveness without weakening the positive effects of cellular senescence before it is applied as an anti-aging drug.
XZ, YZ, NT, and YW initiated and designed the study. YS, YW, and SS wrote the manuscript. YS and YW designed tables and figures. XZ, YZ, and NT revised the paper. All authors contributed to the article and approved the submitted version.
This study was funded by the Zhejiang Provincial Natural Science Foundation of China (LGF21H060011), the National Natural Science Foundation of China (81972094), the Wenzhou Science and Technology Bureau Foundation (Y2020059), and the Lin He’s New Medicine and Clinical Translation Academician Workstation Research Fund (18331213).
The authors declare that the research was conducted in the absence of any commercial or financial relationships that could be construed as a potential conflict of interest.
All claims expressed in this article are solely those of the authors and do not necessarily represent those of their affiliated organizations, or those of the publisher, the editors and the reviewers. Any product that may be evaluated in this article, or claim that may be made by its manufacturer, is not guaranteed or endorsed by the publisher.
Adams, J. M., and Cory, S. (1998). The Bcl-2 Protein Family: Arbiters of Cell Survival. Science 281 (5381), 1322–1326. doi:10.1126/science.281.5381.1322
Adams, M. A., and Roughley, P. J. (2006). What is Intervertebral Disc Degeneration, and what Causes it? Spine 31 (18), 2151–2161. doi:10.1097/01.brs.0000231761.73859.2c
Aguado, J., Chaggar, H. K., Gómez‐Inclán, C., Shaker, M. R., Leeson, H. C., Mackay‐Sim, A., et al. (2021). Inhibition of the cGAS‐STING Pathway Ameliorates the Premature Senescence Hallmarks of Ataxia‐Telangiectasia Brain Organoids. Aging Cell 20 (9), e13468. doi:10.1111/acel.13468
Alvarez-Garcia, O., Matsuzaki, T., Olmer, M., Masuda, K., and Lotz, M. K. (2017). Age-related Reduction in the Expression of FOXO Transcription Factors and Correlations with Intervertebral Disc Degeneration. J. Orthop. Res. 35 (12), 2682–2691. doi:10.1002/jor.23583
Amor, C., Feucht, J., Leibold, J., Ho, Y.-J., Zhu, C., Alonso-Curbelo, D., et al. (2020). Senolytic CAR T Cells Reverse Senescence-Associated Pathologies. Nature 583 (7814), 127–132. doi:10.1038/s41586-020-2403-9
Baker, D. J., Wijshake, T., Tchkonia, T., LeBrasseur, N. K., Childs, B. G., van de Sluis, B., et al. (2011). Clearance of p16Ink4a-Positive Senescent Cells Delays Ageing-Associated Disorders. Nature 479 (7372), 232–236. doi:10.1038/nature10600
Barnes, P. J., Baker, J., and Donnelly, L. E. (2019). Cellular Senescence as a Mechanism and Target in Chronic Lung Diseases. Am. J. Respir. Crit. Care Med. 200 (5), 556–564. doi:10.1164/rccm.201810-1975TR
Barrera-Vázquez, O. S., Gómez-Verjan, J. C., and Magos-Guerrero, G. A. (2021). Chemoinformatic Screening for the Selection of Potential Senolytic Compounds from Natural Products. Biomolecules 11 (3), 467. doi:10.3390/biom11030467
Basisty, N., Kale, A., Jeon, O. H., Kuehnemann, C., Payne, T., Rao, C., et al. (2020). A Proteomic Atlas of Senescence-Associated Secretomes for Aging Biomarker Development. PLoS Biol. 18 (1), e3000599. doi:10.1371/journal.pbio.3000599
Bedore, J., Leask, A., and Séguin, C. A. (2014). Targeting the Extracellular Matrix: Matricellular Proteins Regulate Cell-Extracellular Matrix Communication within Distinct Niches of the Intervertebral Disc. Matrix Biol. 37, 124–130. doi:10.1016/j.matbio.2014.05.005
Bhatti, J. S., Bhatti, G. K., and Reddy, P. H. (2017). Mitochondrial Dysfunction and Oxidative Stress in Metabolic Disorders - A Step towards Mitochondria Based Therapeutic Strategies. Biochim. Biophys. Acta Mol. Basis Dis. 1863 (5), 1066–1077. doi:10.1016/j.bbadis.2016.11.010
Birch, J., and Gil, J. (2020). Senescence and the SASP: many Therapeutic Avenues. Genes Dev. 34 (23–24), 1565–1576. doi:10.1101/gad.343129.120
Borodkina, A., Shatrova, A., Abushik, P., Nikolsky, N., and Burova, E. (2014). Interaction between ROS Dependent DNA Damage, Mitochondria and P38 MAPK Underlies Senescence of Human Adult Stem Cells. Aging 6 (6), 481–495. doi:10.18632/aging.100673
Brinjikji, W., Diehn, F. E., Jarvik, J. G., Carr, C. M., Kallmes, D. F., Murad, M. H., et al. (2015). MRI Findings of Disc Degeneration Are More Prevalent in Adults with Low Back Pain Than in Asymptomatic Controls: A Systematic Review and Meta-Analysis. Am. J. Neuroradiol. 36 (12), 2394–2399. doi:10.3174/ajnr.A4498
Calcinotto, A., Kohli, J., Zagato, E., Pellegrini, L., Demaria, M., and Alimonti, A. (2019). Cellular Senescence: Aging, Cancer, and Injury. Physiol. Rev. 99 (2), 1047–1078. doi:10.1152/physrev.00020.2018
Calero, R., Morchon, E., Martinez-Argudo, I., and Serrano, R. (2017). Synergistic Anti-tumor Effect of 17AAG with the PI3K/mTOR Inhibitor NVP-BEZ235 on Human Melanoma. Cancer Lett. 406, 1–11. doi:10.1016/j.canlet.2017.07.021
Carnemolla, A., Labbadia, J. P., Lazell, H., Neueder, A., Moussaoui, S., and Bates, G. P. (2014). Contesting the Dogma of an Age-Related Heat Shock Response Impairment: Implications for Cardiac-specific Age-Related Disorders. Hum. Mol. Genet. 23 (14), 3641–3656. doi:10.1093/hmg/ddu073
Carrino, J. A., Lurie, J. D., Tosteson, A. N. A., Tosteson, T. D., Carragee, E. J., Kaiser, J., et al. (2009). Lumbar Spine: Reliability of MR Imaging Findings. Radiology 250 (1), 161–170. doi:10.1148/radiol.2493071999
Chan, K. C., Ting, C. M., Chan, P. S., Lo, M. C., Lo, K. W., Curry, J. E., et al. (2013). A Novel Hsp90 Inhibitor AT13387 Induces Senescence in EBV-Positive Nasopharyngeal Carcinoma Cells and Suppresses Tumor Formation. Mol. Cancer 12 (1), 128. doi:10.1186/1476-4598-12-128
Che, H., Li, J., Li, Y., Ma, C., Liu, H., Qin, J., et al. (2020). p16 Deficiency Attenuates Intervertebral Disc Degeneration by Adjusting Oxidative Stress and Nucleus Pulposus Cell Cycle. Elife 9. doi:10.7554/eLife.52570
Chen, D.-D., Peng, X., Wang, Y., Jiang, M., Xue, M., Shang, G., et al. (2021). HSP90 Acts as a Senomorphic Target in Senescent Retinal Pigmental Epithelial Cells. Aging 13 (17), 21547–21570. doi:10.18632/aging.203496
Chen, D., Xia, D., Pan, Z., Xu, D., Zhou, Y., Wu, Y., et al. (2016). Metformin Protects against Apoptosis and Senescence in Nucleus Pulposus Cells and Ameliorates Disc Degeneration In Vivo. Cell Death Dis 7 (10), e2441. doi:10.1038/cddis.2016.334
Chen, H., Wang, J., Hu, B., Wu, X., Chen, Y., Li, R., et al. (2015). MiR-34a Promotes Fas-Mediated Cartilage Endplate Chondrocyte Apoptosis by Targeting Bcl-2. Mol. Cell Biochem. 406 (1-2), 21–30. doi:10.1007/s11010-015-2420-4
Chen, J.-H., Hales, C. N., and Ozanne, S. E. (2007). DNA Damage, Cellular Senescence and Organismal Ageing: Causal or Correlative? Nucleic Acids Res. 35 (22), 7417–7428. doi:10.1093/nar/gkm681
Chen, J., Xie, J.-J., Jin, M.-Y., Gu, Y.-T., Wu, C.-C., Guo, W.-J., et al. (2018). Sirt6 Overexpression Suppresses Senescence and Apoptosis of Nucleus Pulposus Cells by Inducing Autophagy in a Model of Intervertebral Disc Degeneration. Cell Death Dis. 9 (2), 56. doi:10.1038/s41419-017-0085-5
Chen, X., Zhao, X., Cai, H., Sun, H., Hu, Y., Huang, X., et al. (2017). The Role of Sodium Hydrosulfide in Attenuating the Aging Process via PI3K/AKT and CaMKKβ/AMPK Pathways. Redox Biol. 12, 987–1003. doi:10.1016/j.redox.2017.04.031
Cherif, H., Bisson, D. G., Mannarino, M., Rabau, O., Ouellet, J. A., and Haglund, L. (2020). Senotherapeutic Drugs for Human Intervertebral Disc Degeneration and Low Back Pain. Elife 9. doi:10.7554/eLife.54693
Cherif, H., Bisson, D., Jarzem, P., Weber, M., Ouellet, J., and Haglund, L. (2019). Curcumin and O-Vanillin Exhibit Evidence of Senolytic Activity in Human IVD Cells In Vitro. J. Clin. Med. 8 (4), 433. doi:10.3390/jcm8040433
Chien, Y., Scuoppo, C., Wang, X., Fang, X., Balgley, B., Bolden, J. E., et al. (2011). Control of the Senescence-Associated Secretory Phenotype by NF-κB Promotes Senescence and Enhances Chemosensitivity. Genes Dev. 25 (20), 2125–2136. doi:10.1101/gad.17276711
Childs, B. G., Baker, D. J., Wijshake, T., Conover, C. A., Campisi, J., and van Deursen, J. M. (2016). Senescent Intimal Foam Cells are Deleterious at All Stages of Atherosclerosis. Science 354 (6311), 472–477. doi:10.1126/science.aaf6659
Chipuk, J. E., Kuwana, T., Bouchier-Hayes, L., Droin, N. M., Newmeyer, D. D., Schuler, M., et al. (2004). Direct Activation of Bax by P53 Mediates Mitochondrial Membrane Permeabilization and Apoptosis. Science 303 (5660), 1010–1014. doi:10.1126/science.1092734
Chong, S. J. F., Marchi, S., Petroni, G., Kroemer, G., Galluzzi, L., and Pervaiz, S. (2020). Noncanonical Cell Fate Regulation by Bcl-2 Proteins. Trends Cell Biol. 30 (7), 537–555. doi:10.1016/j.tcb.2020.03.004
Criado-Marrero, M., Gebru, N. T., Blazier, D. M., Gould, L. A., Baker, J. D., Beaulieu-Abdelahad, D., et al. (2021). Hsp90 Co-Chaperones, FKBP52 and Aha1, Promote Tau Pathogenesis in Aged Wild-type Mice. Acta Neuropathol. Commun. 9 (1), 65. doi:10.1186/s40478-021-01159-w
Cully, M., You, H., Levine, A. J., and Mak, T. W. (2006). Beyond PTEN Mutations: the PI3K Pathway as an Integrator of Multiple Inputs during Tumorigenesis. Nat. Rev. Cancer 6 (3), 184–192. doi:10.1038/nrc1819
de Magalhães, J. P., and Passos, J. F. (2018). Stress, Cell Senescence and Organismal Ageing. Mech. Ageing Dev. 170, 2–9. doi:10.1016/j.mad.2017.07.001
Delbridge, A. R. D., Grabow, S., Strasser, A., and Vaux, D. L. (2016). Thirty Years of BCL-2: Translating Cell Death Discoveries into Novel Cancer Therapies. Nat. Rev. Cancer 16 (2), 99–109. doi:10.1038/nrc.2015.17
Di Micco, R., Krizhanovsky, V., Baker, D., and d’Adda di Fagagna, F. (2021). Cellular Senescence in Ageing: from Mechanisms to Therapeutic Opportunities. Nat. Rev. Mol. Cell Biol. 22 (2), 75–95. doi:10.1038/s41580-020-00314-w
Dominic, A., Banerjee, P., Hamilton, D. J., Le, N.-T., and Abe, J.-i. (2020). Time-dependent Replicative Senescence vs. Disturbed Flow-Induced Pre-mature Aging in Atherosclerosis. Redox Biol. 37, 101614. doi:10.1016/j.redox.2020.101614
Dote, H., Burgan, W. E., Camphausen, K., and Tofilon, P. J. (2006). Inhibition of Hsp90 Compromises the DNA Damage Response to Radiation. Cancer Res. 66 (18), 9211–9220. doi:10.1158/0008-5472.CAN-06-2181
Dudek, M., Yang, N., Ruckshanthi, J. P., Williams, J., Borysiewicz, E., Wang, P., et al. (2017). The Intervertebral Disc Contains Intrinsic Circadian Clocks that Are Regulated by Age and Cytokines and Linked to Degeneration. Ann. Rheum. Dis. 76 (3), 576–584. doi:10.1136/annrheumdis-2016-209428
Dutta Gupta, S., and Pan, C. H. (2020). Recent Update on Discovery and Development of Hsp90 Inhibitors as Senolytic Agents. Int. J. Biol. Macromol. 161, 1086–1098. doi:10.1016/j.ijbiomac.2020.06.115
Faget, D. V., Ren, Q., and Stewart, S. A. (2019). Unmasking Senescence: Context-Dependent Effects of SASP in Cancer. Nat. Rev. Cancer 19 (8), 439–453. doi:10.1038/s41568-019-0156-2
Farr, J. N., Xu, M., Weivoda, M. M., Monroe, D. G., Fraser, D. G., Onken, J. L., et al. (2017). Targeting Cellular Senescence Prevents Age-Related Bone Loss in Mice. Nat. Med. 23 (9), 1072–1079. doi:10.1038/nm.4385
Finkel, T., and Holbrook, N. J. (2000). Oxidants, Oxidative Stress and the Biology of Ageing. Nature 408 (6809), 239–247. doi:10.1038/35041687
Fouda, M. A., Ghovanloo, M. R., and Ruben, P. C. (2020). Cannabidiol Protects against High Glucose‐induced Oxidative Stress and Cytotoxicity in Cardiac Voltage‐gated Sodium Channels. Br. J. Pharmacol. 177 (13), 2932–2946. doi:10.1111/bph.15020
Freund, A., Patil, C. K., and Campisi, J. (2011). p38MAPK Is a Novel DNA Damage Response-independent Regulator of the Senescence-Associated Secretory Phenotype. EMBO J. 30 (8), 1536–1548. doi:10.1038/emboj.2011.69
Fuhrmann-Stroissnigg, H., Ling, Y. Y., Zhao, J., McGowan, S. J., Zhu, Y., Brooks, R. W., et al. (2017). Identification of HSP90 Inhibitors as a Novel Class of Senolytics. Nat. Commun. 8 (1), 422. doi:10.1038/s41467-017-00314-z
Gandhi, L., Camidge, D. R., Ribeiro de Oliveira, M., Bonomi, P., Gandara, D., Khaira, D., et al. (2011). Phase I Study of Navitoclax (ABT-263), a Novel Bcl-2 Family Inhibitor, in Patients with Small-Cell Lung Cancer and Other Solid Tumors. Jco 29 (7), 909–916. doi:10.1200/JCO.2010.31.6208
Gao, C., Ning, B., Sang, C., and Zhang, Y. (2018). Rapamycin Prevents the Intervertebral Disc Degeneration via Inhibiting Differentiation and Senescence of Annulus Fibrosus Cells. Aging 10 (1), 131–143. doi:10.18632/aging.101364
Ge, M., Hu, L., Ao, H., Zi, M., Kong, Q., and He, Y. (2021). Senolytic Targets and New Strategies for Clearing Senescent Cells. Mech. Ageing Dev. 195, 111468. doi:10.1016/j.mad.2021.111468
Giordano, F. J. (2005). Oxygen, Oxidative Stress, Hypoxia, and Heart Failure. J. Clin. Invest. 115 (3), 500–508. doi:10.1172/jci200524408
Gladyshev, V. N. (2013). The Origin of Aging: Imperfectness-Driven Non-random Damage Defines the Aging Process and Control of Lifespan. Trends Genet. 29 (9), 506–512. doi:10.1016/j.tig.2013.05.004
Gorth, D. J., Shapiro, I. M., and Risbud, M. V. (2019). A New Understanding of the Role of IL‐1 in Age‐Related Intervertebral Disc Degeneration in a Murine Model. J. Bone Miner Res. 34 (8), 1531–1542. doi:10.1002/jbmr.3714
Greenhill, C. (2019). Regulating Metabolism and Ageing - the Role of PI3K. Nat. Rev. Endocrinol. 15 (7), 376–377. doi:10.1038/s41574-019-0208-4
Gruber, H. E., Ingram, J. A., Hoelscher, G. L., Zinchenko, N., Norton, H. J., and Hanley, E. N. (2011). Constitutive Expression of Cathepsin K in the Human Intervertebral Disc: New Insight into Disc Extracellular Matrix Remodeling via Cathepsin K and Receptor Activator of Nuclear Factor-κB Ligand. Arthritis Res. Ther. 13 (4), R140. doi:10.1186/ar3454
Han, S. Y., Ko, A., Kitano, H., Choi, C. H., Lee, M.-S., Seo, J., et al. (2017). Molecular Chaperone HSP90 Is Necessary to Prevent Cellular Senescence via Lysosomal Degradation of p14ARF. Cancer Res. 77 (2), 343–354. doi:10.1158/0008-5472.CAN-16-0613
Han, Y., Yuan, F., Deng, C., He, F., Zhang, Y., Shen, H., et al. (2019). Metformin Decreases LPS-Induced Inflammatory Response in Rabbit Annulus Fibrosus Stem/progenitor Cells by Blocking HMGB1 Release. Aging 11 (22), 10252–10265. doi:10.18632/aging.102453
He, S., and Sharpless, N. E. (2017). Senescence in Health and Disease. Cell 169 (6), 1000–1011. doi:10.1016/j.cell.2017.05.015
He, Y., Li, W., Lv, D., Zhang, X., Zhang, X., Ortiz, Y. T., et al. (2020a). Inhibition of USP7 Activity Selectively Eliminates Senescent Cells in Part via Restoration of P53 Activity. Aging Cell 19 (3), e13117. doi:10.1111/acel.13117
He, Y., Zhang, X., Chang, J., Kim, H.-N., Zhang, P., Wang, Y., et al. (2020b). Using Proteolysis-Targeting Chimera Technology to Reduce Navitoclax Platelet Toxicity and Improve its Senolytic Activity. Nat. Commun. 11 (1), 1996. doi:10.1038/s41467-020-15838-0
Hernández, L., Terradas, M., Camps, J., Martín, M., Tusell, L., and Genescà, A. (2015). Aging and Radiation: Bad Companions. Aging Cell 14 (2), 153–161. doi:10.1111/acel.12306
Herranz, N., Gallage, S., Mellone, M., Wuestefeld, T., Klotz, S., Hanley, C. J., et al. (2015). mTOR Regulates MAPKAPK2 Translation to Control the Senescence-Associated Secretory Phenotype. Nat. Cell Biol. 17 (9), 1205–1217. doi:10.1038/ncb3225
Herranz, N., and Gil, J. (2018). Mechanisms and Functions of Cellular Senescence. J. Clin. Invest. 128 (4), 1238–1246. doi:10.1172/JCI95148
Hickson, L. J., Langhi Prata, L. G. P., Bobart, S. A., Evans, T. K., Giorgadze, N., Hashmi, S. K., et al. (2019). Senolytics Decrease Senescent Cells in Humans: Preliminary Report from a Clinical Trial of Dasatinib Plus Quercetin in Individuals with Diabetic Kidney Disease. EBioMedicine 47, 446–456. doi:10.1016/j.ebiom.2019.08.069
Hoesel, B., and Schmid, J. A. (2013). The Complexity of NF-Κb Signaling in Inflammation and Cancer. Mol. Cancer 12, 86. doi:10.1186/1476-4598-12-86
Hood, S., and Amir, S. (2017). The Aging Clock: Circadian Rhythms and Later Life. J. Clin. Invest. 127 (2), 437–446. doi:10.1172/JCI90328
Horikawa, I. (2020). Balancing and Differentiating P53 Activities toward Longevity and No Cancer? Cancer Res. 80 (23), 5164–5165. doi:10.1158/0008-5472.CAN-20-3080
Hu, B., Zhang, S., Liu, W., Wang, P., Chen, S., Lv, X., et al. (2020). Inhibiting Heat Shock Protein 90 Protects Nucleus Pulposus-Derived Stem/Progenitor Cells from Compression-Induced Necroptosis and Apoptosis. Front. Cell Dev. Biol. 8, 685. doi:10.3389/fcell.2020.00685
Huang, Y.-C., Urban, J. P. G., and Luk, K. D. K. (2014). Intervertebral Disc Regeneration: Do Nutrients Lead the Way? Nat. Rev. Rheumatol. 10 (9), 561–566. doi:10.1038/nrrheum.2014.91
Hubackova, S., Davidova, E., Rohlenova, K., Stursa, J., Werner, L., Andera, L., et al. (2019). Selective Elimination of Senescent Cells by Mitochondrial Targeting Is Regulated by ANT2. Cell Death Differ 26 (2), 276–290. doi:10.1038/s41418-018-0118-3
Huggins, C. J., Malik, R., Lee, S., Salotti, J., Thomas, S., Martin, N., et al. (2013). C/EBPγ Suppresses Senescence and Inflammatory Gene Expression by Heterodimerizing with C/EBPβ. Mol. Cell Biol 33 (16), 3242–3258. doi:10.1128/MCB.01674-12
Jeon, O. H., Kim, C., Laberge, R.-M., Demaria, M., Rathod, S., Vasserot, A. P., et al. (2017). Local Clearance of Senescent Cells Attenuates the Development of Post-Traumatic Osteoarthritis and Creates a Pro-regenerative Environment. Nat. Med. 23 (6), 775–781. doi:10.1038/nm.4324
Jin, W.-N., Shi, K., He, W., Sun, J.-H., Van Kaer, L., Shi, F.-D., et al. (2021). Neuroblast Senescence in the Aged Brain Augments Natural Killer Cell Cytotoxicity Leading to Impaired Neurogenesis and Cognition. Nat. Neurosci. 24 (1), 61–73. doi:10.1038/s41593-020-00745-w
Junqueira, V. B. C., Barros, S. B. M., Chan, S. S., Rodrigues, L., Giavarotti, L., Abud, R. L., et al. (2004). Aging and Oxidative Stress. Mol. Aspects Med. 25 (1-2), 5–16. doi:10.1016/j.mam.2004.02.003
Justice, J. N., Nambiar, A. M., Tchkonia, T., LeBrasseur, N. K., Pascual, R., Hashmi, S. K., et al. (2019). Senolytics in Idiopathic Pulmonary Fibrosis: Results from a First-In-Human, Open-Label, Pilot Study. EBioMedicine 40, 554–563. doi:10.1016/j.ebiom.2018.12.052
Kakiuchi, Y., Yurube, T., Kakutani, K., Takada, T., Ito, M., Takeoka, Y., et al. (2019). Pharmacological Inhibition of mTORC1 but Not mTORC2 Protects against Human Disc Cellular Apoptosis, Senescence, and Extracellular Matrix Catabolism through Akt and Autophagy Induction. Osteoarthritis Cartilage 27 (6), 965–976. doi:10.1016/j.joca.2019.01.009
Kale, J., Osterlund, E. J., and Andrews, D. W. (2018). BCL-2 Family Proteins: Changing Partners in the Dance towards Death. Cell Death Differ 25 (1), 65–80. doi:10.1038/cdd.2017.186
Kang, C. (2019). Senolytics and Senostatics: A Two-Pronged Approach to Target Cellular Senescence for Delaying Aging and Age-Related Diseases. Mol. Cell 42 (12), 821–827. doi:10.14348/molcells.2019.0298
Kang, L., Xiang, Q., Zhan, S., Song, Y., Wang, K., Zhao, K., et al. (2019). Restoration of Autophagic Flux Rescues Oxidative Damage and Mitochondrial Dysfunction to Protect against Intervertebral Disc Degeneration. Oxid. Med. Cell Longev. 2019, 1–27. doi:10.1155/2019/7810320
Khaledian, B., Taguchi, A., Shin‐ya, K., Kondo‐Ida, L., Kagaya, N., Suzuki, M., et al. (2021). Inhibition of Heat Shock Protein 90 Destabilizes Receptor Tyrosine Kinase ROR1 in Lung Adenocarcinoma. Cancer Sci. 112 (3), 1225–1234. doi:10.1111/cas.14786
Khosla, S. (2013). Pathogenesis of Age-Related Bone Loss in Humans. Journals Gerontol. Ser. A: Biol. Sci. Med. Sci. 68 (10), 1226–1235. doi:10.1093/gerona/gls163
Kim, H., Hong, J. Y., Lee, J., Jeon, W.-J., and Ha, I.-H. (2021). IL-1β Promotes Disc Degeneration and Inflammation through Direct Injection of Intervertebral Disc in a Rat Lumbar Disc Herniation Model. Spine J. 21 (6), 1031–1041. doi:10.1016/j.spinee.2021.01.014
Kim, S. R., Jiang, K., Ogrodnik, M., Chen, X., Zhu, X.-Y., Lohmeier, H., et al. (2019). Increased Renal Cellular Senescence in Murine High-Fat Diet: Effect of the Senolytic Drug Quercetin. Transl. Res. 213, 112–123. doi:10.1016/j.trsl.2019.07.005
Kim, Y. C., and Guan, K.-L. (2015). mTOR: A Pharmacologic Target for Autophagy Regulation. J. Clin. Invest. 125 (1), 25–32. doi:10.1172/JCI73939
Korsmeyer, S. J. (1999). BCL-2 Gene Family and the Regulation of Programmed Cell Death. Cancer Res. 59 (7 Suppl), 1693s–1700s.
Koyasu, S. (2003). The Role of PI3K in Immune Cells. Nat. Immunol. 4 (4), 313–319. doi:10.1038/ni0403-313
Kraig, E., Linehan, L. A., Liang, H., Romo, T. Q., Liu, Q., Wu, Y., et al. (2018). A Randomized Control Trial to Establish the Feasibility and Safety of Rapamycin Treatment in an Older Human Cohort: Immunological, Physical Performance, and Cognitive Effects. Exp. Gerontol. 105, 53–69. doi:10.1016/j.exger.2017.12.026
Krupkova, O., Sadowska, A., Kameda, T., Hitzl, W., Hausmann, O. N., Klasen, J., et al. (2018). p38 MAPK Facilitates Crosstalk between Endoplasmic Reticulum Stress and IL-6 Release in the Intervertebral Disc. Front. Immunol. 9, 1706. doi:10.3389/fimmu.2018.01706
Lagoumtzi, S. M., and Chondrogianni, N. (2021). Senolytics and Senomorphics: Natural and Synthetic Therapeutics in the Treatment of Aging and Chronic Diseases. Free Radic. Biol. Med. 171, 169–190. doi:10.1016/j.freeradbiomed.2021.05.003
Lan, C.-Y., Chen, S.-Y., Kuo, C.-W., Lu, C.-C., and Yen, G.-C. (2019). Quercetin Facilitates Cell Death and Chemosensitivity through RAGE/PI3K/AKT/mTOR axis in Human Pancreatic Cancer Cells. J. Food Drug Anal. 27 (4), 887–896. doi:10.1016/j.jfda.2019.07.001
Lans, H., Hoeijmakers, J. H. J., Vermeulen, W., and Marteijn, J. A. (2019). The DNA Damage Response to Transcription Stress. Nat. Rev. Mol. Cell Biol. 20 (12), 766–784. doi:10.1038/s41580-019-0169-4
Lazo-Gomez, R., and Tapia, R. (2017). Quercetin Prevents Spinal Motor Neuron Degeneration Induced by Chronic Excitotoxic Stimulus by a Sirtuin 1-dependent Mechanism. Transl. Neurodegener. 6, 31. doi:10.1186/s40035-017-0102-8
Le Maitre, C., Freemont, A., and Hoyland, J. (2007). Accelerated Cellular Senescence in Degenerate Intervertebral Discs: a Possible Role in the Pathogenesis of Intervertebral Disc Degeneration. Arthritis Res. Ther. 9 (3), R45. doi:10.1186/ar2198
Lee-Theilen, M., Hadhoud, J. R., Volante, G., Fadini, D. D., Eichhorn, J., Rolle, U., et al. (2021). Co-Expression of CD34, CD90, OV-6 and Cell-Surface Vimentin Defines Cancer Stem Cells of Hepatoblastoma, Which are Affected by Hsp90 Inhibitor 17-AAG. Cells 10 (10), 2598. doi:10.3390/cells10102598
L’Hôte, V., Courbeyrette, R., Pinna, G., Cintrat, J. C., Le Pavec, G., Delaunay‐Moisan, A., et al. (2021). Ouabain and Chloroquine Trigger Senolysis of BRAF‐V600E‐induced Senescent Cells by Targeting Autophagy. Aging Cell 20 (9), e13447. doi:10.1111/acel.13447
Li, E.-q., and Zhang, J.-l. (2017). Essential Role of SH3GL1 in interleukin-6(IL-6)- and Vascular Endothelial Growth Factor (VEGF)-triggered P130cas-Mediated Proliferation and Migration of Osteosarcoma Cells. Hum. Cell 30 (4), 300–310. doi:10.1007/s13577-017-0178-6
Li, R.-Z., Fan, X.-X., Duan, F.-G., Jiang, Z.-B., Pan, H.-D., Luo, L.-X., et al. (2018). Proscillaridin A Induces Apoptosis and Suppresses Non-small-cell Lung Cancer Tumor Growth via Calcium-Induced DR4 Upregulation. Cell Death Dis. 9 (6), 696. doi:10.1038/s41419-018-0733-4
Li, X., Lin, F., Wu, Y., Liu, N., Wang, J., Chen, R., et al. (2019). Resveratrol Attenuates Inflammation Environment-Induced Nucleus Pulposus Cell Senescence In Vitro. Biosci. Rep. 39 (5). doi:10.1042/BSR20190126
Li, Z., Zhang, Z., Ren, Y., Wang, Y., Fang, J., Yue, H., et al. (2021). Aging and Age‐related Diseases: from Mechanisms to Therapeutic Strategies. Biogerontology 22 (2), 165–187. doi:10.1007/s10522-021-09910-5
Lieber, M. R., and Karanjawala, Z. E. (2004). Ageing, Repetitive Genomes and DNA Damage. Nat. Rev. Mol. Cell Biol. 5 (1), 69–75. doi:10.1038/nrm1281
Lim, H., Kwon, Y. S., Kim, D., Lee, J., and Kim, H. P. (2020). Flavonoids from Scutellaria Baicalensis Inhibit Senescence-Associated Secretory Phenotype Production by Interrupting IκBζ/C/EBPβ Pathway: Inhibition of Age-Related Inflammation. Phytomedicine 76, 153255. doi:10.1016/j.phymed.2020.153255
Lim, S., An, S. B., Jung, M., Joshi, H. P., Kumar, H., Kim, C., et al. (2021). Local Delivery of Senolytic Drug Inhibits Intervertebral Disc Degeneration and Restores Intervertebral Disc Structure. Adv. Healthc. Mater. 11, 2101483. doi:10.1002/adhm.202101483
Liu, J.-Y., Souroullas, G. P., Diekman, B. O., Krishnamurthy, J., Hall, B. M., Sorrentino, J. A., et al. (2019). Cells Exhibiting strong p16INK4a Promoter Activation In Vivo Display Features of Senescence. Proc. Natl. Acad. Sci. USA 116 (7), 2603–2611. doi:10.1073/pnas.1818313116
Liu, T., Yang, Q., Zhang, X., Qin, R., Shan, W., Zhang, H., et al. (2020). Quercetin Alleviates Kidney Fibrosis by Reducing Renal Tubular Epithelial Cell Senescence Through the SIRT1/PINK1/Mitophagy axis. Life Sci. 257, 118116. doi:10.1016/j.lfs.2020.118116
Lyons, C. E., and Bartolomucci, A. (2020). Stress and Alzheimer’s Disease: A Senescence Link? Neurosci. Biobehav. Rev. 115, 285–298. doi:10.1016/j.neubiorev.2020.05.010
Ma, T., Guo, C. J., Zhao, X., Wu, L., Sun, S. X., and Jin, Q. H. (2015). The Effect of Curcumin on NF-Κb Expression in Rat with Lumbar Intervertebral Disc Degeneration. Eur. Rev. Med. Pharmacol. Sci. 19 (7), 1305–1314.
Mannarino, M., Cherif, H., Li, L., Sheng, K., Rabau, O., Jarzem, P., et al. (2021). Toll-like Receptor 2 Induced Senescence in Intervertebral Disc Cells of Patients with Back Pain can be Attenuated by O-Vanillin. Arthritis Res. Ther. 23 (1), 117. doi:10.1186/s13075-021-02504-z
McGarry, T., Biniecka, M., Veale, D. J., and Fearon, U. (2018). Hypoxia, Oxidative Stress and Inflammation. Free Radic. Biol. Med. 125, 15–24. doi:10.1016/j.freeradbiomed.2018.03.042
McHugh, J. (2020). Linking Cell Mechanobiology and Inflammation in IVD Degeneration. Nat. Rev. Rheumatol. 16 (11), 604. doi:10.1038/s41584-020-00510-0
Méndez-Pertuz, M., Martínez, P., Blanco-Aparicio, C., Gómez-Casero, E., Belen García, A., Martínez-Torrecuadrada, J., et al. (2017). Modulation of Telomere protection by the PI3K/AKT Pathway. Nat. Commun. 8 (1), 1278. doi:10.1038/s41467-017-01329-2
Menolfi, D., and Zha, S. (2020). ATM, ATR and DNA-PKcs Kinases-The Lessons from the Mouse Models: Inhibition ≠ Deletion. Cell Biosci. 10, 8. doi:10.1186/s13578-020-0376-x
Miao, Z., Miao, Z., Wang, S., Shi, X., and Xu, S. (2021). Quercetin Antagonizes Imidacloprid-Induced Mitochondrial Apoptosis through PTEN/PI3K/AKT in Grass Carp Hepatocytes. Environ. Pollut. 290, 118036. doi:10.1016/j.envpol.2021.118036
Miyao, M., Cicalese, S., Kawai, T., Cooper, H. A., Boyer, M. J., Elliott, K. J., et al. (2020). Involvement of Senescence and Mitochondrial Fission in Endothelial Cell Pro-Inflammatory Phenotype Induced by Angiotensin II. Int. J. Mol. Sci. 21 (9), 3112. doi:10.3390/ijms21093112
Morisaki, H., Ando, A., Nagata, Y., Pereira-Smith, O., Smith, J. R., Ikeda, K., et al. (1999). Complex Mechanisms Underlying Impaired Activation of Cdk4 and Cdk2 in Replicative Senescence: Roles of P16, P21, and Cyclin D1. Exp. Cell Res. 253 (2), 503–510. doi:10.1006/excr.1999.4698
Muñoz-Espín, D., and Serrano, M. (2014). Cellular Senescence: from Physiology to Pathology. Nat. Rev. Mol. Cell Biol. 15 (7), 482–496. doi:10.1038/nrm3823
Nam, H. Y., Han, M. W., Chang, H. W., Kim, S. Y., and Kim, S. W. (2013). Prolonged Autophagy by MTOR Inhibitor Leads Radioresistant Cancer Cells into Senescence. Autophagy 9 (10), 1631–1632. doi:10.4161/auto.25879
Ngo, K., Patil, P., McGowan, S. J., Niedernhofer, L. J., Robbins, P. D., Kang, J., et al. (2017). Senescent Intervertebral Disc Cells Exhibit Perturbed Matrix Homeostasis Phenotype. Mech. Ageing Dev. 166, 16–23. doi:10.1016/j.mad.2017.08.007
Nogueira-Recalde, U., Lorenzo-Gómez, I., Blanco, F. J., Loza, M. I., Grassi, D., Shirinsky, V., et al. (2019). Fibrates as Drugs with Senolytic and Autophagic Activity for Osteoarthritis Therapy. EBioMedicine 45, 588–605. doi:10.1016/j.ebiom.2019.06.049
Novais, E. J., Diekman, B. O., Shapiro, I. M., and Risbud, M. V. (2019). p16Ink4a Deletion in Cells of the Intervertebral Disc Affects Their Matrix Homeostasis and Senescence Associated Secretory Phenotype without Altering Onset of Senescence. Matrix Biol. 82, 54–70. doi:10.1016/j.matbio.2019.02.004
Novais, E. J., Tran, V. A., Johnston, S. N., Darris, K. R., Roupas, A. J., Sessions, G. A., et al. (2021). Long-term Treatment with Senolytic Drugs Dasatinib and Quercetin Ameliorates Age-dependent Intervertebral Disc Degeneration in Mice. Nat. Commun. 12 (1), 5213. doi:10.1038/s41467-021-25453-2
Ogrodnik, M. (2021). Cellular Aging beyond Cellular Senescence: Markers of Senescence Prior to Cell Cycle Arrest In Vitro and In Vivo. Aging Cell 20 (4), e13338. doi:10.1111/acel.13338
Orth, M., Albrecht, V., Seidl, K., Kinzel, L., Unger, K., Hess, J., et al. (2021). Inhibition of HSP90 as a Strategy to Radiosensitize Glioblastoma: Targeting the DNA Damage Response and beyond. Front. Oncol. 11, 612354. doi:10.3389/fonc.2021.612354
Ou, H.-L., and Schumacher, B. (2018). DNA Damage Responses and P53 in the Aging Process. Blood 131 (5), 488–495. doi:10.1182/blood-2017-07-746396
Özcan, S., Alessio, N., Acar, M. B., Mert, E., Omerli, F., Peluso, G., et al. (2016). Unbiased Analysis of Senescence Associated Secretory Phenotype (SASP) to Identify Common Components Following Different Genotoxic Stresses. Aging 8 (7), 1316–1329. doi:10.18632/aging.100971
Ozsvari, B., Nuttall, J. R., Sotgia, F., and Lisanti, M. P. (2018). Azithromycin and Roxithromycin Define a New Family of “Senolytic” Drugs that Target Senescent Human Fibroblasts. Aging 10 (11), 3294–3307. doi:10.18632/aging.101633
Pang, L., Li, P., Zhang, R., Xu, Y., Song, L., and Zhou, Q. (2017). Role of P38-MAPK Pathway in the Effects of High-Magnitude Compression on Nucleus Pulposus Cell Senescence in a Disc Perfusion Culture. Biosci. Rep. 37 (5). doi:10.1042/BSR20170718
Panossian, A. G., Efferth, T., Shikov, A. N., Pozharitskaya, O. N., Kuchta, K., Mukherjee, P. K., et al. (2021). Evolution of the Adaptogenic Concept from Traditional Use to Medical Systems: Pharmacology of Stress‐ and Aging‐Related Diseases. Med. Res. Rev. 41 (1), 630–703. doi:10.1002/med.21743
Partridge, L., Fuentealba, M., and Kennedy, B. K. (2020). The Quest to Slow Ageing through Drug Discovery. Nat. Rev. Drug Discov. 19 (8), 513–532. doi:10.1038/s41573-020-0067-7
Passos, J. F., Saretzki, G., and von Zglinicki, T. (2007). DNA Damage in Telomeres and Mitochondria during Cellular Senescence: Is There a Connection? Nucleic Acids Res. 35 (22), 7505–7513. doi:10.1093/nar/gkm893
Patil, P., Dong, Q., Wang, D., Chang, J., Wiley, C., Demaria, M., et al. (2019). Systemic Clearance of P16 INK4a ‐positive Senescent Cells Mitigates Age‐associated Intervertebral Disc Degeneration. Aging Cell 18 (3), e12927. doi:10.1111/acel.12927
Phillips, K. L. E., Jordan-Mahy, N., Nicklin, M. J. H., and Le Maitre, C. L. (2013). Interleukin-1 Receptor Antagonist Deficient Mice Provide Insights into Pathogenesis of Human Intervertebral Disc Degeneration. Ann. Rheum. Dis. 72 (11), 1860–1867. doi:10.1136/annrheumdis-2012-202266
Pungsrinont, T., Sutter, M. F., Ertingshausen, M. C. C. M., Lakshmana, G., Kokal, M., Khan, A. S., et al. (2020). Senolytic Compounds Control a Distinct Fate of Androgen Receptor Agonist- and Antagonist-Induced Cellular Senescent LNCaP Prostate Cancer Cells. Cell Biosci. 10, 59. doi:10.1186/s13578-020-00422-2
Rahmani, M., Nkwocha, J., Hawkins, E., Pei, X., Parker, R. E., Kmieciak, M., et al. (2018). Cotargeting BCL-2 and PI3K Induces BAX-Dependent Mitochondrial Apoptosis in AML Cells. Cancer Res. 78 (11), 3075–3086. doi:10.1158/0008-5472.CAN-17-3024
Rayess, H., Wang, M. B., and Srivatsan, E. S. (2012). Cellular Senescence and Tumor Suppressor Gene P16. Int. J. Cancer 130 (8), 1715–1725. doi:10.1002/ijc.27316
Richter, K., Haslbeck, M., and Buchner, J. (2010). The Heat Shock Response: Life on the Verge of Death. Mol. Cell 40 (2), 253–266. doi:10.1016/j.molcel.2010.10.006
Roberts, S., Evans, H., Trivedi, J., and Menage, J. (2006). Histology and Pathology of the Human Intervertebral Disc. J. Bone Jt. Surg. Am. 88 (Suppl. 2), 10–14. doi:10.2106/jbjs.F.00019
Rodier, F., Coppé, J.-P., Patil, C. K., Hoeijmakers, W. A. M., Muñoz, D. P., Raza, S. R., et al. (2009). Persistent DNA Damage Signalling Triggers Senescence-Associated Inflammatory Cytokine Secretion. Nat. Cell Biol. 11 (8), 973–979. doi:10.1038/ncb1909
Rubinsztein, D. C., Mariño, G., and Kroemer, G. (2011). Autophagy and Aging. Cell 146 (5), 682–695. doi:10.1016/j.cell.2011.07.030
Saibil, H. (2013). Chaperone Machines for Protein Folding, Unfolding and Disaggregation. Nat. Rev. Mol. Cell Biol. 14 (10), 630–642. doi:10.1038/nrm3658
Sakai, D., Nakamura, Y., Nakai, T., Mishima, T., Kato, S., Grad, S., et al. (2012). Exhaustion of Nucleus Pulposus Progenitor Cells with Ageing and Degeneration of the Intervertebral Disc. Nat. Commun. 3, 1264. doi:10.1038/ncomms2226
Salaami, O., Kuo, C.-L., Drake, M. T., Kuchel, G. A., Kirkland, J. L., and Pignolo, R. J. (2021). Antidiabetic Effects of the Senolytic Agent Dasatinib. Mayo Clinic Proc. 96, 3021–3029. doi:10.1016/j.mayocp.2021.06.025
Samaraweera, L., Adomako, A., Rodriguez-Gabin, A., and McDaid, H. M. (2017). A Novel Indication for Panobinostat as a Senolytic Drug in NSCLC and HNSCC. Sci. Rep. 7 (1), 1900. doi:10.1038/s41598-017-01964-1
Sargiacomo, C., Sotgia, F., and Lisanti, M. P. (2020). COVID-19 and Chronological Aging: Senolytics and Other Anti-aging Drugs for the Treatment or Prevention of corona Virus Infection? Aging 12 (8), 6511–6517. doi:10.18632/aging.103001
Sas, K., Szabó, E., and Vécsei, L. (2018). Mitochondria, Oxidative Stress and the Kynurenine System, with a Focus on Ageing and Neuroprotection. Molecules 23 (1), 191. doi:10.3390/molecules23010191
Sasaki, M., Sato, Y., and Nakanuma, Y. (2020). Increased p16INK4a-Expressing Senescent Bile Ductular Cells are Associated with Inadequate Response to Ursodeoxycholic Acid in Primary Biliary Cholangitis. J. Autoimmun. 107, 102377. doi:10.1016/j.jaut.2019.102377
Sato, S., Solanas, G., Peixoto, F. O., Bee, L., Symeonidi, A., Schmidt, M. S., et al. (2017). Circadian Reprogramming in the Liver Identifies Metabolic Pathways of Aging. Cell 170 (4), 664–677. doi:10.1016/j.cell.2017.07.042
Schmeer, C., Kretz, A., Wengerodt, D., Stojiljkovic, M., and Witte, O. W. (2019). Dissecting Aging and Senescence-Current Concepts and Open Lessons. Cells 8 (11), 1446. doi:10.3390/cells8111446
Schmitt, R. (2017). Senotherapy: Growing Old and Staying Young? Pflugers Arch. - Eur. J. Physiol. 469 (9), 1051–1059. doi:10.1007/s00424-017-1972-4
Shao, Z., Wang, B., Shi, Y., Xie, C., Huang, C., Chen, B., et al. (2021). Senolytic Agent Quercetin Ameliorates Intervertebral Disc Degeneration via the Nrf2/NF-κB axis. Osteoarthritis Cartilage 29 (3), 413–422. doi:10.1016/j.joca.2020.11.006
She, Q.-B., Solit, D. B., Ye, Q., O’Reilly, K. E., Lobo, J., and Rosen, N. (2005). The BAD Protein Integrates Survival Signaling by EGFR/MAPK and PI3K/Akt Kinase Pathways in PTEN-Deficient Tumor Cells. Cancer Cell 8 (4), 287–297. doi:10.1016/j.ccr.2005.09.006
Sikora, E., Arendt, T., Bennett, M., and Narita, M. (2011). Impact of Cellular Senescence Signature on Ageing Research. Ageing Res. Rev. 10 (1), 146–152. doi:10.1016/j.arr.2010.10.002
Smith, L. J., Nerurkar, N. L., Choi, K.-S., Harfe, B. D., and Elliott, D. M. (2011). Degeneration and Regeneration of the Intervertebral Disc: Lessons from Development. Dis. Model. Mech. 4 (1), 31–41. doi:10.1242/dmm.006403
Solier, S., Kohn, K. W., Scroggins, B., Xu, W., Trepel, J., Neckers, L., et al. (2012). Heat Shock Protein 90 (HSP90), a Substrate and Chaperone of DNA-PK Necessary for the Apoptotic Response. Proc. Natl. Acad. Sci. U.S.A. 109 (32), 12866–12872. doi:10.1073/pnas.1203617109
Song, Y., Li, S., Geng, W., Luo, R., Liu, W., Tu, J., et al. (2018). Sirtuin 3-Dependent Mitochondrial Redox Homeostasis Protects against AGEs-Induced Intervertebral Disc Degeneration. Redox Biol. 19, 339–353. doi:10.1016/j.redox.2018.09.006
Stitt, T. N., Drujan, D., Clarke, B. A., Panaro, F., Timofeyva, Y., Kline, W. O., et al. (2004). The IGF-1/PI3K/Akt Pathway Prevents Expression of Muscle Atrophy-Induced Ubiquitin Ligases by Inhibiting FOXO Transcription Factors. Mol. Cell 14 (3), 395–403. doi:10.1016/s1097-2765(04)00211-4
Stojanović, S. D., Fiedler, J., Bauersachs, J., Thum, T., and Sedding, D. G. (2020). Senescence-induced Inflammation: An Important Player and Key Therapeutic Target in Atherosclerosis. Eur. Heart J. 41 (31), 2983–2996. doi:10.1093/eurheartj/ehz919
Stokman, G., Kors, L., Bakker, P. J., Rampanelli, E., Claessen, N., Teske, G. J. D., et al. (2017). NLRX1 Dampens Oxidative Stress and Apoptosis in Tissue Injury via Control of Mitochondrial Activity. J. Exp. Med. 214 (8), 2405–2420. doi:10.1084/jem.20161031
Sun, Y.-L., Jiang, W.-Q., Luo, Q.-Y., Yang, D.-J., Cai, Y.-C., Huang, H.-Q., et al. (2019). A Novel Bcl-2 Inhibitor, BM-1197, Induces Apoptosis in Malignant Lymphoma Cells through the Endogenous Apoptotic Pathway. BMC Cancer 20 (1), 1. doi:10.1186/s12885-019-6169-0
Tao, Y.-F., Wang, N.-N., Xu, L.-X., Li, Z.-H., Li, X.-L., Xu, Y.-Y., et al. (2017). Molecular Mechanism of G1 Arrest and Cellular Senescence Induced by LEE011, a Novel CDK4/CDK6 Inhibitor, in Leukemia Cells. Cancer Cell Int. 17, 35. doi:10.1186/s12935-017-0405-y
Triana-Martínez, F., Picallos-Rabina, P., Da Silva-Álvarez, S., Pietrocola, F., Llanos, S., Rodilla, V., et al. (2019). Identification and Characterization of Cardiac Glycosides as Senolytic Compounds. Nat. Commun. 10 (1), 4731. doi:10.1038/s41467-019-12888-x
Turnquist, C., Horikawa, I., Foran, E., Major, E. O., Vojtesek, B., Lane, D. P., et al. (2016). p53 Isoforms Regulate Astrocyte-Mediated Neuroprotection and Neurodegeneration. Cell Death Differ. 23 (9), 1515–1528. doi:10.1038/cdd.2016.37
Unity Biotechnology (2021). Safety and Tolerability Study of UBX1325 in Patients With Diabetic Macular Edema or Neovascular Age-Related Macular Degeneration. San Francisco, CA: Unity Biotechnology, Inc.
van Deursen, J. M. (2019). Senolytic Therapies for Healthy Longevity. Science 364 (6441), 636–637. doi:10.1126/science.aaw1299
van Deursen, J. M. (2014). The Role of Senescent Cells in Ageing. Nature 509 (7501), 439–446. doi:10.1038/nature13193
Vergroesen, P.-P. A., Kingma, I., Emanuel, K. S., Hoogendoorn, R. J. W., Welting, T. J., van Royen, B. J., et al. (2015). Mechanics and Biology in Intervertebral Disc Degeneration: A Vicious Circle. Osteoarthritis Cartilage 23 (7), 1057–1070. doi:10.1016/j.joca.2015.03.028
Victorelli, S., and Passos, J. F. (2017). Telomeres and Cell Senescence - Size Matters Not. EBioMedicine 21, 14–20. doi:10.1016/j.ebiom.2017.03.027
Vo, N. V., Hartman, R. A., Patil, P. R., Risbud, M. V., Kletsas, D., Iatridis, J. C., et al. (2016). Molecular Mechanisms of Biological Aging in Intervertebral Discs. J. Orthop. Res. 34 (8), 1289–1306. doi:10.1002/jor.23195
Wagner, V., and Gil, J. (2020). Senescence as a Therapeutically Relevant Response to CDK4/6 Inhibitors. Oncogene 39 (29), 5165–5176. doi:10.1038/s41388-020-1354-9
Wang, D., He, X., Wang, D., Peng, P., Xu, X., Gao, B., et al. (2020). Quercetin Suppresses Apoptosis and Attenuates Intervertebral Disc Degeneration via the SIRT1-Autophagy Pathway. Front. Cell Dev. Biol. 8, 613006. doi:10.3389/fcell.2020.613006
Wang, F., Cai, F., Shi, R., Wang, X.-H., and Wu, X.-T. (2016). Aging and Age Related Stresses: a Senescence Mechanism of Intervertebral Disc Degeneration. Osteoarthritis Cartilage 24 (3), 398–408. doi:10.1016/j.joca.2015.09.019
Wang, H., Tan, M.-S., Lu, R.-C., Yu, J.-T., and Tan, L. (20142014). Heat Shock Proteins at the Crossroads between Cancer and Alzheimer's Disease. Biomed. Res. Int. 2014, 1–9. doi:10.1155/2014/239164
Wang, J., Huang, C., Lin, Z., Pan, X., Chen, J., Zheng, G., et al. (2018). Polydatin Suppresses Nucleus Pulposus Cell Senescence, Promotes Matrix Homeostasis and Attenuates Intervertebral Disc Degeneration in Rats. J. Cell Mol. Med. 22 (11), 5720–5731. doi:10.1111/jcmm.13848
Wang, L., L., Doherty, G. A., Judd, A. S., Tao, Z.-F., Hansen, T. M., Frey, R. R., et al. (2020). Discovery of A-1331852, a First-In-Class, Potent, and Orally-Bioavailable BCL-XL Inhibitor. ACS Med. Chem. Lett. 11 (10), 1829–1836. doi:10.1021/acsmedchemlett.9b00568
Wang, R., Han, X., You, J., Yu, F., and Chen, L. (2018). Ratiometric Near-Infrared Fluorescent Probe for Synergistic Detection of Monoamine Oxidase B and its Contribution to Oxidative Stress in Cell and Mice Aging Models. Anal. Chem. 90 (6), 4054–4061. doi:10.1021/acs.analchem.7b05297
Wang, Y., Sharpless, N., and Chang, S. (2013). p16INK4a Protects against Dysfunctional Telomere-Induced ATR-Dependent DNA Damage Responses. J. Clin. Invest. 123 (10), 4489–4501. doi:10.1172/JCI69574
Weichhart, T. (2018). mTOR as Regulator of Lifespan, Aging, and Cellular Senescence: A Mini-Review. Gerontology 64 (2), 127–134. doi:10.1159/000484629
Wiley, C. D., Velarde, M. C., Lecot, P., Liu, S., Sarnoski, E. A., Freund, A., et al. (2016). Mitochondrial Dysfunction Induces Senescence with a Distinct Secretory Phenotype. Cell Metab. 23 (2), 303–314. doi:10.1016/j.cmet.2015.11.011
Wu, P. H., Kim, H. S., and Jang, I.-T. (2020). Intervertebral Disc Diseases PART 2: A Review of the Current Diagnostic and Treatment Strategies for Intervertebral Disc Disease. Int. J. Mol. Sci. 21 (6), 2135. doi:10.3390/ijms21062135
Xie, L., Huang, W., Fang, Z., Ding, F., Zou, F., Ma, X., et al. (2019). CircERCC2 Ameliorated Intervertebral Disc Degeneration by Regulating Mitophagy and Apoptosis through miR-182-5p/SIRT1 axis. Cell Death Dis. 10 (10), 751. doi:10.1038/s41419-019-1978-2
Xu, C. L., Sang, B., Liu, G. Z., Li, J. M., Zhang, X. D., Liu, L. X., et al. (2020). SENEBLOC, a Long Non-coding RNA Suppresses Senescence via P53-dependent and Independent Mechanisms. Nucleic Acids Res. 48 (6), 3089–3102. doi:10.1093/nar/gkaa063
Xu, C., Shen, W.-B., Reece, E. A., Hasuwa, H., Harman, C., Kaushal, S., et al. (2021). Maternal Diabetes Induces Senescence and Neural Tube Defects Sensitive to the Senomorphic Rapamycin. Sci. Adv. 7 (27). doi:10.1126/sciadv.abf5089
Xu, D., Liang, S.-Q., Yang, Z., Yang, H., Bruggmann, R., Oberhaensli, S., et al. (2021). Malignant Pleural Mesothelioma Co-opts BCL-XL and Autophagy to Escape Apoptosis. Cell Death Dis. 12 (4), 406. doi:10.1038/s41419-021-03668-x
Xu, J., Li, H., Yang, K., Guo, S., Wang, J., Feng, C., et al. (2019). Hyper-osmolarity Environment-Induced Oxidative Stress Injury Promotes Nucleus Pulposus Cell Senescence In Vitro. Biosci. Rep. 39 (9). doi:10.1042/BSR20191711
Xu, M., Pirtskhalava, T., Farr, J. N., Weigand, B. M., Palmer, A. K., Weivoda, M. M., et al. (2018). Senolytics Improve Physical Function and Increase Lifespan in Old Age. Nat. Med. 24 (8), 1246–1256. doi:10.1038/s41591-018-0092-9
Xu, Q., Fu, Q., Li, Z., Liu, H., Wang, Y., Lin, X., et al. (2021). The Flavonoid Procyanidin C1 Has Senotherapeutic Activity and Increases Lifespan in Mice. Nat. Metab. 3 (12), 1706–1726. doi:10.1038/s42255-021-00491-8
Yang, H., Chen, C., Chen, H., Duan, X., Li, J., Zhou, Y., et al. (2020). Navitoclax (ABT263) Reduces Inflammation and Promotes Chondrogenic Phenotype by Clearing Senescent Osteoarthritic Chondrocytes in Osteoarthritis. Aging 12 (13), 12750–12770. doi:10.18632/aging.103177
Yang, J., Nie, J., Ma, X., Wei, Y., Peng, Y., and Wei, X. (2019). Targeting PI3K in Cancer: Mechanisms and Advances in Clinical Trials. Mol. Cancer 18 (1), 26. doi:10.1186/s12943-019-0954-x
Yang, S., Zhang, F., Ma, J., and Ding, W. (2020). Intervertebral Disc Ageing and Degeneration: The Antiapoptotic Effect of Oestrogen. Ageing Res. Rev. 57, 100978. doi:10.1016/j.arr.2019.100978
Yosef, R., Pilpel, N., Tokarsky-Amiel, R., Biran, A., Ovadya, Y., Cohen, S., et al. (2016). Directed Elimination of Senescent Cells by Inhibition of BCL-W and BCL-XL. Nat. Commun. 7, 11190. doi:10.1038/ncomms11190
Yousefzadeh, M. J., Zhu, Y., McGowan, S. J., Angelini, L., Fuhrmann-Stroissnigg, H., Xu, M., et al. (2018). Fisetin Is a Senotherapeutic that Extends Health and Lifespan. EBioMedicine 36, 18–28. doi:10.1016/j.ebiom.2018.09.015
Zhang, C., Xie, Y., Chen, H., Lv, L., Yao, J., Zhang, M., et al. (2020). FOXO4-DRI Alleviates Age-Related Testosterone Secretion Insufficiency by Targeting Senescent Leydig Cells in Aged Mice. Aging 12 (2), 1272–1284. doi:10.18632/aging.102682
Zhang, P., Kishimoto, Y., Grammatikakis, I., Gottimukkala, K., Cutler, R. G., Zhang, S., et al. (2019). Senolytic Therapy Alleviates Aβ-Associated Oligodendrocyte Progenitor Cell Senescence and Cognitive Deficits in an Alzheimer’s Disease Model. Nat. Neurosci. 22 (5), 719–728. doi:10.1038/s41593-019-0372-9
Zhang, X., Dong, Y., Li, W.-c., Tang, B.-x., Li, J., and Zang, Y. (2021). Roxithromycin Attenuates Bleomycin-Induced Pulmonary Fibrosis by Targeting Senescent Cells. Acta Pharmacol. Sin 42, 2058–2068. doi:10.1038/s41401-021-00618-3
Zhang, X., Zhang, S., Liu, X., Wang, Y., Chang, J., Zhang, X., et al. (2018). Oxidation Resistance 1 is a Novel Senolytic Target. Aging Cell 17 (4), e12780. doi:10.1111/acel.12780
Zhang, Y., He, F., Chen, Z., Su, Q., Yan, M., Zhang, Q., et al. (2019). Melatonin Modulates IL-1β-induced Extracellular Matrix Remodeling in Human Nucleus Pulposus Cells and Attenuates Rat Intervertebral Disc Degeneration and Inflammation. Aging 11 (22), 10499–10512. doi:10.18632/aging.102472
Zhang, Y., Yang, B., Wang, J., Cheng, F., Shi, K., Ying, L., et al. (2020). Cell Senescence: A Nonnegligible Cell State under Survival Stress in Pathology of Intervertebral Disc Degeneration. Oxid. Med. Cell Longev. 2020, 1–12. doi:10.1155/2020/9503562
Zhao, C.-Q., Zhang, Y.-H., Jiang, S.-D., Li, H., Jiang, L.-S., and Dai, L.-Y. (2011). ADAMTS-5 and Intervertebral Disc Degeneration: the Results of Tissue Immunohistochemistry and In Vitro Cell Culture. J. Orthop. Res. 29 (5), 718–725. doi:10.1002/jor.21285
Zhao, J., Zhang, L., Lu, A., Han, Y., Colangelo, D., Bukata, C., et al. (2020). ATM is a Key Driver of NF-κB-dependent DNA-Damage-Induced Senescence, Stem Cell Dysfunction and Aging. Aging 12 (6), 4688–4710. doi:10.18632/aging.102863
Zhao, L., Tian, B., Xu, Q., Zhang, C., Zhang, L., and Fang, H. (2019). Extensive Mechanical Tension Promotes Annulus Fibrosus Cell Senescence Through Suppressing Cellular Autophagy. Biosci. Rep. 39 (4). doi:10.1042/BSR20190163
Zhong, H., Zhou, Z., Guo, L., Liu, F., Zheng, B., Bi, S., et al. (2021). The miR-623/CXCL12 axis Inhibits LPS-Induced Nucleus Pulposus Cell Apoptosis and Senescence. Mech. Ageing Dev. 194, 111417. doi:10.1016/j.mad.2020.111417
Zhou, J., Jiang, Y. Y., Chen, H., Wu, Y. C., and Zhang, L. (2020). Tanshinone I Attenuates the Malignant Biological Properties of Ovarian Cancer by Inducing Apoptosis and Autophagy via the Inactivation of PI3K/AKT/mTOR Pathway. Cell Prolif. 53 (2), e12739. doi:10.1111/cpr.12739
Zhou, N., Lin, X., Dong, W., Huang, W., Jiang, W., Lin, L., et al. (2016). SIRT1 Alleviates Senescence of Degenerative Human Intervertebral Disc Cartilage Endo-Plate Cells via the P53/p21 Pathway. Sci. Rep. 6, 22628. doi:10.1038/srep22628
Zhu, H., Sun, B., Zhu, L., Zou, G., and Shen, Q. (2021). N6-Methyladenosine Induced miR-34a-5p Promotes TNF-α-Induced Nucleus Pulposus Cell Senescence by Targeting SIRT1. Front. Cell Dev. Biol. 9, 642437. doi:10.3389/fcell.2021.642437
Keywords: senolytic, intervertebral disc degeneration, IVDD, senescence-associated secretory phenotype, SASP, cellular senescence, aging
Citation: Wu Y, Shen S, Shi Y, Tian N, Zhou Y and Zhang X (2022) Senolytics: Eliminating Senescent Cells and Alleviating Intervertebral Disc Degeneration. Front. Bioeng. Biotechnol. 10:823945. doi: 10.3389/fbioe.2022.823945
Received: 28 November 2021; Accepted: 21 January 2022;
Published: 02 March 2022.
Edited by:
Umberto Galderisi, University of Campania Luigi Vanvitelli, ItalyReviewed by:
Nicola Alessio, Università della Campania Luigi Vanvitelli, ItalyCopyright © 2022 Wu, Shen, Shi, Tian, Zhou and Zhang. This is an open-access article distributed under the terms of the Creative Commons Attribution License (CC BY). The use, distribution or reproduction in other forums is permitted, provided the original author(s) and the copyright owner(s) are credited and that the original publication in this journal is cited, in accordance with accepted academic practice. No use, distribution or reproduction is permitted which does not comply with these terms.
*Correspondence: Naifeng Tian, bmFpZmVuZ3RpYW5Ad211LmVkdS5jbg==; Yifei Zhou, eWlmZWl6aG91QHdtdS5lZHUuY24=; Xiaolei Zhang, emhhbmd4aWFvbGVpQHdtdS5lZHUuY24=
Disclaimer: All claims expressed in this article are solely those of the authors and do not necessarily represent those of their affiliated organizations, or those of the publisher, the editors and the reviewers. Any product that may be evaluated in this article or claim that may be made by its manufacturer is not guaranteed or endorsed by the publisher.
Research integrity at Frontiers
Learn more about the work of our research integrity team to safeguard the quality of each article we publish.