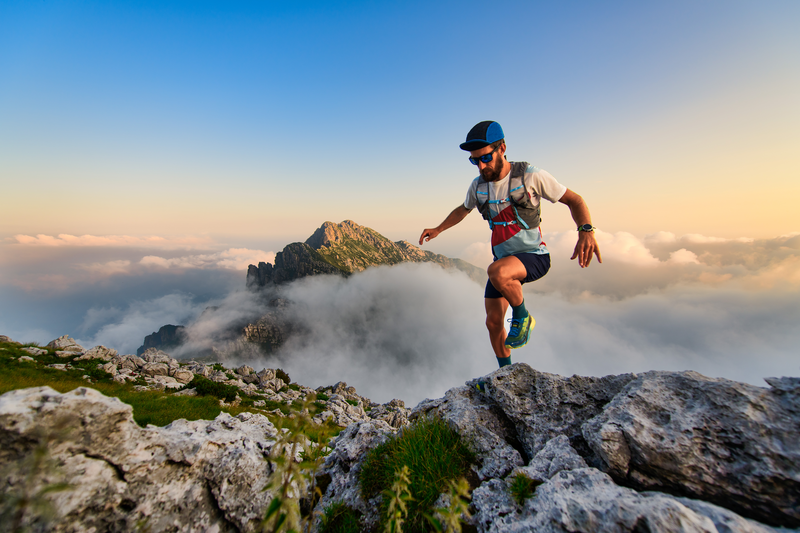
94% of researchers rate our articles as excellent or good
Learn more about the work of our research integrity team to safeguard the quality of each article we publish.
Find out more
REVIEW article
Front. Bioeng. Biotechnol. , 16 September 2022
Sec. Biomaterials
Volume 10 - 2022 | https://doi.org/10.3389/fbioe.2022.1022368
This article is part of the Research Topic Advanced Biomaterials for Osteochondral Regeneration View all 10 articles
Osteoarthritis (OA) is a worldwide and disabling disease, which cause severe pain and heavy socioeconomic burden. However, pharmacologic or surgical therapies cannot mitigate OA progression. Mesenchymal stem cells (MSCs) therapy has emerged as potential approach for OA treatment, while the immunogenicity and ethical audit of cell therapy are unavoidable. Compared with stem cell strategy, EVs induce less immunological rejection, and they are more stable for storage and in vivo application. MSC-EVs-based therapy possesses great potential in regulating inflammation and promoting cartilage matrix reconstruction in OA treatment. To enhance the therapeutic effect, delivery efficiency, tissue specificity and safety, EVs can be engineered via different modification strategies. Here, the application of MSC-EVs in OA treatment and the potential underlying mechanism were summarized. Moreover, EV modification strategies including indirect MSC modification and direct EV modification were reviewed.
Osteoarthritis (OA) is a worldwide and disabling disease, which cause severe pain and heavy socioeconomic burden (Glyn-Jones et al., 2015). The pathogenic risk factors for OA include trauma, aging, obesity, inheritance, etc. (Cisternas et al., 2016). OA is characterized as synovitis, degrading cartilage, damaged menisci and ligaments, and pathologically formed osteophytes (Loeser et al., 2012). Aging-related cell senescence, metabolic disorder and aberrant mechanical load can lead to senescence-associated secretory phenotype (SASP) release and local inflammation, which in turn aggravate cell senescence and cartilage matrix degradation (Glyn-Jones et al., 2015; Appleton, 2018; Boulestreau et al., 2021). Existing therapies for OA are symptomatic strategies like nonsteroidal anti-inflammatory drugs (NSAIDs), and surgical strategies like joint replacement (Tao et al., 2017). However, pharmacologic or surgical therapies cannot mitigate OA progression, or enhance damaged cartilage reconstruction. Recently, mesenchymal stem cells (MSCs) therapy has emerged as potential approach for OA treatment, while the immunogenicity and ethical audit of cell therapy are unavoidable (Zhuang et al., 2022). Effective strategies for inflammation regulation and cartilage regeneration are urgently required.
Extracellular vesicles (EVs), serving as cell-cell communication media, play a vital role in regulating tissue homeostasis and biological process. EVs derived from MSCs (MSCs-EVs) inherit the valuable characteristics of donor MSCs (Liu A. et al., 2021; Liu L. et al., 2021; Zhang X. et al., 2022). Compared with stem cell strategy, EVs induce less immunological rejection, and they are more stable for storage and in vivo application (Brennan et al., 2020). Multiple components (including miRNAs, lipids, proteins) make EVs potential candidates in promoting tissue regeneration and modulating immunity (Yu et al., 2022). MSCs-EVs have been reported to improve chondrocyte phenotype, attenuate cartilage degradation in vitro and ameliorate OA progression in vivo (Zhang S. et al., 2016; Tofiño-Vian et al., 2018; Woo et al., 2020). To enhance the therapeutic effect, delivery efficiency, tissue specificity and safety, EVs can be engineered via different modification strategies.
EV-based therapy possesses great potential in regulating inflammation and promoting cartilage matrix reconstruction in OA treatment. In this review, we summarize the application of MSC-EVs in OA treatment and the potential underlying mechanism. Moreover, EV modification strategies including indirect MSC modification and direct EV modification were also reviewed (Figure 1).
There have been more and more studies indicating that MSC-EVs are potential in controlling inflammation, inhibiting cartilage matrix degradation, and promoting cartilage repair for OA treatment. The applications of EVs derived from different MSCs in OA treatment and the potential underlying mechanisms are summarized here.
The cargos of EVs may vary depending on their donor cells and consist of multiple bioactive molecules including proteins, lipids, and miRNAs, thus leading to specific characteristics of different MSC-EVs (Mianehsaz et al., 2019). MSCs therapy has been proved to attenuate inflammation, prevent cartilage matrix degradation, and ameliorate pain in clinical trials (Toh et al., 2017; Song et al., 2020). The mechanism underlying the MSCs therapeutic effect might be secretion of bioactive molecules (Eleuteri and Fierabracci, 2019), additionally, application of EVs secreted from MSCs possess inherent advantages compared to direct MSC therapy (lower immunogenicity, tumorigenicity, etc.) (Ankrum et al., 2014; Fichtel et al., 2022). Thus, EVs therapy has gained more and more attention in disease treatment and tissue reconstruction. EVs derived from different MSCs source for OA treatment have been summarized here (Table 1).
EVs derived from bone marrow mesenchymal stem cells (BMSC-EVs) has been widely applied for OA treatment. BMSC-EVs can play a role in promoting the proliferation and matrix components secretion of chondrocytes, and improve the framework of subchondral bone. He et al. (2020) treated OA rats with BMSC-EVs, and the results indicated that BMSC-EVs improved chondrocyte phenotype and alleviated pain via ameliorating function of dorsal root ganglion (DRG). Moreover, BMSC-EVs were reported to regulate inflammation through restraining NF-κB pathway (Li et al., 2020), controlling inflammation related factor Autotaxin-YAP (Wang Y. et al., 2021), modulating macrophagocyte polarization (Zhang et al., 2020), and prevent chondrocyte apoptosis (Chen et al., 2020; Wang X. et al., 2021; Jin et al., 2021).
It has been reported that adipose mesenchymal stem cells (ADSCs) also showed potential in protecting cartilage (ter Huurne et al., 2012; Baharlou et al., 2017), and ADSC-EVs could play a role in regulating inflammation. (Mortati et al. (2020) utilized ADSC-EVs for OA treatment, and the results indicated that ADSC-EVs effectively promoted M2 polarization, inhibited inflammation and promoted cartilage matrix deposition. Apart from chondrocytes, ADSC-EVs could target synovial cells, modulating the synthetase, catabolic enzymes and inflammatory cytokines secretion of synovial cells, and positively improve the biological performance of EVs secreted by endogenous synovial cells and chondrocytes (Cavallo et al., 2021). Promoting autophagy of chondrocyte via mTOR pathway could also be one of the mechanisms under the therapeutic effect of ADSC-EVs in preventing OA process (Wu et al., 2019).
Human perinatal stem cells, with outstanding self-renewal capacity, are widely applied in OA treatment (Matas et al., 2019). More and more studies indicated that EVs derived from perinatal stem cells, maintaining the excellent traits of donor cells, are ideal alternatives to MSCs in cartilage repair (Tang et al., 2021; Zhang Q. et al., 2022; Zhang S. et al., 2022; Li et al., 2022; Zhou et al., 2022). EVs derived from embryonic MSCs (EMSCs) were proved to inhibit inflammation, reconstruct cartilage matrix, and alleviate pain in OA model (Zhang S. et al., 2016; Zhang et al., 2019). Umbilical cord MSCs (UMSCs) also secreted EVs that were capable to control inflammation via promoting M2 polarization and inhibiting m6A of NLRP3 in macrophages, and enhance cartilage repair (Park et al., 2017; Li X. et al., 2021; Zhang Q. et al., 2022; Li et al., 2022; Zhou et al., 2022). It has been reported that EVs derived from amniotic MSCs (AMSCs) (Silini et al., 2017; Ragni et al., 2021) and amniotic fluid stem cells (AFSCs) (Maraldi et al., 2013; Zavatti et al., 2020) are also potential in inflammation modulation and OA treatment.
In addition, synovial mesenchymal stem cells (SMSCs) showed stronger potential in chondrocyte differentiation. EVs derived from SMSCs showed great potential in immunomodulation and cartilage repair, and were applied in OA treatment (Zhu et al., 2017; Lu et al., 2021; Qiu et al., 2021).
MSCEVs can effectively promote the synthesis of cartilage extracellular matrix (ECM) (Yeo et al., 2013). The mechanisms under the therapeutic effect of MSC-EVs attract much attention. Multiple MSCs can serve as donor cell source for EV production, and there are masses of various cargos in MSC-EVs, like nucleus acids and proteins. Considering that EVs derived from different MSCs possess similar therapeutic effect, MSC-EVs may share evolutionary conserved key bioactive molecules in their biological activity (Yeo et al., 2013; Toh et al., 2017). Proteins in MSC-EVs contain some housekeeping enzymes that play a role in reconstructing cartilage homeostasis via modulating cell fate of chondrocyte, remodeling bioenergetic metabolism, regulating immune system and promoting cartilage matrix synthesis (Lai et al., 2015).
In OA cartilage micro-environment, oxidative stress, ROS production and inflammatory factors boost intensively, which usually lead to cell apoptosis, cell death and cell dysfunction (Haslauer et al., 2013; Heard et al., 2015; Toh et al., 2016). There have been studies reporting that MSC-EVs could promote cell proliferation via activating the phosphorylation of ERK1/2 and AKT, the factors tightly connected with cell survival. In the repair process of damaged sites, excessive ATP can lead to cell death of neighbouring healthy cells. The ATP is metabolized via hydrolysis into AMP (Toh et al., 2017). CD73, the hallmark of EVs, serve as catalyst to activate the hydrolysis of AMP into adenosine, the activator of survival related enzymes (Colgan et al., 2006; Jacobson and Gao, 2006; Toh et al., 2017). The ability of MSC-EVs in converting ATP into pro-survival kinases make them potential in promoting cell proliferation for cartilage repair. Additionally, MSC-EVs are capable to inhibit apoptosis via mTOR pathway (Li X. et al., 2021; Jin et al., 2021; Lu et al., 2021; Zhou et al., 2022), and promote autophagy (Zhang and Jin, 2022) to improve cell performance of chondrocytes.
Mitochondria, the ATP production organelle, plays a key role in cartilage bioenergy homeostasis. Chondrocytes in OA are reported to suffer mitochondrial dysfunction and reduced electron transport chain (ETC) proteins activity. The inhibited ETC activity and ATP production in chondrocytes lead to abnormal bioenergetics, which then induce increased cell apoptosis, more ROS production, enhanced catabolism and inhibited anabolism of cartilage matrix (Vaamonde-García et al., 2012; Lee et al., 2020). MSC-EVs are enriched in enzymes to promote ATP production for decreased ATP generation compensation in defective chondrocytes, which make them potential in reconstructing bioenergetic homeostasis and repair capability of chondrocytes in OA (Pashoutan Sarvar et al., 2016; Toh et al., 2017).
Immune system is activated rapidly following tissue repair happening, which exerts vital influence on tissue reconstruction. Immune cell like macrophage, neutrophil and synovium cell release amount of pro-inflammation factors (IL-1β, IL-6, IL-8, MMPs, etc.), which then induce the cartilage matrix destruction and OA progression (Haslauer et al., 2013; Heard et al., 2015; Mianehsaz et al., 2019). Moreover, the modulation of macrophage M1-M2 polarization plays a role in maintaining inflammation balance during tissue repair process (Ding et al., 2016; Utomo et al., 2016). There have been many studies indicated that immunomodulation factors in MSC-EVs can synergistically reduce IL-1β, IL-6, TNF-α expression, promote M2 polarization, and enhance IL-10, TGF-β1 secretion, to construct a positive immuno-microenvironment for cartilage repair.
ECM is the important component of cartilage structure and gradually destroyed during OA progression. The inflammatory pathological micro-environment tends to induce cartilage matrix degradation, and cartilage structure loss (Haslauer et al., 2013; Heard et al., 2015). Activating reparative responses and anabolic related gene expression of chondrocytes is important in promoting cartilage matrix re-deposition (Mizuta et al., 2004). MSC-EVs are proved to promote cartilage matrix deposition via upregulating SOX9, aggrecan, col2 expression and inhibit matrix degradation through downregulating MMP13, MMP3, ADAMTS-5 expression (Cosenza et al., 2017; Sun et al., 2019; Zhang et al., 2019; Chen et al., 2020; Woo et al., 2020; Wang X. et al., 2021).
MSC-EVs exert positive influence on immunomodulation, cell fate regulation, bioenergy homeostasis remodeling, and matrix synthesis modulation, which make MSC-EVs considered to be potential candidates for OA treatment. To further improve cargo delivery, cell specification and fusion efficiency, multiple strategies can be utilized to modify EVs, including direct (modify cargo and membrane of EVs) and indirect (modify MSCs) methods (Table 2).
EVs tend to inherit characteristics of their donor cells, and donor MSCs can be modified to obtain correspondingly customized miRNA enriched EVs (Kosaka et al., 2010; Ni et al., 2020). The advantage of indirect MSC modification lies in that the stimulation factors on MSCs are in control and can be quantified.
Gene transfection manipulation, mainly via viral vectors, can not only improve EV yields, but also enhance functional cargos like miRNA, circRNA and LncRNA in engineered EVs for OA treatment Kosaka et al. (Kosaka et al., 2010) found that upregulating the expression of neutral sphingomyelinase 2 (nSMase2) in donor cells promoted the secretion of miRNAs, which are transferable and functional to target cells.
The functions of miRNAs are important in the biological performance of EVs in recipient cells. Through Microarray analysis and literature review, key miRNAs can be found out. Virus infection can effectively manipulate target gene expression of donor cells and cargos of EVS. It has been reported that upregulating expression of miR-26a–5p (Jin et al., 2020), miR-126–3p (Zhou et al., 2021), miR-155–5p (Wang Z. et al., 2021), miR-140–5p (Liu et al., 2022) in MSCs and EVs can effectively inhibit inflammation, matrix degradation, cell apoptosis and improve matrix secretion of chondrocytes. The miRNAs can bind to target mRNAs, induce mRNA degradation, and impact downstream signal pathways (Zhuang et al., 2022). Apart from miRNAs, circRNAs and lncRNAs are also potential in regulating cell behavior. CircRNAs like circRNA_0001236 (Mao et al., 2021), circHIPK3 (Li et al., 2021b) and lncRNAs like lncRNA H19 (Yan et al., 2021; Yang et al., 2021) can interact with target miRNAs, regulate downstream genes, inhibit catabolism and attenuate anabolism of chondrocytes. Thus, overexpression of functional circRNAs and lncRNAs in EVs can also be potential candidates for better OA therapeutic strategy.
Bioactive molecules can be loaded into EVs via co-incubation with donor cells. The characteristic of donor cells obtained following compound co-incubation can be transmitted to EVs. Co-incubated with anti-inflammatory factors like curcumin (Li et al., 2021c; Xu et al., 2022), TGF-β1 (Wang and Xu, 2021) endows EVs with ideal capacity to modulate macrophage polarization, reduce oxidative stress and promote chondrocyte anabolism. Interestingly, IL-1β (Colombini et al., 2021; Kim et al., 2021) and LPS (Duan et al., 2021) preconditioned donor cell derived EVs are proved to inhibit inflammation, improve chondrocyte performance and ameliorate OA progression. The reason accounting for this lies in that MSCs treated with low concentration of LPS or IL-1β response adaptively, which is beneficial for immunomodulation.
MSCculture microenvironment changes can exert influence on the biological effect of EVs. Compared to 2D culture, 3D culture can enhance the yield and functions of EVs (Rocha et al., 2019). Yan et al. (Yan and Wu, 2020) constructed a hollow-fiber bioreactor to build a 3D culture microenvironment for cartilage restoration, and the results indicated that EVs isolated from 3D culture cells showed greater potential in promoting TGF-β1 expression and Smad2/3 pathway. Moreover, dynamic mechanical stimulation is also proved to be able to modulate cell proliferation and differentiation, and improve EV production and functions (Li et al., 2011; Guo et al., 2021). (Liao et al. (2021) utilized ultrasound to load BMSCs with mechanical stimulus, and found that the obtained EVs could inhibit inflammation via NF-κB pathway, and enhance cartilage matrix deposition (Figure 2). Apart from 3D culture and mechanical stimulation, hypoxia microenvironment regulates MSC performance via HIF-1α (Malladi et al., 2007). EVs derived from hypoxia pre-treatment MSCs were proved to promote repair capability of chondrocytes through miR-216a-5p/JAK2/STAT3 pathway (Rong et al., 2021).
FIGURE 2. Low-intensity pulsed ultrasound (LIPUS) dynamic mechanical stimulus promoted the biological performance of MSC-EVs in OA treatment. (A) Safranin O, Toluidine Blue and HE staining for knee joints sections; (B) The mechanism underlying the therapeutic effect of LIPUS-treated MSC-EVs; (C,D) Western blot analysis for anabolism related proteins of chondrocytes, and semi-quantification; (E) RT-qPCR analysis for the expression of anabolism related genes; (F) Western blot analysis for the expression of NF-κB pathway related proteins. Reproduced from Ref. (Liao et al., 2021), International Immunopharmacology, ELSEVIER Publication at 2021.
Indirect methods pre-synthesize components to enrich EV cargos via donor cell modification. Direct engineering strategies, including enriching EV cargos and modifying EV membranes, can endow EVs with specific and controllable functions.
Multiple strategies can be utilized to transfer cargos into EVs, including direct co-incubation, physical and chemical methods. Hydrophobic molecules can pass across EV phospholipid membrane, which makes them suitable for the direct mixture method (Fuhrmann et al., 2015). Li et al. (2021) co-incubated EVs with chitosan oligosaccharides (COS) for 1 h at 37°C to make COS go into EVs and construct COS-EVs. The result indicated that compared to EVs, COS-EVs exhibited better ability in promoting anabolic related genes expression of chondrocytes. Strategies like electroporation (Kooijmans et al., 2013; Pomatto et al., 2018), sonication (Lamichhane et al., 2016), saponin permeabilization (Pomatto et al., 2019), freeze-thawing (Luan et al., 2017), and CaCl2 mediated method (Zhang D. et al., 2016) can be utilized to directly load EVs with bioactive molecules. To enhance drug encapsulation rate, Xu et al. (Xu et al. (2021) encapsulated kartogenin (KGN) into EVs via electroporation, and the encapsulation rate was advanced to 40% compared to 8% of direct co-incubation. The enhanced KGN delivery efficiency could promote cartilage repair.
To further improve the target and drug delivery efficiency of EVs, EVs membrane can be modified. Xu et al. (Xu et al. (2021) modified EV surface with E7 peptide via plasmids transfection of donor cells to target synovial fluid-derived MSCs for enhanced OA treatment. Through plasmids transfection, liang et al. (Liang et al., 2020) fused chondrocyte-affinity peptide (CAP) with EV surface protein, and improved the chondrocyte target ability of CAP-EVs. Apart from protein fusion, regulating EV surface charge potential can promote EV penetration since the block influence of negative cartilage matrix. Feng et al. (2021) utilized ε-polylysine-polyethylene-distearyl phosphatidylethanolamine (PPD) modifying EVs to construct positively charged MSC-EVs for better cartilage matrix penetration. Wei et al. (Wei et al.) endowed EVs with amphiphilic positive potential through surface modification of cationic 1,2-dioleoyl-3-trimethylammonium propane (DOTAP), and the results showed that DOTAP modified EVs could promote the penetration of EVs into cartilage matrix, extend EV retention and attenuate OA destruction.
EVs derived from different MSCs, loaded with abundant cargos including miRNAs, lipids, and proteins, show great potential in OA treatment. The mechanisms underlying the MSC-EVs therapeutic effects lie in that MSC-EVs can play roles in modulating cell fate of chondrocyte, remodeling bioenergetic metabolism, regulating immune system and promoting cartilage matrix synthesis. To further enhance target and delivery efficiency of EVs, gene transfection manipulation, co-incubation and cell culture microenvironment engineering can be utilized to modify donor cells, and EV cargo enrichment and EV membrane modification can be applied to directly modify EVs.Wei Y et al., 2021.
Although the wide application of MSC-EVs, in OA treatment, there are still challenges requiring further explorations.
1) Current studies mostly concentrate on the phenotype of chondrocytes following EV treatment, but the molecular mechanisms underlying the phenomenon are still unclear. For precise medical treatment, the discovery of specific target is crucial. Understanding the molecular mechanism in OA development and treatment can provide a new approach for treatment and facilitate precise intervention for patients.
2) Apart from the therapeutic effect of MSC-EVs, EVs also play a role in the pathological process of OA development. Studying the pathogenic effect of EVs can help better understand the molecular mechanism of OA progress, and facilitate the exploration of new therapeutic strategies. Moreover, EVs can serve as biomarkers for early OA diagnosis, and more specific and effective EVs as biomarkers are required to be found.
3) The MSC-EVs treatment can attenuate OA progression, but the effect of MSC-EVs on reversing chondrocyte function is short of demonstration. Whether applying EVs in early or late period of OA can reverse OA pathological changes, but not only mitigate OA progression, which needs more explorations.
4) The kinetics and bio-distribution of EVs, and dosage of EVs used in vivo experiments and further in clinical trials is still unclear, which needs more researches to clarify.
5) The MSC therapy has undergone clinical trial period, and can be applied in clinic, but the safety of MSC-EVs for clinical application requires more studies to verify.
6) Indirect EV engineering strategies are costly, time-consuming and hard to manipulate; utilizing new gene-editing technologies, like CRISPR-Cas9 can make the gene manipulation more efficient and accurate. Direct EV modification might exert negative influence on morphology and size of engineered EVs, and efficient and scatheless EV engineering strategies are required.
YZ and SJ have written the manuscript text. CY and KL have conceived the concept of this review.
This work was funded by the National Natural Science Foundation of China (82072396, 81871490), Program of Shanghai Academic/Technology Research Leader (19XD1434500), Science and Technology Commission of Shanghai Municipality (21490711700), Double Hundred Plan (20191819), Interdisciplinary Program of Shanghai Jiao Tong University (YG2021ZD12), and CAMS Innovation Fund for Medical Sciences (CIFMS) (2019-I2M-5-037).
The authors declare that the research was conducted in the absence of any commercial or financial relationships that could be construed as a potential conflict of interest.
All claims expressed in this article are solely those of the authors and do not necessarily represent those of their affiliated organizations, or those of the publisher, the editors and the reviewers. Any product that may be evaluated in this article, or claim that may be made by its manufacturer, is not guaranteed or endorsed by the publisher.
Ankrum, J. A., Ong, J. F., and Karp, J. M. (2014). Mesenchymal stem cells: Immune evasive, not immune privileged. Nat. Biotechnol. 32 (3), 252–260. doi:10.1038/nbt.2816 | |
Appleton, C. T. (2018). Osteoarthritis year in review 2017: Biology. Osteoarthr. Cartil. 26 (3), 296–303. doi:10.1016/j.joca.2017.10.008 |
Baharlou, R., Ahmadi-Vasmehjani, A., Faraji, F., Atashzar, M. R., Khoubyari, M., Ahi, S., and Saiedeh, E. (2017). Human adipose tissue-derived mesenchymal stem cells in rheumatoid arthritis: Regulatory effects on peripheral blood mononuclear cells activation. Int. Immunopharmacol. 47, 59–69. doi:10.1016/j.intimp.2017.03.016 | |
Boulestreau, J., Maumus, M., Jorgensen, C., and Noël, D. (2021). Extracellular vesicles from mesenchymal stromal cells: Therapeutic perspectives for targeting senescence in osteoarthritis. Adv. Drug Deliv. Rev. 175, 113836. doi:10.1016/j.addr.2021.113836 | |
Brennan, M. Á., Layrolle, P., and Mooney, D. J. (2020). Biomaterials functionalized with MSC secreted extracellular vesicles and soluble factors for tissue regeneration. Adv. Funct. Mat. 30 (37), 1909125. doi:10.1002/adfm.201909125 | |
Cavallo, C., Merli, G., Borzì, R. M., Zini, N., D’Adamo, S., and Guescini, M., (2021). Small Extracellular Vesicles from adipose derived stromal cells significantly attenuate in vitro the NF-κB dependent inflammatory/catabolic environment of osteoarthritis. Sci. Rep. 11 (1), 1053. doi:10.1038/s41598-020-80032-7 | |
Chen, X., Shi, Y., Xue, P., Ma, X., Li, J., and Zhang, J. (2020). Mesenchymal stem cell-derived exosomal microRNA-136-5p inhibits chondrocyte degeneration in traumatic osteoarthritis by targeting ELF3. Arthritis Res. Ther. 22 (1), 256. doi:10.1186/s13075-020-02325-6 | |
Cisternas, M. G., Murphy, L., Sacks, J. J., Solomon, D. H., Pasta, D. J., and Helmick, C. G. (2016). Alternative methods for defining osteoarthritis and the impact on estimating prevalence in a US population-based survey. Arthritis Care & Res. 68 (5), 574–580. doi:10.1002/acr.22721 |
Colgan, S. P., Eltzschig, H. K., Eckle, T., and Thompson, L. F. (2006). Physiological roles for ecto-5’-nucleotidase (CD73). Purinergic Signal. 2 (2), 351–360. doi:10.1007/s11302-005-5302-5 | |
Colombini, A., Ragni, E., Mortati, L., Libonati, F., Perucca Orfei, C., and Viganò, M., (2021). Adipose-derived mesenchymal stromal cells treated with interleukin 1 beta produced chondro-protective vesicles able to fast penetrate in cartilage. Cells 10 (5), 1180. doi:10.3390/cells10051180 | |
Cosenza, S., Ruiz, M., Toupet, K., Jorgensen, C., and Noël, D. (2017). Mesenchymal stem cells derived exosomes and microparticles protect cartilage and bone from degradation in osteoarthritis. Sci. Rep. 7 (1), 16214. doi:10.1038/s41598-017-15376-8 | |
Ding, J., Chen, B., Lv, T., Liu, X., Fu, X., Wang, Q., and Ran, X. (2016). Bone marrow mesenchymal stem cell-based engineered cartilage ameliorates polyglycolic acid/polylactic acid scaffold-induced inflammation through M2 polarization of macrophages in a pig model. Stem Cells Transl. Med. 5 (8), 1079–1089. doi:10.5966/sctm.2015-0263 | |
Duan, A., Shen, K., Li, B., Li, C., Zhou, H., Kong, R., Wei, L., and Feng, L. (2021). Extracellular vesicles derived from LPS-preconditioned human synovial mesenchymal stem cells inhibit extracellular matrix degradation and prevent osteoarthritis of the knee in a mouse model. Stem Cell. Res. Ther. 12 (1), 427. doi:10.1186/s13287-021-02507-2 | |
Eleuteri, S., and Fierabracci, A. (2019). Insights into the secretome of mesenchymal stem cells and its potential applications. Int. J. Mol. Sci. 20 (18), 4597. doi:10.3390/ijms20184597 |
Feng, K., Xie, X., Yuan, J., Gong, L., Zhu, Z., Zhang, J., and Yang, W. (2021). Reversing the surface charge of MSC-derived small extracellular vesicles by εPL-PEG-DSPE for enhanced osteoarthritis treatment. J. Extracell. Vesicles 10 (13), e12160. doi:10.1002/jev2.12160 | |
Fichtel, P., von Bonin, M., Kuhnert, R., Möbus, K., Bornhäuser, M., and Wobus, M. (2022). Mesenchymal stromal cell-derived extracellular vesicles modulate hematopoietic stem and progenitor cell viability and the expression of cell cycle regulators in an age-dependent manner. Front. Bioeng. Biotechnol. 10, 892661. doi:10.3389/fbioe.2022.892661 | |
Fuhrmann, G., Serio, A., Mazo, M., Nair, R., and Stevens, M. M. (2015). Active loading into extracellular vesicles significantly improves the cellular uptake and photodynamic effect of porphyrins. J. Control. Release 205, 35–44. doi:10.1016/j.jconrel.2014.11.029 | |
Glyn-Jones, S., Palmer, A. J. R., Agricola, R., Price, A. J., Vincent, T. L., and Weinans, H., (2015). Osteoarthritis. Lancet 386 (9991), 376–387. doi:10.1016/S0140-6736(14)60802-3 | |
Guo, S., Debbi, L., Zohar, B., Samuel, R., Arzi, R. S., Fried, A. I., Tahel, C., and Dudi, S. (2021). Stimulating extracellular vesicles production from engineered tissues by mechanical forces. Nano Lett. 21 (6), 2497–2504. doi:10.1021/acs.nanolett.0c04834 | |
Haslauer, C. M., Elsaid, K. A., Fleming, B. C., Proffen, B. L., Johnson, V. M., and Murray, M. M. (2013). Loss of extracellular matrix from articular cartilage is mediated by the synovium and ligament after anterior cruciate ligament injury. Osteoarthr. Cartil. 21 (12), 1950–1957. doi:10.1016/j.joca.2013.09.003 | |
He, L., He, T., Xing, J., Zhou, Q., Fan, L., Liu, C., and Rong, L. (2020). Bone marrow mesenchymal stem cell-derived exosomes protect cartilage damage and relieve knee osteoarthritis pain in a rat model of osteoarthritis. Stem Cell. Res. Ther. 11 (1), 276. doi:10.1186/s13287-020-01781-w | |
Heard, B. J., Barton, K. I., Chung, M., Achari, Y., Shrive, N. G., and Frank, C. B., (2015). Single intra-articular dexamethasone injection immediately post-surgery in a rabbit model mitigates early inflammatory responses and post-traumatic osteoarthritis-like alterations. J. Orthop. Res. 33 (12), 1826–1834. doi:10.1002/jor.22972 | |
Jacobson, K. A., and Gao, Z.-G. (2006). Adenosine receptors as therapeutic targets. Nat. Rev. Drug Discov. 5 (3), 247–264. doi:10.1038/nrd1983 | |
Jin, Y., Xu, M., Zhu, H., Dong, C., Ji, J., Liu, Y., Aidong, D., and Zhifeng, G. (2021). Therapeutic effects of bone marrow mesenchymal stem cells-derived exosomes on osteoarthritis. J. Cell. Mol. Med. 25 (19), 9281–9294. doi:10.1111/jcmm.16860 | |
Jin, Z., Ren, J., and Qi, S. (2020). Human bone mesenchymal stem cells-derived exosomes overexpressing microRNA-26a-5p alleviate osteoarthritis via down-regulation of PTGS2. Int. Immunopharmacol. 78, 105946. doi:10.1016/j.intimp.2019.105946 | |
Kim, M., Shin, D. I., Choi, B. H., and Min, B.-H. (2021). Exosomes from IL-1β-primed mesenchymal stem cells inhibited IL-1β- and TNF-α-mediated inflammatory responses in osteoarthritic SW982 cells. Tissue Eng. Regen. Med. 18 (4), 525–536. doi:10.1007/s13770-020-00324-x | |
Kooijmans, S. A. A., Stremersch, S., Braeckmans, K., de Smedt, S. C., Hendrix, A., and Wood, M. J. A., (2013). Electroporation-induced siRNA precipitation obscures the efficiency of siRNA loading into extracellular vesicles. J. Control. Release 172 (1), 229–238. doi:10.1016/j.jconrel.2013.08.014 | |
Kosaka, N., Iguchi, H., Yoshioka, Y., Takeshita, F., Matsuki, Y., and Ochiya, T. (2010). Secretory mechanisms and intercellular transfer of microRNAs in living cells. J. Biol. Chem. 285 (23), 17442–17452. doi:10.1074/jbc.M110.107821 | |
Lai, R. C., Yeo, R. W. Y., and Lim, S. K. (2015). Mesenchymal stem cell exosomes. Seminars Cell. & Dev. Biol. 40, 82–88. doi:10.1016/j.semcdb.2015.03.001 |
Lamichhane, T. N., Jeyaram, A., Patel, D. B., Parajuli, B., Livingston, N. K., Arumugasaamy, N., et al. (2016). Oncogene knockdown via active loading of small RNAs into extracellular vesicles by sonication. Cell. Mol. Bioeng. 9 (3), 315–324. doi:10.1007/s12195-016-0457-4 | |
Lee, Y.-H., Park, H.-K., Auh, Q.-S., Nah, H., Lee, J. S., Moon, H.-J., In San, K., and Il Keun, K. (2020). Emerging potential of exosomes in regenerative medicine for temporomandibular joint osteoarthritis. Int. J. Mol. Sci. 21 (4), 1541. doi:10.3390/ijms21041541 |
Li, D., Zhou, J., Chowdhury, F., Cheng, J., Wang, N., and Wang, F. (2011). Role of mechanical factors in fate decisions of stem cells. Regen. Med. 6 (2), 229–240. doi:10.2217/rme.11.2 | |
Li, K., Yan, G., Huang, H., Zheng, M., Ma, K., and Cui, X., (2022). Anti-inflammatory and immunomodulatory effects of the extracellular vesicles derived from human umbilical cord mesenchymal stem cells on osteoarthritis via M2 macrophages. J. Nanobiotechnology 20 (1), 38. doi:10.1186/s12951-021-01236-1 | |
Li, S., Liu, J., Liu, S., Jiao, W., and Wang, X. (2021a). Chitosan oligosaccharides packaged into rat adipose mesenchymal stem cells-derived extracellular vesicles facilitating cartilage injury repair and alleviating osteoarthritis. J. Nanobiotechnology 19 (1), 343. doi:10.1186/s12951-021-01086-x |
Li, S., Liu, J., Liu, S., Jiao, W., and Wang, X. (2021b). Mesenchymal stem cell-derived extracellular vesicles prevent the development of osteoarthritis via the circHIPK3/miR-124-3p/MYH9 axis. J. Nanobiotechnology 19 (1), 194. doi:10.1186/s12951-021-00940-2 |
Li, S., Stöckl, S., Lukas, C., Götz, J., Herrmann, M., Federlin, M., and Susanne, G. (2020). hBMSC-derived extracellular vesicles attenuate IL-1β-induced catabolic effects on OA-chondrocytes by regulating pro-inflammatory signaling pathways. Front. Bioeng. Biotechnol. 8, 603598. doi:10.3389/fbioe.2020.603598 | |
Li, S., Stöckl, S., Lukas, C., Herrmann, M., Brochhausen, C., König, M. A., and Susanne, G. (2021c). Curcumin-primed human BMSC-derived extracellular vesicles reverse IL-1β-induced catabolic responses of OA chondrocytes by upregulating miR-126-3p. Stem Cell. Res. Ther. 12 (1), 252. doi:10.1186/s13287-021-02317-6 | |
Li, X., Wang, Y., Cai, Z., Zhou, Q., Li, L., and Fu, P. (2021d). Exosomes from human umbilical cord mesenchymal stem cells inhibit ROS production and cell apoptosis in human articular chondrocytes via the miR-100-5p/NOX4 axis. Cell. Biol. Int. 45 (10), 2096–2106. doi:10.1002/cbin.11657 | |
Liang, Y., Xu, X., Li, X., Xiong, J., Li, B., and Duan, L., (2020). Chondrocyte-targeted MicroRNA delivery by engineered exosomes toward a cell-free osteoarthritis therapy. ACS Appl. Mat. Interfaces 12 (33), 36938–36947. doi:10.1021/acsami.0c10458 | |
Liao, Q., Li, B. J., Li, Y., Xiao, Y., Zeng, H., Liu, J. M., and Liu, G. (2021). Low-intensity pulsed ultrasound promotes osteoarthritic cartilage regeneration by BMSC-derived exosomes via modulating the NF-κB signaling pathway. Int. Immunopharmacol. 97, 107824. doi:10.1016/j.intimp.2021.107824 | |
Liu, A., Lin, D., Zhao, H., Chen, L., Cai, B., and Lin, K., (2021a). Optimized BMSC-derived osteoinductive exosomes immobilized in hierarchical scaffold via lyophilization for bone repair through Bmpr2/Acvr2b competitive receptor-activated Smad pathway. Biomaterials 272, 120718. doi:10.1016/j.biomaterials.2021.120718 | |
Liu, L., Yu, F., Li, L., Zhou, L., Zhou, T., Xu, Y., and Xia, L. (2021b). Bone marrow stromal cells stimulated by strontium-substituted calcium silicate ceramics: Release of exosomal miR-146a regulates osteogenesis and angiogenesis. Acta Biomater. 119, 444–457. doi:10.1016/j.actbio.2020.10.038 | |
Liu, Y., Zeng, Y., Si, H.-B., Tang, L., Xie, H.-Q., and Shen, B. (2022). Exosomes derived from human urine–derived stem cells overexpressing miR-140-5p alleviate knee osteoarthritis through downregulation of VEGFA in a rat model. Am. J. Sports Med. 50 (4), 1088–1105. doi:10.1177/03635465221073991 | |
Loeser, R. F., Goldring, S. R., Scanzello, C. R., and Goldring, M. B. (2012). Osteoarthritis: A disease of the joint as an organ. Arthritis & Rheumatism 64 (6), 1697–1707. doi:10.1002/art.34453 | |
Lu, L., Wang, J., Fan, A., Wang, P., Chen, R., Lu, L., and Yin, F. (2021). Synovial mesenchymal stem cell-derived extracellular vesicles containing microRN555A-26a-5p ameliorate cartilage damage of osteoarthritis. J. Gene Med. 23 (11), e3379. doi:10.1002/jgm.3379 | |
Luan, X., Sansanaphongpricha, K., Myers, I., Chen, H., Yuan, H., and Sun, D. (2017). Engineering exosomes as refined biological nanoplatforms for drug delivery. Acta Pharmacol. Sin. 38 (6), 754–763. doi:10.1038/aps.2017.12 | |
Malladi, P., Xu, Y., Chiou, M., Giaccia, A. J., and Longaker, M. T. (2007). Hypoxia inducible factor-1α deficiency affects chondrogenesis of adipose-derived adult stromal cells. Tissue Eng. 13 (6), 1159–1171. doi:10.1089/ten.2006.0265 | |
Mao, G., Xu, Y., Long, D., Sun, H., Li, H., and Xin, R., (2021). Exosome-transported circRNA_0001236 enhances chondrogenesis and suppress cartilage degradation via the miR-3677-3p/Sox9 axis. Stem Cell. Res. Ther. 12 (1), 389. doi:10.1186/s13287-021-02431-5 | |
Mao, G., Zhang, Z., Hu, S., Zhang, Z., Chang, Z., and Huang, Z., (2018). Exosomes derived from miR-92a-3p-overexpressing human mesenchymal stem cells enhance chondrogenesis and suppress cartilage degradation via targeting WNT5A. Stem Cell. Res. Ther. 9 (1), 247. doi:10.1186/s13287-018-1004-0 | |
Maraldi, T., Riccio, M., Pisciotta, A., Zavatti, M., Carnevale, G., Beretti, F., and Nakamura, E. (2013). Human amniotic fluid-derived and dental pulp-derived stem cells seeded into collagen scaffold repair critical-size bone defects promoting vascularization. Stem Cell. Res. Ther. 4 (3), 53. doi:10.1186/scrt203 | |
Matas, J., Orrego, M., Amenabar, D., Infante, C., Tapia-Limonchi, R., and Cadiz, M. I., (2019). Umbilical cord-derived mesenchymal stromal cells (MSCs) for knee osteoarthritis: Repeated MSC dosing is superior to a single MSC dose and to hyaluronic acid in a controlled randomized phase I/II trial. Stem Cells Transl. Med. 8 (3), 215–224. doi:10.1002/sctm.18-0053 | |
Mianehsaz, E., Mirzaei, H. R., Mahjoubin-Tehran, M., Rezaee, A., Sahebnasagh, R., Pourhanifeh, M. H., and Nakamura, E. (2019). Mesenchymal stem cell-derived exosomes: A new therapeutic approach to osteoarthritis? Stem Cell. Res. Ther. 10 (1), 340. doi:10.1186/s13287-019-1445-0 | |
Mizuta, H., Kudo, S., Nakamura, E., Otsuka, Y., Takagi, K., and Hiraki, Y. (2004). Active proliferation of mesenchymal cells prior to the chondrogenic repair response in rabbit full-thickness defects of articular cartilage. Osteoarthr. Cartil. 12 (7), 586–596. doi:10.1016/j.joca.2004.04.008 | |
Mortati, L., de Girolamo, L., Perucca Orfei, C., Viganò, M., Brayda-Bruno, M., Ragni, E., and Alessandra, C. (2020). In vitro study of extracellular vesicles migration in cartilage-derived osteoarthritis samples using real-time quantitative multimodal nonlinear optics imaging. Pharmaceutics 12 (8), 734. doi:10.3390/pharmaceutics12080734 |
Ni, Z., Zhou, S., Li, S., Kuang, L., Chen, H., Luo, X., et al. (2020). Exosomes: Roles and therapeutic potential in osteoarthritis. Bone Res. 8 (1), 25. doi:10.1038/s41413-020-0100-9 | |
Park, Y.-B., Ha, C.-W., Lee, C.-H., Yoon, Y. C., and Park, Y.-G. (2017). Cartilage regeneration in osteoarthritic patients by a composite of allogeneic umbilical cord blood-derived mesenchymal stem cells and hyaluronate hydrogel: Results from a clinical trial for safety and proof-of-concept with 7 Years of extended follow-up. Stem Cells Transl. Med. 6 (2), 613–621. doi:10.5966/sctm.2016-0157 | |
Pashoutan Sarvar, D., Shamsasenjan, K., and Akbarzadehlaleh, P. (2016). Mesenchymal stem cell-derived exosomes: New opportunity in cell-free therapy. Adv. Pharm. Bull. 6 (3), 293–299. doi:10.15171/apb.2016.041 | |
Pomatto, M. A. C., Bussolati, B., D’Antico, S., Ghiotto, S., Tetta, C., and Brizzi, M. F., (2019). Improved loading of plasma-derived extracellular vesicles to encapsulate antitumor miRNAs. Mol. Ther. - Methods & Clin. Dev. 13, 133–144. doi:10.1016/j.omtm.2019.01.001 |
Pomatto, M. A. C., Gai, C., Deregibus, M. C., Tetta, C., and Camussi, G. (2018). Noncoding RNAs carried by extracellular vesicles in endocrine diseases. Int. J. Endocrinol. 2018, 1–18. doi:10.1155/2018/4302096 | |
Qiu, M., Liu, D., and Fu, Q. (2021). MiR-129-5p shuttled by human synovial mesenchymal stem cell-derived exosomes relieves IL-1β induced osteoarthritis via targeting HMGB1. Life Sci. 269, 118987. doi:10.1016/j.lfs.2020.118987 | |
Ragni, E., Papait, A., Perucca Orfei, C., Silini, A. R., Colombini, A., Viganò, M., et al. (2021). Amniotic membrane-mesenchymal stromal cells secreted factors and extracellular vesicle-miRNAs: Anti-inflammatory and regenerative features for musculoskeletal tissues. Stem Cells Transl. Med. 10 (7), 1044–1062. doi:10.1002/sctm.20-0390 | |
Rocha, S., Carvalho, J., Oliveira, P., Voglstaetter, M., Schvartz, D., Thomsen, A. R., et al. (2019). 3D cellular architecture affects MicroRNA and protein cargo of extracellular vesicles. Adv. Sci. (Weinh). 6 (4), 1800948. doi:10.1002/advs.201800948 | |
Rong, Y., Zhang, J., Jiang, D., Ji, C., liu, W., and Wang, J., (2021). Hypoxic pretreatment of small extracellular vesicles mediates cartilage repair in osteoarthritis by delivering miR-216a-5p. Acta Biomater. 122, 325–342. doi:10.1016/j.actbio.2020.12.034 | |
Silini, A. R., Magatti, M., Cargnoni, A., and Parolini, O. (2017). Is immune modulation the mechanism underlying the beneficial effects of amniotic cells and their derivatives in regenerative medicine? Cell. Transpl. 26 (4), 531–539. doi:10.3727/096368916X693699 |
Song, Y., Zhang, J., Xu, H., Lin, Z., Chang, H., Liu, W., and Kong, L. (2020). Mesenchymal stem cells in knee osteoarthritis treatment: A systematic review and meta-analysis. J. Orthop. Transl. 24, 121–130. doi:10.1016/j.jot.2020.03.015 | |
Sun, H., Hu, S., Zhang, Z., Lun, J., Liao, W., and Zhang, Z. (2019). Expression of exosomal microRNAs during chondrogenic differentiation of human bone mesenchymal stem cells. J. Cell. Biochem. 120 (1), 171–181. doi:10.1002/jcb.27289 | |
Tang, S., Chen, P., Zhang, H., Weng, H., Fang, Z., and Chen, C., (2021). Comparison of curative effect of human umbilical cord-derived mesenchymal stem cells and their small extracellular vesicles in treating osteoarthritis. Int. J. Nanomedicine 16, 8185–8202. doi:10.2147/ijn.S336062 | |
Tao, S.-C., Yuan, T., Zhang, Y.-L., Yin, W.-J., Guo, S.-C., and Zhang, C.-Q. (2017). Exosomes derived from miR-140-5p-overexpressing human synovial mesenchymal stem cells enhance cartilage tissue regeneration and prevent osteoarthritis of the knee in a rat model. Theranostics 7 (1), 180–195. doi:10.7150/thno.17133 | |
ter Huurne, M., Schelbergen, R., Blattes, R., Blom, A., de Munter, W., and Grevers, L. C., (2012). Antiinflammatory and chondroprotective effects of intraarticular injection of adipose-derived stem cells in experimental osteoarthritis. Arthritis & Rheumatism 64 (11), 3604–3613. doi:10.1002/art.34626 |
Tofiño-Vian, M., Guillén, M. I., Pérez del Caz, M. D., Silvestre, A., and Alcaraz, M. J. (2018). Microvesicles from human adipose tissue-derived mesenchymal stem cells as a new protective strategy in osteoarthritic chondrocytes. Cell. Physiol. biochem. 47 (1), 11–25. doi:10.1159/000489739 | |
Toh, W. S., Brittberg, M., Farr, J., Foldager, C. B., Gomoll, A. H., Hui, J. H. P., and Kim, H. K. (2016). Cellular senescence in aging and osteoarthritis. Acta Orthop. 87 (sup363), 6–14. doi:10.1080/17453674.2016.1235087 | |
Toh, W. S., Lai, R. C., Hui, J. H. P., and Lim, S. K. (2017). MSC exosome as a cell-free MSC therapy for cartilage regeneration: Implications for osteoarthritis treatment. Seminars Cell. & Dev. Biol. 67, 56–64. doi:10.1016/j.semcdb.2016.11.008 | |
Utomo, L., van Osch, G. J. V. M., Bayon, Y., Verhaar, J. A. N., and Bastiaansen-Jenniskens, Y. M. (2016). Guiding synovial inflammation by macrophage phenotype modulation: An in vitro study towards a therapy for osteoarthritis. Osteoarthr. Cartil. 24 (9), 1629–1638. doi:10.1016/j.joca.2016.04.013 | |
Vaamonde-García, C., Riveiro-Naveira, R. R., Valcárcel-Ares, M. N., Hermida-Carballo, L., Blanco, F. J., and López-Armada, M. J. (2012). Mitochondrial dysfunction increases inflammatory responsiveness to cytokines in normal human chondrocytes. Arthritis & Rheumatism 64 (9), 2927–2936. doi:10.1002/art.34508 |
Wang, R., and Xu, B. (2021). TGF-β1-modified MSC-derived exosomal miR-135b attenuates cartilage injury via promoting M2 synovial macrophage polarization by targeting MAPK6. Cell. Tissue Res. 384 (1), 113–127. doi:10.1007/s00441-020-03319-1 | |
Wang, X., Li, Z., Cui, Y., Cui, X., Chen, C., and Wang, Z. (2021a). Exosomes isolated from bone marrow mesenchymal stem cells exert a protective effect on osteoarthritis via lncRNA LYRM4-AS1-GRPR-miR-6515-5p. Front. Cell. Dev. Biol. 9, 644380. doi:10.3389/fcell.2021.644380 | |
Wang, Y., Zhao, M., Li, W., Yang, Y., Zhang, Z., Ma, R., et al. (2021b). BMSC-derived small extracellular vesicles induce cartilage reconstruction of temporomandibular joint osteoarthritis via autotaxin–YAP signaling Axis. Front. Cell. Dev. Biol. 9, 656153. doi:10.3389/fcell.2021.656153 | |
Wang, Z., Yan, K., Ge, G., Zhang, D., Bai, J., Guo, X., and Kim, H. K. (2021c). Exosomes derived from miR-155-5p–overexpressing synovial mesenchymal stem cells prevent osteoarthritis via enhancing proliferation and migration, attenuating apoptosis, and modulating extracellular matrix secretion in chondrocytes. Cell. Biol. Toxicol. 37 (1), 85–96. doi:10.1007/s10565-020-09559-9 |
Wei, Y., , , Yan, L., Luo, L., Gui, T., Jang, B., Amirshaghaghi, A., and Kim, H. K. (2021). Phospholipase A2 inhibitor–loaded micellar nanoparticles attenuate inflammation and mitigate osteoarthritis progression. Sci. Adv. 7 (15), eabe6374. doi:10.1126/sciadv.abe6374 | |
Woo, C. H., Kim, H. K., Jung, G. Y., Jung, Y. J., Lee, K. S., Yun, Y. E., and Li, T. (2020). Small extracellular vesicles from human adipose-derived stem cells attenuate cartilage degeneration. J. Extracell. Vesicles 9 (1), 1735249. doi:10.1080/20013078.2020.1735249 | |
Wu, J., Kuang, L., Chen, C., Yang, J., Zeng, W.-N., Li, T., and Liu, Y. (2019). miR-100-5p-abundant exosomes derived from infrapatellar fat pad MSCs protect articular cartilage and ameliorate gait abnormalities via inhibition of mTOR in osteoarthritis. Biomaterials 206, 87–100. doi:10.1016/j.biomaterials.2019.03.022 | |
Xu, C., Zhai, Z., Ying, H., Lu, L., Zhang, J., and Zeng, Y. (2022). Curcumin primed ADMSCs derived small extracellular vesicle exert enhanced protective effects on osteoarthritis by inhibiting oxidative stress and chondrocyte apoptosis. J. Nanobiotechnology 20 (1), 123. doi:10.1186/s12951-022-01339-3 | |
Xu, X., Liang, Y., Li, X., Ouyang, K., Wang, M., and Cao, T., (2021). Exosome-mediated delivery of kartogenin for chondrogenesis of synovial fluid-derived mesenchymal stem cells and cartilage regeneration. Biomaterials 269, 120539. doi:10.1016/j.biomaterials.2020.120539 | |
Yan, L., Liu, G., and Wu, X. (2021). The umbilical cord mesenchymal stem cell-derived exosomal lncRNA H19 improves osteochondral activity through miR-29b-3p/FoxO3 axis. Clin. Transl. Med. 11 (1), e255. doi:10.1002/ctm2.255 | |
Yan, L., and Wu, X. (2020). Exosomes produced from 3D cultures of umbilical cord mesenchymal stem cells in a hollow-fiber bioreactor show improved osteochondral regeneration activity. Cell. Biol. Toxicol. 36 (2), 165–178. doi:10.1007/s10565-019-09504-5 | |
Yang, Q., Yao, Y., Zhao, D., Zou, H., Lai, C., Xiang, G., and Huang, X. (2021). LncRNA H19 secreted by umbilical cord blood mesenchymal stem cells through microRNA-29a-3p/FOS axis for central sensitization of pain in advanced osteoarthritis. Am. J. Transl. Res. 13 (3), 1245–1256. |
Yeo, R. W. Y., Lai, R. C., Zhang, B., Tan, S. S., Yin, Y., Teh, B. J., and Sai Kiang, L. (2013). Mesenchymal stem cell: An efficient mass producer of exosomes for drug delivery. Adv. Drug Deliv. Rev. 65 (3), 336–341. doi:10.1016/j.addr.2012.07.001 | |
Yu, W.-W., Wan, Q.-Q., Wei, Y., Li, Y.-T., Li, Q.-H., and Ye, T., (2022). Engineered extracellular vesicles: Regulating the crosstalk between the skeleton and immune system. Eng. Regen. 3 (3), 270–282. doi:10.1016/j.engreg.2022.06.004 |
Zavatti, M., Beretti, F., Casciaro, F., Bertucci, E., and Maraldi, T. (2020). Comparison of the therapeutic effect of amniotic fluid stem cells and their exosomes on monoiodoacetate-induced animal model of osteoarthritis. BioFactors 46 (1), 106–117. doi:10.1002/biof.1576 | |
Zhang, D., Lee, H., Zhu, Z., Minhas, J. K., and Jin, Y. (2016a). Enrichment of selective miRNAs in exosomes and delivery of exosomal miRNAs in vitro and in vivo. Am. J. Physiology-Lung Cell. Mol. Physiology 312 (1), L110–L121. doi:10.1152/ajplung.00423.2016 | |
Zhang, J., Rong, Y., Luo, C., and Cui, W. (2020). Bone marrow mesenchymal stem cell-derived exosomes prevent osteoarthritis by regulating synovial macrophage polarization. Aging 12 (24), 25138–25152. doi:10.18632/aging.104110 | |
Zhang, Q., Cao, L., Zou, S., Feng, Y., Miao, X., Huang, L., and Yongping, W. (2022a). Human umbilical cord mesenchymal stem cell-derived extracellular vesicles carrying MicroRNA-181c-5p promote BMP2-induced repair of cartilage injury through inhibition of SMAD7 expression. Stem Cells Int. 2022, 1–14. doi:10.1155/2022/1157498 |
Zhang, S., Chu, W. C., Lai, R. C., Lim, S. K., Hui, J. H. P., and Toh, W. S. (2016b). Exosomes derived from human embryonic mesenchymal stem cells promote osteochondral regeneration. Osteoarthr. Cartil. 24 (12), 2135–2140. doi:10.1016/j.joca.2016.06.022 | |
Zhang, S., and Jin, Z. (2022). Bone mesenchymal stem cell-derived extracellular vesicles containing long noncoding RNA NEAT1 relieve osteoarthritis. Oxidative Med. Cell. Longev. 2022, 1–21. doi:10.1155/2022/5517648 |
Zhang, S., Li, J., Li, C., Xie, X., He, J., Ling, F., and Zhilin, L. (2022b). CD73-Positive small extracellular vesicles derived from umbilical cord mesenchymal stem cells promote the proliferation and migration of pediatric urethral smooth muscle cells through adenosine pathway. Front. Bioeng. Biotechnol. 10, 895998. doi:10.3389/fbioe.2022.895998 | |
Zhang, S., Teo, K. Y. W., Chuah, S. J., Lai, R. C., Lim, S. K., and Toh, W. S. (2019). MSC exosomes alleviate temporomandibular joint osteoarthritis by attenuating inflammation and restoring matrix homeostasis. Biomaterials 200, 35–47. doi:10.1016/j.biomaterials.2019.02.006 | |
Zhang, X., Lu, Y., Wu, S., Zhang, S., Li, S., and Tan, J. (2022c). An overview of current research on mesenchymal stem cell-derived extracellular vesicles: A bibliometric analysis from 2009 to 2021. Front. Bioeng. Biotechnol. 10, 910812. doi:10.3389/fbioe.2022.910812 | |
Zhou, H., Shen, X., Yan, C., Xiong, W., Ma, Z., and Tan, Z., (2022). Extracellular vesicles derived from human umbilical cord mesenchymal stem cells alleviate osteoarthritis of the knee in mice model by interacting with METTL3 to reduce m6A of NLRP3 in macrophage. Stem Cell. Res. Ther. 13 (1), 322. doi:10.1186/s13287-022-03005-9 | |
Zhou, Y., Ming, J., Li, Y., Li, B., Deng, M., and Ma, Y., (2021). Exosomes derived from miR-126-3p-overexpressing synovial fibroblasts suppress chondrocyte inflammation and cartilage degradation in a rat model of osteoarthritis. Cell. Death Discov. 7 (1), 37. doi:10.1038/s41420-021-00418-y | |
Zhu, Y., Wang, Y., Zhao, B., Niu, X., Hu, B., and Li, Q., (2017). Comparison of exosomes secreted by induced pluripotent stem cell-derived mesenchymal stem cells and synovial membrane-derived mesenchymal stem cells for the treatment of osteoarthritis. Stem Cell. Res. Ther. 8 (1), 64. doi:10.1186/s13287-017-0510-9 | |
Zhuang, Y., Cheng, M., Li, M., Cui, J., Huang, J., Zhang, C., and Yu, H. (2022). Small extracellular vesicles derived from hypoxic mesenchymal stem cells promote vascularized bone regeneration through the miR-210-3p/EFNA3/PI3K pathway. Acta Biomater. 150, 413–426. doi:10.1016/j.actbio.2022.07.015 | |
Keywords: extracellular vesicles, osteoarthritis, mesenchymal stem cells, therapeutic treatment, EV engineering
Citation: Zhuang Y, Jiang S, Yuan C and Lin K (2022) The potential therapeutic role of extracellular vesicles in osteoarthritis. Front. Bioeng. Biotechnol. 10:1022368. doi: 10.3389/fbioe.2022.1022368
Received: 18 August 2022; Accepted: 01 September 2022;
Published: 16 September 2022.
Edited by:
Qian Feng, Chongqing University, ChinaCopyright © 2022 Zhuang, Jiang, Yuan and Lin. This is an open-access article distributed under the terms of the Creative Commons Attribution License (CC BY). The use, distribution or reproduction in other forums is permitted, provided the original author(s) and the copyright owner(s) are credited and that the original publication in this journal is cited, in accordance with accepted academic practice. No use, distribution or reproduction is permitted which does not comply with these terms.
*Correspondence: Changyong Yuan, eXVhbmNoYW5neW9uZzE5ODNAZm94bWFpbC5jb20=; Kaili Lin, bGlua2FpbGlAc2p0dS5lZHUuY24=
†These authors have contributed equally to this work
Disclaimer: All claims expressed in this article are solely those of the authors and do not necessarily represent those of their affiliated organizations, or those of the publisher, the editors and the reviewers. Any product that may be evaluated in this article or claim that may be made by its manufacturer is not guaranteed or endorsed by the publisher.
Research integrity at Frontiers
Learn more about the work of our research integrity team to safeguard the quality of each article we publish.