- 1Department of Biomaterials, Faculty of Interdisciplinary Science and Technology, Tarbiat Modares University, Tehran, Iran
- 2State Key Laboratory for Modification of Chemical Fibers and Polymer Materials, College of Materials Science and Engineering, Donghua University, Shanghai, China
- 3Department of Chemistry, Carleton University, Ottawa, ON, Canada
- 4National Cell Bank of Iran, Pasteur Institute of Iran, Tehran, Iran
- 5Tissues and Biomaterials Research Group (TBRG), Universal Scientific Education and Research Network (USERN), Tehran, Iran
- 6Department of Pharmacy, Faculty of Medicine and Pharmacy, University of Oradea, Oradea, Romania
- 7Shanghai Key Laboratory for Molecular Engineering of Chiral Drugs, School of Pharmacy, Shanghai Jiao Tong University, Shanghai, China
- 8Department of Natural Product Chemistry, School of Pharmacy, Shanghai Jiao Tong University, Shanghai, China
Bones damaged due to disease or accidents can be repaired in different ways. Tissue engineering has helped with scaffolds made of different biomaterials and various methods. Although all kinds of biomaterials can be useful, sometimes their weakness in cellular activity or osteoconductivity prevents their optimal use in the fabrication of bone scaffolds. To solve this problem, we need additional processes, such as surface modification. One of the common methods is coating with polydopamine. Polydopamine can not only cover the weakness of the scaffolds in terms of cellular properties, but it can also create or increase osteoconductivity properties. Polydopamine creates a hydrophilic layer on the surface of scaffolds due to a large number of functional groups such as amino and hydroxyl groups. This layer allows bone cells to anchor and adheres well to the surfaces. In addition, it creates a biocompatible environment for proliferation and differentiation. Besides, the polydopamine coating makes the surfaces chemically active by catechol and amine group, and as a result of their presence, osteoconductivity increases. In this mini-review, we investigated the characteristics, structure, and properties of polydopamine as a modifier of bone substitutes. Finally, we evaluated the cell adhesion and osteoconductivity of different polydopamine-modified bone scaffolds.
1 Introduction
Bone defects and bone deficiency caused by removing tumors or infections are one of the main problems in orthopedics, oral and maxillofacial, and Implantology surgeries. Bone grafting, therefore, is used as an efficient method for treating and regenerating to stabilize fixtures and implants and complete restoration (Salyer and Taylor, 2009). Bone grafting cannot be used just for treating defected bone but also as a scaffold to improve osteoconductivity and cell adhesion in the purpose area (Lee et al., 2021). Nowadays, there are manifold procedures and new technologies for manufacturing and modification of foreign bone substitutes due to achieve the best results, for example, deproteinization and delipidation of allogenic, and also xenogenic bones (Gardin et al., 2015). A cutting-edge bioengineering method is modifying surfaces of bone substitutes that can improve cell adhesion affinity rate, biodegradability, and biocompatibility (Ghorbani et al., 2019a; Kheilnezhad et al., 2020). Modified bio substitutes such as orthopedic implants that have significantly increased antibacterial and osteoconductive properties by surface modification can play a unique role in promoting bone tissue engineering in the compare with the other materials (Li et al., 2015; Hosseini et al., 2019).
Biopolymers are one of the most potent substances to make a chemical layer to guide cell proliferation, adhesion, and differentiation (Aidun et al., 2019a; 2019b; Ghorbani et al., 2019b). One of the most reliable and functional biopolymers for improving surface properties is PDA (Polydopamine), or polymerized dopamine which has been used as an assistant for surface modification by making a super hydrophilic substrate on a biomaterial interface. This coating is created by an oxidation reaction of dopamine (Lynge et al., 2011). PDA also is comfortably available, and by exposing dopamine to the air in a buffer solution manufactured, and can be linked to metals, oxides, ceramics (Lee et al., 2007), biopolymers (Ghorbani et al., 2019a), carbon nanotubes (Demirci et al., 2021), and magnetic nanoparticles for drug delivery (Si and Yang, 2011; Mrówczyński et al., 2013). Up to now, many structures of PDA have been introduced, but it is fascinating that precise structure has not been ultimately discovered yet (Liebscher et al., 2013).
This article focuses on PDA-modified scaffolds characterization and application for bone tissue regeneration. Firstly, we discussed the properties of different bone substitutes. Furthermore, we report researches carried out on types of microstructures of PDA used for surface modification.
2 Introduction of bone substitutes materials
The bone augmentation process is based on several factors. Osteoblasts, osteoclasts, and their precursors are the provenance of this action (Lischer, 2019). Most bone substitute materials act as a scaffold structure for the osteoconductive ingrowth of osteoblasts and mesenchymal progenitors (SafaeiFiroozabady et al., 2019; Siswanto et al., 2020; Hu J. et al., 2021). Various materials are used in bone tissue engineering as substitutes and scaffolds, either alone such as biopolymers, metals, and ceramics, or in composite form. These fabricated scaffolds sometimes need modification in various ways to improve some properties such as mechanical properties, cellular behavior, or osteoconductivity (Zhang et al., 2017; Ghorbani et al., 2019a; Nosrati et al., 2021).
2.1 Metals
Research showed that metallic materials and their alloys have been accepted as an implant in dentistry and spinal surgery for a few decades (Glenske et al., 2018). Aluminum, in turn, is associated with toxic adverse effects, including anemia, encephalopathy, and osteoporosis (Aaseth et al., 2012; Mozafari et al., 2020). Despite these drawbacks, the composition of aluminum (Al) with Titanium (Ti6Al4V) is widely used as a dental and orthopedic implant. While PDA substrate is formed on Ti6Al4V, a strong interaction between the coating layer and Ti leads to enhanced mechanical properties and corrosive resistance (Hu Y. et al., 2021). Moreover, modification with PDA can increase osteoconductivity and bioactivity properties in metals and their alloys, and also make a more straightforward condition for the differentiation of human mesenchymal stem cells (hMSCs) to endothelial cells (Yu et al., 2014). By immersing the titanium-based scaffolds in prepared dopamine solution for 24 h in the darkroom at room temperature, usually a thin layer of PDA forms on the surface of the scaffolds. The use of oxidation agents can reduce the coating time with PDA. Also, the molar ratio between dopamine and the oxidation agent can bring different characteristics for the scaffold, for example, antibacterial properties or different levels of cytotoxicity. Among oxidizing agents, potassium permanganate (KMnO4) showed possible antipathogenic effects, and in the molar ratio .45:.88 (KMnO4:dopamine) the sample manifested suitable antimicrobial properties, while in the molar ratio .1:1.5 (KMnO4:dopamine) the samples led to more nitric oxide release and being some cytotoxic (Marinas et al., 2021). Li et al. (2019) showed in their study that PDA coating on the 3D printing porous Ti6Al4V scaffolds significantly improves MC3T3-E1 cell attachment after 4 h. They also investigate the osteogenesis by in-vivo evaluation on the adult rabbits within 3–5 kg and the result showed that the PDA-coated group had significantly (p <.01) more newly formed bone tissue and this is evidence of the osteoconductivity of the PDA.
2.2 Biopolymers
Poly (l-lactide-co-glycolide) (PLGA) (Zhang et al., 2017), polycaprolactone (PCL) (Aidun et al., 2019a), poly (L-lactide) (PLLA) (Liu et al., 2019), Poly (methylmethacrylate) (PMMA) (Soleymani Eil Bakhtiari et al., 2021), collagen (Aidun et al., 2019a), polyurethane (Aidun et al., 2019b), and polysaccharides (Aidun et al., 2019c) are biomaterials with various applications. These biomaterials are widely used as substitutes in bone tissue engineering. Although they have suitable biodegrading and biocompatibility, they have no appropriate osteoconductivity (Rocha et al., 2022). Most of them can be used as just bioactive molecules or growth factor carriers (Eap et al., 2015). Research indicated that the properties of their surface can be modified by PDA and the osteoblast growth and after modification by PDA has significantly increased. Ko et al. (2013) in their study showed that PDA can play an important role to immobilize osteoinductive molecules such as growth factors or peptides. They could immobilize bone morphogenetic protein-2 (BMP-2) on the PLGA scaffold by PDA (Ko et al., 2013).
2.3 Ceramics
Ceramics have been used since the 1980s in orthopedics and dentistry and are also made from calcium phosphate (Bohner, 2000). Calcium phosphate ceramics (CPCs) are a kind of bioactive material that has been used for bone tissue engineering. They have a surface that supports cell adhesion, leading to increased osteoconductivity. However, most CPCs have an osteoconductive property but possess different cell growth abilities (Samavedi et al., 2013). Fan et al. (2022) in their study evaluated the effect of the PDA coating and chitosan (CS) coating on the 3D-printed biphasic calcium phosphate (BCP) scaffold. They assessed the cell behavior of the scaffold and showed that MC3T3-E1 cells after 2 days were attached to the BCP/PDA with longer pseudopodia compared with BCP. The Relative gene expression of Runx-2 and COL-1 were significantly (p <.05) higher on BCP/PDA than BCP which indicated that PDA could promote the osteogenic differentiation of MC3T3-E1. The osteoconductivity and mineralization of the scaffold observed and the morphology of the surface mineralization of the scaffolds after 2 weeks showed that the volume of apatite formed in BCP/PDA was more than in BCP.
3 Microstructure and sources of PDA
3.1 Source of PDA
PDA is a polymer inspired by adhesive proteins left in mussel plaques, Because, they are able to adhere to many substances ranging from wood to stones (Ding et al., 2016). Mussel’s plaques are rich in a catechol named 3,4-dihydroxy-L-phenylalanine (DOPA) and lysine amino acids. Therefore, the existence of both amino acids and catechol is the cause of the strong adhesive ability in mussels (Lee et al., 2007). PDA includes both two mentioned groups and Lee et al. (2007) have discovered a method to form PDA coating on surfaces through the simple coating of objects in an aqueous solution of dopamine. Forming of PDA is indicated below.
3.2 Structure of PDA
A large number of structures of PDA are introduced, but to better understand precise PDA structure, the mechanism involved in PDA formulation should be explained. At first, the oxidation of dopamine into dopamine-o-quinone (DQ) made in alkaline PH conditions is noticeable (Velez-Pardo et al., 1995; Lee et al., 2007; Ho and Ding, 2014; Wu et al., 2021). Then, because of the deprotonation of the primary amine, DQ by an irreversible cycle through 1,4 Michael addition converts into leukodopaminechrome (LDC) (Li et al., 2006; Lee et al., 2007). Finally, LDC oxidizes to dopaminechrome (DC) then it isomerizes to 5,6-dihydroxyindole (DHI) before oxidation of DHI to 5,6-indolequinone (IDQ), which is the monomers of PDA (Bisaglia et al., 2007).
The carbon at 2, 3, 4, and 7 conditions in both DHI and IDQ can undergo branching reactions to form different isomers of oligomers and further self-assemble to form PDA. For the oxidation process, solvable oxygen is a required part of these reactions (Ho and Ding, 2014). Although there is a well-defined monomeric progenitor, the precise mechanism of PDA formation is still under discussion, and some researchers believe that the PDA structure might be similar to eumelanin (Watt et al., 2009; Yu et al., 2010; Chen et al., 2013).
4 Super-hydrophilic layer in composites, biopolymers, and ceramics scaffolds with PDA
Dopamine polymerization results in PDA fabrication which can be coated in different substrates including noble metals, oxides, ceramics, biopolymers, and semiconductors. The PDA layer which has a tunable thickness (10–100 nm) could provide valuable properties for coated surfaces including high hydrophilicity, long-term corrosion resistance, and moderate physicochemical properties (Huang et al., 2016). The dopamine concentration, the temperature of the dopamine solution, the speed of the stirrer, oxidative factors such as potassium permanganate, and the scaffold immersion time in the dopamine solution could affect the thickness of the PDA layer and the time of the PDA coating. Based on the literature, The polydopamine layer will be larger at higher concentrations of dopamine, higher solution temperature, and higher stirrer speed (Terrill, 2015; Oymaci et al., 2020).
Wettability of the surface could affect the surface protein adsorption and also cell attachment. Cells tend to attach to the hydrophilic surface and by increasing the contact angle from 0° to 106° the rate of cell attachment decreases and the most rate of fibroblast cell attachment on the surface is between 60°–80° based on the literature. But on the super-hydrophilic surface with a contact angle under 5° is unsuitable for proteins and cells to bind and attach. Super-hydrophilic surfaces may affect the structure, type, and binding strength of the proteins such as albumin, transferrin, and fibronectin from the cell culture medium and this could have a negative effect on the cell attachment (Arora, 2013). Besides, a super-hydrophobic surface with a contact angle of more than 150° could have the same manifestation, and the proteins such as laminin, collagen, and fibronectin in extra cellular matrix adsorbed denatured and this also prohibits cells from adhering to the surfaces (Cai et al., 2020). It has been reported attachment of NIH3T3 fibroblast cells was enhanced following PDA coating (Sun et al., 2011). In another study, Sun et al. (2012) revealed that coating the PDA layer on bio-substitutes could impressively improve the immobilization of rabbit chondrocytes. Table 1 presents more results of other studies.
According to the literature, PDA has a positive effect on increasing the hydrophilicity of the surface of the scaffold. Rezaei et al. (2021) showed in their study that PDA coating could significantly improve the contact angle and absorption capacity (p <.005) of the PLGA-Gelatin (PG) lamellar composite scaffold. The contact angle of the PG scaffold and PDA-coated PG were 61.77° ± .65° and 32.05° ± .86°, respectively. The results of this study indicated that the PDA coating and lamellar structure have a synergistic effect on physicochemical properties such as hydrophilicity.
Recently, PDA is also used specifically in the field of 3D printers for bone tissue engineering. Feng et al. (2021) investigated the effect of the PDA coating in a 3D-printed scaffold. They fabricated the 3D scaffolds with the PDA functionalized PCL powder via a selective laser sintering printer. The results indicated that the scaffolds have abundant catechol groups on the surfaces and also show perfect hydrophilicity because of the specific functional group such as amino and hydroxyl groups.
5 Assessment of osteoconductivity and cell adhesion rate in different types of PDA-modified bone substitutes
Bone grafts, such as autografts, allografts, and xenografts, are frequently used in clinical treatment, although their performance needs to be improved. The optimum scaffold for bio-replacement materials in bone defects intends to promote cell proliferation, osteo-differentiation, and mineralization, which can be achieved by PDA-assisted surface modification (Huang et al., 2016).
A unique, powerful charge transfer interaction could be developed between Ti and the coated surface by applying the PDA layer to the bio-substitutes surface, giving excellent corrosion resistance, higher mechanical properties, and great superficial energy. Furthermore, the PDA-assisted surface modification of Ti and Ti alloys can provide bioinert metallic bone bio-substitutes with osteoinductivity and osteoconductivity, and improve their bioactivities in terms of cell viability, proliferation, and differentiation based on the Yu et al. (2014) study. The result of their study indicated that the PDA and collagen coating have a positive effect on cellular behavior such as cell attachment, proliferation, and also differentiation of the preosteoblasts. The number of cells was significantly (p <.05) higher in PDA-coated groups compared with the control group. The same result was found on the treated surfaces in the ALP activity and the PDA-coated scaffold showed significantly (p <.05) more ALP activity compared with the pristine Ti (Yu et al., 2014).
PDA is one of the mineral inducers that helps to produce mineralized surfaces. With the help of the PDA-coated layer, the hydroxyapatite-coated bio-substitute surface (such as titanium alloy, Ti6Al4V) can immobilize some active biomolecules like BMP-2. These immobilized biomolecules can then be evenly distributed on the surface and show a sustainable release profile when used in a natural environment for bone repair. Cai et al. (2014) showed in their research the role of the PDA in the immobilization of BMP-2 on the surface. They showed the ALP activity was higher significantly in three groups of Ti-PDOP (PDA-grafted Ti6Al4V), Ti-H (Ti-PDOP immersed in the 1.5SBF for 5 days), and Ti-BMP2 (BMP2 peptide absorbed Ti-HA) than in the pristine substrate (p <.05). Also in this research, the number of the adherent cell on the surface of the Ti-BMP2 group was significantly higher than Ti (p <.05, X ± SD, n = 3).
It has been reported that PDA-coated layers can control cellular behaviors, such as enhanced osteoblast cell proliferation and calcium deposition. This impact might be further amplified when growth factors are combined. It has also been widely reported that the PDA coating layer on bio-substitutes improved the immobilization of rabbit chondrocytes; another report stated that PDA coating layers could impressively enhance the attachment of NIH3T3 fibroblast cells (Sun et al., 2012). Moreover, PDA can be employed as a controlled surface modifier to strengthen the scaffold’s cell affinity or biomaterial’s surface selective antifouling (Li et al., 2018). VEGF may also be efficiently immobilized on the Ti-based bio-substitute by the PDA coating layer without impairing VEGF activity for bone implants (Poh et al., 2010). Such layer-by-layer deposited composite bone bio-substitute comprising VEGF, PDA layer, and Ti material significantly promotes endothelial cell adhesion and proliferation. Poh et al. (2010) showed in their study the human dermal microvascular endothelial cells (HDMEC) cell proliferation on the Ti-PDOP-VEGF (VEGFcoated, PDA-grafted Ti) was significantly higher than the cells on the simple Ti (p <.05). Also, the number of cells on the Ti-PDOP-VEGF was about 20% more than the number of attached cells on the pristine Ti which is statistically significant (p <.05). According to this study, HDMEC was used due to the major role of revascularization around the implant. The goal of PDA-assisted surface modification on various metallic surfaces is to introduce novel concepts and processing strategies to improve revascularization during bone regeneration considering various functions obtained by immobilizing different biomolecules.
Synthetic and bioderived biopolymers, such as collagen (Aidun et al., 2019a), starch (Aidun et al., 2019c), and PCL (SafaeiFiroozabady et al., 2019) are another important class of materials used to create bone bio-substitute. Although most polymeric biomaterials have adequate mechanical properties, biodegradability, biocompatibility, and erosion resistance, they do not provide good osteointegration (Rocha et al., 2022). PDA-assisted surface modification can significantly improve the surface properties such as the osteogenesis activity of these biopolymers (Zhou et al., 2017). The ability of osteoblasts to respond to polymeric materials can be markedly enhanced in the presence of immobilized BMP, and the material mineralization can be accelerated in the presence of immobilized growth factors (Pan et al., 2014). Bone filler materials are used in a variety of bone tissue engineering applications, including plastic surgery and bone defects. The cytoaffinity of poly (dimethylsiloxane)-based bone cement can be improved by adding a PDA coating layer to poly (dimethylsiloxane), allowing NIH3T3 fibroblasts’ adherence to the material substrate more easily and thus promoting cell growth (Jun et al., 2014). In a study, Zhong et al. (2020) evaluated the effect of the PDA coating on the 3D-printed titanium for repairing a femoral defect in rabbits. They show on the micro-CT after 12 weeks, the bone volume values of the PDA-coated 3D printed porous titanium (PDA-3D PPT) are significantly higher than the uncoated scaffold and also blank group (p < .05). The uncoated and PDA-coated of the 3D-printed scaffold were illustrated in Figure 1B.
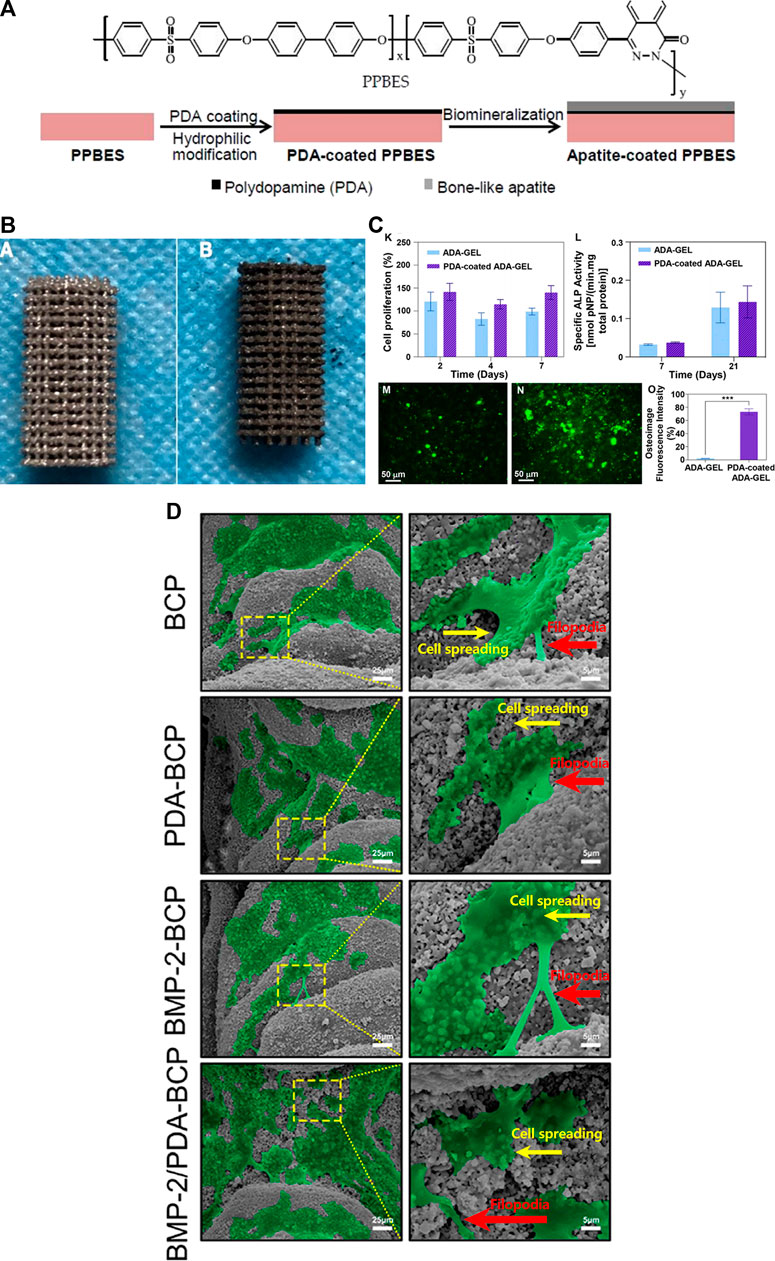
FIGURE 1. (A) The structure of Copoly (phthalazinone biphenyl ether sulfone) (PPBES) and the dopamine polymerization on the PPBES, and finally apatite biomineralization on the surface. [reproduced content is open access- Attribution 4.0 International (CC BY 4.0)] (Liu et al., 2018). (B) The image of the 3D-printed porous titanium (3D PPT) (a), and the PDA coating on 3D-printed porous titanium (PDA-3D PPT) (b). (reproduced content is open access- Attribution 4.0 International (CC BY 4.0)) (Zhong et al., 2020). (C) The proliferation of the MG-63 osteoblast-like cells on the ADA-GEL, and PDA-coated ADA-GEL scaffolds at the 2, 4, 7 days. Although the proliferation of coated scaffolds increased, this increase was not significant (K), The ALP activity of the ADA-GEL, and PDA-coated ADA-GEL scaffolds at the 7, and 21 days. Although the ALP activity of coated scaffolds increased, this increase was not significant (L). (reproduced content is open access- Attribution 4.0 International (CC BY 4.0)) (Ghorbani et al., 2022). (D) Osteoimage mineralization of the uncoated ADA-GEL scaffold (M) and PDA-coated ADA-GEL scaffold (N). The result of the quantitive osteoimage mineralization. The result was significant (***p <.001) (reproduced content is open access- Attribution 4.0 International (CC BY 4.0)) (Yang et al., 2022).
Besides that, the developed PDA coating layer may provide tunable regulating features by mediating numerous parameters, such as multiple cell lines, PDA preparation, and cultivation of cells under various settings. For example, altering the dopamine (PDA precursor) incubation temperature may augment the ratio of quinone to phenolic hydroxyl groups, which will help control the proliferation of smooth muscle cells linked to the bio-substitutes (Luo et al., 2013). Ghorbani et al. (2022) indicated the role of quinone in their study. They showed that the PDA-coated alginate dialdehyde-gelatin (ADA-GEL) is functionalized by various groups which makes the surface of the 3D printed scaffold suitable for biological properties such as cell attachment and proliferation. The results of osteoimage mineralization in the PDA-coated group were significantly higher than the uncoated scaffold after 21 days of cell culture (***p <.001) (Figure 1B). Osteoimage fluorescence intensity of ADA-Gel and PDA-coated ADA-Gel showed 2.5% and 75%, respectively. Although the MG-63 proliferation and also ALP activity of the PDA-coated groups are higher than the uncoated group, these increases were not statistically significant. Due to the fact that the ALP activity plays a crucial role in the preosteoblastic stage of bone cell differentiation, this could be an indicator of osteoblastic differentiation and osteoconductivity of the scaffold (Ghorbani et al., 2020).
Growth factors, which are sensitive biomolecules easily rendered inactive by environmental changes, can be immobilized on PDA-coated bio-substitutes with little or no effect on their functions. The generated PDA coating layer allows growth factors like BMP (Zhang et al., 2017), and Arg-GlyAsp peptide (RGD) (Jeong et al., 2011) to bind with the surface of bio-substitutes. According to studies (Jeong et al., 2011; Cho et al., 2014; Ghorbani et al., 2019a), only the PDA coating layer forming conditions and the inherent characteristics of biomolecules (incubation time, concentration, molecule weight, and isoelectric point) had an impact on the activity of these biomolecules. PDA-assisted immobilization has the potential to significantly improve biomolecules’ ability to anchor when compared to conventional immobilization methods (Vanderleyden et al., 2014). The specific functional groups such as amino and hydroxyl could enhance the proliferation of the MG-63 cells and also cell attachment based on the Feng et al. (2021) study. They pointed out that the presence of these functional groups is due to the presence of catechol and amine groups in primary PDA. Besides, the abundance of catecholamine moieties in PDA has a special role in osteoconductivity. The mechanisms of osteogenesis virtually are related to the catecholamine’s location on the interfaces and also the Ca2+ ion binder which forms and expedites the following hydroxyapatite crystals on the surface of the PDA-coated materials (Ghalandari et al., 2021). PDA functionalize chemically the surface and also plays an important role in creating new hydroxyapatite nuclei on the surfaces of the scaffold (Larrañaga et al., 2015).
6 Conclusion and discussion
According to the superb physicochemical and biological properties of PDA, it could use as a scaffold surface modifier in bone tissue engineering. PDA is virtually a suitable material to coat the surface of the scaffold. Based on numerous studies, it enhances osteoconductivity and also cell adhesion and could be a game changer, especially in bone tissue engineering. Since the properties of PDA are excellent for bone tissue engineering, most of the performed studies are at the in-vitro and in-vivo stages. The main limitation is that there is a lack of clinical studies on this subject until now. With the increase in the number of pre-clinical studies on this functional material in the field of bone tissue engineering, more information will be obtained, which will be of great help in conducting clinical studies. A large number of studies show that PDA has the potential to be used in coating different types of dental implants. It also has a high potential in the treating and healing of bone fractures with the help of various scaffolds such as PDA-coated 3D scaffolds. Looking at the limitations and potentials for the use of PDA, there is a bright future for it.
Author contributions
Conceptualization, AA, AM, SB, and SS; methodology, AA, AM, and SS; validation, AA, AM, MS-K, BH, SB, and MA; data curation, AA, AM, MS-K, BH, and MA; writing “original draft preparation, AA, AM, MS-K, BH, and MA; writing” review and editing, AA, SB, and SS; All authors have read and agreed to the published version of the manuscript.
Funding
The APC was funded by the University of Oradea, and the research was funded by the Romanian Ministry of Research, Innovation, and Digitization through Program 1-Development of the National Research and Development System, Subprogram 1.2—Institutional Performance Projects for funding the excellence in RDI, Contract No. 29 PFE/30.12.2021 with the University of Oradea, Oradea, Romania.
Acknowledgments
The authors thank the University of Oradea, Oradea, Romania, for financial support in publishing this paper.
Conflict of interest
The authors declare that the research was conducted in the absence of any commercial or financial relationships that could be construed as a potential conflict of interest.
The reviewer MM declared a shared affiliation with the author(s) AA at the time of review.
Publisher’s note
All claims expressed in this article are solely those of the authors and do not necessarily represent those of their affiliated organizations, or those of the publisher, the editors and the reviewers. Any product that may be evaluated in this article, or claim that may be made by its manufacturer, is not guaranteed or endorsed by the publisher.
References
Aaseth, J., Boivin, G., and Andersen, O. (2012). Osteoporosis and trace elements - an overview. J. Trace Elem. Med. Biol. 26, 149–152. doi:10.1016/j.jtemb.2012.03.017
Aidun, A., Safaei Firoozabady, A., Moharrami, M., Ahmadi, A., Haghighipour, N., Bonakdar, S., et al. (2019a). Graphene oxide incorporated polycaprolactone/chitosan/collagen electrospun scaffold: Enhanced osteogenic properties for bone tissue engineering. Artif. Organs 43, E264-E281–E281. doi:10.1111/aor.13474
Aidun, A., Zamanian, A., and Ghorbani, F. (2019b). Immobilization of polyvinyl alcohol-siloxane on the oxygen plasma-modified polyurethane-carbon nanotube composite matrix. J. Appl. Polym. Sci. 137, 48477. doi:10.1002/app.48477
Aidun, A., Zamanian, A., and Ghorbani, F. (2019c). Novel bioactive porous starch–siloxane matrix for bone regeneration: Physicochemical, mechanical, and in vitro properties. Biotechnol. Appl. Biochem. 66, 43–52. doi:10.1002/bab.1694
Bisaglia, M., Mammi, S., and Bubacco, L. (2007). Kinetic and structural analysis of the early oxidation products of dopamine: Analysis of the interactions with α-synuclein. J. Biol. Chem. 282, 15597–15605. doi:10.1074/jbc.M610893200
Bohner, M. (2000). Calcium orthophosphates in medicine: From ceramics to calcium phosphate cements. Injury 31, D37–D47. doi:10.1016/S0020-1383(00)80022-4
Cai, S., Wu, C., Yang, W., Liang, W., Yu, H., and Liu, L. (2020). Recent advance in surface modification for regulating cell adhesion and behaviors. Nanotechnol. Rev. 9, 971–989. doi:10.1515/ntrev-2020-0076
Cai, Y., Wang, X., Poh, C. K., Tan, H. C., Soe, M. T., Zhang, S., et al. (2014). Accelerated bone growth in vitro by the conjugation of BMP2 peptide with hydroxyapatite on titanium alloy. Colloids Surfaces B Biointerfaces 116, 681–686. doi:10.1016/j.colsurfb.2013.11.004
Cao, X., Liu, X., Liu, Y., Ma, R., and Sun, S. (2021). The effect of curvature on chondrocytes migration and bone mesenchymal stem cells differentiation. J. Appl. Polym. Sci. 138, 50392. doi:10.1002/app.50392
Chen, C. T., Ball, V., De Almeida Gracio, J. J., Singh, M. K., Toniazzo, V., Ruch, D., et al. (2013). Self-assembly of tetramers of 5, 6-dihydroxyindole explains the primary physical properties of eumelanin: Experiment, simulation, and design. ACS Nano 7, 1524–1532. doi:10.1021/nn305305d
Cho, H. J., Madhurakkat Perikamana, S. K., Lee, J. H., Lee, J., Lee, K. M., Shin, C. S., et al. (2014). Effective immobilization of BMP-2 mediated by polydopamine coating on biodegradable nanofibers for enhanced in vivo bone formation. ACS Appl. Mat. Interfaces 6, 11225–11235. doi:10.1021/am501391z
Demirci, S., Sahiner, M., Suner, S. S., and Sahiner, N. (2021). Improved biomedical properties of polydopamine-coated carbon nanotubes. Micromachines 12, 1280. doi:10.3390/mi12111280
Ding, Y. H., Floren, M., and Tan, W. (2016). Mussel-inspired polydopamine for bio-surface functionalization. Biosurface Biotribology 2, 121–136. doi:10.1016/j.bsbt.2016.11.001
Eap, S., Keller, L., Schiav, J., Huck, O., Jacomine, L., Fioretti, F., et al. (2015). A living thick nanofibrous implant bifunctionalized with active growth factor and stem cells for bone regeneration. Int. J. Nanomedicine 10, 1061–1075. doi:10.2147/IJN.S72670
Fan, S., Wan, Y., Zhao, Z., Wang, H., and Ji, Z. (2022). Biological evaluation of polydopamine and chitosan composite coatings on the 3D printed porous biphasic calcium phosphate scaffold. Ceram. Int. 48, 27942–27956. doi:10.1016/J.CERAMINT.2022.06.098
Feng, P., Liu, M., Peng, S., Bin, S., Zhao, Z., and Shuai, C. (2021). Polydopamine modified polycaprolactone powder for fabrication bone scaffold owing intrinsic bioactivity. J. Mat. Res. Technol. 15, 3375–3385. doi:10.1016/J.JMRT.2021.09.137
Feng, Y., Ma, X., Chang, L., Zhu, S., and Guan, S. (2017). Characterization and cytocompatibility of polydopamine on MAO-HA coating supported on Mg-Zn-Ca alloy. Surf. Interface Anal. 49, 1115–1123. doi:10.1002/sia.6286
Gardin, C., Ricci, S., Ferroni, L., Guazzo, R., Sbricoli, L., De Benedictis, G., et al. (2015). Decellularization and delipidation protocols of bovine bone and pericardium for bone grafting and guided bone regeneration procedures. PLoS One 10, e0132344. doi:10.1371/journal.pone.0132344
Ghalandari, B., Yu, Y., Ghorbani, F., Warden, A. R., Ahmad, K. Z., Sang, X., et al. (2021). Polydopamine nanospheres coated with bovine serum albumin permit enhanced cell differentiation: Fundamental mechanism and practical application for protein coating formation. Nanoscale 13, 20098–20110. doi:10.1039/d1nr07469e
Ghorbani, F., Kim, M., Monavari, M., Ghalandari, B., and Boccaccini, A. R. (2022). Mussel-inspired polydopamine decorated alginate dialdehyde-gelatin 3D printed scaffolds for bone tissue engineering application. Front. Bioeng. Biotechnol. 10, 940070. doi:10.3389/fbioe.2022.940070
Ghorbani, F., Zamanian, A., and Aidun, A. (2019a). Bioinspired polydopamine coating-assisted electrospun polyurethane-graphene oxide nanofibers for bone tissue engineering application. J. Appl. Polym. Sci. 136, 47656–47659. doi:10.1002/app.47656
Ghorbani, F., Zamanian, A., and Aidun, A. (2019b). Conductive electrospun polyurethane-polyaniline scaffolds coated with poly(vinyl alcohol)-GPTMS under oxygen plasma surface modification. Mat. Today Commun. 22, 100752. doi:10.1016/j.mtcomm.2019.100752
Ghorbani, F., Zamanian, A., and Torabinejad, B. (2020). The effect of oxygen plasma pretreatment on the properties of mussel-inspired polydopamine-decorated polyurethane nanofibers. J. Polym. Eng. 40, 109–119. doi:10.1515/polyeng-2019-0219
Glenske, K., Donkiewicz, P., Köwitsch, A., Milosevic-Oljaca, N., Rider, P., Rofall, S., et al. (2018). Applications of metals for bone regeneration. Int. J. Mol. Sci. 19, 826. doi:10.3390/ijms19030826
Ho, C. C., and Ding, S. J. (2014). Structure, properties and applications of mussel-inspired polydopamine. J. Biomed. Nanotechnol. 10, 3063–3084. doi:10.1166/jbn.2014.1888
Hosseini, F. S. F. S., Soleimanifar, F., Aidun, A., Enderami, S. E. S. E., Saburi, E., Marzouni, H. Z. H. Z., et al. (2019). Poly (3-hydroxybutyrate-co-3-hydroxyvalerate) improved osteogenic differentiation of the human induced pluripotent stem cells while considered as an artificial extracellular matrix. J. Cell. Physiol 234, 11537–11544. doi:10.1002/jcp.27807
Hu, J., Wang, Z., Miszuk, J. M., Zhu, M., Lansakara, T. I., Tivanski, A. V., et al. (2021a). Vanillin-bioglass cross-linked 3D porous chitosan scaffolds with strong osteopromotive and antibacterial abilities for bone tissue engineering. Carbohydr. Polym. 271, 118440. doi:10.1016/j.carbpol.2021.118440
Hu, Y., Li, S., Kang, W., Lin, H., and Hu, Y. (2021b). Surface modification of Ti6Al4V alloy by polydopamine grafted GO/ZnO nanocomposite coating. Surf. Coatings Technol. 422, 127534. doi:10.1016/j.surfcoat.2021.127534
Huang, S., Liang, N., Hu, Y., Zhou, X., and Abidi, N. (2016). Polydopamine-assisted surface modification for bone biosubstitutes. Biomed. Res. Int. 2016, 1–9. doi:10.1155/2016/2389895
Jeong, K. J., Wang, L., Stefanescu, C. F., Lawlor, M. W., Polat, J., Dohlman, C. H., et al. (2011). Polydopamine coatings enhance biointegration of a model polymeric implant. Soft Matter 7, 8305. doi:10.1039/c1sm05918a
Jun, D. R., Moon, S. K., and Choi, S. W. (2014). Uniform polydimethylsiloxane beads coated with polydopamine and their potential biomedical applications. Colloids Surfaces B Biointerfaces 121, 395–399. doi:10.1016/j.colsurfb.2014.06.027
Kheilnezhad, B., Firoozabady, A. S., and Aidun, A. (2020). An overview of polyaniline in tissue engineering. J. Tissues Mat. 3, 6. doi:10.22034/jtm.2020.142186
Ko, E., Yang, K., Shin, J., and Cho, S. W. (2013). Polydopamine-assisted osteoinductive peptide immobilization of polymer scaffolds for enhanced bone regeneration by human adipose-derived stem cells. Biomacromolecules 14, 3202–3213. doi:10.1021/bm4008343
Larrañaga, A., Ramos, D., Amestoy, H., Zuza, E., and Sarasua, J. R. (2015). Coating of bioactive glass particles with mussel-inspired polydopamine as a strategy to improve the thermal stability of poly(l-lactide)/bioactive glass composites. RSC Adv. 5, 65618–65626. doi:10.1039/c5ra09495j
Lee, D. J., Lee, Y. T., Zou, R., Daniel, R., and Ko, C. C. (2017). Polydopamine-laced biomimetic material stimulation of bone marrow derived mesenchymal stem cells to promote osteogenic effects. Sci. Rep. 7, 12984. doi:10.1038/s41598-017-13326-y
Lee, D. K., Ki, M. R., Kim, E. H., Park, C. J., Ryu, J. J., Jang, H. S., et al. (2021). Biosilicated collagen/β-tricalcium phosphate composites as a BMP-2-delivering bone-graft substitute for accelerated craniofacial bone regeneration. Biomater. Res. 25, 13. doi:10.1186/s40824-021-00214-w
Lee, H., Dellatore, S. M., Miller, W. M., and Messersmith, P. B. (2007). Mussel-inspired surface chemistry for multifunctional coatings. Sci. (80-. ) 318, 426–430. doi:10.1126/science.1147241
Li, L., Li, Y., Yang, L., Yu, F., Zhang, K., Jin, J., et al. (2019). Polydopamine coating promotes early osteogenesis in 3D printing porous Ti6Al4V scaffolds. Ann. Transl. Med. 7, 240. doi:10.21037/atm.2019.04.79
Li, Y., Liu, M., Xiang, C., Xie, Q., and Yao, S. (2006). Electrochemical quartz crystal microbalance study on growth and property of the polymer deposit at gold electrodes during oxidation of dopamine in aqueous solutions. Thin Solid Films 497, 270–278. doi:10.1016/j.tsf.2005.10.048
Li, Y., Shi, S., Cao, H., Zhao, Z., Su, C., and Wen, H. (2018). Improvement of the antifouling performance and stability of an anion exchange membrane by surface modification with graphene oxide (GO) and polydopamine (PDA). J. Memb. Sci. 566, 44–53. doi:10.1016/j.memsci.2018.08.054
Li, Y., Yang, W., Li, X., Zhang, X., Wang, C., Meng, X., et al. (2015). Improving osteointegration and osteogenesis of three-dimensional porous Ti6Al4V scaffolds by polydopamine-assisted biomimetic hydroxyapatite coating. ACS Appl. Mat. Interfaces 7, 5715–5724. doi:10.1021/acsami.5b00331
Liebscher, J., Mrówczyński, R., Scheidt, H. A., Filip, C., Haìdade, N. D., Turcu, R., et al. (2013). Structure of polydopamine: A never-ending story? Langmuir 29, 10539–10548. doi:10.1021/la4020288
Lischer, C. (2019). Tissue particularities and problems related to bone healing and fracture repair. Ciencias Vet. 37, 13–17. doi:10.15359/rcv.37-3.4
Liu, C., Li, Y., Wang, J., Liu, C., Liu, W., and Jian, X. (2018). Improving hydrophilicity and inducing bone-like apatite formation on PPBES by polydopamine coating for biomedical application. Molecules 23, 1643. doi:10.3390/molecules23071643
Liu, Y., Xu, C., Gu, Y., Shen, X., Zhang, Y., Li, B., et al. (2019). Polydopamine-modified poly(L-lactic acid) nanofiber scaffolds immobilized with an osteogenic growth peptide for bone tissue regeneration. RSC Adv. 9, 11722–11736. doi:10.1039/c8ra08828d
Luo, R., Tang, L., Zhong, S., Yang, Z., Wang, J., Weng, Y., et al. (2013). In vitro investigation of enhanced hemocompatibility and endothelial cell proliferation associated with quinone-rich polydopamine coating. ACS Appl. Mat. Interfaces 5, 1704–1714. doi:10.1021/am3027635
Lynge, M. E., Van Der Westen, R., Postma, A., and Städler, B. (2011). Polydopamine - a nature-inspired polymer coating for biomedical science. Nanoscale 3, 4916. doi:10.1039/c1nr10969c
Marinas, I. C., Tihauan, B. M., Diaconu, A. G., Filip, X., Petran, A., Grosu, I. G., et al. (2021). Polydopamine-assisted surface modification of Ti-6Al-4V alloy with anti-biofilm activity for dental implantology applications. Coatings 11, 1385. doi:10.3390/coatings11111385
Meng, Z., Liu, Y., Xu, K., Sun, X., Yu, Q., Wu, Z., et al. (2021). Biomimetic polydopamine-modified silk fibroin/curcumin nanofibrous scaffolds for chemo-photothermal therapy of bone tumor. ACS Omega 6, 22213–22223. doi:10.1021/acsomega.1c02903
Mozafari, M., Bordbar-Khiabani, A., and Yarmand, B. (2020). Emerging magnesium-based biomaterials for orthopedic implantation. Emerg. Mat. Res. 8, 305–319. doi:10.1680/jemmr.18.00048
Mrówczyński, R., Turcu, R., Leostean, C., Scheidt, H. A., and Liebscher, J. (2013). New versatile polydopamine coated functionalized magnetic nanoparticles. Mat. Chem. Phys. 138, 295–302. doi:10.1016/j.matchemphys.2012.11.059
Nosrati, H., Sarraf-Mamoory, R., Zolfaghari Emameh, R., Aidun, A., and Canillas Perez, M. (2021). Enhancing mechanical properties of hydroxyapatite-reduced graphene oxide nanocomposites by increasing the spark plasma sintering temperature. Inorg. Nano-Metal Chem., 1–11. doi:10.1080/24701556.2020.1852251
Oymaci, P., Nijmeijer, K., and Borneman, Z. (2020). Development of polydopamine forward osmosis membranes with low reverse salt flux. Membr. (Basel) 10, 94. doi:10.3390/membranes10050094
Pan, H., Zheng, Q., Yang, S., and Guo, X. (2014). Effects of functionalization of PLGA-[Asp-PEG]n copolymer surfaces with Arg-Gly-Asp peptides, hydroxyapatite nanoparticles, and BMP-2-derived peptides on cell behavior in vitro. J. Biomed. Mat. Res. - Part A. 102, 4526–4535. doi:10.1002/jbm.a.35129
Poh, C. K., Shi, Z., Lim, T. Y., Neoh, K. G., and Wang, W. (2010). The effect of VEGF functionalization of titanium on endothelial cells in vitro. Biomaterials 31, 1578–1585. doi:10.1016/j.biomaterials.2009.11.042
Rezaei, H., Shahrezaee, M., Jalali Monfared, M., Ghorbani, F., Zamanian, A., and Sahebalzamani, M. (2021). Mussel-inspired polydopamine induced the osteoinductivity to ice-templating PLGA–gelatin matrix for bone tissue engineering application. Biotechnol. Appl. Biochem. 68, 185–196. doi:10.1002/bab.1911
Rocha, C. V., Gonçalves, V., da Silva, M. C., Bañobre-López, M., and Gallo, J. (2022). PLGA-based composites for various biomedical applications. Int. J. Mol. Sci. 23, 2034. doi:10.3390/ijms23042034
SafaeiFiroozabady, A., Aidun, A., Kowsari-Esfahan, R., and Allahyari, A. (2019). Characterization and evaluation of graphene oxide incorporated into nanofibrous scaffold for bone tissue engineering. J. Tissues Mat. 2, 1–13. doi:10.22034/jtm.2019.83189
Salyer, K. E., and Taylor, D. P. (2009). Bone grafts in craniofacial surgery. Craniomaxillofac. Trauma Reconstr. 2, 125–134. doi:10.1055/S-0029-1215875
Samavedi, S., Whittington, A. R., and Goldstein, A. S. (2013). Calcium phosphate ceramics in bone tissue engineering: A review of properties and their influence on cell behavior. Acta Biomater. 9, 8037–8045. doi:10.1016/j.actbio.2013.06.014
Si, J., and Yang, H. (2011). Preparation and characterization of bio-compatible Fe3O 4@Polydopamine spheres with core/shell nanostructure. Mat. Chem. Phys. 128, 519–524. doi:10.1016/j.matchemphys.2011.03.039
Siswanto, S., Hikmawati, D., Kulsum, U., Rudyardjo, D. I., Apsari, R., and Aminatun, A. (2020). Biocompatibility and osteoconductivity of scaffold porous composite collagen-hydroxyapatite based coral for bone regeneration. Open Chem. 18, 584–590. doi:10.1515/chem-2020-0080
Soleymani Eil Bakhtiari, S., Bakhsheshi-Rad, H. R., Karbasi, S., Tavakoli, M., Hassanzadeh Tabrizi, S. A., Ismail, A. F., et al. (2021). Poly(methyl methacrylate) bone cement, its rise, growth, downfall and future. Polym. Int. 70, 1182–1201. doi:10.1002/pi.6136
Sun, K., Song, L., Xie, Y., Liu, D., Wang, D., Wang, Z., et al. (2011). Using self-polymerized dopamine to modify the antifouling property of oligo(ethylene glycol) self-assembled monolayers and its application in cell patterning. Langmuir 27, 5709–5712. doi:10.1021/la2012099
Sun, K., Xie, Y., Ye, D., Zhao, Y., Cui, Y., Long, F., et al. (2012). Mussel-inspired anchoring for patterning cells using polydopamine. Langmuir 28, 2131–2136. doi:10.1021/la2041967
Vanderleyden, E., Van Bael, S., Chai, Y. C., Kruth, J. P., Schrooten, J., and Dubruel, P. (2014). Gelatin functionalised porous titanium alloy implants for orthopaedic applications. Mat. Sci. Eng. C 42, 396–404. doi:10.1016/j.msec.2014.05.048
Velez-Pardo, C., Jimenez del Rio, M., Ebinger, G., and Vauquelin, G. (1995). Manganese and copper promote the binding of dopamine to “serotonin binding proteins” in bovine frontal cortex. Neurochem. Int. 26, 615–622. doi:10.1016/0197-0186(94)00163-O
Wang, H., Lin, C., Zhang, X., Lin, K., Wang, X., and Shen, S. G. (2019). Mussel-inspired polydopamine coating: A general strategy to enhance osteogenic differentiation and osseointegration for diverse implants. ACS Appl. Mat. Interfaces 11, 7615–7625. doi:10.1021/acsami.8b21558
Watt, A. A. R., Bothma, J. P., and Meredith, P. (2009). The supramolecular structure of melanin. Soft Matter 5, 3754. doi:10.1039/b902507c
Wu, D., Zhou, J., Creyer, M. N., Yim, W., Chen, Z., Messersmith, P. B., et al. (2021). Phenolic-enabled nanotechnology: Versatile particle engineering for biomedicine. Chem. Soc. Rev. 50, 4432–4483. doi:10.1039/d0cs00908c
Xu, Y., Li, H., Wu, J., Yang, Q., Jiang, D., and Qiao, B. (2018). Polydopamine-induced hydroxyapatite coating facilitates hydroxyapatite/polyamide 66 implant osteogenesis: An in vitro and in vivo evaluation. Int. J. Nanomedicine. 13, 8179–8193. doi:10.2147/IJN.S181137
Yang, Z., Xie, L., Zhang, B., Zhang, G., Huo, F., Zhou, C., et al. (2022). Preparation of BMP-2/PDA-BCP bioceramic scaffold by DLP 3D printing and its ability for inducing continuous bone formation. Front. Bioeng. Biotechnol. 10, 854693. doi:10.3389/fbioe.2022.854693
Yu, F., Chen, S., Chen, Y., Li, H., Yang, L., Chen, Y., et al. (2010). Experimental and theoretical analysis of polymerization reaction process on the polydopamine membranes and its corrosion protection properties for 304 Stainless Steel. J. Mol. Struct. 982, 152–161. doi:10.1016/j.molstruc.2010.08.021
Yu, X., Walsh, J., and Wei, M. (2014). Covalent immobilization of collagen on titanium through polydopamine coating to improve cellular performances of MC3T3-E1 cells. RSC Adv. 4, 7185. doi:10.1039/c3ra44137g
Zhang, J., Li, J., Jia, G., Jiang, Y., Liu, Q., Yang, X., et al. (2017). Improving osteogenesis of PLGA/HA porous scaffolds based on dual delivery of BMP-2 and IGF-1: Via a polydopamine coating. RSC Adv. 7, 56732–56742. doi:10.1039/c7ra12062a
Zhong, W., Li, J., Hu, C., Quan, Z., Jiang, D., Huang, G., et al. (2020). 3D-printed titanium implant-coated polydopamine for repairing femoral condyle defects in rabbits. J. Orthop. Surg. Res. 15, 102. doi:10.1186/s13018-020-01593-x
Zhou, J., Guo, X., Zheng, Q., Wu, Y., Cui, F., and Wu, B. (2017). Improving osteogenesis of three-dimensional porous scaffold based on mineralized recombinant human-like collagen via mussel-inspired polydopamine and effective immobilization of BMP-2-derived peptide. Colloids Surfaces B Biointerfaces 152, 124–132. doi:10.1016/j.colsurfb.2016.12.041
Keywords: biomaterials, scaffold, tissue engineering, bone, polydopamine, surface modification, cell adhesion, osteoconductivity
Citation: Mahnavi A, Shahriari-Khalaji M, Hosseinpour B, Ahangarian M, Aidun A, Bungau S and Hassan SSu (2023) Evaluation of cell adhesion and osteoconductivity in bone substitutes modified by polydopamine. Front. Bioeng. Biotechnol. 10:1057699. doi: 10.3389/fbioe.2022.1057699
Received: 29 September 2022; Accepted: 30 December 2022;
Published: 13 January 2023.
Edited by:
Farnaz Ghorbani, University of Erlangen Nuremberg, GermanyReviewed by:
Behrad Shaghaghi, Radboud University, NetherlandsMorteza Mehrjoo, Pasteur Institute of Iran (PII), Iran
Melika Sahranavard, Materials and Energy Research Center, Iran
Copyright © 2023 Mahnavi, Shahriari-Khalaji, Hosseinpour, Ahangarian, Aidun, Bungau and Hassan. This is an open-access article distributed under the terms of the Creative Commons Attribution License (CC BY). The use, distribution or reproduction in other forums is permitted, provided the original author(s) and the copyright owner(s) are credited and that the original publication in this journal is cited, in accordance with accepted academic practice. No use, distribution or reproduction is permitted which does not comply with these terms.
*Correspondence: Amir Aidun, a_aidun@aut.ac.ir; Simona Bungau, simonabungau@gmail.com; Syed Shams ul Hassan, shams1327@yahoo.com