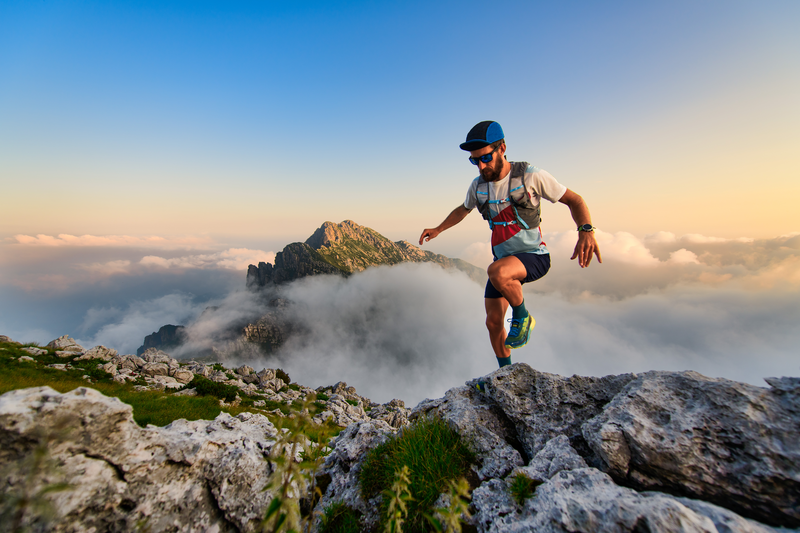
95% of researchers rate our articles as excellent or good
Learn more about the work of our research integrity team to safeguard the quality of each article we publish.
Find out more
ORIGINAL RESEARCH article
Front. Bioeng. Biotechnol. , 22 November 2021
Sec. Biomaterials
Volume 9 - 2021 | https://doi.org/10.3389/fbioe.2021.806151
This article is part of the Research Topic Biomaterials In Asia View all 36 articles
The coverage of stents with healthy endothelium is crucial to the success of cardiovascular stent implantation. Immobilizing bioactive molecules on stents is an effective strategy to generate such stents. Glycogen synthase kinase-3β inhibitor (GSKi) is a bioactive molecule that can effectively accelerate vascular endothelialization. In this work, GSKi was covalently conjugated on 316L stainless steel through polydopamine to develop a stable bioactive surface. Fourier transform infrared spectroscopy (FTIR), scanning electron microscopy (SEM) and water contact angle results revealed the successful introduction of GSKi onto 316L stainless steel. The GSKi coating did not obviously affect the hemocompatibility of plates. The adhesion and proliferation of human coronary artery endothelial cells (HCAECs) on stainless steel was significantly promoted by the addition of GSKi. In summary, this work provides a universal and stable strategy of immobilizing GSKi on the stent surface. This method has the potential for widespread application in the modification of vascular stents.
Coronary artery disease (CAD), especially myocardial infarction, is becoming a major cause of death worldwide and is responsible for more than 17.5 million deaths annually (Cui et al., 2018). Drug-eluting stents (DESs) have been successfully used in the treatment of CAD. Despite the success of DESs, a previous study demonstrated that in-stent restenosis (ISR) and late stent thrombosis (LST) represent obstacles to long-term application in the clinic (Otsuka et al., 2012; Liang et al., 2016). It was soon realized that the occurrences of ISR and LST are ultimately due to endothelial injury or the re-endothelialization delay of the stents (Kakinoki et al., 2018). A functionally intact vessel endothelium not only prevents thrombosis but also mediates intimal hyperplasia. As a result, re-endothelialization on the stent effectively prevents ISR and LST (Woods and Marks, 2004). Considerable efforts in the surface modification of stents have been made to improve their ability to rapidly re-endothelialize (Avci-Adali et al., 2010; Qi et al., 2014; Pang et al., 2015). However, the selection of appropriate bioactive molecules and the strategy of immobilizing molecules on stents still need to be investigated.
Glycogen synthase kinase-3β (GSK3β) acts as a nodal point of converging signaling pathways in endothelial cells to adjust vessel growth. It is a pro-apoptotic kinase given that it’s overexpression makes cells sensitive to apoptosis. The inhibitor of GSK3β (GSKi) increases the expression of β-catenin in endothelial cells and promote the expression of vascular endothelial growth factor (VEGF) (Doble and Woodgett, 2003; Skurk et al., 2005; Choi et al., 2007), which is an important regulatory molecule of endothelial cells and plays an important role in vascular development (Ferrara et al., 2003; Eichmann and Simons, 2012). Zhang et al. found that GSKi had a protective effect on endothelial cells by phosphorylating inactivated GSK3β and inhibiting endothelial cell apoptosis (Zhang et al., 2004). Benjamin Hibbert et al. treated endothelial progenitor cells with GSKi to promote the secretion of VEGF and then injected them into immunocompromised mice. They found that GSKi promoted re-endothelialization and reduced neointimal formation after arterial injury (Hibbert et al., 2009). These exciting results indicated that GSKi can be used to accelerate vascular endothelialization after coronary stent implantation. However, the clinical feasibility of transplantation in operant cells in vitro may be limited given that the process is labor intensive and impractical.
To date, only a few studies have reported on GSKi-modified stents. Ma et al. coated stents with a mixture of lubricating jelly and active compounds [GSKi and rapamycin (a key DES agent)]. The experimental results revealed that GSKi-coated stents enhance the adhesion of endothelial progenitor cells and efficiently ameliorate the vascular response to stent implantation. Furthermore, GSKi-coated stents can redeem the deleterious endothelial effects of rapamycin-coated stents (Ma et al., 2010). However, this method of coating stents may lead to weak adhesion between the coating and stents and the rapid loss of active compounds caused by simple blending.
In recent years, mussel-inspired polydopamine coatings have attracted researchers’ attention because they have outstanding ability to bind strongly to almost all types of substrates (Lee et al., 2006; Lee et al., 2007a; Lee et al., 2007b; Luo et al., 2013; Zhang et al., 2014; Wu et al., 2016; Zhang et al., 2016; Zhang et al., 2019). Polydopamine coatings can be realized through the simple dip-coating step of the substrates in the aqueous solution of dopamine and provides secondary reactivity for conjugating bioactive molecules. The reaction conditions are mild and easy to perform (Madhurakkat Perikamana et al., 2015; Yang et al., 2015). The bioactive molecules are connected on the material surface by a stable covalent bond between the sulydryl/amine groups of the molecule and the phenolic hydroxyl/quinone groups of polydopamine (Lynge et al., 2011; Qi et al., 2014).
In this study, GSKi was immobilized onto 316L stainless steel plates (316L-SS) by polydopamine coating. The surfaces of the modified 316L-SS were observed by SEM and water contact angle. The hemocompatibility of the modified 316L-SS was studied by blood clotting time tests and platelet adhesion. The viability, adhesion, proliferation and morphology of human coronary artery endothelial cells (HCAECs) on the modified plates were examined using in vitro cell experiments.
The 316L-SS substrates were ultrasonically washed with acetone, ethanol and distilled water for 5 min. The preparation procedure of functionalized surfaces was as follows: 316L-SS was immersed in a 2 mg/L dopamine hydrochloride (Aladdin, United States) solution (dissolved in 10 mM Tris buffer, pH 8.5) for 24 h at room temperature and then rinsed with 10 mM Tris buffer (pH 8.5) thrice to remove the poorly bound polydopamine. The obtained polydopamine-modified stainless steel plates were air-dried and labeled PDAM-SS.
Then, 2 ml GSKi (CHIR-98014, Abmole, United States) solution (2.5 mg/ml DMSO solution) was added to 48 ml distilled water to obtain the supersaturated aqueous solution of GSKi. Then, PDAM-SS was placed into a culture plate and incubated with the supersaturated aqueous solution of GSKi at room temperature for 24 h. Finally, the GSKi-modified samples were rinsed thrice with distilled water and air-dried (labeled GSKi-SS).
Fourier transform infrared spectroscopy (FTIR) (Bruker ALPHA) with the diffuse reflectance mode was used to determine the chemical structure of 316L-SS, PDAM-SS and GSKi-SS.
The surface morphologies of 316L-SS, PDAM-SS and GSKi-SS were observed using a Gemini 300/VP field emission scanning electron microscope (Zeiss, Germany).
The wettability studies were performed using a contact angle analyzer (DSA10-MK2, Krüss, Germany). The measurement was performed at five different points on each stainless steel plate to obtain the average contact angle.
Platelet-poor plasma (PPP) was obtained by centrifuging fresh human whole blood at 3,000 rpm for 15 min. The samples were incubated with 500 μL PPP in a 24-well plate for 30 min at 37°C. The dotting time of incubated PPP was detected using a coagulometer (ACL TOP 500, Werfen, United States).
Fresh peripheral blood was obtained from a healthy human volunteer. Platelet-rich plasma (PRP) was obtained by centrifugation of whole blood at 1,500 rpm for 15 min. The samples were placed in a 24-well plate, and 100 μL PRP was added to each sample surface. After incubation at 37°C for 2 h, the PRP on the sample was absorbed and rinsed with PBS thrice. The rinsed samples were then fixed in 2.5% glutaraldehyde for 2 h, rinsed again with PBS thrice, and dehydrated in graded ethanol solution (50, 75, 90, and 100%). Finally, the samples were dried and observed by SEM (Gemini 300/VP field emission scanning electron microscope, Zeiss, Germany).
Complete DMEM was incubated with different samples in a 24-well plate for 24 h to obtain conditioned media, and media without substrate was used as a control. HCAECs were cultured at a density of 3,000 cells per well in a 96-well plate and cultured with normal DMEM complete media overnight. The next day, the media was changed to conditioned media and cultured for 24 h. Then, cell viability was detected using the Cell Counting Kit-8 (CCK-8, Dojindo) assay.
HCAECs were seeded onto different samples at a density of 15,000 cells per well in a 24-well plate. After 2 h of incubation, the cells were fixed in 4% paraformaldehyde for 20 min and blocked with PBS containing 5% BSA for 30 min. Then, 4′,6-diamidino-2-phenylindole (DAPI, 1 μg/ml, Sigma) was used to stain nuclei for 5 min. Then, the adhesion of cells was observed by a confocal laser microscope (LSM 780, Zeiss, Germany). Six images in each channel were obtained for each sample. Image-Pro Plus software was used to calculate the number of cells.
A CCK-8 assay was performed to investigate cell proliferation in different samples. After 24 or 48 h of incubation, 50 μL CCK8 was added to 500 μL culture media and incubated with cells for 1 h at 37°C. One hundred microliters of supernatant was transferred to a 96-well plate, and the optical density was measured with a microplate reader at 450 nm wavelength after the instrument was blanked with PBS solution. Three replicates were prepared for each sample.
Cell morphology was determined by DAPI and fluorescein isothiocyanate (FITC)-labeled phalloidin (Sigma) staining. These fluorescent dyes are indicators of the cell nucleus and skeleton. Briefly, HCAECs were incubated on different samples for 2 or 24 h. Then, samples were fixed in 4% paraformaldehyde and blocked with PBS containing 5% BSA for 20 and 30 min, respectively. The cytoskeleton was labeled with 300 nM FITC-labeled phalloidin for 30 min followed by washing with PBS containing 0.1% Tween 20 thrice. DAPI (1 μg/ml) was used to stain nuclei for 5 min. Then, the cells were mounted on slides in glycerol with cover slips and observed with a confocal laser microscope.
All numerical data are presented as the mean ± SD (n ≥ 3). Statistical analysis was performed using one-way ANOVA. If the p-value was less than 0.05 (*p < 0.05), the differences were considered statistically significant.
The FTIR results in Figure 1 show that 316L-SS has no obvious absorption peak. The characteristic adsorption peaks of PDAM-SS at ∼3,300 cm−1 (stretching vibration of phenolic O-H and N-H), 1,603 cm−1 (superposition of N-H bending vibration and stretching vibration of aromatic ring), 1,513 cm−1 (N-H shearing vibration), and 1,290 cm−1 (C-O stretching vibration) proved the existence of polydopamine layer on 316L stainless steel. Compared with PDAM-SS, GSKi-SS exhibits many changes in the FTIR spectrum. The peak at 1,541 cm−1 was assigned to amide II (N-H bending vibration), which indicated that GSKi was successfully immobilized on PDAM-SS. The catecholic groups in polydopamine coating offered the active sites to immobilize GSKi molecules.
A schematic diagram of the surface modification procedure of 316L-SS is shown in Figure 2. After 24 h of immersion in dopamine solution, the 31-SS substrates turned light brown in our experiments. The color of PDAM-SS did not change much after immersion in GSKi solution for an additional 24 h. Under weakly alkaline conditions, dopamine self-polymerized and formed a light brown polydopamine coating on the 316L-SS surface. The polydopamine layer is rich in catechol groups. These groups are easily oxidized to phenolic hydroxyl/quinone groups and undergo a Schiff base reaction or a Michael addition reaction with the primary amino group (-NH2) or secondary amino group (−NH) of GSKi (Yang et al., 2014; Madhurakkat Perikamana et al., 2015). Then, GSKi was covalently immobilized on the PDAM-SS surface through polydopamine coating.
The SEM results of 31-SS, PDAM-SS and GSKi-SS are shown in Figure 3. First, the stripes left on the surface of the original 316L-SS were caused by the polishing process. After 24 h of polydopamine coating, the surface morphology of 316L-SS changed significantly. Some nanoscale aggregation was observed on the PDAM-SS surface. However, the surface morphology changed slightly after GSKi was immobilized on the surface of PDAM-SS.
Dopamine is polymerized under weakly alkaline conditions (pH 8.5), and polymerized dopamine can be spontaneously deposited on many types of substrates, such as metals and polymers (Lee et al., 2007a; Yang et al., 2015). After the long-term coating process, polymerized dopamine changed the surface morphology of 316L-SS. It formed a thin adhesive layer and even appeared as nanoaggregates or clumps on the surface of stainless steel plates, which was also reported in other studies (Yang et al., 2012b; Hong et al., 2013; Yang et al., 2015).
The surface hydrophobicity was evaluated by measuring the water contact angle of each sample. The results of 316L-SS, PDAM-SS and GSKi-SS are 53.9 ± 7.4°, 6.4 ± 1.1° and 40.7 ± 2.3°, respectively. The addition of polydopamine obviously improved the surface hydrophilicity of stainless steel plates. GSKi-SS is more hydrophobic than PDAM-SS.
The large amount of hydrophilic functional groups, such as phenolic hydroxyl groups and amino groups of the polydopamine coating, are potentially responsible for the improved hydrophilicity of PDAM-SS (Yang et al., 2010; Ding et al., 2014). The increased hydrophobicity of GSKi-SS may be due to the connection of GSKi with the phenolic hydroxyl group of the polydopamine coating, which resulted in a significant reduction of the hydrophilic group. The change in contact angle of the three samples indirectly demonstrated the successful coating of polydopamine and GSKi on the 316L-SS surface.
For cardiovascular implant devices, improperly modified materials can trigger thrombus cascade reactions, which lead to implantation failure. APTT is a simple and highly reliable assay for the evaluation of the anticoagulant capacity of material surfaces (Lu et al., 2012). When stents with poor blood compatibility are implanted, the coagulation factor will bind to the stent surface to initiate the endogenous coagulation reaction, and the clotting time will be reduced during APTT detection (Basmadjian et al., 1997). The APTT results of 316L-SS, PDAM-SS, and GSKi-SS are shown in Figure 4. Compared with 316L-SS (31.33 ± 0.55 s), the values of PDAM-SS (31.63 ± 1.95 s) and GSKi-SS (32.3 ± 0.44 s) were not drastically altered. No significant difference was noted among the three groups (p > 0.05), indicating that these groups have similar anticoagulant properties. Therefore, the polydopamine and GSKi coating on 316L-SS did not obviously activate the endogenous coagulation system.
As a key step of thrombosis, platelet adhesion has been widely used to characterize the thrombogenic ability of materials. The adhesion morphology of platelets on materials can be divided into five grades: discoid, dendritic, partially spreading, spreading and aggregation (Goodman et al., 1989). To study the thrombogenic properties of PDAM- and GSKi-modified 316L stainless steel, platelets adhered to these sample surfaces were observed by SEM (as shown in Figure 5). The amount and morphology of platelets were similar in the three groups. All platelets showed discoid or partial dendritic morphology. This SEM result indicated that 316L-SS, PDAM-SS and GSKi-SS had similar anticoagulant properties, which was consistent with the results of the APTT test. Therefore, the polydopamine and GSKi coating on 316L-SS did not obviously activate the endogenous coagulation system.
To explore the neovascularization ability of modified stainless steel plates, it is necessary to study the growth behaviors of HCAECs in the three groups. Figure 6 shows the cell cytotoxicity of 316L-SS, PDAM-SS and GSKi-SS after 24 h of cell culture. The tissue culture plate served as a control. The cell viability of each sample was not significantly different from that of the control group and was greater than 85%. This finding indicated that 316L-SS, PDAM-SS and GSKi-SS had good biocompatibility. The polydopamine and GSKi coating of 316L-SS did not lead to obvious cell cytotoxicity.
The adhesion and proliferation of endothelial cells on stent surfaces is necessary for the re-endothelialization of stents. Figure 7 shows the number of cells attached to different stainless steel samples after 2 h of incubation. The cell adhesion numbers for PDAM-SS and GSKi-SS were significantly greater than that of 316L-SS. No significant difference was noted between PDAM-SS and GSKi-SS. The surface properties of materials, such as topography, wettability and functional groups, play important roles in regulating cell behaviors (Flemming et al., 1999; Alves et al., 2010; Yuan et al., 2018; Amani et al., 2019; Shi et al., 2019). In this study, nanopolydopamine dots that appeared on the surface of PDAM-SS and GSKi-SS were thought to contribute to improving the adhesion of HCAECs. The strong protein adsorption capacity of the polydopamine coating also promoted the adhesion of cells on PDAM-SS (Ku et al., 2010; Yang et al., 2012b). Ma et al. reported that GSKi increases the attachment of endothelial progenitor cells to GSKi-coated stents (Ma et al., 2010). In our experiments, the HCAEC attachment efficiency of GSKi-SS was similar to that of PDAM-SS when cells were cultured for 2 h. The cell adhesion results indicated that PDAM-SS and GSKi-SS provided a more suitable microenvironment for the adhesion of HCAECs than 316L-SS.
FIGURE 7. Cell adhesion numbers on various samples after 2 h of incubation (mean ± SD, ***p < 0.001).
The cell proliferation results of different samples are shown in Figure 8. Compared with 316L-SS, PDAM-SS did not obviously promote HCAEC proliferation until 48 h of culture. For the GSKi-SS group, cell proliferation increased after incubation for 24 h and was further significantly enhanced after 48 h. These results showed that both polydopamine and GSKi coating on stainless steel plates can promote HCAEC proliferation. GSKi more effectively improves cell proliferation than PDAM. GSKi protects endothelial cells by phosphorylating inactivated GSK3β, resulting in the accumulation of β-catenin in the endothelial nucleus, activating the Wnt signaling pathway and inhibiting endothelial cell apoptosis (Zhang et al., 2004). Choi et al. reported that GSKi stimulates VEGF expression in endothelial cells (Choi et al., 2004). In vitro studies we found that VEGF-immobilized surfaces accelerate endothelialization by increasing the adhesion, proliferation and migration of endothelial cells (Shin et al., 2012). Therefore, GSKi-SS yields better cell proliferation results than 316L-SS and PDAM-SS.
FIGURE 8. Proliferation of HCAECs on various samples under serum condition (mean ± SD, *p < 0.05, ***p < 0.001).
The morphology of HCAECs on different samples is shown in Figure 9. After culturing for 2 h, only a few cells adhered to 316L-SS. The number of adherent cells on PDAM-SS and GSKi-SS was significantly increased. Cells on PDAM-SS and GSKi-SS began to spread and showed similar morphological features. After 24 h of culture, the cell numbers obviously increased, and the cells completely spread on all three samples. GSKi-SS showed a significant increase in cell coverage and proliferation compared with PDAM-SS and 316L-SS.
FIGURE 9. Fluorescence images of HCAECs cultured on different samples for 2 and 24 h; the scale bar is 200 μm.
The nano and microscale architecture of material surfaces can modulate endothelial cell structure and function (Leclech et al., 2020). Cells on along the microgrooves on the stainless steel surface in the GSKi-SS samples exhibited a more elongated, unidirectional and polarized morphology than those in the 316L-SS and PDAM-SS groups. These slender HCAECs were thought to not only have stronger proliferative capacity and extracellular matrix secretion but also have a faster migration rate than fully spread cells (Yang et al., 2012a). This fluorescence result was consistent with the cell adhesion and proliferation results shown in Figure 8.
In this study, GSKi was successfully introduced on the surface of 316L stainless steel through polydopamine to form a stable bioactive coating. Blood compatibility tests indicated that the addition of GSKi did not obviously affect the hemocompatibility of 316L-SS. Cell experiments revealed that the GSKi coating significantly promoted the adhesion and proliferation of HCAECs on stainless steel surfaces. Compared with 316L-SS and PDAM-SS, cells on GSKi-SS exhibited a more elongated, unidirectional and polarized morphology along the microgrooves on the stainless steel surface. These results suggested that this surface modification strategy of stable GSKi coating has potential application in the modification of cardiovascular stents.
The original contributions presented in the study are included in the article/Supplementary Material, further inquiries can be directed to the corresponding author.
Writing—original draft preparation, MZ; writing—review and editing, XS; investigation, HS; methodology, DX; software, YG; data curation, XW and JQZ; conceptualization—review and editing, JCZ; All authors have read and agreed to the published version of the manuscript. All authors contributed to manuscript revision and read and approved the submitted version.
This project was supported by the National Key Research and Development Program of China (2016YFC1100701), the Capital Construction Funds within the Provincial Budget (2019C045-6), the Natural Science Foundation of Jilin Province (20200201381JC), the Science Foundation of Jilin Education Department (JJKH20201097KJ), and the Graduate Innovation Fund of Jilin University (101832018C072).
The authors declare that the research was conducted in the absence of any commercial or financial relationships that could be construed as a potential conflict of interest.
All claims expressed in this article are solely those of the authors and do not necessarily represent those of their affiliated organizations, or those of the publisher, the editors and the reviewers. Any product that may be evaluated in this article, or claim that may be made by its manufacturer, is not guaranteed or endorsed by the publisher.
Alves, N. M., Pashkuleva, I., Reis, R. L., and Mano, J. F. (2010). Controlling Cell Behavior through the Design of Polymer Surfaces. Small 6 (20), 2208–2220. doi:10.1002/smll.201000233
Amani, H., Arzaghi, H., Bayandori, M., Dezfuli, A. S., Pazoki‐Toroudi, H., Shafiee, A., et al. (2019). Controlling Cell Behavior through the Design of Biomaterial Surfaces: A Focus on Surface Modification Techniques. Adv. Mater. Inter. 6 (13), 1900572. doi:10.1002/admi.201900572
Avci-Adali, M., Ziemer, G., and Wendel, H. P. (2010). Induction of EPC Homing on Biofunctionalized Vascular Grafts for Rapid In Vivo Self-Endothelialization - A Review of Current Strategies. Biotechnol. Adv. 28 (1), 119–129. doi:10.1016/j.biotechadv.2009.10.005
Basmadjian, D., Sefton, M. V., and Baldwin, S. A. (1997). Coagulation on Biomaterials in Flowing Blood: Some Theoretical Considerations. Biomaterials 18 (23), 1511–1522. doi:10.1016/s0142-9612(97)80002-6
Choi, J.-H., Hur, J., Yoon, C.-H., Kim, J.-H., Lee, C.-S., Youn, S.-W., et al. (2004). Augmentation of Therapeutic Angiogenesis Using Genetically Modified Human Endothelial Progenitor Cells with Altered Glycogen Synthase Kinase-3β Activity. J. Biol. Chem. 279 (47), 49430–49438. doi:10.1074/jbc.M402088200
Choi, S.-E., Kang, Y., Jang, H.-J., Shin, H.-C., Kim, H.-E., Kim, H.-S., et al. (2007). Involvement of Glycogen Synthase Kinase-3β in Palmitate-Induced Human Umbilical Vein Endothelial Cell Apoptosis. J. Vasc. Res. 44 (5), 365–374. doi:10.1159/000102321
Cui, Y., Zhou, F., Wei, L., Song, Q., Tan, J., Zeng, Z., et al. (2018). In Situ Endothelialization Promoted by SEMA4D and CXCL12 for Titanium-Based Biomaterials. Semin. Thromb. Hemost. 44 (1), 070–080. doi:10.1055/s-0037-1605569
Ding, Y., Yang, Z., Bi, C. W. C., Yang, M., Xu, S. L., Lu, X., et al. (2014). Directing Vascular Cell Selectivity and Hemocompatibility on Patterned Platforms Featuring Variable Topographic Geometry and Size. ACS Appl. Mater. Inter. 6 (15), 12062–12070. doi:10.1021/am502692k
Doble, B. W., and Woodgett, J. R. (2003). GSK-3: Tricks of the Trade for a Multi-Tasking Kinase. J. Cel. Sci. 116, 1175–1186. doi:10.1242/jcs.00384
Eichmann, A., and Simons, M. (2012). VEGF Signaling inside Vascular Endothelial Cells and beyond. Curr. Opin. Cel. Biol. 24 (2), 188–193. doi:10.1016/j.ceb.2012.02.002
Ferrara, N., Gerber, H.-P., and LeCouter, J. (2003). The Biology of VEGF and its Receptors. Nat. Med. 9 (6), 669–676. doi:10.1038/nm0603-669
Flemming, R. G., Murphy, C. J., Abrams, G. A., Goodman, S. L., and Nealey, P. F. (1999). Effects of Synthetic Micro- and Nano-Structured Surfaces on Cell Behavior. Biomaterials 20 (6), 573–588. doi:10.1016/s0142-9612(98)00209-9
Goodman, S. L., Grasel, T. G., Cooper, S. L., and Albrecht, R. M. (1989). Platelet Shape Change and Cytoskeletal Reorganization on Polyurethaneureas. J. Biomed. Mater. Res. 23 (1), 105–123. doi:10.1002/jbm.820230109
Hibbert, B., Ma, X., Pourdjabbar, A., Holm, E., Rayner, K., Chen, Y.-X., et al. (2009). Inhibition of Endothelial Progenitor Cell Glycogen Synthase Kinase-3β Results in Attenuated Neointima Formation and Enhanced Re-endothelialization after Arterial Injury. Cardiovasc. Res. 83 (1), 16–23. doi:10.1093/cvr/cvp156
Hong, S., Kim, J., Na, Y. S., Park, J., Kim, S., Singha, K., et al. (2013). Poly(norepinephrine): Ultrasmooth Material-independent Surface Chemistry and Nanodepot for Nitric Oxide. Angew. Chem. Int. Ed. 52 (35), 9187–9191. doi:10.1002/anie.201301646
Kakinoki, S., Takasaki, K., Mahara, A., Ehashi, T., Hirano, Y., and Yamaoka, T. (2018). Direct Surface Modification of Metallic Biomaterials via Tyrosine Oxidation Aiming to Accelerate the Re-endothelialization of Vascular Stents. J. Biomed. Mater. Res. 106 (2), 491–499. doi:10.1002/jbm.a.36258
Ku, S. H., Ryu, J., Hong, S. K., Lee, H., and Park, C. B. (2010). General Functionalization Route for Cell Adhesion on Non-wetting Surfaces. Biomaterials 31 (9), 2535–2541. doi:10.1016/j.biomaterials.2009.12.020
Leclech, C., Natale, C. F., and Barakat, A. I. (2020). The Basement Membrane as a Structured Surface - Role in Vascular Health and Disease. J. Cel. Sci. 133 (18), jcs239889. doi:10.1242/jcs.239889
Lee, H., Dellatore, S. M., Miller, W. M., and Messersmith, P. B. (2007a). Mussel-inspired Surface Chemistry for Multifunctional Coatings. Science 318 (5849), 426–430. doi:10.1126/science.1147241
Lee, H., Lee, B. P., and Messersmith, P. B. (2007b). A Reversible Wet/dry Adhesive Inspired by Mussels and Geckos. Nature 448 (7151), 338–341. doi:10.1038/nature05968
Lee, H., Scherer, N. F., and Messersmith, P. B. (2006). Single-molecule Mechanics of Mussel Adhesion. Proc. Natl. Acad. Sci. 103 (35), 12999–13003. doi:10.1073/pnas.0605552103
Liang, C., Hu, Y., Wang, H., Xia, D., Li, Q., Zhang, J., et al. (2016). Biomimetic Cardiovascular Stents for In Vivo Re-endothelialization. Biomaterials 103, 170–182. doi:10.1016/j.biomaterials.2016.06.042
Lu, L., Li, Q.-L., Maitz, M. F., Chen, J.-L., and Huang, N. (2012). Immobilization of the Direct Thrombin Inhibitor-Bivalirudin on 316L Stainless Steel via Polydopamine and the Resulting Effects on Hemocompatibility In Vitro. J. Biomed. Mater. Res. 100 (9), 2421. doi:10.1002/jbm.a.34143
Luo, R., Tang, L., Zhong, S., Yang, Z., Wang, J., Weng, Y., et al. (2013). In Vitro investigation of Enhanced Hemocompatibility and Endothelial Cell Proliferation Associated with Quinone-Rich Polydopamine Coating. ACS Appl. Mater. Inter. 5 (5), 1704–1714. doi:10.1021/am3027635
Lynge, M. E., van der Westen, R., Postma, A., and Städler, B. (2011). Polydopamine-a Nature-Inspired Polymer Coating for Biomedical Science. Nanoscale 3 (12), 4916–4928. doi:10.1039/c1nr10969c
Ma, X., Hibbert, B., Dhaliwal, B., Seibert, T., Chen, Y.-X., Zhao, X., et al. (2010). Delayed Re-endothelialization with Rapamycin-Coated Stents Is Rescued by the Addition of a Glycogen Synthase Kinase-3β Inhibitor. Cardiovasc. Res. 86 (2), 338–345. doi:10.1093/cvr/cvq047
Madhurakkat Perikamana, S. K., Lee, J., Lee, Y. B., Shin, Y. M., Lee, E. J., Mikos, A. G., et al. (2015). Materials from Mussel-Inspired Chemistry for Cell and Tissue Engineering Applications. Biomacromolecules 16 (9), 2541–2555. doi:10.1021/acs.biomac.5b00852
Otsuka, F., Finn, A. V., Yazdani, S. K., Nakano, M., Kolodgie, F. D., and Virmani, R. (2012). The Importance of the Endothelium in Atherothrombosis and Coronary Stenting. Nat. Rev. Cardiol. 9 (8), 439–453. doi:10.1038/nrcardio.2012.64
Pang, J. H., Farhatnia, Y., Godarzi, F., Tan, A., Rajadas, J., Cousins, B. G., et al. (2015). In Situ Endothelialization: Bioengineering Considerations to Translation. Small 11 (47), 6248–6264. doi:10.1002/smll.201402579
Qi, P., Chen, S., Liu, T., Chen, J., Yang, Z., Weng, Y., et al. (2014). New Strategies for Developing Cardiovascular Stent Surfaces with Novel Functions (Review). Biointerphases 9 (2), 029017. doi:10.1116/1.4878719
Shi, X., Cui, L., Sun, H., Jiang, N., Heng, L., Zhuang, X., et al. (2019). Promoting Cell Growth on Porous PLA Microspheres through Simple Degradation Methods. Polym. Degrad. Stab. 161, 319–325. doi:10.1016/j.polymdegradstab.2019.01.003
Shin, Y. M., Lee, Y. B., Kim, S. J., Kang, J. K., Park, J.-C., Jang, W., et al. (2012). Mussel-inspired Immobilization of Vascular Endothelial Growth Factor (VEGF) for Enhanced Endothelialization of Vascular Grafts. Biomacromolecules 13 (7), 2020–2028. doi:10.1021/bm300194b
Skurk, C., Maatz, H., Rocnik, E., Bialik, A., Force, T., and Walsh, K. (2005). Glycogen-Synthase Kinase3β/β-Catenin Axis Promotes Angiogenesis through Activation of Vascular Endothelial Growth Factor Signaling in Endothelial Cells. Circ. Res. 96 (3), 308–318. doi:10.1161/01.RES.0000156273.30274.f7
Woods, T. C., and Marks, A. R. (2004). Drug-eluting Stents. Annu. Rev. Med. 55, 169–178. doi:10.1146/annurev.med.55.091902.105243
Wu, F., Li, J., Zhang, K., He, Z., Yang, P., Zou, D., et al. (2016). Multifunctional Coating Based on Hyaluronic Acid and Dopamine Conjugate for Potential Application on Surface Modification of Cardiovascular Implanted Devices. ACS Appl. Mater. Inter. 8 (1), 109–121. doi:10.1021/acsami.5b07427
Yang, Y., Qi, P., Ding, Y., Maitz, M. F., Yang, Z., Tu, Q., et al. (2015). A Biocompatible and Functional Adhesive Amine-Rich Coating Based on Dopamine Polymerization. J. Mater. Chem. B 3 (1), 72–81. doi:10.1039/c4tb01236d
Yang, Y., Qi, P., Wen, F., Li, X., Xia, Q., Maitz, M. F., et al. (2014). Mussel-Inspired One-step Adherent Coating Rich in Amine Groups for Covalent Immobilization of Heparin: Hemocompatibility, Growth Behaviors of Vascular Cells, and Tissue Response. ACS Appl. Mater. Inter. 6 (16), 14608–14620. doi:10.1021/am503925r
Yang, Z., Tu, Q., Wang, J., and Huang, N. (2012a). The Role of Heparin Binding Surfaces in the Direction of Endothelial and Smooth Muscle Cell Fate and Re-endothelialization. Biomaterials 33 (28), 6615–6625. doi:10.1016/j.biomaterials.2012.06.055
Yang, Z., Tu, Q., Zhu, Y., Luo, R., Li, X., Xie, Y., et al. (2012b). Mussel-inspired Coating of Polydopamine Directs Endothelial and Smooth Muscle Cell Fate for Re-endothelialization of Vascular Devices. Adv. Healthc. Mater. 1 (5), 548–559. doi:10.1002/adhm.201200073
Yang, Z., Wang, J., Luo, R., Maitz, M. F., Jing, F., Sun, H., et al. (2010). The Covalent Immobilization of Heparin to Pulsed-Plasma Polymeric Allylamine Films on 316L Stainless Steel and the Resulting Effects on Hemocompatibility. Biomaterials 31 (8), 2072–2083. doi:10.1016/j.biomaterials.2009.11.091
Yuan, Y., Shi, X., Gan, Z., and Wang, F. (2018). Modification of Porous PLGA Microspheres by Poly-L-Lysine for Use as Tissue Engineering Scaffolds. Colloids Surf. B: Biointerfaces 161, 162–168. doi:10.1016/j.colsurfb.2017.10.044
Zhang, F., Cheng, J., Hackett, N. R., Lam, G., Shido, K., Pergolizzi, R., et al. (2004). Adenovirus E4 Gene Promotes Selective Endothelial Cell Survival and Angiogenesis via Activation of the Vascular Endothelial-cadherin/Akt Signaling Pathway. J. Biol. Chem. 279 (12), 11760–11766. doi:10.1074/jbc.M312221200
Zhang, F., Zhang, Q., Li, X., Huang, N., Zhao, X., and Yang, Z. (2019). Mussel-inspired Dopamine-CuII Coatings for Sustained In Situ Generation of Nitric Oxide for Prevention of Stent Thrombosis and Restenosis. Biomaterials 194, 117–129. doi:10.1016/j.biomaterials.2018.12.020
Zhang, H., Xie, L., Deng, J., Zhuang, W., Luo, R., Wang, J., et al. (2016). Stability Research on Polydopamine and Immobilized Albumin on 316L Stainless Steel. Regen. Biomater. 3 (5), 277–284. doi:10.1093/rb/rbw030
Keywords: glycogen synthase kinase-3β inhibitor (GSKi), polydopamine, 316L stainless steel, endothelialization, human coronary artery endothelial cells
Citation: Zhang M, Shi X, Sun H, Xu D, Gao Y, Wu X, Zhang J and Zhang J (2021) Immobilization of Glycogen Synthase Kinase-3β Inhibitor on 316L Stainless Steel via Polydopamine to Accelerate Endothelialization. Front. Bioeng. Biotechnol. 9:806151. doi: 10.3389/fbioe.2021.806151
Received: 31 October 2021; Accepted: 04 November 2021;
Published: 22 November 2021.
Edited by:
Mingqiang Li, Sun Yat-sen University, ChinaReviewed by:
Haiqing Li, Nanjing Tech University, ChinaCopyright © 2021 Zhang, Shi, Sun, Xu, Gao, Wu, Zhang and Zhang. This is an open-access article distributed under the terms of the Creative Commons Attribution License (CC BY). The use, distribution or reproduction in other forums is permitted, provided the original author(s) and the copyright owner(s) are credited and that the original publication in this journal is cited, in accordance with accepted academic practice. No use, distribution or reproduction is permitted which does not comply with these terms.
*Correspondence: Jichang Zhang, amljaGFuZ0BqbHUuZWR1LmNu
†These authors have contributed equally to this work and share first authorship
Disclaimer: All claims expressed in this article are solely those of the authors and do not necessarily represent those of their affiliated organizations, or those of the publisher, the editors and the reviewers. Any product that may be evaluated in this article or claim that may be made by its manufacturer is not guaranteed or endorsed by the publisher.
Research integrity at Frontiers
Learn more about the work of our research integrity team to safeguard the quality of each article we publish.