- 1Department of Sports Medicine, The First Affiliated Hospital of Shenzhen University, Shenzhen Second People’s Hospital, Shenzhen, China
- 2Clinical Medical College, Anhui Medical University, Hefei, China
- 3Guangdong Key Laboratory of Tissue Engineering, The First Affiliated Hospital of Shenzhen University, Shenzhen Second People’s Hospital, Shenzhen, China
- 4Clinical Medical College, Guangxi University of Chinese Medicine, Nanning, China
Articular cartilage lesion is a common disease to be treated by arthroscopic surgery. It will eventually progress to osteoarthritis without proper management, which can affect patients’ work and daily life seriously. Although mechanical debridement and laser have been used clinically for its treatment, due to their respective drawbacks, radiofrequency has drawn increasing attention from clinicians as a new technique with more advantages. However, the safety and efficacy of radiofrequency have also been questioned. In this article, the scope of application of radiofrequency was reviewed following an introduction of its development history and mechanism, and the methods to ensure the safety and effectiveness of radiofrequency through power and temperature control were summarized.
Introduction
Articular cartilage (AC), a kind of hyaline cartilage covering the articular surface, is a viscoelastic connective tissue composed of chondrocytes and the extracellular matrix (ECM), while ECM is mainly composed of collagen, aggregated proteoglycan and water (Maly et al., 2021). In normal cartilage, the interaction between water, collagen and proteoglycan-rich matrix constitutes the compressive properties and tensile strength of AC. In addition, AC also has excellent wear resistance and lubrication ability (Dutcheshen et al., 2012; Deng et al., 2018).
While participation in sports and daily physical activities, acute impact or chronic injury accumulated by repeated impact of the joint may damage the articular surface and cause AC lesions (Zhang et al., 2019). For example, an earlier study reported full-thickness focal cartilage defects in more than 1/3 of 931 athletes (Flanigan et al., 2010). The cartilage defects play a vital role in cartilage degeneration. Without effective treatment, such degeneration will eventually lead to osteoarthritis (OA) that can affect the patients’ work and daily life seriously and represent a significant health and economic burden on society (Mahmoudian et al., 2021). In a recent epidemiological study, OA occurred in 54.4 million (22.7%) adults, and 43.5% of the patients reported negative impact on their life due to limitation of motion (Barbour et al., 2017). Unfortunately, cartilage has a limited self-repair ability, due to lack of blood vessels, nerves, and lymph tissue. Therefore, the treatment of articular cartilage lesions is still a great challenge in clinical practice (Buckwalter and Mankin, 1998; Ganguly et al., 2010; Huang et al., 2021; Zhou et al., 2021).
Chondroplasty is an effective surgical option for the treatment of AC lesions (Barber and Iwasko, 2006; Gowd et al., 2019). This technique debrides the fibroblast cartilage in the joint to form a smooth and stable articular surface to avoid further degeneration caused by delamination, fragmentation and fibrillation of the injured AC. Chondroplasty includes mechanical debridement, laser, and radiofrequency chondroplasty (Khan and Dillingham, 2002; Rocco et al., 2016). Traditionally, mechanical debridement has been used to debride and smooth the damaged articular surface, but this method usually removes adjacent normal cartilage while treating focal lesions. In addition, it cannot completely smooth the surface of cartilage, which will cause fibrillation on the cartilage surface, leading to further degeneration (Turner et al., 1998; Spahn et al., 2016). As a thermal energy technology, laser has also been applied to treat AC lesions, but it has raised a great concern from clinicians due to safety and cost considerations (Shellock and Shields, 2000; Wienecke and Lobenhoffer, 2003; Cook et al., 2004; Ganguly et al., 2010). In view of the respective deficiencies of the two techniques aforementioned, radiofrequency chondroplasty has recently become a new hotspot as a safer and more effective option (Khan and Dillingham, 2002). In this article, the development history, parameter control, biological research, clinical research, indications, and complications of radiofrequency were comprehensively reviewed.
Manuscript Formatting
History of Radiofrequency
The Origin and Development of Radiofrequency
In 1891, d’Arsonval et al. reported that radiofrequency waves could increase the temperature of local tissue when passed through, making this thermal energy feasible to be used for clinical application (D'Arsonval, 1891). At the beginning of the 20th century, Cushing et al. used the bovie electric knife to assist in intra-cranial tumor resection, which was a technique using the thermal energy generated by radiofrequency waves to stanch bleeding and remove abnormal tissues (Cushing and Bovie, 1928). In 1975, radiofrequency was applied to treat chronic pain in unilateral limbs (Pawl, 1975). Subsequently, it has been widely used in neurology, oncology, cardiology and other fields (Pawl, 1975; Dickson and Calderwood, 1980; Huang et al., 1987).
Application of Radiofrequency in Orthopedic Arthroscopy
Due to the numerous side effects of laser chondroplasty, there was an urgent need to find an alternative method for the treatment of AC lesions (Whipple et al., 1985; Miller et al., 1989; Lane et al., 1997). The application of radiofrequency under arthroscopy played a significant role in the development of orthopedic surgery. In 1986, Schosheim et al. applied radiofrequency under arthroscopy for the first time to perform meniscectomy on rabbit knee joints (Schosheim and Caspari, 1986). The authors found that radiofrequency treatment caused less damage to soft tissues. Turner et al. compared 6 histological variables of the cartilage treated by mechanical debridement and radiofrequency, and reported that the radiofrequency group achieved more favorable results in all the variables, indicating a superior performance of radiofrequency to mechanical debridement for AC lesions (Turner et al., 1998). Since then, an increasing number of studies have supported that radiofrequency is an effective treatment for AC lesions (Allen et al., 2006; Uthamanthil et al., 2006; Edwards et al., 2007). In recent years, there has been a proliferation of relevant clinical studies. However, while gaining more experience, there are still many problems and controversies to be addressed.
Mechanism of Radiofrequency
Types and Grades of Cartilage Lesions
Acute impact or chronic injury of the joint may both damage the articular surface, cause cartilage degeneration, and lead to biomechanical and histological changes of the cartilage, such as joint pain, dysfunction and joint effusion (Buckwalter, 2002). Cartilage lesions can be divided into three types depending on the depth of the damage, namely, partial-thickness defects, full-thickness defects and osteochondral defects. In partial- or full-thickness defects, the damage is completely confined to the cartilaginous tissue and does not penetrate the subchondral bone (Nukavarapu and Dorcemus, 2013). A commonly-used method for classifying cartilage lesions was described by Outerbridge et al. (Slattery and Kweon, 2018). According to the classification of cartilage lesions observed under arthroscopy by the improved Outerbridge system, Grade Ⅰ lesions refer to softened cartilage surface only, which usually does not need to be treated; Grade Ⅱ or Ⅲ lesions refer to a partial-thickness defect with fissures of the cartilage with a diameter less than (Ⅱ) or more than (Ⅲ) 0.5 inches in diameter, which can be treated by mechanical debridement and radiofrequency; Grade Ⅳ lesions refer to subchondral bone exposure, which cannot be effectively treated by radiofrequency, mechanical debridement and other interventions, and needs to take further measures, such as autologous chondrocyte implantation, osteochondral allograft transplantation and so on (Osti et al., 2010). However, the Outerbridge grading system does not take into account the depth of the lesion. On the contrary, the classification system of the International Cartilage Repair Society (ICRS) mainly focuses on the lesion depth (Brittberg and Winalski, 2003). The ICRS Grade Ⅰ lesions are only superficial, such as soft indentation or superficial fissures and cracks. The ICRS Grade Ⅱ lesions have extended to less than half of the cartilage depth, while the ICRS Grade Ⅲ lesions have extended to half or more of the cartilage depth but not yet into the subchondral bone. The ICRS Grade Ⅳ lesions are osteochondral lesions. In addition, some other classification systems focus on histopathology, such as the Osteoarthritis Research Society International (OARSI) system (Pritzker et al., 2006). The OARSI system was classified as 1) uneven but intact chondral surface (OARSI Grade Ⅰ), 2) surface discontinuity (OARSI Grade Ⅱ), 3) vertical fissures (OARSI Grade Ⅲ), 4) erosion (OARSI Grade Ⅳ), 5) denudation of the cartilage (OARSI Grade Ⅴ), or 6) deformation and osteophytes formation of the joint (OARSI Grade Ⅵ).
Biomechanical and Histological Changes
Radiofrequency can debride the damaged cartilage surface to create a smooth cartilage surface and improve the mechanical integrity and function of the treated cartilage (Dutcheshen et al., 2012). Through biomechanical modification of the cartilage, the function of the joint can be restored, the degeneration process can be delayed, and the patients’ function and quality of life can be improved. Cook et al. demonstrated that no significant difference in compression stiffness between radiofrequency-treated and untreated AC (Cook et al., 2004). Thus, they believed that radiofrequency had no adverse effect on the biomechanical properties of AC. However, it was obviously not sufficient to judge the biomechanical properties of cartilage only by testing its stiffness. On the other hand, due to the local high temperature caused by the probe and the cartilage defects in the treatment area, the use of radiofrequency might cause secondary damage to the border of the chondromalacic and non-chondromalacic area (Cook et al., 2004). The cartilage defects in the treated area made it impossible to contribute to the bearing capacity of nearby areas. The heat energy would induce the denaturation of collagen, make the collagen shrink, and reduce the immediate and short-term stiffness (Lopez et al., 1998; Edwards et al., 2002a; Uthamanthil et al., 2006). Based on a fiber-reinforced biphasic cartilage model, Dutcheshen et al. inferred that the collagen fibril modulus of the treated cartilage decreased, which might reduce the instantaneous or short-term stiffness (resistance to shear and tension) of AC while increasing the matrix modulus (Dutcheshen et al., 2012). This was beneficial to the restoration of the long-term stiffness (load-bearing capacity) of cartilage.
Laboratory evidence showed that the use of radiofrequency chondroplasty could not only improve mechanical stability but also reduce the release of inflammatory mediators, which might benefit from the reduction of permeability of the cartilage due to the annealing effect of bipolar radiofrequency energy (bRFE) (Uthamanthil et al., 2006; Dutcheshen et al., 2012). Although the decrease in permeability had a potentially positive effect (i.e., it reduced the release of inflammatory mediators), it affected the exchange of nutrients at the same time. Cook et al. pointed out that the matrix metalloproteinase (MMP)-13 immunoreactivity increased after the bRFE therapy, and the long-term increase of the MMP-13 activity in ECM might lead to a negative balance between ECM synthesis and degradation, which would further accelerate the progression of OA (Cook et al., 2004). MMP-1 can cleave native triple-helical collagens at a single bond, whereas MMP-2 plays a non-specific role in the degradation of fibrillar collagens, basement membrane components, and matrix molecules such as fibronectin. Yasura et al. observed that the secretion of MMP-1 and MMP-2 in AC decreased after the use of monopolar radiofrequency energy (mRFE), which was helpful in preventing the further degeneration of AC (Yasura et al., 2006). Enochson et al. revealed that the expressions of interleukin (IL)-6 and IL-8 in the cartilage were up-regulated after the radiofrequency treatment (Enochson et al., 2012). IL-6 played a role in promoting and inhibiting the chondrocyte proliferation. In addition, it had been proven that IL-6 and IL-8 contributed to proliferation of mesenchymal stem cell which would differentiate into chondrocytes (Figure 1).
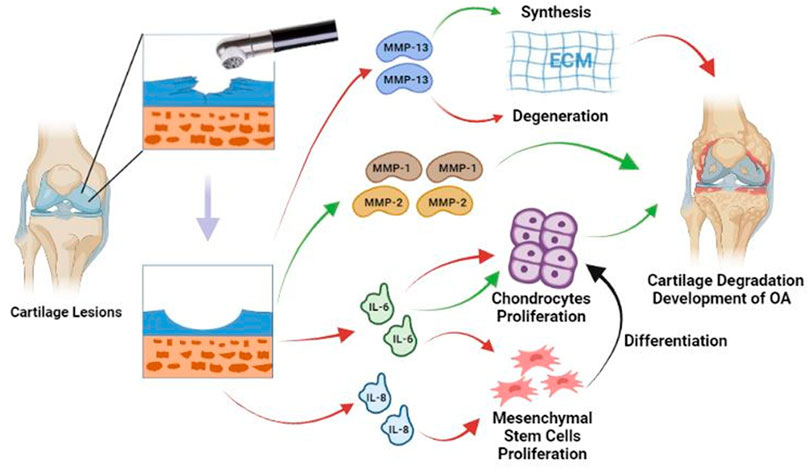
FIGURE 1. Radiofrequency debrides the damaged cartilage surface to create a smooth cartilage surface. After treatment, MMP-13 tissue immunoreactivity increases, which may lead to a negative shift in the balance between synthesis and degradation of the ECM. IL-6 plays a role in promoting and inhibiting the chondrocyte proliferation. IL-6 and IL-8 promote the proliferation of mesenchymal stem cell. Mesenchymal stem cells can differentiate into chondrocytes and enhance cartilage repair. Secretion of MMP-1 and MMP-2 is significantly reduced, which may help prevent further degradation. Red lines = Stimulating; Green lines = Suppressing; Black line = Differentiation.
Monopolar and Bipolar Radiofrequency Energy
Radiofrequency equipment includes mRFE (Figure 2) and bRFE (Figure 3), which differ in work mode and temperature distribution (Khan and Dillingham, 2002; Uribe, 2002).
In mRFE, the current from the box reaches the target tissue through the probe in contact with the tissue and then is derived from the return negative plate connected to the patient. Subsequently, the current goes back to the box to form a complete circuit. Because the target tissue has a higher resistance than the rest of the circuit, heat is generated in the target tissue. The system requires the probe to be in contact with the target tissue during the process of treatment, and the energy is directly transmitted to the target tissue, thereby causing an effect similar to electrical damage (Khan and Dillingham, 2002; Ganguly et al., 2010).
Compared with the working mode of mRFE that generates heat, the mode of bRFE is ablation (Good et al., 2009). The bRFE plasma system is a low-temperature ablation technique, which does not directly act on the tissue, but applies a voltage to the conductive fluid between the electrode and the target tissue to generate a plasma field (Wienecke and Lobenhoffer, 2003; Voloshin et al., 2007). Through the impact of charged particles in the plasma field with the target tissue, the molecular chain of the target tissue is broken, thus playing the role of tissue cutting and removal.
The two types of radiofrequency differ greatly not only in work mode, but also in treatment results. Lu et al. reported that significant chondrocyte death occurred after treatment with both mRFE and bRFE, and the depth of death was higher in the bRFE group than in the mRFE group (Lu et al., 2001; Lu et al., 2002a). Edwards et al. explored the temperature distribution of mRFE and bRFE in different depths of cartilage matrix, and the results showed that bRFE led to a higher temperature and more significant chondrocyte death than mRFE (Edwards et al., 2002a; Edwards et al., 2002b). Caffey et al. studied 5 commercially available radiofrequency instruments in human cartilage under simulated surgical conditions, and the results showed that both mRFE and bRFE caused significant cell death in the cartilage under minimal setting conditions (Caffey et al., 2005).
Many studies have suggested that radiofrequency can lead to remarkable chondrocyte death, especially in bRFE, relative to mRFE. However, the cartilage surface becomes smooth after receiving radiofrequency treatment, providing AC with resistance to wearing and tearing, which can delay the progress of cartilage degeneration. Meanwhile, cartilage has a certain self-repair capability (Caplan et al., 1997). Therefore, whether radiofrequency is suitable for treating cartilage lesions in terms of safety and practicability still requires further in vivo experiments to validate.
Control Factors of Radiofrequency
The effectiveness of the radiofrequency therapy can be affected by several variables, including the choice of treatment device, exposure time, power setting, and temperature control and speed of the lavage fluid flow (Table 1).
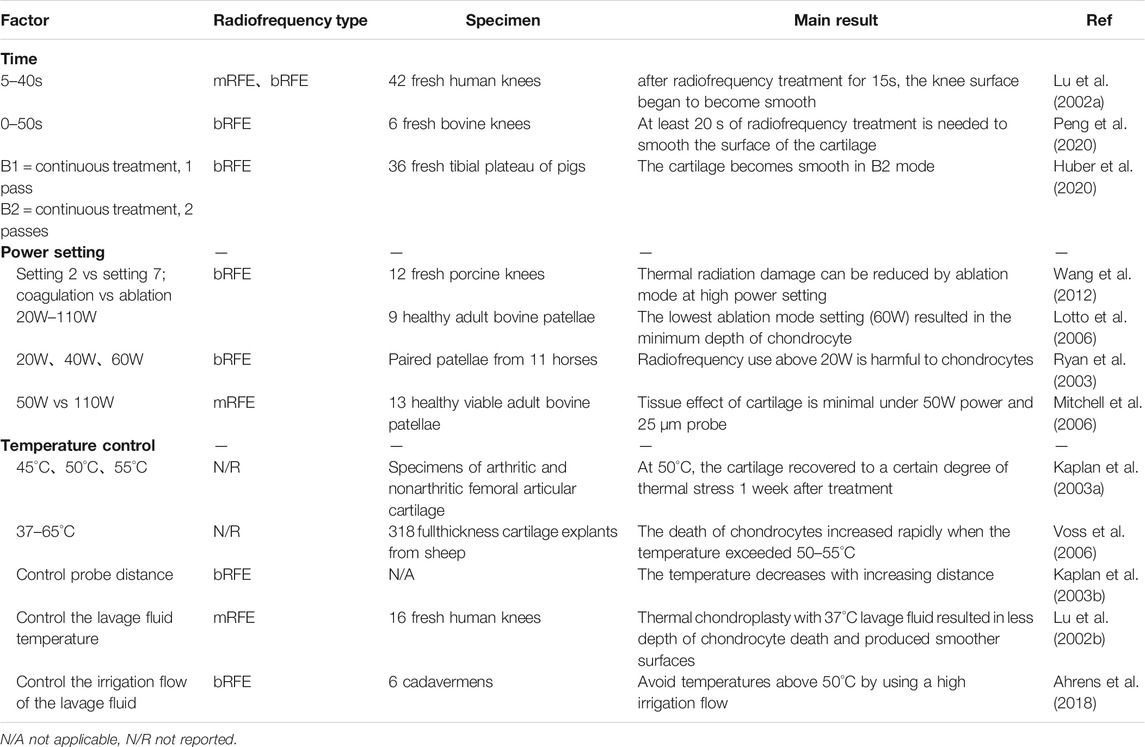
TABLE 1. Influence of radiofrequency time, power setting, temperature control and lavage fluid on articular cartilage.
Time
Clinicians are prone to premature termination of the treatment due to limited arthroscopic magnification when using radiofrequency to treat cartilage lesions, resulting in a less smooth cartilage surface after treatment. Therefore, it is necessary to accurately control the use time of radiofrequency.
Lu et al. performed mRFE and bRFE treatments on fresh osteochondral sections for 5–40s (Lu et al., 2002a). The electron microscope observation showed that the AC surface became smooth with the color gradually turning to yellow after 15 s of radiofrequency treatment in both groups. Excessive treatment time resulted in a significant increase in the depth of chondrocyte death. Similar result was obtained by Peng et al. (Peng et al., 2020). They noted that the glycosaminoglycan content was negatively correlated with exposure time, suggesting that radiofrequency treatment was also negatively correlated with exposure time and had time-dependent damage to the metabolic chondrocyte vitality. Huber et al. compared different modes of radiofrequency therapy. In order to treat a 1 cm2 cartilage defect area, one continuous pattern, at least 17 s, is required to smooth the articular cartilage (Huber et al., 2020). The cartilage surface only appeared to become smooth after two continuous radiofrequency treatments. With this continuous treatment pattern extending for a long time, the chondrocyte death could reach up to 95%.
These results indicate that radiofrequency needs to be used for more than 15 s in order to smooth the AC surface during clinical treatment. On the contrary, the exposure time should be controlled as short as possible to reduce the effect on the chondrocyte vitality. Due to different specimens used and obvious variation in the thickness of cartilage in different studies, it is difficult to reach a consensus on this issue.
Power Setting
Wang et al. showed that high-power settings in the ablation mode could reduce the thermal radiation injury and was therefore more suitable for the treatment of cartilage lesions (Wang et al., 2012). Lotto et al. discovered that a continuous “char-like layer” was observed on the cartilage surface when the power setting was greater than 60W, which significantly reduced the depth of cell death (Lotto et al., 2006). As the power increased, the current increased significantly. A higher electrical current correlated with increased cell death, even though “char-like layer” appeared in all groups with power higher than 60W. Therefore, they concluded that chondrocytes had the minimum death depth when the cartilage surface was treated at 60W. In a study on the safety of radiofrequency chondroplasty, Ryan et al. found that the cell survival rate of the 40W and 60W groups decreased to 81 and 73%, respectively (Ryan et al., 2003). However, the local peak temperature of the articular surface cartilage was lower than 50°C, at which the chondrocyte vitality might be able to restore (Kaplan et al., 2003a; Voss et al., 2006). Mitchell et al. proposed that in addition to power setting, the selection of probe was also an important factor. Compared with the 110W power and 125 μm probe, the 50W power setting and 25 μm probe had the least influence on the tissue (Mitchell et al., 2006).
In general, different types and manufacturers of radiofrequency will lead to different conclusions. In addition, the experiments mentioned above are only in vitro studies or zero-time studies after treatment. Long-term in vivo studies are expected to simulate the actual clinical conditions in the future. Since all the manufacturers have provided guidelines for the use of radiofrequency, clinicians can follow these guidelines in clinical practice.
Temperature Control
It has been reported in previous studies that radiofrequency chondroplasty can cause AC to be subjected to destructive heat stress, which will further lead to chondrocyte death. Therefore, it is necessary to explore whether there is a temperature “safe zone” when using radiofrequency, at which it will not cause the death of chondrocytes. Kaplan et al. placed cartilage specimens in water baths at 45°C, 50°C and 55°C respectively for up to 3 min and evaluated the chondrocyte vitality immediately and 1 week after treatment (Kaplan et al., 2003a). The chondrocyte vitality was restored 1 week after treatment in the 50°C group, but not in the 55°C group. Voss et al. also confirmed that there was a strong relationship between temperature increase and chondrocyte death (Voss et al., 2006). The chondrocytes were more likely to restore their vitality after thermal injury when the temperature was less than 50°C (Mitchell et al., 2006). Therefore, the local temperature should also be properly controlled to ensure the safety of AC while achieving the desired clinical results.
In another study by Kaplan et al., the temperature was controlled by changing the distance between the bRFE probe and the cartilage surface (Kaplan et al., 2003b). Lu et al. noted that the use of higher temperature lavage fluid could shorten the time required to reach the preset temperature at the beginning of treatment, thus prolonging the effective treatment time (Lu et al., 2002b). In order to prevent the radiofrequency temperature from exceeding 50°C, Ahrens et al. used a higher lavage fluid flow in arthroscopic surgery to reduce the temperature (Ahrens et al., 2018). This method had also been mentioned and confirmed by Good et al. (Good et al., 2009).
In clinical practice, attention should be paid to controlling the local temperature of radiofrequency to prevent the environment of cartilage from exceeding 55°C for a long time. The radiofrequency temperature can be controlled by increasing the lavage fluid flow, shortening the working time of radiofrequency, controlling the distance of the radiofrequency probe, and increasing the initial temperature of the lavage fluid.
Arthroscopic Application of Radiofrequency
In the arthroscopic application of radiofrequency for the treatment of cartilage lesions, the scope of application and indications of this technique are worthy of attention. The types of patients included in the related clinical studies and the procedures in which the radiofrequency system was used were presented in Table 2.
Knee Arthroscopy
Partial-thickness cartilage defects can be detected in more than 60% of the cases that received knee arthroscopy, with Outerbridge Grade Ⅲ lesions of the patella being the dominant one (Curl et al., 1997). Voloshin et al. investigated 15 patients who underwent knee arthroscopic radiofrequency chondroplasty (a total of 25 cartilage lesions, including 11 patellofemoral lesions and 14 tibiofemoral lesions, of which 23 were Outerbridge Grade Ⅲ lesions) (Voloshin et al., 2007). At the second-look, significant reductions in the mean extent of the lesions compared with the preoperative status were observed, and partial or complete filling of the cartilage defects in 56% of the patients and continued progression of cartilage degeneration in only 3 cases (12%) were detected. Similar results were reported in another case report by Voloshin et al. (Voloshin et al., 2005). Gharaibeh et al. retrospectively reviewed 824 patients who underwent bRFE for the treatment of cartilage lesions, where the most common lesion involved was the medial femoral condyle (27%), followed by patella (21%) and pulley (9%), with a lesion area ranging 7–820 mm2 (mean 358 mm2) (Gharaibeh et al., 2018). Neither postoperative complications nor reoperation in patients were found to be directly related to the use of bRFE. Barber et al. treated 28 patients with Outerbridge Grade Ⅲ lesions of the femoral condyle (1.5–3.0 cm in diameter) using radiofrequency combined with mechanical debridement (Barber and Iwasko, 2006). Similarly, the follow-up study of Kang et al. included patients with Outerbridge Grade Ⅱ (7 cases) or Ⅲ (8 cases) lesions of the medial or lateral condyle of the femur, who were also treated by radiofrequency combined with mechanical debridement (Kang et al., 2008). The clinical results of these patients were significantly improved compared with their preoperative status (Spahn et al., 2016).
Consistently, in several earlier clinical studies, the patients treated by radiofrequency chondroplasty were also classified as Outerbridge Grade Ⅱ or Ⅲ lesions. Most cartilage lesions were on the medial condyle of the femur, followed by lateral condyle, patella and trochlea, while the lesion area was generally less than 800 mm2. A majority of the patients included under this standard had achieved good clinical results, with a low incidence of complications. The detailed indication for the use of radiofrequency in knee arthroscopy needs further research and more long-term follow-up studies to confirm.
Shoulder Arthroscopy
Radiofrequency has long been used in other arthroscopies as well, and its earliest application was in shoulder arthroscopy to reduce periarticular soft tissue laxity and treat shoulder instability. However, in a review by Solomon et al., it was found that most published studies on the relationship between post-arthroscopic glenohumeral chondrolysis (PAGCL) and surgical factors focused on radiofrequency (Solomon et al., 2009). Around 45% of the patients with PAGCL were treated by radiofrequency during surgery. Hence, the side effects of radiofrequency have discouraged its application in shoulder arthroscopy (McFarland et al., 2002).
Hip Arthroscopy
There are few studies on the use of radiofrequency in the treatment of cartilage lesions in hip arthroscopy, and in the relevant studies, radiofrequency was mostly used in iliopsoas release, ligament debridement, treatment for osteoid osteoma and so on (Suarez-Ahedo et al., 2015). In two case reports on the use of radiofrequency to treat acetabular cartilage lesions, both patients developed chondrolysis within 6 months after surgery (Rehan-Ul-Ha et al., 2010; Más et al., 2015). Although there is no direct evidence indicating the cause of chondrolysis by radiofrequency, it has been mentioned in previous reports that the local high temperature produced by radiofrequency led to the death of chondrocytes and even destruction of the entire cartilage layer. Thus, the authors believed that this could be a side effect of radiofrequency.
Wrist Arthroscopy
With the rapid development of the radiofrequency technology, small radiofrequency probes have been created to be used in the wrist and ankle joints, mainly for “thermal shrinkage” and “joint capsule shrinkage” treatment of small joint lesions (Huber et al., 2013; Han et al., 2014; Huber et al., 2015; Leclercq and Mathoulin, 2016; Giddins et al., 2020). Jang et al. executed radiofrequency shrinkage in 4 patients with scaphoid ligament injury and achieved significant improvement during a follow-up of 4.8 years (Jang et al., 2014). Zhu et al. reported 7 cases of excision of lesions by radiofrequency in ankle arthroscopy with no complications complained during the follow-up (Zhu et al., 2019).
Summary of Radiofrequency Applications in Arthroscopy
Although some laboratory studies had shown that the shoulder temperature could be effectively reduced under the condition of continuous lavage fluid flow, it might still exceed 50°C in a short time interval of limited flow, causing thermal damage to the cartilage (Lu et al., Arthroscopy, 2005, 21, 592–596; Good et al., J Bone Joint Surg Am, 2009, 91, 429–434; Zoric et al., J Bone Joint Surg Am, 2009, 91, 2,448–2,454). For the hip joint, McCormick et al. proposed that 5s-interval pulse irrigation was effective in maintaining the intra-articular temperature below 50°C (McCormick et al., Arthroscopy, 2013, 29, 336–342). For the wrist and ankle joints, the local temperature of cartilage lesions could often exceed 50°C due to a small size and varying cartilage thickness (Huber et al., J Hand Surg Am, 2016, 41, 1,080–1,086). It was also reported that the lavage fluid could not effectively dissipate heat, resulting in PAGCL, chondrolysis, distal radioulnar joint and local skin necrosis (Curtin and Friebe, Orthopedics, 2014, 37, e746–e749). Hence, treatment of cartilage lesions by radiofrequency is rarely seen in other than knee arthroscopy, and the use of “thermal shrinkage” and “joint capsule shrinkage” techniques to ablate the diseased tissue requires particular attention to controlling the local temperature, so as to prevent the occurrence of complications.
Radiofrequency Compared With Other Treatments
Radiofrequency Versus Mechanical Debridement
In 1998, Turner et al. reported that, compared with mechanical debridement, less histological changes and less destruction of cartilage were observed in the treatment with bRFE (Turner et al., 1998). Uthamanthil et al. showed that the postoperative cartilage thickness and stiffness were significantly higher in the mRFE group than in the mechanical debridement group (Uthamanthil et al., 2006). Allen et al. used bRFE and mechanical debridement to treat meniscus injury and cartilage lesions respectively (Allen et al., 2006). The results showed no difference between bRFE and mechanical debridement in terms of the effect on chondrocyte vitality. However, compared with the “tearing” approach of mechanical debridement, radiofrequency could treat cartilage lesions more precisely, create a smoother cartilage surface, and avoid damage to the articular surface.
The earliest prospective clinical study using radiofrequency for the treatment of cartilage lesions was conducted by Owens et al. (Owens et al., 2002). They included 39 patients with Outerbridge Grade Ⅱ or Ⅲ lesions of the patella to compare radiofrequency chondroplasty with mechanical debridement chondroplasty. The patients were evaluated before and after surgery based on the Fulkerson-Shea Patellofemoral Joint Evaluation Score, and the results showed that radiofrequency chondroplasty achieved better clinical outcomes. Clearing the cartilage lesions by radiofrequency cannot regenerate the original tissue, but is effective in relieving symptoms and delaying the progression of cartilage degeneration. This encouraging clinical effect can be explained by the microscopic observations that radiofrequency chondroplasty removes fibrotic cartilage, which is the source of chemical and mechanical irritation in the joint.
Although several studies have reported short-term and medium-term follow-up of radiofrequency treatment of AC lesions, long-term follow-up has not been reported yet. Recently, Spahn et al. published the 1-year, 4-years and 10-years follow-up results of bRFE versus mechanical debridement, respectively (Spahn et al., 2008; Spahn et al., 2010; Spahn et al., 2016). It was found that the subjective Knee injury and Osteoarthritis Outcome Score in the radiofrequency group was better than that in the control group. Although the Tegner score of the two groups was at the same level in the 10-years follow-up results, the radiofrequency group reached a higher level of exercise in the 1- and 4-years follow-up results. The medial joint space narrowed in both groups during the follow-up period, and was narrowing obviously faster in the mechanical debridement group. The results of long-term clinical follow-up showed that radiofrequency chondroplasty achieved a better subjective effect. The level of vitality at the 10-years follow-up was lower than that before operation, which might be explained by the increase in age and the progression of OA. Although neither of the two treatment methods can completely prevent the progression of OA, radiofrequency chondroplasty is able to delay the progression of OA more effectively.
Radiofrequency Versus Microfracture
Microfracture has also been used to treat cartilage lesions, relieve knee pain, and restore knee function. Techniques for repairing cartilage injuries by microfracture have been reported in the existing literature (Cerynik et al., 2009). Osti et al. applied radiofrequency and microfracture to treat postoperative Outerbridge Grade Ⅰ-Ⅱ lesions and Outerbridge Grade Ⅲ-Ⅳ lesions, respectively (Osti et al., 2010). At the 2-years and 5-years postoperative follow-up, the findings suggested that microfracture achieved similar results to RF in terms of short-term functional improvement; however, microfracture also failed to prevent the continued progression of OA.
Radiofrequency Chondroplasty: A Cost-Effective Technology
Cartilage lesions can eventually progress to OA, which has a enormous impact on the social economy and health (Mahmoudian et al., 2021). Early chondroplasty for cartilage lesions avoids the need for chondrocyte implantation or arthroplasty if the disease progresses further, which potentially decrease the socio-economic burden. In a study on the analysis of the economic benefits of comparing radiofrequency and mechanical debridement, the results showed that radiofrequency chondroplasty resulted in a total cost saving of more than 3000 USD per patient over a 4-years follow-up period. This was attributed to the better efficacy and lower revision rate of radiofrequency (Adeyemi et al., 2020). Moreover, radiofrequency is also considered superior to mechanical debridement in terms of operation time and contributes to the reduction in bleeding, which is facilitated by the coagulation effect of radiofrequency on the small vessels in the adjacent tissue (Camillieri et al., 2001; Cetik et al., 2009). For doctors and patients, this is clearly the treatment of choice.
Safety and Efficacy
Thermal Damage
It has been mentioned above that the use of radiofrequency may expose the surrounding healthy cartilage to heat stress and affect the chondrocyte vitality, but when the temperature is controlled at 50°C, the chondrocyte vitality will be able to restore to a certain extent after 1 week (Kaplan et al., 2003a). In order to avoid the thermal damage caused by radiofrequency, it is necessary to set appropriate working conditions. Firstly, the probe with a chondroprotective design can be used, and the power should be set appropriately according to the manufacturer’s guideline before the surgery (Huang et al., 2014). Secondly, the use time and probe distance must be precisely controlled during the surgery, because the damage of radiofrequency on the cartilage is time-dependent and the increase of probe distance can significantly reduce local temperature (Kaplan et al., 2003b). Last but not least, appropriate initial temperature and sufficient lavage fluid flow are required to ensure that radiofrequency can reach the working temperature more quickly and maintain an appropriate temperature environment in the joint (Lu et al., 2002b; Ahrens et al., 2018).
Osteonecrosis
The local high temperature generated by thermal energy equipment will not only affect the chondrocyte vitality, but also cause deeper bone damage. As mentioned earlier, Lu et al. revealed that bRFE penetrated deeper into the cartilage than mRFE, and hence the energy might also penetrate deeper into the subchondral bone and lead to osteonecrosis (Lu et al., 2001). It has been reported that subchondral bone necrosis occurs after the use of laser and radiofrequency thermal energy equipment in arthroscopic meniscectomy (Muscolo et al., 1996; Rozbruch et al., 1996; Encalada and Richmond, 2004; Cetik et al., 2009). Mehmet et al. found that the addition of radiofrequency chondroplasty to meniscectomy did not increase the incidence of osteonecrosis (Türker et al., 2015). Therefore, the occurrence of osteonecrosis following the use of thermal energy equipment during meniscectomy may be attributed to the increase of tibiofemoral contact pressure due to the decrease of weight-bearing area after meniscectomy, which may lead to subchondral bone microfracture and synovial fluid leakage in the bone, thereby resulting in osteonecrosis (Encalada and Richmond, 2004). In comparison, the use of radiofrequency equipment will not cause osteonecrosis. Similarly, what is desired is to guarantee that radiofrequency is used in a relatively safe environment, which can be achieved by taking the same steps as preventing thermal damage to ensure that local temperature does not exceed 50°C.
Is Radiofrequency Chondroplasty Really Safe?
After over 10 years of clinical application, accumulated evidence has supported the effectiveness of radiofrequency in the treatment of cartilage injury, which appears to be superior to mechanical debridement. This includes the long-term follow-up study conducted by Spahn et al. for 10 years. Recently, Koller et al. put forward an opposite point of view (Koller et al., 2020). They planned to use the magnetic resonance imaging T2 Mapping technique to quantitatively evaluate the efficacy of radiofrequency chondroplasty in patients with Outerbridge Grade Ⅱ lesions of the patella 1 year after surgery. However, the trial was terminated prematurely because the T2 Mapping quantitative analysis of 5 patients showed postoperative cartilage lesions. Koller et al. believed that radiofrequency was often combined with meniscus repair in treating cartilage lesions, so the improvement in the postoperative functional score might be due to meniscus repair. T2 Mapping is a new and sensitive MR technique, which can provide information about the interaction between extracellular matrix and cartilage water molecules, and better reflect the cartilage recovery of postoperative patients (Banjar et al., 2021). In particular, specific techniques for glycosaminoglycan (GAG) assessment, such as delayed gadolinium-enhanced MRI has also shown utility in detecting AC damage (Link et al., 2017). T2 Mapping has been widely used for the assessment of cartilage activity as a non-invasive technique, and MRI techniques such as delayed gadolinium-enhanced MRI are also promising. Future studies on the efficacy of radiofrequency will need to make greater use of these techniques.
Future Outlook
Radiofrequency has been proposed as an effective method for the treatment of cartilage lesions. However, since the local high temperature produced by radiofrequency will affect the chondrocyte vitality, it is necessary to control the use time, power setting and temperature adjustment precisely, so as to obtain a sufficiently smooth surface of the cartilage while avoiding the side effects of radiofrequency. In terms of clinical application, although a large number of clinical studies (even including 10-years follow-up studies) have proven the effectiveness and safety of radiofrequency, there is still a lack of follow-up studies to evaluate the cartilage growth at the biochemical level. Therefore, more effort is needed to include other indicators such as T2 Mapping, which can be used to evaluate the status of cartilage in vivo, to further confirm the safety of radiofrequency.
Conclusions
Cartilage lesion is a disease of AC loss caused by acute injury or repeated injury, which will eventually develop into OA without proper management. As an alternative to mechanical debridement and laser for the treatment of cartilage lesions, radiofrequency has achieved encouraging results in the past 2 decades. However, there is insufficient evidence to support its indications and safety. At present, we believe that, when radiofrequency is used to treat cartilage lesions, it is necessary to control its indications, mainly for Outerbridge Grade Ⅱ or Ⅲ lesions of the knee cartilage, and the range of lesions should generally not exceed 800 mm2. The use of radiofrequency in such cases seems to be safe with fewer complications.
Author Contributions
ZD contributed to the conception and design of this review article. CL and ZD performed searches, analyses, and interpretations. CL and ZD drafted the paper. JX, WZ, WL, KC and YZ substantially revised the paper. ZD and WZ gave final approval of the version to be submitted.
Funding
This study was supported by the National Natural Science Foundation of China (81902303, 81902682), Guangdong Basic and Applied Basic Research Foundation (2020A151501048, 2021A1515011122), Shenzhen Double Chain Project for Innovation and Development Industry supported by Bureau of Industry and Information Technology of Shenzhen (201806081524201510), Shenzhen Science and Technology Project (JCYJ20190806164216661, RCBS20200714114856299), Science and Technology Commission of Shanghai Municipality (Shanghai Sailing Program 19YF1408900), and Clinical Research Project of Shenzhen Second People's Hospital (20203357028).
Conflict of Interest
The authors declare that the research was conducted in the absence of any commercial or financial relationships that could be construed as a potential conflict of interest.
Publisher’s Note
All claims expressed in this article are solely those of the authors and do not necessarily represent those of their affiliated organizations, or those of the publisher, the editors, and the reviewers. Any product that may be evaluated in this article, or claim that may be made by its manufacturer, is not guaranteed or endorsed by the publisher.
Abbreviations
AC: articular cartilage; bRFE: bipolar radiofrequency energy; ECM: extracellular matrix; ICRS: International Cartilage Repair Society; IL: interleukin; MMP: matrix metalloproteinase; mRFE: monopolar radiofrequency energy; OA: osteoarthritis; OARSI: Osteoarthritis Research Society International; PAGCL: post-arthroscopic glenohumeral chondrolysis.
References
Adeyemi, A., Nherera, L., Trueman, P., and Ranawat, A. (2020). Cost-effectiveness Analysis of Coblation versus Mechanical Shaver Debridement in Patients Following Knee Chondroplasty. Cost Effectiveness and Resource Allocation 18, 1–44. doi:10.1186/s12962-020-00240-w
Ahrens, P., Mueller, D., Siebenlist, S., Lenich, A., Stoeckle, U., and Sandmann, G. H. (2018). The Influence of Radio Frequency Ablation on Intra-articular Fluid Temperature in the Ankle Joint - a Cadaver Study. BMC Musculoskelet. Disord. 19, 413. doi:10.1186/s12891-018-2347-5
Allen, R. T., Tasto, J. P., Cummings, J., Robertson, C. M., and Amiel, D. (2006). Meniscal Debridement with an Arthroscopic Radiofrequency Wand versus an Arthroscopic Shaver: Comparative Effects on Menisci and Underlying Articular Cartilage. Arthroscopy 22, 385–393. doi:10.1016/j.arthro.2005.12.007
Banjar, M., Horiuchi, S., Gedeon, D. N., and Yoshioka, H. (2021). Review of Quantitative Knee Articular Cartilage MR Imaging. Magn. Reson. Med. Sci. doi:10.2463/mrms.rev.2021-0052
Barber, F. A., and Iwasko, N. G. (2006). Treatment of Grade III Femoral Chondral Lesions: Mechanical Chondroplasty versus Monopolar Radiofrequency Probe. Arthrosc. J. Arthroscopic Relat. Surg. 22, 1312–1317. doi:10.1016/j.arthro.2006.06.008
Barbour, K. E., Helmick, C. G., Boring, M., and Brady, T. J. (2017). Vital Signs: Prevalence of Doctor-Diagnosed Arthritis and Arthritis-Attributable Activity Limitation - United States, 2013-2015. MMWR Morb. Mortal. Wkly. Rep. 66, 246–253. doi:10.15585/mmwr.mm6609e1
Brittberg, M., and Winalski, C. S. (2003). Evaluation of Cartilage Injuries and Repair. J. Bone Jt. Surg Am 85-A (Suppl. 2), 58–69. doi:10.2106/00004623-200300002-00008
Buckwalter, J. A., and Mankin, H. J. (1998). Articular Cartilage: Degeneration and Osteoarthritis, Repair, Regeneration, and Transplantation. Instr. Course Lect 47, 487–504.
Buckwalter, J. A. (2002). Articular Cartilage Injuries. Clin. Orthop. Relat. Res., 21–37. doi:10.1097/00003086-200209000-00004
Caffey, S., McPherson, E., Moore, B., Hedman, T., and Vangsness, C. J. (2005). Effects of Radiofrequency Energy on Human Articular Cartilage: An Analysis of 5 Systems. Am. J. Sports Med. 33, 1035–1039. doi:10.1177/0363546504271965
Camillieri, G., Margheritini, F., Maresca, G., and Mariani, P. P. (2001). Postoperative Bleeding Following Notchplasty in Anterior Cruciate Ligament Reconstruction: Thermal Radio Frequency versus Powered Instrumentation. Knee Surg. Sports Traumatol. Arthrosc. 9, 12–14. doi:10.1007/s001670000176
Caplan, A. I., Elyaderani, M., Mochizuki, Y., Wakitani, S., and Goldberg, V. M. (1997). Principles of Cartilage Repair and Regeneration. Clin. Orthop. Relat. Res., 254–269. doi:10.1097/00003086-199709000-00033
Cerynik, D. L., Lewullis, G. E., Joves, B. C., Palmer, M. P., and Tom, J. A. (2009). Outcomes of Microfracture in Professional Basketball Players. Knee Surg. Sports Traumatol. Arthrosc. 17, 1135–1139. doi:10.1007/s00167-009-0765-5
Cetik, O., Cift, H., Comert, B., and Cirpar, M. (2009). Risk of Osteonecrosis of the Femoral Condyle after Arthroscopic Chondroplasty Using Radiofrequency: A Prospective Clinical Series. Knee Surg. Sports Traumatol. Arthrosc. 17, 24–29. doi:10.1007/s00167-008-0604-0
Cook, J., Kuroki, K., Kenter, K., Marberry, K., Brawner, T., Geiger, T., et al. (2004). Bipolar and Monopolar Radiofrequency Treatment of Osteoarthritic Knee Articular Cartilage - Acute and Temporal Effects on Cartilage Compressive Stiffness, Permeability, Cell Synthesis, and Extracellular Matrix Composition. J. Knee Surg. 17, 99–108. doi:10.1055/s-0030-1248205
Curl, W. W., Krome, J., Gordon, E. S., Rushing, J., Smith, B. P., and Poehling, G. G. (1997). Cartilage Injuries: A Review of 31,516 Knee Arthroscopies. Arthroscopy 13, 456–460. doi:10.1016/s0749-8063(97)90124-9
Curtin, B., and Friebe, I. (2014). Dermal Burn during Hip Arthroscopy. Orthopedics 37, e746–e749. doi:10.3928/01477447-20140728-93
Cushing, H., and Bovie, W. T. (1928). Electro-surgery as an Aid to the Removal of Intracranial Tumors. Surg. Gynecol. Obstet. 47, 751–784.
D'Arsonval, M. A. (1891). A. Referate I. Niederösterreich. Oesterr. Botan. Z. 41, 283–286. doi:10.1007/bf01790749
Deng, Z. H., Li, Y. S., Gao, X., Lei, G. H., and Huard, J. (2018). Bone Morphogenetic Proteins for Articular Cartilage Regeneration. Osteoarthritis and Cartilage 26, 1153–1161. doi:10.1016/j.joca.2018.03.007
Dickson, J. A., and Calderwood, S. K. (1980). Temperature Range and Selective Sensitivity of Tumors to Hyperthermia: A Critical Review. Ann. NY Acad. Sci. 335, 180–205. doi:10.1111/j.1749-6632.1980.tb50749.x
Dutcheshen, N., Maerz, T., Rabban, P., Haut, R. C., Button, K. D., Baker, K. C., et al. (2012). The Acute Effect of Bipolar Radiofrequency Energy thermal Chondroplasty on Intrinsic Biomechanical Properties and Thickness of Chondromalacic Human Articular Cartilage. J. Biomech. Eng. 134, 81007. doi:10.1115/1.4007105
Edwards, R. R., Lu, Y., Nho, S., Cole, B. J., and Markel, M. D. (2002a). Thermal Chondroplasty of Chondromalacic Human Cartilage. An Ex Vivo Comparison of Bipolar and Monopolar Radiofrequency Devices. Am. J. Sports Med. 30, 90–97. doi:10.1177/03635465020300012801
Edwards, R. R., Lu, Y., Rodriguez, E., Markel, M. D., Athanasiou, K. A., et al. (2002b). Thermometric Determination of Cartilage Matrix Temperatures during thermal Chondroplasty: Comparison of Bipolar and Monopolar Radiofrequency Devices. Arthroscopy 18, 339–346. doi:10.1053/jars.2002.29893
Edwards, R. R., Lu, Y., Uthamanthil, R. K., Bogdanske, J. J., Muir, P., et al. (2007). Comparison of Mechanical Debridement and Radiofrequency Energy for Chondroplasty in an In Vivo Equine Model of Partial Thickness Cartilage Injury. Osteoarthritis Cartilage 15, 169–178. doi:10.1016/j.joca.2006.06.021
Encalada, I., and Richmond, J. C. (2004). Osteonecrosis after Arthroscopic Meniscectomy Using Radiofrequency. Arthroscopy 20, 632–636. doi:10.1016/j.arthro.2004.04.075
Enochson, L., Sönnergren, H. H., Mandalia, V. I., and Lindahl, A. (2012). Bipolar Radiofrequency Plasma Ablation Induces Proliferation and Alters Cytokine Expression in Human Articular Cartilage Chondrocytes. Arthroscopy 28, 1275–1282. doi:10.1016/j.arthro.2012.01.005
Flanigan, D. C., Harris, J. D., Trinh, T. Q., Siston, R. A., and Brophy, R. H. (2010). Prevalence of Chondral Defects in Athletes' Knees. Med. Sci. Sports Exerc. 42, 1795–1801. doi:10.1249/MSS.0b013e3181d9eea0
Ganguly, K., McRury, I. D., Goodwin, P. M., Morgan, R. E., and Augé II, W. K. (2010). Histopomorphic Evaluation of Radiofrequency Mediated Débridement Chondroplasty. Toorthj 4, 211–220. doi:10.2174/1874325001004010211
Gharaibeh, M., Szomor, A., Chen, D. B., and MacDessi, S. J. (2018). A Retrospective Study Assessing Safety and Efficacy of Bipolar Radiofrequency Ablation for Knee Chondral Lesions. Cartilage 9, 241–247. doi:10.1177/1947603517703731
Giddins, G., Shewring, D., and Downing, N. (2020). Articular Cartilage and Soft Tissue Damage from Radiofrequency thermal Ablation Wands at Wrist Arthroscopy. J. Hand Surg. Eur. Vol, 655655035. doi:10.1177/1753193420980347
Good, C. R., Shindle, M. K., Griffith, M. H., Wanich, T., and Warren, R. F. (2009). Effect of Radiofrequency Energy on Glenohumeral Fluid Temperature during Shoulder Arthroscopy. J. Bone Jt. Surg Am 91, 429–434. doi:10.2106/JBJS.G.01261
Han, G., Xu, B., Geng, C., and Cheng, X. (2014). Effectiveness of Arthroscopy for Ankle Impingement Syndrome. Zhongguo Xiu Fu Chong Jian Wai Ke Za Zhi 28, 673–676.
Huang, G., Jiang, W., Xie, W., Lu, W., Zhu, W., and Deng, Z. (2021). Role of Peroxisome Proliferator-Activated Receptors in Osteoarthritis (Review). Mol. Med. Rep. 23. doi:10.3892/mmr.2020.11798
Huang, S. K., Bharati, S., Graham, A. R., Lev, M., Marcus, F. I., and Odell, R. C. (1987). Closed Chest Catheter Desiccation of the Atrioventricular junction Using Radiofrequency Energy-Aa New Method of Catheter Ablation. J. Am. Coll. Cardiol. 9, 349–358. doi:10.1016/s0735-1097(87)80388-1
Huang, Y., Zhang, Y., Ding, X., Liu, S., and Sun, T. (2014). Working Conditions of Bipolar Radiofrequency on Human Articular Cartilage Repair Following thermal Injury during Arthroscopy. Chin. Med. J. (Engl) 127, 3881–3886.
Huber, M., Eder, C., Loibl, M., Berner, A., Zellner, J., Kujat, R., et al. (2015). RFE Based Chondroplasty in Wrist Arthroscopy Indicates High Risk for Chrondocytes Especially for the Bipolar Application. BMC Musculoskelet. Disord. 16, 6. doi:10.1186/s12891-015-0460-2
Huber, M., Eder, C., Mueller, M., Kujat, R., Roll, C., Nerlich, M., et al. (2013). Temperature Profile of Radiofrequency Probe Application in Wrist Arthroscopy: Monopolar versus Bipolar. Arthroscopy 29, 645–652. doi:10.1016/j.arthro.2012.11.006
Huber, M., Loibl, M., Eder, C., Kujat, R., Nerlich, M., Gehmert, S., et al. (2016). Effects on the Distal Radioulnar Joint of Ablation of Triangular Fibrocartilage Complex Tears with Radiofrequency Energy. J. Hand Surg. Am. 41, 1080–1086. doi:10.1016/j.jhsa.2016.08.013
Huber, M., Schlosser, D., Stenzel, S., Maier, J., Pattappa, G., Kujat, R., et al. (2020). Quantitative Analysis of Surface Contouring with Pulsed Bipolar Radiofrequency on Thin Chondromalacic Cartilage. Biomed. Res. Int. 2020, 1242086. doi:10.1155/2020/1242086
Jang, E., Danoff, J. R., Rajfer, R. A., and Rosenwasser, M. P. (2014). Revision Wrist Arthroscopy after Failed Primary Arthroscopic Treatment. J. Wrist Surg. 3, 30–36. doi:10.1055/s-0033-1364090
Kang, R. W., Gomoll, A. H., Nho, S. J., Pylawka, T. K., and Cole, B. J. (2008). Outcomes of Mechanical Debridement and Radiofrequency Ablation in the Treatment of Chondral Defects: A Prospective Randomized Study. J. Knee Surg. 21, 116–121. doi:10.1055/s-0030-1247805
Kaplan, L. D., Chu, C. R., Bradley, J. P., Fu, F. H., and Studer, R. K. (2003a). Recovery of Chondrocyte Metabolic Activity after thermal Exposure. Am. J. Sports Med. 31, 392–398. doi:10.1177/03635465030310031101
Kaplan, L. D., Ernsthausen, J. M., Bradley, J. P., Fu, F. H., and Farkas, D. L. (2003b). The thermal Field of Radiofrequency Probes at Chondroplasty Settings. Arthroscopy 19, 632–640. doi:10.1016/s0749-8063(03)00128-2
Khan, A. M., and Dillingham, M. F. (2002). Electrothermal Chondroplasty-Monopolar. Clin. Sports Med. 21, 663–674. doi:10.1016/s0278-5919(02)00022-4
Koller, U., Springer, B., Rentenberger, C., Szomolanyi, P., Waldstein, W., Windhager, R., et al. (2020). Radiofrequency Chondroplasty May Not Have a Long-Lasting Effect in the Treatment of Concomitant Grade II Patellar Cartilage Defects in Humans. J. Clin. Med. 9. doi:10.3390/jcm9041202
Lane, J. G., Amiel, M. E., Monosov, A. Z., and Amiel, D. (1997). Matrix Assessment of the Articular Cartilage Surface after Chondroplasty with the Holmium:YAG Laser. Am. J. Sports Med. 25, 560–569. doi:10.1177/036354659702500421
Leclercq, C., and Mathoulin, C. (2016). Complications of Wrist Arthroscopy: A Multicenter Study Based on 10,107 Arthroscopies. J. Wrist Surg. 5, 320–326. doi:10.1055/s-0036-1584163
Link, T. M., Neumann, J., and Li, X. (2017). Prestructural Cartilage Assessment Using MRI. J. Magn. Reson. Imaging 45, 949–965. doi:10.1002/jmri.25554
Lopez, M. J., Hayashi, K., Fanton, G. S., Thabit, G. R., and Markel, M. D. (1998). The Effect of Radiofrequency Energy on the Ultrastructure of Joint Capsular Collagen. Arthroscopy 14, 495–501. doi:10.1016/s0749-8063(98)70078-7
Lotto, M. L., Lu, Y., Mitchell, M. E., Wright, E. J., Lubowitz, J. H., and Markel, M. D. (2006). An Ex Vivo thermal Chondroplasty Model: The Association of a Char-like Layer and Underlying Cell Death. Arthroscopy 22, 1159–1162. doi:10.1016/j.arthro.2006.06.018
Lu, Y., Bogdanske, J., Lopez, M., Cole, B. J., and Markel, M. D. (2005). Effect of Simulated Shoulder thermal Capsulorrhaphy Using Radiofrequency Energy on Glenohumeral Fluid Temperature. Arthroscopy 21, 592–596. doi:10.1016/j.arthro.2005.02.013
Lu, Y., Edwards, R. R., Cole, B. J., and Markel, M. D. (2001). Thermal Chondroplasty with Radiofrequency Energy. An In Vitro Comparison of Bipolar and Monopolar Radiofrequency Devices. Am. J. Sports Med. 29, 42–49.
Lu, Y., Edwards, R. R., Nho, S., Cole, B. J., and Markel, M. D. (2002b). Lavage Solution Temperature Influences Depth of Chondrocyte Death and Surface Contouring during thermal Chondroplasty with Temperature-Controlled Monopolar Radiofrequency Energy. Am. J. Sports Med. 30, 667–673. doi:10.1177/03635465020300050601
Lu, Y., Edwards, R. R., Nho, S., Heiner, J. P., Cole, B. J., Markel, M. D., et al. (2002a). Thermal Chondroplasty with Bipolar and Monopolar Radiofrequency Energy: Effect of Treatment Time on Chondrocyte Death and Surface Contouring. Arthroscopy 18, 779–788. doi:10.1053/jars.2002.32840
Mahmoudian, A., Lohmander, L. S., Mobasheri, A., Englund, M., and Luyten, F. P. (2021). Early-stage Symptomatic Osteoarthritis of the Knee - Time for Action. Nat. Rev. Rheumatol. 17, 621–632. doi:10.1038/s41584-021-00673-4
Maly, K., Andres Sastre, E., Farrell, E., Meurer, A., and Zaucke, F. (2021). COMP and TSP-4: Functional Roles in Articular Cartilage and Relevance in Osteoarthritis. Ijms 22, 2242. doi:10.3390/ijms22052242
Más, M. J., Sanz, R. J., Morales, S. M., and Bustamante, S. D. P. D. (2015). Chondrolysis after Hip Arthroscopy. Arthroscopy 31, 167–172. doi:10.1016/j.arthro.2014.06.028
McCormick, F., Alpaugh, K., Nwachukwu, B. U., Xu, S., and Martin, S. D. (2013). Effect of Radiofrequency Use on Hip Arthroscopy Irrigation Fluid Temperature. Arthroscopy 29, 336–342. doi:10.1016/j.arthro.2012.10.001
McFarland, E. G., Kim, T. K., Banchasuek, P., and McCarthy, E. F. (2002). Histologic Evaluation of the Shoulder Capsule in normal Shoulders, Unstable Shoulders, and after Failed thermal Capsulorrhaphy. Am. J. Sports Med. 30, 636–642. doi:10.1177/03635465020300050201
Miller, D. V., O'Brien, S. J., Arnoczky, S. S., Kelly, A., Fealy, S. V., and Warren, R. F. (1989). The Use of the Contact Nd:YAG Laser in Arthroscopic Surgery: Effects on Articular Cartilage and Meniscal Tissue. Arthroscopy 5, 245–253. doi:10.1016/0749-8063(89)90137-0
Mitchell, M. E., Kidd, D., Lotto, M. L., Lorang, D. M., Dupree, D. M., Wright, E. J., et al. (2006). Determination of Factors Influencing Tissue Effect of thermal Chondroplasty: An Ex Vivo Investigation. Arthroscopy 22, 351–355. doi:10.1016/j.arthro.2006.01.006
Muscolo, D. L., Costa-Paz, M., Makino, A., and Ayerza, M. A. (1996). Osteonecrosis of the Knee Following Arthroscopic Meniscectomy in Patients over 50-years Old. Arthroscopy 12, 273–279. doi:10.1016/s0749-8063(96)90058-4
Nukavarapu, S. P., and Dorcemus, D. L. (2013). Osteochondral Tissue Engineering: Current Strategies and Challenges. Biotechnol. Adv. 31, 706–721. doi:10.1016/j.biotechadv.2012.11.004
Osti, L., Papalia, R., Del, B. A., Amato, C., Denaro, V., and Maffulli, N. (2010). Good Results Five Years after Surgical Management of Anterior Cruciate Ligament Tears, and Meniscal and Cartilage Injuries. Knee Surg. Sports Traumatol. Arthrosc. 18, 1385–1390. doi:10.1007/s00167-009-1035-2
Owens, B. D., Stickles, B. J., Balikian, P., and Busconi, B. D. (2002). Prospective Analysis of Radiofrequency versus Mechanical Debridement of Isolated Patellar Chondral Lesions. Arthroscopy 18, 151–155. doi:10.1053/jars.2002.29906
Pawl, R. P. (1975). Percutaneous Radiofrequency Electrocoagulation in the Control of Chronic Pain. Surg. Clin. North America 55, 167–179. doi:10.1016/s0039-6109(16)40540-2
Peng, L., Li, Y., Zhang, K., Chen, Q., Xiao, L., Geng, Y., et al. (2020). The Time-dependent Effects of Bipolar Radiofrequency Energy on Bovine Articular Cartilage. J. Orthop. Surg. Res. 15, 106. doi:10.1186/s13018-020-01626-5
Pritzker, K. P., Gay, S., Jimenez, S. A., Ostergaard, K., Pelletier, J. P., Revell, P. A., et al. (2006). Osteoarthritis Cartilage Histopathology: Grading and Staging. Osteoarthritis Cartilage 14, 13–29. doi:10.1016/j.joca.2005.07.014
Rehan-Ul-Ha, , Yang, H. K., Park, K. S., Lee, K. B., and Yoon, T. R. (2010). An Unusual Case of Chondrolysis of the Hip Following Excision of a Torn Acetabular Labrum. Arch. Orthopaedic Trauma Surg. 130, 65–70. doi:10.1007/s00402-009-0837-5
Rocco, P., Lorenzo, D. B., Guglielmo, T., Michele, P., Nicola, M., and Vincenzo, D. (2016). Radiofrequency Energy in the Arthroscopic Treatment of Knee Chondral Lesions: A Systematic Review. Br. Med. Bull. 117, 149–156. doi:10.1093/bmb/ldw004
Rozbruch, S. R., Wickiewicz, T. L., DiCarlo, E. F., and Potter, H. G. (1996). Osteonecrosis of the Knee Following Arthroscopic Laser Meniscectomy. Arthroscopy 12, 245–250. doi:10.1016/s0749-8063(96)90022-5
Ryan, A., Bertone, A. L., Kaeding, C. C., Backstrom, K. C., and Weisbrode, S. E. (2003). The Effects of Radiofrequency Energy Treatment on Chondrocytes and Matrix of Fibrillated Articular Cartilage. Am. J. Sports Med. 31, 386–391. doi:10.1177/03635465030310031001
Schosheim, P. M., and Caspari, R. B. (1986). Evaluation of Electrosurgical Meniscectomy in Rabbits. Arthroscopy 2, 71–76. doi:10.1016/s0749-8063(86)80015-9
Shellock, F. G., and Shields, C. L. (2000). Radiofrequency Energy-Induced Heating of Bovine Articular Cartilage Using a Bipolar Radiofrequency Electrode. Am. J. Sports Med. 28 (5), 720–724. doi:10.1177/03635465000280051701
Slattery, C., and Kweon, C. Y. (2018). Classifications in Brief: Outerbridge Classification of Chondral Lesions. Clin. Orthop. Relat. Res. 476, 2101–2104. doi:10.1007/s11999.0000000000000255
Solomon, D. J., Navaie, M., Stedje-Larsen, E. T., Smith, J. C., and Provencher, M. T. (2009). Glenohumeral Chondrolysis after Arthroscopy: A Systematic Review of Potential Contributors and Causal Pathways. Arthroscopy 25, 1329–1342. doi:10.1016/j.arthro.2009.06.001
Spahn, G., Hofmann, G. O., and von Engelhardt, L. V. (2016). Mechanical Debridement versus Radiofrequency in Knee Chondroplasty with Concomitant Medial Meniscectomy: 10-Year Results from a Randomized Controlled Study. Knee Surg. Sports Traumatol. Arthrosc. 24, 1560–1568. doi:10.1007/s00167-015-3810-6
Spahn, G., Kahl, E., Mückley, T., Hofmann, G. O., and Klinger, H. M. (2008). Arthroscopic Knee Chondroplasty Using a Bipolar Radiofrequency-Based Device Compared to Mechanical Shaver: Results of a Prospective, Randomized, Controlled Study. Knee Surg. Sports Traumatol. Arthrosc. 16, 565–573. doi:10.1007/s00167-008-0506-1
Spahn, G., Klinger, H. M., Mückley, T., and Hofmann, G. O. (2010). Four-year Results from a Randomized Controlled Study of Knee Chondroplasty with Concomitant Medial Meniscectomy: Mechanical Debridement versus Radiofrequency Chondroplasty. Arthroscopy 26, S73–S80. doi:10.1016/j.arthro.2010.02.030
Suarez-Ahedo, C., Pavan, V. S., Stake, C. E., Finley, Z. A., Martin, T. J., Gui, C., et al. (2015). What Are the Current Indications for Use of Radiofrequency Devices in Hip Arthroscopy? A Systematic Review. J. Hip Preserv Surg. 2, 323–331. doi:10.1093/jhps/hnv055
Türker, M., Çetik, Ö., Çırpar, M., Durusoy, S., and Cömert, B. (2015). Postarthroscopy Osteonecrosis of the Knee. Knee Surg. Sports Traumatol. Arthrosc. 23, 246–250. doi:10.1007/s00167-013-2450-y
Turner, A., Tippett, J., Powers, B., Dewell, R., and Mallinckrodt, C. (1998). Radiofrequency (Electrosurgical) Ablation of Articular Cartilage: A Study in Sheep. Arthrosc. J. Arthroscopic Relat. Surg. 14, 585–591. doi:10.1016/s0749-8063(98)70054-4
Uribe, J. W. (2002). Electrothermal Chondroplasty-Bbipolar. Clin. Sports Med. 21, 675. doi:10.1016/s0278-5919(02)00016-9
Uthamanthil, R. K., Edwards, R. B., Lu, Y., Manley, P. A., Athanasiou, K. A., and Markel, M. D. (2006). In Vivo study on the Short-Term Effect of Radiofrequency Energy on Chondromalacic Patellar Cartilage and its Correlation with Calcified Cartilage Pathology in an Equine Model. J. Orthop. Res. 24, 716–724. doi:10.1002/jor.20108
Voloshin, I., DeHaven, K. E., Steadman, J. R., and DeHaven, K. E. (2005). Second-look Arthroscopic Observations after Radiofrequency Treatment of Partial Thickness Articular Cartilage Defects in Human Knees: Report of Four Cases. J. Knee Surg. 18, 116–122. doi:10.1055/s-0030-1248168
Voloshin, I., Morse, K. R., Allred, C. D., Bissell, S. A., Maloney, M. D., et al. (2007). Arthroscopic Evaluation of Radiofrequency Chondroplasty of the Knee. Am. J. Sports Med. 35, 1702–1707. doi:10.1177/0363546507304328
Voss, J. R., Lu, Y., Edwards, R. B., Bogdanske, J. J., and Markel, M. D. (2006). Effects of thermal Energy on Chondrocyte Viability. Am. J. Vet. Res. 67, 1708–1712. doi:10.2460/ajvr.67.10.1708
Wang, N., Liu, Y. J., Xue, J., Li, H. F., He, W., and Wang, J. L. (2012). Effects of Radiofrequency Energy on Porcine Articular Cartilage: Higher-Power Settings in Ablation Mode Show Lower thermal Radiation Injury. Knee Surg. Sports Traumatol. Arthrosc. 20, 1901–1906. doi:10.1007/s00167-011-1798-0
Whipple, T. L., Caspari, R. B., and Meyers, J. F. (1985). Arthroscopic Laser Meniscectomy in a Gas Medium. Arthroscopy 1, 2–7. doi:10.1016/s0749-8063(85)80071-2
Wienecke, H., and Lobenhoffer, P. (2003). Grundlagen radiochirurgischer Systeme und ihre Anwendungen in der Arthroskopie. Der Unfallchirurg 106, 2–12. doi:10.1007/s00113-002-0559-4
Yasura, K., Nakagawa, Y., Kobayashi, M., Kuroki, H., and Nakamura, T. (2006). Mechanical and Biochemical Effect of Monopolar Radiofrequency Energy on Human Articular Cartilage: An In Vitro Study. Am. J. Sports Med. 34, 1322–1327. doi:10.1177/0363546506287742
Zhang, Y., Feng, G., Xu, G., and Qi, Y. (2019). Microporous Acellular Extracellular Matrix Combined with Adipose-Derived Stem Cell Sheets as a Promising Tissue Patch Promoting Articular Cartilage Regeneration and Interface Integration. Cytotherapy 21, 856–869. doi:10.1016/j.jcyt.2019.02.005
Zhou, Z., Deng, Z., Liu, Y., Zheng, Y., Yang, S., Lu, W., et al. (2021). Protective Effect of SIRT1 Activator on the Knee with Osteoarthritis. Front. Physiol. 12, 661852. doi:10.3389/fphys.2021.661852
Zhu, X., Yang, L., and Duan, X. (2019). Arthroscopically Assisted Anterior Treatment of Symptomatic Large Talar Bone Cyst. J. Foot Ankle Surg. 58, 151–155. doi:10.1053/j.jfas.2018.03.028
Keywords: cartilage lesions, radiofrequency, articular cartilage, thermal energy, chondroplasty
Citation: Lin C, Deng Z, Xiong J, Lu W, Chen K, Zheng Y and Zhu W (2022) The Arthroscopic Application of Radiofrequency in Treatment of Articular Cartilage Lesions. Front. Bioeng. Biotechnol. 9:822286. doi: 10.3389/fbioe.2021.822286
Received: 25 November 2021; Accepted: 29 December 2021;
Published: 20 January 2022.
Edited by:
Feng-Juan Lyu, South China University of Technology, ChinaReviewed by:
Senbo An, Shandong Provincial Hospital, ChinaHuan-Tian Zhang, First Affiliated Hospital of Jinan University, China
Copyright © 2022 Lin, Deng, Xiong, Lu, Chen, Zheng and Zhu. This is an open-access article distributed under the terms of the Creative Commons Attribution License (CC BY). The use, distribution or reproduction in other forums is permitted, provided the original author(s) and the copyright owner(s) are credited and that the original publication in this journal is cited, in accordance with accepted academic practice. No use, distribution or reproduction is permitted which does not comply with these terms.
*Correspondence: Zhenhan Deng, ZGVuZ3poZW5oYW5AZW1haWwuc3p1LmVkdS5jbg==; Weimin Zhu, c3poendtQGVtYWlsLnN6dS5lZHUuY24=
†These authors have contributed equally to this work and share first authorship