- 1School of Basic Medical Sciences and Forensic Medicine, Hangzhou Medical College, Hangzhou, China
- 2Jiangsu Co-innovation Center of Efficient Processing and Utilization of Forest Resources, College of Chemical Engineering, Nanjing Forestry University, Nanjing, China
Supplementing commercial xylanase and cellulase with selected debranching enzymes only resulted in slight enhancement of the enzymatic hydrolysis of wheat bran autohydrolysis residues (WBAR) which was obtained at 160°C over a 30-min period of autohdyrolysis, while a blend of enzymes from Aspergillus niger and Eupenicillium parvum achieved synergistic efficacy in this context. Using an equal mixture blend of these enzymes at a 0.5% (w/w) enzyme loading dosage with the addition of ferulic acid esterase (1 U/g substrate), the obtained hydrolysis yields were desirable, including 84.98% of glucose, 84.74% of xylose, 80.24% of arabinose, and 80.86% of ferulic acid. Following further separation using an HP-20 resin, the final ferulic acid recovery levels were as high as 62.5% of the esterified ferulic acid present within the initial WBAR input. Together, these data suggest that a combination of autohydrolysis and enzymatic hydrolysis using crude enzyme blends can efficiently achieve wheat bran enzymatic saccharification and associated ferulic acid release.
Introduction
Wheat bran, which accounts for 25% of the weight of wheat grains, is a byproduct of the wheat production process. Approximately 150 million tons of wheat bran are generated globally each year, and this bran is most commonly utilized as a feed for livestock (Si et al., 2020). However, bran-based feeds are of low commercial value, leading to efforts in the wheat industry to convert this byproduct into a higher value commodity. Wheat bran is composed primarily of arabinoxylan (38–55% of dry mass), cellulose (16–30%), and esterified ferulic acid ( ∼ 1%). Ferulic acid (FA) offers value as an antimicrobial compound with antioxidant properties, and it can additionally serve as a preservative for food products (Li et al., 2021; Hu et al., 2022). Moreover, it can be converted into vanillin for use as a flavoring agent in the food and perfume industries (Ferri et al., 2020). Wheat bran thus represents an ideal low-cost, abundant, organic source of natural FA production and a valuable source for other biochemical compounds of potential commercial value.
Most studies to date have primarily focused on preparing and processing wheat bran-derived xylooligosaccharides (Mathew et al., 2017; Wu et al., 2017; Bhattacharya et al., 2020; Sonkar et al., 2021) or arabinoxylan (Aguedo et al., 2014; Sánchez-Bastardo et al., 2017; Kaur et al., 2021). Other researchers have sought to improve wheat bran utilization efficiency by developing approaches to generating fermentable sugars as products of wheat bran decomposition, providing an efficient source for the precursors necessary for bioethanol preparation. Enzymatic saccharification can maintain the production of these fermentable sugars without favoring the concomitant production of inhibitory compounds (Huang et al., 2022). However, achieving complete enzyme-mediated hydrolysis of raw wheat bran remains challenging owing to the complexity and heterogeneity of arabinoxylan. Wheat bran arabinoxylan exhibits a high degree of substitution, with a β-1,4-linked xylopyranose backbone linked to α-L-arabinofuranose units that can either be unsubstituted or exhibit xylose C(O)-2 and/or C(O)-3 substitutions (Gullón et al., 2014). Moreover, these molecules can exhibit α-1,2-linked glucuronic, acetyl, D-galactopyranosyl or methyl-glucuronic acid residue substituents, and ferulic acid can be esterified to arabinose units at the O-5 position (Mathew and Abraham, 2004; Ma et al., 2012; Peng et al., 2012). Additionally, ferulic acid can form dehydrodimers that can facilitate arabinoxylan polymer cross-linking (Mandalari et al., 2005). Owing to these complexities, highly efficient enzymatic cocktails are essential to fully unlock the commercial and biological potential of wheat bran polysaccharides. On the other hand, in prior studies, the underlying utility of wheat bran-derived ferulic acid generated in the context of enzymatic hydrolysis has largely been overlooked, highlighting an untapped resource that has the potential to be enriched from prepared enzymatic hydrolysates.
To alter the chemical and microscopic structural properties of wheat bran and to increase its amenability to subsequent enzymatic hydrolysis, bran initially undergoes pretreatment processing. Prior studies focused on bioethanol production have utilized H2SO4, H3PO4, or other acids to liberate sugars from wheat bran (Palmarola-Adrados et al., 2005; Cripwell et al., 2015; Nair et al., 2015). While this resulted in acceptable rates of sugar recovery, such harsh acidic pretreatment ultimately results in the degradation of a portion of the sugar molecules. In contrast, hydrothermal autohydrolysis-based pretreatment efforts are cost-effective, straightforward, and do not incur significant environmental harm (Batalha et al., 2015; Khalili and Amiri, 2020; Wang et al., 2021). Mild heat-based autohydrolysis treatment can facilitate partial arabinoxylan depolymerization and debranching, breaking down complex hemicellulose molecular networks within wheat bran. However, the full degradation of wheat bran substrates necessitates the use of a complex enzymatic mixture including both cellulolytic and hemicellulolytic enzymes. Owing to enzymatic synergy such that the products of a given reaction can serve as substrates for a different enzyme, crude enzyme extract blends with extensive enzyme activities can enhance lignocellulose hydrolytic efficiency (Saini et al., 2016). For example, α-L-arabinofuranosidases are unable to liberate L-arabinofuranosyl residue (Araf) that are esterified with phenolic acids (Biely et al., 2016). Therefore, the prior action of ferulic acid esterase (FAE) to liberate trans-ferulic acid from 5-O-feruloylated Araf is essential for the subsequent action of α-L-arabinofuranosidases. Besides, WB xylan backbone is highly substituted with arabinofuranosyl substituents singly attached to C(O)-2/C(O)-3, or doubly linked to C(O)-2,3 of the xylose residues (Sørensen et al., 2007a; Sakamoto et al., 2011). These arabinose substitutions partly impede the action of endo-xylanase by causing steric hindrance for accessing the β-1,4-bonds in xylan, resulting in the limited release of xylobiose, xylotriose and other short-chain xylooligosaccharides from partially shaved xylan backbone (Sørensen et al., 2005; Sørensen et al., 2007a). In other words, the depolymerization of xylan backbone by endo-xylanase is depended on the removal of the arabinofuranosyl residues from arabinoxylan by arabinofuranosidases catalysis, and this depolymerization also makes β-xylosidase perform better on the catalysis release of xylose from produced xylobiose, xylotriose, and short-chain xylooligosaccharides (Sørensen et al., 2005; Sørensen et al., 2007b). As such, wheat bran autohydrolysis residue (WBAR) derived from the pretreatment of de-starched wheat bran using hot water was subjected to further enzymatic hydrolysis in order to facilitate monosaccharide and FA recovery through synergistic enzymatic activity using a blend of crude enzymatic extracts derived from Aspergillus niger and Eupenicillium parvum. This strategy offers potential value as a reliable approach to biomass hydrolysis for the preparation of fermentable sugars and natural FA. Many different fungal strains such as those belonging to Aspergillus, Trichoderma as well as Penicillium, and their combinations have been used to prepare synergistic cellulase blends either by co-culture or by mixing of broths in the past. However, as far as our knowledge is concerned, there are fewer reports on the synergistic crude enzyme preparations from Eupenicillium and Aspergillus.
Materials and Methods
Materials
Wheat bran was obtained from Nanyang, Henan Province, China, and was treated with papain and amylase (Imperial Jade Biotechnology Co., Ltd., Ningxia, China) based on a modified version of the protocol developed by Rose and Inglett to yield de-starched WB (DSWB) (Rose and Inglett, 2010). One hundred grams of wheat bran were treated with 0.3% amylase enzyme solution at 65°C for 30 min, and then incubated at 55°C for 30 min after addition of 0.3% (w/v) papain enzyme, followed by heat deactivation at 99°C for 20 min and extensive washing with distilled water to remove starch. Commercial cellulase, β-glucosidase, and xylanase were obtained from Sigma Aldrich (MO, USA). Arabinofuranosidase (AF) and acetyl xylan esterase (AXE) were from Megazyme (Bray, Ireland). FA esterase (FAE) derived from Myceliopthora thermophila (ATCC 42464) that had been recombinantly expressed in Pichia pastoris strain X33 was provided as a kind gift by Prof. Ding of Nanjing Forestry University, who also provided the hemicellulolytic enzyme-producing E. parvum 4–14 strain. E. parvum 4–14 was isolated from soil (Nanjing, China) and deposited in the China Center for Type Culture Collection (CCTCC) (Long et al., 2016). All other chemicals used herein were of analytical grade and were from Sinopharm Chemical Reagent Co., Ltd. (Shanghai, China). A. niger TRIIM 3.00944 was from Tianjin Institute of Industrial Microorganism in China.
Solid-State Fermentation
Crude enzymatic preparations were prepared via SSF from A. niger and E. parvum strains grown in modified Mandel’s medium in which wheat bran served as a carbon source as per the methods described previously by Long et al. (Long et al., 2016). In a 250 ml Erlenmeyer flask, for every 1.5 g of delignified wheat straw (1–2 mm), 1.5 g of DSWB was mixed with 5 ml of 10 × Mandel’s medium without any other carbon source, followed by sterilization at 121°C for 20 min. A fungal block derived from a PDA slant was then inoculated into a 250 ml Erlenmeyer flask containing 50 ml of liquid medium, followed by culture for 7 days at 37°C with constant agitation (200 rpm). Then, 2 ml of the prepared fungal culture was used to inoculate the Erlenmeyer flask containing SSF medium prepared above, followed by fermentation for 10 days at 37°C with 70% humidity. Following the completion of this fermentation step, flasks were mixed well with 25 ml of sterilized H2O supplemented with 0.1% (v/v) Tween-80, shaken (120 rpm) for 2 h at 28°C, and centrifuged for 10 min at 7,000 × g. Supernatants were then transferred to fresh tubes for enzymatic analyses. Tetracycline was added to crude enzyme preparations (0.05%, w/v), followed by storage at 4°C.
WBAR Preparation
A stainless steel batch reactor (model YRG2-10 × 1.25 L, ZhengJie Technology and Development Co., Ltd., Nanjing, China) was utilized for autohydrolysis. Briefly, DSWB (50 g) was added to the reactor and mixed with ultrapure water (500 ml) followed by immersion in an oil bath. Autohydrolysis was then conducted for 30 min at 160°C, not including periods for heating and cooling. Following the completion of autohydrolysis, reactors were immersed in cool water to lower the reaction system temperature to the ambient temperature. Solid residues were then washed repeatedly using tap water, collected on a filtration cloth, and dried under vacuum for 24 h at 40°C. Samples were then sealed in zipper-locked bags prior to subsequent composition analyses and enzymatic hydrolysis.
WBAR Hydrolysis Using Commercial Enzymes or Crude Enzyme Blends
WBAR was used as a substrate for enzymatic hydrolysis performed in 50 ml conical flasks at a substrate loading of 5% (w/v, 5 ml total volume) at 50°C using 50 mM sodium citrate buffer (pH 5.0). Flasks were constantly agitated (150 rpm) for 72 h, with added enzymes including 15 CBU β-glucosidase (Novozyme 188, 269 CBU/g), 15 FPU cellulase (Sigma C2730, 117 FPU/g), and 200 U xylanase (Sigma X2629, 7700 U/g) per gram of dry biomass. Tetracycline (0.05%, w/v) was added to prevent bacterial contamination. Reactions were terminated via transferring the flasks into boiling water for 10 min and then clarifying hydrolysates via centrifugation. Supernatant glucose, xylose, and arabinose levels were assessed via HPLC. Analyses were repeated in duplicate. Glucose and xylose yields were determined with the following equations:
The conversion factor for dehydration on polymerization to cellulose was 162/180 (0.9) for glucose; to xylan and arabinan, it was 132/150 (0.88) for xylose and arabinose, respectively.
In experiments in which debranching enzymes were added, experimental protocols were as above with the addition of 1 U of AF (Megazeme, E-ABFAN), AXE (Megazeme, E-AXEAO), and FAE complemented with 15 FPU of cellulase, 15 CBU of β-glucosidase, and 200 U of xylanase. FA oxidation was prevented via the addition of sodium hydrogen sulfite (100 mg/L). Glucose and xylose yields were calculated as above, while arabinose, FA, and acetic acid yields were calculated with the following equations:
WBAR enzymatic hydrolysis using crude blends of enzymes derived from E. parvum and A. niger was conducted as above at a range of experimentally appropriate enzyme doses. Monosaccharide and FA hydrolysis yields were calculated using Equations 1–4.
Carbohydrate, Ferulic Acid, and Acetic Acid Analyses
A two-step sulfuric acid-based hydrolysis approach was used to measure xylan, cellulose, arabinan, and other structural carbohydrates derived from WBAR (Sluiter et al., 2005). An HPLC approach was utilized to measure levels of glucose, xylose, and arabinose in the acid hydrolysate samples by using an Agilent 1,100 (USA) instrument with a Bio-Rad Aminex HPX-87H column (300 mm × 7.8 mm; USA) and a refractive index detector. This analytical column was used at a constant 55°C temperature with a mobile phase composed of H2SO4 (5 mM) and a constant 0.6 ml/min flow rate. The conversion factor for dehydration on polymerization to cellulose was 162/180 (0.9) for glucose; to xylan and arabinan, it was 132/150 (0.88) for xylose and arabinose. All analyses were conducted in triplicate.
Levels of acetic acid and monosaccharides present within enzymatic hydrolysates were measured via HPLC as above. Esterified FA levels in WBAR were measured following NaOH saponification as in a prior report (Jiang et al., 2016). Levels of free FA were assessed via HPLC (Agilent Technologies 1,260 Infinity) with a ZORBAX Eclipse Plus C18 column (4.6 × 100 mm, Agilent, CA, USA) at 30°C with a mobile phase composed of acetic acid (0.1%)-methanol (65:35) and a 0.8 ml/min flow rate. An external standard-based method was utilized for final quantitative analyses at 320 nm. Levels of FA in enzymatic hydrolysates were directly assessed via HPLC as above. All analyses were conducted in triplicate.
Crude Enzyme Activity Analyses
Crude enzyme activity levels were assessed at 50°C in 50 mM sodium phosphate buffer (pH 5.0). Endoglucanase (CMCase) and xylanase activities were assayed in 2.5 ml reaction mixtures containing 100 μL crude enzymatic extract and carboxymethyl cellulose (CMC-Na) or beechwood xylan (Sigma, St. Louis, MO) at the final concentration of 1% or 0.2% (w/v) respectively. Reaction mixtures were incubated at 50°C for 30 min for CMCase or 10 min for xylanase, and the released reducing sugar was quantified by the Somogyi-Nelson method using glucose or xylose standard curves. Enzymatic activity on filter paper was determined in a similar method that was used to determine endoglucanase activity, by taking 30 mg of Whatman No. 1 filter paper in 2.5 ml of 50 mM sodium citrate buffer (pH 5.0) as the substrate. The activities of β-xylosidase, β-glucosidase, arabinofuranosidase, and glucuronidase were assessed in 1 ml reaction mixtures containing 900 μL of 50 mM sodium citrate buffer (pH 5.0), 50 μL enzyme solution, and respective 50 μL of 50 mM p-nitrophenyl β-D-xyloside, p-nitrophenyl β-D-glucoside, p-nitrophenyl α-L-arabinoside, or p-nitrophenyl β-D-glucuronide (Sigma, St. Louis, MO) as the substrates. After incubating at 50°C for 30 min, the reaction was stopped by adding 4 ml of glycine buffer (0.4 M, pH 10.8), and the liberated p-nitrophenol was measured at 405 nm. One unit (U) of enzyme activity was defined as the amount of enzyme required to liberate 1 μmol p-nitrophenol from the corresponding substrates per millilitre per minute under the assay conditions. The AXE activity was determined spectrophotometrically at 50°C by measuring the increasing in A354 nm during the initial 1 min of the assay resulting from the release of 4-methylumbelliferone from 4-methylumbelliferyl acetate. Reaction mixtures consisted of 1,390 μL 50 mM sodium citrate buffer (pH 5.0), 100 μL of 10 mM 4-methylumbelliferyl acetate and 10 μL crude enzyme. One unit of enzyme activity (U) was defined as the quantity of enzyme required to release 1 μmol of 4-methylumbelliferone per minute. FAE activity was assessed based upon the rate of methyl-ferulate (MFA) conversion into FA. Briefly, 100 μL of crude enzyme was mixed with 900 μL of 50 mM sodium citrate buffer (pH 5.0) containing 5.0 mM MFA. After incubating at 50°C for 30 min, the reaction was terminated at 99°C for 10 min. The released free FA was analysed using HPLC. One unit (U) of FAE activity was defined as the amount of enzyme liberating 1 μmol of free FA per min under the standard assay conditions. Benzyl alcohol release from benzyl-D-glucuronate was measured via HPLC to assess glucuronoyl esterase activity levels, which was similar to the determination of FAE activity. All assays were conducted in triplicate and the results were averaged together for final report.
Date Analysis
One way analysis of variance (ANOVA) was conducted by SPSS software (Version 19.0) at p < 0.05 probability level. Multiple comparisons were conducted by Duncan method.
Results and Discussion
Wheat Bran Sample Composition
DSWB used in the present study was primarily composed of cellulose, xylan, and arabinan, accounting for 19.1, 29.2, and 22.2% of the total weight, respectively, demonstrating the high levels of carbohydrates therein (Table 1). Esterified FA and acetic acid levels in DSWB were 1.0 and 0.5%, respectively. The high FA and polysaccharide levels within this wheat bran input underscore the promise of DSWB as a source of fermentable monosaccharides and natural FA. Following autohydrolysis treatment (30 min at 160°C), marked arabinan depolymerization had occurred such that it accounted for just 13.1% of WBAR. Moreover, xylan content declined from 29.2 to 25.2%, indicating that arabinan and xylan exhibit different levels of autohydrolysis sensitivity. These findings are consistent with prior studies (Silva-Fernandes et al., 2015). Other component levels were also reduced following autohydrolysis with the exception of cellulose, the levels of which rose from 19.1 to 35.1%, suggesting that autohydrolysis did not significantly promote cellulose dissolution (Jiang et al., 2018; Tang et al., 2021). Additionally, it was found that there were also some acid insoluble constituents in both DSWB and WBAR, respectively accounting for 11.7 and 13.5%. These acid insoluble constituents might include lignin and ashes, etc.
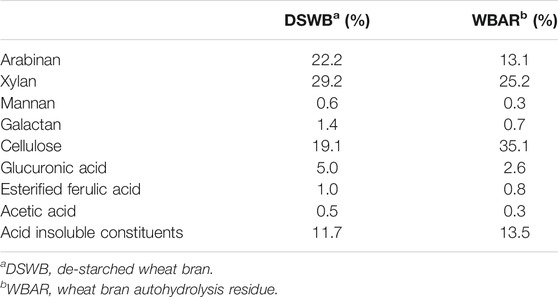
TABLE 1. The content of different components in de-starched wheat bran and wheat bran autohydrolysis residue.
The Impact of Commercial Enzymatic Preparations on the Release of FA and Monosaccharides From WBAR
Next, the impacts of the debranching enzymes FAE, AXE, and AF on the release of FA, monosaccharide, and acetic acid release from WBAR were assessed. As illustrated in Figure 1, the addition of both cellulase and β-glucosidase leaded to a glucose yield of 35.27% and xylose yield of 11.47% as the commercial cellulase also showed some xylanase activities. The addition of xylanase to cellulase and β-glucosidase resulted in respective increases in glucose and xylose yields by 55.68 and 78.47%, although overall xylose yield remained relatively low (20.47%). Adding AF to this enzymatic mixture increased arabinose yield by 57.92% to a relatively low final yield of 12.46%, while FAE addition improved FA yield by 52.17% to a final yield of 11.23%. Adding AXE to the enzymatic mixture did not significantly increase acetic acid yield. Finally, the combined addition of AF, FAE, and AXE as accessory enzymes resulted in respective xylose, arabinose, FA, and acetic acid yields of 22.58, 13.12, 13.25, and 17.22%. The heterogeneous makeup of wheat bran-derived arabinoxylan thus makes it difficult for these combinations of debranching enzymes to effectively degrade, with hydrolysis likely being restricted by steric hindrance or an absence of other enzymes including glucuronidase, β-xylosidase, glucuronoyl esterase, etc, which can catalyze the hydrolysis of certain atypical bond types including glucuronide linkages, glycosidic bonds, glucuronoyl esters, etc (Biely et al., 2016). A range of enzymes with different activity profiles is essential to enhance monosaccharide and FA release from wheat bran.
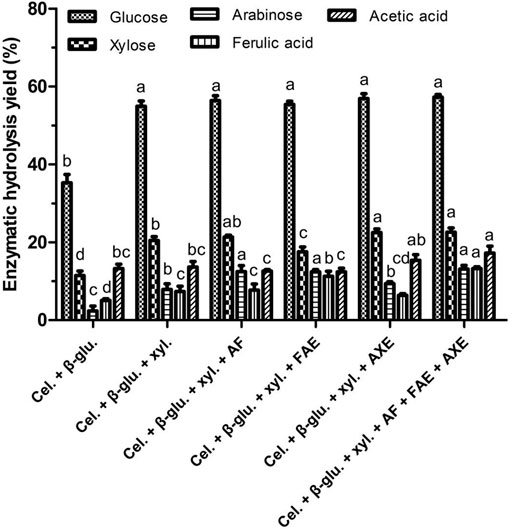
FIGURE 1. Monosaccharides and ferulic acid release from wheat bran autohydrolysis residue by commercial enzyme mixtures. Cel., cellulase; β-glu., β-glucosidase; Xyl., xylanase; AF, arabinofuranosidase; FAE, ferulic acid esterase; AXE, acetyl xylan esterase. Enzymatic hydrolysis yield of the same product between different treatments were conducted for one way analysis of variance (ANOVA) and multiple comparisons, where different lowercase letters indicate significant difference at p < 0.05 probability level.
Assessment of the Synergistic Hydrolytic Activity of Crude Enzymes Derived From Two Fungal Species
As shown in Figure 2, the enzymatic yields of glucose, xylose, arabinose and ferulic acid were respectively 61.38, 74.39, 66.23 and 65.17% when using the crude enzyme of 1% (w/w) from E. parvum, while that were respectively 78.35, 70.02, 57.37 and 70.12% when using the same enzyme dose from A. niger. However, 83.46% of glucose, 81.28% of xylose, 70.34% of arabinose and 67.89% of ferulic acid were respectively yielded when using an equal-parts mixture of 0.5% (w/w) of each crude enzyme extract. Therefore, generally, an enzymatic blend derived from A. niger and E. parvum, which consisted of an equal-parts mixture of 0.5% (w/w) of each crude enzyme extract, achieved greater hydrolysis efficiency than did either enzyme crude individually at an equivalent dose (1%, w/w). Relative to an equivalent dose of E. parvum-derived enzymes, the enzymatic blend yielded glucose, xylose, and arabinose levels that were 35.97, 9.26, and 6.21% higher, respectively, with FA yield showing no significant difference. Similarly, relative to A. niger-derived enzyme yields, the enzymatic blend yielded glucose, xylose, and arabinose levels that were 6.52, 16.08, and 22.61% higher, while FA yield showed no significant difference either. Table 2 compiles the cellulolytic and hemicellulolytic activity profiles for these crude enzyme preparations from A. niger and E. parvum. E. parvum enzyme extracts exhibited higher levels of hemicellulase activity (including xylanase and arabinofuranosidase), whereas A. niger enzyme extracts exhibited more robust cellulase activity (including filter paper, CMC-Na, and β-glucosidase activity). The activities of xylanase and arabinofuranosidase from E. parvum respectively reached 53.4 U/mL and 76.8 U/mL, while that from A. niger were only 43.9 U/mL and 38.3 U/mL, respectively. On the other hand, the activities of filter paper, CMC-Na, and β-glucosidase from E. parvum reached 0.1 U/mL, 15.7 U/mL and 0.7 U/mL, whereas that from A. niger were significantly higher (0.2 U/mL, 46.3 U/mL and 3.8 U/mL, respectively). Both of these fungal enzyme preparations exhibited other accessory hydrolase activities for other side groups, including acetyl xylan esterase, FA esterase, and glucuronoyl esterase activity levels. The synergistically enhanced enzymatic yields of xylose and arabinose might be partly due to different glycoside hydrolase (GH) families which the related enzyme components were classified in the crude enzymes from A. niger and E. parvum. Such enhanced enzymatic hydrolysis appears to be attributable to the complementary activities of enzymes with different action modes and substrate specificities derived from these two fungi. Cooperative interactions between two or more hydrolytic components such that the product of one enzymatic reaction can serve as a substrate for another, produce combined total effects that are more than the sum of the effects of the components individually (E.M. Visser, et al., 2013; Saini et al., 2016). For example, α-L-arabinofuranosidases can be divided into GH 43 and GH 51. GH 51 shows enzyme activity on Xylp (D-xylopyranosyl residue) monosubstituted by Araf at either position 2 or 3, while GH 43 is specific for doubly arabinosylated Xylp from which they selectively liberate only the α-1,3-linked Araf, leaving the α-1,2-linked Araf on the main chain (Sørensen et al., 2006; Sørensen et al., 2007b). As WB xylan is highly substituted with α-L-arabinofuranosyl residues singly attached to C(O)-2/C(O)-3, or doubly linked to both C(O)-2,3 of the xylose residues, the mutual action of these two groups of α-L-arabinofuranosidases can facilitate the synergistic debranching of all the α-L-arabinofuranosyls from xylan backbone and the complete enzymatic degradation of arabinoxylan into monosaccharides by the subsequent synergy action of endo-xylanase and β-xylosidase (Sørensen et al., 2006; Sørensen et al., 2007b). On the other hand, all the different groups of FA esterases can liberate trans-ferulic acid from 5-O-feruloylated Araf (Biely et al., 2016). Furthermore, generally, trans-ferulic acid is terminally positioned on arabinofuranosyl in the short heterogeneous side chains or on the arabinofuranosyl moiety, and mainly exists in the form of 5-O-feruloylated Araf (Saulnier et al., 1995; Agger et al., 2010). These might cause no significant synergistic enhancement of enzymatic ferulic acid yield using the enzyme blends as shown in Figure 2. Therefore, the crude enzymatic blend may thus be better suited to overall improvements in hydrolytic efficiency at a given enzyme dosage with concomitant reductions in costs.
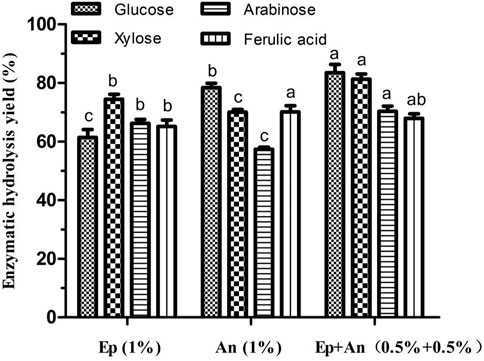
FIGURE 2. Effects of individual or blended enzyme on the enzymatic hydrolysis of wheat bran autohydrolysis residue. Ep, crude enzyme from E. parvum; An, crude enzyme from A. niger. Enzymatic hydrolysis yield of the same product between different treatments were conducted for one way analysis of variance (ANOVA) and multiple comparisons, where different lowercase letters indicate significant difference at p < 0.05 probability level.
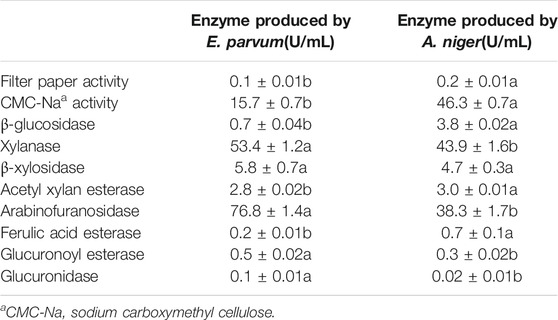
TABLE 2. Activity profiles of the crude enzymes from solid state fermentation by E. parvum and A. oryzae. The activities of crude enzyme extracts from E. parvum and A. niger were conducted for one way analysis of variance (ANOVA) and multiple comparisons, where different lowercase letters indicate significant difference at p < 0.05 probability level.
The Effects of Different Enzyme Blends Dosages on Hydrolytic Efficiency
The effects of different A. niger and E. parvum enzyme blends (0.25–1%, w/w) on WBAR enzymatic hydrolysis were next assessed. Glucose, arabinose, xylose, and FA yields rose with increasing enzyme dosage (Figure 3). The yields of glucose, xylose, arabinose, and FA were respectively 69.26, 65.79, 57.82 and 62.78% with an addition of 0.25% enzyme dose. An enzyme dose of 0.5% was associated with glucose yield of 83.46%, xylose yield of 81.28%, arabinose yield of 70.34% and FA yield of 67.89%, achieving respective 20.50, 23.54, 21.65, and 8.14% improvements in glucose, xylose, arabinose, and FA yields relative to a 0.25% dose. However, these yields were respectively 86.37, 87.58, 76.89 and 69.37% when using 1% enzyme dose, with xylose and arabinose only respectively increased by 7.75 and 9.31%, yet glucose and FA yields displaying no significant difference relative to 0.5% dose. This suggested that the 0.5% dose was close to the saturation point. Besides, when additional FAE (1 U/g substrate) was added to this enzymatic mixture, FA and arabinose yields significantly rose by 19.10 and 14.07%, respectively. Arabinoxylan is heterogeneous and exhibits extensive covalent cross-linking between arabinofuranosyl and feruloyl residues. FAE was able to disrupt these linkages, thereby releasing arabinose and FA. Final respective glucose, xylose, arabinose, and FA yields were 84.98, 84.74, 80.24, and 80.86% of input. Reisinger et al. (Reisinger et al., 2013) previously reported glucose, xylose, and arabinose yields of 68, 55, and 48%, respectively, relative to raw wheat bran following hydrothermal treatment (30 min at 160°C) and enzymatic treatment with a mixture of 2% cellulase and 0.52% xylanase (w/w). Jiang et al. (Jiang and Guo, 2016) were only able to achieve respective glucose, xylose, and arabinose yields of 6, 12, and 1.5% when processing wheat bran via steam explosion (1.0 Mpa, 120 s) despite the application of high xylanase and cellulase doses (3% each, w/w). As such, these findings suggest that the enzymatic blend used in the present study can achieve hydrolytic outcomes superior to those in prior studies relying on commercial enzymes.
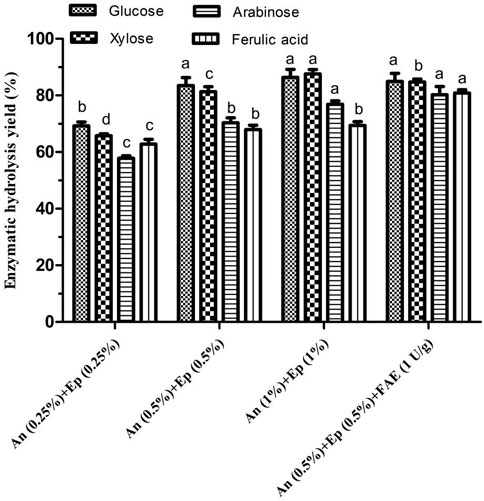
FIGURE 3. Effects of different blended enzyme dosages on the enzymatic hydrolysis of wheat bran autohydrolysis residue. Ep, crude enzyme from E. parvum; An, crude enzyme from A. niger; FAE, ferulic acid esterase. Enzymatic hydrolysis yield of the same product between different treatments were conducted for one way analysis of variance (ANOVA) and multiple comparisons, where different lowercase letters indicate significant difference at p < 0.05 probability level.
Overall Mass Balance
The overall composition of and mass balance for 1,000 g of dried WBAR over the course of the enzymatic hydrolysis process is outlined in Figure 4. Enzymatic hydrolysis was conducted at 50°C for 72 h using an enzymatic blend (0.5% derived from A. niger and 0.5% derived from E. parvum with an addition of 1 U/g FAE). This hydrolytic processing step yielded 325 g glucose, 237 g xylose, 116 g arabinose, and 6 g FA. Following the use of an HP-20 resin column for further FA enrichment, the final FA yield was 5 g, corresponding to 62.5% of the esterified FA present within the initial WBAR input. Levels of glucose, xylose, and arabinose in the resultant filtrate were 308 g, 224 g, and 109 g, respectively, corresponding to 79.0, 78.2, and 73.2% of the levels present within the initial WBAR input, with no FA remaining detectable within this filtrate. As such, FA can be reliably enriched from enzymatic hydrolysates through resin-based adsorption and subsequent ethanol elution.
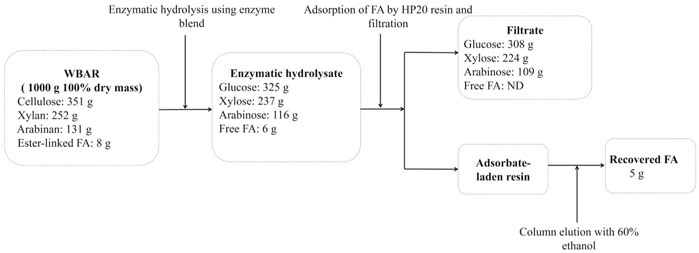
FIGURE 4. Mass balance of the monosaccharides and ferulic acid from wheat bran autohydrolysis residue. FA, ferulic acid; ND, not detected.
Conclusion
Herein, an enzyme blend derived from A. niger and E. parvum was used to achieve the enzymatic hydrolysis of wheat bran autohydrolysis residue, thereby achieving ferulic acid and monosaccharide co-production with high efficiency. Final ferulic acid recovery rates were as high as 62.5% of the levels of esterified ferulic acid present within the starting WBAR input, with limited monosaccharide loss following additional HP-20 resin-mediated separation. Together, these results suggest that wheat bran offers value as a biomass source that can be leveraged to prepare ferulic acid and to facilitate efficient enzymatic saccharification following autohydrolysis pretreatment.
Data Availability Statement
The raw data supporting the conclusions of this article will be made available by the authors, without undue reservation.
Author Contributions
ZL: Methodology, Formal analysis, Investigation. ES: Investigation, Validation, Resources. FM: Investigation, Data curation. XZ: Formal analysis. KJ: Funding acquisition, Writing - original draft, Supervision, Conceptualization, Writing - review and editing. All authors have read and agreed to the published version of the manuscript.
Funding
The works were supported by Scientific Research Fund of Zhejiang Provincial Education Department (Y202146043), and the National Financial Support of Undergraduate Training Program for Innovation (202113023019).
Conflict of Interest
The authors declare that the research was conducted in the absence of any commercial or financial relationships that could be construed as a potential conflict of interest.
Publisher’s Note
All claims expressed in this article are solely those of the authors and do not necessarily represent those of their affiliated organizations, or those of the publisher, the editors and the reviewers. Any product that may be evaluated in this article, orclaim that may be made by its manufacturer, is not guaranteed or endorsed by the publisher.
Acknowledgments
We sincerely thank for the support of the Advanced Analysis and Testing Center of Nanjing Forestry University.
References
Agger, J., Viksø-Nielsen, A., and Meyer, A. S. (2010). Enzymatic Xylose Release from Pretreated Corn Bran Arabinoxylan: Differential Effects of Deacetylation and Deferuloylation on Insoluble and Soluble Substrate Fractions. J. Agric. Food Chem. 58, 6141–6148. doi:10.1021/jf100633f
Aguedo, M., Fougnies, C., Dermience, M., and Richel, A. (2014). Extraction by Three Processes of Arabinoxylans from Wheat Bran and Characterization of the Fractions Obtained. Carbohydr. Polym. 105, 317–324. doi:10.1016/j.carbpol.2014.01.096
Batalha, L. A. R., Han, Q., Jameel, H., Chang, H.-m., Colodette, J. L., and Borges Gomes, F. J. (2015). Production of Fermentable Sugars from Sugarcane Bagasse by Enzymatic Hydrolysis after Autohydrolysis and Mechanical Refining. Bioresour. Techn. 180, 97–105. doi:10.1016/j.biortech.2014.12.060
Bhattacharya, A., Ruthes, A., Vilaplana, F., Karlsson, E. N., Adlecreutz, P., and Stålbrand, H. (2020). Enzyme Synergy for the Production of Arabinoxylo-Oligosaccharides from Highly Substituted Arabinoxylan and Evaluation of Their Prebiotic Potential. Lwt 131, 109762. doi:10.1016/j.lwt.2020.109762
Biely, P., Singh, S., and Puchart, V. (2016). Towards Enzymatic Breakdown of Complex Plant Xylan Structures: State of the Art. Biotechnol. Adv. 34, 1260–1274. doi:10.1016/j.biotechadv.2016.09.001
Cripwell, R., Favaro, L., Rose, S. H., Basaglia, M., Cagnin, L., Casella, S., et al. (2015). Utilisation of Wheat Bran as a Substrate for Bioethanol Production Using Recombinant Cellulases and Amylolytic Yeast. Appl. Energ. 160, 610–617. doi:10.1016/j.apenergy.2015.09.062
Ferri, M., Happel, A., Zanaroli, G., Bertolini, M., Chiesa, S., Commisso, M., et al. (2020). Advances in Combined Enzymatic Extraction of Ferulic Acid from Wheat Bran. New Biotechnol. 56, 38–45. doi:10.1016/j.nbt.2019.10.010
Gullón, B., Gullón, P., Tavaria, F., Pintado, M., Gomes, A. M., Alonso, J. L., et al. (2014). Structural Features and Assessment of Prebiotic Activity of Refined Arabinoxylooligosaccharides from Wheat Bran. J. Funct. Food. 6, 438–449. doi:10.1016/j.jff.2013.11.010
Hu, Y., Yan, B., Stephen Chen, Z., Wang, L., Tang, W., and Huang, C. (2022). Recent Technologies for the Extraction and Separation of Polyphenols in Different Plants: A Review. J. Renew. Mater. 10, 1–20. doi:10.32604/jrm.2022.018811
Huang, C., Jiang, X., Shen, X., Hu, J., Tang, W., Wu, X., et al. (2022). Lignin-enzyme Interaction: A Roadblock for Efficient Enzymatic Hydrolysis of Lignocellulosics. Renew. Sustain. Energ. Rev. 154, 111822. doi:10.1016/j.rser.2021.111822
Jiang, K., Li, L., Long, L., and Ding, S. (2016). Comparison of Alkali Treatments for Efficient Release of P-Coumaric Acid and Enzymatic Saccharification of Sorghum Pith. Bioresour. Techn. 207, 1–10. doi:10.1016/j.biortech.2016.01.116
Jiang, K., Li, L., Long, L., and Ding, S. (2018). Comprehensive Evaluation of Combining Hydrothermal Pretreatment (Autohydrolysis) with Enzymatic Hydrolysis for Efficient Release of Monosaccharides and Ferulic Acid from Corn Bran. Ind. Crops Prod. 113, 348–357. doi:10.1016/j.indcrop.2018.01.047
Jiang, S.-T., and Guo, N. (2016). The Steam Explosion Pretreatment and Enzymatic Hydrolysis of Wheat Bran. Energy Sourc. A: Recovery, Utilization, Environ. Effects. 38, 295–299. doi:10.1080/15567036.2012.744118
Kaur, A., Singh, B., Yadav, M. P., Bhinder, S., and Singh, N. (2021). Isolation of Arabinoxylan and Cellulose-Rich Arabinoxylan from Wheat Bran of Different Varieties and Their Functionalities. Food Hydrocolloids. 112, 106287. doi:10.1016/j.foodhyd.2020.106287
Khalili, F., and Amiri, H. (2020). Integrated Processes for Production of Cellulosic and Hemicellulosic Biobutanol from Sweet Sorghum Bagasse Using Autohydrolysis. Ind. Crops Prod. 145, 111918. doi:10.1016/j.indcrop.2019.111918
Li, D., Rui, Y.-x., Guo, S.-d., Luan, F., Liu, R., and Zeng, N. (2021). Ferulic Acid: A Review of its Pharmacology, Pharmacokinetics and Derivatives. Life Sci. 284, 119921. doi:10.1016/j.lfs.2021.119921
Long, L., Ding, D., Han, Z., Zhao, H., Lin, Q., and Ding, S. (2016). Thermotolerant Hemicellulolytic and Cellulolytic Enzymes from Eupenicillium parvum 4-14 Display High Efficiency upon Release of Ferulic Acid from Wheat Bran. J. Appl. Microbiol. 121, 422–434. doi:10.1111/jam.13177
Ma, M.-G., Jia, N., Zhu, J.-F., Li, S.-M., Peng, F., and Sun, R.-C. (2012). Isolation and Characterization of Hemicelluloses Extracted by Hydrothermal Pretreatment. Bioresour. Techn. 114, 677–683. doi:10.1016/j.biortech.2012.03.0410.1016/j.biortech.2012.03.048
Mandalari, G., Faulds, C. B., Sancho, A. I., Saija, A., Bisignano, G., LoCurto, R., et al. (2005). Fractionation and Characterisation of Arabinoxylans from Brewers' Spent Grain and Wheat Bran. J. Cereal Sci. 42, 205–212. doi:10.1016/j.jcs.2005.03.001
Mathew, S., and Abraham, T. E. (2004). Ferulic Acid: an Antioxidant Found Naturally in Plant Cell walls and Feruloyl Esterases Involved in its Release and Their Applications. Crit. Rev. Biotechnol. 24, 59–83. doi:10.1080/07388550490491467
Mathew, S., Karlsson, E. N., and Adlercreutz, P. (2017). Extraction of Soluble Arabinoxylan from Enzymatically Pretreated Wheat Bran and Production of Short Xylo-Oligosaccharides and Arabinoxylo-Oligosaccharides from Arabinoxylan by Glycoside Hydrolase Family 10 and 11 Endoxylanases. J. Biotechnol. 260, 53–61. doi:10.1016/j.jbiotec.2017.09.006
Murlidhar Sonkar, R., Savata Gade, P., Bokade, V., Mudliar, S. N., and Bhatt, P. (2021). Ozone Assisted Autohydrolysis of Wheat Bran Enhances Xylooligosaccharide Production with Low Generation of Inhibitor Compounds: A Comparative Study. Bioresour. Techn. 338, 125559. doi:10.1016/j.biortech.2021.125559
Nair, R. B., Lundin, M., Brandberg, T., Lennartsson, P. R., and Taherzadeh, M. J. (2015). Dilute Phosphoric Acid Pretreatment of Wheat Bran for Enzymatic Hydrolysis and Subsequent Ethanol Production by Edible Fungi Neurospora Intermedia. Ind. Crops Prod. 69, 314–323. doi:10.1016/j.indcrop.2015.02.038
Palmarola-Adrados, B., Chotěborská, P., Galbe, M., and Zacchi, G. (2005). Ethanol Production from Non-starch Carbohydrates of Wheat Bran. Bioresour. Techn. 96, 843–850. doi:10.1016/j.biortech.2004.07.004
Peng, F., Peng, P., Xu, F., and Sun, R.-C. (2012). Fractional Purification and Bioconversion of Hemicelluloses. Biotechnol. Adv. 30, 879–903. doi:10.1016/j.biotechadv.2012.01.018
Reisinger, M., Tirpanalan, Ö., Prückler, M., Huber, F., Kneifel, W., and Novalin, S. (2013). Wheat Bran Biorefinery - A Detailed Investigation on Hydrothermal and Enzymatic Treatment. Bioresour. Techn. 144, 179–185. doi:10.1016/j.biortech.2013.06.088
Rose, D. J., and Inglett, G. E. (2010). Production of Feruloylated Arabinoxylo-Oligosaccharides from maize (Zea mays) Bran by Microwave-Assisted Autohydrolysis. Food Chem. 119, 1613–1618. doi:10.1016/j.foodchem.2009.09.053
Saini, J. K., Singhania, R. R., Satlewal, A., Saini, R., Gupta, R., Tuli, D., et al. (2016). Improvement of Wheat Straw Hydrolysis by Cellulolytic Blends of Two Penicillium Spp. Renew. Energ. 98, 43–50. doi:10.1016/j.renene.2016.01.025
Sakamoto, T., Ogura, A., Inui, M., Tokuda, S., Hosokawa, S., Ihara, H., et al. (2011). Identification of a GH62 α-l-arabinofuranosidase Specific for Arabinoxylan Produced by Penicillium chrysogenum. Appl. Microbiol. Biotechnolappl. Microbiol. Biot. 90, 137–146. doi:10.1007/s00253-010-2988-2
Sánchez-Bastardo, N., Romero, A., and Alonso, E. (2017). Extraction of Arabinoxylans from Wheat Bran Using Hydrothermal Processes Assisted by Heterogeneous Catalysts. Carbohydr. Polym. 160, 143–152. doi:10.1016/j.carbpol.2016.12.035
Saulnier, L., Vigouroux, J., and Thibault, J.-F. (1995). Isolation and Partial Characterization of Feruloylated Oligosaccharides from maize Bran. Carbohydr. Res. 272, 241–253. doi:10.1016/0008-6215(95)00053-V
Si, D., Shang, T., Liu, X., Zheng, Z., Hu, Q., Hu, C., et al. (2020). Production and Characterization of Functional Wheat Bran Hydrolysate Rich in Reducing Sugars, Xylooligosaccharides and Phenolic Acids. Biotechnol. Rep. 27, e00511. doi:10.1016/j.btre.2020.e00511
Silva-Fernandes, T., Duarte, L. C., Carvalheiro, F., Loureiro-Dias, M. C., Fonseca, C., and Gírio, F. (2015). Hydrothermal Pretreatment of Several Lignocellulosic Mixtures Containing Wheat Straw and Two Hardwood Residues Available in Southern Europe. Bioresour. Techn. 183, 213–220. doi:10.1016/j.biortech.2015.01.059
Sluiter, A., Hames, B., Ruiz, R., Scarlata, C., Sluiter, J., Templeton, D., et al. (2005). Determination of Structural Carbohydrates and Lignin in Biomass. Denver, Colorado: Laboratory Analytical Procedure, the National Renewable Energy Laboratory Biomass Analysis Technology (NREL BAT) Team.
Sørensen, H. R., Jørgensen, C. T., Hansen, C. H., Jørgensen, C. I., Pedersen, S., and Meyer, A. S. (2006). A Novel GH43 α-l-arabinofuranosidase from Humicola Insolens: Mode of Action and Synergy with GH51 α-l-arabinofuranosidases on Wheat Arabinoxylan. Appl. Microbiol. Biotechnol. 73, 850–861. doi:10.1007/s00253-006-0543-y
Sørensen, H. R., Pedersen, S., Jorgensen, C. T., and Meyer, A. S. (2007a). Enzymatic Hydrolysis of Wheat Arabinoxylan by a Recombinant "Minimal" Enzyme Cocktail Containing β-Xylosidase and Novel Endo-1,4-β-Xylanase and α-L-Arabinofuranosidase Activities. Biotechnol. Prog. 23, 100–107. doi:10.1021/bp0601701
Sørensen, H. R., Pedersen, S., and Meyer, A. S. (2007b). Synergistic Enzyme Mechanisms and Effects of Sequential Enzyme Additions on Degradation of Water Insoluble Wheat Arabinoxylan. Enzyme Microb. Techn. 40, 908–918. doi:10.1016/j.enzmictec.2006.07.026
Sørensen, H. R., Pedersen, S., Viksø-Nielsen, A., and Meyer, A. S. (2005). Efficiencies of Designed Enzyme Combinations in Releasing Arabinose and Xylose from Wheat Arabinoxylan in an Industrial Ethanol Fermentation Residue. Enzyme Microb. Techn. 36, 773–784. doi:10.1016/j.enzmictec.2005.01.007
Tang, W., Wu, X., Huang, C., Ling, Z., Lai, C., and Yong, Q. (2021). Revealing Migration Discipline of Lignin during Producing Fermentable Sugars from Wheat Straw through Autohydrolysis. Ind. Crops Prod. 171, 113849. doi:10.1016/j.indcrop.2021.113849
Visser, E. M., Falkoski, D. L., de Almeida, M. N., Maitan-Alfenas, G. P., and Guimarães, V. M. (2013). Production and Application of an Enzyme Blend from Chrysoporthe Cubensis and Penicillium pinophilum with Potential for Hydrolysis of Sugarcane Bagasse. Bioresour. Techn. 144, 587–594. doi:10.1016/j.biortech.2013.07.015
Wang, Z.-K., Huang, C., Zhong, J.-L., Wang, Y., Tang, L., Li, B., et al. (2021). Valorization of Chinese hickory Shell as Novel Sources for the Efficient Production of Xylooligosaccharides. Biotechnol. Biofuels. 14, 226. doi:10.1186/s13068-021-02076-9
Keywords: wheat bran autohydrolysis residue, enzymatic hydrolysis, monosaccharide, ferulic acid, enzyme blend
Citation: Liu Z, Shi E, Ma F, Zhou X and Jiang K (2022) Improved Release of Monosaccharides and Ferulic Acid Using Enzyme Blends From Aspergillus Niger and Eupenicillium Parvum. Front. Bioeng. Biotechnol. 9:814246. doi: 10.3389/fbioe.2021.814246
Received: 13 November 2021; Accepted: 17 December 2021;
Published: 27 January 2022.
Edited by:
Helen Treichel, Universidade Federal da Fronteira Sul, BrazilReviewed by:
Anne S. Meyer, Technical University of Denmark, DenmarkHéctor A. Ruiz, Universidad Autónoma de Coahuila, Mexico
Copyright © 2022 Liu, Shi, Ma, Zhou and Jiang. This is an open-access article distributed under the terms of the Creative Commons Attribution License (CC BY). The use, distribution or reproduction in other forums is permitted, provided the original author(s) and the copyright owner(s) are credited and that the original publication in this journal is cited, in accordance with accepted academic practice. No use, distribution or reproduction is permitted which does not comply with these terms.
*Correspondence: Kankan Jiang, jiangkankan@126.com