- Department of Prosthodontics, School and Hospital of Stomatology, Jilin University, Changchun, China
Objective: This study aimed to evaluate the role of collagen cross-linkers in the bonding performance of the resin-dentin interface through a systematic review and a network meta-analysis.
Sources: The literature search was conducted in several databases like PubMed, EMBASE, Cochrane, Scopus and Web of Science from their inception till 30 April 2022.
Study selection: The inclusion criteria consisted of in vitro studies evaluating the micro-tensile and micro-shear bond strengths of different cross-linkers acting on dentin. Bayesian network meta-analysis was conducted using RStudio.
Data: Out of the 294 studies evaluated in the full-text analysis, 40 were included in the systematic review and meta-analysis. Most studies have used cross-linkers as primer (65.1%), followed by incorporating them into in adhesives and acid etching agents. The application methods of the adhesive system were classified as “etch-and-rinse (ER) adhesives” (77%) and “self-etching (SE) adhesives”. Moreover, there were six types of cross-linkers in this presented review, of which the most numerous were polyphenols.
Conclusion: Different application methods of cross-linkers, the long-term results showed that were only effective when used for longer durations, the immediate results were not statistically different. According to immediate and long-term results, etch-and-rinse (ER) adhesives showed a greater bonding performance than the control groups (p ≤ 0.05), whereas self-etching (SE) adhesives showed similar bond strength values (p ≥ 0.05). The result of network meta-analysis (NMA) showed that Dope like compound showed higher long-term bonding performance than other cross-linkers.
Clinical significance: Long-term clinical studies may be needed to determine the effect of the cross-linkers on the bonding properties.
Introduction
Precise adhesion between resin composites and dentin substrate requires the infiltration of resin monomers into the demineralized dentin matrix after the partial dissolution of the mineralized layer (Matuda et al., 2016). Recent advancements in adhesive bonding techniques have resulted in the use of resin composite materials for dentin substrates, which includes collagen fibers, non-collagenous proteins, and carbonate apatite. The resin composite materials used in restorative procedures are made up of collagen, non-collagen and carbonate apatite. To support the tissues, the C-terminal and globular N-terminal propeptidesand non-separable terminal peptides of collagen accommodate hydroxyapatite crystals (Tjäderhane et al., 2013). Moreover, the infiltration of adhesive monomers to demineralized dentin creates a hybrid layer (HL), which remains the weakest region of adhesively-based restorations (Comba et al., 2020).
The goal of excellent adhesion is to achieve an effective resin-dentin interface, that is, stable, and provides good retention, marginal integrity, and clinical durability (Tjäderhane, 2015). Despite these advances, HL created on the variable organic dentin phase is imperfect and may degrade over time, leading to marginal discoloration, nanoleakage, and decreased composite retention (Mjör et al., 2002; Maravic et al., 2017). Several in vitro and in vivo studies have tried to elucidate the potential causes resin-dentin adhesion progression. Therefore, the most likely contributors to interfacial degradation are a hydrolytic breakdown of the polymerized resin compounds and endogenous protease-initiated degradation of the demineralized dentin collagen matrix (Hashimoto et al., 2000; Armstrong et al., 2004; Mazzoni et al., 2018; Comba et al., 2019). However, 1 year of water storage resulted in a significant fall in bond strength of 31%–70% (Hashimoto et al., 2000; Mazzoni et al., 2013a). Therefore, different strategies have been proposed to minimize the degradation of HL over time, such as enhancing collagen fibrils within HL, inactivating endogenous enzymes, or combining the two strategies (Breschi et al., 2008; Mazzoni et al., 2013b; Bedran-Russo et al., 2014).
Cross-linking of dentin matrix collagen is a naturally occurring mechanism in dentin that provides tensile strength (Bedran-Russo et al., 2008; Bedran-Russo et al., 2014). Hence, collagen cross-linkers were introduced as an alternative dentin pre-treatment to improve the durability of dentin-resin bonds (Fawzy et al., 2012; Fawzy et al., 2013). Furthermore, they enhance the collagen fibrils network by inducing intra and intermolecular cross-linking by several mechanisms. Firstly, cross-linking agents increase collagen structural architecture and inactivate the catalytic site of these enzymes, thus, disabling the access and posterior hydrolysis of collagenases (Hass et al., 2016a). Secondly, the use of competitive (or non-competitive) enzyme inhibitors creates an enzyme-substrate complex that prevents the hydrolysis of the collagen substrate (Baena et al., 2020; Chen et al., 2021).
Cross-linking agents, like adhesives, increase the hardness of the HL collagen matrix as improved biomechanical properties of the dentin matrix increase resin-dentin bond durability (Cai et al., 2018). A previous meta-analysis evaluating the effects of plant extracts as a primer on dentin bonding strength demonstrated an improvement in the immediate bond strength of adhesive (Zhao et al., 2022). Nevertheless, whether collagen cross-linkers can provide stable and long-lasting bonding strength to the adhesive interface is still debatable.
Therefore, this study aimed to systematically review all in vitro studies that assessed the role of collagen cross-linkers in the bonding performance of the resin-dentin interface immediately and for longer durations. The null hypothesis was that there would be no difference in bond strength values when the collagen cross-linkers were used in the bonding procedures.
Materials and methods
Registered with PROSPERO (CRD42022365877), this study was conducted following updated guidelines for the PRISMA 2020 Statement: Systematic Review Reports (Page et al., 2021); the research question was “Can collagen cross-linkers improve the bonding performance of resin-dentin interface?”
Literature search and information sources
The literature search strategy consisted of the following terms: cross-linkers, adhesives, and bond strength, as detailed in Supplementary Material S1. Two independent reviewers performed the literature search and screened five electronic databases (PubMed, EMBASE, Cochrane library, Scopus, and Web of Science) to identify relevant manuscripts that could be included. The database search was extended until 30 April 3022, because no publication year or language restrictions were used. Additionally, the reviewers also manually searched the reference lists of the collected manuscripts for additional relevant studies that met the inclusion criteria.
Inclusion criteria
Inclusion criteria were in vitro or ex vivo studies evaluating the effects of cross-linkers on dentin and in vitro studies assessing immediate and long-term bond strengths, in the experimental group and control groups treated with cross-linkers and without them, respectively. Micro-tensile and micro-shear bond strengths (MTBS and MSBS) of adhesives (unit: MPa) studies, as well as using caries-affected or sound teeth. Studies focusing on deciduous and material-based substrates (e.g., resin composites, ceramics, metals), were excluded; studies lacking substrate data were not available after at least two email requests to the authors. The summary study design (PICOS) was as follows: P, dental adhesive; I, cross-linkers; C, without cross-linkers; O, bond strength.
Study selection and data extraction
Duplicate records were removed after importing the articles into EndNoteX9 (Thomson Reuters); in case of any disagreement, a third review (SZ) was recruited to reach a consensus. Two researchers (HC and DS) extracted data independently using Microsoft Office Excel 2013 spreadsheets (Microsoft Corporation, Redmond, WA, United States) and tabulated relevant data. The following datas were extracted: study (year of publication), type of aging, dental adhesives used, cross-linkers used, and additional tests performed, such as scanning electron microscopy (SEM) evaluation. The corresponding authors were contacted via email to retrieve missing data of specific bond strength values and display results graphically or numerically or any other information.
Quality assessment
Adapted from a previous study (Montagner et al., 2014), the quality assessment was done by two investigators based on the following parameters: teeth randomization, teeth free of caries/restoration, materials used according to manufacturers’ instructions, adhesive procedures performed by a single operator, sample size calculation, and operation blinding. The study was given a “Y” if the parameter was included and performed appropriately and an “N” if the parameter was missing or inadequately performed. The number of parameters that scored “Y”, 1 or 2 indicated a high risk, 3 to 3 medium, and 5 to 6 indicated a low risk of bias. Any differences between the two investigators were resolved by interviewing the third investigator.
Statistical analysis
Meta-analyses were performed using Review Manager software version 5.3.5 (Nordic Cochrane Center, Cochrane Collaboration, Copenhagen, Denmark). The pooled effect estimates were derived using a random-effects model that compared the mean difference between bond strength values. The studies were divided into three categories based on how cross-linkers were used: (Matuda et al., 2016): applied to dentin as a pre-treatment solution that remains in contact with the surface (commonly used), (Tjäderhane et al., 2013), incorporated within the adhesive system, or (Comba et al., 2020) incorporated into the acid etching agent that is rinsed away from the surface. The adhesive system’s application methods were classified as “etch-and-rinse (ER) adhesives” and “self-etching (SE) adhesives”. It is worth noting that ER mode of universal adhesive is assigned to ER adhesives and the SE mode is assigned to SE adhesives. A comprehensive effect estimate was obtained by comparing standardized average differences between bond strength values of the experiment and the control groups. Furthermore, studies evaluating samples before and after the long-term process were analyzed separately. All p-values < 0.05 were considered statistically significant. Statistical heterogeneity of treatment effect among studies was assessed using the Cochran Q test and the inconsistent I2 test.
Bayesian network meta-analysis (NMA) was performed on bond strength data of various cross-linkers, and it was classified as 1) control; 2) aldehydes, including glutaraldehyde (GA), acrylic primer, 4-formylphenyl acrylate (FA); 3) 1-Ethyl-3-(3-dimethylaminopropyl) carbodiimide (EDC); 4) polyphenols, including grape seed extracts (main component: proanthocyanidins (PA), epigallocatechin-3-gallate (EGCG), quercetin, etc; 5) chitosan; 6) riboflavin; 7) Dope like compound such as mussel adhesive proteins (MAP) and dopamine methacrylamide (DMA). Additionally, Bayesian random effects pairwise and NMA were performed to derive pairwise, indirect and network estimates, one for immediate and the other for long-term bond strength. Separated analyses were conducted for immediate and long-term results. The JAGS program implemented in the R package gemtc 0.8–2 (Valkenhoef et al., 2012) evaluated Network plots and league tables using Bayesian random effects modeling and Markov chain Monte Carlo simulations (van Ravenzwaaij et al., 2018), with 20,000 iterations for adaptation. Bayesian random-effects NMA estimated the effect as mean difference (MD) with 95% credible intervals (95% CrI). A p-value < 0.05 was considered statistically significant. Additionally, the average ranking and cumulative ranking curves (SUCRA) were used to rank groups (Salanti et al., 2011), which were shown graphically, and the pairs or values were generated from the NMA table (Rouse et al., 2017).
Results
Search strategy
From 2,100 potentially eligible studies, 294 and 78 were selected for full-text analysis, and the systematic review, respectively (Figure 1). A total of 216 studies were not included; many studies were excluded based on the eligibility criteria (182); data not available (Mazzoni et al., 2013b); without long-term bond strength (Hass et al., 2016a), and conducted on bovine teeth (Comba et al., 2020). Finally, seven-eight studies were included in the review (Cova et al., 2011; Castellan et al., 2013; Chiang et al., 2013; Andre et al., 2015; Carvalho et al., 2016; Abunawareg et al., 2017; Bacelar-Sa et al., 2017; Albuquerque et al., 2019; Costa et al., 2019; Czech et al., 2019; Baena et al., 2020; Beck and Ilie, 2020; Comba et al., 2020; Abdelshafi et al., 2021; Baldion et al., 2021; Chen et al., 2021; Dacoreggio et al., 2021; Abd El-Aal et al., 2022; Beck and Ilie, 2022) and meta-analysis for which the primary data (mean bond strength, standard deviation, and the number of test samples) could be retrieved.
Descriptive analysis
All studies included in the review were published between 2011 and 2022. Cross-linkers were applied as a pre-treatment solution in the majority of studies (65.1%), followed by incorporation into adhesive systems (30.1%), and only four studies (4.8%) added them into the acid etching agent to evaluate bonding performance (Table 1). Concerning the resin composites used to prepare restorations, the most commonly used materials are purchased from the 3M ESPE industry (e.g., Filtek Z250, Filtek Z350, Filtek Supreme, and Filtek P60 (3M ESPE)), followed by Kulzer (Charisma), FGM (Opallis), VOCO (Grandio) and Kuraray (Clearfil A-PX), Ivoclar-Vivadent (Tetric Ceram) and Ivoclar-Vivadent (Bluephase). The majority of studies (76.9%) applied a #600-grit SiC abrasive paper at the dentin surface before applying adhesive In contrast, others used grit sizes ranging from #180-grit to #1200-grit SiC or a sequence of SiC at varying final grits.
Figure 2 depicts the adhesive systems used in this review, which were allocated according to their application strategies. Although Single Bond Universal (3M ESPE) and Adper Single Bond 2 (3M ESPE) were the most frequently used adhesives, eight kinds of universal adhesives were utilized in total: Single Bond Universal (3M ESPE), All Bond Universal (3M ESPE), Tetric N-Bond Universal (Ivoclar), Ambar Universal (FGM), Clearfil Universal (Kuraray), G-Bond (GC Corp), OptiBond-All-In-One (Kerr) and UA Zipbond (SDI), while ER adhesives included Adper Single Bond 2 (3M ESPE), Adper Single Bond Plus (3M ESPE), XP Bond Adhesive (Dentsply), Adper Scotchbond 1XT (3M ESPE), Prime and Bond Elect (Dentsply), Optibond FL (Kerr), All-Bond 3 (Bisco), One Step Plus (Bisco), Scotchbond multi-purpose Adhesive (3M ESPE), Adper Easy One (3M ESPE), Gluma Comfort Bond (Heraeus), Gluma 2Bond (Heraeus) and Optibond S (Kerr); Clearfil SE Bond (Kuraray) and G-Aenial (GC Corp) were SE adhesives. Additionally, nine studies did not use any commercial adhesives, but used adhesives configured according to the experiment.
Risk of bias
Based on the bias analysis parameters (Table 2), most of the studies had a low risk of bias in teeth free of caries/restoration (89.7%), and materials were used according to manufacturer’s instructions (89.7%). The majority of regarding teeth randomization (84.6) and sample size calculations were classified as having a medium risk of bias (84.6). Overall, the majority (88.5%) of them showed a moderate risk of bias, followed by low risk (7.7%) and high risk (3.8%). Furthermore, no studies reported blinding and only 11 (14.1%) reported single-operator adhesive procedures.
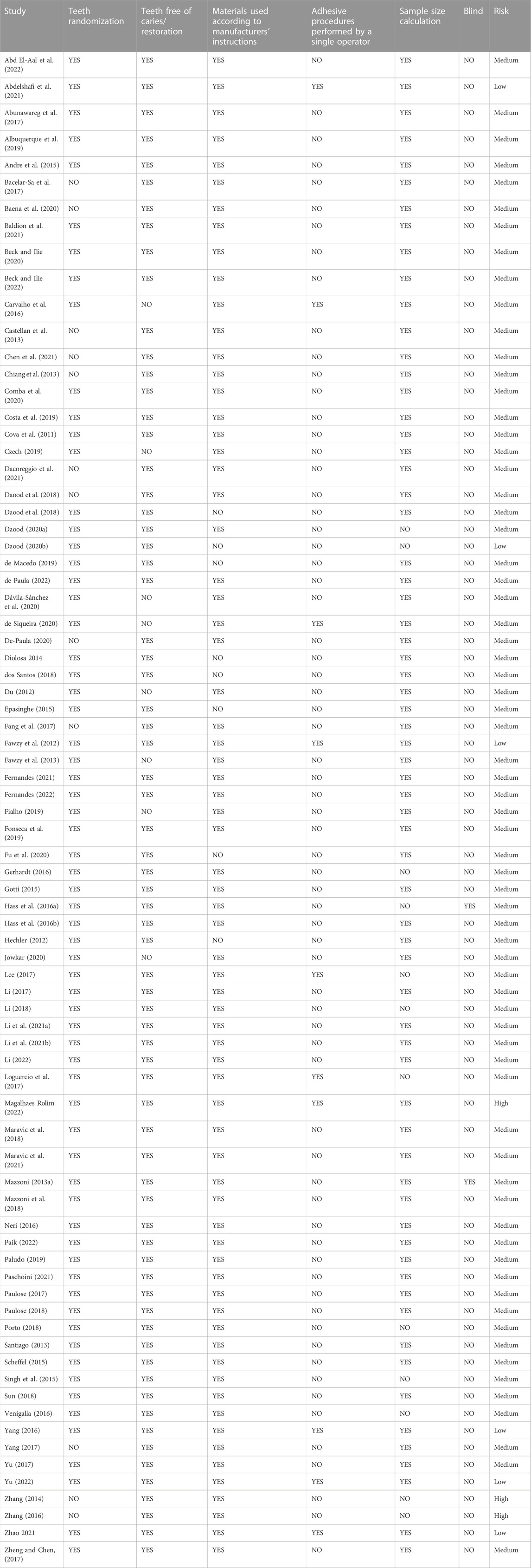
TABLE 2. Risk of bias of the studies considering aspects reported in the materials and methods section.
Meta-analyses
Due to the heterogeneous distribution of adhesives used, a global meta-analysis of all 78 studies was not performed. Hence, cross-linkers were first assigned to the subgroups (immediate or long-term) based on their mode of application (used as a pre-treatment solution, incorporated in adhesive systems or in acid etching agent). Figure 3 represents the meta-analysis results on immediate and long-term bond strengths with different application methods of cross-linkers, respectively. Overall, there was a significant difference between the groups, showing evidence that the presence of cross-linkers produced superior resin–dentin bonds than the control group (p < 0.05). For immediate results, the mean differences between the experimental and the control groups were higher when used as a pre-treatment solution (p ≤ 0.03), but not used by incorporating it into adhesive systems and acid etching agent (p > 0.05). In terms of long-term bond strength, regardless of the application method, the groups presented better bond potentials (p ≤ 0.05), and the heterogeneity of the three analyzed sets was high (I2 ≥ 70%).
The meta-analysis on different adhesives used (Figure 4) showed significant differences between the groups using ER adhesives in favor of cross-linkers; both immediate (p ≤ 0.02) and long-term results (p < 0.00001) showed that the control group exhibited lower bonding potential than the experimental group. However, similar bonding effects were observed for SE adhesives between the cross-linkers and the control group (p > 0.05): immediate (effect size: 0.03, 95% CI: 0.41, 0.34; p = 0.86) and long-term results (effect size: 0.38, 95% CI: 0.02, 0.34; p = 0.79).
The NMA was conducted on studies grouped according to the presence or absence of as well as the type of cross-linkers (six categories), so a total of seven arms were compared with each other. Two sets of NMA were created, one for immediate (Figure 5) and the other for the long-term (Figure 6) data storage. Most pairwise comparisons were made between the “polyphenols” and the “control” groups (Figures 5A, 6A), while direct comparisons were made for all groups in the immediate and long-term subgroups, as shown in the league table (Figures 5B, 6B).
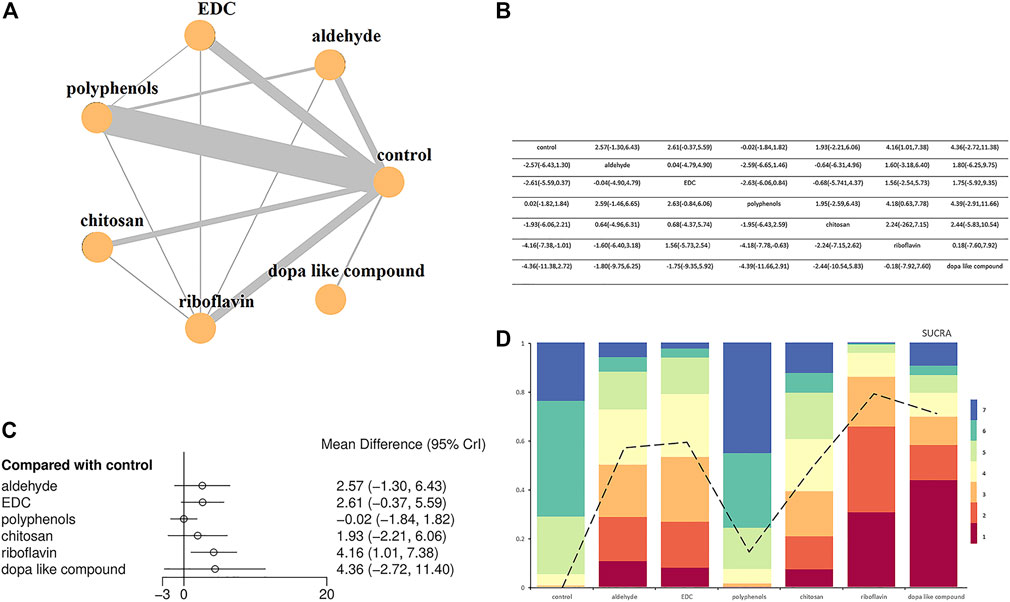
FIGURE 5. Network meta-analysis comparing bond strengths among seven group arms. (A) Network plot where each node indicates a direct comparison (control, aldehyde, EDC, polyphenols, chitosan, riboflavin and dopa like compound) with the thickness of connecting lines between nodes representing the number of studies compared. (B) League table showing Bayesian comparisons for all groups: this table shows the results for all group pairs in the upper (direct comparison) and lower (indirect comparison) triangles, but the comparisons have been switched; For the leading diagonal above and below, the result is the grouping at the top of the same column versus the grouping to the left of the same row. (C) Bayesian random effect consistency model forest plot of the pooled effects estimates of bond strengths expressed in mean difference (MD) and respective 95% credible intervals (95% CrI) for different adhesive groups compared with the control group. (D) Cumulative ranks and SUCRA-values.
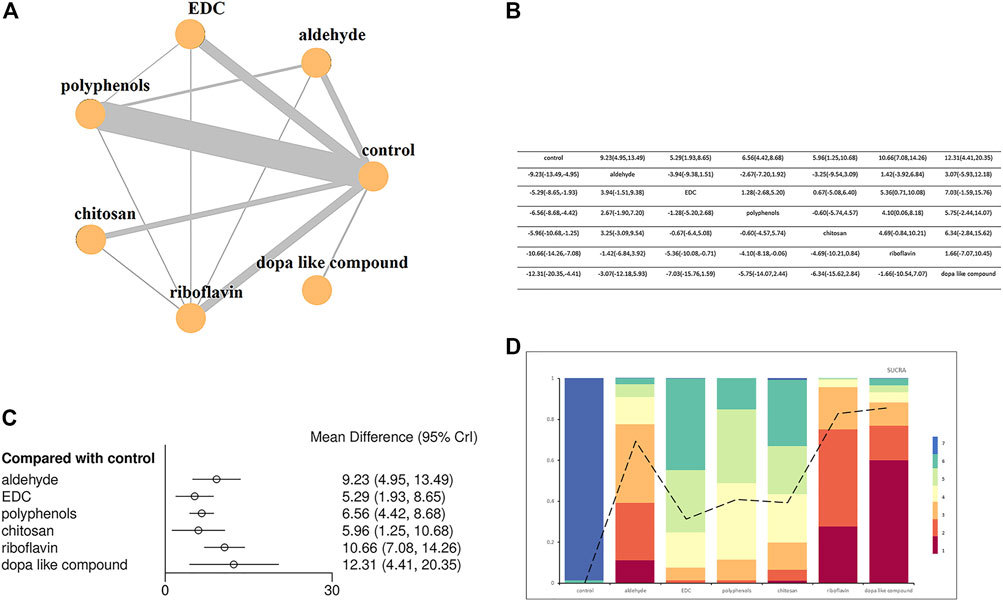
FIGURE 6. Network meta-analysis comparing the long-term bond strength between the control group and the six types cross-linkers. (A) Network plot where each node indicates a direct comparison (control, aldehyde, EDC, polyphenols, chitosan, riboflavin and dopa like compound) with the thickness of connecting lines between nodes representing the number of studies compared. (B) League table showing Bayesian comparisons for all groups: this table shows the results for all group pairs in the upper (direct comparison) and lower (indirect comparison) triangles, but the comparisons have been switched; For the leading diagonal above and below, the result is the grouping at the top of the same column versus the grouping to the left of the same row. (C) Bayesian random effect consistency model forest plot of the pooled effects estimates of bond strengths expressed in mean difference (MD) and respective 95% credible intervals (95% CrI) for different adhesive groups compared with the control group. (D) Cumulative ranks and SUCRA-values.
Forest plots comparing different types of crosslinkers with the “control” group demonstrated that the immediate bonding effect of crosslinkers and controls was similar, except for the “riboflavin” group (effect size: 4.16, 95% CrI: 1.01, 7.38) (Figure 5C), but long-term results showed that the former was associated with smaller resin-dentin bonding, with a reduction of 5.29–12.31 MPa (Figure 6C).
When evaluating the sorting probability and resulting SUCRA images (Figures 5D, 6D), riboflavin showed a higher immediate bond strength, while Dope like compound, EDC, aldehydes, chitosan, and polyphenols showed similar bonding performance as the control group (Figure 5B). In long-term outcomes, Dope like compound ranked highest, followed by riboflavin, aldehydes, polyphenols, chitosan, and EDC. The control group had the lowest ranking, while the possibility of smaller bonding properties was the highest, with limited differences (Figure 6D).
Discussion
This is the first study to perform an NMA to compare the bonding performance of different adhesive systems to dentin, depending on the cross-linkers. The purpose of this study was to aee if the longevity lifespans of dentin bond strength could be effectively increased using a homogeneous systematic review. As much information was collected about the relevant studies to achieve reliability. In general, resin-dentin adhesion was favorable in the case of cross-linkers, depending on their types and application modes.
Effect of different application methods of cross-linkers
In this meta-analysis, the majority of cross-linking agents were used as a pre-treatment solution (∼65.1%), followed by addition into the adhesive system (∼30.1%), and few were mixing in acid etching agents (4.8%) (Table 1). The analyses showed that the usage of cross-linkers as primers led to a significant improvement in the immediate and long-term bond strength values (p = 0.03 and p < 0.00001). The long-term bond strength was improved after adding cross-linkers to the adhesive system and acid etching agent (adhesive system, p = 0.0001; acid etching agent, p = 0.05).
It is already known that cross-linkers used alone as primers have gained popularity in the last two decades due to their superior crosslinking ability without compromising the degree of conversion of adhesives (Comba et al., 2020; Li et al., 2021a; Yu et al., 2022). Taking into consideration that cross-linkers were used only as a primer, they imparted greater bond strengths to dentin as compared to the control group (Figure 3). The disadvantage using cross-linkers as primers in clinical practice is that adds an extra step to the bonding protocol, making the process more difficult for the clinician (Liu et al., 2014). Incorporating cross-linking agents into the adhesive system or acid etching agent can significantly improve bonding durability and not impair the immediate result (Figure 3), which is due to the formation of a stable HL with dentin (45, 50–52). For example, the ability to react with the dentin matrix and the consequent impact on reducing proteolytic degradation, maintains the integrity of HL, thereby improving its longevity (53, 54). Notably, cross-linkers possess three desirable properties that may favor superior dentin bonds: 1) Maintain an elaborate collagen network and facilitate inter-diffusion of solvent and hydrophilic monomers; 2) Increase the hardness of demineralized dentin, thereby reducing the plasticizing effect of absorbed water; 3) Inhibit collagenase activity, resulting in a reduced in collagen biodegradation rate at the resin-dentin interface (Kishen et al., 2016).
Effect of the application category of the adhesive system
Based on 78 studies included in this review, a total of 20 different types of cross-linkers were investigated (Figure 2), with Adper Single Bond 2 representing the most frequently reported adhesive material (∼35.9%). Most of the analyzed bond strength data were derived from ER adhesives (∼77%), followed by SE adhesives. In the case of ER adhesives, cross-linkers showed greater dentin bond strengths as compared to the control group (Figure 4), which may be due to some characteristics of ER adhesives that allow the formation of a strong HL with dentin (Pashley et al., 2011). However, the results of SE adhesives, the dentin bond strengths were similarly distributed between the experiment and the control groups (Figure 4). Another study has shown that cross-linkers are more effective in preservation and enzyme silencing when used with ER adhesives due to the process of dentin etching which allows the cross-linker to interact freely with collagen molecules, and substrate that has been degraded by matrix metalloproteinases (MMPs) (Mazzoni et al., 2006). Cross-linkers may still have an effect on the resin-sparse, water-rich collagen fibril layer at the bottom of HL during long-term storage (Maravic et al., 2021). Furthermore, in SE adhesives, the dentin tubules are blocked by the smear layer, and cross-linking agents significantly reduce the exposed MMPs activity and the cross-linking of exposed collagen fibrils; thus, presenting exposed dentinal tubules along with spare collagen fibrils. Therefore, they are not so effective in strengthening the collagen matrix (Singh et al., 2015; Maravic et al., 2021).
Effect of the different types of cross-linkers
In the presented review, all bond strength data were divided into seven different groups aiming for an NMA. The groups varied in terms of the presence/absence of cross-linkers as well as their classification as control, aldehydes, EDC, polyphenols, chitosan, riboflavin and Dope like compound. Overall, cross-linkers favor longer lifespans of the resin-dentin bonds by stabilizing HL (Breschi et al., 2018; Cai et al., 2018). Additionally, Figures 5, 6 demonstrate the efficacy of Dope like compound and riboflavin when compared to the other classes, especially in long-term results. This is an important finding that highlights which cross-linkers should be used to prevent the degradation of HL, thus providing directions for future research.
According to the network analysis, Dope like compound achieved the highest ranking in terms of bond strength, both immediate and long-term. It was observed that cross-linking occurs between the catechol groups of MAP and amino groups of collagen fibrils through covalent bonds, which causes collagen to become stiff by preventing its triple-helix conformation from uncoiling (Sabatini and Pashley, 2014). Furthermore, MAP also enhances the resistance of the crosslinked collagen to enzymatic degradation, and can directly interfere with the active and the changed enzyme sites of the group (Fang et al., 2017). DMA consists of three different parts, namely carbon-carbon double bond, polyphenol structure, and connection group, made up of amide compound (Martinez Rodriguez et al., 2015). The carbon-carbon double bond can be combined with the grafting monomer. Whereas the two hydroxyl polyphenol groups are intersected with dentin collagen fibrils. Unlike the ester base, the amide base is more stable and provides durability in a moist environment (Rodrigues et al., 2015). As DMA can combine the adhesives and dentin as a whole unit, this unique feature allows it to enhance the strength of the collagen matrix stability and protect HL from hydrolysis (Li et al., 2020; Li et al., 2021a; Li et al., 2021b).
Riboflavin is a cross-linker producing free radicals via photooxidation (UVA), which improves the rigidity and mechanical stability of the collagen matrix, as well as the penetration capacity of the adhesive resin (Frassetto et al., 2016). Daood et al. proposed that the addition of riboflavin (0.1%) to the adhesive significantly increases the bond strength and maintains the resin-dentin bond’s durability without negatively affecting the degree of conversion (Fu et al., 2020). In the cross-linking mechanism of riboflavin, covalent bonds are formed within the collagen amino group (Zhang et al., 2011) and are cross-linked to proline and/or lysine in collagen via functional hydroxyl groups in riboflavin (Wollensak et al., 2007). It also inhibits MMPs activity, increases the stiffness of dentin collagen, and improves resin-dentin bonding (Cova et al., 2011; Chiang et al., 2013). Therefore, riboflavin is a relatively effective cross-linking agent, and this conclusion was consistent with the meta-analysis results.
It has been broadly accepted that GA performs better than other cross-linkers, since it increases type I collagen covalent bonds by cross-linking amino groups that bridge the lysine and hydroxylysine residues of different collagen polypeptide chains, as well as improves the mechanical properties of dentin, contributing to better dental bonds (54, 55). But its clinical application is limited due to its depolymerization effect and the high cytotoxicity of uncured molecules (47, 55). Recently, researchers have successively synthesized acrolein and FA, which, similarly to GA, bind to exposed collagen fibers, form stable covalent bonds, and produce intermolecular cross-links with adjacent collagen matrix (Maravic et al., 2018; Yu et al., 2022). Furthermore, they also inhibit the activity of collagenolytic enzymes in the deeper regions of HL, providing more efficacy than GA (Hass et al., 2016a). How to overcome GA’s cytotoxicity while ensuring an excellent cross-linking effect of the aldehyde group is a current research hotspot. Our results demonstrated that aldehydes could promote the bond strength of dentin.
The results suggest that polyphenols can effectively improve long-term bond strength without compromising immediate bond strength. Since the structure of natural polyphenols contains multiple phenolic hydroxyl groups, they enhance the structural stability of collagen molecules through hydrogen bonds. Several polyphenolic cross-linkers are now being used, among which PA and EGCG have the highest frequency. As a natural cross-linking agent, PA has antioxidant, antibacterial, and anti-inflammatory properties, and its low toxicity makes it a widely studied (Chen et al., 2022). A study has shown that using PA (6.5%) for dentin can promote long-term bond strength (Dávila-Sánchez et al., 2020). Moreover, PA provides a better collagen network and increased fiber volume, which enhance the penetration of adhesives and produce a higher-quality HL with greater bond strength (Zheng and Chen, 2017). Under clinically relevant circumstances, PA can effectively stabilize demineralized dentin collagen in anti-enzymatic activity, due to its non-covalent nature and covalent, electrostatic, and hydrophobic interactions with collagen molecules (Perdigão et al., 2013). Another study confirmed that strong bonds could be formed between the amide carbonyl group of collagen and the phenolic hydroxyl group of PA; resulting in the formation of proline-PA complexes (Parise Gré et al., 2018). Recent studies on the incorporation of PA into acid etching agents and experimental adhesives have demonstrated that they stabilize the dentin bonding interface without any adverse effects (Hass et al., 2016b; Loguercio et al., 2017).
It is well known that EGCG is a collagen cross-linker obtained from green tea with low toxicity and anti-inflammatory properties (Perdigão et al., 2013; Albuquerque et al., 2019), that stabilizes the collagen chain (Goo et al., 2003). It is worth mentioning that it reduces collagen biodegradation and increase the number of collagen crosslinks through hydrogen molecular interactions of acyl groups (Goo et al., 2003). This study outcomes are in accordance with previous studies that showed no adverse effects for the long-term bond efficacy to dentin, and displayed promoting effects without changing the degree of polymerization of experimental adhesives (Yu et al., 2017; Czech et al., 2019). Another cross-linker, chitosan, is a naturally hydrophilic polycationic biopolymer with inherent, adhesive potential and antibacterial properties, along with a wide range of dental applications (Shrestha and Kishen, 2012; Daood et al., 2013). Since chitosan displays properties of cross-linkage, a large number of free hydroxyl and amino groups form ionic complexes with collagen, which can produce microfiber arrangement in the collagen structure (Abd El-Hack et al., 2020). Notably, these cross-linked collagen matrices also possess antibacterial and anti-biofilm activities (Daood et al., 2018; Fonseca et al., 2019) The incorporation of chitosan into dentin adhesives increases long-term bond strength and creates an interface with antibacterial properties (Elsaka and Elnaghy, 2012; Diolosà et al., 2014) The usage of the chitosan-riboflavin combination enhanced the mechanical properties of dentin and synergistically reduced the degradation of the resin-dentin interface (Daood et al., 2018). The study results, in contrast to previous results (Hardan et al., 2022), refute the finding that chitosan is a weak crosslinker and does not have a significant effect when used alone.
According to thisanalysis, both the immediate and long-term bond strength levels improved after using EDC; it can be a good alternative to GA by forming amide bonds between the carboxyl and amino groups of collagen molecules. Moreover, it does not participate in the cross-linking process and shows greater biocompatibility due to urea derivatives (Nimni, 1988; Mazzoni et al., 2013a). In addition to the cross-linked collagen, EDC also interacts with the extracellular dentin matrix through MMPs inactivation by cross-linking the catalytic or non-catalytic parts of MMPS so that the substrate is unrecognized and gets cleaved, or the triple helix of the collagen molecule cannot be unwound (Mazzoni et al., 2017). EDC also cross-links proteins (collagen) by donating O-acyl urea groups, which activate the carboxylic acid groups of glutamate as well as aspartic acid peptide residues within 1-h treatment time and is clinically unacceptable (Cammarata et al., 2015). A recent in vitro study on EDC application on demineralized dentin for 60s suggested that EDC can persist in HL for 5 years, in term of bond strength, collagen structure preservation and dentinal enzyme silencing (Maravic et al., 2021). However, this might be relevant to the results of this study.
Research prospects
The presented results showed that most of the studies focused on ER adhesives, while only a few the number of studies were on SE adhesives. Furthermore, the majority of the studies in this network analysis focused on control and polyphenols, followed by EDC and riboflavin. Due to its promising results, cross-linking can be considered a simple and clinically applicable method to improve bonding durability and reduce collagen degradation in HL. Currently, studies on various cross-linkers are still conducted in the laboratory, as it is difficult to simulate the challenges of the oral environment (pH, occlusal load and thermal stress, etc.). However, more clinical studies are needed to confirm the beneficial effects of these cross-linkers in vivo.
Advantages and limitations of the study
This study has several advantages. It is the first study to investigate the effects of cross-linkers different factors on the bond strength of cross-linkers in dentin. The effects of different cross-linkers application methods and adhesive types on bond strength were analyzed by conventional meta-analysis, while the different types of cross-linkers were ranked by Bayesian analysis. However, this study also had some limitations. Since the heterogeneity was relatively large, it could have affected the accuracy.
Conclusion
Due to a moderate heterogeneity in most studies based on this meta-analysis, an overall advantage of using cross-linkers for better dentine bond potential was observed. The results were dependent only on the application category of the adhesive system and were not affected by different application methods and the types of cross-linkers. The use of cross-linkers on acid-etched dentin increased the beneficial effects of cross-linkers and demineralized collagen and inhibited matrix metalloproteinases at the interface, which benefited dental bonding. Based on this meta-analysis, it is possible to conclude can be concluded that the application of different cross-linkers such as Dope like compound, riboflavin, GA, polyphenols, chitosan, and EDC improved the long-term bonding performance. It is worth noting that, of the cross-linkers examined in this review, Dope like compound have higher bonding potential to dentin than other classes of cross-linkers.
Data availability statement
The original contributions presented in the study are included in the article/Supplementary Material, further inquiries can be directed to the corresponding author.
Author contributions
All authors contributed to the study conception and design, HC and GS: Conceptualization, methodology, software, writing–original draft, data curation, visualization were performed; HW and SY: Investigation, writing–original draft, writing–reviewing and editing were performed; ZT: Methodology, software, writing–original draft were performed; SZ: Conceptualization, supervision, project administration, funding acquisition were performed. All authors read and approved the final manuscript.
Funding
This work was supported by National Natural Science Foundation of China (82071163).
Conflict of interest
The authors declare that the research was conducted in the absence of any commercial or financial relationships that could be construed as a potential conflict of interest.
Publisher’s note
All claims expressed in this article are solely those of the authors and do not necessarily represent those of their affiliated organizations, or those of the publisher, the editors and the reviewers. Any product that may be evaluated in this article, or claim that may be made by its manufacturer, is not guaranteed or endorsed by the publisher.
Supplementary material
The Supplementary Material for this article can be found online at: https://www.frontiersin.org/articles/10.3389/fbioe.2022.1100894/full#supplementary-material
References
Abd El-Aal, N. H., Abed El-Haliem, H., and Zaghloul, N. M. (2022). Effect of grape seed extract on the bond strength and adhesion durability of universal adhesive to dentin. Int. J. Adhesion Adhesives 113, 103073. doi:10.1016/j.ijadhadh.2021.103073
Abd El-Hack, M. E., El-Saadony, M. T., Shafi, M. E., Zabermawi, N. M., Arif, M., Batiha, G. E., et al. (2020). Antimicrobial and antioxidant properties of chitosan and its derivatives and their applications: A review. Int. J. Biol. Macromol. 164, 2726–2744. doi:10.1016/j.ijbiomac.2020.08.153
Abdelshafi, M. A., Fathy, S. M., Elkhooly, T. A., Reicha, F. M., and Osman, M. F. (2021). Bond strength of demineralized dentin after synthesized collagen/hydroxyapatite nanocomposite application. J. Mech. Behav. Biomed. Mater. 121, 104590. doi:10.1016/j.jmbbm.2021.104590
Abunawareg, M., Abuelenain, D. A., Elkassas, D., Abu Haimed, T., Al-Dharrab, A., Zidan, A., et al. (2017). Role of dentin cross-linking agents in optimizing dentin bond durability. Int. J. Adhesion Adhesives 78, 83–88. doi:10.1016/j.ijadhadh.2017.06.009
Albuquerque, N., Neri, J. R., Lemos, M., Yamauti, M., de Sousa, F., and Santiago, S. L. (2019). Effect of polymeric microparticles loaded with catechin on the physicochemical properties of an adhesive system. Oper. Dent. 44 (4), E202–E211. doi:10.2341/18-112-l
Andre, C. B., Almeida Gomes, F. B. P., Duque, T. M., Stipp, R. N., Chan, D. C. N., Bovi Ambrosano, G. M., et al. (2015). Dentine bond strength and antimicrobial activity evaluation of adhesive systems. J. Dent. 43 (4), 466–475. doi:10.1016/j.jdent.2015.01.004
Armstrong, S. R., Vargas, M. A., Chung, I., Pashley, D. H., Campbell, J. A., Laffoon, J. E., et al. (2004). Resin-dentin interfacial ultrastructure and microtensile dentin bond strength after five-year water storage. Oper. Dent. 29 (6), 705–712.
Bacelar-Sa, R., Giannini, M., Ambrosano, G. M. B., and Bedran-Russo, A. K. (2017). Dentin sealing and bond strength evaluation of hema-free and multi-mode adhesives to biomodified dentin. Braz. Dent. J. 28 (6), 731–737. doi:10.1590/0103-6440201701522
Baena, E., Cunha, S. R., Maravić, T., Comba, A., Paganelli, F., Alessandri-Bonetti, G., et al. (2020). Effect of chitosan as a cross-linker on matrix metalloproteinase activity and bond stability with different adhesive systems. Mar. Drugs 18 (5), 263. doi:10.3390/md18050263
Baldion, P. A., Cortes, C. C., Castellanos, J. E., and Betancourt, D. E. (2021). Effect of myricetin on odontoblast-like cells and its potential to preserve resin–dentin Bonds. J. Mech. Behav. Biomed. Mater. 117, 104392. doi:10.1016/j.jmbbm.2021.104392
Beck, F., and Ilie, N. (2020). Antioxidants and collagen-crosslinking: Benefit on bond strength and clinical applicability. Materials 13 (23), 5483. doi:10.3390/ma13235483
Beck, F., and Ilie, N. (2022). Riboflavin and its effect on dentin bond strength: Considerations for clinical applicability-an in vitro study. Bioengineering-Basel. 9 (1), 34. doi:10.3390/bioengineering9010034
Bedran-Russo, A. K., Pashley, D. H., Agee, K., Drummond, J. L., and Miescke, K. J. (2008). Changes in stiffness of demineralized dentin following application of collagen crosslinkers. J. Biomed. Mater. Res. Part B, Appl. biomaterials 86 (2), 330–334. doi:10.1002/jbm.b.31022
Bedran-Russo, A. K., Pauli, G. F., Chen, S. N., McAlpine, J., Castellan, C. S., Phansalkar, R. S., et al. (2014). Dentin biomodification: Strategies, renewable resources and clinical applications. Dent. Mater. 30 (1), 62–76. doi:10.1016/j.dental.2013.10.012
Breschi, L., Maravic, T., Cunha, S. R., Comba, A., Cadenaro, M., Tjäderhane, L., et al. (2018). Dentin bonding systems: From dentin collagen structure to bond preservation and clinical applications. Dent. Mater 34 (1), 78–96. doi:10.1016/j.dental.2017.11.005
Breschi, L., Mazzoni, A., Ruggeri, A., Cadenaro, M., Di Lenarda, R., and De Stefano Dorigo, E. (2008). Dental adhesion review: Aging and stability of the bonded interface. Dent. Mater 24 (1), 90–101. doi:10.1016/j.dental.2007.02.009
Cai, J., Palamara, J. E. A., and Burrow, M. F. (2018). Effects of collagen crosslinkers on dentine: A literature review. Calcif. Tissue Int. 102 (3), 265–279. doi:10.1007/s00223-017-0343-7
Cammarata, C. R., Hughes, M. E., and Ofner, C. M. (2015). Carbodiimide induced cross-linking, ligand addition, and degradation in gelatin. Mol. Pharm. 12 (3), 783–793. doi:10.1021/mp5006118
Carvalho, C., Fernandes, F. P., Freitas Vda, P., França, F. M., Basting, R. T., Turssi, C. P., et al. (2016). Effect of green tea extract on bonding durability of an etch-and-rinse adhesive system to caries-affected dentin. J. Appl. oral Sci. revista FOB 24 (3), 211–217. doi:10.1590/1678-775720150518
Castellan, C. S., Bedran-Russo, A. K., Antunes, A., and Pereira, P. N. (2013). Effect of dentin biomodification using naturally derived collagen cross-linkers: One-year bond strength study. Int. J. Dent. 2013, 1–6. doi:10.1155/2013/918010
Chen, H., Wang, W., Yu, S., Wang, H., Tian, Z., and Zhu, S. (2022). Procyanidins and their therapeutic potential against oral diseases. Mol. Basel, Switz. 27 (9), 2932. doi:10.3390/molecules27092932
Chen, W., Jin, H., Zhang, H., Wu, L., Chen, G., Shao, H., et al. (2021). Synergistic effects of graphene quantum dots and carbodiimide in promoting resin-dentin bond durability. Dent. Mater 37 (10), 1498–1510. doi:10.1016/j.dental.2021.07.004
Chiang, Y. S., Chen, Y. L., Chuang, S. F., Wu, C. M., Wei, P. J., Han, C. F., et al. (2013). Riboflavin-ultraviolet-A-induced collagen cross-linking treatments in improving dentin bonding. Dent. Mater. official Publ. Acad. Dent. Mater. 29 (6), 682–692. doi:10.1016/j.dental.2013.03.015
Comba, A., Maravic, T., Valente, L., Girlando, M., Cunha, S. R., Checchi, V., et al. (2019). Effect of benzalkonium chloride on dentin bond strength and endogenous enzymatic activity. J. Dent. 85, 25–32. doi:10.1016/j.jdent.2019.04.008
Comba, A., Maravić, T., Villalta, V., Tozzola, S., Mazzitelli, C., Checchi, V., et al. (2020). Effect of an ethanol cross-linker on universal adhesive. Dent. Mater 36 (12), 1645–1654. doi:10.1016/j.dental.2020.10.004
Costa, C. A. G., Passos, V. F., Neri, J. R., Mendonça, J. S., and Santiago, S. L. (2019). Effect of metalloproteinase inhibitors on bond strength of a self-etching adhesive on erosively demineralized dentin. J. Adhes. Dent. 21 (4), 337–344. doi:10.3290/j.jad.a42930
Cova, A., Breschi, L., Nato, F., Ruggeri, A., Carrilho, M., Tjäderhane, L., et al. (2011). Effect of UVA-activated riboflavin on dentin bonding. J. Dent. Res. 90 (12), 1439–1445. doi:10.1177/0022034511423397
Czech, R., Oliveira, C. A. R., Franca, F. M. G., Basting, R. T., Turssi, C. P., and Amaral, F. L. B. (2019). Incorporation of EGCG into an etch-and-rinse adhesive system: Mechanical properties and bond strength to caries affected dentin. J. Adhesion Sci. Technol. 33 (22), 2430–2442. doi:10.1080/01694243.2019.1642978
Dacoreggio, R., Bridi, E. C., Basting, R. T., Tenuti, J. G. B., Franca, F. M. G., Turssi, C. P., et al. (2021). Incorporation of chitosan into a universal adhesive system: Physicochemical characteristics, gelatinolytic activity, bond strength and interface micromorphology analyses. Int. J. Adhesion Adhesives 106, 102814. doi:10.1016/j.ijadhadh.2021.102814
Daood, U., Iqbal, K., Nitisusanta, L. I., and Fawzy, A. S. (2013). Effect of chitosan/riboflavin modification on resin/dentin interface: Spectroscopic and microscopic investigations. J. Biomed. Mater. Res. Part A 101 (7), 1846–1856. doi:10.1002/jbm.a.34482
Daood, U., Omar, H., Tsoi, J. K. H., and Fawzy, A. S. (2018). Long-term bond strength to dentine of a chitosan-riboflavin modified two-step etch-and-rinse adhesives. Int. J. Adhesion Adhesives 85, 263–273. doi:10.1016/j.ijadhadh.2018.06.015
Daood, U., Omar, H., Qasim, S., Nogueira, L. P., Pichika, M. R., Mak, K. K., et al. (2020a). New antimicrobial and collagen crosslinking formulated dentin adhesive with improved bond durability. J. Mech. Behav. Biomed. Mater. 110, 103927. doi:10.1016/j.jmbbm.2020.103927
Daood, U., Sauro, S., Pichika, M. R., Omar, H., Lin, S. L., and Fawzy, A. S. (2020b). Novel riboflavin/VE-TPGS modified universal dentine adhesive with superior dentine bond strength and self-crosslinking potential. Dent. Mater. 36 (1), 145–156. doi:10.1016/j.dental.2019.11.003
Dávila-Sánchez, A., Gutierrez, M. F., Bermudez, J. P., Méndez-Bauer, M. L., Hilgemberg, B., Sauro, S., et al. (2020). Influence of flavonoids on long-term bonding stability on caries-affected dentin. Dent. Mater. official Publ. Acad. Dent. Mater. 36 (9), 1151–1160. doi:10.1016/j.dental.2020.05.007
de Macedo, F. A. A., Souza, N. O., Lemos, M. V. S., De-Paula, D. M., Santiago, S. L., and Feitosa, V. P. (2019). Dentin bonding and physicochemical properties of adhesives incorporated with epigallocatechin-3-gallate. Odontology 107 (1), 23–28. doi:10.1007/s10266-018-0367-0
de Paula, D. M., Lomonaco, D., Parente da Ponte, A. M., Cordeiro, K. E., Magalhães Moreira, M., Giovarruscio, M., et al. (2022). Collagen cross-linking lignin improves the bonding performance of etch-and-rinse adhesives to dentin. Mater. Basel, Switz. 15 (9).
De-Paula, D. M., Lomonaco, D., Ponte, A. M. P., Cordeiro, K. E., Moreira, M. M., Mazzetto, S. E., et al. (2020). Influence of collagen cross-linkers addition in phosphoric acid on dentin biomodification and bonding of an etch-and-rinse adhesive. Dent. Mater. 36 (1), e1–e8. doi:10.1016/j.dental.2019.11.019
de Siqueira, F. S. F., Hilgemberg, B., Araujo, L. C. R., Hass, V., Bandeca, M. C., Gomes, J. C., et al. (2020). Improving bonding to eroded dentin by using collagen cross-linking agents: 2 years of water storage. Clin. Oral Investig. 24 (2), 809–822.
Diolosà, M., Donati, I., Turco, G., Cadenaro, M., Di Lenarda, R., Breschi, L., et al. (2014). Use of methacrylate-modified chitosan to increase the durability of dentine bonding systems. Biomacromolecules 15 (12), 4606–4613. doi:10.1021/bm5014124
dos Santos, A., Andre, C. B., Martim, G. C., Schuquel, I. T. A., Pfeifer, C. S., Ferracane, J. L., et al. (2018). Methacrylate saccharide-based monomers for dental adhesive systems. Int. J. Adhesion Adhesives 87, 1–11. doi:10.1016/j.ijadhadh.2018.09.009
Du, X., Huang, X., Huang, C., Wang, Y., and Zhang, Y. (2012). Epigallocatechin-3-gallate (EGCG) enhances the therapeutic activity of a dental adhesive. J. Dent. 40 (6), 485–492. doi:10.1016/j.jdent.2012.02.013
Elsaka, S., and Elnaghy, A. (2012). Effect of addition of chitosan to self-etching primer: Antibacterial activity and push-out bond strength to radicular dentin. J. Biomed. Res. 26 (4), 288–294. doi:10.7555/jbr.26.20120042
Epasinghe, D. J., Yiu, C. K. Y., and Burrow, M. F. (2015). Effect of proanthocyanidin incorporation into dental adhesive on durability of resin-dentin bond. Int. J. Adhesion Adhesives 63, 145–151. doi:10.1016/j.ijadhadh.2015.09.006
Fang, H., Li, Q. L., Han, M., Mei, M. L., and Chu, C. H. (2017). Anti-proteolytic property and bonding durability of mussel adhesive protein-modified dentin adhesive interface. Dent. Mater 33 (10), 1075–1083. doi:10.1016/j.dental.2017.07.008
Fawzy, A. S., Nitisusanta, L. I., Iqbal, K., Daood, U., Beng, L. T., and Neo, J. (2013). Chitosan/Riboflavin-modified demineralized dentin as a potential substrate for bonding. J. Mech. Behav. Biomed. Mater 17, 278–289. doi:10.1016/j.jmbbm.2012.09.008
Fawzy, A. S., Nitisusanta, L. I., Iqbal, K., Daood, U., and Neo, J. (2012). Riboflavin as a dentin crosslinking agent: ultraviolet A versus blue light. Dent. Mater 28 (12), 1284–1291. doi:10.1016/j.dental.2012.09.009
Fernandes, F. P., Adorno, C. C., da Silva, T. M., França, F. M. G., Turssi, C. P., Basting, R. T., et al. (2021). Addition of EGCG to self-etching primer: Effect on adhesive properties and bond stability to dentin. J. Adhesion Sci. Technol. 35 (17), 1895–1908. doi:10.1080/01694243.2020.1862450
Fernandes, A. B. F., Silva, R. C., Müller, M. A., da Cunha, L. F., Correr, G. M., and Gonzaga, C. C. (2022). Influence of two carbodiimides on the bond strength of universal adhesives to dentin. Odontology 110 (1), 99–105. doi:10.1007/s10266-021-00642-z
Fialho, M. P. N., Hass, V., Nogueira, R. P., Franca, F. M. G., Turssi, C. P., Basting, R. T., et al. (2019). Effect of epigallocatechin-3- gallate solutions on bond durability at the adhesive interface in caries-affected dentin. J. Mech. Behav. Biomed. Mater. 91, 398–405. doi:10.1016/j.jmbbm.2018.11.022
Fonseca, B. M., Barcellos, D. C., Silva, T. M. D., Borges, A. L. S., Cavalcanti, B. D. N., Prakki, A., et al. (2019). Mechanical-physicochemical properties and biocompatibility of catechin-incorporated adhesive resins. J. Appl. oral Sci. revista FOB 27, e20180111. doi:10.1590/1678-7757-2018-0111
Frassetto, A., Breschi, L., Turco, G., Marchesi, G., Di Lenarda, R., Tay, F. R., et al. (2016). Mechanisms of degradation of the hybrid layer in adhesive dentistry and therapeutic agents to improve bond durability--A literature review. Dent. Mater 32 (2), e41–e53. doi:10.1016/j.dental.2015.11.007
Fu, C., Deng, S., Koneski, I., Awad, M. M., Akram, Z., Matinlinna, J., et al. (2020). Multiscale in-vitro analysis of photo-activated riboflavin incorporated in an experimental universal adhesive. J. Mech. Behav. Biomed. Mater. 112, 104082. doi:10.1016/j.jmbbm.2020.104082
Gerhardt, K. M. F., Oliveira, C. A. R., Franca, F. M. G., Basting, R. T., Turssi, C. P., and Amaral, F. L. B. (2016). Effect of epigallocatechin gallate, green tea extract and chlorhexidine application on long-term bond strength of self-etch adhesive to dentin. Int. J. Adhesion Adhesives 71, 23–27. doi:10.1016/j.ijadhadh.2016.08.005
Goo, H. C., Hwang, Y. S., Choi, Y. R., Cho, H. N., and Suh, H. (2003). Development of collagenase-resistant collagen and its interaction with adult human dermal fibroblasts. Biomaterials 24 (28), 5099–5113. doi:10.1016/s0142-9612(03)00431-9
Gotti, V. B., Feitosa, V. P., Sauro, S., Correr-Sobrinho, L., Leale, F. B., Stansbury, J. W., et al. (2015). Effect of antioxidants on the dentin interface bond stability of adhesives exposed to hydrolytic degradation. J. Adhesive Dent. 17 (1), 35–44. doi:10.3290/j.jad.a33515
Hardan, L., Daood, U., Bourgi, R., Cuevas-Suárez, C. E., Devoto, W., Zarow, M., et al. (2022). Effect of collagen crosslinkers on dentin bond strength of adhesive systems: A systematic review and meta-analysis. Cells 11 (15), 2417. doi:10.3390/cells11152417
Hashimoto, M., Ohno, H., Kaga, M., Endo, K., Sano, H., and Oguchi, H. (2000). In vivo degradation of resin-dentin bonds in humans over 1 to 3 years. J. Dent. Res. 79 (6), 1385–1391. doi:10.1177/00220345000790060601
Hass, V., Luque-Martinez, I., Angel Munoz, M., Gutierrez Reyes, M. F., Abuna, G., Coelho Sinhoreti, M. A., et al. (2016). The effect of proanthocyanidin-containing 10% phosphoric acid on bonding properties and MMP inhibition. Dent. Mater. 32 (3), 468–475. doi:10.1016/j.dental.2015.12.007
Hass, V., Luque-Martinez, I. V., Gutierrez, M. F., Moreira, C. G., Gotti, V. B., Feitosa, V. P., et al. (2016). Collagen cross-linkers on dentin bonding: Stability of the adhesive interfaces, degree of conversion of the adhesive, cytotoxicity and in situ MMP inhibition. Dent. Mater. official Publ. Acad. Dent. Mater. 32 (6), 732–741. doi:10.1016/j.dental.2016.03.008
Hechler, B., Yao, X., and Wang, Y. (2012). Proanthocyanidins alter adhesive/dentin bonding strengths when included in a bonding system. Am. J. Dent. 25 (5), 276–280.
Jowkar, Z., Firouzmandi, M., and Tabibi, S. (2021). The effect of proanthocyanidin and casein phosphopeptide-amorphous calcium phosphate on the bond strength durability to caries-affected dentin. Clin. Exp. Dent. Res. 7 (3), 338–343. doi:10.1002/cre2.368
Kishen, A., Shrestha, S., Shrestha, A., Cheng, C., and Goh, C. (2016). Characterizing the collagen stabilizing effect of crosslinked chitosan nanoparticles against collagenase degradation. Dent. Mater 32 (8), 968–977. doi:10.1016/j.dental.2016.05.005
Lee, J., and Sabatini, C. (2017). Glutaraldehyde collagen cross-linking stabilizes resin-dentin interfaces and reduces bond degradation. Eur. J. oral Sci. 125 (1), 63–71. doi:10.1111/eos.12317
Li, K., Yang, H., Yan, H., Sun, Y., Chen, X., Guo, J., et al. (2017). Quercetin as a simple but versatile primer in dentin bonding. Rsc Adv. 7 (58), 36392–36402. doi:10.1039/c7ra07467k
Li, J., Chen, B., Hong, N., Wu, S., and Li, Y. (2018). Effect of baicalein on matrix metalloproteinases and durability of resin-dentin bonding. Oper. Dent. 43 (4), 426–436. doi:10.2341/17-097-l
Li, K., Sun, Y., Tsoi, J. K. H., and Yiu, C. K. Y. (2020). The application of mussel-inspired molecule in dentin bonding. J. Dent. 99, 103404. doi:10.1016/j.jdent.2020.103404
Li, K., Yao, C., Sun, Y., Wang, K., Wang, X., Wang, Z., et al. (2021). Enhancing resin-dentin bond durability using a novel mussel-inspired monomer. Mater. Today Bio 12, 100174. doi:10.1016/j.mtbio.2021.100174
Li, K., Zhang, Z., Sun, Y., Yang, H., Tsoi, J. K. H., Huang, C., et al. (2021). In vitro evaluation of the anti-proteolytic and cross-linking effect of mussel-inspired monomer on the demineralized dentin matrix. J. Dent. 111, 103720. doi:10.1016/j.jdent.2021.103720
Li, M. X., Duan, L., Chen, M. L., Tian, F. C., and Fu, B. P. (2022). Effect of an extrafibrillar dentin demineralization strategy on the durability of the resin–dentin bond. J. Mech. Behav. Biomed. Mater. 126, 105038. doi:10.1016/j.jmbbm.2021.105038
Liu, R. R., Fang, M., Zhang, L., Tang, C. F., Dou, Q., and Chen, J. H. (2014). Anti-proteolytic capacity and bonding durability of proanthocyanidin-biomodified demineralized dentin matrix. Int. J. oral Sci. 6 (3), 168–174. doi:10.1038/ijos.2014.22
Loguercio, A. D., Malaquias, P., Dos Santos, F. P., Hass, V., Stanislawczuk, R., Lima, S. N. L., et al. (2017). Acid etching with modified phosphoric acid to increase the longevity of the bonded interface. J. adhesive Dent., 195–201. doi:10.3290/j.jad.a38413
Magalhaes Rolim, D. C., Souza, L. C., Hass, V., Barros Silva, P. G., Signori, C., Cenci, M. S., et al. (2022). Effect of cross-linker's incorporation into two adhesive systems with self-etch mode applied on sound and caries-affected dentin. Int. J. Adhesion Adhesives 113, 103074. doi:10.1016/j.ijadhadh.2021.103074
Maravic, T., Breschi, L., Comba, A., Cunha, S. R., Angeloni, V., Nucci, C., et al. (2018). Experimental use of an acrolein-based primer as collagen cross-linker for dentine bonding. J. Dent. 68, 85–90. doi:10.1016/j.jdent.2017.11.006
Maravic, T., Mancuso, E., Comba, A., Checchi, V., Generali, L., Mazzitelli, C., et al. (2021). Dentin cross-linking effect of carbodiimide after 5 years. J. Dent. Res. 100 (10), 1090–1098. doi:10.1177/00220345211014799
Maravic, T., Mazzoni, A., Comba, A., Scotti, N., Checchi, V., and Breschi, L. J. C. O. H. R. (2017). How stable is dentin as a substrate for bonding? Curr. Oral Health Rep. 4 (3), 248–257. doi:10.1007/s40496-017-0149-8
Martinez Rodriguez, N. R., Das, S., Kaufman, Y., Wei, W., Israelachvili, J. N., and Waite, J. H. (2015). Mussel adhesive protein provides cohesive matrix for collagen type-1α. Biomaterials 51, 51–57. doi:10.1016/j.biomaterials.2015.01.033
Matuda, L. S., Marchi, G. M., Aguiar, T. R., Leme, A. A., Ambrosano, G. M., and Bedran-Russo, A. K. (2016). Dental adhesives and strategies for displacement of water/solvents from collagen fibrils. Dent. Mater 32 (6), 723–731. doi:10.1016/j.dental.2016.03.009
Mazzoni, A., Angeloni, V., Apolonio, F. M., Scotti, N., Tjäderhane, L., Tezvergil-Mutluay, A., et al. (2013). Effect of carbodiimide (EDC) on the bond stability of etch-and-rinse adhesive systems. Dental materials: official publication of the Academy of Dental Materials 29 (10), 1040–1047. doi:10.1016/j.dental.2013.07.010
Mazzoni, A., Angeloni, V., Comba, A., Maravic, T., Cadenaro, M., Tezvergil-Mutluay, A., et al. (2018). Cross-linking effect on dentin bond strength and MMPs activity. Dent. Mater. official Publ. Acad. Dent. Mater. 34 (2), 288–295. doi:10.1016/j.dental.2017.11.009
Mazzoni, A., Angeloni, V., Sartori, N., Duarte, S., Maravic, T., Tjäderhane, L., et al. (2017). Substantivity of carbodiimide inhibition on dentinal enzyme activity over time. J. Dent. Res. 96 (8), 902–908. doi:10.1177/0022034517708312
Mazzoni, A., Pashley, D. H., Nishitani, Y., Breschi, L., Mannello, F., Tjäderhane, L., et al. (2006). Reactivation of inactivated endogenous proteolytic activities in phosphoric acid-etched dentine by etch-and-rinse adhesives. Biomaterials 27 (25), 4470–4476. doi:10.1016/j.biomaterials.2006.01.040
Mazzoni, A., Scaffa, P., Carrilho, M., Tjäderhane, L., Di Lenarda, R., Polimeni, A., et al. (2013). Effects of etch-and-rinse and self-etch adhesives on dentin MMP-2 and MMP-9. J. Dent. Res. 92 (1), 82–86. doi:10.1177/0022034512467034
Mjör, I. A., Shen, C., Eliasson, S. T., and Richter, S. (2002). Placement and replacement of restorations in general dental practice in Iceland. Oper. Dent. 27 (2), 117–123.
Montagner, A. F., Sarkis-Onofre, R., Pereira-Cenci, T., and Cenci, M. S. (2014). MMP inhibitors on dentin stability: A systematic review and meta-analysis. J. Dent. Res. 93 (8), 733–743. doi:10.1177/0022034514538046
Neri, J. R., Yamauti, M., Silveira, F., Mendonça, J., Carvalho, R., Santiago, S. L. J. I. JoA., et al. (2016). Influence of dentin biomodification with epigallocatechin-3-gallate on the bond strength of self-etch adhesive: Twelve-month results. Int. J. Adhesion Adhesives 71. doi:10.1016/j.ijadhadh.2016.08.007
Nimni, M. E. (1988). The cross-linking and structure modification of the collagen matrix in the design of cardiovascular prosthesis. J. cardiac Surg. 3 (4), 523–533. doi:10.1111/j.1540-8191.1988.tb00446.x
Page, M. J., McKenzie, J. E., Bossuyt, P. M., Boutron, I., Hoffmann, T. C., Mulrow, C. D., et al. (2021). The PRISMA 2020 statement: An updated guideline for reporting systematic reviews. BMJ Clin. Res. ed 372, n71. doi:10.1136/bmj.n71
Paik, Y., Kim, J. H., Yoo, K. H., Yoon, S. Y., and Kim, Y. I. (2022). Dentin biomodification with flavonoids and calcium phosphate ion clusters to improve dentin bonding stability. Materials 15 (4), 1494. doi:10.3390/ma15041494
Paludo, T., Marcondes, M. L., Souto, A. A., Lopes, G. C., Loguércio, A. D., and Spohr, A. M. (2019). Effect of grape seed extract-containing phosphoric acid formulations on bonding to enamel and dentin. Braz Oral Res. 33, e098. doi:10.1590/1807-3107bor-2019.vol33.0098
Parise Gré, C., Pedrollo Lise, D., Ayres, A. P., De Munck, J., Tezvergil-Mutluay, A., Seseogullari-Dirihan, R., et al. (2018). Do collagen cross-linkers improve dentin's bonding receptiveness? Dent. Mater 34 (11), 1679–1689. doi:10.1016/j.dental.2018.08.303
Paschoini, V. L., Ziotti, I. R., Neri, C. R., Milori Corona, S. A., and Souza-Gabriel, A. E. (2021). Chitosan improves the durability of resin-dentin interface with etch-and-rinse or self-etch adhesive systems. J. Appl. Oral Sci. 29, e20210356. doi:10.1590/1678-7757-2021-0356
Pashley, D. H., Tay, F. R., Breschi, L., Tjäderhane, L., Carvalho, R. M., Carrilho, M., et al. (2011). State of the art etch-and-rinse adhesives. Dent. Mater 27 (1), 1–16. doi:10.1016/j.dental.2010.10.016
Paulose, N. E., and Fawzy, A. S. (2017). Effect of grape seed extract on the bond strength and durability of resin-dentin interface. J. Adhesion Sci. Technol. 31 (23), 2525–2541. doi:10.1080/01694243.2017.1308304
Paulose, N. E., and Fawzy, A. S. (2018). Effect of carbodiimide on the bond strength and durability of resin-dentin interface. J. Adhesion Sci. Technol. 32. doi:10.1080/01694243.2017.1393927
Perdigão, J., Reis, A., and Loguercio, A. D. (2013). Dentin adhesion and MMPs: A comprehensive review. J. Esthet. Restor. Dent. 25 (4), 219–241. doi:10.1111/jerd.12016
Porto, I., Nascimento, T. G., Oliveira, J. M. S., Freitas, P. H., Haimeur, A., and França, R. (2018). Use of polyphenols as a strategy to prevent bond degradation in the dentin-resin interface. Eur. J. Oral Sci. 126 (2), 146–158. doi:10.1111/eos.12403
Rodrigues, S. B., Collares, F. M., Leitune, V. C., Schneider, L. F., Ogliari, F. A., Petzhold, C. L., et al. (2015). Influence of hydroxyethyl acrylamide addition to dental adhesive resin. Dent. Mater 31 (12), 1579–1586. doi:10.1016/j.dental.2015.10.005
Rouse, B., Chaimani, A., and Li, T. (2017). Network meta-analysis: An introduction for clinicians. Intern Emerg. Med. 12 (1), 103–111. doi:10.1007/s11739-016-1583-7
Sabatini, C., and Pashley, D. H. (2014). Mechanisms regulating the degradation of dentin matrices by endogenous dentin proteases and their role in dental adhesion. A review. Am. J. Dent. 27 (4), 203–214.
Salanti, G., Ades, A. E., and Ioannidis, J. P. (2011). Graphical methods and numerical summaries for presenting results from multiple-treatment meta-analysis: An overview and tutorial. J. Clin. Epidemiol. 64 (2), 163–171. doi:10.1016/j.jclinepi.2010.03.016
Santiago, S. L., Osorio, R., Neri, J. R., de Carvalho, R. M., and Toledano, M. (2013). Effect of the flavonoid epigallocatechin-3-gallate on resin-dentin bond strength. J. Adhesive Dent. 15 (6), 535–540. doi:10.3290/j.jad.a29532
Scheffel, D. L. S., Delgado, C. C., Soares, D. G., Basso, F. G., De Souza Costa, C. A., Pashley, D. H., et al. (2015). Increased durability of resin-dentin bonds following cross-linking treatment. Oper. Dent. 40 (5), 533–539. doi:10.2341/13-211-l
Shrestha, A., and Kishen, A. (2012). The effect of tissue inhibitors on the antibacterial activity of chitosan nanoparticles and photodynamic therapy. J. Endod. 38 (9), 1275–1278. doi:10.1016/j.joen.2012.05.006
Singh, S., Nagpal, R., Tyagi, S. P., and Manuja, N. (2015). Effect of EDTA conditioning and carbodiimide pretreatment on the bonding performance of all-in-one self-etch adhesives. Int. J. Dent. 2015, 1–7. doi:10.1155/2015/141890
Sun, Q., Gu, L., Quan, J. Q., Yu, X., Huang, Z., Wang, R., et al. (2018). Epigallocatechin-3-gallate enhance dentin biomodification and bond stability of an etch-and-rinse adhesive system. Int. J. Adhes. Adhes. 80, 115–121. doi:10.1016/j.ijadhadh.2017.11.001
Tjäderhane, L. (2015). Dentin bonding: Can we make it last? Oper. Dent. 40 (1), 4–18. doi:10.2341/14-095-bl
Tjäderhane, L., Nascimento, F. D., Breschi, L., Mazzoni, A., Tersariol, I. L., Geraldeli, S., et al. (2013). Optimizing dentin bond durability: Control of collagen degradation by matrix metalloproteinases and cysteine cathepsins. Dent. Mater 29 (1), 116–135. doi:10.1016/j.dental.2012.08.004
Valkenhoef, G. V., Lu, G., de Brock, B., Hillege, H., Ades, A., and Welton, N. J. (2012). Automating network meta-analysis. Res. Synth. Methods 3 (4), 285–299. doi:10.1002/jrsm.1054
van Ravenzwaaij, D., Cassey, P., and Brown, S. D. (2018). A simple introduction to Markov chain Monte-Carlo sampling. Psychonomic Bull. Rev. 25 (1), 143–154. doi:10.3758/s13423-016-1015-8
Venigalla, B. S., Jyothi, P., Kamishetty, S., Reddy, S., Cherukupalli, R. C., and Reddy, D. A. (2016). Resin bond strength to water versus ethanol-saturated human dentin pretreated with three different cross-linking agents. J. conservative Dent. JCD 19 (6), 555–559. doi:10.4103/0972-0707.194019
Wollensak, G., Aurich, H., Pham, D. T., and Wirbelauer, C. (2007). Hydration behavior of porcine cornea crosslinked with riboflavin and ultraviolet A. J. cataract Refract. Surg. 33 (3), 516–521. doi:10.1016/j.jcrs.2006.11.015
Yang, H., Guo, J., Deng, D., Chen, Z., and Huang, C. (2016). Effect of adjunctive application of epigallocatechin-3-gallate and ethanol-wet bonding on adhesive-dentin bonds. J. Dent. 44, 44–49. doi:10.1016/j.jdent.2015.12.001
Yang, H., Li, K., Yan, H., Liu, S., Wang, Y., and Huang, C. (2017). High-performance therapeutic quercetin-doped adhesive for adhesive-dentin interfaces. Sci. Rep. 7, 8189. doi:10.1038/s41598-017-08633-3
Yu, H. H., Zhang, L., Yu, F., Li, F., Liu, Z. Y., and Chen, J. H. (2017). Epigallocatechin-3-gallate and epigallocatechin-3-O-(3-O-methyl)-gallate enhance the bonding stability of an etch-and-rinse adhesive to dentin. Mater. (Basel, Switz. 10 (2), 183. doi:10.3390/ma10020183
Yu, S. Y., Zhang, J. H., Li, K. X., Chen, H., Wang, H. M., He, X., et al. (2022). A novel chemical binding primer to improve dentin bonding durability. J. Dent. Res. 101, 777–784. doi:10.1177/00220345221074910
Zhang, Y., Conrad, A. H., and Conrad, G. W. (2011). Effects of ultraviolet-A and riboflavin on the interaction of collagen and proteoglycans during corneal cross-linking. J. Biol. Chem. 286 (15), 13011–13022. doi:10.1074/jbc.m110.169813
Zhang, L., Wang, D. Y., Fan, J., Li, F., Chen, Y. J., and Chen, J. H. (2014). Stability of bonds made to superficial vs. deep dentin, before and after thermocycling. Dent. Mater 30 (11), 1245–1251. doi:10.1016/j.dental.2014.08.362
Zhang, Z., Beitzel, D., Majd, H., Mutluay, M., Tezvergil-Mutluay, A., Tay, F. R., et al. (2016). Effect of carbodiimide on the fatigue crack growth resistance of resin-dentin bonds. Dent. Mater. 32 (2), 211–222. doi:10.1016/j.dental.2015.11.024
Zhao, Y., He, X., Wang, H., Zhu, J., Wang, H., Zheng, Y., et al. (2021). Synthesis of an urushiol derivative and its use for hydrolysis resistance in dentin adhesive. RSC Adv. 11 (30), 18448–18457.
Zhao, S., Hua, F., Yan, J., Yang, H., and Huang, C. (2022). Effects of plant extracts on dentin bonding strength: A systematic review and meta-analysis. Front. Bioeng. Biotechnol. 10, 836042. doi:10.3389/fbioe.2022.836042
Keywords: cross-linkers, dentin adhesives, bonding performance, network meta-analysis, systematic review
Citation: Chen H, Sun G, Wang H, Yu S, Tian Z and Zhu S (2023) Effect of collagen cross-linkers on dentin bond strength: A systematic review and network meta-analysis. Front. Bioeng. Biotechnol. 10:1100894. doi: 10.3389/fbioe.2022.1100894
Received: 17 November 2022; Accepted: 14 December 2022;
Published: 24 January 2023.
Edited by:
Jianxun Ding, Changchun Institute of Applied Chemistry (CAS), ChinaReviewed by:
Rene García Contreras, National Autonomous University of Mexico, MexicoXiaodong Li, Zhejiang University, China
Hector Flores, Autonomous University of San Luis Potosí, Mexico
Copyright © 2023 Chen, Sun, Wang, Yu, Tian and Zhu. This is an open-access article distributed under the terms of the Creative Commons Attribution License (CC BY). The use, distribution or reproduction in other forums is permitted, provided the original author(s) and the copyright owner(s) are credited and that the original publication in this journal is cited, in accordance with accepted academic practice. No use, distribution or reproduction is permitted which does not comply with these terms.
*Correspondence: Song Zhu, emh1c29uZzE5NjVAMTYzLmNvbQ==