- 1Department of Scientific Research, Zhuzhou Hospital Affiliated to Xiangya School of Medicine, Central South University, Zhuzhou, China
- 2Department of Blood Transfusion, Zhuzhou Hospital Affiliated to Xiangya School of Medicine, Central South University, Zhuzhou, China
- 3Department of Clinical Laboratory, Zhuzhou Hospital Affiliated to Xiangya School of Medicine, Central South University, Zhuzhou, China
- 4Department of Cardiovascular Medicine, Zhuzhou Hospital Affiliated to Xiangya School of Medicine, Central South University, Zhuzhou, China
The outbreak of the coronavirus (COVID-19) has heightened awareness of the importance of quick and easy testing. The convenience, speed, and timely results from point-of-care testing (POCT) in all vitro diagnostic devices has drawn the strong interest of researchers. However, there are still many challenges in the development of POCT devices, such as the pretreatment of samples, detection sensitivity, specificity, and so on. It is anticipated that the unique properties of nanomaterials, e.g., their magnetic, optical, thermal, and electrically conductive features, will address the deficiencies that currently exist in POCT devices. In this review, we mainly analyze the work processes of POCT devices, especially in nucleic acid detection, and summarize how novel nanomaterials used in various aspects of POCT products can improve performance, with the ultimate aims of offering new ideas for the application of nanomaterials and the overall development of POCT devices.
Introduction
Point-of-care testing (POCT), which refers to detection immediately after sampling—without the need for professional personnel and equipment—has the advantages of being in real-time, fast, low cost, and so on (Buckle et al., 2021; Xu et al., 2021). Operators do not need to be medical professionals, and testing does not need to be in a fixed site, but can take place anywhere, e.g., in an emergency room, ambulance, outdoors or in the home (Ferreira et al., 2018; Yan et al., 2021). WHO has defined the criteria for POCT products as “ASSURED” (i.e., affordable, sensitive, specific, user-friendly, rapid and robust, equipment-free, and deliverable to end-users) (Land et al., 2019). However, there is a challenge in fulfilling these criteria, especially the sensitivity and specificity of the device. Thus, novel methods are urgently needed to ensure the rapid development of POCT devices.
Nanomaterials can be defined as materials possessing at least one external dimension within the nanometer scale of 1–100 nm (Suan Ng et al., 2022). Due to their special features, nanomaterials offer a wide range of possibilities in various fields, such as biocompatibility, fluorescence, electrical and thermal conductivity, and magnetism (Guo et al., 2018; Li et al., 2020; Tang et al., 2020; Gong N et al., 2021; Li J et al., 2022a). Meanwhile, nanomaterials have different shapes and can be divided into four types: 0D (e.g., spherical nanomaterials), 1D (e.g., nanotubes and nanowires), 2D (e.g., graphenes), and 3D (e.g., nanoprisms and nanoflowers). In line with their advantages and disadvantages, nanomaterials have been applied in different fields, such as the diagnosis of disease, detection of metal ions, and so on (Lai et al., 2018; Quesada-Gonzalez et al., 2018; Ma et al., 2020; Li J et al., 2021). Owing to their specific features, nanomaterials have also become integral in enhancing the performance of POCT devices.
In recent years, nanomaterials have been applied in multiple POCT devices to improve the efficiency of nucleic acid detection, especially in the face of the coronavirus (COVID-19) pandemic. Kim et al. (2021) found nanomaterial-based thin films were superior transducers that can reliably detect about 100 copies of the virus without being disturbed by interfering agents in a virus culture medium (WHO considers a viral load under 106 copies/mL negative). Interestingly, the relations between various thin film properties and the sensitivity of immunosensors were systematically analyzed using a special calculation method that showed good correlation. Hasanzadeh et al. (2021) introduced the application of nanomaterials in protective equipment, the loading and delivery of vaccines, and COVID-19 detection equipment. Xiao C et al. (2022) also systematically elaborated relevant studies regarding the use of nanomaterials in preventing viral spread, preparing vaccines, and diagnosing coronavirus. These works show that nanomaterials have penetrated all stages in the diagnosis and treatment of COVID-19, and hence their integration with POCT devices will be an increasing trend in the future detection market.
A clear understanding of the core technology of POCT devices is of paramount importance. The sensor as receiver controls the accuracy and sensitivity of the devices, because it can register the measured information and output as an electrical signal or in other required forms. A nanosensor is also a type of sensor, and has the advantages of miniaturization, digitalization, intelligence, multi-functionality, systematization, and network capacity, which shows its good application prospects in the development of POCT devices (Yang et al., 2018; Wu et al., 2020b; Li Q et al., 2021; Suan Ng et al., 2022; Xiao M. F et al., 2022). As Mitasha has described, using various biomolecules to detect specific analytes, activatable sensors in POCT products can be divided into immunological-based tests, nucleic acid (NA)-based tests, and other biomarker-based tests (Bharadwaj et al., 2021). Activatable sensors can improve specificity and sensitivity compared to traditional detection, hence providing more comprehensive information and obtaining faster detection speed (Wiraja et al., 2019; Ma et al., 2020; Xie et al., 2020). Therefore, the advantages of activatable sensors all meet the demands for POCT, and are widely used in POCT devices.
The NA-based nanosensor is an activatable sensor, which not only targets NA or proteins (e.g. aptamer), but can also combine antisense oligonucleotides or interfering RNAs to serve both therapeutic and diagnostic purposes (theragnostic) (Liu et al., 2017; Xie et al., 2020; He et al., 2021; Khan et al., 2021). Use of an NA-based sensor as a screening tool to detect COVID-19 produces highly specific and sensitive results (Dronina et al., 2021; Zhang M et al., 2022). In recent decades, the NA-based sensor has been used as a detector to assess heavy metal ion concentration, where it has also demonstrated good performance (Liu et al., 2018; Guo et al., 2021; Chen et al., 2022). In summary, deeper insights into POCT devices and their application include disease diagnosis and therapy, pathogen diagnosis, heavy metal ion monitoring, and so on (Cho et al., 2018; Zeng et al., 2020; Kim H et al., 2021; Mo et al., 2021; Jani and Peter., 2022). However, using a NA-based sensor to achieve the purpose of detection in POCT, to meet the abovementioned WHO-defined criteria, requires greater accuracy and shorter response times, and can, to a certain extent, be achieved by combination with nanomaterials.
In this review, we firstly clarify the current status and trends in POCT in recent years to elicit the latest information, as these aspects have been broadly reviewed in general terms (Prattis et al., 2021; Zhang N et al., 2022). Moreover, we focus on introducing the role of nanomaterials in the work processes of POCT devices, as illustrated in Figure 1, including loading the sample, detection, and data display. In relation to detection especially, we elaborate two functions: amplification and non-amplification, and highlight the important role of nanomaterials in these functions. Overall, this work aims to promote further development of POCT devices, and in particular, POCT devices related to nucleic acid detection. It also offers new ideas for the continued development of POCT devices with high sensitivity, low assay cost, and low power requirements.
Loading the sample
To reduce interference material in the sample, and to avoid influence on the determination of the result, sample purification is an important step that cannot be ignored. Currently, for the extraction and purification of NA, common methods are magnetic bead-based, column-based, and phenol-chloroform-based (Mou et al., 2019; Wang S et al., 2021; White et al., 2022). In the early days of POCT devices, few researchers considered the project of integrating sample preprocessing into POCT devices. It is well known that the complex and time-consuming sample preparation process should be performed by professionals, because direct loading of samples under unpurified conditions can cause high false positives and negative results. These processes are difficult to promote, which hinders further development of POCT technology.
The application of nanomaterials can speed up and automate sample purification, which also helps users simplify sample pre-processing and hence improve accuracy in the POCT procedure. Magnetic nanoparticles have a pivotal position, particularly in NA purification (He et al., 2017; Tang et al., 2018; Dong et al., 2020). A variety of extraction methods involving magnetic nanomaterials are emerging. Kang et al. (2021) developed a simple NA purification system performed in a plastic Pasteur pipette, using magnetic nanoparticles that enter the NA extraction process, as shown in Figure 2. Unlike traditional approaches, the system could complete NA extraction from various samples, including swabs, serum, milk, and pork, in 15 min without assistance from electrical instruments, and the obtained extract had a lower limit for subsequent amplification detection. The system satisfies the requirements for POCT and offers a new idea for integrated detection in POCT devices.
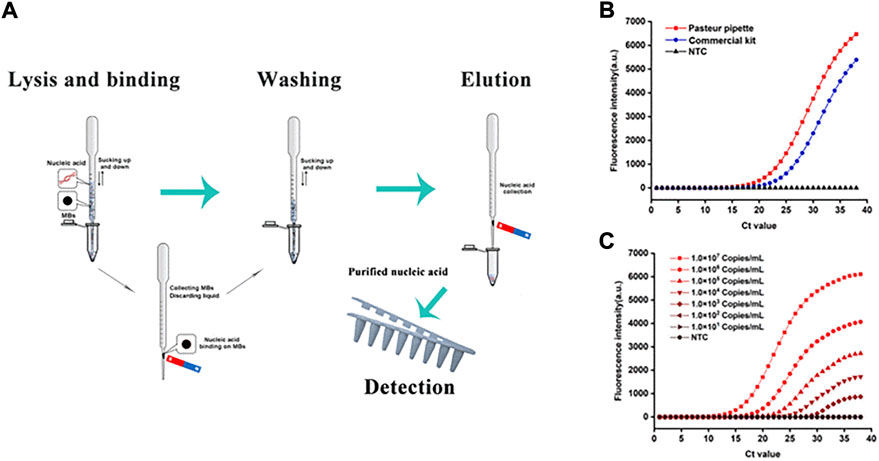
FIGURE 2. Nucleic acid extraction in Pasteur pipette system and detection results. (A) The schematic illustration of lysis and binding step, washing step, elution step and nucleic acid extraction in Pasteur pipette system, and detection step. (B) Comparison of extraction effect between Pasteur pipette system and the commercial kit. (C) The sensitivity of the Pasteur pipette system. Copyright 2021 Elsevier Inc.
There is a similar study from Pearlman et al. (2020), and work is ongoing to improve the capturing ability of magnetic beads. Bacteria have a different cell surface hydrophobicity, and when the contact angle of the material and water is approximately 90°, the highest bacterial adhesion is shown (Krasowska and Sigler., 2014; Li et al., 2019). In line with this feature, Lee et al., (2021) synthesized tryptamine-functionalized magnetic nanoparticles. Their strategy was to adopt the enrichment of bacteria before DNA extraction. The result demonstrated the extraction efficiency of 1 CFU/ml was 10 times better than that of the commercial kit, and that the process had good stability and accuracy. A novel g-Fe2O3/PEDOT hybrid nanocomposite was synthesized by da Silva et al. (2021). Here modified nanomagnetic beads were used to extract the DNA from blood and bacteria, and the yields after verification were 6.4 mg and 7.3 mg, respectively, and the captured DNA could be directly used for downstream experiments, such as PCR amplification, without being detached from the nanocomposite.
The limitation of magnetic nanoparticles is their slow response to external magnetic stimuli, which means that they need to form larger magnetic complexes by using high nanoparticle concentrations or have long incubation times (Burklund et al., 2020). Poncelet et al. (2021) developed suspended magnetic nanoparticle assemblies (M-clouds) for DNA extraction. The detailed principle is shown in Figure 3. The M-clouds have a strong magnetic field, and can enable almost instant contact between capture sites and the target analyte when the sample solution is flowing. The real result shows that the efficiency of E.coli capture and DNA extraction by M-cloud devices was in the range of 17.3–34.1% when the concentration was 103 CFU/ml in environmental water samples. Extraction reagents surely also affect the efficiency of DNA capture (Tang et al., 2013; He et al., 2019). In short, the purpose of pre-test treatment for complex samples is to improve the purity and yield of NA, and all the work has been done to this end. The purification and enrichment of bio samples to obtain high quantity NA is crucial for downstream detection and analysis. Pre-processing of complex samples is therefore essential, and from proper processing it will be easier to obtain highly accurate detection results.
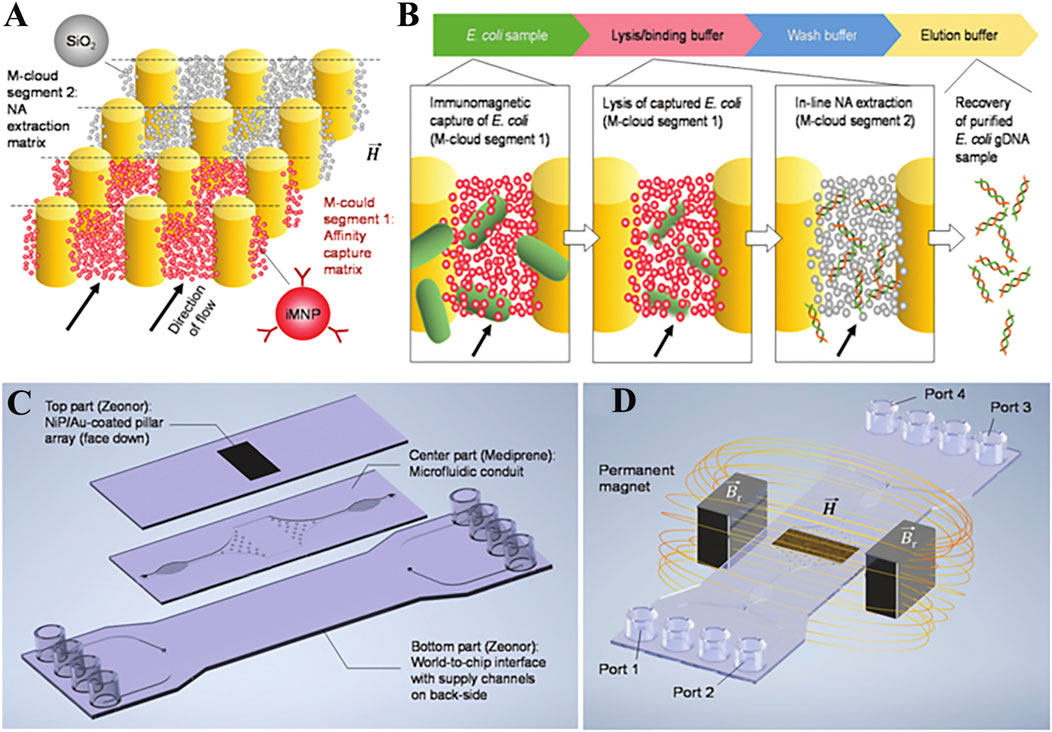
FIGURE 3. Concept of in situ microbial capture and DNA isolation. (A) Schematic illustration of segmented, multifunctional M-cloud configuration used within this work. (B) Flow chart detailing the order in which assay components are introduced to the M-cloud, along with illustrations of the main steps. (C) Schema of the microfluidic chip developed for process integration. (D) Illustration of the experimental setup used to generate M-cloud capture regions inside the microfluidic chip. Copyright 2022 Royal Society of Chemistry.
Detection
Obtaining high-quality DNA is the first step in optimizing detection results, but improvement in the sensitivity and specificity of detection should not be ignored. Therefore, researchers are taking great pains to improve detection methods in order to obtain more exact outcomes. At present, there are two methods for NA detection: amplification and non-amplification. They each have their advantages and disadvantages, which are unavoidable to some extent. Researchers strive to improve these advantages and reduce the disadvantages. The use of nanomaterials can help to address some of the key issues, and in the next section we mainly introduce NA detection methods based on use of nanomaterials in POCT devices.
Amplification
Exponential amplification in NA detection helps researchers obtain sufficient information from low NA levels. Zhang W et al. (2022) reviewed the application of different nanomaterials (0D, 1D, 2D, and 3D) in isothermal NA amplification, and identified the following advantages of nanomaterials: increasing the activity and stability of the amplification enzyme, activating the activity of enzymes, inhibiting nonspecific amplification, and promoting helicase-dependent denaturation. These advantages are all driving forces in promoting the development of POCT devices.
POCT devices have some limitations, such as complex temperature controls, considerable weight, and high background signals. To solve these problems, more novel technologies have been developed. For example, in the work of Lee et al. (2020), a novel system based on a nanogap-embedded, active whispering-gallery mode resonator was developed to detect the product of recombinase polymerase amplification. Resonators are the core components of detection systems. At present, nano-gap active resonators, disk resonators, and ring resonators are the most common. Ultra-high quality factor optical resonators (Chen et al., 2017) are among the disk resonators, while silicon microring resonators (Park et al., 2013) are ring resonators. Detailed parameter comparisons are given in Table 1, with nanogap active resonators possessing higher sensitivity and lower background signals compared to other resonators. Meanwhile, the author thinks the system can become a POCT device by combining it with a visible LED source, because the size and performance of the entire system fits the demands for POCT devices. Direct detection without NA extraction has become a hot topic, because of the simplified detection steps, shortened detection time, and decreased detection costs, although distractions in the sample or impure NA will affect the detection results.
Similarly, Yi et al. (2021) have synthesized a nanoporous hydrogel with self-cleaning properties for application to direct NA analysis without the need for any sample pretreatment. The detection principle is shown in Figure 4. The inhibitor was adsorbed and removed by the formation of a cross-linking network structure of hydrogel, and next an amplification reaction was produced. The method gives us the new idea that nanoporous hydrogel can become a sort of “dustman” in removing some adverse factors and achieving an efficient NA amplification reaction. Appropriately amplifying the detection signal is necessary. For example, Yang et al. (2020), reviewed detection signal amplification strategies for nanomaterial-based photoelectrochemical (PEC) biosensors, and put forward PEC instruments that are simpler, more cost-effective, with higher analytical detection performance and easier miniaturization. In addition, the PEC sensors can reduce background signals, and have higher sensitivity than conventional electrochemical methods. Alafeef et al. (2021) synthesized the antisense oligonucleotide (ASO)-capped gold nanoparticles (AuNPs) to detect the product of loop-mediated isothermal amplification. By using clinical sample evolution, the accuracy, sensitivity, and specificity of the detection were >98.4%, >96.6%, and 100%, respectively, with a detection limit of 10 copies/μL.
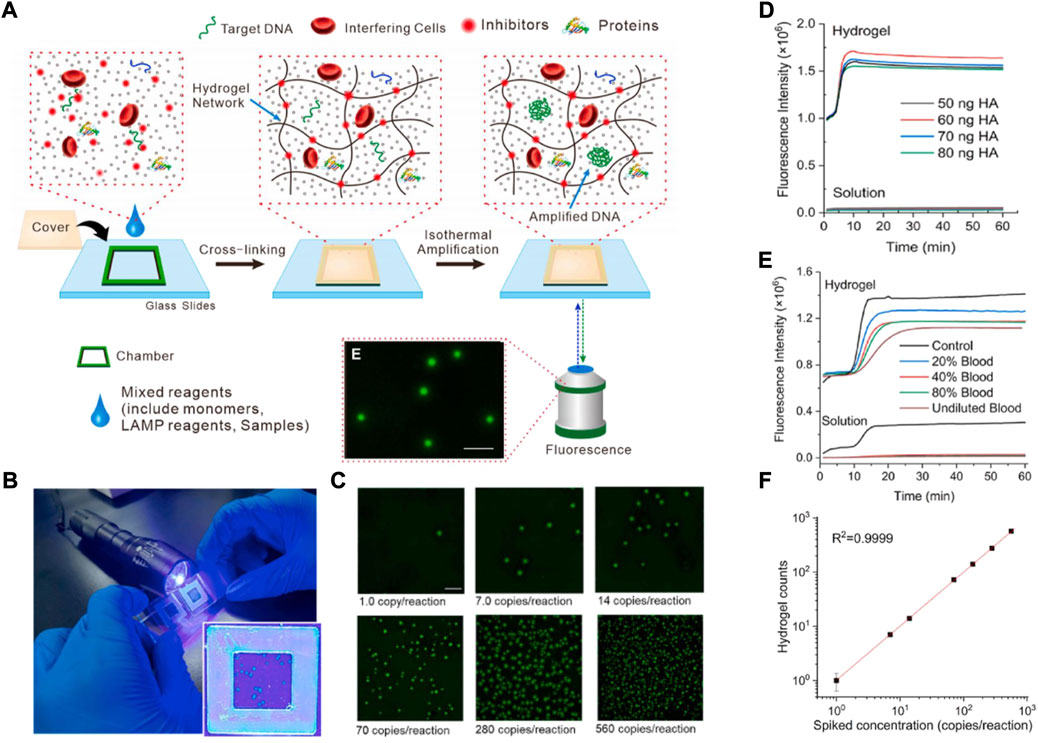
FIGURE 4. (A) Schematic illustration of isothermal amplification inside the nano porous hydrogel for digital LAMP in complex matrices. (B) The photograph for naked-eye counts of amplicon dots in the hydrogel under the LED flashlight. (C) Endpoint fluorescence images of hydrogel after LAMP reaction for unprocessed whole blood spiked with a series concentration of the E.coli genome. Scale bar of 1 mm. (D) The impact of different concentrations of whole blood on LAMP reaction performed in the hydrogel or solution. (E) The impact of different concentrations of HA on LAMP reaction in the hydrogel or solution. (F) Comparison of hydrogel counts to the spiked E. coli genome concentration in unprocessed blood. LAMP: loop-mediated isothermal amplification. Copyright 2021 Elsevier B.V.
Rolling circle amplification (RCA) is an isothermal NA amplification method and has ultrahigh specificity for nucleotide variants. Tian et al. (2020), performed two rounds of RCA, and the product of the second round of RCA combined magnetic nanoparticles to generate amplicon coils. The opto magnetic signal can then be charged with the increased amplification product, thus achieving real-time NA detection. Similarly, Wang et al. proposed a lateral flow gene assay (LFGA), combining recombinase polymerase amplification (RPA) and nucleic acid lateral flow (NALF), to directly detect a DNA sample using an oligonucleotide probe. The detection process is described in detail in Figure 5. AuNPs were applied as a dye in RPA amplification product analysis. The whole detection process was completed under 37–42°C in 30 min. This work provides a portable POCT platform without the need for any costly instruments (Wang Z et al., 2021).
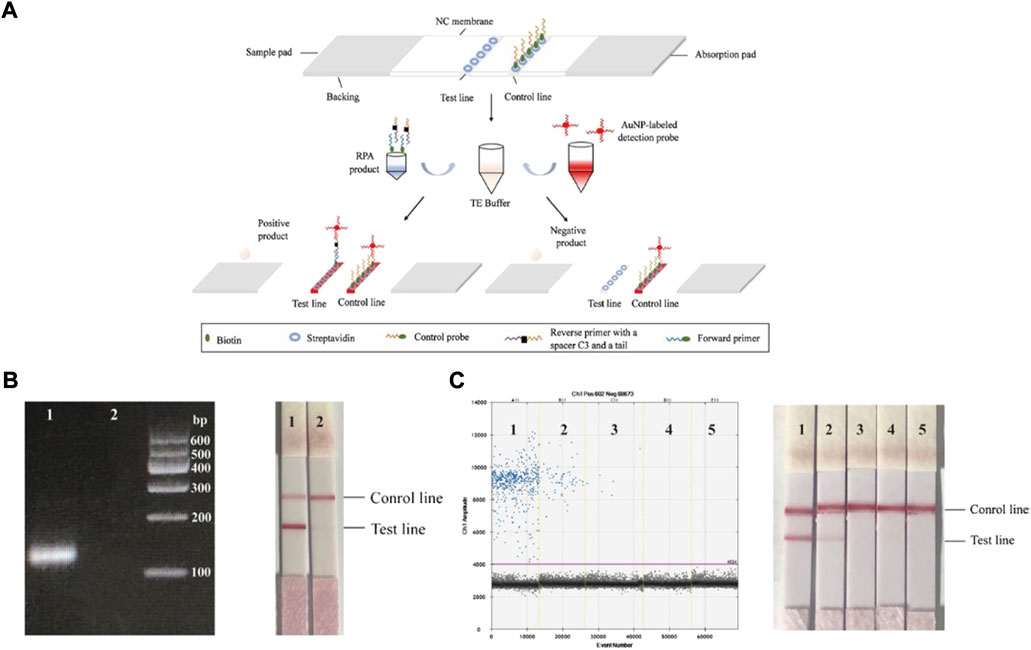
FIGURE 5. (A) The principle of lateral flow gene assay. The RPA product and the AuNP-labeled detection probe were added to TE buffer, and then the mixed droplets were added to the sample pad of the strip. In the presence of positive products, there will be two red lines on both the test line and control line. In the presence of negative products, there will be only one red line on the control line. (B) The detection result of sample, Lane 1: target DNA; Lane 2: double-distilled H2O. (C) A series of tenfold DNA diluents from 101 to 104 extracted from blood infected with ASFV were used to determine the LOD, Lane 1: 10-fold dilution; Lane 2: 102-fold dilution; Lane 3: 103-fold dilution; Lane 4: 104-fold dilution; Lane 5: NTC. Copyright 2022 Springer Nature Switzerland AG.
These results show that nanomaterials make a contribution to POCT devices in the matter of NA amplification detection, mainly concerning amplified detection signals and reduced nonspecific amplification, and a large body of literature supports this result (Jiang et al., 2013; Nie et al., 2014; Yang et al., 2017; Jiang et al., 2018; Wu et al., 2020a). Overall, nanomaterials have made an outstanding contribution in optimizing NA amplification, and can not only improve the accuracy of detection, but can also shorten detection time and optimize the detection process. Amplification detection requires enzyme assistance, and enzymes need a separate chamber in order to work, and specifically, most reactions require temperature to activate the enzymes. This means that POCT products need to be larger to some extent and are more difficult to operate. However, the above methods can be applied to miniaturized instruments to achieve POCT detection.
Non-amplification
Direct detection of NA without amplification is more suitable for POCT devices. Meanwhile, the requirement for sample purification is also lower without the involvement of an amplifying enzyme. Nevertheless, here the greatest limitation is low sensitivity, and how to capture more NA in samples and collect as many real signals as possible are some of the problems that researchers need to think about (Mondal et al., 2021; Sun 2022). AuNPs can be designed to suit diversified functionalization options, due to easy surface modification (Lai et al., 2018; Sarfraz and Khan, 2021; Li J et al., 2022b; Liu Y et al., 2022).
AuNPs have the feature of localized surface plasmon resonance, which give them a signal 10- to 10,000-times higher than fluorescent dyes or quantum dots, and this can be detected in simple dark-field microscope. Li et al. (2016) developed a nonamplification sandwich assay for NA detection, and the detection principle is shown in Figure 6. The result shows the length and concentration of target DNA: while the dose of AuNPs can affect the detection efficiency, the detection rate of the target DNA is almost unaffected in a complex matrix containing a variety of proteins and other contaminants. Interestingly, the limit of detection of a clinic sample is 3 fM, and only requires a simple dark-field microscope, which shows that the assay has high simplicity and sensitivity. Ota et al. (2021) used 4-cyano-N-(2-mercaptoethyl) benzamide (4CMB) and oligonucleotides to modify the surface of gold nanorods and finally form a sandwich structure which can detect the target DNA under 1 nM. The limitations of the structure are that the concentration of target DNA is unquantifiable and needs the auxiliary detection of a micro-Raman spectroscope.
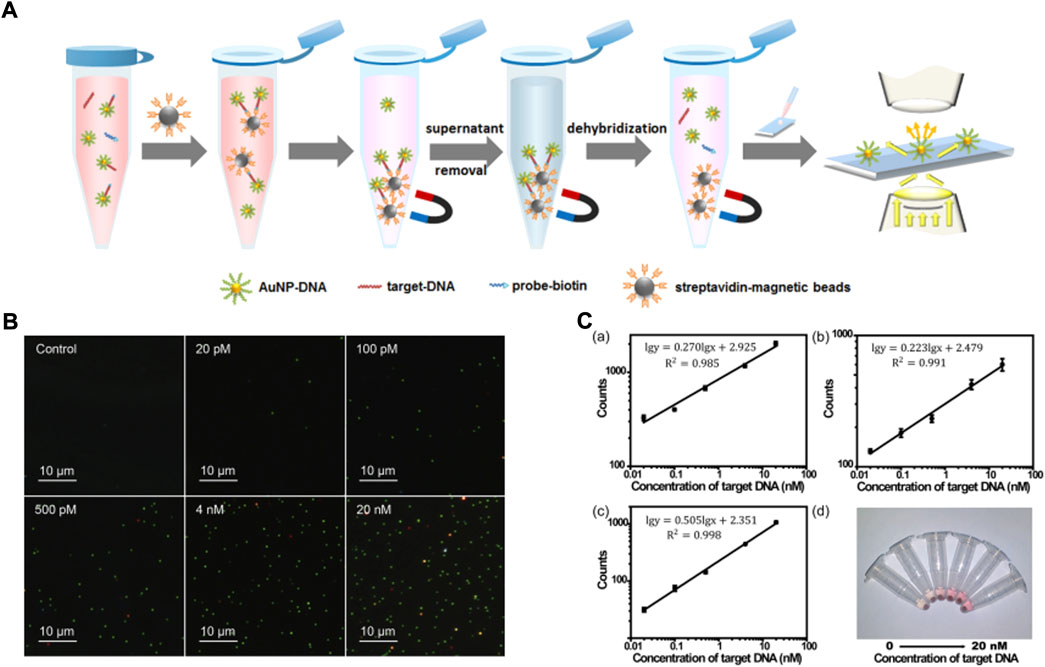
FIGURE 6. (A) The schematic illustration of nucleic acid extraction method combining the sandwich-type DNA hybridization strategy and magnetic separation. (B) Dark-field images of AuNP-DNA with different concentrations of 40-nucleotide target DNA. (C) Automatic counting results for target DNA with different lengths: (a) 24 nucleotides, (b) 40 nucleotides, and (c) 60 nucleotides; (d) the photograph of the supernatant with different concentrations of 40-nucleotide target DNA (from left to right, 0 p.m., 20 p.m., 100 p.m., 500 p.m., 4 nM, and 20 nM). Copyright 2016 American Chemical Society. Copyright 2021 Elsevier B.V.
A DNA nanostructure is a technique for artificially designing and producing useful NA structures, which, as a bridge, connect the relationship between optoelectronics, thermology dynamics, and genetic information (Zhang et al., 2020; Henry and Stephanopoulos, 2021; Li S et al., 2021; Qin et al., 2022). Although not active, a DNA nanostructure has these advantages: low toxicity, high resistance to degradation in biological media, and an ideal ability to enter cells without transfection agents. These advantages make it a good tool for detecting the NA in live cells (Zhou et al., 2021; Li G et al., 2022a; Li G et al., 2022b). Li et al. (2019) assembled a DNA tetrahedral structure using oligonucleotides to protect the DNAzyme and fluorescent probe. To prevent the uncontrollability of the DNAzyme, the author designed a unique structure to lock its activity, with specific miRNA as the “key” to activating the DNAzyme. The DNAzyme can obtain the dissociated fluorescent signal by separating out the fluorescence-quenched structure, thus achieving the purpose of fluorescence detection. This detection method, compared with its nonamplification molecular beacon counterpart, exhibited at least 10 times higher detection sensitivity (the limit of detection is 16 p.m.) and was able to distinguish miRNA targets from corresponding family members. Of course, the structures can not only detect the miRNA, but are also applied in NA detection, especially short fragment NA. Non-amplification makes NA detection convenient and speedy, and with its shortcomings addressed by some of the above work, is an important part of the development of POCT technology.
In summary, non-amplification NA detection still has huge difficulties, especially for samples with low levels of NA, but it has advantages for direct detection in complex samples. Therefore, it is important to select appropriate detection methods for POCT products, given the method has an important relationship with the samples tested. When developing new POCT products, it is essential to consider the object for detection.
Data display
In this section, we only discuss the forms of data presentation, and not how the data is processed. The data display has very little to do with the nanomaterial, but it is an integral part of POCT, especially in terms of the user experience. Currently, the personal computer (PC) and built-in touch screen are the two main forms for presenting data visualization, but their disadvantages cannot be ignored. For example, the PC is an expensive and bulky device compared with some POCT products, which is bad news for users. The built-in touch screen solves the problem of needing the supplementary PC, but the use of screens in POCT devices, while on the one hand increasing the instrument’s size, on the other offers a poor user experience.
Given its popularity, the smartphone is a good choice as a tool for data display. Its characteristics, such as simplicity, affordability and user-friendliness, makes it acceptable to the public. Meanwhile, three data transmission modes, wired, wireless, and Bluetooth, can meet user needs in different situations. Furthermore, smartphones are widely used as detector and analysis devices (Pujari 2021; Pujari et al., 2021; Zhang Z et al., 2022), due to their built-in high-definition cameras. Li Y et al. (2021) built a novel optical detection system for use with a smartphone. Fluorescent images are taken from the camera of a smartphone, and the application is driven by an Android smartphone to analyze the fluorescent signal, and ultimately obtain detection results. The specific detection process and results are shown in Figure 7. Liu S et al. (2022) did the same thing, choosing SYTO9 as a fluorescence reagent to stain the amplification product, which was then observed and analyzed by smartphone. Their result was exciting, and the limit of detection for pathogenic bacteria was 6 copies/mL in the green channel, with the whole process carried out under a constant temperature. Powerful smartphones have brought benefits to productivity, and the lives of people in all aspects, and provide a new idea for the development of POCT. The strong fluorescence of nanomaterials (Li et al., 2018; Mei et al., 2021; Ye et al., 2021) means they can be accurately captured by smartphones, which might create a trend in POCT development in the future.
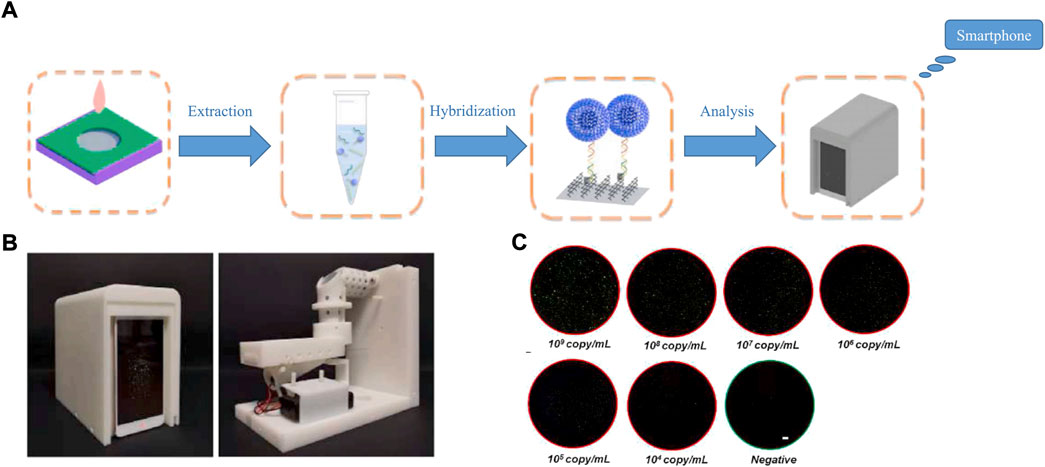
FIGURE 7. (A) Flow chart of the amplification-free smartphone-based detecting technique. (B) Portable fluorescence nucleic acid detector. (C) Captured FoVs corresponding to different virus concentration. White bar represents 1 μm. Copyright 2021 Elsevier B.V.
However, in order to obtain accurate test results, an adequate fluorescence signal and sensitive detection are indispensable. It is also worth noting the smartphone is not professional camera equipment, and can experience data loss or sensitivity decrease, and should thus be treated with caution when used as a detector.
Discussion and conclusion
Nanomaterials have attracted increased attention due to their unique physical and chemical properties, generating considerable interest in many fields, including biotechnology, catalysis, electronics, optics, and drug-loading (Liu et al., 2020; Gong L et al., 2021; Zhang M et al., 2021; Choi et al., 2022; Gao et al., 2022; Pang et al., 2022). In this review, we focused on the area of NA detection in POCT devices. There is no doubt that the high efficiency and accuracy of NA detection can greatly improve the performance of POCT devices. It is thus important to obtain high quality NA and highly sensitive identification processes. Nanomaterials play a crucial role in NA detection, with detection results showing high performance in terms of sensitivity and selectivity. A lot of the literature reveals that modified nanomaterials not only improve detection capability (about 10–1000 fold compared to routine PCR), but also address the complexity of the sample (Chen et al., 2020; Fang et al., 2021; Liu et al., 2021; Zhang C et al., 2022), such as in the detection of Salmonella Typhimurium in complex food environments using a novel fluorescent platform of DNA-stabilized silver nanoclusters. The authors assessed the effectiveness of the main method in terms of sensitivity, specificity, and detection rate in complex samples and their results showed that the detection rate had a linear concentration range of Salmonella Typhimurium from 4.6×102 to 4.6 × 107 CFU/ml, with the limit of detection reaching 6.6 × 102 CFU/ml in food samples (Yang et al., 2021). When novel nanomaterials are applied in NA detection, the detection limit for biomolecules is one of the key parameters in evaluating the feasibility of the assay. Hence researchers want to improve the sensitivity of detection by combining the specificity and versatility of NA with the intrinsic properties of nanomaterials. In summary, the design of nanomaterials has become a primary research goal in the biomedical field. With advancements in the artificial intelligence age, new smart technologies have an indispensable place in POCT devices. Sow et al. (2020) summarized the advantages of using smart materials in different work stages of POCT devices, but the challenge presented by high signal-to-noise ratio and accurate detection of analytes in the POCT platform still exists. In general, the main means of promoting the POCT industry in the future will be to use the characteristics of the nanomaterials combined with suitable detection methods.
At the same time, the limitations of the use of nanomaterials also attracts the attention of researchers. Toxicity, stability, and biocompatibility are major problems hindering in vivo application, and when it comes to in vitro testing, stability is the primary consideration (Ahmed et al., 2021; Jobdeedamrong et al., 2021; Li Y et al., 2021; Zhang Q et al., 2021). No matter how well designed, the deviation in repeated experiment results is too large, which leads to the greatly reduced reliability of such experimental results. Agglomeration of nanoparticles is another main factor that may affect their stability (Magesa et al., 2020; Sun et al., 2021; Diephuis et al., 2022). These properties are nevertheless beneficial in many fields, such as vascular tissue engineering (Rahimnejad et al., 2021). In terms of NA detection, aggregation will affect the efficiency of extraction and detection, and cause the failure of the POCT device. Moreover, the environmental pollution caused by the degradation-resistant nanomaterials should not be ignored, for as the food chain circulates, it accumulates, and eventually enters the body and harms human health. Therefore, easily degradable, non-toxic, and highly stable nanomaterials are the focus for researchers, and the key factors driving the sustainable development of the field.
Application of nanomaterials in POCT devices has become a hot topic for research in recent years, owing in part to the impact of COVID-19, which further stimulated POCT device development. POCT devices represent the future trend in the field of molecular diagnosis, given there is no need for a fixed testing site, as reagents and instruments are portable and can be operated in a timely manner. A problem was posed: i.e., the need to ensure that requirements for speed, quality, and cost all are met in COVID-19 detection (Ding et al., 2020). Beach et al. (2021) thought that POCT could become a key diagnostic tool for health-care providers carrying out COVID-19 rapid-testing, and they identified what needed attention when using POCT devices for detection, such as an established quality assurance framework and the evaluation of clinical performance. While POCT devices have many advantages as a detection tool, they are still a long way from reaching the diagnostic market and being widely used. Therefore, according to the WHO-defined ASSURED criteria, integrating appropriate detection methods to be applied in different fields will shape the direction of our efforts.
Operable paper-based POCT devices have advantages, such as self-driven flow, high surface to volume ratio, and superb biocompatibility. Researchers have thus intensively studied these, and some have produced products that have become commercialized (Liu et al., 2021). For example, Jia et al. (2021) commented on applications of a paper-based POCT device, putting forward reagent storage and sample preparation as the key research directions for the device, in the hope it would have high accuracy while basically meeting WHO’s ASSURED criteria through the endeavors of academia and industry.
Overall, we mainly introduced herein the application of POCT devices to NA testing. The detection aspect is crucial in POCT devices, owing to the development of detection technology closely related to obtaining high quality data. Of course, the other two aspects, loading sampling and data display, cannot be ignored either. Nanomaterials have been widely used in POCT devices, so we have summarized their advantages and use in various parts of these devices, so that researchers might learn about the latest developments in relation to NA detection. However, POCT devices still have their challenges, including low accuracy of detection, the requirement for specialized reagents, and lack of a uniform evaluation system. Of note, in NA detection, fully enclosed detection can reduce the hazards of nucleic acid aerosols, and hence increase the credibility of the result. Some POCT devices associated with NA detection are only a simple combination of a nucleic acid extraction instrument and detector. Strictly speaking, they do not belong to the category of POCT devices. Therefore, developing uniform industry standards is a priority. Second, improving the accuracy and sensitivity of POCT devices will be the future developmental trend, while the use of nanomaterials will be the intrinsic driving force promoting the development of POCT devices. The review we have presented here will be helpful to researchers in promoting POCT products, and will also provide new ideas for creating better healthcare systems for current and future types of disease detection.
Author contributions
The author contributions as follows: ZH: writing, original draft preparation, and methodology; CL, ZL, and ZC: investigation; XC and XC: visualization and figure processing; YG: supervision and funding acquisition. All authors reviewed the final manuscript.
Funding
This work was supported by Hunan Health Committee Scientific Research Project of China (Grant No. 202103010009).
Conflict of interest
The authors declare that the research was conducted in the absence of any commercial or financial relationships that could be construed as a potential conflict of interest.
Publisher’s note
All claims expressed in this article are solely those of the authors and do not necessarily represent those of their affiliated organizations, or those of the publisher, the editors, and the reviewers. Any product that may be evaluated in this article, or claim that may be made by its manufacturer, is not guaranteed or endorsed by the publisher.
References
Ahmed, J., Gultekinoglu, M., Bayram, C., Kart, D., Ulubayram, K., and Edirisinghe, M. (2021). Alleviating the toxicity concerns of antibacterial cinnamon-polycaprolactone biomaterials for healthcare-related biomedical applications. MedComm 2, 236–246. doi:10.1002/mco2.71
Alafeef, M., Moitra, P., Dighe, K., and Pan, D. (2021). Rna-extraction-free nano-amplified colorimetric test for point-of-care clinical diagnosis of Covid-19. Nat. Protoc. 16, 3141–3162. doi:10.1038/s41596-021-00546-w
Beach, L. A., Fung, A. W. S., Knauer, M. J., Shaw, J. L. V., and Taher, J. (2021). Rapid Covid-19 testing: Speed, quality and cost. Can you have all three? Clin. Biochem. 95, 13–14. doi:10.1016/j.clinbiochem.2021.05.009
Bharadwaj, M., Bengtson, M., Golverdingen, M., Waling, L., and Dekker, C. (2021). Diagnosing point-of-care diagnostics for neglected tropical diseases. PLoS Negl. Trop. Dis. 15, e0009405. doi:10.1371/journal.pntd.0009405
Buckle, P., Micocci, M., Tulloch, J., Kierkegaard, P., Parvulescu, P., Thompson, C., et al. (2021). Covid-19 point-of-care testing in care homes: What are the lessons for policy and practice? Age Ageing 50, 1442–1444. doi:10.1093/ageing/afab101
Burklund, A., Petryk, J. D., Hoopes, P. J., and Zhang, J. X. J. (2020). Microfluidic enrichment of bacteria coupled to contact-free lysis on a magnetic polymer surface for downstream molecular detection. Biomicrofluidics 14, 034115. doi:10.1063/5.0011908
Chen, D., Kovach, A., Shen, X., Poust, S., and Armani, A. M. (2017). On-chip ultra-high-q silicon oxynitride optical resonators. ACS Photonics 4, 2376–2381. doi:10.1021/acsphotonics.7b00752
Chen, M., Nguyen, T. T., Varongchayakul, N., Grazon, C., Chern, M., Baer, R. C., et al. (2020). Surface immobilized nucleic acid-transcription factor quantum dots for biosensing. Adv. Healthc. Mat. 9, e2000403. doi:10.1002/adhm.202000403
Chen, Z., Zhao, K., He, Z., Luo, X., Qin, Z., Tan, Y., et al. (2022). Development and evaluation of a thermostatic nucleic acid testing device based on magnesium pyrophosphate precipitation for detecting enterocytozoon hepatopenaei. Chin. Chem. Lett. 33, 4053–4056. doi:10.1016/j.cclet.2022.01.072
Cho, I. H., Lee, J., Kim, J., Kang, M. S., Paik, J. K., Ku, S., et al. (2018). Current technologies of electrochemical immunosensors: Perspective on signal amplification. Sensors (Basel). 18, 207. doi:10.3390/s18010207
Choi, S. H., Lee, J. S., Choi, W. J., Seo, J. W., and Choi, S. J. (2022). Nanomaterials for iot sensing platforms and point-of-care applications in South Korea. Sensors (Basel) 22, 610. doi:10.3390/s22020610
da Silva, R. J., Pedro, G. C., Gorza, F. D. S., Maciel, B. G., Ratkovski, G. P., Mojica-Sanchez, L. C., et al. (2021). DNA purification using a novel gamma-fe2o3/pedot hybrid nanocomposite. Anal. Chim. Acta X. 1178, 338762. doi:10.1016/j.aca.2021.338762
Diephuis, W. R., Molloy, A. L., Boltz, L. L., Porter, T. B., Aragon Orozco, A., Duron, R., et al. (2022). The effect of agglomeration on arsenic adsorption using iron oxide nanoparticles. Nanomater. (Basel) 12, 1598. doi:10.3390/nano12091598
Ding, H., Yin, S., Cheng, Y., Cai, Y., Huang, W., and Deng, W. (2020). Neurologic manifestations of nonhospitalized patients with Covid-19 in wuhan, China. MedComm 1, 253–256. doi:10.1002/mco2.13
Dong, H., Tang, C., He, Z., Liu, H., Xu, Y., Huang, H., et al. (2020). Rapid identification of diarrheagenic escherichia coli based on barcoded magnetic bead hybridization. Chin. Chem. Lett. 31, 1812–1816. doi:10.1016/j.cclet.2020.03.002
Dronina, J., Bubniene, U. S., and Ramanavicius, A. (2021). The application of DNA polymerases and cas9 as representative of DNA-modifying enzymes group in DNA sensor design (review). Biosens. Bioelectron. X. 175, 112867. doi:10.1016/j.bios.2020.112867
Fang, Y., Liu, H., Wang, Y., Su, X., Jin, L., Wu, Y., et al. (2021). Fast and accurate control strategy for portable nucleic acid detection (pnad) system based on magnetic nanoparticles. J. Biomed. Nanotechnol. 17, 407–415. doi:10.1166/jbn.2021.3028
Ferreira, C. E. S., Guerra, J. C. C., Slhessarenko, N., Scartezini, M., Franca, C. N., Colombini, M. P., et al. (2018). Point-of-care testing: General aspects. Clin. Lab. 64, 1–9. doi:10.7754/Clin.Lab.2017.170730
Gao, Y., Chen, X., Tian, T., Zhang, T., Gao, S., Zhang, X., et al. (2022). A lysosome-activated tetrahedral nanobox for encapsulated sirna delivery. Adv. Mat., e2201731. doi:10.1002/adma.202201731
Gong, L., Zhao, L., Tan, M., Pan, T., He, H., Wang, Y., et al. (2021). Two-photon fluorescent nanomaterials and their applications in biomedicine. J. Biomed. Nanotechnol. 17, 509–528. doi:10.1166/jbn.2021.3052
Gong, N., Sheppard, N. C., Billingsley, M. M., June, C. H., and Mitchell, M. J. (2021). Nanomaterials for t-cell cancer immunotherapy. Nat. Nanotechnol. 16, 25–36. doi:10.1038/s41565-020-00822-y
Guo, L., Wang, T., Chen, Z., He, N., Chen, Y., and Yuan, T. (2018). Light scattering based analyses of the effects of bovine serum proteins on interactions of magnetite spherical particles with cells. Chin. Chem. Lett. 29, 1291–1295. doi:10.1016/j.cclet.2017.11.017
Guo, W., Zhang, C., Ma, T., Liu, X., Chen, Z., Li, S., et al. (2021). Advances in aptamer screening and aptasensors' detection of heavy metal ions. J. Nanobiotechnology 19, 166. doi:10.1186/s12951-021-00914-4
Hasanzadeh, A., Alamdaran, M., Ahmadi, S., Nourizadeh, H., Bagherzadeh, M. A., Mofazzal Jahromi, M. A., et al. (2021). Nanotechnology against Covid-19: Immunization, diagnostic and therapeutic studies. J. Control. Release 336, 354–374. doi:10.1016/j.jconrel.2021.06.036
He, L., Huang, R., Xiao, P., Liu, Y., Jin, L., Liu, H., et al. (2021). Current signal amplification strategies in aptamer-based electrochemical biosensor: A review. Chin. Chem. Lett. 32, 1593–1602. doi:10.1016/j.cclet.2020.12.054
He, L., Yang, H., Xiao, P., Singh, R., He, N., Liu, B., et al. (2017). Highly selective, sensitive and rapid detection of Escherichia Coli O157:H7 using duplex pcr and magnetic nanoparticle-based chemiluminescence assay. J. Biomed. Nanotechnol. 13, 1243–1252. doi:10.1166/jbn.2017.2422
He, Z., Tang, C., Chen, X., Liu, H., Yang, G., Xiao, Z., et al. (2019). Based on magnetic beads to develop the kit for extraction of high-quality cell-free DNA from blood of breast cancer patients. Mat. express 9, 956–961. doi:10.1166/mex.2019.1579
Henry, S. J. W., and Stephanopoulos, N. (2021). Functionalizing DNA nanostructures for therapeutic applications, 13. Wiley Interdiscip Rev Nanomed Nanobiotechnol, e1729. doi:10.1002/wnan.1729
Jani, I. V., and Peter, T. F. (2022). Nucleic acid point-of-care testing to improve diagnostic preparedness. Clin. Infect. Dis. 75, 723–728. doi:10.1093/cid/ciac013
Jiang, H., Zeng, X., Xi, Z., Liu, M., Li, C., Li, Z., et al. (2013). Improvement on controllable fabrication of streptavidin-modified three-layer core-shell fe3o4@sio2@au magnetic nanocomposites with low fluorescence background. J. Biomed. Nanotechnol. 9, 674–684. doi:10.1166/jbn.2013.1575
Jiang, P., Wang, Y., Zhao, L., Ji, C., Chen, D., and Nie, L. (2018). Applications of gold nanoparticles in non-optical biosensors. Nanomater. (Basel) 8, 977. doi:10.3390/nano8120977
Jobdeedamrong, A., Theerasilp, M., Nasongkla, N., and Crespy, D. (2021). Nanocapsules with excellent biocompatibility and stability in protein solutions. Biomater. Sci. 9, 5781–5784. doi:10.1039/d1bm00510c
Kang, J., Li, Y., Zhao, Y., Wang, Y., Ma, C., and Shi, C. (2021). Nucleic acid extraction without electrical equipment via magnetic nanoparticles in pasteur pipettes for pathogen detection. Anal. Biochem. 635, 114445. doi:10.1016/j.ab.2021.114445
Khan, M. I., Nur, S. M., Adhami, V., and Mukhtar, H. (2021). Epigenetic regulation of rna sensors: Sentinels of immune response. Semin. Cancer Biol. 83, 413–421. doi:10.1016/j.semcancer.2020.12.028
Kim, H., Huh, H. J., Park, E., Chung, D. R., and Kang, M. (2021). Multiplex molecular point-of-care test for syndromic infectious diseases. BioChip J. 15, 14–22. doi:10.1007/s13206-021-00004-5
Kim, S. Y, S. Y., Lee, J. C., Seo, G., Woo, J. H., Lee, M., Nam, J., et al. (2021). Computational method-based optimization of carbon nanotube thin-film immunosensor for rapid detection of sars-cov-2 virus. Small Sci. 2, 2100111. doi:10.1002/smsc.202100111
Krasowska, A., and Sigler, K. (2014). How microorganisms use hydrophobicity and what does this mean for human needs? Front. Cell. Infect. Microbiol. 4, 112. doi:10.3389/fcimb.2014.00112
Lai, Y., Deng, Y., Yang, G., Li, S., Zhang, C., and Liu, X. (2018). Molecular imprinting polymers electrochemical sensor based on aunps/pth modified gce for highly sensitive detection of carcinomaembryonic antigen. J. Biomed. Nanotechnol. 14, 1688–1694. doi:10.1166/jbn.2018.2617
Land, K. J., Boeras, D. I., Chen, X. S., Ramsay, A. R., and Peeling, R. W. (2019). Reassured diagnostics to inform disease control strategies, strengthen health systems and improve patient outcomes. Nat. Microbiol. 4, 46–54. doi:10.1038/s41564-018-0295-3
Lee, E. Y., Kim, Y., Koo, B., Noh, G. S., Lee, H., and Shin, Y. (2020). A novel nucleic acid amplification system based on nano-gap embedded active disk resonators. Sensors Actuators B Chem. 320, 128351. doi:10.1016/j.snb.2020.128351
Lee, S. Y., Chen, F., and Lee, T. Y. (2021). Tryptamine-functionalized magnetic nanoparticles for highly sensitive detection of salmonella typhimurium. Analyst 146, 2559–2566. doi:10.1039/d0an02458a
Li, C., Xue, C., Wang, J., Luo, M., Shen, Z., and Wu, Z. S. (2019). Oriented tetrahedron-mediated protection of catalytic DNA molecular-scale detector against in vivo degradation for intracellular mirna detection. Anal. Chem. 91, 11529–11536. doi:10.1021/acs.analchem.9b00860
Li, Q., Xia, Y., Wan, X., Yang, S., Cai, Z., Ye, Y., et al. (2020). Morphology-dependent mno2/nitrogen-doped graphene nanocomposites for simultaneous detection of trace dopamine and uric acid. Mater. Sci. Eng. C 109, 110615. doi:10.1016/j.msec.2019.110615
Li, T., Xu, X., Zhang, G., Lin, R., Chen, Y., Li, C., et al. (2016). Nonamplification sandwich assay platform for sensitive nucleic acid detection based on aunps enumeration with the dark-field microscope. Anal. Chem. 88, 4188–4191. doi:10.1021/acs.analchem.6b00535
Li, T., Yi, H., Liu, Y., Wang, Z., Liu, S., He, N., et al. (2018). One-step synthesis of DNA templated water-soluble au-ag bimetallic nanoclusters for ratiometric fluorescence detection of DNA. J. Biomed. Nanotechnol. 14, 150–160. doi:10.1166/jbn.2018.2491
Li, Z., and Guo, Z. (2019). Bioinspired surfaces with wettability for antifouling application. Nanoscale 11, 22636–22663. doi:10.1039/c9nr05870b
Li, G., Qi, X., Wu, J., Xu, L., Wan, X., Liu, Y., et al. (2022a). Ultrasensitive, label-free voltammetric determination of norfloxacin based on molecularly imprinted polymers and au nanoparticle-functionalized black phosphorus nanosheet nanocomposite. J. Hazard. Mat. 436, 129107. doi:10.1016/j.jhazmat.2022.129107
Li, G., Qi, X., Zhang, G., Wang, S., Li, K., Wu, J., et al. (2022b). Low-cost voltammetric sensors for robust determination of toxic cd(ii) and pb(ii) in environment and food based on shuttle-like α-fe2o3 nanoparticles decorated β-bi2o3 microspheres. Microchem. J. 179, 107515. doi:10.1016/j.microc.2022.107515
Li, J., Lai, Y., Li, M., Chen, X., Zhou, M., Wang, W., et al. (2022a). Repair of infected bone defect with clindamycin-tetrahedral DNA nanostructure complex-loaded 3d bioprinted hybrid scaffold. Chem. Eng. J. 435, 134855. doi:10.1016/j.cej.2022.134855
Li, J., Wu, X., Li, Y., Wang, X., Huang, H., Jian, D., et al. (2021). Amplification-free smartphone-based attomolar hbv detection. Biosens. Bioelectron. X. 194, 113622. doi:10.1016/j.bios.2021.113622
Li, J., Yao, Y., Wang, Y., Xu, J., Zhao, D., Liu, M., et al. (2022b). Modulation of the crosstalk between schwann cells and macrophages for nerve regeneration: A therapeutic strategy based on a multifunctional tetrahedral framework nucleic acids system. Adv. Mat., 2202513. doi:10.1002/adma.202202513
Li, Q., Wu, J. T., Liu, Y., Qi, X. M., Jin, H. G., Yang, C., et al. (2021). Recent advances in black phosphorus-based electrochemical sensors: A review. Anal. Chim. Acta X. 1170, 338480. doi:10.1016/j.aca.2021.338480
Li, S., Liu, Y., Tian, T., Zhang, T., Lin, S., Zhou, M., et al. (2021). Bioswitchable delivery of microrna by framework nucleic acids: Application to bone regeneration. Small 17, e2104359. doi:10.1002/smll.202104359
Li, Y., Yang, H. P., Chen, S., Wu, X. J., and Long, Y. F. (2021). Simple preparation of carbon dots and application in cephalosporin detection. J. Nanosci. Nanotechnol. 21, 6024–6034. doi:10.1166/jnn.2021.19520
Liu, J., Chen, J., Wu, D., Huang, M., Chen, J., Pan, R., et al. (2021). Crispr-/cas12a-mediated liposome-amplified strategy for the surface-enhanced Raman scattering and naked-eye detection of nucleic acid and application to food authenticity screening. Anal. Chem. 93, 10167–10174. doi:10.1021/acs.analchem.1c01163
Liu, M., Yu, X., Chen, Z., Yang, T., Yang, D., Liu, Q., et al. (2017). Aptamer selection and applications for breast cancer diagnostics and therapy. J. Nanobiotechnology 15, 81. doi:10.1186/s12951-017-0311-4
Liu, Y., Deng, Y., Li, T., Chen, Z., Chen, H., Li, S., et al. (2018). Aptamer-based electrochemical biosensor for mercury ions detection using aunps-modified glass carbon electrode. J. Biomed. Nanotechnol. 14, 2156–2161. doi:10.1166/jbn.2018.2655
Liu, Y., Sun, Y., Li, S., Liu, M., Qin, X., Chen, X., et al. (2020). Tetrahedral framework nucleic acids deliver antimicrobial peptides with improved effects and less susceptibility to bacterial degradation. Nano Lett. 20, 3602–3610. doi:10.1021/acs.nanolett.0c00529
Liu, S., He, X., Zhang, T., Zhao, K., Xiao, C., Tong, Z., et al. (2022). Highly sensitive smartphone-based detection of listeria monocytogenes using syto9. Chin. Chem. Lett. 33, 1933–1935. doi:10.1016/j.cclet.2021.11.051
Liu, Y., Li, T., Yang, G., Deng, Y., Mou, X., and He, N. (2022). A simple aunps-based colorimetric aptasensor for chlorpyrifos detection. Chin. Chem. Lett. 33, 1913–1916. doi:10.1016/j.cclet.2021.11.025
Ma, T., Huang, H., Guo, W., Zhang, C., Chen, Z., Li, S., et al. (2020). Recent progress in black phosphorus sensors. J. Biomed. Nanotechnol. 16, 1045–1064. doi:10.1166/jbn.2020.2963
Magesa, F., Wu, Y., Dong, S., Tian, Y., Li, G., Vianney, J. M., et al. (2020). Electrochemical sensing fabricated with TA2O5 nanoparticle-electrochemically reduced graphene oxide nanocomposite for the detection of oxytetracycline. Biomolecules 10, 110. doi:10.3390/biom10010110
Mei, X., Wang, D., Zhang, L., Li, J., and Dong, C. (2021). Synthesis of carbon dots for al(3+) sensing in water by fluorescence assay. Luminescence 36, 1469–1475. doi:10.1002/bio.4088
Mo, X., Wang, X., Zhu, Z., Yu, Y., Chang, D., Zhang, X., et al. (2021). Quality management for point-of-care testing of pathogen nucleic acids: Chinese expert consensus. Front. Cell. Infect. Microbiol. 11, 755508. doi:10.3389/fcimb.2021.755508
Mondal, S., Feirer, N., Brockman, M., Preston, M. A., Teter, S. J., Ma, D., et al. (2021). A direct capture method for purification and detection of viral nucleic acid enables epidemiological surveillance of sars-cov-2. Sci. Total Environ. 795, 148834. doi:10.1016/j.scitotenv.2021.148834
Mou, X. S., Chen, Z., Liu, M., Liu, Y., Deng, Yan, et al. (2019). In-situ mutation detection by magnetic beads-probe based on single base extension and its application in genotyping of Hepatitis B virus pre-c region 1896nt locus single nucleotide polymorphisms. J. Biomed. Nanotechnol. 15, 2393–2400. doi:10.1166/jbn.2019.2862
Nie, L., Liu, F., Ma, P., and Xiao, X. (2014). Applications of gold nanoparticles in optical biosensors. J. Biomed. Nanotechnol. 10, 2700–2721. doi:10.1166/jbn.2014.1987
Ota, R., Takagi, N., Imaizumi, Y., Waku, T., and Kobori, A. (2021). Sandwich-type detection of nucleic acids by bioorthogonal sers probes. Nucleosides Nucleotides Nucleic Acids 40, 166–177. doi:10.1080/15257770.2020.1849718
Pang, R., Zhu, Q., Wei, J., Meng, X., and Wang, Z. (2022). Enhancement of the detection performance of paper-based analytical devices by nanomaterials. Molecules 27, 508. doi:10.3390/molecules27020508
Park, M. K., Kee, J. S., Quah, J. Y., Netto, V., Song, J., Fang, Q., et al. (2013). Label-free aptamer sensor based on silicon microring resonators. Sensors Actuators B Chem. 176, 552–559. doi:10.1016/j.snb.2012.08.078
Pearlman, S. I., Leelawong, M., Richardson, K. A., Adams, N. M., Russ, P. K., Pask, M. E., et al. (2020). Low-resource nucleic acid extraction method enabled by high-gradient magnetic separation. ACS Appl. Mat. Interfaces 12, 12457–12467. doi:10.1021/acsami.9b21564
Poncelet, L., Malic, L., Clime, L., Geissler, M., Morton, K. J., Nassif, C., et al. (2021). Multifunctional magnetic nanoparticle cloud assemblies for in situ capture of bacteria and isolation of microbial DNA. Analyst 146, 7491–7502. doi:10.1039/d1an01297e
Prattis, I., Hui, E., Gubeljak, P., Kaminski Schierle, G. S., Lombardo, A., and Occhipinti, L. G. (2021). Graphene for biosensing applications in point-of-care testing. Trends Biotechnol. 39, 1065–1077. doi:10.1016/j.tibtech.2021.01.005
Pujari, A. (2021). Smartphone ophthalmoscopy: Is there a place for it? Clin. Ophthalmol. 15, 4333–4337. doi:10.2147/OPTH.S243103
Pujari, A., Saluja, G., Agarwal, D., Sinha, A., Kumar, A., and Sharma, N. (2021). Clinical role of smartphone fundus imaging in diabetic retinopathy and other neuro-retinal diseases. Curr. Eye Res. 46, 1605–1613. doi:10.1080/02713683.2021.1958347
Qin, X., Xiao, L., Li, N., Hou, C., Li, W., Li, J., et al. (2022). Tetrahedral framework nucleic acids-based delivery of microrna-155 inhibits choroidal neovascularization by regulating the polarization of macrophages. Bioact. Mat. 14, 134–144. doi:10.1016/j.bioactmat.2021.11.031
Rahimnejad, M., Nasrollahi Boroujeni, N., Jahangiri, S., Rabiee, N., Rabiee, M., Makvandi, P., et al. (2021). Prevascularized micro-/nano-sized spheroid/bead aggregates for vascular tissue engineering. Nanomicro. Lett. 13, 182. doi:10.1007/s40820-021-00697-1
Sarfraz, N., and Khan, I. (2021). Plasmonic gold nanoparticles (aunps): Properties, synthesis and their advanced energy, environmental and biomedical applications. Chem. Asian J. 16, 720–742. doi:10.1002/asia.202001202
Suan Ng, S., Ling Lee, H., Bothi Raja, P., and Doong, R. A. (2022). Recent advances in nanomaterial-based optical biosensors as potential point-of-care testing (poct) probes in carcinoembryonic antigen detection. Chem. Asian J. 17, e202200287. doi:10.1002/asia.202200287
Sun, H., Jiao, R., An, G., Xu, H., and Wang, D. (2021). Influence of particle size on the aggregation behavior of nanoparticles: Role of structural hydration layer. J. Environ. Sci. 103, 33–42. doi:10.1016/j.jes.2020.10.007
Sun, X. (2022). Glucose detection through surface-enhanced Raman spectroscopy: A review. Anal. Chim. Acta X. 1206, 339226. doi:10.1016/j.aca.2021.339226
Tang, C., He, Z., Liu, H., Xu, Y., Huang, H., Yang, G., et al. (2020). Application of magnetic nanoparticles in nucleic acid detection. J. Nanobiotechnology 18, 62. doi:10.1186/s12951-020-00613-6
Tang, Y., Ali, Z., Dai, J., Liu, X., Wu, Y., Chen, Z., et al. (2018). Single-nucleotide polymorphism genotyping of exos in pseudomonas aeruginosa using dual-color fluorescence hybridization and magnetic separation. J. Biomed. Nanotechnol. 14, 206–214. doi:10.1166/jbn.2018.2525
Tang, Y., Li, Z., He, N., Zhang, L., Ma, C., Li, X., et al. (2013). Preparation of functional magnetic nanoparticles mediated with peg-4000 and application in pseudomonas aeruginosa rapid detection. J. Biomed. Nanotechnol. 9, 312–317. doi:10.1166/jbn.2013.1493
Tian, B., Gao, F., Fock, J., Dufva, M., and Hansen, M. F. (2020). Homogeneous circle-to-circle amplification for real-time optomagnetic detection of sars-cov-2 rdrp coding sequence. Biosens. Bioelectron. X. 165, 112356. doi:10.1016/j.bios.2020.112356
Wang, S., Shanthan, G., Bouzga, M. M., Thi Dinh, H. M., Haas, C., and Fonnelop, A. E. (2021). Evaluating the performance of five up-to-date DNA/rna co-extraction methods for forensic application. Forensic Sci. Int. 328, 110996. doi:10.1016/j.forsciint.2021.110996
Wang, Z., Yu, W., Xie, R., Yang, S., and Chen, A. (2021). A strip of lateral flow gene assay using gold nanoparticles for point-of-care diagnosis of african swine fever virus in limited environment. Anal. Bioanal. Chem. 413, 4665–4672. doi:10.1007/s00216-021-03408-2
White, T. J., Rye, M. S., and Tay, J. W. (2022). Developmental validation of an efficient differential separation method incorporating the i‐sep ® DL spin column with high sperm DNA recovery for the processing of sexual assault samples. J. Forensic Sci. 67, 1679–1689. doi:10.1111/1556-4029.15043
Wiraja, C., Yeo, D. C., Lio, D. C. S., Zheng, M., and Xu, C. (2019). Functional imaging with nucleic-acid-based sensors: Technology, application and future healthcare prospects. Chembiochem 20, 437–450. doi:10.1002/cbic.201800430
Wu, Y., Deng, P., Tian, Y., Ding, Z., Li, G., Liu, J., et al. (2020a). Rapid recognition and determination of tryptophan by carbon nanotubes and molecularly imprinted polymer-modified glassy carbon electrode. Bioelectrochemistry 131, 107393. doi:10.1016/j.bioelechem.2019.107393
Wu, Y., Deng, P., Tian, Y., Feng, J., Xiao, J., Li, J., et al. (2020b). Simultaneous and sensitive determination of ascorbic acid, dopamine and uric acid via an electrochemical sensor based on pvp-graphene composite. J. Nanobiotechnology 18, 112. doi:10.1186/s12951-020-00672-9
Xiao, C., Guo, Y., Zhao, K., Liu, S., He, N., He, Y., et al. (2022). Prognostic value of machine learning in patients with acute myocardial infarction. J. Cardiovasc. Dev. Dis. 9, 56. doi:10.3390/jcdd9020056
Xiao, M. F, M. F., Zeng, C., Li, S. H., and Yuan, F. L. (2022). Applications of nanomaterials in Covid-19 pandemic. Rare Met. 41, 1–13. doi:10.1007/s12598-021-01789-y
Xie, H., Di, K., Huang, R., Khan, A., Xia, Y., Xu, H., et al. (2020). Extracellular vesicles based electrochemical biosensors for detection of cancer cells: A review. Chin. Chem. Lett. 31, 1737–1745. doi:10.1016/j.cclet.2020.02.049
Xu, Y., Wang, T., Chen, Z., Jin, L., Wu, Z., Yan, J., et al. (2021). The point-of-care-testing of nucleic acids by chip, cartridge and paper sensors. Chin. Chem. Lett. 32, 3675–3686. doi:10.1016/j.cclet.2021.06.025
Yan, T., Zhang, G., Chai, H., Qu, L., and Zhang, X. (2021). Flexible biosensors based on colorimetry, fluorescence, and electrochemistry for point-of-care testing. Front. Bioeng. Biotechnol. 9, 753692. doi:10.3389/fbioe.2021.753692
Yang, G., Lai, Y., Xiao, Z., Tang, C., and Deng, Y. (2018). Ultrasensitive electrochemical immunosensor of carcinoembryonic antigen based on gold-label silver-stain signal amplification. Chin. Chem. Lett. 29, 1857–1860. doi:10.1016/j.cclet.2018.11.030
Yang, H., Liu, M., Jiang, H., Zeng, Y., Jin, L., Luan, T., et al. (2017). Copy number variation analysis based on gold magnetic nanoparticles and fluorescence multiplex ligation-dependent probe amplification. J. Biomed. Nanotechnol. 13, 655–664. doi:10.1166/jbn.2017.2386
Yang, L., Zhang, S., Liu, X., Tang, Y., Zhou, Y., and Wong, D. K. Y. (2020). Detection signal amplification strategies at nanomaterial-based photoelectrochemical biosensors. J. Mat. Chem. B 8, 7880–7893. doi:10.1039/d0tb01191f
Yang, X., Wang, L., Pang, L., Fu, S., Qin, X., Chen, Q., et al. (2021). A novel fluorescent platform of DNA-stabilized silver nanoclusters based on exonuclease iii amplification-assisted detection of salmonella typhimurium. Anal. Chim. Acta X. 1181, 338903. doi:10.1016/j.aca.2021.338903
Ye, H. L., Shang, Y., Wang, H. Y., Ma, Y. L., He, X. W., Li, W. Y., et al. (2021). Determination of fe ion and cellular bioimaging based on a novel photoluminescent silicon nanoparticles. Talanta 230, 122294. doi:10.1016/j.talanta.2021.122294
Yi, C., Luo, Z., Lu, Y., Belwal, T., Pan, X., and Lin, X. (2021). Nanoporous hydrogel for direct digital nucleic acid amplification in untreated complex matrices for single bacteria counting. Biosens. Bioelectron. X. 184, 113199. doi:10.1016/j.bios.2021.113199
Zeng, Q., Qi, X., Zhang, M., Tong, X., Jiang, N., Pan, W., et al. (2020). Efficient decontamination of heavy metals from aqueous solution using pullulan/polydopamine hydrogels. Int. J. Biol. Macromol. 145, 1049–1058. doi:10.1016/j.ijbiomac.2019.09.197
Zhang, T., Tian, T., Zhou, R., Li, S., Ma, W., Zhang, Y., et al. (2020). Design, fabrication and applications of tetrahedral DNA nanostructure-based multifunctional complexes in drug delivery and biomedical treatment. Nat. Protoc. 15, 2728–2757. doi:10.1038/s41596-020-0355-z
Zhang, C., Belwal, T., Luo, Z., Su, B., and Lin, X. (2022). Application of nanomaterials in isothermal nucleic acid amplification. Small 18, e2102711. doi:10.1002/smll.202102711
Zhang, M., Cui, X., and Li, N. (2022). Smartphone-based mobile biosensors for the point-of-care testing of human metabolites. Mat. Today Bio 14, 100254. doi:10.1016/j.mtbio.2022.100254
Zhang, M., Shao, S., Yue, H., Wang, X., Zhang, W., Chen, F., et al. (2021). High stability au nps: From design to application in nanomedicine. Int. J. Nanomedicine 16, 6067–6094. doi:10.2147/IJN.S322900
Zhang, N., Li, J., Liu, B., Zhang, D., Zhang, C., Guo, Y., et al. (2022). Signal enhancing strategies in aptasensors for the detection of small molecular contaminants by nanomaterials and nucleic acid amplification. Talanta 236, 122866. doi:10.1016/j.talanta.2021.122866
Zhang, Q., Lin, S., Wang, L., Peng, S., Tian, T., Li, S., et al. (2021). Tetrahedral framework nucleic acids act as antioxidants in acute kidney injury treatment. Chem. Eng. J. 413, 127426. doi:10.1016/j.cej.2020.127426
Zhang, W., He, Y., Feng, Z., and Zhang, J. (2022). Recent advances of functional nucleic acid-based sensors for point-of-care detection of sars-cov-2. Microchim. Acta 189, 128. doi:10.1007/s00604-022-05242-4
Zhang, Z., Ma, P., Ahmed, R., Wang, J., Akin, D., Soto, F., et al. (2022). Advanced point-of-care testing technologies for human acute respiratory virus detection. Adv. Mat. 34, e2103646. doi:10.1002/adma.202103646
Keywords: nanomaterial, nucleic acid detection, point-of-care testing devices, sensor, nucleic acid enrichment
Citation: He Z, Liu C, Li Z, Chu Z, Chen X, Chen X and Guo Y (2022) Advances in the use of nanomaterials for nucleic acid detection in point-of-care testing devices: A review. Front. Bioeng. Biotechnol. 10:1020444. doi: 10.3389/fbioe.2022.1020444
Received: 16 August 2022; Accepted: 23 September 2022;
Published: 13 October 2022.
Edited by:
Guangli Li, Hunan University of Technology, ChinaReviewed by:
Xianbo Mou, Ningbo University, ChinaXinjiang Cao, Anyang Institute of Technology, China
Qi You, Nanjing University, China
Copyright © 2022 He, Liu, Li, Chu, Chen, Chen and Guo. This is an open-access article distributed under the terms of the Creative Commons Attribution License (CC BY). The use, distribution or reproduction in other forums is permitted, provided the original author(s) and the copyright owner(s) are credited and that the original publication in this journal is cited, in accordance with accepted academic practice. No use, distribution or reproduction is permitted which does not comply with these terms.
*Correspondence: Yuan Guo, Z3VveXVhbjA4MTVAMTYzLmNvbQ==