- 1State Key Laboratory of Subtropical Silviculture, Zhejiang A&F University, Hangzhou, Zhejiang, China
- 2The Engineering Research Institute of Agriculture and Forestry, Ludong University, Yantai, Shandong, China
Introduction
Compared to seed propagation, clonal propagation is a simpler, faster, and more efficient method to rapidly expand millions of cuttings from elite germplasms (Gonin et al., 2019; Solgi et al., 2022). Adventitious roots (AR) formed from above-ground organs such as stems and leaves are crucial for clonal propagation, which is mainly controlled by the balance of endogenous and exogenous hormones (Lakehal and Bellini, 2019). Therefore, understanding the mechanisms of AR formation in woody species is important for large-scale vegetative propagation of economically and horticulturally important tree species.
Due to the recalcitrance of AR formation in many tree species, the application of exogenous hormones becomes a major approach for optimizing clonal propagation (Legué et al., 2014). Here, we focused on woody species and compared the selection and dosages of exogenous hormones that promote AR formation in cuttings or tissue culture. In addition, we proposed the opinion of promoting AR formation by balancing endogenous and exogenous hormones, thereby accelerating the tree breeding process.
Adventitious root formation is controlled by endogenous hormonal balance
Four stages are included in the AR formation process (Figure 1): activation of competent cells, cell cycle reactivation, AR primordium formation, and AR outgrowth (Legué et al., 2014). Previous studies have suggested that the formation of AR is controlled by multiple endogenous and exogenous factors (Gonin et al., 2019). Among these, phytohormones play an important role (Pacurar et al., 2014), and auxin seems to be the master regulator controlling AR formation, as it responds to rooting-competent tissue, plays a decisive role in cell fate, and activates signaling regulatory networks (Druege et al., 2016).
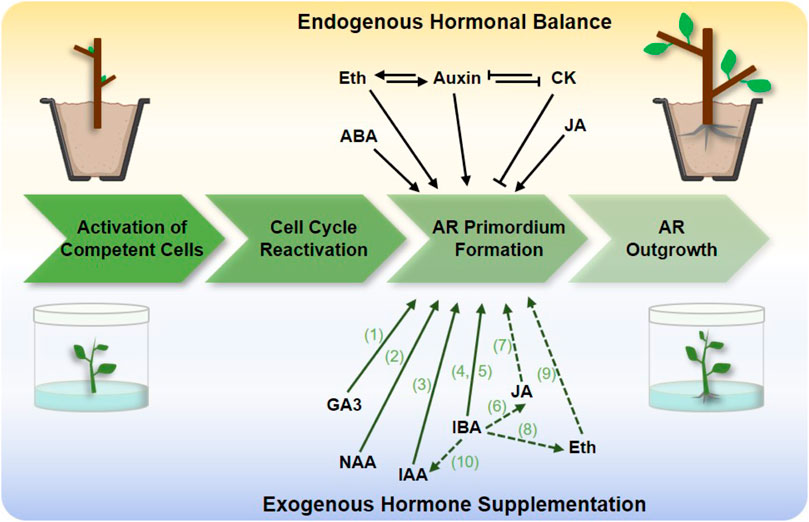
FIGURE 1. Hormones affecting adventitious root (AR) formation in clonal propagation by cuttings and tissue culture of a woody plant. The top panel is endogenous factors that directly induce AR primordium formation, the lower panel represents the effects of exogenous hormone supplementation for rooting. The phytohormones include jasmonic acid (JA), ethylene (Eth), cytokinins (CKs), abscisic acid (ABA), indole-3-butyric acid (IBA), indole acetic acid (IAA), 1-naphthalene acetic acid (NAA), gibberellin 3 (GA3). The dash lines represent the endogenous hormone response to exogenous hormone application. Green letters represent references 1–10: 1) Ford et al. (2002); 2) de Almeida et al. (2015); 3) de Almeida et al. (2015); 4) Fett-Neto et al. (2001); 5) Harfouche et al. (2007); 6) Liu et al. (2021); 7) Liu et al. (2021); 8) Bai et al. (2020); 9) Kilkenny et al. (2012); 10) Munir et al. (2021). The arrows and “T” at the end of lines represent positive and negative regulation, respectively.
For changes of endogenous hormones in AR formation in woody species, multiple experimental evidences confirmed that the induction of auxin-related processes during AR formation in black walnut (Juglans nigra L.) (Stevens et al., 2018), black locust (Robinia pseudoacacia L.) (Uddin et al., 2022), and Populus tremula (Vaičiukynė et al., 2019), which may be related to auxin-promoted cell wall loosening and stretching (Wei et al., 2019). Furthermore, the difference between easy-to-root and difficult-to-root genotypes is attributed to changes in the concentration of inactive forms of auxin conjugates (Gonin et al., 2019). Moreover, there is a complex regulation, balance, and signaling cross-talk between auxin and other phytohormones (Figure 1). For example, ethylene (Eth) positively regulates AR formation through modulating auxin transport in tomato (Negi et al., 2010), and cytokinin interacts with Eth and auxin pathways to antagonize AR development in poplar (Ramírez-Carvajal et al., 2009). Besides that, other hormonal signaling pathways are also known to affect rooting independent of auxin. For example, abscisic acid (ABA) accumulation is found in AR formation in birch (Betula pendula) B1 genotype 6-fold higher than in non-rooting birch B2 genotype (Vaičiukynė et al., 2019); jasmonic acid (JA) content was accumulated after cutting injury to beneficial for rooting in Platycladus orientalis via activating the regeneration of stem cells (Liu et al., 2021). Therefore, it is necessary to maintain the endogenous hormone balance in rooting during the clonal propagation process.
Exogenous hormones supplementation promotes AR formation
For perennial woody plants, stem cutting and tissue culture are the most commonly used clonal propagation techniques (Winkelmann, 2013). According to the changes in the endogenous hormone balance in rooting, the application of exogenous hormones is the most effective means to promote AR formation (Figure 1). For tissue culture propagation, rooting of microshoots was achieved in vitro in the presence of exogenous hormones supplementation and was also successfully grown in a rooting mixture of peat and perlite (Figure 1).
As a central player in AR formation, natural auxins and their synthetic analogs are not only the most powerful exogenous stimulators but also used empirically for rooting cuttings in different species (Legué et al., 2014). The exogenous supplementation of cuttings with the auxin analog indole-3-butyric acid (IBA) can strongly promote AR induction in woody species such as chestnuts (Castanea) (Vielba et al., 2020), eucalyptus (Eucalyptus globulus) (Fett-Neto et al., 2001; Vilasboa et al., 2019), and teak (Tectona grandis) (Azamal and Sayyada, 2012) (Table 1). In C. sativa, endogenous IAA content increased with IBA treatment (Vielba et al., 2020), suggesting that IBA treatment affects AR development by modulating endogenous auxin level. In addition, GA3 pre-treatment of cherry (Prunus avium) stock resulted in the AR formation of cuttings increased (Ford et al., 2002). Therefore, the application of appropriate exogenous hormones has a significant effect on the rooting of cuttings of different species.
Application of exogenous auxin in cutting and tissue culture propagation of woody species
Cutting and tissue culture offer prospects for faster multiplication of elite trees (Winkelmann, 2013). Unfortunately, many species, such as cashew (Anacardium occidentale L.) and chestnut, are highly recalcitrant to in vitro culture and clonal propagation as the astrict to form AR (Camille et al., 2021). In order to overcome the difficulty in rooting, the basal part of cuttings can usually be treated with high concentrations of auxin for root production and then plant for cutting regeneration, or the stem segment explants are cultured in Murashige and Skoog (MS) medium supplemented with various concentrations of auxin or its analogs, such as indole acetic acid (IAA), IBA, and 1-naphthalene acetic acid (NAA) (Winkelmann, 2013). Transcriptomic profiles of NAA- or IBA-induced AR formation indicated that exogenous NAA and IBA stimulated multiple pathways including phytohormone signal transduction and metabolic pathways to promote rooting in black locust tissue culture (Munir et al., 2021) and nodal cuttings of tea (Camellia sinensis L.) (Wei et al., 2014), respectively. This suggests that the promotion of AR by exogenous auxin may involve other hormones or metabolic signals.
In cutting, the dipping method of auxin application is commonly used to induce AR formation, which means a short pulse of a high concentration of the hormone enters the plant tissues through the cut surface of cuttings and will eventually enter the cells by pH trapping or the activity of influx carrier proteins. Shoot cuttings of poplar grown in vitro in the presence of IAA inhibitors caused complete inhibition of rooting (Bellamine et al., 1998). In contrast, IBA- or NAA-treatment induced root primordia in white poplar (Populus alba) cuttings and increased the number of AR per cutting (Harfouche et al., 2007). Moreover, Browne et al. (1997) compared the rooting frequency of jack pine (Pinus banksiana) cuttings of different tree ages (3, 7, and 12 years) and found that NAA treatment generally increased rooting by 2-3 fold compared to controls. In apple (Malus domestica Borkh.) propagation, the rooting rate in cutting is significantly higher after treatment under 3 g/L IBA with 50 mM H2O2 since H2O2 significantly enhances the effect of IBA on rooting (Xiao et al., 2014), or only with 3 g/L IBA (Li X et al., 2021) for 1 min and then inserted into fine sand and incubated in a mist solar greenhouse, and study has demonstrated that IBA stimulates the production of Eth to stimulate AR formation in apple rootstock propagation (Bai et al., 2020; Devi et al., 2021). Olive is one of the hard-to-rooting plants, the hardwood cuttings of olive (Olea europaea ‘Manzanilla') treated under 3 g/L IBA showed a 1.8-fold increase in rooting rate (Khajehpour et al., 2014). The basal of cherry cuttings dip in 1.25 g/L IBA and then plant in pots containing peat significantly increases the number of AR (Ford et al., 2002). The studies described indicate that IBA and NAA as commonly used exogenous auxin in the propagation of forest tree cuttings promote AR formation by the dipping method.
During the root regeneration from tree microshoots, exogenous application of NAA or IBA promotes rooting mainly in two ways: prolonged incubation with low concentrations in the medium or transient dipping. For the dipping method, the effect of pulse treatment of 50 mg/L IBA on in vitro propagated shoots for 48 h and then transferred to MS medium provided a maximum percentage of root induction for sandalwood (Santalum album) (Bhargava et al., 2018). Cuttings were treated with concentrations of 9 g/L IBA for 10 s and then transferred to MS medium for AR inducing in chestnut propagation. Moreover, effective root regeneration of eucalyptus microshoots is obtained by a 4-days exposure to 10 mg/L IBA during the AR induction step (Fett-Neto et al., 2001). The previous study shows that plantlets obtained by culturing in media with exogenous adjuvants are healthier compared to transient immersion (Vielba et al., 2019). Therefore, all of the shoots of neem (Azadirachta indica) formed AR under MS medium with 1 mg/L IBA supplementation (Quraishi et al., 2004), and the highest percentage of AR formation was cultured with 0.1 mg/L IBA in the medium of cashew propagation (Camille et al., 2021). Exogenous application of 0.3 mg/L NAA into MS medium stimulated AR formation in black locust propagation (Munir et al., 2021). Based on the above, the application of exogenous IBA or NAA is essential for ex vitro rooting of tree species in which cuttings or tissue culture is the main propagation method.
Poplar is not only an important economic crop but also a woody model species. It comprises about 30 species with huge varieties in rooting ability, which is related to their sensitivity to exogenous hormones (Luo et al., 2021). Although several poplar species are easy-to-root, exogenous hormone application can accelerate root initiation and promote root quality in hybrid poplars. For example, 0.05 mg/L IBA with 0.02 mg/L NAA is used to promote root initiation in hybrid poplar ‘84K' (P. alba × P. glandulosa) (Wen et al., 2022), while 0.3 mg/L IBA with 0.5 mg/L NAA are required in hybrid poplar (Populus × euramericana ‘Neva') rooting induction medium (Liu et al., 2016). In addition, exogenous IAA impacts differently on gene expression modifications in cuttings of different species of Eucalyptus easy- and difficult-to-root, e.g., IAA improves root number and length in E. grandis while with no significant effect on these parameters being observed in E. globulus (de Almeida et al., 2015). Together, trees with interspecific variations require different kinds and appropriate contents of auxin to promote AR formation in clonally propagated species.
Dose-effect relationships of exogenous auxins in AR formation
The proper concentrations of plant growth regulators are important because excessive auxin concentrations may inhibit AR initiation (Pant et al., 2023), suggesting a dose-effect of exogenous hormones on AR formation. For instance, 1 mg/L IBA has a less promoting effect on AR formation than 0.1 mg/L IBA with grape (Vitis sp.) cuttings (Chang et al., 2022). In addition, 0.1 mg/L and 10 mg/L NAA promote and inhibit AR formation in apple cuttings, respectively (Li K et al., 2021). Conversely, increasing auxin levels correlated positively with rooting success using ‘M116' apple clonal rootstock (Patial et al., 2021), and Eucalyptus (Eucalyptus pellita × E. grandis) cuttings formed significantly more adventitious roots when the cuttings dipped with 8 g/L IBA than that formed in 3 g/L IBA (Kilkenny et al., 2012). Therefore, mastering the optimal concentration of IBA or NAA application in the clonal propagation of diverse woody plants can improve the rooting rate and achieve efficient in vitro multiplication.
Future perspective
Adventitious rooting is indispensable for the vegetative propagation of forestry and horticultural plants. A good rooting system is necessary for plants to adapt to various environments and increase yields, as it can absorb more nutrients for the growth of the above-ground parts. Therefore, advances in the knowledge of AR formation will pave the way for optimizing clonal propagation in woody species. Internal and external factors impact AR formation, of which, auxin as the master hormone regulator seems to be the most important and central one. Despite advances in exogenous hormone selection and concentration over the past few decades, the response of inter-species variation in woody plants to different hormones and their impact on AR formation remain unclear. Therefore, the mechanism of auxin and other hormones in AR formation in diverse woody plants still needs to be analyzed, in order to improve the efficiency of clonal propagation by applying exogenous hormones.
Author contributions
JZ conceived the study and edited the manuscript. YZ drafted the manuscript. YC, CJ, M-ZL, and JZ revised the manuscript. All authors contributed to the article and approved the final version.
Funding
This work was supported by the Key Scientific and Technological Grant of Zhejiang for Breeding New Agricultural Varieties (2021C02070-1), the National Science Foundation of Zhejiang Province for Distinguished Young Scholars (LR22C160001), the Zhejiang A&F University Research and Development Fund Talent Startup Project (2018FR013, 2021LFR013), and the National Key Research and Development Program of China (2021YFD2200205).
Conflict of interest
The authors declare that the research was conducted in the absence of any commercial or financial relationships that could be construed as a potential conflict of interest.
Publisher’s note
All claims expressed in this article are solely those of the authors and do not necessarily represent those of their affiliated organizations, or those of the publisher, the editors and the reviewers. Any product that may be evaluated in this article, or claim that may be made by its manufacturer, is not guaranteed or endorsed by the publisher.
References
Azamal, H., and Sayyada, K. (2012). Role of anthraquinones as a marker of juvenility and maturity in response to adventitious rooting of Tectona grandis. Am. J. Plant Physiology 7, 220–231. doi:10.3923/ajpp.2012.220.231
Bai, T., Dong, Z., Zheng, X., Song, S., Jiao, J., Wang, M., et al. (2020). Auxin and its interaction with ethylene control adventitious root formation and development in Apple rootstock. Front. Plant Sci. 11, 574881. doi:10.3389/fpls.2020.574881
Bellamine, J., Penel, C., Greppin, H., and Gaspar, T. (1998). Confirmation of the role of auxin and calcium in the late phases of adventitious root formation. Plant Growth Regul. 26, 191–194. doi:10.1023/A:1006182801823
Bhargava, P., Ravindra, N., and Singh, G. (2018). A modified and improved protocol development for in vitro clonal propagation of Santalum album L. from internodal explants. Trop. Plant Res. 5, 193–199. doi:10.22271/tpr.2018.v5.i2.024
Browne, R., Davidson, C., Steeves, T., and Dunstan, D. (1997). Effects of ortet age on adventitious rooting of jack pine (Pinus banksiana) long-shoot cuttings. Can. J. For. Res. 27, 91–96. doi:10.1139/x96-160
Camille, K., Laurent, K. K., Martine, B. M., and Irié, Z. B. (2021). Clonal propagation of cashew (Anacardium occidentale L.) by stem cuttings and in vitro adventitious shoots and roots formation. J. Animal Plant Sci. 49, 8845–8855. doi:10.35759/JAnmPlSci.v49-2
Chang, X., Zhang, K., Yuan, Y., Ni, P., Ma, J., Liu, H., et al. (2022). A simple, rapid, and quantifiable system for studying adventitious root formation in Grapevine. Plant Growth Regul., 1–10. doi:10.21203/rs.3.rs-1318845/v1
de Almeida, M. R., de Bastiani, D., Gaeta, M. L., de Araújo Mariath, J. E., de Costa, F., Retallick, J., et al. (2015). Comparative transcriptional analysis provides new insights into the molecular basis of adventitious rooting recalcitrance in Eucalyptus. Plant Sci. 239, 155–165. doi:10.1016/j.plantsci.2015.07.022
Devi, J., Kumar, R., Singh, K., Gehlot, A., Bhushan, S., and Kumar, S. (2021). In vitro adventitious roots: a non-disruptive technology for the production of phytoconstituents on the industrial scale. Crit. Rev. Biotechnol. 41, 564–579. doi:10.1080/07388551.2020.1869690
Druege, U., Franken, P., and Hajirezaei, M. R. (2016). Plant hormone homeostasis, signaling, and function during adventitious root formation in cuttings. Front. Plant Sci. 7, 381. doi:10.3389/fpls.2016.00381
Fett-Neto, A. G., Fett, J. P., Veira Goulart, L. W., Pasquali, G., Termignoni, R. R., and Ferreira, A. G. (2001). Distinct effects of auxin and light on adventitious root development in Eucalyptus saligna and Eucalyptus globulus. Tree Physiol. 21, 457–464. doi:10.1093/treephys/21.7.457
Ford, Y.-Y., Taylor, J., Blake, P., and Marks, T. (2002). Gibberellin A3 stimulates adventitious rooting of cuttings from cherry (Prunus avium). Plant Growth Regul. 37, 127–133. doi:10.1023/A:1020584627919
Gonin, M., Bergougnoux, V., Nguyen, T. D., Gantet, P., and Champion, A. (2019). What makes adventitious roots? Plants (Basel) 8, 240. doi:10.3390/plants8070240
Harfouche, A., Baoune, N., and Merazga, H. (2007). Main and interaction effects of factors on softwood cutting of white poplar (Populus alba L.). Silvae Genet. 56, 287–294. doi:10.1515/sg-2007-0041
Khajehpour, G., Jam'eizadeh, V., and Khajehpour, N. (2014). Effect of different concentrations of IBA (indulebutyric acid) hormone and cutting season on the rooting of the cuttings of olive (Olea europaea Var Manzanilla). Int. J. Adv. Biol. Biomed. Res. 2 (12), 2920–2924. doi:10.17660/actahortic.2011.924.12
Kilkenny, A. J., Wallace, H. M., Walton, D. A., Adkins, M. F., and Trueman, S. J. (2012). Improved root formation in eucalypt cuttings following combined auxin and anti-ethylene treatments. J. Plant Sci. 7, 138–153. doi:10.3923/jps.2012.138.153
Lakehal, A., and Bellini, C. (2019). Control of adventitious root formation: insights into synergistic and antagonistic hormonal interactions. Physiol. Plant. 165 (1), 90–100. doi:10.1111/ppl.12823
Legué, V., Rigal, A., and Bhalerao, R. P. (2014). Adventitious root formation in tree species: involvement of transcription factors. Physiol. Plant. 151, 192–198. doi:10.1111/ppl.12197
Li, K., Wei, Y.-H., Wang, R.-H., Mao, J.-P., Tian, H.-Y., Chen, S.-Y., et al. (2021). Mdm-MIR393b-mediated adventitious root formation by targeted regulation of MdTIR1A expression and weakened sensitivity to auxin in apple rootstock. Plant Sci. 308, 110909. doi:10.1016/j.plantsci.2021.110909
Li, X., Shen, F., Xu, X., Zheng, Q., Wang, Y., Wu, T., et al. (2021). An HD-ZIP transcription factor, MxHB13, integrates auxin-regulated and juvenility-determined control of adventitious rooting in Malus xiaojinensis. Plant J. 107, 1663–1680. doi:10.1111/tpj.15406
Liu, D., Zhang, J., Dong, Y., Zhang, X., Yang, M., and Gao, B. (2016). Genetic transformation and expression of Cry1Ac–Cry3A–NTHK1 genes in Populus × euramericana ‘neva'. Acta Physiol. Plant. 38, 177–211. doi:10.1007/s11738-016-2195-6
Liu, G., Zhao, J., Liao, T., Wang, Y., Guo, L., Yao, Y., et al. (2021). Histological dissection of cutting-inducible adventitious rooting in Platycladus orientalis reveals developmental endogenous hormonal homeostasis. Indu. Crop. Prod. 170, 113817. doi:10.1016/j.indcrop.2021.113817
Luo, J., Nvsvrot, T., and Wang, N. (2021). Comparative transcriptomic analysis uncovers conserved pathways involved in adventitious root formation in poplar. Physiol. Mol. Biol. Plants 27, 1903–1918. doi:10.1007/s12298-021-01054-7
Munir, M. Z., Ud Din, S., Imran, M., Zhang, Z., Pervaiz, T., Han, C., et al. (2021). Transcriptomic and anatomic profiling reveal etiolation promotes adventitious rooting by exogenous application of 1-Naphthalene Acetic Acid in Robinia pseudoacacia L. Forests 12, 789. doi:10.3390/f12060789
Negi, S., Sukumar, P., Liu, X., Cohen, J. D., and Muday, G. K. (2010). Genetic dissection of the role of ethylene in regulating auxin-dependent lateral and adventitious root formation in tomato. Plant J. 61 (1), 3–15. doi:10.1111/j.1365-313X.2009.04027.x
Pacurar, D. I., Perrone, I., and Bellini, C. (2014). Auxin is a central player in the hormone cross-talks that control adventitious rooting. Physiol. Plant. 151, 83–96. doi:10.1111/ppl.12171
Pant, M., Bhandari, A., and Husen, A. (2023). “Adventitious root formation and clonal propagation of forest-based tree species,” in Environmental, physiological and chemical controls of adventitious rooting in cuttings (Cambridge: Academic Press), 471–490.
Patial, S., Chandel, J., Sharma, N., and Verma, P. (2021). Influence of auxin on rooting in hardwood cuttings of apple (Malus × domestica Borkh.) clonal rootstock ‘M 116' under malus domestica mist chamber conditions. Indian J. Ecol. 48, 429–433.
Quraishi, A., Koche, V., Sharma, P., and Mishra, S. (2004). In vitro clonal propagation of neem (Azadirachta indica). Plant Cell, Tissue Organ Cult. 78, 281–284. doi:10.1023/B:TICU.0000025647.58548.3d
Ramírez-Carvajal, G. A., Morse, A. M., Dervinis, C., and Davis, J. M. (2009). The cytokinin type-B response regulator PtRR13 is a negative regulator of adventitious root development in Populus. Plant Physiol. 150 (2), 759–771. doi:10.1104/pp.109.137505
Ribeiro, Y. R. D. S., Aragão, V. P. M., Sousa, K. R. D., Macedo, A. F., Floh, E. I. S., Silveira, V., et al. (2022). Involvement of differentially accumulated proteins and endogenous auxin in adventitious root formation in micropropagated shoot cuttings of Cedrela fissilis Vellozo (Meliaceae). Plant Cell, Tissue Organ Cult. 148 (1), 119–135. doi:10.1007/s11240-021-02171-7
Solgi, M., Taghizadeh, M., and Bagheri, H. (2022). Response of black mulberry onto white mulberry rootstock to stenting (cutting-grafting) techniques and IBA concentrations. Ornam. Hortic. 28, 78–84. doi:10.1590/2447-536X.v28i1.2413
Stevens, M. E., Woeste, K. E., and Pijut, P. M. (2018). Localized gene expression changes during adventitious root formation in black walnut (Juglans nigra L.). Tree Physiol. 38, 877–894. doi:10.1093/treephys/tpx175
Uddin, S., Munir, M. Z., Gull, S., Khan, A. H., Khan, A., Khan, D., et al. (2022). Transcriptome profiling reveals role of microRNAs and their targeted genes during adventitious root formation in dark-pretreated micro-shoot cuttings of tetraploid Robinia pseudoacacia L. Genes (Basel) 13, 441. doi:10.3390/genes13030441
Vaičiukynė, M., Žiauka, J., Žūkienė, R., Vertelkaitė, L., and Kuusienė, S. (2019). Abscisic acid promotes root system development in birch tissue culture: a comparison to aspen culture and conventional rooting-related growth regulators. Physiol. Plant. 165, 114–122. doi:10.1111/ppl.12860
Vielba, J. M., Vidal, N., José, M. C. S., Rico, S., and Sánchez, C. (2020). Recent advances in adventitious root formation in chestnut. Plants (Basel) 9, 1543. doi:10.3390/plants9111543
Vielba, J. M., Vidal, N., Ricci, A., Castro, R., Sanchez, C., Carmen San-Jose, M., et al. (2019). Effects of auxin and urea derivatives on adventitious rooting in chestnut and oak microshoots. Isr. J. Plant Sci. 67, 52–68. doi:10.1163/22238980-20191113
Vilasboa, J., Da Costa, C. T., and Fett-Neto, A. G. (2019). Rooting of eucalypt cuttings as a problem-solving oriented model in plant biology. Prog. Biophysics Mol. Biol. 146, 85–97. doi:10.1016/j.pbiomolbio.2018.12.007
Wei, K., Ruan, L., Wang, L., and Cheng, H. (2019). Auxin-induced adventitious root formation in nodal cuttings of Camellia sinensis. Int. J. Mol. Sci. 20, 4817. doi:10.3390/ijms20194817
Wei, K., Wang, L.-Y., Wu, L.-Y., Zhang, C.-C., Li, H.-L., Tan, L.-Q., et al. (2014). Transcriptome analysis of indole-3-butyric acid-induced adventitious root formation in nodal cuttings of Camellia sinensis (L.). PLoS One 9, e107201. doi:10.1371/journal.pone.0107201
Wen, S. S., Ge, X. L., Wang, R., Yang, H. F., Bai, Y. E., Guo, Y. H., et al. (2022). An efficient agrobacterium-mediated transformation method for hybrid poplar 84K (Populus alba × P. glandulosa) using calli as explants. Int. J. Mol. Sci. 23, 2216. doi:10.3390/ijms23042216
Winkelmann, T. (2013). Recent advances in propagation of woody plants. Acta Hortic. 990, 375–381. doi:10.17660/actahortic.2013.990.47
Keywords: exogenous hormone, auxin, adventitious root formation, woody plants, clonal propagation
Citation: Zhao Y, Chen Y, Jiang C, Lu M-Z and Zhang J (2022) Exogenous hormones supplementation improve adventitious root formation in woody plants. Front. Bioeng. Biotechnol. 10:1009531. doi: 10.3389/fbioe.2022.1009531
Received: 02 August 2022; Accepted: 19 August 2022;
Published: 13 September 2022.
Edited by:
Guanjun Liu, Northeast Forestry University, ChinaReviewed by:
Jing Hou, Nanjing Forestry University, ChinaMingyang Quan, Beijing Forestry University, China
Yikai Zhang, China National Rice Research Institute (CAAS), China
Copyright © 2022 Zhao, Chen, Jiang, Lu and Zhang. This is an open-access article distributed under the terms of the Creative Commons Attribution License (CC BY). The use, distribution or reproduction in other forums is permitted, provided the original author(s) and the copyright owner(s) are credited and that the original publication in this journal is cited, in accordance with accepted academic practice. No use, distribution or reproduction is permitted which does not comply with these terms.
*Correspondence: Jin Zhang, emhhbmdqQHphZnUuZWR1LmNu, b3JjaWQub3JnLzAwMDAtMDAwMi04Mzk3LTUwNzg=
†These authors have contributed equally to this work
‡ORCID: Jin Zhang, http://orcid.org/0000-0002-8397-5078