- 1Tianjin Key Laboratory of Pulp and Paper, Tianjin University of Science and Technology, Tianjin, China
- 2Guangxi Key Laboratory of Clean Pulp & Papermaking and Pollution Control, College of Light Industry and Food Engineering, Guangxi University, Nanning, China
- 3Department of Medicinal Bioscience, Konkuk University (Glocal Campus), Chungju-si, Chungcheongbuk-do, South Korea
- 4Department of Forest Biomaterials Engineering, College of Forest & Environmental Sciences, Kangwon National University, Chuncheon, South Korea
- 5State Key Laboratory of Tree Genetics and Breeding, Northeast Forestry University, Harbin, China
With the emerging of the problems of environmental pollution and energy crisis, the development of high-efficiency energy storage technology and green renewable energy is imminent. Supercapacitors have drawn great attention in wearable electronics because of their good performance and portability. Electrodes are the key to fabricate high-performance supercapacitors with good electrochemical properties and flexibility. As a biomass based derived material, nanocellulose has potential application prospects in supercapacitor electrode materials due to its biodegradability, high mechanical strength, strong chemical reactivity, and good mechanical flexibility. In this review, the research progress of nanocellulose/two dimensional nanomaterials composites is summarized for supercapacitors in recent years. First, nanocellulose/MXene composites for supercapacitors are reviewed. Then, nanocellulose/graphene composites for supercapacitors are comprehensively elaborated. Finally, we also introduce the current challenges and development potential of nanocellulose/two dimensional nanomaterials composites in supercapacitors.
1 Introduction
The emission of greenhouse gases and the depletion of fossil fuels have led to an increasing global demand for renewable energy, which has promoted human beings to invest more and more funds in the development, utilization, and storage of new energy (Gross et al., 2003; Lund, 2007; Si et al., 2009a; Si et al., 2013a; Du et al., 2016; Dai et al., 2019; Yang et al., 2019a; Yang et al., 2020; Liu et al., 2021a; Xiong et al., 2021a; Huang et al., 2021; Liu et al., 2022a; Xu et al., 2022). As an advanced energy storage technology, supercapacitors have been widely used in portable electronic products, memory backup, electric vehicles, and military equipment, due to their environmental friendliness, high power density, maintenance-free, and long-life properties (Si et al., 2008; Si et al., 2009b; Si et al., 2013b; Dubal et al., 2015; Salunkhe et al., 2016a; Salunkhe et al., 2016b; Salunkhe et al., 2017; Xu et al., 2021a; Liu et al., 2021b; Xiong et al., 2021b; Liu et al., 2021c; Liu et al., 2021d; Ma et al., 2021). Hybrid capacitors, pseudocapacitors, and Electric double-layer capacitors (EDLCs) can be distinguished depending on the storage mechanism or cell configuration. The key characteristic of EDLCs is the double-layer capacitance, for example, it generates at the interface between the liquid electrolyte and the adjacent conductive electrode. At this boundary, two layers of charges of opposite polarity are formed, one in the electrolyte and the other on the electrode surface (Zhang and Zhao, 2009; Zhai et al., 2011; Qiang et al., 2021; Zhang et al., 2021). Figure 1A shows the working mechanism of EDLCs during the charging and discharging process. When charging, the positive and negative electrodes generate a stable electric field. The cathode attracts the anions in the electrolyte, and the anode attracts the cations in the electrolyte to generate an electric double layer; when discharging, the anions and cations in the electrolyte return to the electrolyte to form an electrically neutral solution. As shown in Figure 1B, pseudocapacitors store charges through redox reactions, electrosorption, or intercalation mechanisms (Conway et al., 1997; Zhao et al., 2011; Augustyn et al., 2014). These electrochemical reactions allow pseudocapacitors to achieve higher specific capacitance than EDLCs. Hybrid capacitors have two different electrodes, one electrode mainly exhibits electrostatic capacitance and the other electrode mostly exhibits electrochemical capacitance (Figure 1C) (Ma et al., 2007).
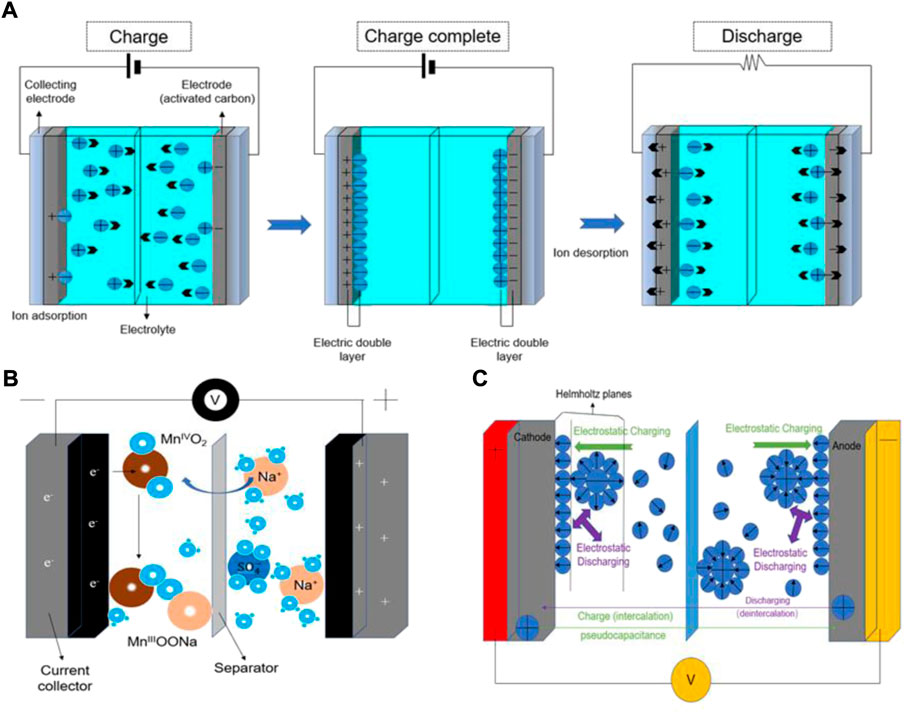
FIGURE 1. The working mechanism of supercapacitors. (A) Working principle of EDLC during charging and discharging, (B) pseudocapacitor, and (C) hybrid capacitor.
Cellulose, as the most abundant natural polymer on Earth, consists of hundreds of strands β (1 → 4) linked D-glucose units (Updegraff, 1969; Crawford, 1981; Hu, et al., 2019; Liu et al., 2021e; Liu et al., 2022b). Nanocellulose has been extensively studied as the rapid development of nanotechnology. Nanocellulose is a kind of polymer that reduces the size of cellulose in a certain dimension to the nanometer level through chemical, physical and biological methods (Figure 2) (O’sullivan, 1997; Lu et al., 2019; Du et al., 2019; Du et al., 2021a Du et al., 2021b; Du et al., 2022; Liu et al., 2020a; Xu et al., 2020a; Xu et al., 2021b; Xu et al., 2020b; Xu et al., 2020c). In addition to the characteristics of cellulose, nanocellulose also demonstrates large aspect ratio, and specific surface area, excellent mechanical strength, and good chemical reactivity (Dai et al., 2017; An et al., 2019; Li et al., 2019; Li et al., 2020a; An et al., 2020; Wang et al., 2020a; Dai et al., 2020; Si and Xu, 2020; Liu et al., 2021e; Wang et al., 2021; Wang et al., 2022). According to the morphological structure, nanocellulose can be divided into three classes: bacterial cellulose (BC), cellulose nanofibers (CNFs), and cellulose nanocrystals (CNCs) (Ostadhossein et al., 2015; Tayeb et al., 2018; Xie et al., 2018; Xie et al., 2019; Chen et al., 2020a; Chen et al., 2020b; Lu et al., 2020; Li et al., 2022a). Nanocellulose could be mixed with other conductive materials as electrode materials for supercapacitors (Kang et al., 2012; Li et al., 2018; Nie et al., 2018; Zhang et al., 2018; Li et al., 2020b; Liu et al., 2020b; Li et al., 2022b).
Conductive materials are essential in the preparation of energy storage equipments and are considered objects that permit the flow of electrical current. Both MXene and graphene are emerging two-dimensional materials that have been successfully used for supercapacitors as electrode materials. However, the high cost of preparing graphene and easy aggregation affects the surface wettability and dispersibility of graphene in the electrolyte, which reduces the electrical conductivity and thus reduces the effective specific surface area. In the same way, the large van der Waals forces between layers of MXene cause the expansion of inactive pores, limiting the transfer of electrolyte ions, resulting in the decreasing of the electrical conductivity. The introduction of nanocellulose can avoid graphene aggregation and enlarge the active pores between the MXene sheets. At the same time, due to its good properties, including surface wettability, flexibility, high mechanical strength, thermal stability, and hydrophilicity, biomass raw materials can be converted into value-added products, which could promote the economic cycle. Therefore, the composite of nanocellulose with graphene and MXene is the promising electrode materials for supercapacitors (Gao et al., 2013a; Li et al., 2014; Yan et al., 2014). Figures 3, 4 show the recent development of composites (MXene/CNF and graphene/CNF) as electrodes for supercapacitors, respectively.
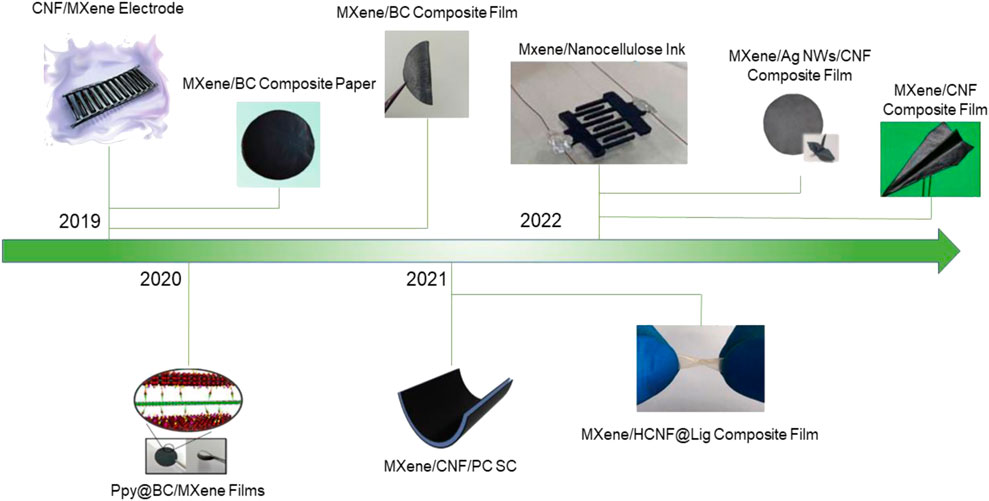
FIGURE 3. Brief timeline of nanocellulose/MXene composites for supercapacitors. Reproduced from: Tian et al. (2019), Wiley-VCH; Jiao et al. (2019), Wiley-VCH; Wang et al. (2019), Wiley-VCH; Song et al. (2020), Springer Nature; Chen et al. (2021a), Elsevier; Chang et al.(2021), Royal Society of Chemistry. Zhou et al. (2022), Wiley-VCH; Tang et al. (2022), Wiley-VCH; Chen et al. (2022), Elsevier.
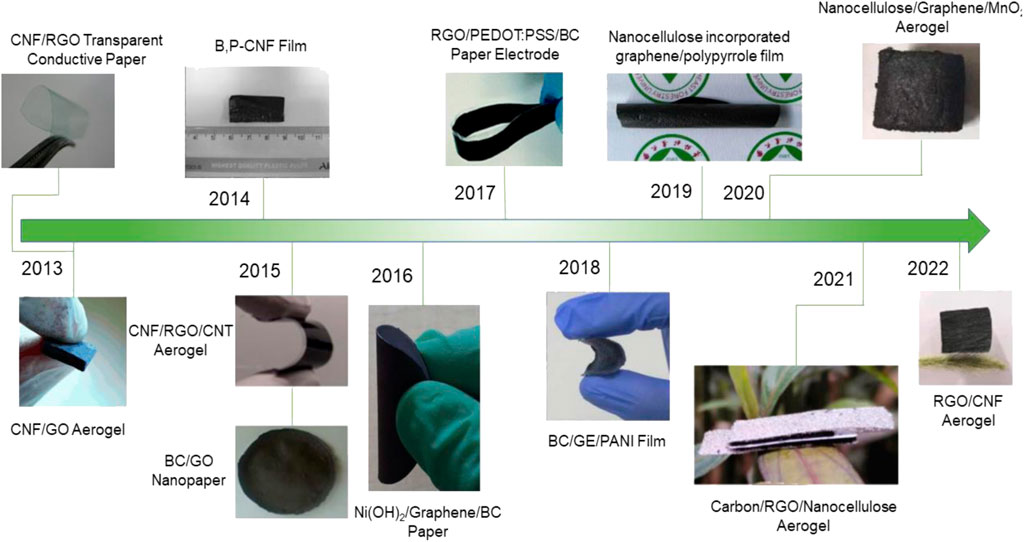
FIGURE 4. Brief timeline of nanocellulose/graphene composites for supercapacitors. Reproduced from: Gao et al. (2013b), Elsevier. Hamedi et al. (2013), Wiley-VCH; Chen et al. (2014), Wiley-VCH; Zheng et al. (2015), American Chemical Society; Liu et al. (2015), Royal Society of Chemistry. Ma et al. (2016a), Elsevier. Zhao et al. (2017), American Chemical Society; Luo et al. (2018), Elsevier. Hou et al. (2019), Elsevier. Wang et al. (2020b), Springer; Chen et al. (2021b), Elsevier; Liu et al. (2022c), Springer.
2 Nanocellulose/two dimensional nanomaterials composites
2.1 Nanocellulose/MXene composites for supercapacitors
2.1.1 MXene and composite strategy
MXene, comprising of transition metal nitrides, carbides, or carbonitrides, is a class of two-dimensional inorganic compounds. It was first reported in 2011 that MXene materials possess metallic conductivity of transition metal carbides due to hydroxyl groups or terminal oxygens on its surface (Naguib et al., 2011; Naguib et al., 2014). Generally, MXene is prepared by selectively etching the A layer from the MAX phases, and the etching solution contains fluoride ions, such as hydrofluoric acid (HF) (Naguib et al., 2014), ammonium bifluoride (NH4HF2) (Halim et al., 2014) or hydrochloric acid (HCl), and lithium fluoride (LiF) (Ghidiu et al., 2014). Because of the strong interlayer van der Waals interaction, MXene is easy to stack, which hinders the effective utilization of surfactant sites and the rapid transport of ions (Yang et al., 2019b). To solve the above problems, the “spacer” is needed to increase the space between the MXene sheets, thereby increasing the surfactant sites to achieve effective ion migration.
Nanocellulose is one-dimensional fibrous structure, that can act as a “spacer” and improves ion transport across the electrode/electrolyte interface. For example, Tian et al. (2019) prepared a two-dimensional film composite of nanocellulose and MXene for supercapacitor electrodes with high electronic conductivity (2.95 × 104 S/m). This is because the small width of the CNFs (around 3.5 nm) makes it possible to have better conductivity between two-dimensional sheets. Simultaneously, since the addition of CNFs did not limit the ability of ion transport and pseudocapacitance storage, its gravimetric capacitance was as high as 298 F/g. As shown in Figure 5A, Wang et al. (2019) reported 3D porous BC/MXene self-supporting film using a simple and time-saving method. The prepared BC/MXene film has a good hierarchical porous structure, excellent mechanical properties, and high flexibility and showed ultra-high capacitance of 416 F/g. In addition, the assembled asymmetric supercapacitor with negative electrode of BC/MXene film and positive electrode of BC/polyaniline (PANI) had a high energy density (252 μWh/cm). This work developed a simple method to assemble high performance 3D porous films electrodes of advanced energy storage devices with 2D MXene materials.
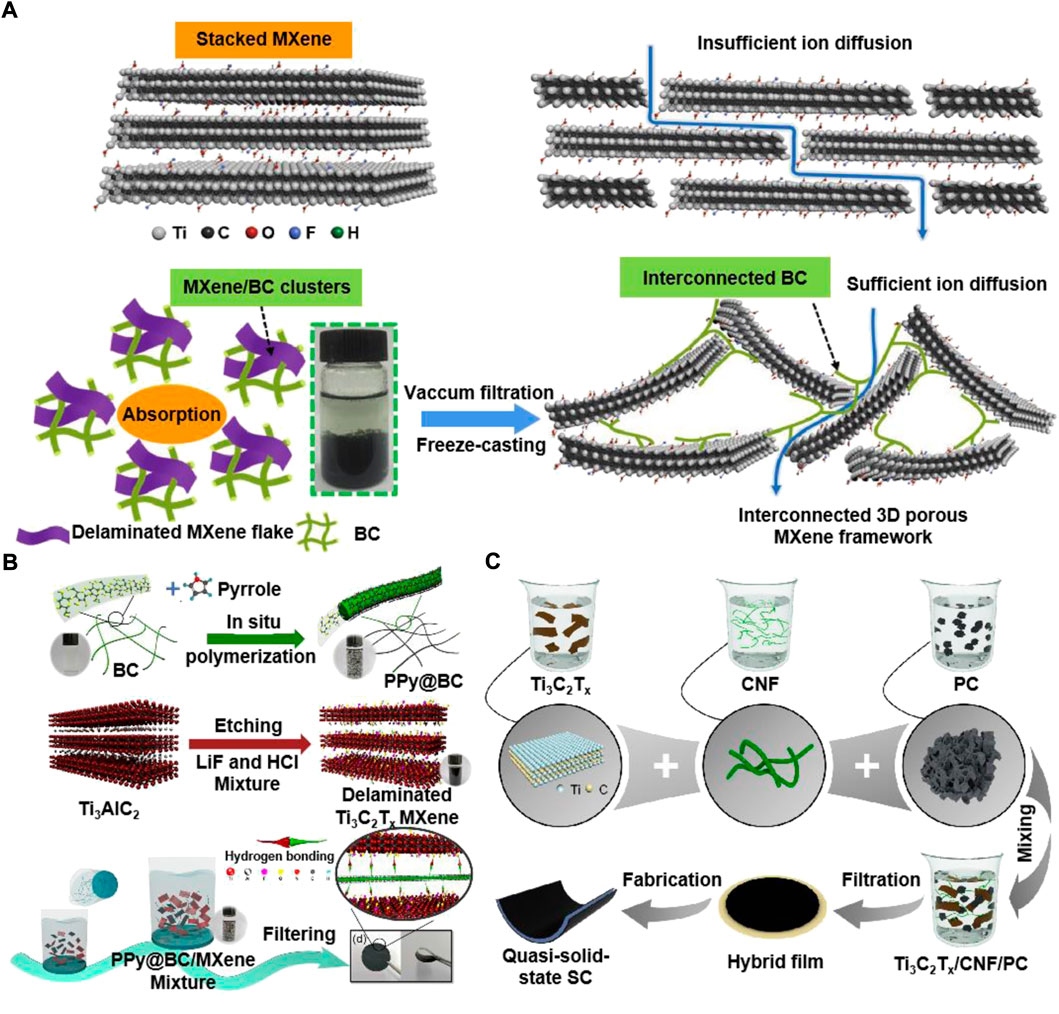
FIGURE 5. The preparation process of the (A) porous MXene architecture, (B) PPy@BC/MXene composite paper, and (C) Ti3C2Tx/CNF/PC hybrid film. Reproduced from: (A) Wang et al. (2019), Wiley. (B) Song et al. (2020), Springer. (C) Chen et al. (2021a), Elsevier.
2.1.2 Nanocellulose/MXene-based supercapacitors
So far, investigation on nanocellulose-MXene based electrodes for supercapacitors with basic compositions and electrochemical performance have been carried out, as showed in Table 1 in literatures. It can be seen that the future study of nanocellulose and MXene composite materials as electrodes for supercapacitors can be divided into two directions.
One is to pursue higher performance supercapacitors, and the other is to develop multifunctional supercapacitors. Because of the potential synergistic effect, the composite hybrid materials of different compositions are expected to be an effective strategy. For example, Song et al. (2020) prepared an enhanced electrochemical performance freestanding electrode of polypyrrole (PPy)@BC/MXene composite film (Figure 5B). The BC as a template used for depositing PPy nanoparticles uniformly, and PPy@BC nanofibers can also be embedded in the MXene layer to effectively prevent the re-accumulation of MXene and expand its interlayer space, which provided a wide range of accessible electrochemical active sites. The prepared PPy@BC/MXene electrode showed good specific capacitance of 550 F/g and long cycle life (83.5% capacitance retention after 10,000 cycles). The assembled symmetric supercapacitor by PPy@BC/MXene electrodes exhibited a high energy density of 33.1 Wh/kg. As shown in Figure 5C, Chen et al. (2021a) prepared a porous flexible MXene/CNFs/porous carbon (PC) hybrid film through a simple method of vacuum filtration. Abundant micropores were provided by three-dimensional PC for charge storage and amount of meso/macropores were provided by three-dimensional PC for the rapid diffusion of ions. One dimensional CNFs enables the hybrid film to have high mechanical properties by combining adjacent MXene sheets and PC. Supercapacitors assembled with MXene/CNFs/PC films as electrodes showed high capacitance of 143 mF/cm2 and high energy density of 2.4 μWh/cm2.
More rational dimensional structure design should be explored. For example, to combine with MXene nanoflakes, Cheng et al. (2021) constructed one-dimensional conductive BC@PPy nanofibers with core-shell structure. The obtained MXene/BC@PPy film electrodes displayed high capacitance of 388 mF/cm2. Based on a two-dimensional film composite with nanocellulose and MXene, Cai et al. (2021) added SnS2 to assemble into a nacre-like structure material. The prepared composite material exhibited excellent mechanical strength (78.3 MPa) without sacrificing toughness. The supercapacitor electrodes based on this material maintained 91.5% capacitance, provided 6.7 μWh/cm2 energy density after 4000 cycles, and exhibited excellent flexibility (over 90% capacitance retention after 500 folding/unfolding cycles). Similarly, Chang et al. (2021) synthesized a nacre-like composite film obtained from MXene, CNFs, and lignosulfonate by a hydrothermal process (Figures 6A–C). The composite film exhibited good mechanical strength (114 MPa) compared with the CNFs film (95 MPa). The supercapacitor assembled by the composite films exhibited an excellent specific capacitance (748.96 F/cm3).
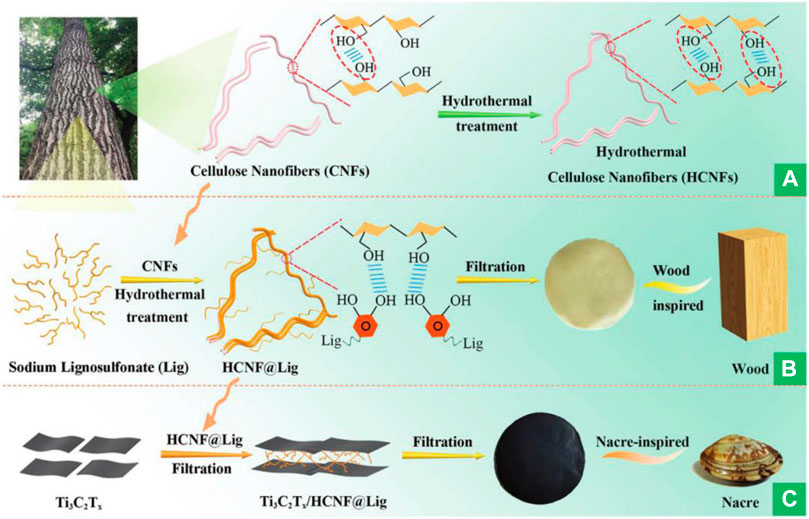
FIGURE 6. The preparation process of the nacre-inspired MXene/HCNF@Lig composite films. Reproduced from: Chang et al. (2021), Royal Society of Chemistry.
2.2 Nanocellulose/graphene composites for supercapacitors
2.2.1 Graphene and composite strategy
Graphene has a hexagonal honeycomb lattice structure and is a two-dimensional nanomaterial composed of carbon atoms with sp2 hybrid orbitals. It has high electron mobility, high specific surface area, excellent mechanical properties, and stable chemical properties. It shows great potential for applications in photothermal conversion, electrochemical energy storage, electronic screens, and industrial catalysis (Novoselov et al., 2012; Ferrari et al., 2015). However, due to the easy accumulation of graphene, the dispersibility and surface wettability of graphene in the electrolyte are affected, and the electrical conductivity is greatly affected. In the composite process of nanocellulose and graphene, nanocellulose acts as a nano-spacer layer, providing a continuous conductive path between different graphene nano-sheet layers and effectively preventing graphene aggregation. This change solves the problem of ion diffusion difficulty in graphene-based materials in electrolytes (Gao et al., 2013a). Table 2 shows the performance of composite materials based on nanocellulose and graphene as electrodes for supercapacitors in recent years. Recent research on supercapacitors mainly focuses on improving electrochemical performance. Nanocellulose-graphene based materials are suitable for selecting electrode materials for high-energy and high-power density supercapacitors. Sheng et al. (2019) constructed a flexible, high-performance fiber-based supercapacitor with synthesized PPy, 2,2,6,6-Tetramethylpiperidine-1-oxyl (TEMPO)-oxidized BC (TOBC), and graphene oxide (GO) into a fiber by wet spinning (Figure 7A). The assembled supercapacitor showed excellent electrochemical performance because TOBC can prevent the aggregation of graphene nanosheets. Carboxylic acid groups and pyrrole monomers penetrated the fiber’s interior, resulting in the generation of PPy inside the fiber, and this unique layered structure provided excellent electrochemical stability. The fiber-based supercapacitor exhibited a high energy density (8.8 mWh/cm3).
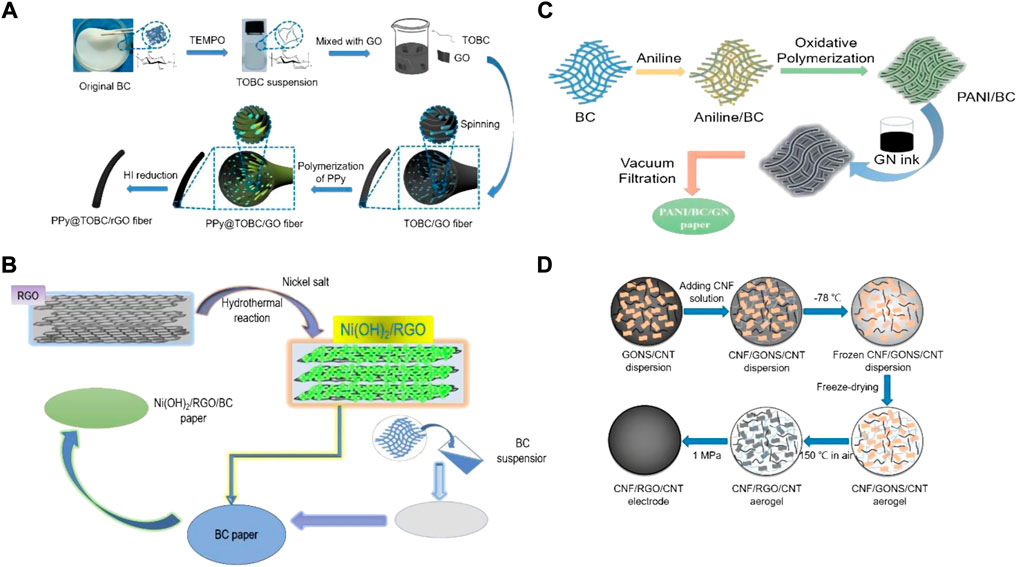
FIGURE 7. The preparation process of the (A) PPy@TOBC/rGO macrofibers, (B) Ni(OH)2/RGO/BC electrode, (C) PANI/BC/GN freestanding paper electrodes, and (D) CNF/RGO/CNT electrode. Reproduced from: (A) Sheng et al. (2019), Elsevier. (B) Ma et al. (2016a), Elsevier. (C) Liu et al. (2016), Royal Society of Chemistry. (D) Zheng et al. (2015), American Chemical Society.
2.2.2 Nanocellulose/graphene-based supercapacitors
Nanocellulose-based two-dimensional paper or film have attracted more and more attention in the field of flexible electrodes for supercapacitors. Vacuum filtration is one of the most common methods to prepare nanopaper or composite film. For instance, Ma et al. (2016a) coated graphene-wrapped Ni(OH)2 on BC to prepare a flexible film using the hydrothermal method and filtration technology (Figure 7B). This method provided an effective pore volume for high-quality loading and provided a fast channel for ion and electron transport. The prepared film electrode achieved a significant area capacitance of 10.44 F/cm2 under a load of 11.9 mg/cm2 and had a capacitance retention rate of 93.6% after 15000 cycles. Later, they used simple in-situ polymerization and filtration methods to prepare a freestanding conductive film from PPy/BC composite nanofibers combined with graphene (Ma et al., 2016b). The introduction of graphene effectively solves the problem of poor conductivity of PPy/BC to ensure high electron and ion transfer. The prepared flexible electrode showed good flexibility and could be bent to a large extent.
The symmetric supercapacitor made of two PPy/BC/RGO paper electrodes exhibited a large area capacitance (1.67 F/cm2) and a high energy density of 0.23 mWh/cm at a power density of 23.5 mW/cm2. The excellent electrochemical performance made it a promising candidate for flexible energy storage devices. Similarly, Liu et al. (2016) prepared a flexible film electrode using PANI, BC and graphene by a simple in-situ chemical polymerization and vacuum filtration method, as shown in Figure 7C. The prepared film electrode possessed a regular interconnected pore channel network, which not only contributed to electron transport and ion dispersion in the whole interconnected network, but also overcame the aggregation of graphene and PANI/BC in three-dimensional conductive paper. The symmetric supercapacitor assembled by the film electrodes can provide high area capacitance (1.93 F/cm2) and energy density (0.17 mWh/cm2).
Liu et al. (2015) constructed BC/GO nanopaper by covalently intercalating GO and BC fiber through one-step esterification. The tensile strength of the prepared reinforced BC/GO composite increased by 12.2 times and the elongation at break increased by 20.9% compared with the original GO. The prepared composite showed excellent conductivity (171 S/m) and high specific capacitance (160 F/g) and had an outstanding capacitance retention of 90.3% after 2,000 cycles. Compared with the original graphene, porous graphene has a multi-layered pore structure on the graphene base surface, which can not only promote the storage and diffusion of electrolyte ions, but also enhance the electrochemical performance of graphene-based electrode materials. Guan et al. (2019) prepared holey GO (HRGO)/BC composite film materials with porous structure, good wettability, and excellent mechanical flexibility through biological assembly method. HRGO/BC composite film has good foldability and can produce high tensile strength of 204 MPa and elongation of 13.8%. The symmetric supercapacitor assembled by HRGO/BC composite film electrode showed a specific capacity (65.9 F/g) and an energy density (9.2 Wh/kg).
Electrode materials with three-dimensional network structure and high conductivity are the keys to developing high-performance supercapacitors. Therefore, three-dimensional aerogel with porous structure has gradually become a popular candidate for supercapacitor electrode materials. Zheng et al. (2015) used CNFs, RGO, and carbon nanotubes (CNTs) to synthesize aerogels as electrodes (Figure 7D). The assembled supercapacitors exhibited suitable areal capacitance, areal power density, and energy density of 216 mF/cm2, 9.5 mW/cm2, and 28.4 μWh/cm2, respectively. Yang et al. (2019c) used TEMPO-oxidized CNFs (TOCN) and GO as precursors to prepare carbon aerogel (TOCN/RGO) by ion exchange, freeze drying and high-temperature carbonization. The obtained carbon aerogel electrode showed a high specific capacitance (398.5 F/g). At the same time, the carbon aerogel electrode also had excellent capacitance retention of 99.77% after 10,000 charge and discharge cycles. In addition, Gao et al. (2013a) used CNFs/RGO hybrid hydrogel as raw material to prepare hybrid aerogel by supercritical CO2 drying, and used it as electrode material for all-solid-state flexible supercapacitor. CNFs were used as nano spacers and electrolyte nano reservoirs of hybrid aerogel. The results showed that the supercapacitor had excellent surface capacitance (207 F/g) and energy density (20 mWh/cm2).
3 Conclusion
Most research on nanocellulose-based supercapacitors mainly concentrated on nanocellulose as a spacer or substrate in composites. As mentioned above, the electrodes of supercapacitors can be a composite of nanocellulose and other conductive materials. Currently, it is still a challenge to produce supercapacitors with high energy density and high capacitance. Therefore, to further improve the electrochemical performance of electrode materials, researchers need to add other materials (such as polypyrrole, sodium lignin thiosulfonate, etc.) to nanocellulose/MXene and nanocellulose/graphene composites. In addition, the composite structure can be changed to obtain different properties. For graphene/MXene and nanocellulose composites, improving the synergy between composites by changing the structure, morphology, distribution, and number of components is the focus of future research (Klemm et al., 2005; Panwar et al., 2011; Liu et al., 2021f; Liu et al., 2022d; Liu et al., 2022e; Liu et al., 2022f; Liu et al., 2022g).
Author contributions
QL, YW, YX, YY, and QZ; investigation and writing—original draft. TX, XS, S-EC, and CS: supervision. QL, TX, S-HH, M-SK, Y-HJ, and SY: writing—review and editing. All authors contributed to the article and approved the submitted version.
Funding
This work was financially supported by the Opening Project of State Key Laboratory of Tree Genetics and Breeding (K2022104), the China Postdoctoral Science Foundation (2021M702456), the Foundation (2021KF32, 2021KF02) of Guangxi Key Laboratory of Clean Pulp & Papermaking and Pollution Control, College of Light Industry and Food Engineering, Guangxi University, and Tianjin Excellent Special Commissioner for Agricultural Science & Technology Project (22ZYCGSN00350, 22YDTPJC00930). This research was also supported by the R&D program for Forest Science Technology (2019151D10-2223-0301) provided by the Korea Forest Service (Korea Forestry Promotion Institute) and partially supported by nurturing open-lab universities connected with regional industries in 2022 to S-EC.
Conflict of interest
The authors declare that the research was conducted in the absence of any commercial or financial relationships that could be construed as a potential conflict of interest.
Publisher’s note
All claims expressed in this article are solely those of the authors and do not necessarily represent those of their affiliated organizations, or those of the publisher, the editors and the reviewers. Any product that may be evaluated in this article, or claim that may be made by its manufacturer, is not guaranteed or endorsed by the publisher.
References
An, L., Si, C., Wang, G., Sui, W., and Tao, Z. (2019). Enhancing the solubility and antioxidant activity of high-molecular-weight lignin by moderate depolymerization via in situ ethanol/acid catalysis. Ind. Crops Prod. 128, 177–185. doi:10.1016/j.indcrop.2018.11.009
An, L., Si, C., Bae, J. H., Jeong, H., and Kim, Y. S. (2020). One-step silanization and amination of lignin and its adsorption of Congo red and Cu(II) ions in aqueous solution. Int. J. Biol. Macromol. 159, 222–230. doi:10.1016/j.ijbiomac.2020.05.072
Aphale, A., Chattopadhyay, A., Mahakalakar, K., and Patra, P. (2015). Synthesis and electrochemical analysis of algae cellulose-polypyrrole-graphene nanocomposite for supercapacitor electrode. J. Nanosci. Nanotechnol. 15 (8), 6225–6229. doi:10.1166/jnn.2015.10280
Augustyn, V., Simon, P., and Dunn, B. (2014). Pseudocapacitive oxide materials for high-rate electrochemical energy storage. Energy Environ. Sci. 7 (5), 1597–1614. doi:10.1039/C3EE44164D
Cai, C., Zhou, W., and Fu, Y. (2021). Bioinspired MXene nacre with mechanical robustness for highly flexible all-solid-state photothermo-supercapacitor. Chem. Eng. J. 418, 129275. doi:10.1016/j.cej.2021.129275
Chang, L., Peng, Z., Zhang, T., Yu, C., and Zhong, W. (2021). Nacre-inspired composite films with high mechanical strength constructed from MXenes and wood-inspired hydrothermal cellulose-based nanofibers for high performance flexible supercapacitors. Nanoscale 13 (5), 3079–3091. doi:10.1039/D0NR08090J
Chen, L. F., Huang, Z. H., Liang, H. W., Gao, H. L., and Yu, S. H. (2014). Three‐dimensional heteroatom‐doped carbon nanofiber networks derived from bacterial cellulose for supercapacitors. Adv. Funct. Mat. 24 (32), 5104–5111. doi:10.1002/adfm.201400590
Chen, S., Wang, G., Sui, W., Parvez, A. M., Dai, L., and Si, C. (2020a). Novel lignin-based phenolic nanosphere supported palladium nanoparticles with highly efficient catalytic performance and good reusability. Ind. Crops Prod. 145, 112164. doi:10.1016/j.indcrop.2020.112164
Chen, S., Wang, G., Sui, W., Parvez, A. M., and Si, C. (2020b). Synthesis of lignin-functionalized phenolic nanosphere supported Ag nanoparticles with excellent dispersion stability and catalytic performance. Green Chem. 22 (9), 2879–2888. doi:10.1039/C9GC04311J
Chen, R., Li, X., Huang, Q., Ling, H., Yang, Y., and Wang, X. (2021a). Self-assembled porous biomass carbon/RGO/nanocellulose hybrid aerogels for self-supporting supercapacitor electrodes. Chem. Eng. J. 412, 128755. doi:10.1016/j.cej.2021.128755
Chen, W., Zhang, D., Yang, K., Luo, M., Yang, P., and Zhou, X. (2021b). Mxene (Ti3C2Tx)/cellulose nanofiber/porous carbon film as free-standing electrode for ultrathin and flexible supercapacitors. Chem. Eng. J. 413, 127524. doi:10.1016/j.cej.2020.127524
Chen, J., Chen, H., Chen, M., Zhou, W., Tian, Q., and Wong, C. P. (2022). Nacre-inspired surface-engineered MXene/nanocellulose composite film for high-performance supercapacitors and zinc-ion capacitors. Chem. Eng. J. 428, 131380. doi:10.1016/j.cej.2021.131380
Cheng, W., Fu, J., Hu, H., and Ho, D. (2021). Interlayer structure engineering of MXene‐based capacitor‐type electrode for hybrid micro‐supercapacitor toward battery‐level energy density. Adv. Sci. 8 (16), 2100775. doi:10.1002/advs.202100775
Conway, B. E., Birss, V., and Wojtowicz, J. (1997). The role and utilization of pseudocapacitance for energy storage by supercapacitors. J. Power Sources 66 (1-2), 1–14. doi:10.1016/S0378-7753(96)02474-3
Dai, L., Liu, R., Hu, L.-Q., Zou, Z.-F., and Si, C.-L. (2017). Lignin nanoparticle as a novel green carrier for the efficient delivery of resveratrol. ACS Sustain. Chem. Eng. 5 (9), 8241–8249. doi:10.1021/acssuschemeng.7b01903
Dai, L., Cao, Q., Wang, K., Han, S., Si, C., Liu, D., et al. (2020). High efficient recovery of L-lactide with lignin-based filler by thermal degradation. Ind. Crops Prod. 143, 111954. doi:10.1016/j.indcrop.2019.111954
Dai, L., Zhu, W., Lu, J., Kong, F., Si, C., and Ni, Y. (2019). A lignin-containing cellulose hydrogel for lignin fractionation. Green. Chem. 21, 5222–5230. doi:10.1039/c9gc01975h
Du, H., Liu, C., Mu, X., Gong, W., Lv, D., Hong, Y., et al. (2016). Preparation and characterization of thermally stable cellulose nanocrystals via a sustainable approach of FeCl3-catalyzed formic acid hydrolysis. Cellulose. 23 (4), 2389–2470. doi:10.1007/s10570-016-0963-5
Du, H., Liu, W., Zhang, M., Si, C., Zhang, X., and Li, B. (2019). Cellulose nanocrystals and cellulose nanofibrils based hydrogels for biomedical applications. Carbohydr. Polym. 209, 130–144. doi:10.1016/j.carbpol.2019.01.020
Du, H., Parit, M., Liu, K., Zhang, M., Jiang, Z., Huang, T.-S., et al. (2021a). Engineering cellulose nanopaper with water resistant, antibacterial, and improved barrier properties by impregnation of chitosan and the followed halogenation. Carbohydr. Polym. 270, 118372. doi:10.1016/j.carbpol.2021.118372
Du, H., Parit, M., Liu, K., Zhang, M., Jiang, Z., Huang, T.-S., et al. (2021b). Multifunctional cellulose nanopaper with superior water-resistant, conductive, and antibacterial properties functionalized with chitosan and polypyrrole. ACS Appl. Mat. Interfaces 13 (27), 32115–32125. doi:10.1021/acsami.1c06647
Du, H., Zhang, M., Liu, K., Parit, M., Jiang, Z., Zhang, X., et al. (2022). Conductive PEDOT:PSS/cellulose nanofibril paper electrodes for flexible supercapacitors with superior areal capacitance and cycling stability. Chem. Eng. J. 428, 131994. doi:10.1016/j.cej.2021.131994
Dubal, D. P., Ayyad, O., Ruiz, V., and Gomez-Romero, P. (2015). Hybrid energy storage: The merging of battery and supercapacitor chemistries. Chem. Soc. Rev. 44 (7), 1777–1790. doi:10.1039/C4CS00266K
Ferrari, A. C., Bonaccorso, F., Fal'Ko, V., Novoselov, K. S., Roche, S., Bøggild, P., et al. (2015). Science and technology roadmap for graphene, related two-dimensional crystals, and hybrid systems. Nanoscale 7 (11), 4598–4810. doi:10.1039/C4NR01600A
Gao, K., Shao, Z., Li, J., Wang, X., Peng, X., Wang, W., et al. (2013a). Cellulose nanofiber–graphene all solid-state flexible supercapacitors. J. Mat. Chem. A 1 (1), 63–67. doi:10.1039/C2TA00386D
Gao, K., Shao, Z., Wu, X., Wang, X., Li, J., Zhang, Y., et al. (2013b). Cellulose nanofibers/reduced graphene oxide flexible transparent conductive paper. Carbohydr. Polym. 97 (1), 243–251. doi:10.1016/j.carbpol.2013.03.067
Ghidiu, M., Lukatskaya, M. R., Zhao, M. Q., Gogotsi, Y., and Barsoum, M. W. (2014). Conductive two-dimensional titanium carbide ‘clay’with high volumetric capacitance. Nature 516 (7529), 78–81. doi:10.1038/nature13970
Gross, R., Leach, M., and Bauen, A. (2003). Progress in renewable energy. Environ. Int. 29 (1), 105–122. doi:10.1016/S0160-4120(02)00130-7
Guan, F., Chen, S., Sheng, N., Chen, Y., Yao, J., Pei, Q., et al. (2019). Mechanically robust reduced graphene oxide/bacterial cellulose film obtained via biosynthesis for flexible supercapacitor. Chem. Eng. J. 360, 829–837. doi:10.1016/j.cej.2018.11.202
Halim, J., Lukatskaya, M. R., Cook, K. M., Lu, J., Smith, C. R., Näslund, L. Å., et al. (2014). Transparent conductive two-dimensional titanium carbide epitaxial thin films. Chem. Mat. 26 (7), 2374–2381. doi:10.1021/cm500641a
Hamedi, M., Karabulut, E., Marais, A., Herland, A., Nyström, G., and Wågberg, L. (2013). Nanocellulose aerogels functionalized by rapid layer‐by‐layer assembly for high charge storage and beyond. Angew. Chem. Int. Ed. Engl. 125 (46), 12038–12042. doi:10.1002/anie.201305137
Hou, M., Xu, M., Hu, Y., and Li, B. (2019). Nanocellulose incorporated graphene/polypyrrole film with a sandwich-like architecture for preparing flexible supercapacitor electrodes. Electrochimica Acta 313, 245–254. doi:10.1016/j.electacta.2019.05.037
Hu, L., Du, H., Liu, C., Zhang, Y., Yu, G., Zhang, X., et al. (2019). Comparative evaluation of the efficient conversion of corn husk filament and corn husk powder to valuable materials via a sustainable and clean biorefinery process. ACS Sustain. Chem. Eng. 7, 1327–1336. doi:10.1021/acssuschemeng.8b05017
Huang, L.-Z., Ma, M.-G., Ji, X.-X., Choi, S.-E., and Si, C. (2021). Recent developments and applications of hemicellulose from wheat straw: A review. Front. Bioeng. Biotechnol. 9, 690773. doi:10.3389/fbioe.2021.690773
Jiao, S., Zhou, A., Wu, M., and Hu, H. (2019). Kirigami patterning of MXene/bacterial cellulose composite paper for all‐solid‐state stretchable micro‐supercapacitor arrays. Adv. Sci. (Weinh). 6 (12), 1900529. doi:10.1002/advs.201900529
Kang, Y. J., Chun, S. J., Lee, S. S., Kim, B. Y., Kim, J. H., Chung, H., et al. (2012). All-solid-state flexible supercapacitors fabricated with bacterial nanocellulose papers, carbon nanotubes, and triblock-copolymer ion gels. ACS Nano 6 (7), 6400–6406. doi:10.1021/nn301971r
Klemm, D., Heublein, B., Fink, H. P., and Bohn, A. (2005). Cellulose: Fascinating biopolymer and sustainable raw material. Angew. Chem. Int. Ed. 44 (22), 3358–3393. doi:10.1002/anie.200460587
Li, S., Huang, D., Yang, J., Zhang, B., Zhang, X., Yang, G., et al. (2014). Freestanding bacterial cellulose–polypyrrole nanofibres paper electrodes for advanced energy storage devices. Nano Energy 9, 309–317. doi:10.1016/j.nanoen.2014.08.004
Li, W., Yang, Y., Sha, J., Zhou, J., Qin, C., and Wang, S. (2018). The influence of mechanical refining treatments on the rheosedimentation properties of bleached softwood pulp suspensions. Cellulose 25 (6), 3609–3618. doi:10.1007/s10570-018-1808-1
Li, X., Xu, R., Yang, J., Nie, S., Liu, D., Liu, Y., et al. (2019). Production of 5-hydroxymethylfurfural and levulinic acid from lignocellulosic biomass and catalytic upgradation. Ind. Crops Prod. 130, 184–197. doi:10.1016/j.indcrop.2018.12.082
Li, X., Lu, X., Liang, M., Xu, R., Yu, Z., Duan, B., et al. (2020a). Conversion of waste lignocellulose to furfural using sulfonated carbon microspheres as catalyst. Waste Manag. 108, 119–126. doi:10.1016/j.wasman.2020.04.039
Li, X., Lu, X., Nie, S., Liang, M., Yu, Z., Duan, B., et al. (2020b). Efficient catalytic production of biomass-derived levulinic acid over phosphotungstic acid in deep eutectic solvent. Ind. Crops Prod. 145, 112154. doi:10.1016/j.indcrop.2020.112154
Li, W., Wang, G., Sui, W., Xu, T., Li, Z., Parvez, A. M., et al. (2022a). Facile and scalable preparation of cage-like mesoporous carbon from lignin-based phenolic resin and its application in supercapacitor electrodes. Carbon 196, 819–827. doi:10.1016/j.carbon.2022.05.053
Li, X., Lu, X., Hu, W., Xu, H., Chen, J., Xiong, J., et al. (2022b). Phosphotungstic acid functionalized biochar for furfural production from corncob. Fuel Process. Technol. 229, 107178. doi:10.1016/j.fuproc.2022.107178
Liu, Y., Zhou, J., Zhu, E., Tang, J., Liu, X., and Tang, W. (2015). Facile synthesis of bacterial cellulose fibres covalently intercalated with graphene oxide by one-step cross-linking for robust supercapacitors. J. Mat. Chem. C Mat. 3 (5), 1011–1017. doi:10.1039/C4TC01822B
Liu, R., Ma, L., Huang, S., Mei, J., Xu, J., and Yuan, G. (2016). Large areal mass, flexible and freestanding polyaniline/bacterial cellulose/graphene film for high-performance supercapacitors. RSC Adv. 6 (109), 107426–107432. doi:10.1039/C6RA21920A
Liu, W., Du, H., Liu, H., Xie, H., Xu, T., Zhao, X., et al. (2020a). Highly efficient and sustainable preparation of carboxylic and thermostable cellulose nanocrystals via FeCl3-catalyzed innocuous citric acid hydrolysis. ACS Sustain. Chem. Eng. 8 (44), 16691–16700. doi:10.1021/acssuschemeng.0c06561
Liu, W., Du, H., Zhang, M., Liu, K., Liu, H., Xie, H., et al. (2020b). Bacterial cellulose-based composite scaffolds for biomedical applications: A review. ACS Sustain. Chem. Eng. 8 (20), 7536–7562. doi:10.1021/acssuschemeng.0c00125
Liu, H., Du, H., Zheng, T., Liu, K., Ji, X., Xu, T., et al. (2021a). Cellulose based composite foams and aerogels for advanced energy storage devices. Chem. Eng. J. 426, 130817. doi:10.1016/j.cej.2021.130817
Liu, H., Xu, T., Liu, K., Zhang, M., Liu, W., Li, H., et al. (2021b). Lignin-based electrodes for energy storage application. Ind. Crops Prod. 165, 113425. doi:10.1016/j.indcrop.2021.113425
Liu, K., Du, H., Zheng, T., Liu, H., Zhang, M., Zhang, R., et al. (2021c). Recent advances in cellulose and its derivatives for oilfield applications. Carbohydr. Polym. 259, 117740. doi:10.1016/j.carbpol.2021.117740
Liu, K., Du, H., Zheng, T., Liu, W., Zhang, M., Liu, H., et al. (2021d). Lignin-containing cellulose nanomaterials: Preparation and applications. Green Chem. 23 (24), 9723–9746. doi:10.1039/D1GC02841C
Liu, S., Du, H., Liu, K., Ma, M.-G., Kwon, Y.-E., Si, C., et al. (2021e). Flexible and porous Co3O4-carbon nanofibers as binder-free electrodes for supercapacitors. Adv. Compos. Hybrid. Mat. 4 (4), 1367–1383. doi:10.1007/s42114-021-00344-8
Liu, W., Du, H., Liu, K., Liu, H., Xie, H., Si, C., et al. (2021f). Sustainable preparation of cellulose nanofibrils via choline chloride-citric acid deep eutectic solvent pretreatment combined with high-pressure homogenization. Carbohydr. Polym. 267, 118220. doi:10.1016/j.carbpol.2021.118220
Liu, H., Xu, T., Liang, Q., Zhao, Q., Zhao, D., and Si, C. (2022a). Compressible cellulose nanofibrils/reduced graphene oxide composite carbon aerogel for solid-state supercapacitor. Adv. Compos. Hybrid. Mat. 5, 1168–1179. doi:10.1007/s42114-022-00427-0
Liu, H., Xu, T., Cai, C., Liu, K., Liu, W., Zhang, M., et al. (2022b). Multifunctional superelastic, superhydrophilic, and ultralight nanocellulose-based composite carbon aerogels for compressive supercapacitor and strain sensor. Adv. Funct. Mat. 32 (26), 2113082. doi:10.1002/adfm.202113082
Liu, K., Du, H., Liu, W., Liu, H., Zhang, M., Xu, T., et al. (2022c). Cellulose nanomaterials for oil exploration applications. Polym. Rev. 62 (3), 585–625. doi:10.1080/15583724.2021.2007121
Liu, K., Du, H., Liu, W., Zhang, M., Wang, Y., Liu, H., et al. (2022d). Strong, flexible, and highly conductive cellulose nanofibril/PEDOT:PSS/MXene nanocomposite films for efficient electromagnetic interference shielding. Nanoscale, Advance Article. doi:10.1039/D2NR00468B
Liu, K., Liu, W., Li, W., Duan, Y., Zhou, K., Zhang, S., et al. (2022e). Strong and highly conductive cellulose nanofibril/silver nanowires nanopaper for high performance electromagnetic interference shielding. Adv. Compos. Hybrid. Mat. 5, 1078–1089. doi:10.1007/s42114-022-00425-2
Liu, W., Liu, K., Du, H., Zheng, T., Zhang, N., Xu, T., et al. (2022f). Cellulose nanopaper: Fabrication, functionalization, and applications. Nano-Micro Lett. 14 (1), 104–127. doi:10.1007/s40820-022-00849-x
Liu, W., Liu, K., Wang, Y., Lin, Q., Liu, J., Du, H., et al. (2022g). Sustainable production of cellulose nanofibrils from Kraft pulp for the stabilization of oil-in-water Pickering emulsions. Ind. Crops Prod. 185, 115123. doi:10.1016/j.indcrop.2022.115123
Lu, J., Zhu, W., Dai, L., Si, C., and Ni, Y. (2019). Fabrication of thermo-and pH-sensitive cellulose nanofibrils-reinforced hydrogel with biomass nanoparticles. Carbohydr. Polym. 215, 289–295. doi:10.1016/j.carbpol.2019.03.100
Lu, J., Han, X., Dai, L., Li, C., Wang, J., Zhong, Y., et al. (2020). Conductive cellulose nanofibrils-reinforced hydrogels with synergetic strength, toughness, self-adhesion, flexibility and adjustable strain responsiveness. Carbohydr. Polym. 250, 117010. doi:10.1016/j.carbpol.2020.117010
Lund, H. (2007). Renewable energy strategies for sustainable development. Energy 32 (6), 912–919. doi:10.1016/j.energy.2006.10.017
Luo, H., Dong, J., Zhang, Y., Li, G., Guo, R., Zuo, G., et al. (2018). Constructing 3D bacterial cellulose/graphene/polyaniline nanocomposites by novel layer-by-layer in situ culture toward mechanically robust and highly flexible freestanding electrodes for supercapacitors. Chem. Eng. J. 334, 1148–1158. doi:10.1016/j.cej.2017.11.065
Ma, S. B., Nam, K. W., Yoon, W. S., Yang, X. Q., Ahn, K. Y., Oh, K. H., et al. (2007). A novel concept of hybrid capacitor based on manganese oxide materials. Electrochem. Commun. 9 (12), 2807–2811. doi:10.1016/j.elecom.2007.09.015
Ma, L., Liu, R., Liu, L., Wang, F., Niu, H., and Huang, Y. (2016a). Facile synthesis of Ni(OH) 2/graphene/bacterial cellulose paper for large areal mass, mechanically tough and flexible supercapacitor electrodes. J. Power Sources 335, 76–83. doi:10.1016/j.jpowsour.2016.10.006
Ma, L., Liu, R., Niu, H., Wang, F., Liu, L., and Huang, Y. (2016b). Freestanding conductive film based on polypyrrole/bacterial cellulose/graphene paper for flexible supercapacitor: Large areal mass exhibits excellent areal capacitance. Electrochimica Acta 222, 429–437. doi:10.1016/j.electacta.2016.10.195
Ma, C., Ma, M.-G., Si, C., Ji, X.-X., and Wan, P. (2021). Flexible MXene-based composites for wearable devices. Adv. Funct. Mat. 31 (22), 2009524. doi:10.1002/adfm.202009524
Naguib, M., Kurtoglu, M., Presser, V., Lu, J., Niu, J., Heon, M., et al. (2011). Two‐dimensional nanocrystals produced by exfoliation of Ti3AlC2. Adv. Mat. 23 (37), 4248–4253. doi:10.1002/adma.201102306
Naguib, M., Mochalin, V. N., Barsoum, M. W., and Gogotsi, Y. (2014). 25th anniversary article: MXenes: A new family of two‐dimensional materials. Adv. Mat. 26 (7), 992–1005. doi:10.1002/adma.201304138
Nie, S., Zhang, K., Lin, X., Zhang, C., Yan, D., Liang, H., et al. (2018). Enzymatic pretreatment for the improvement of dispersion and film properties of cellulose nanofibrils. Carbohydr. Polym. 181, 1136–1142. doi:10.1016/j.carbpol.2017.11.020
Novoselov, K. S., Colombo, L., Gellert, P. R., Schwab, M. G., and Kim, K. (2012). A roadmap for graphene. nature 490 (7419), 192–200. doi:10.1038/nature11458
O'sullivan, A. C. (1997). Cellulose: The structure slowly unravels. Cellul. (Lond). 4 (3), 173–207. doi:10.1023/A:1018431705579
Ostadhossein, F., Mahmoudi, N., Morales-Cid, G., Tamjid, E., Navas-Martos, F. J., Soriano-Cuadrado, B., et al. (2015). Development of chitosan/bacterial cellulose composite films containing nanodiamonds as a potential flexible platform for wound dressing. Materials 8 (9), 6401–6418. doi:10.3390/ma8095309
Panwar, N. L., Kaushik, S. C., and Kothari, S. (2011). Role of renewable energy sources in environmental protection: A review. Renew. Sustain. energy Rev. 15 (3), 1513–1524. doi:10.1016/j.rser.2010.11.037
Qiang, Y., Liao, Y., Liu, J., Tian, C., Xu, H., and Wu, Y. (2021). Research progress of wood-drived energy storage materials. J. For. Eng. 6 (5), 1–13. doi:10.13360/j.issn.2096-1359.202012046
Salunkhe, R. R., Tang, J., Kobayashi, N., Kim, J., Ide, Y., Tominaka, S., et al. (2016a). Ultrahigh performance supercapacitors utilizing core–shell nanoarchitectures from a metal–organic framework-derived nanoporous carbon and a conducting polymer. Chem. Sci. 7 (9), 5704–5713. doi:10.1039/C6SC01429A
Salunkhe, R. R., Young, C., Tang, J., Takei, T., Ide, Y., Kobayashi, N., et al. (2016b). A high-performance supercapacitor cell based on ZIF-8-derived nanoporous carbon using an organic electrolyte. Chem. Commun. 52 (26), 4764–4767. doi:10.1039/C6CC00413J
Salunkhe, R. R., Kaneti, Y. V., and Yamauchi, Y. (2017). Metal–organic framework-derived nanoporous metal oxides toward supercapacitor applications: Progress and prospects. ACS Nano 11 (6), 5293–5308. doi:10.1021/acsnano.7b02796
Sheng, N., Chen, S., Yao, J., Guan, F., Zhang, M., Wang, B., et al. (2019). Polypyrrole@TEMPO-oxidized bacterial cellulose/reduced graphene oxide macrofibers for flexible all-solid-state supercapacitors. Chem. Eng. J. 368, 1022–1032. doi:10.1016/j.cej.2019.02.173
Si, C.-L., Liu, Z., Kim, J.-K., and Bae, Y.-S. (2008). Structure elucidation of phenylethanoid glycosides from Paulownia tomentosa Steud. var. tomentosa wood. Holzforschung 62, 197–200. doi:10.1515/HF.2008.047
Si, C.-L., Kim, J.-K., Bae, Y.-S., and Li, S.-M. (2009a). Phenolic compounds in the leaves of Populus ussuriensis and their antioxidant activities. Planta Med. 75, 1165–1167. doi:10.1055/s-0029-1185476
Si, C.-L., Wu, L., Zhu, Z.-Y., Kim, J.-K., Kwon, D.-J., and Bae, Y.-S. (2009b). Apigenin derivatives from Paulownia tomentosa Steud. var. tomentosa stem barks. Holzforschung 63 (4), 440–442. doi:10.1515/HF.2009.063
Si, C.-L., Jiang, J.-Z., Liu, S.-C., Hu, H.-Y., Ren, X.-D., Yu, G.-J., et al. (2013a). A new lignan glycoside and phenolics from the branch wood of Pinus banksiana Lambert. Holzforschung 67, 357–363. doi:10.1515/hf-2012-0137
Si, C.-L., Shen, T., Jiang, Y.-Y., Wu, L., Yu, G.-J., Ren, X.-D., et al. (2013b). Antioxidant properties and neuroprotective effects of isocampneoside II on hydrogen peroxide-induced oxidative injury in PC12 cells. Food Chem. Toxicol. 59, 145–152. doi:10.1016/j.fct.2013.05.051
Si, C., and Xu, J. (2020). Recent advances in bio-medicinal and pharmaceutical applications of bio-based materials. Curr. Med. Chem. 27 (28), 4581–4583. doi:10.2174/092986732728200621210700
Song, Q., Zhan, Z., Chen, B., Zhou, Z., and Lu, C. (2020). Biotemplate synthesis of polypyrrole@ bacterial cellulose/MXene nanocomposites with synergistically enhanced electrochemical performance. Cellulose 27 (13), 7475–7488. doi:10.1007/s10570-020-03310-7
Tang, H., Chen, R., Huang, Q., Ge, W., Zhang, X., Yang, Y., et al. (2022). Scalable manufacturing of leaf‐like MXene/Ag NWs/cellulose composite paper electrode for all‐solid‐state supercapacitor. EcoMat, e12247. doi:10.1002/eom2.12247
Tayeb, A. H., Amini, E., Ghasemi, S., and Tajvidi, M. (2018). Cellulose nanomaterials—binding properties and applications: A review. Molecules 23 (10), 2684. doi:10.3390/molecules23102684
Tian, W., VahidMohammadi, A., Reid, M. S., Wang, Z., Ouyang, L., Erlandsson, J., et al. (2019). Multifunctional nanocomposites with high strength and capacitance using 2D MXene and 1D nanocellulose. Adv. Mat. 31 (41), 1902977. doi:10.1002/adma.201902977
Updegraff, D. M. (1969). Semimicro determination of cellulose inbiological materials. Anal. Biochem. 32 (3), 420–424. doi:10.1016/s0003-2697(69)80009-6
Wang, Y., Wang, X., Li, X., Bai, Y., Xiao, H., Liu, Y., et al. (2019). Engineering 3D ion transport channels for flexible MXene films with superior capacitive performance. Adv. Funct. Mat. 29 (14), 1900326. doi:10.1002/adfm.201900326
Wang, H., Xie, H., Du, H., Wang, X., Liu, W., Duan, Y., et al. (2020a). Highly efficient preparation of functional and thermostable cellulose nanocrystals via H2SO4 intensified acetic acid hydrolysis. Carbohydr. Polym. 239, 116233. doi:10.1016/j.carbpol.2020.116233
Wang, Y. Y., Fu, Q. J., Bai, Y. Y., Ning, X., and Yao, C. L. (2020b). Construction and application of nanocellulose/graphene/MnO2 three-dimensional composites as potential electrode materials for supercapacitors. J. Mat. Sci. Mat. Electron. 31 (2), 1236–1246. doi:10.1007/s10854-019-02635-9
Wang, H., Du, H., Liu, K., Liu, H., Xu, T., Zhang, S., et al. (2021). Sustainable preparation of bifunctional cellulose nanocrystals via mixed H2SO4/formic acid hydrolysis. Carbohydr. Polym. 266, 118107. doi:10.1016/j.carbpol.2021.118107
Wang, H., Zhang, M., Hu, J., Du, H., Xu, T., and Si, C. (2022). Sustainable preparation of surface functionalized cellulose nanocrystals and their application for Pickering emulsions. Carbohydr. Polym. 297, 120062. doi:10.1016/j.carbpol.2022.120062
Xie, H., Du, H., Yang, X., and Si, C. (2018). Recent strategies in preparation of cellulose nanocrystals and cellulose nanofibrils derived from raw cellulose materials. Int. J. Polym. Sci. 2018, 1–25. doi:10.1155/2018/7923068
Xie, H., Zou, Z., Du, H., Zhang, X., Wang, X., Yang, X., et al. (2019). Preparation of thermally stable and surface-functionalized cellulose nanocrystals via mixed H2SO4/Oxalic acid hydrolysis. Carbohydr. Polym. 223, 115116. doi:10.1016/j.carbpol.2019.115116
Xiong, R., Hua, D., Hoeck, J. V., Berdecka, D., Léger, M., Munter, S. D., et al. (2021a). Photothermal nanofibres enable safe engineering of therapeutic cells. Nat. Nanotechnol. 16, 1281–1291. doi:10.1038/s41565-021-00976-3
Xiong, R., Xu, R. X., Huang, C., De Smedt, S., and Braeckmans, K. (2021b). Stimuli-responsive nanobubbles for biomedical applications. Chem. Soc. Rev. 50, 5746–5776. doi:10.1039/C9CS00839J
Xu, J., Li, C., Dai, L., Xu, C., Zhong, Y., Yu, F., et al. (2020a). Biomass fractionation and lignin fractionation towards lignin valorization. Chemsuschem 13 (17), 4284–4295. doi:10.1002/cssc.202001491
Xu, R., Liu, K., Du, H., Liu, H., Cao, X., Zhao, X., et al. (2020b). Falling leaves return to their roots: A review on the preparation of gamma-valerolactone from lignocellulose and its application in the conversion of lignocellulose. Chemsuschem 13 (24), 6461–6476. doi:10.1002/cssc.202002008
Xu, R., Si, C., Kong, F., and Li, X. (2020c). Synthesis of γ-valerolactone and its application in biomass conversion. J. For. Eng. 5 (2), 20–28. doi:10.13360/j.issn.2096-1359.201904004
Xu, R., Du, H., Liu, C., Liu, H., Wu, M., Zhang, X., et al. (2021a). An efficient and magnetic adsorbent prepared in a dry process with enzymatic hydrolysis residues for wastewater treatment. J. Clean. Prod. 313, 127834. doi:10.1016/j.jclepro.2021.127834
Xu, T., Du, H., Liu, H., Liu, W., Zhang, X., Si, C., et al. (2021b). Advanced nanocellulose‐based composites for flexible functional energy storage devices. Adv. Mat. 33 (48), 2101368. doi:10.1002/adma.202101368
Xu, T., Liu, K., Sheng, N., Zhang, M., Liu, W., Liu, H., et al. (2022). Biopolymer-based hydrogel electrolytes for advanced energy storage/conversion devices: Properties, applications, and perspectives. Energy Storage Mater. 48, 244–262. doi:10.1016/j.ensm.2022.03.013
Yan, C., Wang, J., Kang, W., Cui, M., Wang, X., Foo, C. Y., et al. (2014). Highly stretchable piezoresistive graphene–nanocellulose nanopaper for strain sensors. Adv. Mat. 26 (13), 2022–2027. doi:10.1002/adma.201304742
Yang, J., Bao, W., Jaumaux, P., Zhang, S., Wang, C., and Wang, G. (2019a). MXene‐based composites: Synthesis and applications in rechargeable batteries and supercapacitors. Adv. Mat. Interfaces 6 (8), 1802004. doi:10.1002/admi.201802004
Yang, Q., Yang, J., Gao, Z., Li, B., and Xiong, C. (2019b). Carbonized cellulose nanofibril/graphene oxide composite aerogels for high-performance supercapacitors. ACS Appl. Energy Mat. 3 (1), 1145–1151. doi:10.1021/acsaem.9b02195
Yang, X., Xie, H., Du, H., Zhang, X., Zou, Z., Zou, Y., et al. (2019c). Facile extraction of thermally stable and dispersible cellulose nanocrystals with high yield via a green and recyclable FeCl3-catalyzed deep eutectic solvent system. ACS Sustain. Chem. Eng. 7 (7), 7200–7208. doi:10.1021/acssuschemeng.9b00209
Yang, J., Si, C., Liu, K., Liu, H., Li, X., and Liang, M. (2020). Production of levulinic acid from lignocellulosic biomass and application. J. For. Eng. 5 (2), 21–27. doi:10.13360/j.issn.2096-1359.201905013
Zhai, Y., Dou, Y., Zhao, D., Fulvio, P. F., Mayes, R. T., and Dai, S. (2011). Carbon materials for chemical capacitive energy storage. Adv. Mat. 23 (42), 4828–4850. doi:10.1002/adma.201100984
Zhang, L. L., and Zhao, X. S. (2009). Carbon-based materials as supercapacitor electrodes. Chem. Soc. Rev. 38 (9), 2520–2531. doi:10.1039/B813846J
Zhang, K., Zhang, Y., Yan, D., Zhang, C., and Nie, S. (2018). Enzyme-assisted mechanical production of cellulose nanofibrils: Thermal stability. Cellulose 25 (9), 5049–5061. doi:10.1007/s10570-018-1928-7
Zhang, M., Du, H., Liu, K., Nie, S., Xu, T., Zhang, X., et al. (2021). Fabrication and applications of cellulose-based nanogenerators. Adv. Compos. Hybrid. Mat. 4, 865–884. doi:10.1007/s42114-021-00312-2
Zhao, X., Sánchez, B. M., Dobson, P. J., and Grant, P. S. (2011). The role of nanomaterials in redox-based supercapacitors for next generation energy storage devices. Nanoscale 3 (3), 839–855. doi:10.1039/C0NR00594K
Zhao, D., Zhang, Q., Chen, W., Yi, X., Liu, S., Wang, Q., et al. (2017). Highly flexible and conductive cellulose-mediated PEDOT: PSS/MWCNT composite films for supercapacitor electrodes. ACS Appl. Mat. Interfaces 9 (15), 13213–13222. doi:10.1021/acsami.7b01852
Zheng, Q., Cai, Z., Ma, Z., and Gong, S. (2015). Cellulose nanofibril/reduced graphene oxide/carbon nanotube hybrid aerogels for highly flexible and all-solid-state supercapacitors. ACS Appl. Mat. Interfaces 7 (5), 3263–3271. doi:10.1021/am507999s
Keywords: supercapacitor, nanocellulose, MXene, graphene, electrode
Citation: Liang Q, Wang Y, Yang Y, Xu T, Xu Y, Zhao Q, Heo S-H, Kim M-S, Jeong Y-H, Yao S, Song X, Choi S-E and Si C (2022) Nanocellulose/two dimensional nanomaterials composites for advanced supercapacitor electrodes. Front. Bioeng. Biotechnol. 10:1024453. doi: 10.3389/fbioe.2022.1024453
Received: 21 August 2022; Accepted: 09 September 2022;
Published: 04 October 2022.
Edited by:
Lei Wang, Ocean University of China, ChinaReviewed by:
Wenyang Xu, Åbo Akademi University, FinlandQiong Wu, Qingdao University of Science and Technology, China
Copyright © 2022 Liang, Wang, Yang, Xu, Xu, Zhao, Heo, Kim, Jeong, Yao, Song, Choi and Si. This is an open-access article distributed under the terms of the Creative Commons Attribution License (CC BY). The use, distribution or reproduction in other forums is permitted, provided the original author(s) and the copyright owner(s) are credited and that the original publication in this journal is cited, in accordance with accepted academic practice. No use, distribution or reproduction is permitted which does not comply with these terms.
*Correspondence: Ting Xu, eHV0aW5nQHR1c3QuZWR1LmNu; Xueping Song, c3hfcGluZ0BneHUuZWR1LmNu; Sun-Eun Choi, b3JlZ29uaW5Aa2FuZ3dvbi5hYy5rcg==; Chuanling Si, c2ljaGxpQHR1c3QuZWR1LmNu
†These authors have contributed equally to this work and share first authorship