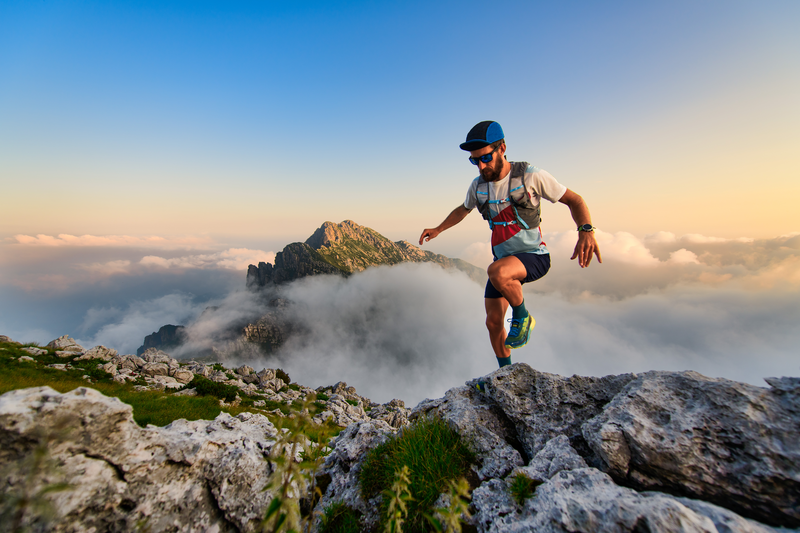
94% of researchers rate our articles as excellent or good
Learn more about the work of our research integrity team to safeguard the quality of each article we publish.
Find out more
REVIEW article
Front. Bioeng. Biotechnol. , 09 December 2022
Sec. Biosensors and Biomolecular Electronics
Volume 10 - 2022 | https://doi.org/10.3389/fbioe.2022.1013354
This article is part of the Research Topic Nanomaterials for Biosensing and Bioassays View all 5 articles
Schistosomiasis is one of the neglected tropical diseases that affect millions of people worldwide. Globally, it affects economically poor countries, typically due to a lack of proper sanitation systems, and poor hygiene conditions. Currently, no vaccine is available against schistosomiasis, and the preferred treatment is chemotherapy with the use of praziquantel. It is a common anti-schistosomal drug used against all known species of Schistosoma. To date, current treatment primarily the drug praziquantel has not been effective in treating Schistosoma species in their early stages. The drug of choice offers low bioavailability, water solubility, and fast metabolism. Globally drug resistance has been documented due to overuse of praziquantel, Parasite mutations, poor treatment compliance, co-infection with other strains of parasites, and overall parasitic load. The existing diagnostic methods have very little acceptability and are not readily applied for quick diagnosis. This review aims to summarize the use of nanotechnology in the treatment, diagnosis, and prevention. It also explored safe and effective substitute approaches against parasitosis. At this stage, various nanomaterials are being used in drug delivery systems, diagnostic kits, and vaccine production. Nanotechnology is one of the modern and innovative methods to treat and diagnose several human diseases, particularly those caused by parasite infections. Herein we highlight the current advancement and application of nanotechnological approaches regarding the treatment, diagnosis, and prevention of schistosomiasis.
Schistosomiasis is a serious tropical disease caused by parasitic flatworms of the genus Schistosoma, often recognized as blood flukes (Ullah et al., 2020a). Schistosomiasis is the second most prevalent parasitic disease after malaria, owing to its catastrophic impact on public health, which in turn impacts the global economy. Infection with different Schistosoma species may cause more intricate situations such as urinary bladder, colorectal, and liver malignancies (Li et al., 2019). S. haematobium infection may develop infertility in males. Testicular infarction can develop from ova occluding the spermatic venous plexus and subsequent granuloma formation (Adisa et al., 2012). As per the world health organization (WHO) data, 236.6 million individuals (WHO, 2022) in 78 countries (Ullah et al., 2022a; Ullah et al., 2022b), required schistosomiasis preventive therapy (Qadeer et al., 2021), including forty million females in the procreative phase (Cioli et al., 2014). The yearly death toll from schistosomiasis in developing nations is estimated to be over 0.3 million (Colley et al., 2014).
S. mansoni, S. haematobium, S. Japonicum, S. intercalatum, and S. mekongi are the five clinically important schistosomal species (Siqueira et al., 2017). The intermediate host freshwater snail infected with miracidia is needed for infection with these worms. The miracidia develop into cercaria and the cercaria penetrates the host skin after water exposure (Adekiya et al., 2020). The intermediate form produces in the aquatic gastropod snail. Generally, the intermediate host for S. japonicum is Oncomelania, Biomphalaria for S. mansoni, and Bulinus for S. haematobium. The life cycle of schistosomiasis is initiated by the exit of cercariae from the snail into the water and the penetration of the skin of humans and animals when they swim or interact with contaminated water (Gryseels et al., 2006). The adult parasites inhabit many sites of the mesenteric veins of the vertebrate host, and it is seen to be definite for every species. They are found in the venous plexus of the bladder and, on rare occasions, in the rectal venules in S. haematobium infection. While, adults of S. japonicum and S. mansoni are found in the superior mesenteric vein draining to the small intestine and superior mesenteric vein draining to the large intestine, respectively (Tomiotto-Pellissier et al., 2017).
Disease spread is facilitated by poor sanitary conditions as well as low economic development (Rollinson et al., 2013). The World Health Assembly passed a resolution in 2012, in Geneva, Switzerland, urging increased investment in schistosomiasis control and supporting the launch of elimination programs in endemic countries (Americas, Asia, and Africa). Schistosomiasis control and treatment are restricted to less accessible chemotherapeutic agents that show effectiveness, care, and thrmissibility (Tomiotto-Pellissier et al., 2017). Schistosomiasis is currently controlled through snail host elimination, health education, hygiene preference, clean water availability, and improved sanitation (Ferrari et al., 2003). Precise diagnosis and extensive drug administration are critical for disease management (Ullah et al., 2020b).
Praziquantel (PZQ) is the preferred treatment for schistosomiasis; it was developed in the late 1970s and is known to be an active anti-schistosomal medicine. Though, PZQ cannot treat immature worms nor avert reinfection (Dkhil et al., 2015a). Drug resistance has been observed across the world as a result of parasite mutation, inadequate tretreatment ofseases, overuse of PZQ, and mixed infection (Adekiya et al., 2020). PZQ usage is generally restricted due to its poor bioavailability and water solubility (Lindenberg et al., 2004). The metabolism leads to the formation of a less effective molecule, which must thus be given at larger doses (Mourao et al., 2005). Such factors motivate scientists, and thysician to look for technological alternatives for a safe and efficient oral absorption of PZQ, which may be utilized in disease prevention and treatment (Yang M. et al., 2009b; Ribeiro de Souza et al., 2012).
In the twenty-first century, nanotechnology emerged as one of the progressive and developing technologies. Norio Taniguchi coined the term “nanotechnology” in 1974. Nanotechnology (from the Latin nanus, which means the “dwarf”). is defined as the design, manufacture, and application of materials and technologies with he least functional content on a nanoscale (1–100 nm), or one billionth of a meter (10−9) (Moniruzzaman and Min, 2020). The progress of a broad variety of nanoscale technologies is the start of changing the basis of diagnosis, healthcare, and disease prevention. These technological advances are known as nanomedicines (Moghimi et al., 2005).
Nanomedicine is the application of nanotechnology to treat, monitor, and prevent diseases. For the effective use of nanomedicine, identification of the exact targets (cell and/or receptors) and precised delivery system are necessary (Abaza, 2016). Nanomedicines can play a significant role in drug delivery and disease by ensuring that a sufficient amount of the drug enters the body, that the drug does enter the body should stay for an appropriate amount of time, and that it is target-specific to the areas that require treatment (Agrawal, 2016). Nanotechnology has been used worldwide in various fields, and certain metals or metal oxides nanoparticles (NPs) are now broadly applied as drugs to cure several diseases (Elechiguerra et al., 2005; Jebali and Kazemi, 2013). Nanotechnology has the ability to restore the use of hazardous medications by the use of multifaceted structures that permit drugs to be carried to the pathogen, protecting host cells and using its impact with less toxicity (Forrest and Kwon, 2008). Previously, nanotechnology was employed as a drug delivery system (DDS) in several parasitic diseases such as malaria, toxoplasmosis, trypanosomiasis, and leishmaniasis (Date et al., 2007).
Nanotechnology and the use of nano-enabled drug delivery systems are believed to be effective in schistosomiasis treatment if combined with PZQ (Veerasamy et al., 2011). By offering more intensive drug delivery, nano-enabled drug delivery systems can improve the bioavailability, therapeutic effectiveness, and adverse effect profile of PZQ (or other medicines), (Adekiya et al., 2020). The objective of this appraisal is to emphasize and address the present state of research employing various nanoparticles as drug delivery systems that permit for drug vectorization, treatment, diagnosis, and control of schistosomiasis.
In 1984, chemotherapy was suggested as the best way of treating and removing schistosomiasis by the WHO experts committee. Schistosomiasis control and treatment depend upon the single-choice treatment using PZQ. PZQ is widely used among other antischistosomal products. It is effective against all Schistosoma species responsible for schistosomiasis. It reduces parasitic load and can lower the intensity of symptom. Because of its easy administration, efficiency, and affordability, it is also the most preferred medication. The mechanism of action for the treatment of schistosomiasis with PZQ is not well understood, however, variations in the musculature of the worm is a suggested mechanism (Adekiya et al., 2020). Younger forms are exposed to lower levels of unchanged PZQ in the bloodstream than older forms found in the liver. PZQ is broadly changed into an inactive or significantly less effective compound after oral administration (Xiao et al., 1985; Mourao et al., 2005). In the absence of alternative schistosomicides, it is critical to implant strategies to prevent, or at least delay, the evolution of drug resistance, as well as to seek remidies to overcome some of PZQ shortcomings, such as its lack of activity on immature worms (Doenhoff et al., 2008). Due to these circumstances, scientist and clinicians were compelled to seek a safer and more effective method of treating oral absorption (Yang et al., 2009a; de Almeida et al., 2012).
Due to the shortcomings of the drugs scientists have developed drug delivery technologies (nanotechnology) to offer further focused therapies to all stages of the schistosome parasite, and the medicines may be more effective by targeting the juvenile parasites. This novel approach can also decrease drug resistance by clearing the host’s schistosome and preventing reinfection.
The adult schistosome tegument is a type of outer surface structure composed of a cytoplasmic syncytium linked to the basal cell bodies by a thin cytoplasmic linkage. Their bodies house the nuclei, ribosomes, endoplasmic reticulum, mitochondria, and Golgi apparatus, and their vesicular products, known as discoid bodies and multilaminate vesicles, are carried to the tegument syncytium via the cytoplasmic connections (Pérez-Sánchez et al., 2008). The apical surface of the tegument, like the glycocalyx of eukaryotic cells, is made of a normal plasma membrane underlain by a membrane-like secretion, which has been dubbed membranocalyx (Skelly and Alan Wilson, 2006; Pérez-Sánchez et al., 2008).
This tegument, which encompasses the entire exterior of the worm, acts as an important connection between both the parasite and its host and has been vitally elucidated in the multifaceted host - pathogen interactions, which encompasses nutrient supply, excretion, osmoregulation, sensory reception, signal transduction, and interaction with the host’s immature and hemostatic systems (Van Hellemond et al., 2006; Ramajo-Hernández et al., 2007). Proteins expressed at the surface membranes of newly formed schistosomula are thus believed to be excellent candidates for the growth of novel schistosomiasis vaccines and medicines (Sotillo et al., 2015). As a result, identifying and characterizing schistosome tegumental compounds is critical for comprehending the host-parasite interaction and creating novel immunologic, therapeutic, and diagnostic methods (Skelly and Alan Wilson, 2006).
On the surface of the tegument, several targets have been identified. These are required to target schistosome glucose transporter 1 (SGTP1), schistosome glucose transporter 4 (SGTP4), acetylcholinesterase (AChE), and a nicotinic type of acetylcholine receptor (nAChR) for designed drug-loaded nanoparticles, which are mostly found on the surface of male schistosomes. Dynein, aquaporins, and tetraspanins are some of the other surface proteins present on the targeted tegument. Such compounds discovered on the surface of the tegument can be used to generate novel medicinal drugs and Schistosoma parasite vaccines (Adekiya et al., 2020).
Several studies have found that nano-delivery systems can improve the therapeutic effects of various substances in disease treatment (Zadeh Mehrizi et al., 2018). A nano-enabled drug delivery system can improve or modify the pharmacokinetics of active pharmaceutical ingredients (API), as well as protect API from chemical, physical, and biological degradation. However, the nano sizes of such systems improve the efficiency of biological barrier bridging, tissue tolerance, and cellular absorption and transportation, enabling for adequate delivery of therapeutic drugs to target regions such as the liver, brain, and solid tumors (Date et al., 2007; Adekiya et al., 2020). The use of solid lipid nanoparticles in the treatment of schistosomiasis would also be valuable in terms of cost-effectiveness, as they are cost-effective, comparatively less toxic, stable, and easy to decompose (Cheng et al., 2017). Lipid-based preparations can increase drug availability by changing the solubility and pace at which drugs can be freed to promote and enhance drug absorption across biological barriers. This method will be useful and successful for treating all forms of schistosomes (Adekiya et al., 2020). It has been shown that NPs can activate higher cell defense, which can prevent disease recurrence and reinfection, by specifically targeting overexpressed schistosome antigens in the human host (Tousif et al., 2017).
In S. mansoni infected mice, SLN-PZQ at a dose rate of 500 mg/kg showed better bioavailability, absorption rate, and antischistosomal action in all treatment groups, with a higher degree of worm reduction in all tested doses. The effective dose 95 (ED95) of solid lipid nanoparticles-PZQ was 5.29 times lower than that of market praziquantel (M-PZQ), with considerably larger intestinal tissue egg burdens and virtually total elimination of immature deposited eggs in all studied groups (Radwan et al., 2019). Another research found the greatest substantial reductions in total worm count, egg/Gram liver tissue, and intestine were seen using liposome-encapsulated PZQ at a dose rate of 500 mg/kg (97.2%, 99.3%, and 99.5% respectively). Liposomal-PZQ (Lip.-PZQ) showed greater efficacy than that of free PZQ in every aspect, particularly when administered at 45 days Post infection (Labib El Gendy et al., 2019). Table 1 lists the various nano-delivery systems utilized to improve PZQ therapeutic effectiveness in the treatment of schistosomal infections.
TABLE 1. List of different Nano-delivery systems used to improve the treatment efficacy of drug against schistosomal infections.
Drugs can be administered via enteral or parental routes using NPs delivery systems (Tawfeek et al., 2019). NPs have potent antiparasitic effects by acting as a drug carrier for commonly used drugs, such as praziquantel in the treatment of schistosomiasis (Kolenyak-Santos et al., 2015). SLNs-loaded PZQ were found to enhance PZQ’s pharmacological and safety characteristics in vitro on S. mansoni cultures (Kolenyak-Santos et al., 2015). To treat schistosomiasis, malaria, viserial leishmaniasis (VL), and visceral larva migrans, metal NPs, chitosan (CS), and liposomes are conjugated with a range of medications, including praziquantel, chloroquine, amphotericin B, rifampicin, and albendazole. NPs have the ability to be employed as a vaccine candidate for toxoplasmosis and malaria, as an additive to boost immune response against schistosomiasis, VL, and Chagas disease, and as an additive to increase immunological response against schistosomiasis, Visceral leishmaniasis (VL), and Chagas disease (Abaza, 2016). Gold nanorods were combined with a recombinant S. mansoni tegument protein Sm29 to immunise mice against schistosomiasis (Assis et al., 2018). AuNPs are being used to alleviate oxidative stress in mice spleen tissue produced by S. mansoni infection (Dkhil et al., 2017). Other nanoparticles, such as liposome nanoparticles, were also used in vitro to transport epiisopiloturine, with promising results (Tawfeek et al., 2019), as well as poly (lactic-co-glycolic acid) (PLGA) nanoparticles loaded with lignan (-)-6,6′-dinitrohinokinin (DNHK) for schistosomiasis treatment (Tawfeek et al., 2019).
Nanoparticles are used in various types of products for different type’s activities. Nanoparticles uses in various fields in given in (Figure 1). Based on shape, size, and chemical characteristics, NPs are commonly classified into different categories. Some of the well-known NPs used in drug delivery, diagnosis, and vaccine are shown in (Figure 2) and each one is discussed below in detail.
FIGURE 2. Schematic diagram of various commonly used nanoparticles in schistosomiasis treatment, diagnosis, and prevention.
AuNPs have recently been actively used in several fields of nanomedicine for diagnostic and therapeutic purposes. Due to the promising properties of AuNPs, they can be used in near future for medical purposes in almost all fields (Dykman and Khlebtsov, 2011). The gold complexes have also shown possible antileishmanial and antimalarial action (Vieites et al., 2009). AuNPs also show anthelminthic efficacy as investigated in the invivo study (Kar et al., 2014). AuNPs were discovered to cause cestode paralysis and death, which the scientists ascribe to alterations in the parasite’s enzymatic activity (Tikariha et al., 2012). Consequently, the use of Au is significant for the treatment of tropical human diseases (de Almeida and Carabineiro, 2013). For example, when AuNPs are used in a polymerase chain reaction (PCR), it increases and improves the specificity of this diagnostic technique (Li and Ji, 2015). In schistosomiasis AuNPs inoculated intraperitoneally can reduce the total worm load, eggs in the liver, and granuloma size in S. mansoni infected mice. In addition, the treatment can decrease liver damage due to its anti-inflammatory and antioxidant properties (Dkhil et al., 2015a). Another study discovered that AuNps have a unique therapeutic potential against renal diseases caused by S. mansoni infection. AuNPs decreased oxidative stress and histological damage in the kidney. They also restored the expression of the damaged kidney genes (Dkhil M. A. et al., 2016b). Meanwhile, other researchers are employing AuNPs as a means of delivering nuclear-oriented drugs into biological cells (Gu et al., 2009).
AgNPs are amongst several metallic NPs which are commonly used in a range of biomedical applications owing to their fascinating antimicrobial and anticancer properties. In the field of nanoscience and nanotechnology, especially in nanomedicine AgNPs plays a pivotal part in the treatment of various disease (Zhang et al., 2016). The biological activity of AgNPs is determined by factors such as surface chemistry, mass, shape, atom morphology, coating/capping, agglomeration, dissolving rate, and atom reactivity in solution. The efficacy of ion discharge capacity, cell types, and the kind of reducing agent employed in the production of AgNPs are all important factors in determining cytotoxicity (Carlson et al., 2008). AgNPs’ exploitation has attracted considerable courtesy and effort in numerous fields. Though, the antibacterial actions of the new silver type are still largely at the center of the healthcare arena. AgNPs have been widely used in commercialized products and entered into clinical practice, taking benefit of the sterilizer and antibacterial properties of AgNPs (Lansdown, 2006; Cohen et al., 2007). Few studies have provided detail on AgNP’s (silver ions Ag+), showing anti-schistosomal activity (King and Highashi, 1992; Cheng et al., 2013). It was discovered that Ag+ was toxic to S. mansoni cercariae at concentrations more than 0.09 mM, but that Ag+ was non-toxic at lower levels yet hindered their penetration into linolenic acid-impregnated agar (King and Highashi, 1992). Another study evaluated the impact of AgNPs on schistosome cercariae and its potential use in schistosomiasis prevention, specifically in terms of pathogen transmission. Ag + may contribute largely to the effects of AgNPs on schistosome cercariae. Yet, the possible contribution of nano dimensions of AgNPs could not be ruled out, as remarkably unusual physicochemical properties and biological activities of various nano-sized materials including AgNPs have been demonstrated (Cheng et al., 2013). Owing to the traditional adverse properties of chemotherapy and radiation therapy, scientists and the industry have investigated the idea of utilizing AgNPs as a therapeutic and anti-cancer agent (Zhang et al., 2016).
SLNs are solid colloidal elements that are available in many sizes ranging from 30–1,000 nm (Ayan et al., 2017; Ganesan et al., 2018). SLN is made up of solid lipids (lipids at room and body temperatures) that have been stabilized by surfactants. The lipids could be purified triglycerides, complicated glyceride combinations, or even waxes (Wissing et al., 2004). SLNs are encapsulated or embedded in lipid nuclei to form a stable colloidal DDS, and the carriers are usually natural or synthetic solid lipid materials such as saturated fatty acid glycerides, stearic acid, beeswax, cholesterol, cetyl palmitate, monostearate mononitrate, and solid paraffin (Tapeinos et al., 2017; Mishra et al., 2018). High compression homogenization, a microemulsion method, film-ultrasonic diffusion, an emulsification-solvent evaporation method, and hot melt ultrasound technology may all be used to produce SLN (Sohail et al., 2020).
SLNs have extensive application in the delivery of Phyto-bioactive compounds for the treatment of various chronic infections. Because of their outstanding biocompatibility and biodegradability, SLNs have been demonstrated to be a viable alternative to drug delivery methods (Qi et al., 2012; Lasoń et al., 2013). The several benefits of SLNs delivery contain bypassing 120–200 nm particle size in the spleen or liver filtration, improved stability, leakage stoppage, and increase lymphatic endorsement of the bioactive compound through oral delivery (Ayan et al., 2017; Ganesan et al., 2018). SLNs, on the other hand, have drawbacks such as restricted drug-loading capacity and drug ejection due to crystallization during loading circumstances (Rajabi and Mousa, 2016). SLNs can be made from triglycerides, complex glycerides, and waxes (Mader, 2006). Because of their tiny size, SLNs may be used in several modes, including parenteral, pulmonary, and cutaneous applications, as well as an intravenous injection (Üner and Yener, 2007). SLNs have been widely studied for drug administration of dynamic anti-cancer complexes, improving medication oral bioavailability, protecting labile anti-cancer medicines, and reducing adverse effects by selectively targeting the active site (Pindiprolu et al., 2019).
SLN enhanced PZQ’s antischistosomal action, resulting in significantly prolonged drug release due to the encapsulated drug’s protection from enzymatic destruction. Despite the prolonged residency in the systemic circulation, oral administration of this SLN-PZQ demonstrated a substantial improvement in bioavailability and effectiveness with a safer profile when compared to traditional medication (Radwan et al., 2019). Another study found that PZQ-loaded SLN might be a new drug delivery method for schistosomiasis therapy, particularly in marginalized communities, improving therapeutic effectiveness while reducing PZQ side effects (de Souza et al., 2014). The use of hydrogenated castor oil in SLN increased PZQ bioavailability as well as drug residence duration after oral delivery (Xie et al., 2010). PZQ-loaded SLN was used to investigate the biological uses of this technology for PZQ intestinal penetration (de Souza et al., 2014).
LNCs feature a hybrid shape between polymeric nanocapsules and liposomes due to their oily core enclosed by a rigid capsule (Huynh et al., 2009). Miltefosine, a medication used to treat cutaneous breast cancer metastases and Visceral leishmaniasis, was shown to have improved availability and activity due to improved gastrointestinal transit and schistosomicidal activity when encapsulated in LNCs and taken orally, according to one study (Eissa et al., 2015). Due to the schistosomes’ better-absorbed tegument, which has an attraction for the phospholipid bilayer, the usage of LNCs has recently received significant attention, notably in the treatment of schistosomiasis. Because of their amphipathic character, LNCs can play a prominent part in modifying the solubility and rate at which drugs such as PZQ can be targeted, therefore increasing penetration across biological barriers (Cheng et al., 2017).
Moreover, a single dose of PZQ-LNCs substantially improve efficacy and decreased worm burden, hepatic pathology improvement, and substantial damage to the fluke suckers and tegument (Amara et al., 2018). Whereas PZQ-lipid Nano-capsule at a dose rate of 250 mg PZQ-20 mg miltefosine/kg demonstrates a substantial increase in antischistosomal efficacy in terms of statistical augmentation in mean worm load, particularly against invasive and early-stage worms, as well as improvement in hepatic granulomas (Eissa et al., 2020b), The prophylactic role of a single oral dose of PZQ-MFS LNCs was evaluated and demonstrate that when compared to an infected non-treated control, administration of the nano combination one or 7 days before infection resulted in a significantly substantial drop in mean worm burden and granuloma size, as well as improvement in hepatic pathology (Eissa et al., 2020a).
Likewise in one more investigation, the miltefosine encapsulation method was employed in LNCs remodeled with cetyltrimethylammonium bromide (CTAB), a +ve charge conveyance substance, or oleic acid with dose directed orally in S. mansoni infected mice. According to the findings of this investigation, a single oral dose of both encapsulated miltefosine resulted in a drop in parasite load as well as a reduction in liver granulomas in infected mice. Following the previous study findings, the investigators found that the role of encapsulated miltefosine is a feasible nanomedicine for mass schistosomiasis chemotherapy, particularly its application as an oral single dosage (El-Moslemany et al., 2016).
ZnO-NPs are employed in a variety of industrial products such as rubber, dye, coating, and cosmetics. ZnO-NPs is one of the most extensively utilized metal oxide NPs in biological applications due to their excellent biocompatibility, low toxicity, and low cost. ZnO-NPs have emerged as potentially useful in biomedicine, particularly in the anticancer and antibacterial domains, due to their ability to trigger the creation of extra reactive oxygen species (ROS), the release of zinc ions, and the induction of apoptosis (Jiang et al., 2018). Dietary ZnO-NPs improved oxidative status and improved the efficiency of several blood enzymes. As a result, ZnO-NPs were employed as a novel leishmania therapy (Ahmadi et al., 2014; Nadhman et al., 2014), and schistosomiasis (Bauomy, 2020). The photo-activated disinfectant effectiveness of the Ag@Sm-doped ZnO/CB nanocomposite for Staphylococcus aureus (80%), Pseudomonas. Aeruginosa (60%), and S. mansoni cercariae (100%) was associated with gradual degradation in the cercarial body. This nanocomposite is also effective for adult S. mansoni worms, leading to near-complete worm mortality and substantial worm body destruction. These findings validated Ag@Sm-doped ZnO/CB as a powerful biocide weapon capable of destroying harmful bacteria and parasites in both dark and light settings (Darwish et al., 2018). Another study revealed that administering ZnO NPs and/or L-carnitine to schistosome-infested mice decreased brain oxidative stress measures, with glutathione levels and catalase activity much greater than in the schistosome-infested group. On the contrary, the therapy dramatically reduced the levels of nitrite/nitrate, malondialdehyde, and reactive oxygen species. Furthermore, the treatment with ZnO nanoparticles and/or L-carnitine cured the neuro-schistosomiasis-related brain histological abnormalities (Bauomy, 2020).
Liposomes are small structures used as a drug delivery system to reach tissues, delivering only a portion of the drug to the target site (Tomiotto-Pellissier et al., 2017). Liposomes are amphiphilic, meaning they can transport both hydrophobic and hydrophilic medicines. It can protect the encapsulated medications by isolating them from the outer environment, increasing the solubility of the lipophilic drug, and extending the action period of the drugs (Muthu et al., 2011). Liposomes’ physiochemical characteristics may be changed, permitting the encapsulated drug to be delivered to the exact targets, resulting in greater therapeutic efficiency than traditional dose forms (Ammar et al., 1994). Because of their great biocompatibility and low toxicity, liposomes have sparked a lot of interest as a drug delivery system (Mufamadi et al., 2011).
In the treatment of S. mansoni, praziquantel (300 mg/kg) encapsulated in liposomes significantly reduced worm load, stool and intestinal egg count, and the number of hepatic granulomas (Frezza et al., 2013). In S. mansoni infected mice sulfated polysaccharide -D-glucan extracted from Ramalina celastri encapsulated in liposomes significantly reduced hepatic granuloma (Frezza et al., 2015). Thus, liposomes have the benefit of not restricting the drug’s physicochemical characteristics that are not enclosed, such as hydrophilicity or membrane, improving the therapeutic efficacy, regulating intake and tissue distribution, and producing less toxicological effects that encourage schistosomiasis treatment (Tomiotto-Pellissier et al., 2017).
MSNs are utilized as a nano-drug carrier because of their unique organization and remarkable features such as higher specific surface area (>900 m2/g−1) and lower volume (>1 cm3/g-1), ordered apertures, variable pore diameter (2–50 nm), excellent biocompatibility, and thermal stability (Li et al., 2017). These consistent surface characteristics and structures are ideal for encapsulating proteins, microbes, and other sorts of drugs. Furthermore, MSNs’ mesoporous characteristics allow them to carry a high amount of anticancer drugs and enable drug assortment via nano-sized components via passive targeting to the tumor location. As a result, MSNs are employed as an effective drug delivery system for a variety of diseases (Sohail et al., 2020). Furthermore, suitable surface functionalization on the external surface of MSNs can be performed to get a dynamic targeting mechanism. Such as chemicals sensitive to exterior stimuli like as pH, magnetic field, temperature, redox, and enzymes are utilized to manufacture environmentally friendly and controllable DDS to improve anticancer medication targeting and diminish side effects (Pan et al., 2012). Considering MSNPs’ potential to increase PZQ bioavailability and shorten half-life in the exchange (Amara et al., 2018). PZQ-Si has superior physiochemical properties such as tiny constant size (105 nm), spherical shape, and PZQ trapping efficacy (83%). In terms of overall worm load, tissue egg count, oogram pattern, and liver granuloma count and diameter, the PZQ-Si therapy produces the best antischistosomal results when taken orally. According to the findings, mesoporous silica NP is a likely safe nanocarrier for PZQ, enhancing its antischistome, antioxidant, immunomodulatory, and anti-inflammatory action in S. mansoni-infected mice (Tawfeek et al., 2019). Silica nanoparticles were effectively produced and analyzed, showing acceptable features for in vivo uses. Soluble worm antigenic preparation (SWAP) has been included in the MSNs. SWAP-loaded MSNs outperformed a conventional vaccination system in terms of immunization performance (SWAP-associated aluminum salt). These findings imply that SWAP-loaded MSNs is a potential approach for improving the immune response to S. mansoni (de Pádua Oliveira et al., 2016). MSNs have revolutionized nanobiotechnology due to their ability to contain a high number of drug molecules. In comparison to crude drug material, they were extremely successful in the delivery of hydrophobic drugs, considerably improving their solubility and bioavailability after oral administration (Zhang et al., 2012). Increasing the performance of already existing medicines is thought to be a more beneficial strategy from an economic standpoint (Tomiotto-Pellissier et al., 2017).
Nanoemulsions are tiny biphasic particles where it is one component is firmly dispersed into the next in the shape of microscopic droplets ranging in diameter from 10–600 nm and are used to transport oil-soluble substances (de Araújo et al., 2007). It can be an oil-in-water nanoemulsion or a bi-continuous nanoemulsion with oil and water interspersed throughout the system (Tomiotto-Pellissier et al., 2017).
Although aminoalkanethiosulfuric acids are a class of chemicals with schistosomicidal action, their use as a pharmaceutical preparation is restricted due to their low water solubility (de Oliveira Penido et al., 2008). The efficacy of 2-(butyl amino)-1-phenyl-1-ethane thiosulphuric acid (BphEA) utilizing nanoemulsions incorporating BphEA is enhanced. This nanoemulsion was capable of inducing worm motor activity loss, tegument disruption, female worm death, and male worm sluggish movement. When the parasites were treated with a free compound, these outcomes were not observed (de Araújo et al., 2007).
In the field of nanomedicine, the use of nanotechnology aims to improve public health and quality of life (Jia, 2005). Currently, nanotechnologies are used in various fields such as nano-medicine, nano-biotechnology, and nano-diagnosis as shown in (Figure 3).
Nanomaterials have been industrialized for their usage in medicine and several types of gadgets. Because of their antimicrobial properties, NPs of certain metals or metal oxides are now broadly used to treat various disorders and improve human health. NPs with particular physicochemical properties have demonstrated antimicrobial, antiviral, and antiparasitic properties (Jebali and Kazemi, 2013). Because of their small particle size and charged surface, nanoparticles can easily enter pathogenic cells and interfere with cellular contents such as protein and DNA, inducing programmed cell death (Sharmin et al., 2021). Antileishmanial capabilities are exhibited by gold and silver nanoparticles, as well as zinc, titanium, and magnesium oxide nanoparticles (Jebali and Kazemi, 2013). Furthermore, gold nanoparticles (GNPs) exhibited antischistosomal activity in the liver and brain of mice (Dkhil et al., 2015a). Treatment is a substantial therapeutic action. While it is favored that prevention of diseases is better than treatment, therapy for an already present disorder is necessary (Emerich, 2005). The main objective of treatment is to make the patient as comfortable as possible. For millennia, therapy has been evolving at a rapid pace. The introduction of Nano therapy healthcare has given rise to a new promise in medicine (Moghimi et al., 2005; Murthy, 2007). The application of nanomaterial as a new addition to enhance the conventional therapeutic procedure can be very useful (Nakayama and Okano, 2005; Gulati and Gupta, 2012). Nanomaterials and the use of a nano-enabled drug delivery system (Figure 4) have been a key emphasis with the hope of enhancing treatment results for schistosomiasis using PZQ. Table 2 lists the various nano-therapeutic compounds utilized as anti-schistosomal therapy for the treatment of diseases.
FIGURE 4. Schematic illustration of nanotechnology and nano-enable drug delivery system use in schistosomiasis treatment. (1) The liver and digestive system containing schistosomes that enter the body by skin penetration and reach the specified organ through the bloodstream (2) The oral and intravenous administration of a nanotechnologically based medication causes the worms’ membrane (tegument) to rupture, permitting the drug to be distributed and kill the worms.
TABLE 2. List of various nanoparticles used therapeutically in the treatment of schistosomal infections.
Schistosomiasis diagnosis is essential for the recognition and treatment of the diseases in prevalent and non-prevalent areas, as case finding, morbidity valuation, and control plans are all based on the result from diagnosis (Ajibola et al., 2018; Odundo et al., 2018). Current diagnostic methods for schistosomiasis include traditional parasitological methods, immunological diagnosis, as well as molecular methods (Katz et al., 1972). Common traditional parasitological methods include the use of a microscope for parasitic egg determination in urine and stool, or by immunological method (antibody or antigen detection) (van Etten et al., 1994; Odundo et al., 2018). Kato-Katz method is cheap and convenient and offers a high degree of specificity (Caldeira et al., 2012). The sensitivity of the test is contingent on the degree of the disease, the technique, and the judgment of the post-infection host. The understanding of existing antibody analyses is not ideal (ranges from 65 to 86 percent), (Odundo et al., 2018). Screen printing electrode biosensors have recently attracted significant attention in clinical sciences, food, and drug analysis process control. These sensors can determine very minute amounts of analytes by detecting the changes in potential, current, and conductance caused by an immune response (Taleat et al., 2014). Nanotechnology has been used to enhance the correctness and improve existing methods, but also provide new techniques unexpectedly (Lin et al., 2008; Yang M. et al., 2009b). The use of NPs in immunosensing has demonstrated great potential in the development of highly sensitive, versatile diagnostic care devices (Dequaire et al., 2000; Baptista et al., 2008; Wan et al., 2013). The list of various nanosensors used to boost the diagnostic effectiveness against schistosomal infections is provided in Table 3.
TABLE 3. List of Nano-sensor/Nano-material used in improving the diagnostic ability against schistosomal infections.
Vaccination has a significant influence on infectious disease control. There are a lot of serious diseases, yet no effective vaccine is available for them (Gregory et al., 2013). Nanotechnology is playing an increasingly important role in vaccine development. Measures that improve antigen efficiency are becoming increasingly essential as vaccination development focuses on less immunogenic “minimalist” formulations. The use of NPs in vaccine manufacturing increases not only antigen storage and immunogenicity but also permits targeted administration and slow release (Zhao et al., 2014). Nanotechnology usage has grown exponentially in recent decades, giving rise to the term “nanovaccinology” (Mamo and Poland, 2012). Nanoparticles are used in both prevention and treatment. It can be used as a vehicle for antigen delivery or as an immunostimulant adjuvant to activate or boost immunity. Prophylactic nanovaccinology is used to regulate and prevent various illnesses, whilst therapeutic nanovaccinology is used to cure cancer (Bolhassani et al., 2011; Krishnamachari et al., 2011). Traditional vaccinations contain live attenuated microorganisms, dead bacteria, or components of microbes. While many of these vaccinations were essential for controlling infectious organisms, many of them did not give protection against specific diseases (Gregory et al., 2013). A range of infectious diseases lacks licensed vaccines, as most of them are purified proteins, polysaccharides, or naked DNA encoding a protective antigen (Harandi et al., 2010). Control methods for schistosomiasis are primarily grounded on chemotherapy, but given eras of bulk action, the number of people diseased remains persistent (Harder, 2002). General prevalent zones have continuous reinfection of people and poor sanitation situations in the evolving countries, making drug treatment alone inadequate (Bergquist and Colley, 1998). Researchers find that the long period strategy for managing schistosomiasis is through combining immunization with drug treatment (Bergquist, 2002). An antischistosomal vaccine that even partially reduces worm loads might significantly decrease the pathology and limit the infection with a parasite (Chitsulo et al., 2004). The researcher has now redirected the usage of nanotechnology as vaccine delivery vehicle for treatment. The vaccine antigen would either be enclosed or imprinted on the exterior of the NPs. By packaging antigenic components, NPs allows for the administration of antigens that would otherwise be quickly degraded after injection or trigger a localized immune response. The conjugation of antigens to NPs allows the immunogen to be given to the immune system in the same way as the pathogen would, resulting in a comparable reaction (Gregory et al., 2013). Nanotechnology is used because of its greater stability and easier accessibility to the target site. The list of various nanoparticles used along with vaccine materials against schistosomal infections is given in Table 4.
TABLE 4. List of various nanoparticles used along with vaccine materials against schistosomal infections.
A lot of research has been conducted on nanotechnology because nanoparticles with dimensions of 100 nm or fewer usually have a high specific zone, a high adsorption capacity, and a variety of particular physicochemical, optical, and electrical characteristics (Ghormade et al., 2011). Nano-loading of NPs in nano-drug delivery systems can alter the permeability, of the membrane and remove special big barriers, thereby endorsing straight drug diffusion and intracellular delivery (Sun et al., 2008; Kunzmann et al., 2011). A nano drug delivery system can therefore increase consumption levels and curative effects, and decrease drug side effects. Biosensors are an influential and creative analytical tool with admirable classifications, very sensitive, quick, simple, and low-cost. It can be used in the field of drug finding, diagnosis, biomedicine, food safety, and environmental monitoring (Ullah et al., 2020c). As a result, these devices have become extensively established and employed in a variety of biological disciplines, including cancer detection, diagnostic reagent creation, and gene therapy. The relatively high cost and adverse environmental impact of the most often utilized NPs, such as gold, iron oxide, or ZnO-like NPs, severely limit their agro-biotechnological uses (Sun et al., 2008; Tang et al., 2012). The biosensor is also not commercially available for the majority of infections; further research is needed to evolve the technology (Janissen et al., 2017; Ullah et al., 2020c).
This review comprehensively documented the application of nanoparticles in schistosomiasis treatment, prevention, and diagnosis. Despite various treatments, option schistosomiasis is frequently seen in endemic as well as non-endemic areas. Further, the basic hurdle in schistosomiasis treatment is the low bioavailability of anti-schistosomal materials, the resistance of parasites due to misuse of drugs, and improper diagnosis. Researchers are working on the understanding treatment, diagnosis, prevention, and control of schistosomiasis. Unluckily there has not been any promising approach toward anti-schistosomal therapy. The involvement of scientists would confidently tackle the improvement of the treatment plans for this deliberating disease. Nanomedicine is an important application of nanotechnology for medical science for the last 20 years, which has grown as one of the most favorable techniques for the precision of conservative chemotherapies and diagnosis. The drug delivery system has the capability of administration of a low dose of drug and target-specific activity could be achieved by using nanotechnology. However, the fabrication and manipulation of nanomaterials in a repeatable and cost-effective way are still in their early stages. But it is expected that the application of NMs in schistosomiasis therapy will improve the current methods used in the detection, treatment, and control. The challenges involved in anti-schistosomal chemotherapy will be minimized by nanomedicine. The use of nanotechnology will increase bioavailability, decrease the quantity, toxicity, and side effects, and surge patient compliance. Hopefully, nanomedicine will deliver fairly improved and economical anti-schistosomal treatment practices than conventional chemotherapy, and probably will decrease the fiscal load of this tropical neglected disease. Therefore, nanotechnology approaches will encompass a wide range of solutions over conventional approaches in the upcoming days, for fast diagnosis, control, and prevention of this tropical neglected disease. It will be critical to discuss alternative ways to improve existing nanomedicine or nanotechnology approaches to improve schistosomiasis diagnosis and treatment.
Schistosomiasis continues to increase globally in tropical regions. It upsets the world’s deprived countries where there is a lack of basic facilities like safe water, sanitation, and other hygiene environmental conditions. The increasing shortcomings in the use of PZQ and other commonly used diagnostic methods have paved toward the use of potential alternative drug therapies. In this analysis, we have focused on the current application of nanomaterials used in biomedicine. Because of their distinct properties, nanomaterials have attracted a great deal of attention, and they have been utilized in the improvement of diagnostic techniques, therapeutic targets, and schistosomiasis prevention and vaccine. NPs are novel drug delivery systems for PZQ, which is a powerful anti-schistosomal medication that may be employed against different stages of Schistosoma. Silver, gold, selenium, silica, liposome, etc. are considered potential nanomaterials used for the treatment, and as vaccine candidates against schistosomiasis. Nanoparticles are easy to develop, have low toxicity, improve drug bioavailability by solubility modification, and improve drug absorption across the biological barrier. Nanotechnology also improves the sensitivity and efficacy of diagnostic kits. Therefore, the combination of these nanomaterial products may change the existing situation of therapeutics, control, and medical diagnosis.
AQ, HU, MS, and SA: write and formatted draft, SS: did visualization, AR, TS, PS, and PH did validation, review and editing.
The authors are thankful to the Innovation of practices for the establishment of mixed cultures of alfalfa to improve soil quality and produce safe forage (TH04030258) for financial assistance for this article. Also thankful to King Fahd University of Petroleum and Mineral Resources for financial support under project No. DF191016.
The authors declare that the research was conducted in the absence of any commercial or financial relationships that could be construed as a potential conflict of interest.
All claims expressed in this article are solely those of the authors and do not necessarily represent those of their affiliated organizations, or those of the publisher, the editors and the reviewers. Any product that may be evaluated in this article, or claim that may be made by its manufacturer, is not guaranteed or endorsed by the publisher.
Abaza, S. M. (2016). Applications of nanomedicine in parasitic diseases. Parasitol. United J. 9 (1), 1. doi:10.4103/1687-7942.192997
Adekiya, T. A., Kondiah, P. P. D., Choonara, Y. E., Kumar, P., and Pillay, V. (2020). A review of nanotechnology for targeted anti-schistosomal therapy. Front. Bioeng. Biotechnol. 8, 32. doi:10.3389/fbioe.2020.00032
Adisa, J., Egbujo, E. M., Yahaya, B. A., and Echejoh, G. (2012). Primary infertility associated with Schistosoma mansoni: A case report from the jos plateau, north central Nigeria. Afr. Health Sci. 12 (4), 563–565. doi:10.4314/ahs.v12i4.26
Agrawal, P. (2016). Potential prospects of future medicine: Nano medicine. J. Pharmacovigil. 4 (1), 1000–1149. doi:10.4172/2329-6887.1000e149
Ahmadi, F., Ebrahimnezjad, Y., Ghalehkandi, J., and Sis, N. (2014). “The effect of dietary zinc oxide nanoparticles on the antioxidant state and serum enzymes activity in broiler chickens during starter stage,” in International conference on biological, civil and environmental engineering (Dubai (UAE): IICBE), 26–28.
Ajibola, O., Gulumbe, B. H., Eze, A. A., and Obishakin, E. (2018). Tools for detection of schistosomiasis in resource limited settings. Med. Sci. (Basel). 6 (2), 39. doi:10.3390/medsci6020039
Amara, R. O., Ramadan, A. A., El-Moslemany, R. M., Eissa, M. M., El-Azzouni, M. Z., and El-Khordagui, L. K. (2018). Praziquantel–lipid nanocapsules: An oral nanotherapeutic with potential Schistosoma mansoni tegumental targeting. Int. J. Nanomedicine 13, 4493–4505. doi:10.2147/ijn.s167285
Ammar, H., El-Ridy, M., Ghorab, M., and Ghorab, M. (1994). Evaluation of the antischistosomal effect of praziquantel in a liposomal delivery system in mice. Int. J. Pharm. 103 (3), 237–241. doi:10.1016/0378-5173(94)90173-2
Araújo, R. V., Melo-Júnior, M. R., Beltrao, E. I., Mello, L. A., Iacomini, M., Carneiro-Leao, A. M., et al. (2011). Evaluation of the antischistosomal activity of sulfated α-D-glucan from the lichen Ramalina celastri free and encapsulated into liposomes. Braz. J. Med. Biol. Res. 44 (4), 311–318. doi:10.1590/s0100-879x2011000400007
Assis, N. R. G., Caires, A. J., Figueiredo, B. C., Morais, S. B., Mambelli, F. S., Marinho, F. V., et al. (2018). The use of gold nanorods as a new vaccine platform against schistosomiasis. J. Control. Release 275, 40–52. doi:10.1016/j.jconrel.2018.02.004
Ayan, A. K., Yenilmez, A., and Eroglu, H. (2017). Evaluation of radiolabeled curcumin-loaded solid lipid nanoparticles usage as an imaging agent in liver-spleen scintigraphy. Mater. Sci. Eng. C 75, 663–670. doi:10.1016/j.msec.2017.02.114
Baptista, P., Pereira, E., Eaton, P., Doria, G., Miranda, A., Gomes, I., et al. (2008). Gold nanoparticles for the development of clinical diagnosis methods. Anal. Bioanal. Chem. 391 (3), 943–950. doi:10.1007/s00216-007-1768-z
Bauomy, A. A. (2020). Zinc oxide nanoparticles and l-carnitine effects on neuro-schistosomiasis mansoni induced in mice. Environ. Sci. Pollut. Res. 27, 18699–18707. doi:10.1007/s11356-020-08356-5
Bergquist, N. R., and Colley, D. G. (1998). Schistosomiasis vaccine:research to development. Parasitol. Today 14 (3), 99–104. doi:10.1016/s0169-4758(97)01207-6
Bergquist, N. R. (2002). Schistosomiasis: From risk assessment to control. Trends Parasitol. 18 (7), 309–314. doi:10.1016/s1471-4922(02)02301-2
Bolhassani, A., Safaiyan, S., and Rafati, S. (2011). Improvement of different vaccine delivery systems for cancer therapy. Mol. Cancer 10, 3. doi:10.1186/1476-4598-10-3
Caldeira, K., Teixeira, C. F., Silveira, M. B. d., Fries, L. C. C. d., Romanzini, J., Bittencourt, H. R., et al. (2012). Comparison of the Kato-Katz and Helmintex methods for the diagnosis of schistosomiasis in a low-intensity transmission focus in Bandeirantes, Paraná, southern Brazil. Mem. Inst. Oswaldo Cruz 107 (5), 690–692. doi:10.1590/s0074-02762012000500019
Carlson, C., Hussain, S. M., Schrand, A. M., Braydich-Stolle, K. L., Hess, K. L., Jones, R. L., et al. (2008). Unique cellular interaction of silver nanoparticles: Size-dependent generation of reactive oxygen species. J. Phys. Chem. B 112 (43), 13608–13619. doi:10.1021/jp712087m
Cheng, W., Li, X., Zhang, C., Chen, W., Yuan, H., and Xu, S. (2017). Preparation and in vivo-in vitro evaluation of polydatin-PhospholipidComplex with improved dissolution and bioavailability. Int. J. Drug Dev. Res. 9.
Cheng, Y., Chen, X., Song, W., Kong, Z., Li, P., and Liu, Y. (2013). Contribution of silver ions to the inhibition of infectivity of Schistosoma japonicum cercariae caused by silver nanoparticles. Parasitology 140 (5), 617–625. doi:10.1017/s0031182012002211
Chitsulo, L., Loverde, P., and Engels, D. (2004). Focus: Schistosomiasis. Nat. Rev. Microbiol. 2 (1), 12–13. doi:10.1038/nrmicro801
Cioli, D., Pica-Mattoccia, L., Basso, A., and Guidi, A. (2014). Schistosomiasis control: Praziquantel forever? Mol. Biochem. Parasitol. 195 (1), 23–29. doi:10.1016/j.molbiopara.2014.06.002
Cohen, M. S., Stern, J. M., Vanni, A. J., Kelley, R. S., Baumgart, E., Field, D., et al. (2007). In vitro analysis of a nanocrystalline silver-coated surgical mesh. Surg. Infect. (Larchmt). 8 (3), 397–404. doi:10.1089/sur.2006.032
Colley, D. G., Bustinduy, A. L., Secor, W. E., and King, C. H. (2014). Human schistosomiasis. Lancet 383 (9936), 2253–2264. doi:10.1016/s0140-6736(13)61949-2
Darwish, A. S., Bayaumy, F. E. A., and Ismail, H. M. (2018). Photoactivated water-disinfecting, and biological properties of Ag NPs@Sm-doped ZnO nanorods/cuttlefish bone composite: In-vitro bactericidal, cercaricidal and schistosomicidal studies. Mater. Sci. Eng. C 93, 996–1011. doi:10.1016/j.msec.2018.09.007
Date, A. A., Joshi, M. D., and Patravale, V. B. (2007). Parasitic diseases: Liposomes and polymeric nanoparticles versus lipid nanoparticles. Adv. Drug Deliv. Rev. 59 (6), 505–521. doi:10.1016/j.addr.2007.04.009
de Almeida, A. E., Souza, A. L. R., Cassimiro, D. L., Gremiao, M. P. D., Ribeiro, C. A., and Crespi, M. S. (2012). Thermal characterization of solid lipid nanoparticles containing praziquantel. J. Therm. Anal. Calorim. 108 (1), 333–339. doi:10.1007/s10973-011-1814-0
de Almeida, M. P., and Carabineiro, S. A. (2013). The role of nanogold in human tropical diseases: Research, detection and therapy. Gold Bull. 46 (2), 65–79. doi:10.1007/s13404-013-0086-9
de Araújo, S. C., de Mattos, A. C., Teixeira, H. F., Coelho, P. M., Nelson, D. L., and de Oliveira, M. C. (2007). Improvement of in vitro efficacy of a novel schistosomicidal drug by incorporation into nanoemulsions. Int. J. Pharm. X. 337 (1-2), 307–315. doi:10.1016/j.ijpharm.2007.01.009
de Melo, A. L., Silva-Barcellos, N. M., Demicheli, C., and Frézard, F. (2003). Enhanced schistosomicidal efficacy of tartar emetic encapsulated in pegylated liposomes. Int. J. Pharm. X. 255 (1-2), 227–230. doi:10.1016/s0378-5173(03)00125-x
de Oliveira Penido, M. L., Zech Coelho, P. M., de Mello, R. T., Piló-Veloso, D., de Oliveira, M. C., Kusel, J. R., et al. (2008). Antischistosomal activity of aminoalkanethiols, aminoalkanethiosulfuric acids and the corresponding disulfides. Acta Trop. 108 (2-3), 249–255. doi:10.1016/j.actatropica.2008.10.005
de Pádua Oliveira, D. C., de Barros, A. L. B., Belardi, R. M., de Goes, A. M., de Oliveira Souza, B. K., and Soares, D. C. F. (2016). Mesoporous silica nanoparticles as a potential vaccine adjuvant against Schistosoma mansoni. J. Drug Deliv. Sci. Technol. 35, 234–240. doi:10.1016/j.jddst.2016.07.002
de Souza, A. L. R., Andreani, T., De Oliveira, R. N., Kiill, C. P., dos Santos, F. K., Allegretti, S. M., et al. (2014). In vitro evaluation of permeation, toxicity and effect of praziquantel-loaded solid lipid nanoparticles against Schistosoma mansoni as a strategy to improve efficacy of the schistosomiasis treatment. Int. J. Pharm. 463 (1), 31–37. doi:10.1016/j.ijpharm.2013.12.022
Dequaire, M., Degrand, C., and Limoges, B. (2000). An electrochemical metalloimmunoassay based on a colloidal gold label. Anal. Chem. 72 (22), 5521–5528. doi:10.1021/ac000781m
Dkhil, M. A., Bauomy, A. A., Diab, M. S., and Al-Quraishy, S. (2015a). Antioxidant and hepatoprotective role of gold nanoparticles against murine hepatic schistosomiasis. Int. J. Nanomedicine 10, 7467–7475. doi:10.2147/ijn.s97622
Dkhil, M. A., Bauomy, A. A., Diab, M. S., Wahab, R., Delic, D., and Al-Quraishy, S. (2015b). Impact of gold nanoparticles on brain of mice infected with Schistosoma mansoni. Parasitol. Res. 114 (10), 3711–3719. doi:10.1007/s00436-015-4600-2
Dkhil, M. A., Khalil, M. F., Bauomy, A. A., Diab, M. S., and Al-Qura, S. (2016b). Efficacy of gold nanoparticles against nephrotoxicity induced by Schistosoma mansoni infection in mice. Biomed. Environ. Sci. 29 (11), 773–781. doi:10.3967/bes2016.104
Dkhil, M. A., Khalil, M. F., Diab, M. S., Bauomy, A. A., and Al-Quraishy, S. (2017). Effect of gold nanoparticles on mice splenomegaly induced by schistosomiasis mansoni. Saudi J. Biol. Sci. 24 (6), 1418–1423. doi:10.1016/j.sjbs.2016.12.017
Dkhil, M. A., Khalil, M. F., Diab, M. S., Bauomy, A. A., Santourlidis, S., Al-Shaebi, E. M., et al. (2019). Evaluation of nanoselenium and nanogold activities against murine intestinal schistosomiasis. Saudi J. Biol. Sci. 26 (7), 1468–1472. doi:10.1016/j.sjbs.2018.02.008
Dkhil, M., Bauomy, A., Diab, M., and Al-Quraishy, S. (2016a). Protective role of selenium nanoparticles against Schistosoma mansoni induced hepatic injury in mice. Biomed. Res. 27, 214–219.
Doenhoff, M. J., Cioli, D., and Utzinger, J. (2008). Praziquantel: Mechanisms of action, resistance and new derivatives for schistosomiasis. Curr. Opin. Infect. Dis. 21 (6), 659–667. doi:10.1097/qco.0b013e328318978f
Dykman, L. A., and Khlebtsov, N. G. (2011). Gold nanoparticles in biology and medicine: Recent advances and prospects. Acta Naturae 3 (2), 34–55. doi:10.32607/20758251-2011-3-2-34-55
Eissa, M. M., El-Azzouni, M. Z., El-Khordagui, L. K., Abdel Bary, A., El-Moslemany, R. M., and Abdel Salam, S. A. (2020a). Evaluation of prophylactic efficacy and safety of praziquantel-miltefosine nanocombination in experimental Schistosomiasis mansoni. Acta Trop. 212, 105714. doi:10.1016/j.actatropica.2020.105714
Eissa, M. M., El-Azzouni, M. Z., El-Khordagui, L. K., Abdel Bary, A., El-Moslemany, R. M., and Abdel Salam, S. A. (2020b). Single oral fixed-dose praziquantel-miltefosine nanocombination for effective control of experimental schistosomiasis mansoni. Parasit. Vectors 13 (1), 474. doi:10.1186/s13071-020-04346-1
Eissa, M. M., El-Moslemany, R. M., Ramadan, A. A., Amer, E. I., El-Azzouni, M. Z., and El-Khordagui, L. K. (2015). Miltefosine lipid nanocapsules for single dose oral treatment of schistosomiasis mansoni: A preclinical study. PloS one 10 (11), e0141788. doi:10.1371/journal.pone.0141788
El Ridy, M., Khalil, R., Moustafa, D., El-Rashdy, M., Mohamed, M., Osman, A., et al. (1997). Chemoprophylaxis of schistosomiasis using liposome encapsulated oxamniquine. Drug Dev. industrial Pharm. 23 (8), 771–782. doi:10.3109/03639049709150548
El-Moslemany, R. M., Eissa, M. M., Ramadan, A. A., El-Khordagui, L. K., and El-Azzouni, M. Z. (2016). Miltefosine lipid nanocapsules: Intersection of drug repurposing and nanotechnology for single dose oral treatment of pre-patent schistosomiasis mansoni. Acta trop. 159, 142–148. doi:10.1016/j.actatropica.2016.03.038
El-Ridy, M., Akbarieh, M., Kassem, M., Sharkawi, M., and Tawashi, R. (1989). Chemoprophylaxis of schistosomiasis using liposome-encapsulated tartar emetic. Int. J. Pharm. 56 (1), 23–27. doi:10.1016/0378-5173(89)90056-2
Elechiguerra, J. L., Burt, J. L., Morones, J. R., Camacho-Bragado, A., Gao, X., Lara, H. H., et al. (2005). Interaction of silver nanoparticles with HIV-1. J. Nanobiotechnology 3, 6. doi:10.1186/1477-3155-3-6
Emerich, D. F. (2005). Nanomedicine–prospective therapeutic and diagnostic applications. Taylor & Francis.
Ferrari, M. L. A., Coelho, P. M. Z., Antunes, C. M. F., Tavares, C. A. P., and da Cunha, A. S. (2003). Efficacy of oxamniquine and praziquantel in the treatment of Schistosoma mansoni infection: A controlled trial. Bull. World Health Organ. 81 (3), 190–196.
Forrest, M. L., and Kwon, G. S. (2008). Clinical developments in drug delivery nanotechnology. Adv. Drug Deliv. Rev. 60 (8), 861–862. doi:10.1016/j.addr.2008.02.013
Frézard, F., and de Melo, A. L. (1997). Evaluation of the schistosomicidal efficacy of liposome-entrapped oxamniquine. Rev. Inst. Med. Trop. S. Paulo 39 (2), 97–100. doi:10.1590/s0036-46651997000200006
Frezza, T. F., de Souza, A. L. R., Prado, C. C. R., de Oliveira, C. N. F., Gremiao, M. P. D., Giorgio, S., et al. (2015). Effectiveness of hyperbaric oxygen for experimental treatment of schistosomiasis mansoni using praziquantel-free and encapsulated into liposomes: Assay in adult worms and oviposition. Acta trop. 150, 182–189. doi:10.1016/j.actatropica.2015.07.022
Frezza, T. F., Gremiao, M. P. D., Zanotti-Magalhaes, E. M., Magalhaes, L. A., de Souza, A. L. R., and Allegretti, S. M. (2013). Liposomal-praziquantel: Efficacy against Schistosoma mansoni in a preclinical assay. Acta trop. 128 (1), 70–75. doi:10.1016/j.actatropica.2013.06.011
Ganesan, P., Ramalingam, P., Karthivashan, G., Ko, Y. T., and Choi, D. K. (2018). Recent developments in solid lipid nanoparticle and surface-modified solid lipid nanoparticle delivery systems for oral delivery of phyto-bioactive compounds in various chronic diseases. Int. J. Nanomedicine 13, 1569–1583. doi:10.2147/ijn.s155593
Ghormade, V., Deshpande, M. V., and Paknikar, K. M. (2011). Perspectives for nano-biotechnology enabled protection and nutrition of plants. Biotechnol. Adv. 29 (6), 792–803. doi:10.1016/j.biotechadv.2011.06.007
Gregory, A. E., Williamson, D., and Titball, R. (2013). Vaccine delivery using nanoparticles. Front. Cell. Infect. Microbiol. 3, 13. doi:10.3389/fcimb.2013.00013
Gryseels, B., Polman, K., Clerinx, J., and Kestens, L. (2006). Human schistosomiasis. Lancet 368 (9541), 1106–1118. doi:10.1016/s0140-6736(06)69440-3
Gu, Y. J., Cheng, J., Lin, C. C., Lam, Y. W., Cheng, S. H., and Wong, W. T. (2009). Nuclear penetration of surface functionalized gold nanoparticles. Toxicol. Appl. Pharmacol. 237 (2), 196–204. doi:10.1016/j.taap.2009.03.009
Guimaraes, M. A., Campelo, Y. D., Véras, L. M., Colhone, M. C., Lima, D. F., Ciancaglini, P., et al. (2014). Nanopharmaceutical approach of epiisopiloturine alkaloid carried in liposome system: Preparation and <I>in vitro</I> schistosomicidal activity. J. Nanosci. Nanotechnol. 14 (6), 4519–4528. doi:10.1166/jnn.2014.8248
Gulati, N., and Gupta, H. (2012). Nanomedicine: Potential devices for diagnostics. Recent Pat. Nanomedicine 2 (2), 146–155. doi:10.2174/1877912311202020146
Harandi, A. M., Medaglini, D., and Shattock, R. J. (2010). Vaccine adjuvants: A priority for vaccine research. Vaccine 28 (12), 2363–2366. doi:10.1016/j.vaccine.2009.12.084
Harder, A. (2002). Chemotherapeutic approaches to schistosomes: Current knowledge and outlook. Parasitol. Res. 88 (5), 395–397. doi:10.1007/s00436-001-0588-x
Huynh, N. T., Passirani, C., Saulnier, P., and Benoit, J. P. (2009). Lipid nanocapsules: A new platform for nanomedicine. Int. J. Pharm. X. 379 (2), 201–209. doi:10.1016/j.ijpharm.2009.04.026
Janissen, R., Sahoo, P. K., Santos, C. A., da Silva, A. M., von Zuben, A. A. G., Souto, D. E. P., et al. (2017). InP nanowire biosensor with tailored biofunctionalization: Ultrasensitive and highly selective disease biomarker detection. Nano Lett. 17 (10), 5938–5949. doi:10.1021/acs.nanolett.7b01803
Jebali, A., and Kazemi, B. (2013). Nano-based antileishmanial agents: A toxicological study on nanoparticles for future treatment of cutaneous leishmaniasis. Toxicol. Vitro 27 (6), 1896–1904. doi:10.1016/j.tiv.2013.06.002
Jia, L. (2005). Nanoparticle formulation increases oral bioavailability of poorly soluble drugs: Approaches, experimental evidences and theory. Curr. Nanosci. 1 (3), 237–243. doi:10.2174/157341305774642939
Jiang, J., Pi, J., and Cai, J. (2018). The advancing of zinc oxide nanoparticles for biomedical applications. Bioinorg. Chem. Appl. 2018, 1–18. doi:10.1155/2018/1062562
Kame, M., Elbaz, H., Demerdash, Z., Elmoneem, E., Hendawy, M., and Bayoumi, I. (2016). Nano-immunoassay for diagnosis of active schistosomal infection. World J. Med. Sci. 13, 27.
Kar, P. K., Murmu, S., Saha, S., Tandon, V., and Acharya, K. (2014). Anthelmintic efficacy of gold nanoparticles derived from a phytopathogenic fungus, Nigrospora oryzae. PLoS One 9 (1), e84693. doi:10.1371/journal.pone.0084693
Katz, N., Chaves, A., and Pellegrino, J. (1972). A simple device for quantitative stool thick-smear technique in schistosomiasis mansoni. Rev. Inst. Med. Trop. Sao Paulo 14 (6), 397–400.
King, C. L., and Highashi, G. I. (1992). Schistosoma mansoni: Silver ion (Ag +) stimulates and reversibly inhibits lipid-induced cercarial penetration. Exp. Parasitol. 75 (1), 31–39. doi:10.1016/0014-4894(92)90119-u
Kolenyak-Santos, F., Garnero, C., de Oliveira, R. N., de Souza, A. L., Chorilli, M., Allegretti, S. M., et al. (2015). Nanostructured lipid carriers as a strategy to improve the <I>in vitro</I> schistosomiasis activity of praziquantel. J. Nanosci. Nanotechnol. 15 (1), 761–772. doi:10.1166/jnn.2015.9186
Krishnamachari, Y., Geary, S. M., Lemke, C. D., and Salem, A. K. (2011). Nanoparticle delivery systems in cancer vaccines. Pharm. Res. 28 (2), 215–236. doi:10.1007/s11095-010-0241-4
Kunzmann, A., Andersson, B., Thurnherr, T., Krug, H., Scheynius, A., and Fadeel, B. (2011). Toxicology of engineered nanomaterials: Focus on biocompatibility, biodistribution and biodegradation. Biochimica Biophysica Acta - General Subj. 1810 (3), 361–373. doi:10.1016/j.bbagen.2010.04.007
Labib El Gendy, A. E. M., Mohammed, F. A., Abdel-Rahman, S. A., Shalaby, T. I. A., Fathy, G. M., Mohammad, S. M., et al. (2019). Effect of nanoparticles on the therapeutic efficacy of praziquantel against Schistosoma mansoni infection in murine models. J. Parasit. Dis. 43 (3), 416–425. doi:10.1007/s12639-019-01106-6
Lansdown, A. B. (2006). Silver in health care: Antimicrobial effects and safety in use. Curr. Probl. Dermatol. 33, 17–34. doi:10.1159/000093928
Lasoń, E., Sikora, E., and Ogonowski, J. (2013). Influence of process parameters on properties of Nanostructured Lipid Carriers (NLC) formulation. Acta Biochim. Pol. 60 (4), 773–777. doi:10.18388/abp.2013_2056
Li, G., Huang, S., Lian, L., Song, X., Sun, W., Miao, J., et al. (2019). Derivation and external validation of a model to predict 2-year mortality risk of patients with advanced schistosomiasis after discharge. EBioMedicine 47, 309–318. doi:10.1016/j.ebiom.2019.08.028
Li, Y., Li, N., Pan, W., Yu, Z., Yang, L., and Tang, B. (2017). Hollow mesoporous silica nanoparticles with tunable structures for controlled drug delivery. ACS Appl. Mat. Interfaces 9 (3), 2123–2129. doi:10.1021/acsami.6b13876
Li, Z., and Ji, X. (2015). Association of tissue transglutaminase and NLRP3 inflammasome in liver inflammation after Schistosoma japonicum infection (INM6P.343). J. Immunol. 194 (1), 193–117.
Lin, C. C., Chen, L. C., Huang, C. H., Ding, S. J., Chang, C. C., and Chang, H. C. (2008). Development of the multi-functionalized gold nanoparticles with electrochemical-based immunoassay for protein A detection. J. Electroanal. Chem. 619, 39–45. doi:10.1016/j.jelechem.2008.03.014
Lindenberg, M., Kopp, S., and Dressman, J. B. (2004). Classification of orally administered drugs on the World Health Organization Model list of Essential Medicines according to the biopharmaceutics classification system. Eur. J. Pharm. Biopharm. 58 (2), 265–278. doi:10.1016/j.ejpb.2004.03.001
Mader, K. (2006). “Solid lipid nanoparticles as drug carriers,” in Nanoparticulates as drug carriers (London: Imperial College Press), 187–212.
Mamo, T., and Poland, G. A. (2012). Nanovaccinology: The next generation of vaccines meets 21st century materials science and engineering. Vaccine 30 (47), 6609–6611. doi:10.1016/j.vaccine.2012.08.023
Mbanefo, E. C., Kumagai, T., Kodama, Y., Kurosaki, T., Furushima-Shimogawara, R., Cherif, M. S., et al. (2015). Immunogenicity and anti-fecundity effect of nanoparticle coated glutathione S-transferase (SjGST) DNA vaccine against murine Schistosoma japonicum infection. Parasitol. Int. 64 (4), 24–31. doi:10.1016/j.parint.2015.01.005
Mishra, V., Bansal, K. K., Verma, A., Yadav, N., Thakur, S., Sudhakar, K., et al. (2018). Solid lipid nanoparticles: Emerging colloidal nano drug delivery systems. Pharmaceutics 10 (4), 191. doi:10.3390/pharmaceutics10040191
Moghimi, S. M., Hunter, A. C., and Murray, J. C. (2005). Nanomedicine: Current status and future prospects. FASEB J. 19 (3), 311–330. doi:10.1096/fj.04-2747rev
Mokbel, K. E. M., Baiuomy, I. R., Sabry, A. E. A., Mohammed, M. M., and El-Dardiry, M. A. (2020). In vivo assessment of the antischistosomal activity of curcumin loaded nanoparticles versus praziquantel in the treatment of Schistosoma mansoni. Sci. Rep. 10 (1), 15742. doi:10.1038/s41598-020-72901-y
Moniruzzaman, M., and Min, T. (2020). Curcumin, curcumin nanoparticles and curcumin nanospheres: A review on their pharmacodynamics based on monogastric farm animal, poultry and fish nutrition. Pharmaceutics 12 (5), 447. doi:10.3390/pharmaceutics12050447
Mourao, S. C., Costa, P. I., Salgado, H. R., and Gremiao, M. P. (2005). Improvement of antischistosomal activity of praziquantel by incorporation into phosphatidylcholine-containing liposomes. Int. J. Pharm. X. 295 (1-2), 157–162. doi:10.1016/j.ijpharm.2005.02.009
Moustafa, M. A., Mossalem, H. S., Sarhan, R. M., Abdel-Rahman, A. A., and Hassan, E. M. (2018). The potential effects of silver and gold nanoparticles as molluscicides and cercaricides on Schistosoma mansoni. Parasitol. Res. 117 (12), 3867–3880. doi:10.1007/s00436-018-6093-2
Mufamadi, M. S., Pillay, V., Choonara, Y. E., Du Toit, L. C., Modi, G., Naidoo, D., et al. (2011). A review on composite liposomal technologies for specialized drug delivery. J. Drug Deliv. 2011, 939851–939919. doi:10.1155/2011/939851
Murthy, S. K. (2007). Nanoparticles in modern medicine: State of the art and future challenges. Int. J. Nanomedicine 2 (2), 129–141.
Muthu, M. S., Kulkarni, S. A., Xiong, J., and Feng, S. S. (2011). Vitamin E TPGS coated liposomes enhanced cellular uptake and cytotoxicity of docetaxel in brain cancer cells. Int. J. Pharm. X. 421 (2), 332–340. doi:10.1016/j.ijpharm.2011.09.045
Nadhman, A., Nazir, S., Khan, M. I., Arooj, S., Bakhtiar, M., Shahnaz, G., et al. (2014). PEGylated silver doped zinc oxide nanoparticles as novel photosensitizers for photodynamic therapy against Leishmania. Free Radic. Biol. Med. 77, 230–238. doi:10.1016/j.freeradbiomed.2014.09.005
Nakayama, M., and Okano, T. (2005). Drug delivery systems using nano-sized drug carriers. Gan Kagaku Ryoho. 32 (7), 935–940.
Naumih, J. O., Noah, N., Andala, D., Janet, K., Ndikau, M., Kimani, G., et al. (2016). Spectroelectrochemical characterization of anti-schistosoma-gold nanoparticle conjugate for use in immunoassays. J. Kenya Chem. Soc. 9.
Odundo, J., Noah, N., Andala, D., Kiragu, J., and Masika, E. (2018). Development of an electrochemical nano-biosensor for rapid and sensitive diagnosis of bilharzia in Kenya. S. Afr. J. Chem. 71 (1), 127–134. doi:10.17159/0379-4350/2018/v71a16
Oliveira, C. R., Rezende, C. M., Silva, M. R., Borges, O. M., Pêgo, A. P., and Goes, A. M. (2012a). Oral vaccination based on DNA-chitosan nanoparticles against Schistosoma mansoni infection. Sci. World J. 2012, 1–11. doi:10.1100/2012/938457
Oliveira, C. R., Rezende, C. M., Silva, M. R., Pêgo, A. P., Borges, O., and Goes, A. M. (2012b). A new strategy based on SmRho protein loaded chitosan nanoparticles as a candidate oral vaccine against schistosomiasis. PLoS Negl. Trop. Dis. 6 (11), e1894. doi:10.1371/journal.pntd.0001894
Pan, L., He, Q., Liu, J., Chen, Y., Ma, M., Zhang, L., et al. (2012). Nuclear-targeted drug delivery of TAT peptide-conjugated monodisperse mesoporous silica nanoparticles. J. Am. Chem. Soc. 134 (13), 5722–5725. doi:10.1021/ja211035w
Pérez-Sánchez, R., Valero, M. L., Ramajo-Hernández, A., Siles-Lucas, M., Ramajo-Martín, V., and Oleaga, A. (2008). A proteomic approach to the identification of tegumental proteins of male and female Schistosoma bovis worms. Mol. Biochem. Parasitol. 161 (2), 112–123. doi:10.1016/j.molbiopara.2008.06.011
Pindiprolu, S., Chintamaneni, P. K., Krishnamurthy, P. T., and Ratna Sree Ganapathineedi, K. (2019). Formulation-optimization of solid lipid nanocarrier system of STAT3 inhibitor to improve its activity in triple negative breast cancer cells. Drug Dev. Ind. Pharm. 45 (2), 304–313. doi:10.1080/03639045.2018.1539496
Qadeer, A., Giri, B. R., Ullah, H., and Cheng, G. (2021). Transcriptional profiles of genes potentially involved in extracellular vesicle biogenesis in Schistosoma japonicum. Acta Trop. 217, 105851. doi:10.1016/j.actatropica.2021.105851
Qi, J., Lu, Y., and Wu, W. (2012). Absorption, disposition and pharmacokinetics of solid lipid nanoparticles. Curr. Drug Metab. 13 (4), 418–428. doi:10.2174/138920012800166526
Radwan, A., El-Lakkany, N. M., William, S., El-Feky, G. S., Al-Shorbagy, M. Y., Saleh, S., et al. (2019). A novel praziquantel solid lipid nanoparticle formulation shows enhanced bioavailability and antischistosomal efficacy against murine S. mansoni infection. Parasit. Vectors 12 (1), 304. doi:10.1186/s13071-019-3563-z
Rajabi, M., and Mousa, S. A. (2016). Lipid nanoparticles and their application in nanomedicine. Curr. Pharm. Biotechnol. 17 (8), 662–672. doi:10.2174/1389201017666160415155457
Ramajo-Hernández, A., Pérez-Sánchez, R., Ramajo-Martín, V., and Oleaga, A. (2007). Schistosoma bovis: Plasminogen binding in adults and the identification of plasminogen-binding proteins from the worm tegument. Exp. Parasitol. 115 (1), 83–91. doi:10.1016/j.exppara.2006.07.003
Ribeiro de Souza, A., Andreani, T., Nunes, F. M., Cassimiro, D., Almeida, A., Ribeiro, C., et al. (2012). Loading of praziquantel in the crystal lattice of solid lipid nanoparticles: Studies by DSC and SAXS. J. Therm. Anal. Calorim. 108, 353–360. doi:10.1007/s10973-011-1871-4
Rollinson, D., Knopp, S., Levitz, S., Stothard, J. R., Tchuem Tchuenté, L.-A., Garba, A., et al. (2013). Time to set the agenda for schistosomiasis elimination. Acta Trop. 128 (2), 423–440. doi:10.1016/j.actatropica.2012.04.013
Santos, G. S., Andrade, C. A., Bruscky, I. S., Wanderley, L. B., Melo, F. L., and Oliveira, M. D. (2017). Impedimetric nanostructured genosensor for detection of schistosomiasis in cerebrospinal fluid and serum samples. J. Pharm. Biomed. analysis 137, 163–169. doi:10.1016/j.jpba.2017.01.031
Santos, G. S., Caldas, R. G., Melo, F. L., Bruscky, I. S., Silva, M. A., Wanderley, L. B., et al. (2019). Label-free nanostructured biosensor for Schistosoma mansoni detection in complex biological fluids. Talanta 204, 395–401. doi:10.1016/j.talanta.2019.05.111
Sharmin, S., Rahaman, M. M., Sarkar, C., Atolani, O., Islam, M. T., and Adeyemi, O. S. (2021). Nanoparticles as antimicrobial and antiviral agents: A literature-based perspective study. Heliyon 7 (3), e06456. doi:10.1016/j.heliyon.2021.e06456
Shohayeb, M., Arida, H., Mersal, G. A., and El-Badawy, M. (2016). Development of a nanotechnology-based screen-printed biosensor for detection of Schistosoma mansoni antibodies. Int. J. Electrochem. Sci. 11, 1337–1344.
Shou-fu, J., Xiao-ping, Z., Bao-liang, L., Yan-yan, H., Jing, L., Yue-hong, T., et al. (2014). Evaluation of partially purified soluble egg antigens in colloidal gold immunochromatography assay card for rapid detection of anti-Schistosoma japonicum antibodies. Southeast Asian J. Trop. Med. Public Health 45 (3), 568–575.
Siqueira, L. D. P., Fontes, D. A. F., Aguilera, C. S. B., Timóteo, T. R. R., Ângelos, M. A., Silva, L., et al. (2017). Schistosomiasis: Drugs used and treatment strategies. Acta Trop. 176, 179–187. doi:10.1016/j.actatropica.2017.08.002
Skelly, P. J., and Alan Wilson, R. (2006). Making sense of the schistosome surface. Adv. Parasitol. 63, 185–284. doi:10.1016/s0065-308x(06)63003-0
Sohail, M., Guo, W., Li, Z., Xu, H., Zhao, F., Chen, D., et al. (2020). Nanocarrier-based drug delivery system for cancer therapeutics: A review of the last decade. Curr. Med. Chem. 28, 3753–3772. doi:10.2174/0929867327666201005111722
Sotillo, J., Pearson, M., Becker, L., Mulvenna, J., and Loukas, A. (2015). A quantitative proteomic analysis of the tegumental proteins from Schistosoma mansoni schistosomula reveals novel potential therapeutic targets. Int. J. Parasitol. 45 (8), 505–516. doi:10.1016/j.ijpara.2015.03.004
Sun, X., Liu, Z., Welsher, K., Robinson, J. T., Goodwin, A., Zaric, S., et al. (2008). Nano-graphene oxide for cellular imaging and drug delivery. Nano Res. 1 (3), 203–212. doi:10.1007/s12274-008-8021-8
Taleat, Z., Khoshroo, A., and Mazloum-Ardakani, M. (2014). Screen-printed electrodes for biosensing: A review (2008–2013). Microchim. Acta 181 (9-10), 865–891. doi:10.1007/s00604-014-1181-1
Tang, F., Li, L., and Chen, D. (2012). Mesoporous silica nanoparticles: Synthesis, biocompatibility and drug delivery. Adv. Mat. 24 (12), 1504–1534. doi:10.1002/adma.201104763
Tapeinos, C., Battaglini, M., and Ciofani, G. (2017). Advances in the design of solid lipid nanoparticles and nanostructured lipid carriers for targeting brain diseases. J. Control. Release 264, 306–332. doi:10.1016/j.jconrel.2017.08.033
Tawfeek, G. M., Baki, M. H. A., Ibrahim, A. N., Mostafa, M. A. H., Fathy, M. M., and Diab, M. (2019). Enhancement of the therapeutic efficacy of praziquantel in murine Schistosomiasis mansoni using silica nanocarrier. Parasitol. Res. 118 (12), 3519–3533. doi:10.1007/s00436-019-06475-8
Tikariha, S., Singh, S., Banerjee, S., and Vidyarthi, A. (2012). Biosynthesis of gold nanoparticles, scope and application: A review. Int. J. Pharm. Sci. Res. 3 (6), 1603.
Tomiotto-Pellissier, F., Miranda-Sapla, M. M., Machado, L. F., da Silva Bortoleti, B. T., Sahd, C. S., Chagas, A. F., et al. (2017). Nanotechnology as a potential therapeutic alternative for schistosomiasis. Acta trop. 174, 64–71. doi:10.1016/j.actatropica.2017.06.025
Tousif, S., Singh, D. K., Mukherjee, S., Ahmad, S., Arya, R., Nanda, R., et al. (2017). Nanoparticle-formulated curcumin prevents posttherapeutic disease reactivation and reinfection with Mycobacterium tuberculosis following isoniazid therapy. Front. Immunol. 8, 739. doi:10.3389/fimmu.2017.00739
Ullah, H., Arbab, S., Khan, M. I. U., Li, K., Muhammad, N., Suleman, , et al. (2020a). Circulating cell-free mitochondrial DNA fragment: A possible marker for early detection of Schistosoma japonicum. Infect. Genet. Evol. 88, 104683. doi:10.1016/j.meegid.2020.104683
Ullah, H., Arbab, S., Li, K., Khan, M. I. U., Qadeer, A., and Muhammad, N. (2022a). Schistosomiasis related circulating cell-free DNA: A useful biomarker in diagnostics. Mol. Biochem. Parasitol. 251, 111495. doi:10.1016/j.molbiopara.2022.111495
Ullah, H., Qadeer, A., and Giri, B. R. (2020b). Detection of circulating cell-free DNA to diagnose Schistosoma japonicum infection. Acta Trop. 211, 105604. doi:10.1016/j.actatropica.2020.105604
Ullah, H., Qadeer, A., Rashid, M., Rashid, M. I., and Cheng, G. (2020c). Recent advances in nucleic acid-based methods for detection of helminth infections and the perspective of biosensors for future development. Parasitology 147 (4), 383–392. doi:10.1017/s0031182019001665
Ullah, H., Tian, Y., Arbab, S., Li, K., Khan, M. I. U., Rahman, S. U., et al. (2022b). Circulatory microRNAs in helminthiases: Potent as diagnostics biomarker, its potential role and limitations. Front. Vet. Sci. 9, 1018872. doi:10.3389/fvets.2022.1018872
Üner, M., and Yener, G. (2007). Importance of solid lipid nanoparticles (SLN) in various administration routes and future perspectives. Int. J. Nanomedicine 2 (3), 289–300.
van Etten, L., Folman, C. C., Eggelte, T. A., Kremsner, P. G., and Deelder, A. M. (1994). Rapid diagnosis of schistosomiasis by antigen detection in urine with a reagent strip. J. Clin. Microbiol. 32 (10), 2404–2406. doi:10.1128/jcm.32.10.2404-2406.1994
Van Hellemond, J. J., Retra, K., Brouwers, J. F., van Balkom, B. W., Yazdanbakhsh, M., Shoemaker, C. B., et al. (2006). Functions of the tegument of schistosomes: Clues from the proteome and lipidome. Int. J. Parasitol. 36 (6), 691–699. doi:10.1016/j.ijpara.2006.01.007
Veerasamy, R., Xin, T. Z., Gunasagaran, S., Xiang, T. F. W., Yang, E. F. C., Jeyakumar, N., et al. (2011). Biosynthesis of silver nanoparticles using mangosteen leaf extract and evaluation of their antimicrobial activities. J. Saudi Chem. Soc. 15 (2), 113–120. doi:10.1016/j.jscs.2010.06.004
Vieites, M., Smircich, P., Guggeri, L., Marchán, E., Gómez-Barrio, A., Navarro, M., et al. (2009). Synthesis and characterization of a pyridine-2-thiol N-oxide gold(I) complex with potent antiproliferative effect against Trypanosoma cruzi and Leishmania sp. insight into its mechanism of action. J. Inorg. Biochem. 103 (10), 1300–1306. doi:10.1016/j.jinorgbio.2009.02.011
Wan, Y., Su, Y., Zhu, X., Liu, G., and Fan, C. (2013). Development of electrochemical immunosensors towards point of care diagnostics. Biosens. Bioelectron. 47, 1–11. doi:10.1016/j.bios.2013.02.045
Wang, X., Dai, Y., Zhao, S., Tang, J., Li, H., Xing, Y., et al. (2014). PAMAM-Lys, a novel vaccine delivery vector, enhances the protective effects of the SjC23 DNA vaccine against Schistosoma japonicum infection. PLoS One 9 (1), e86578. doi:10.1371/journal.pone.0086578
WHO (2022). Schistosomiasis. Available at: https://www.who.int/news-room/fact-sheets/detail/schistosomiasis.
Wissing, S. A., Kayser, O., and Müller, R. H. (2004). Solid lipid nanoparticles for parenteral drug delivery. Adv. Drug Deliv. Rev. 56 (9), 1257–1272. doi:10.1016/j.addr.2003.12.002
Xiao, S. H., Catto, B. A., and Webster, L. T. (1985). Effects of praziquantel on different developmental stages of Schistosoma mansoni in vitro and in vivo. J. Infect. Dis. 151 (6), 1130–1137. doi:10.1093/infdis/151.6.1130
Xie, S., Pan, B., Wang, M., Zhu, L., Wang, F., Dong, Z., et al. (2010). Formulation, characterization and pharmacokinetics of praziquantel-loaded hydrogenated castor oil solid lipid nanoparticles. Nanomedicine 5 (5), 693–701. doi:10.2217/nnm.10.42
Xu, R., Feng, J., Hong, Y., Lv, C., Zhao, D., Lin, J., et al. (2017). A novel colloidal gold immunochromatography assay strip for the diagnosis of schistosomiasis japonica in domestic animals. Infect. Dis. Poverty 6 (1), 84. doi:10.1186/s40249-017-0297-z
Yang, L., Geng, Y., Li, H., Zhang, Y., You, J., and Chang, Y. (2009a). Enhancement the oral bioavailability of praziquantel by incorporation into solid lipid nanoparticles. Pharmazie 64 (2), 86–89.
Yang, M., Kostov, Y., Bruck, H. A., and Rasooly, A. (2009b). Gold nanoparticle-based enhanced chemiluminescence immunosensor for detection of Staphylococcal Enterotoxin B (SEB) in food. Int. J. food Microbiol. 133 (3), 265–271. doi:10.1016/j.ijfoodmicro.2009.05.029
Zadeh Mehrizi, T., Shafiee Ardestani, M., Haji Molla Hoseini, M., Khamesipour, A., Mosaffa, N., and Ramezani, A. (2018). Novel nanosized chitosan-betulinic acid against resistant leishmania major and first clinical observation of such parasite in kidney. Sci. Rep. 8 (1), 11759. doi:10.1038/s41598-018-30103-7
Zhang, X.-F., Liu, Z.-G., Shen, W., and Gurunathan, S. (2016). Silver nanoparticles: Synthesis, characterization, properties, applications, and therapeutic approaches. Int. J. Mol. Sci. 17 (9), 1534. doi:10.3390/ijms17091534
Zhang, Y., Wang, J., Bai, X., Jiang, T., Zhang, Q., and Wang, S. (2012). Mesoporous silica nanoparticles for increasing the oral bioavailability and permeation of poorly water soluble drugs. Mol. Pharm. 9 (3), 505–513. doi:10.1021/mp200287c
Zhao, L., Seth, A., Wibowo, N., Zhao, C. X., Mitter, N., Yu, C., et al. (2014). Nanoparticle vaccines. Vaccine 32 (3), 327–337. doi:10.1016/j.vaccine.2013.11.069
Zoghroban, H. S., El-Kowrany, S. I., Aboul Asaad, I. A., El Maghraby, G. M., El-Nouby, K. A., and Abd Elazeem, M. A. (2019). Niosomes for enhanced activity of praziquantel against Schistosoma mansoni: In vivo and in vitro evaluation. Parasitol. Res. 118 (1), 219–234. doi:10.1007/s00436-018-6132-z
AChE Acetylcholinesterase
AgNPs Silver nanoparticles
AuNPs Gold nanoparticles
BN Boron nitride
BNNSs Boron nitride nano spheres
Cur-GNPs Curcumin loaded gold nanocapsules
DNA Deoxyribonucleic acid
CTAB Cetyltrimethylammonium bromide
DDS Drug delivery system
ED95 Effective dose 95
ENMs Engineered nanomaterial’s
GICA Colloidal gold immunochromatography assay
Lip.PZQ Liposomal-Praziquantel
LNCs Lipid nanocapsules
LOXA Liposomes entrapped oxamniquine
nAChR Nicotinic type of acetylcholine receptor
NMs Nanomaterials
NPs Nanoparticles
M-PZQ Market praziquantel
MFS-LNC Miltefosine lipid nanocapusles
MSNs Mesoporous silica nanoparticles
PZQ Praziquantel
PZQ-Si Praziquantel-mesoporous silica
PZQ-NLC2 Praziquantel-Nanostructured lipid carriers 2
PZQ-NLC4 Praziquantel- Nanostructured lipid carriers
ROS Reactive oxygen species
S Schistosoma
Se-NPs Selenium-nanoparticles
SGTP1 Schistosome glucose transporter 1
SGTP4 Schistosome glucose transporter 4
SLNs Solid nanoparticles
SLN-PZQ Solid nanoparticles praziquantel
SWAP Soluble worm antigenic preparation
VL Visceral leishmaniasis
WHO World health organization
ZnO-NPs Zinc oxide nanoparticles
Keywords: schistosomiasis, praziquantel, nanotechnology, drug delivery system, liposome, nanoparticles, diagnosis
Citation: Qadeer A, Ullah H, Sohail M, Safi SZ, Rahim A, Saleh TA, Arbab S, Slama P and Horky P (2022) Potential application of nanotechnology in the treatment, diagnosis, and prevention of schistosomiasis. Front. Bioeng. Biotechnol. 10:1013354. doi: 10.3389/fbioe.2022.1013354
Received: 06 August 2022; Accepted: 30 November 2022;
Published: 09 December 2022.
Edited by:
Wenyue Gao, Shandong University, ChinaReviewed by:
Giovina Ruberti, Institute of Biochemistry and Cell Biology, ItalyCopyright © 2022 Qadeer, Ullah, Sohail, Safi, Rahim, Saleh, Arbab, Slama and Horky. This is an open-access article distributed under the terms of the Creative Commons Attribution License (CC BY). The use, distribution or reproduction in other forums is permitted, provided the original author(s) and the copyright owner(s) are credited and that the original publication in this journal is cited, in accordance with accepted academic practice. No use, distribution or reproduction is permitted which does not comply with these terms.
*Correspondence: Abdur Rahim, cmFoaW1raGFuNTMzQGdtYWlsLmNvbQ==; Petr Slama, cGV0ci5zbGFtYUBtZW5kZWx1LmN6; Pavel Horky, cGF2ZWwuaG9ya3lAbWVuZGVsdS5jeg==
Disclaimer: All claims expressed in this article are solely those of the authors and do not necessarily represent those of their affiliated organizations, or those of the publisher, the editors and the reviewers. Any product that may be evaluated in this article or claim that may be made by its manufacturer is not guaranteed or endorsed by the publisher.
Research integrity at Frontiers
Learn more about the work of our research integrity team to safeguard the quality of each article we publish.