- 1Hubei Provincial Key Laboratory of Green Materials for Light Industry, Hubei University of Technology, Wuhan, China
- 2State Key Laboratory of Biobased Material and Green Papermaking, Shandong Academy of Sciences, Qilu University of Technology, Jinan, Shandong, China
- 3Key Laboratory of Fermentation Engineering (Ministry of Education), Cooperative Innovation Center of Industrial Fermentation (Ministry of Education and Hubei Province), College of Bioengineering, Hubei University of Technology, Wuhan, China
Introduction: A combination of deep eutectic solvent with ethanol was developed for pretreatment of Broussonetia papyrifera to effectively extract lignin and promote the subsequent enzymatic hydrolysis.
Methods: In order to further explore the optimal conditions for enzymatic hydrolysis, a central composite design method was applied.
Results and Discussion: The correlation between each factor and glucose yield was obtained, and the optimal conditions was 160°C, 60 min, the ratio of DES to E was 1/1 (mol/mol). The results showed that compared with control, the glucose yield increased by 130.67% under the optimal pretreatment conditions. Furthermore, the specific surface area of biomass was increased by 66.95%, and the content of xylan and lignin was decreased by 86.71% and 85.83%. The correlation between xylan/lignin removal and enzymatic hydrolysis showed that the removal of lignin facilitated the glucose yield more significantly than that of xylan. To further explore the lignin valorization, the structural and antioxidant analysis of recovered lignin revealed that high temperature was favorable for lignin with good antioxidant performance. This pretreatment is a promising method for separating lignin with high antioxidant activity and improving cellulose digestibility.
1 Introduction
In recent years, the sharp increase in the consumption of fossil fuels results in the rise of crude oil prices and serious environmental problems. As a kind of green energy, renewable energy can replace fossil fuel, which is widely concerned worldwide (Thi and Lee, 2019; Peng et al., 2021). One of them is bioethanol derived from lignocellulose. The composition of lignocellulose biomass is complex, which includes carbohydrate (cellulose, hemicellulose) and aromatic polymer (lignin) (Wang Y. et al., 2022). In the plant cell wall, hemicellulose and lignin are connected by covalent bonds and hydrogen bonds to form heterogenous structure, which is coated on cellulose to form is a strong “natural anti degradation barrier”. This barrier greatly limits the efficiency of biomass refinery (Penín et al., 2020). Pretreatment is an essential process to overcome the recalcitrance of biomass and achieve efficient fractionation of biomass components.
Deep eutectic solvents (DESs) are composed of hydrogen bond receptors (HBAs) and hydrogen bond donors (HBDs), which contain large and asymmetric ions, resulting in low lattice energy and therefore low melting point (Gontrani et al., 2019; Xu et al., 2020; Yu et al., 2021). Because DES could form rich hydrogen bonds, it has good solvability for polar and non-polar compounds (Pandey et al., 2017). DES pretreatment has achieved remarkable results in biomass fractionation and digestibility improvement (Hou et al., 2022). The cellulose conversion of DES pretreated corn stover and corncob exceeds 90% (Xu et al., 2016; Zhang et al., 2016; Hou et al., 2017). Furthermore, DES shows advantages of simple preparation, low toxicity, recyclability and biodegradability, thus wide application potential in the utilization of lignocellulose (Bu et al., 2021; Wang W. et al., 2022).
DES based on choline chloride (ChCL) can effectively break linkages within lignin carbohydrate complex (LCC) (Shen et al., 2019; 2021; Zhou et al., 2021). It was found that the addition of the third HBD component to the ChCL based DES can change fluidity and thermal stability of DES, thus improving the pretreatment performance of biomass (Xia et al., 2018). The most widely applied DES is composed of ChCL/LA (lactic acid). Earlier research showed that lignin re condensed during pretreatment (Bjelic et al., 2022). In our previous research, it was found that DES with ethanol was more efficient in digestibility improvement than ChCL/LA (Yao et al., 2022). However, the previous research on the optimization of pretreatment conditions is still incomplete.
Lignin is an important component that needs to be valorized to obtain total biomass utilization for biorefinery (Wang et al., 2022). Lignin itself has different kinds of active functional groups, which has advantages in scavenging free radicals. Therefore, it is of great interest to explore the value-added utilization of lignin. Organosolv lignins are the most studied one as antioxidant. Our previous research showed that physicochemical properties had great impact on the capability of scavenging free radicals (Yao et al., 2020). To be specific, the contents of phenolic hydroxyl groups had positive effects on the antioxidant activity of lignin. To further explore the impact of DES-E pretreatment on recovered lignin and its valorization, more research needs to be done. In this study, DES with ethanol was synthesized with choline chloride lactic acid and ethanol. The central composite design method was employed to obtain the best pretreatment conditions (mol ratio of DES to ethanol, temperature and time) to promote the enzymatic hydrolysis and saccharification of pretreated biomass. Furthermore, the structural properties and antioxidant activity of recovered lignin after pretreatment were analyzed. The relationship between the structural properties of lignin and its antioxidant activity was studied.
2 Material and methods
2.1 Materials
Broussonetia papyrifera was given by a local farmer in Wuhan, Hubei Province. After size reduction to 60–80 meshes, it was extracted with benzene-alcohol for 6 h. The component analysis results showed that the content of glucan, xylan, lignin and ash was 36.31%, 27.89%, 19.99%, and 1.01% respectively. Cellulase is provided by Baiyin Sainuo Technology Co., Ltd. (Gansu Province, China), with 160U/g filter paper activity. All other chemical reagents were purchased from Sigma without further purification.
2.2 Preparation of DES
After vacuum oven dried at 50°C for 24 h, lactic acid and choline chloride was mixed in a round bottom flask (2:1 M ratio) in an oil bath at 90°C for 2 h to form a uniform, stable and transparent liquid. After cooling to room temperature, it was vacuum oven dried for 1 week before use.
2.3 Pretreatment and lignin isolation
50 g dried wood powder and 500 g DES-E were mixed into a 1-L rotary electrothermal pressure digester (Parr Instrument Company). After keeping at a certain temperature for a period of time, the pretreated liquid and biomass was separated by filtration. The solid residues were first washed with excess ethanol to remove DES, and then washed with deionized water until the pH was neutral. The pretreated solid residues were stored at 4°C for further cellulase saccharification. In order to obtain the optimal pretreatment conditions, pretreatment temperature, time, and the ratio of DES to ethanol were optimized by central composite design (CCD). The statistical analysis software ‘‘Statistica 10.0” and ‘‘Minitab” (Minitab Inc.) were used for the experimental design and data analysis (p-value analysis), respectively. Pretreatment yield, component analysis and removal rate was calculated as follows:
After pretreatment, lignin rich liquid fractions were concentrated by rotary evaporation. The recovered lignin was then obtained by precipitating in excess amount of cold DI water. The precipitated lignins (140°C—lignin, 150°C—lignin, and 160°C—lignin) were obtained by centrifugation, washed with DI water and 90% ethanol, and finally freeze-dried, as shown in Figure 1.
2.4 Enzymatic hydrolysis
Pretreated Broussonetia papyrifera were enzymatically hydrolyzed with cellulase. The enzyme loading was 25 FPU/g, and the solid concentration was 2%. The enzyme hydrolysis was conducted at 50°C for 72 h. The glucose content was analyzed by HPLC (Shimadzu, Kyoto, Japan) with a refractive index detector (Shimadzu) on an Aminex HPX-87P column (Bio-Rad, Hercules, CA, United States), with water as eluent. The cellulose digestibility and glucose yield were calculated as follows:
2.5 SEM and BET determination of pretreated biomass
Scanning electron microscope (SU8010; HITACHI Ltd., Tokyo, Japan) was applied to observe the fiber morphology under 5 kV accelerating voltage.
The surface of pretreated biomass was determined by nitrogen adsorption method. The adsorption/desorption isotherms of nitrogen at 77 K were measured applying a BELSORP mini-II surface area and pore size analyzer (MicrotracBEL, Japan).
2.6 Determination of physical and chemical properties of recovered lignin
FT-IR 710 infrared spectrophotometer was employed for FTIR analysis. The Gel permeation chromatography was applied to determine the molecular weight of lignin (LC-10AD, Shimadzu Co., Ltd., Japan) after being acetylated (Kumar et al., 2013). Phenolic hydroxyl group content was determined by ultraviolet spectrophotometer (UV-2550, Shimadzu Co., Ltd., Japan) (Tan et al., 2019).
2.7 Determination of antioxidant activity by DPPH method
The antioxidant activity of lignin was determined by 1,1-diphenyl-2-trinitrophenylhydrazine (DPPH) method. Briefly, lignin was dissolved in 90% dioxane water (v/v) solution with different concentrations. Then lignin was mixed with DPPH solution in dark for 1 h, after which the ultraviolet absorption value of each solution was determined at 517 nm. The free radical scavenging activity and antioxidant activity indexes were calculated according to previous study (Sadeghifar et al., 2017).
3 Results and discussion
3.1 Optimization of pretreatment condition by response surface method
Different DESs (various mol ratios) were synthesized, and their performance in improving glucose yield of Broussonetia papyrifera at different temperature and time was evaluated. Based on the preliminary results, three factors (mol ratio of DES to E, temperature, time) were optimized by central composite design. The pretreatment conditions, cellulose digestibility and glucose yield were shown in Table 1.
Trials No. 9, 15, and 10 were conducted with the same pretreatment temperature and time, and the molar ratio of DES to ethanol was 1/2, 1/1 and 2/1, respectively. The results showed that pretreatment yield was decreased with the enhanced DES content (52.56%, 49.45%, and 46.06%), indicating that more DES could dissolve more biomass during pretreatment. The cellulose digestibility (83.31%, 93.52%, and 91.11%) and the glucose yield (43.78%, 46.25%, and 41.98%) was increased first and then decreased with more of DES content. The results showed that more DES loading did not favor enzymatic hydrolysis of pretreated Broussonetia papyrifera. This may be due to that the excessive removal of lignin and xylan, which led to the collapse of cellulose structure and the reduction of the available surface area of cellulose for cellulase adsorption (Shen et al., 2019).
Broussonetia papyrifera were pretreated at the same DES to E ratio and time at 150°C, 160°C, and 170°C in scheme No 11, 15, and 12, respectively. It was obvious to see that solid residue yield was decreased at higher pretreatment temperature (63.11%, 49.45%, and 45.15%). The cellulose digestibility was 66.27%, 93.52%, and 97.57%, respectively, indicating that the increase of pretreatment temperature was in favor of cellulose conversion. Earlier literature showed that the cellulose conversion of pretreated poplars with DES was significantly increased with the increase of pretreatment temperature (Yang et al., 2021). Due to the decreased pretreatment yield with increased pretreatment temperature, the glucose yield was increased first and then decreased (41.75%, 46.25%, and 44.03%).
Scheme No 13, 15, and 14 were pretreated with the same DES to E ratio and temperature, but with various time (0.5, 1, and 1.5 h). Results showed that the pretreatment yield was decreased with extension of time (56.91%, 49.45%, and 46.25%). After pretreatment with DES-E, the ether linkage in lignin carbohydrate complex (LCC) was broken (Li et al., 2022). With the extension of pretreatment time, more surfaces and pores were exposed for cellulase, thus increasing the cellulose digestibility (64.61%, 93.52%, and 94.27%). Taking pretreatment yield into consideration, glucose yield was first increased and then decreased with extended time (36.77%, 46.25%, and 43.57%).
The highest glucose yield (46.25%) was in trials No 15, which was 130.67% higher than that of control. Figure 2 was the response surface curves based on the interaction between mol ratio of DES to E, temperature and time, taking glucose yield as the response value.
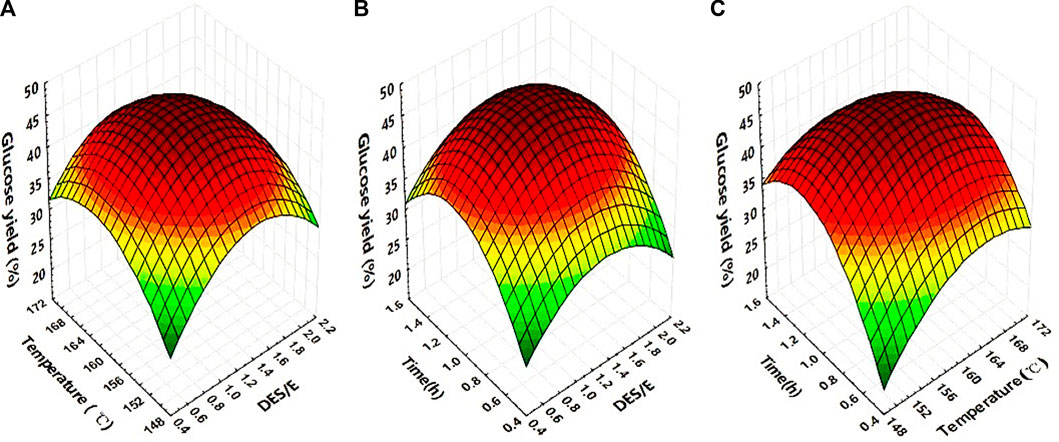
FIGURE 2. Response surface curve representing the interactive effect of DES to E ratio, temperature and time on digestibility of Broussonetia papyrifera: effect of temperature and DES to E ratio (A). Effect of DES to E ratio and time (B). Effect of temperature and time (C).
Table 2 was the variance analysis of the glucose yield of the three studied factors. From the results, it was obvious to see that the regression of this model was extremely significant (p < 0.0001). The determination coefficient R2 was 0.9830, indicating that the linear relationship between the dependent variable and the independent variable was significant. Comparing the value of F, the order of factors affecting the glucose yield was: time > DES to E ratio > temperature. The results also showed that the DES to E ratio, temperature and time, as well as the interaction of the three factors, had a very significant effect on the glucose yield (p < 0.01).
3.2 Component analysis of Broussonetia papyrifera after pretreatment
The glucose yield is closely related to the composition of biomass. The influence of different pretreatment conditions on chemical composition of Broussonetia papyrifera was shown in Table 3. The content of cellulose, xylan and lignin in untreated raw materials was 36.31%, 23.89%, and 19.99%, respectively. After pretreatment, the cellulose content was increased and that of xylan and lignin was decreased at various extent. This may be because during the pretreatment of DES-E, the chemical linkages between hemicellulose and lignin was cleaved, resulting in removal of hemicellulose and lignin, retaining most of the cellulose, which is consistent with the previous researches (Chen et al., 2019; Li et al., 2022).
Trials No. 9, 15, and 10 were conducted with the same pretreatment temperature and time, the molar ratio of DES to E was 1/2, 1/1 and 2/1, respectively. The removal of cellulose (9.98%, 15.82%, and 16.75%), xylan (85.7%, 86.71%, and 89.76%) and lignin (76.15%, 85.83%, and 88.69%) was increased with the increase of DES to E ratio. DES promotes the cleavage of C-O bond, which favors the dissolution of lignin. However, during pretreatment lignin might re-polymerize with polysaccharide degradation products (such as furfural) to produce pseudo lignin (Li and Zheng, 2017). DES (ChCl/OA/EG) was synthesized to pretreat birch, in which the degree of lignin condensation increased with the increased OA amount (Liu et al., 2021). Therefore, when the DES content increased, the lactic acid content increased accordingly, resulting in enhanced repolymerization of lignin, which made the removal rate of lignin decreased.
Broussonetia papyrifera were pretreated at 150°C, 160°C, and 170°C with the same DES to E ratio and time in schemes 11, 15, and 12. The removal of cellulose (14.61%, 14.61%, and 17.76%), xylan (62.22%, 86.71%, and 89.81%) and lignin (53.09%, 85.83%, and 92.25%) were increased with higher pretreatment temperature. The results indicated that high temperature was in favor for breaking the chemical linkage between cellulose, hemicellulose and lignin, thus the removal of hemicellulose and lignin. However, earlier research showed that pseudo lignin could form under high temperature, which leads to rising in lignin content and a decrease in lignin removal rate (Li et al., 2022).
Schemes 13, 15, and 14 were conducted with the same DES to E ratio and temperature for 0.5, 1, and 1.5 h. The removal of cellulose (15.08%, 15.82%, and 18.35%), xylan (77.13%, 86.71%, and 90.92%) and lignin (67.32%, 85.83%, and 90.42%) were all increased with the extension of time. Earlier study also showed that solubility of pretreated beech by multiple groups of DES was increased with the extension of pretreatment time (Mamilla et al., 2019).
In conclusion, when the DES to E ratio, temperature and time increased independently, the removal of cellulose, hemicellulose and lignin increased. The removal of cellulose was much less than that of hemicellulose and lignin. When the DES to E ratio, temperature and time were increased simultaneously, the changes of chemical composition were the most significant. Pretreatment condition of Scheme 8 was conducted at DES to E of 2:1, 170°C for 1.5 h. The cellulose content in the solid residue was 74.10% and 0.44% xylan and 2 .88% lignin. The cellulose removal of this scheme was relatively high (22.37%), which was not in favor of higher glucose yield.
3.3 Factors affecting cellulose conversion of Broussonetia papyrifera
From the component analysis, it can be observed that DES-E has a strong ability in biomass fractionation. In order to determine whether the improvement of cellulose digestibility is due to the removal of xylan and lignin during pretreatment, the correlation between their removal and cellulose digestibility were evaluated, as shown in Figure 3. It was observed that xylan removal (R2 = 0.66, Figure 3A) and lignin removal (R2 = 0.74, Figure 3B) showed great impact on enzyme digestibility of pretreated Broussonetia papyrifera. In addition, linear correlation between lignin removal and xylan removal was also found (R2 = 0.94, Figure 3C). The results indicated that the removal of xylan and lignin had a positive effect on cellulose conversion to glucose. The removal of lignin exhibited a more obvious effect than that of xylan. Earlier studies showed that removal of xylan can improve the accessibility of enzyme to cellulose surface, thus promoting cellulase hydrolysis (Martínez et al., 2015). In addition, our previous research results showed that there was a non-productive binding between lignin and cellulase. Removal of lignin could make more free cellulase for cellulose degradation (Yao et al., 2022). Bamboo residues were pretreated with DES by different molar ratios of choline chloride/lactic acid by Lin and his coworkers (Lin et al., 2020). The results showed that the xylan and lignin removal were positively correlated with the cellulose degradation, which was consistent with our research results.
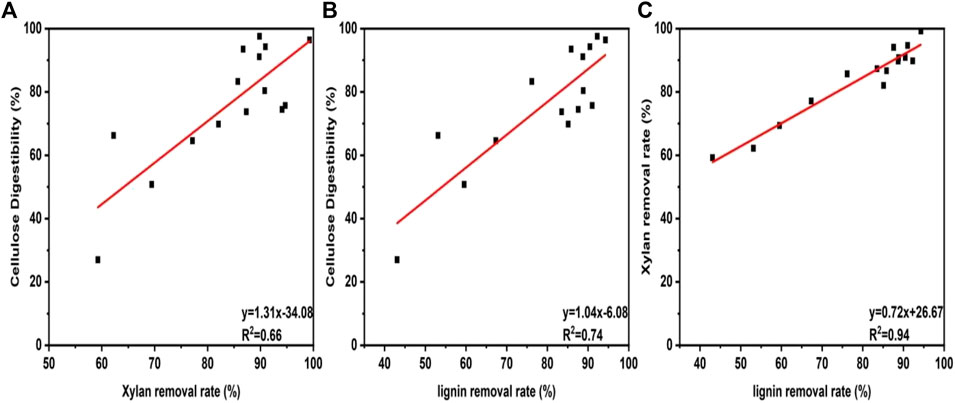
FIGURE 3. Relationship between xylan removal rate and cellulose digestibility (A). Relationship lignin removal rate and cellulose digestibility (B). Relationship between lignin removal rate and xylan removal rate (C).
3.4 Physical property changes after pretreatment
The SEM images of the samples before and after pretreatment by DES-E at were shown in Figure 4. SEM images of untreated Broussonetia papyrifera showed a compact and dense cell wall with complete surface (Figure 4A). However, it can be observed that the structure was loose and rough after DES-E pretreatment. In addition, cracks were observed on the pretreated samples obviously (Figure 4B). The chemical linkages between cellulose, hemicellulose and lignin was destroyed during DES-E pretreatment, removing a large amount of xylan and lignin, leaving residue rich in cellulose (Li et al., 2022). Therefore, due to its rough fiber surface after pretreatment with DES-E, the accessibility of cellulose was improved, thus promoting cellulose digestibility. SEM image analysis after DES pretreatment of beech and corn strover showed that the surface is rougher and looser (Zhao et al., 2018; Mamilla et al., 2019), which was also found in the present study.
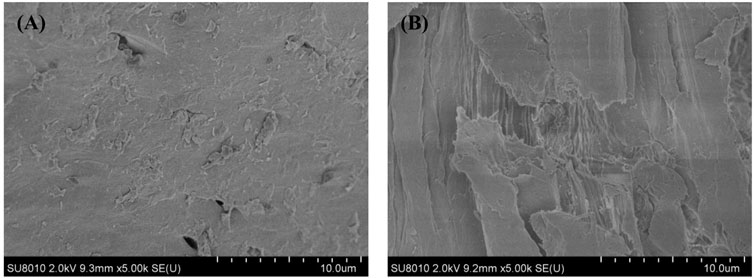
FIGURE 4. SEM images of Broussonetia papyrifera before pretreatment (A). After pretreatment at the best conditions (B).
The average pore size and specific surface area are the two most commonly applied parameters to evaluate the pore structure of porous materials (Maloney and Paulapuro, 1999). SEM analysis showed that DES-E pretreatment had a certain impact on the fiber structure. To further explore the impact, average pore size and specific surface area changes after pretreatment was determined, as shown in Figure 5 and Table 4.
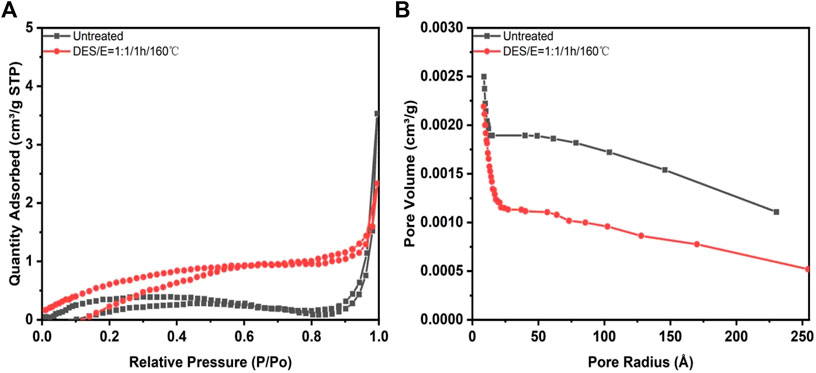
FIGURE 5. Nitrogen adsorption desorption isotherm curve (A). And BJH pore size distribution diagram (B). Of Broussonetia papyrifera before and after pretreatment.
It was showed in Figure 5A that both Broussonetia papyrifera was non-porous structured polymer before and after pretreatment (Altwala and Mokaya, 2020). When the relative pressure is less than 0.3, the nitrogen adsorption capacity of Broussonetia papyrifera after pretreatment was higher than that of the untreated one, indicating that the surface area was increased after pretreatment. Porous materials were classified into three categories according to the pore size: micropores (radius < 2 nm), mesopore (radius <50 nm); macropore (radius >50 nm). It can be seen from Figure 5B that the wood fibers before and after pretreatment showed obvious double pore distribution characteristics. After pretreatment, more microporous structures were formed, which is beneficial to the cellulase hydrolysis. Results in Table 4 indicated that the pore volume after pretreatment was relatively reduced, but the BET surface area and surface area density were increased. BET surface area was increased from 1.5987 to 2.6691 m2/g after pretreatment. In an early study, bamboo was pretreated with DES composed by choline chloride, oxalic acid and ethylene glycol. After pretreatment, the surface area was increased significantly (Li et al., 2022). The increase of specific surface area can improve the accessibility of cellulose, so as to achieve high cellulose digestibility and promote the increase of glucose yield.
3.5 Physiochemical characteristic analysis of recovered lignin
FTIR is a widely employed method to analysis different functional groups of lignin. A comparison between recovered lignin at different temperatures showed that the common property and signals in the fingerprint region of the three lignin samples were quite similar. The assignment of important signals in FTIR was referred to previous studies (Yang et al., 2016; 2020). As shown in Figure 6, signals at 3424 cm−1 were attributed to the stretching vibration of hydroxyl group (O-H) in lignin. The absorption bands at 2938 cm−1 was assigned to the C-H stretching. Signals centered at 1731 cm−1 was from the carbonyl stretching not conjugated with the aromatic ring. Strong signals at 1598, 1509, and 1423 cm−1 corresponding to aromatic rings were clearly observed in all lignin samples. Furthermore, crosspeak at 1365cm−1 was corresponded to syringyl and condensed guaiacyl units. Signals at 1226cm−1 was assigned to the C-O absorption of guaiacyl based units. Therefore, the recovered lignin from Broussonetia papyrifera pretreated by DES-E was composed by guaiacyl and syringyl.
The ratio of relative signal intensity of different functional groups to that at 1509 cm−1 was shown in Table 5 (Yao et al., 2018b). By comparing the relative signal intensity of the three recovered lignin, it can be seen that signal from carbonyl not conjugated with the aromatic ring centered at 1731 cm−1 was not found in recovered lignin at 160°C, indicating that C=O in the lignin structure was destroyed at higher pretreatment temperature. The intensity of signals at 1051 cm−1 was the strongest in recovered lignin at 150°C. The signal peaks at 1126 and 1114 cm−1 were corresponded to guaiacyl-type and syringyl-type lignin, respectively. Comparing the three lignin samples, the S/G of 140°C- lignin was significantly higher than that of the other two lignin samples.
The molecular weight analysis could elucidate the variations in depolymerization/repolymerization reactions of lignin during pretreatment. GPC method was employed to determine and compare the weight average molecular weight (Mw), number average molecular weight (Mn) and polydispersity index (PDI) of each lignin sample. As shown in Table 6, Mn of lignin sample recovered in pretreatment liquid decreased with the increase of temperature, which was consistent with the previous research results (Yao et al., 2020a). Mw was increased with the increase of temperature, indicating of enhanced lignin repolymerization occurring with increased temperature during acid-catalyzed pretreatment process (Yao et al., 2018). Earlier studies showed that the extensive repolymerization reactions took over with increasing temperature, forming larger and heterogeneous lignin macromolecules (Wang et al., 2012), which could explain the increased Mw and PDI with the increased pretreatment temperature in the present study.
The results in Table 6 showed that the phenolic hydroxyl content in the recovered lignin increased with the increase of temperature. This may be due to the broken of α, β-ether linkage during pretreatment, resulting in an increase in phenolic hydroxyl group (Wang et al., 2020). When the pretreatment temperature was at 160°C, the content of phenolic hydroxyl group content was 0.40 mmol/g, as twice as that at 140°C. The result of -OH group content determined by FT-IR and UV was contradictory. The possible reason was that UV method was employed to determine the phenolic hydroxyl group, while in the FTIR, the signal around 3400 cm-1 was assigned to total hydroxyl group. The least signal intensity of lignin recovered at pretreatment temperature of 160°C might be caused by less content of aliphatic -OH group (Yao et al., 2020). Earlier studies showed that with the increase of temperature, the content of phenolic hydroxyl group of lignin obtained by hydrothermal pretreatment increased by 53.79% (Chen et al., 2016), which was consistent with our research results. More phenolic OH groups is a desired feature for potentially application of lignin as antioxidant, as reported by other researches previously (Sadeghifar et al., 2017; Yao et al., 2018a).
3.6 Antioxidant activity determination of recovered lignin
Lignin is a natural aromatic polymer composed of three phenylpropane structural units (p-hydroxyphenyl (H), guaiacyl (G) and syringal (S)) connected by various ether bonds and C-C bonds (Sadeghifar and Argyropoulos, 2015). As a compound rich in active functional groups, lignin can capture and neutralize free radicals and shows good antioxidant activity (Lu et al., 2022). In the present study, the in vitro antioxidant activity of lignin recovered from the DES-E pretreatment liquid was determined by DPPH method as described earlier (Sadeghifar et al., 2017). The DPPH radical scavenging rates of lignin extracted at different temperatures and water-soluble vitamin E were shown in Figure 7. In the range of 0–50 mg/L, with the increase of lignin concentration, the free radical scavenging capacity of recovered lignin and water-soluble vitamin E was enhanced, and the concentration of lignin is linearly correlated with the free radical scavenging rate (R2 ≥ 0.95). The IC50 and antioxidant activity index (AAI) were shown in Table 7. IC50 represents the concentration of antioxidant required to inhibit 50% free radicals under study. The lower IC50 indicates the stronger antioxidant activity. The results showed that compared with vitamin E, the antioxidant activity of recovered lignin pretreated by DES-E was higher. More importantly, the IC50 of these lignin samples was lower than most of published studies (An et al., 2017; Yao et al., 2018b; Dong et al., 2020), indicating advantage of DES-E pretreatment in producing lignin with higher antioxidant activity.
With the increase of pretreatment temperature, the broken of ether linkage and C-C bond was increased (Li et al., 2022), and the content of phenolic hydroxyl group was increased, which favored the capture of free radicals by lignin. The antioxidant properties of industrial lignin from different sources was studied earlier, and it was found that the phenolic hydroxyl group content in lignin structure was positively correlated with AAI (y = 0.797x + 0.99, R2 = 0.978), and the phenolic hydroxyl in lignin played a crucial role in the antioxidant properties (Sadeghifar et al., 2017). The antioxidant activity of lignin sample is mainly from their ability to donate H and quench the free radical intermediate, which is primarily from free phenolic hydroxyl groups. That could explain the importance of the phenolic hydroxyl groups in the antioxidant capability of lignin sample. In another research, it was indicated that the carbonyl group in the side chain inhibited the free radical scavenging activity (Dizhbite et al., 2004). Combined with the FTIR analysis results, there was no obvious signal from C=O in recovered lignin at 160°C, which led to the improvement of its antioxidant capacity. Furthermore, the S/G of the three lignin samples were 1.52, 1.18 and 1.27, respectively, based on the FTIR analysis. It was indicated before that AL with a higher S/G ratio had stronger antioxidant activities (Gong et al., 2016), which was not observed in the present study. However, it was found that lignin with higher Mw and PDI showed higher antioxidant ability, which was contradictory with previous study (Yao et al., 2018b). It might due to limited data in this study. The impact of molecular weight and distribution of lignin on its antioxidant capacity needs further investigation.
4 Conclusion
Broussonetia papyrifera was pretreated with DES-E to improve the glucose yield and obtain the recovered lignin for further application. When the ratio of DES to ethanol was 1:1, the pretreatment temperature was 160°C, and time was 1h, the highest glucose yield was 46.25%. The physical and chemical properties of the pretreated solid residue and dissolved lignin were analyzed. It was found that compared with the untreated one, the content of lignin and hemicellulose decreased significantly. The specific surface area was also increased after pretreatment. With the increase of temperature, the content of phenolic hydroxyl of recovered lignin was increased, which was beneficial to the antioxidant capacity.
Data availability statement
The original contributions presented in the study are included in the article/Supplementary Material, further inquiries can be directed to the corresponding authors.
Author contributions
PC: writing, validation. ZY: formal analysis. MC: review and editing. JY: review and editing. YX: review and editing. HY: review and editing, funding acquisition. LY: review and editing, investigation.
Funding
The authors are grateful for the support from the National Natural Science Foundation of China (No. 21978074 and 31871789), the China Scholarship Council (No. 2011842330 and 201508420257), key project of Hubei Provincial Department of Education (D20211404), and key Laboratory of Fermentation Engineering (Ministry of Education) (No. 202105FE04).
Conflict of interest
The authors declare that the research was conducted in the absence of any commercial or financial relationships that could be construed as a potential conflict of interest.
Publisher’s note
All claims expressed in this article are solely those of the authors and do not necessarily represent those of their affiliated organizations, or those of the publisher, the editors and the reviewers. Any product that may be evaluated in this article, or claim that may be made by its manufacturer, is not guaranteed or endorsed by the publisher.
References
Altwala, A., and Mokaya, R. (2020). Predictable and targeted activation of biomass to carbons with high surface area density and enhanced methane storage capacity. Energy Environ. Sci 13, 2967–2978. doi:10.1039/d0ee01340d
An, L., Wang, G., Jia, H., Liu, C., Sui, W., and Si, C. (2017). Fractionation of enzymatic hydrolysis lignin by sequential extraction for enhancing antioxidant performance. Int. J. Biol Macromol 99, 674–681. doi:10.1016/j.ijbiomac.2017.03.015
Bjelic, A., Hočevar, B., Grilc, M., Novak, U., and Likozar, B. (2022). A review of sustainable lignocellulose biorefining applying (natural) deep eutectic solvents (DESs) for separations, catalysis and enzymatic biotransformation processes. Rev. Chem. Eng 38, 243–272. doi:10.1515/revce-2019-0077
Bu, C. Y., Yan, Y. X., Zou, L. H., Ouyang, S. P., Zheng, Z. J., and Ouyang, J. (2021). Comprehensive utilization of corncob for furfuryl alcohol production by chemo-enzymatic sequential catalysis in a biphasic system. Bioresour. Technol 319, 124156. doi:10.1016/j.biortech.2020.124156
Chen, X., Li, H., Sun, S., Cao, X., and Sun, R. (2016). Effect of hydrothermal pretreatment on the structural changes of alkaline ethanol lignin from wheat straw. Sci. Rep 6, 39354–39359. doi:10.1038/srep39354
Chen, Z., Jacoby, W. A., and Wan, C. (2019). Ternary deep eutectic solvents for effective biomass deconstruction at high solids and low enzyme loadings. Bioresour. Technol 279, 281–286. doi:10.1016/j.biortech.2019.01.126
Dizhbite, T., Telysheva, G., Jurkjane, V., and Viesturs, U. (2004). Characterization of the radical scavenging activity of lignins - natural antioxidants. Bioresour. Technol 95, 309–317. doi:10.1016/j.biortech.2004.02.024
Dong, H., Zheng, L., Yu, P., Jiang, Q., Wu, Y., and Huang, C., (2020). Characterization and application of lignin-carbohydrate complexes from lignocellulosic materials as antioxidants for scavenging in vitro and in vivo reactive oxygen species. ACS Sustain. Chem. Eng 8, 256–266. doi:10.1021/acssuschemeng.9b05290
Gong, W., Xiang, Z., Ye, F., and Zhao, G. (2016). Composition and structure of an antioxidant acetic acid lignin isolated from shoot shell of bamboo (Dendrocalamus Latiforus). Ind. Crops Prod. 91, 340–349. doi:10.1016/j.indcrop.2016.07.023
Gontrani, L., Plechkova, N. V., and Bonomo, M. (2019). In-depth physico-chemical and structural investigation of a dicarboxylic acid/choline chloride natural deep eutectic solvent (nades): A spotlight on the importance of a rigorous preparation procedure. ACS Sustain. Chem. Eng 7, 9b02402–12543. doi:10.1021/acssuschemeng.9b02402
Hou, S., Shen, B., Zhang, D., Li, R., Xu, X., Wang, K., et al. (2022). Understanding of promoting enzymatic hydrolysis of combined hydrothermal and deep eutectic solvent pretreated poplars by Tween 80. Bioresour. Technol. 362, 127825. doi:10.1016/j.biortech.2022.127825
Hou, X. D., Feng, G. J., Ye, M., Huang, C. M., and Zhang, Y. (2017). Significantly enhanced enzymatic hydrolysis of rice straw via a high-performance two-stage deep eutectic solvents synergistic pretreatment. Bioresour. Technol 238, 139–146. doi:10.1016/j.biortech.2017.04.027
Kumar, R., Hu, F., Hubbell, C. A., Ragauskas, A. J., and Wyman, C. E. (2013). Comparison of laboratory delignification methods, their selectivity, and impacts on physiochemical characteristics of cellulosic biomass. Bioresour. Technol 130, 372–381. doi:10.1016/j.biortech.2012.12.028
Li, N., Meng, F., Yang, H., Shi, Z., Zhao, P., and Yang, J. (2022). Enhancing enzymatic digestibility of bamboo residues using a three-constituent deep eutectic solvent pretreatment. Bioresour. Technol 346, 126639. doi:10.1016/j.biortech.2021.126639
Li, X., and Zheng, Y. (2017). Lignin-enzyme interaction: Mechanism, mitigation approach, modeling, and research prospects. Biotechnol. Adv 35, 466–489. doi:10.1016/j.biotechadv.2017.03.010
Lin, W., Xing, S., Jin, Y., Lu, X., Huang, C., and Yong, Q. (2020). Insight into understanding the performance of deep eutectic solvent pretreatment on improving enzymatic digestibility of bamboo residues. Bioresour. Technol 306, 123163. doi:10.1016/j.biortech.2020.123163
Liu, Y., Deak, N., Wang, Z., Yu, H., Hameleers, L., and Jurak, E., (2021). Tunable and functional deep eutectic solvents for lignocellulose valorization. Nat. Commun 12, 5424. doi:10.1038/s41467-021-25117-1
Lu, X., Gu, X., and Shi, Y. (2022). A review on lignin antioxidants: Their sources, isolations, antioxidant activities and various applications. Int. J. Biol. Macromol 210, 716–741. doi:10.1016/j.ijbiomac.2022.04.228
Maloney, T. C., and Paulapuro, H. (1999). The formation of pores in the cell wall. J. Pulp Pap. Sci 25, 430–436.
Mamilla, J. L. K., Novak, U., Grilc, M., and Likozar, B. (2019). Natural deep eutectic solvents (DES) for fractionation of waste lignocellulosic biomass and its cascade conversion to value-added bio-based chemicals. Biomass Bioenerg. 120, 417–425. doi:10.1016/j.biombioe.2018.12.002
Martínez, P. M., Bakker, R., Harmsen, P., Gruppen, H., and Kabel, M. (2015). Importance of acid or alkali concentration on the removal of xylan and lignin for enzymatic cellulose hydrolysis. Ind. Crops Prod 64, 88–96. doi:10.1016/j.indcrop.2014.10.031
Pandey, A., BhawnaDhingra, D., and Pandey, S. (2017). Hydrogen bond donor/acceptor cosolvent-modified choline chloride-based deep eutectic solvents. J. Phys. Chem. B 16, 4202–4212. doi:10.1021/acs.jpcb.7b01724
Peng, J., Xu, H., Wang, W., Kong, Y., Su, Z., and Li, B. (2021). Techno-economic analysis of bioethanol preparation process via deep eutectic solvent pretreatment. Ind. Crops Prod 172, 114036. doi:10.1016/j.indcrop.2021.114036
Penín, L., López, M., Santos, V., Alonso, J. L., and Parajó, J. C. (2020). Technologies for Eucalyptus wood processing in the scope of biorefineries: A comprehensive review. Bioresour. Technol 311, 123528. doi:10.1016/j.biortech.2020.123528
Sadeghifar, H., and Argyropoulos, D. S. (2015). Correlations of the antioxidant properties of softwood kraft lignin fractions with the thermal stability of its blends with polyethylene. ACS Sustain. Chem. Eng 3, 349–356. doi:10.1021/sc500756n
Sadeghifar, H., Wells, T., Le, R. K., Sadeghifar, F., Yuan, J. S., and Ragauskas, A. J. (2017). Fractionation of organosolv lignin using acetone:water and properties of the obtained fractions. ACS Sustain. Chem. Eng 5, 580–587. doi:10.1021/acssuschemeng.6b01955
Shen, B., Hou, S., Jia, Y., Yang, C., Su, Y., and Ling, Z., (2021). Synergistic effects of hydrothermal and deep eutectic solvent pretreatment on co-production of xylo-oligosaccharides and enzymatic hydrolysis of poplar. Bioresour. Technol 341, 125787. doi:10.1016/j.biortech.2021.125787
Shen, X. J., Wen, J. L., Mei, Q. Q., Chen, X., Sun, D., and Yuan, T. Q., (2019). Facile fractionation of lignocelluloses by biomass-derived deep eutectic solvent (DES) pretreatment for cellulose enzymatic hydrolysis and lignin valorization. Green Chem 21, 275–283. doi:10.1039/c8gc03064b
Tan, Y. T., Ngoh, G. C., and Chua, A. S. M. (2019). Effect of functional groups in acid constituent of deep eutectic solvent for extraction of reactive lignin. Bioresour. Technol 281, 359–366. doi:10.1016/j.biortech.2019.02.010
Thi, S., and Lee, K. M. (2019). Comparison of deep eutectic solvents (DES) on pretreatment of oil palm empty fruit bunch (OPEFB): Cellulose digestibility, structural and morphology changes. Bioresour. Technol 282, 525–529. doi:10.1016/j.biortech.2019.03.065
Wang, K., Gao, S., Lai, C., Xie, Y., Sun, Y., Wang, J., et al. (2022). Upgrading wood biorefinery: An integration strategy for sugar production and reactive lignin preparation. Ind. Crops Prod. 187, 115366. doi:10.1016/j.indcrop.2022.115366
Wang, K., Yang, H., Yao, X., Xu, F., and Sun, R. C. (2012). Structural transformation of hemicelluloses and lignin from triploid poplar during acid-pretreatment based biorefinery process. Bioresour. Technol 116, 99–106. doi:10.1016/j.biortech.2012.04.028
Wang, S., Li, H., Xiao, L. P., and Song, G. (2020). Unraveling the structural transformation of wood lignin during deep eutectic solvent treatment. Front. Energy Res 8. doi:10.3389/fenrg.2020.00048
Wang, W., Zhu, B., Xu, Y., Li, B., and Xu, H. (2022). Mechanism study of ternary deep eutectic solvents with protonic acid for lignin fractionation. Bioresour. Technol 363, 127887. doi:10.1016/j.biortech.2022.127887
Wang, Y., Zhang, W. J., Yang, J. Y., Li, M. F., Peng, F., and Bian, J. (2022). Efficient fractionation of woody biomass hemicelluloses using cholinium amino acids-based deep eutectic solvents and their aqueous mixtures. Bioresour. Technol 354, 127139. doi:10.1016/j.biortech.2022.127139
Xia, Q., Liu, Y., Meng, J., Cheng, W., Chen, W., Liu, S., et al. (2018). Multiple hydrogen bond coordination in three-constituent deep eutectic solvents enhances lignin fractionation from biomass. Green Chem 20, 2711–2721. doi:10.1039/c8gc00900g
Xu, G. C., Ding, J. C., Han, R. Z., Dong, J. J., and Ni, Y. (2016). Enhancing cellulose accessibility of corn stover by deep eutectic solvent pretreatment for butanol fermentation. Bioresour. Technol 203, 364–369. doi:10.1016/j.biortech.2015.11.002
Xu, H., Peng, J., Kong, Y., Liu, Y., Su, Z., Li, B., et al. (2020). Key process parameters for deep eutectic solvents pretreatment of lignocellulosic biomass materials: A review. Bioresour. Technol. 310, 123416. doi:10.1016/j.biortech.2020.123416
Yang, H., Xie, Y., Zheng, X., Pu, Y., Huang, F., Meng, X., et al. (2016). Comparative study of lignin characteristics from wheat straw obtained by soda-AQ and kraft pretreatment and effect on the following enzymatic hydrolysis process. Bioresour. Technol 207, 361–369. doi:10.1016/j.biortech.2016.01.123
Yang, H., Yoo, C. G., Meng, X., Pu, Y., Muchero, W., Tuskan, G. A., et al. (2020). Structural changes of lignins in natural Populus variants during different pretreatments. Bioresour. Technol 295, 122240. doi:10.1016/j.biortech.2019.122240
Yang, J., Wang, Y., Zhang, W., Li, M., Peng, F., and Bian, J. (2021). Alkaline deep eutectic solvents as novel and effective pretreatment media for hemicellulose dissociation and enzymatic hydrolysis enhancement. Int. J. Biol. Macromol 193, 1610–1616. doi:10.1016/j.ijbiomac.2021.10.223
Yao, L., Chen, C., Yoo, C. G., Meng, X., Li, M., Pu, Y., et al. (2018a). Insights of ethanol organosolv pretreatment on lignin properties of Broussonetia papyrifera. ACS Sustain. Chem. Eng 6, 14767–14773. doi:10.1021/acssuschemeng.8b03290
Yao, L., Cui, P., Chen, X., Yoo, C. G., Liu, Q., Meng, X., et al. (2022). A combination of deep eutectic solvent and ethanol pretreatment for synergistic delignification and enhanced enzymatic hydrolysis for biorefinary process. Bioresour. Technol 350, 126885. doi:10.1016/j.biortech.2022.126885
Yao, L., Xiong, L., Yoo, C. G., Dong, C., Meng, X., Dai, J., et al. (2020). Correlations of the physicochemical properties of organosolv lignins from Broussonetia papyrifera their antioxidant activities. Sustain. Energ. Fuels. 4, 5114–5119. doi:10.1039/d0se00940g
Yao, L., Yoo, C. G., Meng, X., Li, M., Pu, Y., Ragauskas, A. J., et al. (2018b). A structured understanding of cellobiohydrolase Ⅰ binding to poplar lignin fractions after dilute acid pretreatment. Biotechnol. Biofuels. 11, 96. doi:10.1186/s13068-018-1087-y
Yu, D., Xue, Z., and Mu, T. (2021). Eutectics: Formation, properties, and applications. Chem. Soc. Rev 50, 8596–8638. doi:10.1039/d1cs00404b
Zhang, C. W., Xia, S. Q., and Ma, P. S. (2016). Facile pretreatment of lignocellulosic biomass using deep eutectic solvents. Bioresour. Technol 219, 1–5. doi:10.1016/j.biortech.2016.07.026
Zhao, Z., Chen, X., Ali, M. F., Abdeltawab, A. A., Yakout, S. M., and Yu, G. (2018). Pretreatment of wheat straw using basic ethanolamine-based deep eutectic solvents for improving enzymatic hydrolysis. Bioresour. Technol 263, 325–333. doi:10.1016/j.biortech.2018.05.016
Keywords: DES, pretreatment, enzymatic saccharification, lignin, antioxidant
Citation: Cui P, Ye Z, Chai M, Yuan J, Xiong Y, Yang H and Yao L (2023) Effective fractionation of lignocellulose components and lignin valorization by combination of deep eutectic solvent with ethanol. Front. Bioeng. Biotechnol. 10:1115469. doi: 10.3389/fbioe.2022.1115469
Received: 04 December 2022; Accepted: 19 December 2022;
Published: 09 January 2023.
Edited by:
Chen Huang, Chinese Academy of Forestry, ChinaReviewed by:
Chenhuan Lai, Nanjing Forestry University, ChinaYanzhu Guo, Dalian Polytechnic University, China
Copyright © 2023 Cui, Ye, Chai, Yuan, Xiong, Yang and Yao. This is an open-access article distributed under the terms of the Creative Commons Attribution License (CC BY). The use, distribution or reproduction in other forums is permitted, provided the original author(s) and the copyright owner(s) are credited and that the original publication in this journal is cited, in accordance with accepted academic practice. No use, distribution or reproduction is permitted which does not comply with these terms.
*Correspondence: Haitao Yang, cHBodHlhbmcxOTc5QGFsaXl1bi5jb20=; Lan Yao, eWFvbGlzbGFuMTk4MkBhbGl5dW4uY29t
†These authors have contributed equally to this work