- 1Department of Biochemical Engineering, School of Chemistry, Federal University of Rio de Janeiro (UFRJ), Rio de Janeiro, Brazil
- 2Goiano Federal Institute, Rio Verde, Brazil
- 3School of Pharmaceutical Sciences, São Paulo State University (UNESP), Araraquara, Brazil
Bioactive compounds can provide health benefits beyond the nutritional value and are originally present or added to food matrices. However, because they are part of the food matrices, most bioactive compounds remain in agroindustrial by-products. Agro-industrial by-products are generated in large quantities throughout the food production chain and can—when not properly treated—affect the environment, the profit, and the proper and nutritional distribution of food to people. Thus, it is important to adopt processes that increase the use of these agroindustrial by-products, including biological approaches, which can enhance the extraction and obtention of bioactive compounds, which enables their application in food and pharmaceutical industries. Biological processes have several advantages compared to nonbiological processes, including the provision of extracts with high quality and bioactivity, as well as extracts that present low toxicity and environmental impact. Among biological approaches, extraction from enzymes and fermentation stand out as tools for obtaining bioactive compounds from various agro-industrial wastes. In this sense, this article provides an overview of the main bioactive components found in agroindustrial by-products and the biological strategies for their extraction. We also provide information to enhance the use of these bioactive compounds, especially for the food and pharmaceutical industries.
1 Introduction
The world produces large amounts of agroindustrial raw materials, mainly used for human and animal consumption and energy production (FAO, 2017; Sadh et al., 2018a). However, losses of up to 50% of the raw materials are estimated and occur mainly during harvest, post-harvest, slaughter, transport, processing, storage, and consumption (Arah et al., 2016; Lemes et al., 2020a). The losses can represent about 680 billion dollars per year (Dora et al., 2020) and correspond to about 25%–35% of the food produced in the world. These losses of raw material can be equivalent to 1.3 billion tons a year of material that is no longer consumed or transformed from appropriate processes (Ishangulyyev et al., 2019).
Due to its composition, residues can show slow degradability, resulting in accumulation and negative environmental impact (Sadh et al., 2018a). Thus, it is relevant to identify new applications to convert these residues into high-value-added products (Irmak, 2017). In general, agro-industrial residues present considerable concentrations of compounds such as fibers, lipids, carbohydrates, peptides, carotenoids, phenolic compounds, and other compounds, which have multiple functionalities and bioactivities and can be applied as ingredients in other products (Varzakas et al., 2016; Coman et al., 2020; Lopes and Ligabue-Braun, 2021).
The bioactive compounds present in the residue matrices can be potentially used in the prevention and treatment of several diseases, such as hypertension (Oliveira Filho et al., 2020), diabetes (Valencia-Mejía et al., 2019), cardiovascular disease (Rangel-Huerta et al., 2015), and neurological disease (Mohd Sairazi and Sirajudeen, 2020). In addition, bioactive compounds can be incorporated into foods, increasing their nutritional, sensorial, and technological properties (e.g., water and oil holding capacities, foaming, emulsion, and gelatinization) (Egea et al., 2018; Guimarães et al., 2019).
The proper use of agro-industrial matrices requires the production/extraction of bioactive compounds through ecofriendly strategies instead of conventional processes, followed by optimizing process conditions (Lemes et al., 2016a; Heemann et al., 2019). In this context, biological processes stand out, as they can enhance the production, extraction, and application of components from agro-industrial matrices in a more attractive way (Jegatheesan et al., 2020). Due to their selectivity, biological strategies present some advantages, including the production of extracts with high quality and bioactivity, as well as low toxicity (Chen, 2015; Habeebullah et al., 2020; Wang and Lü, 2021). Among the biological approaches, one consists of (1) extraction using enzymes that release compounds from the matrix under optimized conditions, making the process efficient (Marathe et al., 2019), and another is the (2) fermentation using different microorganisms that transform waste into products of interest, such as ethanol, proteins, peptides, enzymes, and pigments (Sadh et al., 2018b; Martínez-Espinosa, 2020).
In this sense, this article provides an overview of the main bioactive components found in agro-industrial by-products and the biological strategies for their extraction. We also provide information to enhance the use of these bioactive compounds, especially for the food and pharmaceutical industries.
2 Generation of Agro-industrial Waste
The agroindustry generates large amounts of waste regardless of the production chain step (Palhares et al., 2020; Chauhan et al., 2021). This waste generation can impact the environment according to factors that include the degree of development of the countries, education, population awareness, public policies, overexploitation, and waste of natural resources (Bedoić et al., 2019; Palhares et al., 2020). For example, in the steps involving food processing, losses reaching up to 40% of production are verified, mainly due to inefficiency in the production processing and management system, deformed or damaged products, and packaging disposable, among others, which generate refusal on the part of consumers (Dora et al., 2020).
Waste can be from plant or animal sources (Figure 1). The vegetable by-products include leaves, stems, seeds, bark, straw, fibers, bagasse, and fruit skins, among others (Ezejiofor et al., 2014). For fruits and vegetables, for example, the production of industrial solid waste is verified, which includes items removed from fruits and vegetables during cleaning, processing, cooking, and packaging (EPA, 2012). For cereals, the waste generation corresponding to 35% of the total production is verified, including liquid residues (rice milling wastewater, parboiled rice effluent, corn steep liquor, and bakery wastewater) and solid wastes (corn pericarp, corn grits, and brewer’s spent grain), which are highly polluting due to large amounts of organic load, solid waste, and nutrients (Hassan et al., 2021). In general, vegetable residues present high carbohydrates (starch, cellulose, and hemicellulose), lignin, organic acids, minerals, and vitamins (Kumar et al., 2020).
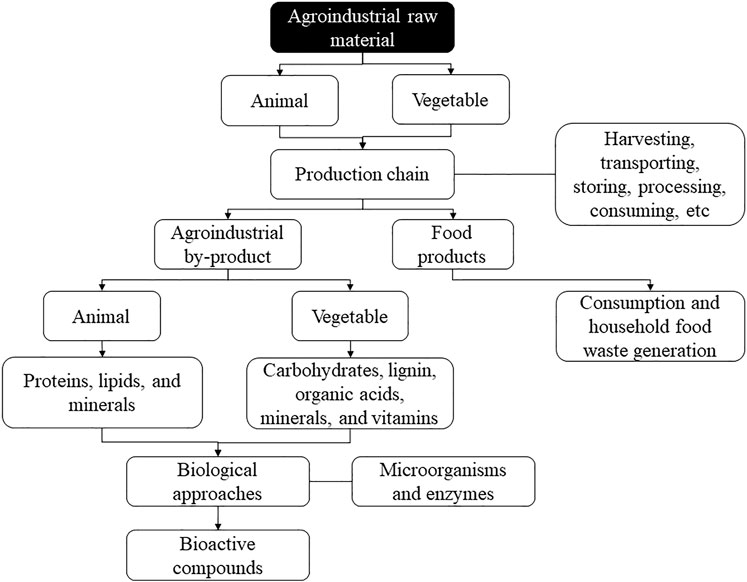
FIGURE 1. General steps that involve the generation of agroindustrial wastes and their use to produce bioactive components.
On the other hand, animal by-products comprise large amounts of carcasses, skins, hooves, heads, feathers, viscera, bones, fat, meat trimmings, blood, and other animal fluids (Ockerman and Hansen, 2000; Waldron, 2007), as well as meat out of specification and significant amounts of milk processing residues such as whey and other fractions from the separation process (Ben-Othman et al., 2020). The meat sector, for example, records losses of up to 23% of everything produced, including consumption losses, industrial processing, distribution, inadequate storage conditions, and failures in the freezing process (Karwowska et al., 2021). The dairy industry generates around 4 to 11 million tons of waste per year, including whey, dairy sludge, and wastewater (processing, cleaning, and sanitary), with great pollutant potential (Ahmad et al., 2019; Lemes et al., 2020a). In general, animal residues present high levels of proteins, lipids, and minerals (Jayathilakan et al., 2012; Jain and Anal, 2016; Maysonnave et al., 2020).
Due to the complex chemical composition, animal and vegetable residues can be used as a low-cost raw material to obtain bioactive compounds using suitable processes (Lemes et al., 2016a; Prado et al., 2020).
3 Bioactive Compounds
Bioactive compounds can be used with functions like to (1) improve quality in conventional food (nutritional, sensory, and technological properties), (2) produce functional foods that provide physiological benefits in terms of essential nutritional aspects, (3) produce nutraceuticals, isolated components of food or agroindustrial wastes that provide proven physiological benefits (Birch and Bonwick, 2018; Daliu et al., 2018; Aguiar et al., 2019; Reque and Brandelli, 2021), (4) and compose films for application as smart, active, and/or bioactive food packaging (Nogueira et al., 2020; Oliveira Filho et al., 2021). This wide application of bioactive compounds occurs due to several effects attributed to bioactive compounds, including protection of the immune system, anti-inflammatory action, reduction of damage from cell oxidation, and the occurrence of chronic noncommunicable diseases (Silva et al., 2019; Alongi and Anese, 2021).
Several wastes can be used to obtain bioactive compounds, including cereal bran, which is rich in phenolic compounds, flavonoids, glucans, and pigments (Pauline et al., 2020); fruit and vegetable wastes, which are sources of phenolic compounds (Trombino et al., 2021); and complex carbohydrates (Pérez et al., 2002), as well as animal wastes, e.g., fish wastes rich in omega 3 (Bonilla-Méndez and Hoyos-Concha, 2018) and milk processing wastes as sources of peptides (Pires et al., 2021).
Among the main bioactive compounds found in agro-industrial wastes with more interest for application in the food and pharmaceutical industries are (1) bioactive peptides, (2) phenolic compounds, (3) carbohydrates, and (4) other molecules with distinct biological and technological properties. Below we describe these bioactive compounds of interest.
1) Bioactive peptides are protein fragments with up to 20 amino acid residues and that have an impact on body functions, which depend on their composition and amino acid sequence in the structure (Lemes et al., 2016b). Due to their high protein value, cakes and meals can serve as a source of peptides or amino acids that, once released, demonstrate higher biological activity such as antioxidant, antihypertensive, anti-inflammatory, and immune-modulating activities (Lemes et al., 2016b; Lemes et al., 2020b; Velliquette et al., 2020).
Peptides with antioxidant activity exert biological effects on the human body and have attracted great interest for their safety and wide distribution (Brandelli et al., 2015). When applied directly to food, peptides can decrease the occurrence and speed of oxidation reactions, which is especially interesting in replacing synthetic antioxidants related to toxic effects on human health. Currently, studies report the antioxidant activities of plant-derived hydrolysates, such as soybean (Yang et al., 2019), sunflower (Prado et al., 2020), corn (Zhang et al., 2019), beans (Paula et al., 2020), and peanut flour (Yu et al., 2021), as well as hydrolysates from animal by-products such as fish (Hemker et al., 2020) and poultry (Bouhamed et al., 2020).
The antihypertensive property has been mentioned for several peptide molecules with the potential to inhibit the activity of renin, angiotensin-converting enzyme, and angiotensin II receptors in vitro and in vivo, increasing the levels of nitric oxide in the blood (Lemes et al., 2020b). As a result, peptides show potential for application in antihypertensive prevention and treatment, reducing cardiovascular complications, mainly when associated with physical activity and a healthy diet.
2) Phenolic compounds are one of the main groups of secondary metabolites produced by plants and are of particular interest due to their bioactive properties such as antioxidant, antihypertensive, and antimicrobial activities and inhibition of carcinogenesis (Tanase et al., 2014; Tanase et al., 2019). In addition, phenolic compounds have been suggested for applications in food as active agents to control lipid oxidation and microbial growth in foods (Huang et al., 2020; Zhang et al., 2020) and in the pharmaceutical and cosmetic industries such as mouthwashes, eye creams, and different herbal cosmetics (Petti and Scully, 2009; Saraf and Kaur, 2010; Gaur and Agnihotri, 2014).
3) Carbohydrates are an important energy source and play numerous key roles in all living organisms (Jiang et al., 2021). They are at high levels in residues of vegetable origin, especially starch, lignocellulose (cellulose, hemicellulose, and lignin), and β-glucans, among others (Kumar et al., 2020). Starch is the main storage carbohydrate in plants and is a mixture of two glucose polymers: amylose and amylopectin (Lovegrove et al., 2017). Starches are used in various sectors of the industry in a wide range of products besides food applications (Di-Medeiros-Leal et al., 2021). Lignocellulose is the main polymeric compound formed by plant metabolism as structural material and is widely present in agro-industrial waste. This carbohydrate type has variable amounts of cellulose, hemicellulose, and lignin and can be converted into different high-value products, contributing to waste reduction (Fortunati et al., 2016). β-Glucan is a polysaccharide with several biological activities with scientifically proven beneficial health effects. Cereal wastes from barley, oats, and residual yeast biomass can also be used as a source of β-glucan (Tosh et al., 2010; Du et al., 2014; Vieira et al., 2017; Guedes et al., 2019; Liu et al., 2021).
4) Other molecules can be obtained, including lipid molecules such as lycopene-type carotenoids that can act as natural pigments in their application in foods and fatty acids.
Lycopene is a carotenoid, not a vitamin A precursor, mainly found in tomatoes and by-products of tomato processing (Anarjan and Jouyban, 2017). In addition to acting as a natural pigment giving a red-orange color, lycopene can react to free radicals, preventing cellular compounds’ degradation, including DNA (Moritz and Tramonte, 2006; Caseiro et al., 2020; Adetunji et al., 2021). All fractions of tomato can be used, including the skin, which contains about 510–734 mg of lycopene/kg of dry matter (DM), in addition to significant amounts of lutein, β-carotene, and cis-β-carotene (Knoblich et al., 2005; Nour et al., 2018), and the seed, which has a lycopene content of ∼130 µg lycopene/kg DM (Knoblich et al., 2005). Solid fractions can also be used for lycopene extraction (Trombino et al., 2021) from the use of solvents, supercritical extraction (Machmudah et al., 2012; Urbonaviciene and Viskelis, 2017; Hatami et al., 2019), pulsed electric field-assisted extraction (Pataro et al., 2020), and ohmic technology (Coelho et al., 2019), among others. In addition to lycopene, tomato by-products contain tocopherols, sterols and terpenes, fatty acids, phenolic compounds, and flavonoids, showing great versatility in obtaining several bioactive compounds (Kalogeropoulos et al., 2012).
Polyunsaturated fatty acids (PUFAs) of the omega-3 and omega-6 types are in vegetable oils, fish, and nuts. As with marine products, vegetable and nut by-products can be a source of PUFA that is underutilized. Fish by-products that are sources of marine oils can be used in the production of enzymatic PUFA synthesis of acylglycerols directly from glycerol and omega-3 fatty acid concentrates. The main acids present in omega-3 are docosahexaenoic acid (DHA), eicosapentaenoic acid (EPA), and α-linolenic acid, while acids present in omega-6 are arachidonic and linolenic acids (Dave and Routray, 2018).
4 Biological vs. Non-Biological Approaches
The recovery of compounds of interest from agricultural wastes and by-products involves conventional and novel solid–liquid and liquid–liquid extractions. Conventional methods are based on the extraction capacity of different solvents and applying thermal factors and/or homogenization, such as maceration, infusion, Soxhlet extraction, and hydro-distillation. However, these methods have presented some disadvantages such as long duration in the case of solid–liquid extraction, e.g., low extraction selectivity and specificity, decomposition of thermolabile compounds, and low purity of the product after the purification process, as well as high operation pressure, energy need, and amount of solvent with high purity (Gligor et al., 2019; Becerra et al., 2021).
On the other hand, novel extraction methods had been proposed, such as substituting molecular solvents with ionic liquids, eutectic solvents, and supercritical fluids or using different nonthermal energies (ultrasound, microwave, and pulsed electric field). However, the cell wall of plant matrices (and their components, cellulose, hemicellulose, starch, pectin, lignin, and proteins) can make the extraction of compounds a challenge.
In biological conversion processes (enzymatic or fermentation), cell wall recalcitrance is a resistance of plant cell walls to biological deconstruction for enzymes and microorganisms (Zeng et al., 2017) that varies among plant species and phenotypes (Silveira et al., 2013). However, extensive research has been carried out to establish effective protocols for pretreatment of cell wall material, such as lignocellulose, before using the waste for biological conversion (Baruah et al., 2018; Mankar et al., 2021). Available pretreatments include physical (milling, microwave, extrusion, and ultrasonication), chemical (alkali, acid, ionic liquids, organosolv, and deep eutectic solvents), physicochemical (steam, ammonia and CO2 explosion, and liquid hot water), and biological (whole-cell and enzymatic pretreatment) methods (Baruah et al., 2018).
Biological processes using microorganisms or enzymes can hydrolyze molecules, disrupt cell walls, increase permeability, and allow intracellular materials to be accessible for extraction. The microorganisms can utilize agricultural wastes as substrates under specific pH, temperature, moisture, and water activity conditions for their growth and production of the compounds of interest. The use of enzymes from microorganisms, plants, and mammalian cells and tissues—which catalyze reactions with high specificity, regioselectivity, and mild conditions—could improve the extraction efficiency of different compounds (polyphenols, carotenoids, terpenoids, and others) or even convert this compounds into valuable compounds as biofuels, surfactants, and pharmaceuticals (Gligor et al., 2019; Marathe et al., 2019; Becerra et al., 2021; Sharma et al., 2021).
4.1 Extraction of Bioactive Components With Enzymes From Agro-industrial Wastes
The enzyme-assisted extraction (EAE) method can be employed in pretreatments of raw materials, improving extraction time, solvent use, and the quality and purity of a product while lowering production costs compared with classical extraction processes. Different enzymes could be applied including cellulases, hemicellulases, pectinases, amylases, proteases, and lipases, as free or immobilized forms. The enzyme behavior depends on operational conditions such as pH, temperature, enzyme and substrate concentration, solid/liquid ratio, the particle size of the substrate, and reaction time (Becerra et al., 2021).
Among the advantages related to enzyme’s use at the industrial scale is the cost reduction since enzymes acting as catalysts provide process savings compared to conventional strategies (Singh et al., 2016). To further reduce the cost of applying processes using enzymes, agro-industrial waste available in large quantities can be used in the production of enzymes (Lemes et al., 2016a) using simpler purification protocols or coupling techniques to purify the target product (Lemes et al., 2014; Lemes et al., 2019). Another factor that supports the application of enzymes and cost reduction in the process is the possibility of their immobilization, resulting in their recyclable use, allowing their application in defined cycles, and maintaining their selectivity, catalytic activity, and the generation of products in large quantities (Braga et al., 2014; Basso and Serban, 2019).
Greener processes have also been proposed by combining enzymes with ultrasound, microwave, and alternative solvent-based extraction methods, which can result in higher product quality, decreased production costs and solvents, or increased enzymatic treatment efficiency and extraction yields. These complementary treatments may be employed before or after EAE and simultaneously with the process, and their features consist of shortened extraction periods, nontoxicity, non-flammability, use of recyclable solvents, overall simplified steps, and customizable process parameters (Gligor et al., 2019; Wen et al., 2020; Picot-Allain et al., 2021). Table 1 shows examples of the use of enzymes in the extraction and recovery of these bioactive compounds from agro-industrial wastes.
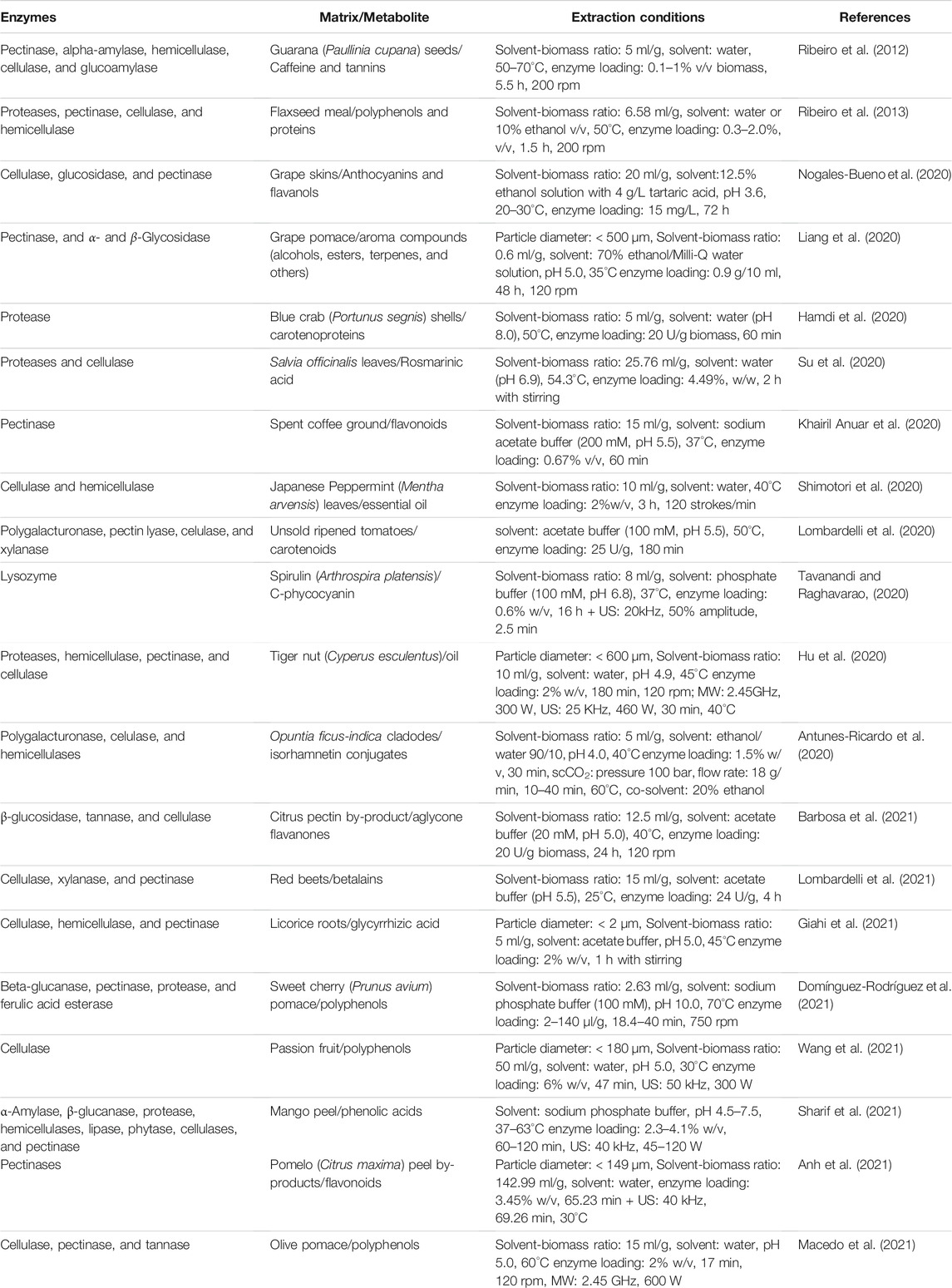
TABLE 1. Examples of enzyme-assisted extraction of bioactive compounds from agroindustrial by-products.
4.2 Fermentation Processes as a Tool to Obtain Bioactive Compounds From Agro-industrial Wastes
The fermentation process as a tool for obtaining bioactive compounds from agroindustrial wastes can be seen under different perspectives (Figure 2) as follows: (1) the target compound is the main product of microbial fermentation of agro-wastes, (2) the target compound is one of the products resulting from microbial fermentation of agro-wastes, and (3) microbial fermentation of agro-wastes produces enzymes, which will be applied to recover the target compound from a particular substrate.
4.2.1 Production of Bioactive Compounds Using Fermentation of Agro-industrial Wastes
The production of bioactive compounds through fermentation can be carried out with various microorganisms and their respective species (bacteria, yeasts, filamentous fungi, and others) (Moreira et al., 2018; Shin et al., 2019; Gulsunoglu et al., 2020; Jiang et al., 2020; Silva et al., 2020; Sinha et al., 2021). In addition, wild or genetically modified microorganisms (Cipolatti et al., 2019; Yang et al., 2020) can be applied in fermentative processes to obtain high-added-value compounds. The fermentation product can be part of the cell metabolism of microbial species or be extracted from the substrate by the microorganism’s action. The target compound can also be produced by the microorganism intracellularly (Rodrigues et al., 2019)—which needs cell rupture steps after fermentation for the compound recovery (Gomes et al., 2020)—or extracellularly (Acosta et al., 2020).
Fermentative strategies differ between solid (SSF) and submerged (SmF) states. The choice of the fermentation process will depend on the used microorganism and the process recovery of target compounds. In the SmF approach, microorganisms are grown in a liquid medium containing the nutrients (Dey et al., 2016). The target compounds are secreted into the fermentation medium and then recovered in a separation step, such as centrifugation. SmF offers better control of cultivation conditions and is most suitable for bacteria and yeasts requiring high moisture content. SmF also allows the proper mixing of nutrients due to the high amount of free water and is a method of easy handling and scaling up. Nevertheless, the target products tend to be diluted at the end of fermentation (Bagewadi et al., 2018; Sánchez et al., 2021).
In contrast, SSF utilizes solid substrates in the absence or near absence of free water, which is a more appropriate condition for the growth of filamentous fungi (Soccol et al., 2017). Microbial growth and product formation occur on the surface of a solid substrate that works as support or on an inert material impregnated with nutrient solution (Thomas et al., 2013). After the SSF process, the target compounds are recovered through extraction and separation steps. SSF presents minimal problems with microbial contamination due to the low water contents in the medium and offers high volumetric productivity, concentrated target compounds, tolerance of high substrate concentration, and less wastewater generation (Manan and Webb, 2017; Krishania et al., 2018).
In both fermentation strategies, agro-industrial wastes can be utilized as a nutritional source for microorganism species to obtain bioactive compounds (Kaur et al., 2019; Reque et al., 2019; Abdeshahian et al., 2020; Jiang et al., 2020; Sharma and Ghoshal, 2020; Sinha et al., 2021). Regarding the complex matrices of some wastes, pretreatments are applied before the fermentation to facilitate the microorganism’s access to nutrients (Acosta et al., 2020).
The choice of substrate will depend on the nutritional needs of the microbial species to produce the target compounds. A combination of wastes from different sources can also be an alternative to supply the nutritional requirements for microbial growth (Otero et al., 2019). In addition, the fermentation of wastes to produce bioactive compounds can be optimized through some approaches including response surface methodology (Moayedi et al., 2018; Rodrigues et al., 2019; Abdeshahian et al., 2020; Yang et al., 2020) and one factor at a time (Amorim et al., 2019; Kaur et al., 2019). The main bioactive compounds that can be produced using the biological approach were highlighted, as well as the particularities of each process.
Protein hydrolysates with biological actives can be obtained using one-step fermentation from wastes (Mechmeche et al., 2017; Fontoura et al., 2019). Moayedi et al. (2016) established a fermentative process with the Bacillus subtilis strain to convert tomato waste proteins into antioxidant and antibacterial hydrolysates. Mechmeche et al. (2017) investigated the bioconversion of tomato seed meal extract into antioxidant peptides through a fermentative process with Lactobacillus planetarium, which showed a promising ability to degrade and convert tomato seed proteins into peptides, also contributing to the antioxidant activity of the hydrolysates. Maciel et al. (2017) explored the keratinolytic potential of Chryseobacterium sp. and Bacillus sp. to convert feathers into protein hydrolysates with better in vitro nutritional features, suggesting a good prospect for their use in animal feed. Similarly, Fontoura et al. (2019) obtained protein hydrolysates with antioxidant properties through SmF of feathers with Chryseobacterium sp. Jiang et al. (2020) proposed an optimized production of bioactive peptides with antioxidant activity by B. subtilis from corn gluten meals.
Some studies have investigated the production of phenolic compounds through bioconversion of wastes. Shin et al. (2019) evaluated the fermentation of black rice by Aspergillus species under SSF to produce antioxidant phenolic compounds. After 3 days of fermentation, a maximum production of 1,660 µg protocatechuic acid/g of substrate was achieved. The authors also pointed the requirement to pretreat the waste for the extraction of phenolic compounds. Reque et al. (2017) addressed the bioprocessing of wheat middlings by Bacillus sp. to increase its antioxidant phenolic compound content. Besides changes in the phenolic profiles, the bioprocessed wheat middlings exhibited higher antioxidant capacity and total phenolic amounts than the unfermented waste. In the same way, Gulsunoglu et al. (2020) evaluated the effect of SSF with four Aspergillus spp. as a strategy to enhance the contents of phenolic compounds of apple peels. As a result, the 7-day fermentation enhanced apple peels’ phenolic contents and antioxidant activity by between threefold and fivefold. The enhancement of antioxidant phenolic compounds through bioprocessing of by-products from fig (Buenrostro-Figueroa et al., 2017) and apricot (Dulf et al., 2017) is also reported. The bioprocessing of brewer’s spent grain can favor both its phenolic compound and bioactive peptides contents, thus contributing to its antioxidant activity (Verni et al., 2020).
Potential prebiotic oligomers can be produced under microbial fermentation of wastes (Amorim et al., 2019; Reque et al., 2019). Wheat middlings, a by-product from wheat flour production, showed good aspects to be utilized as a substrate for xylooligosaccharide (XOS) production by B. subtilis, demonstrating prebiotic activity through in vitro tests with Lactobacillus acidophilus, a commercial probiotic strain (Reque et al., 2019). Likewise, brewers’ spent grain was fermented by a genetically modified B. subtilis to produce arabino-xylooligosaccharides (AXOS), and after optimizing the fermentation process, AXOS with a degree of polymerization (DP) of 2–6 were obtained. Furthermore, AXOS yield using a genetically modified strain increased 33% in comparison to the wild type (Amorim et al., 2018). In both cases, the bioconversion of wastes into xylooligomers occurred due to the ability of strains to secrete xylanases, the main enzymes involved in XOS production. Yang et al. (2020) proposed an efficient preparation of oligogalacturonides (OGS) using fermentation of citrus peel wastes with an engineered Pichia pastoris strain. The one-step fermentation of mandarin and orange peel wastes resulted in OGS with a DP of 2–7 and 2–6, respectively, and a maximal OGS yield of 26.1% after process optimization.
β-Glucan, a polysaccharide with several biological activities, is commonly extracted from cereal by-products (Karimi et al., 2019) and brewing/winery spent yeasts (Pinto et al., 2015; Varelas, 2016). However, β-glucan can also be produced by fermentation processes from wastes. Abdeshahian et al. (2020) investigated the production of extracellular β-glucan from sugarcane straw by Lasiodiplodia theobromae. The highest β-glucan yield and productivity were 0.047 g/g glucose and 0.014 g/L·h, respectively, at 72 h of fermentation. Acosta et al. (2020) evaluated the use of soybean molasses (unhydrolyzed and hydrolyzed forms) as a raw material for the fermentative production of β-glucan by L. theobromae. Maximum β-glucan production (1.06 g/L) and yield (0.13 g/g) were obtained in fermentations using unhydrolyzed molasses. Bzducha-Wróbel et al. (2020) proposed the valorization of waste potato juice water for β-glucan preparation with the Candida utilis strain, resulting in a β-glucan yield of 63 g/100 g yeast dry weight after 72 h of fermentation.
Natural pigments as carotenoids can be produced through biotechnological processes from wastes (Cipolatti et al., 2019; Otero et al., 2019). Corn steep liquor and sugarcane molasses were used as substrates to produce carotenoids by the Rhodotorula mucilaginosa strain through batch and fed-batch fermentation. Among the two fermentation approaches evaluated, the fed-batch process increased the carotenoid production by 400% compared to the batch process (Rodrigues et al., 2019). Onion peels, potato skin, mung bean husk, and pea pods were also evaluated as substrates to produce carotenoids under SmF with R. mucilaginosa. These wastes were chosen based on the high concentrations of sugars (onion peels and potato skin) and nitrogen (mung bean and pea pods). As a result, it was found that onion peels and mung bean husk are potential substrates for the production of microbial carotenoids as β-carotene, phytoene, torulene, and torularhodin (Sharma and Ghoshal, 2020). Olive mill wastes (Ghilardi et al., 2020), coffee pulp and husk (Moreira et al., 2018), and wastes from the vegetable and fruit markets (Sinha et al., 2021) were explored in carotenoid production by Rhodotorula species, showing good prospects as cheap substrates. Orange, carrot, and papaya peels were used as substrates to produce β-carotene under SSF with Blakeslea trispora, resulting in good yields in synthetic media (Kaur et al., 2019).
4.2.2 Low-Cost Microbial Enzymes for Further Recovery of Bioactive Compounds
Microbial enzymes produced by the fermentation of wastes are extensively applied to recover bioactive compounds (Zanutto-Elgui et al., 2019; Gautério et al., 2021a). Several studies have already demonstrated the effective use of wastes to obtain microbial hydrolases such as proteases, lipases, and carbohydrases (Pereira et al., 2019; Ahmad et al., 2020; Gautério et al., 2020).
The use of agro-industrial wastes reduces the production costs of microbial enzymes, being an alternative to replace synthetic and commercial substrates. The expenses related to the recovery of target compounds are also positively affected, as enzymatic extracts have a lower cost than pure commercial enzymes. Nevertheless, it is necessary to verify if the application requires purified enzymatic extracts since the inclusion of purification steps would result in a more costly process (Lemes et al., 2014; Lemes et al., 2019).
Zanutto-Elgui et al. (2019) produced bioactive peptides from bovine and goat milk subjected to the proteolytic activity of Aspergillus oryzae and Aspergillus flavipes proteases. Proteolytic enzymes from fungal species were effectively produced under SSF in wheat bran and then applied in the hydrolysis of milk proteins. The milk peptides showed broad antimicrobial and antioxidant activities in vitro, thus demonstrating a good prospect for biotechnological applications of these bioactive compounds. Oliveira et al. (2015) evaluated the production of soy protein hydrolysates with a microbial protease preparation. First, the protease production occurred using SmF from feather meal broth with the Chryseobacterium sp. strain. Then the enzymatic hydrolysis of soy protein isolate (SPI) occurred using the microbial protease extract, thus evaluating soluble peptides, antioxidant activity, and emulsifying capabilities of the hydrolysates. The authors pointed out that enzymatic hydrolysis increased soluble peptide content and positively affected SPI’s antioxidant and emulsifying properties. Moreover, the enzymatic treatment was demonstrated as a promising approach to obtain antioxidant compounds for food application, besides providing functional properties.
Gautério et al. (2020) utilized rice bran as a xylan source to produce xylanases by the Aureobasidium pullulans strain. In a subsequent study, crude and partially purified xylanases from A. pullulans CCT 1261 were applied in beechwood xylan hydrolysis, resulting in XOS, and the pretreatment did not influence the total concentration of XOS (Gautério et al., 2021a). The optimization of the hydrolytic process also demonstrated the successful use of crude xylanase to obtain XOS with low xylose release (Gautério et al., 2021b).
Kupski et al. (2018) applied a fungal cellulolytic complex to provide functional compounds—mainly proteins and phenolic compounds—from soybean meal (SBM) and corn husk (CH). The cellulolytic enzymes were obtained using SSF of rice husk and bran with Rhizopus oryzae CCT 7560 and then used to hydrolyze SBM and CH. Enzymatic hydrolysis resulted in 34% cellulose reduction in SBM, whereas, in CH, it was 55%. This reduction increased the protein (74%) and starch (95%) digestibility in SBM. In CH, in turn, the reduction allowed the release of phenolic compounds (21%). As mentioned by the authors, available protein in SBM can be used as a food supplement, whereas the phenolic contents from CH can be applied as a food additive.
4.2.3 Simultaneous Production of Bioactive Compounds Using Fermentation of Agro-industrial Wastes
The use of agro-industrial wastes to produce bioactive compounds via fermentation strategies has undergone several advances. One of them refers to co-production, which is cost-efficient and meets the sustainable context of the circular economy. Some examples include the simultaneous production of antioxidant compounds and proteolytic enzymes (Lemes et al., 2016a); lignocellulolytic enzymes and phenolic compounds (Leite et al., 2019); xylanases and xylooligomers (Menezes et al., 2017; Pereira et al., 2018); proteolytic enzymes and protein hydrolysates (Bernardo et al., 2019); lipids and carotenoids (Kot et al., 2019; Costa et al., 2020; Silva et al., 2020); and antioxidant peptides and pigments (Bertolini et al., 2021), among others.
The co-production has some challenges to be overcome such as (1) optimization of the fermentation process, which becomes more complicated when the aim is to obtain the maximum yield of all the target compounds; (2) application of treatments to wastes, which can sometimes favor the production of only the target compound; and (3) separation of compounds produced, where questions related to the application and purity of the target compounds—use of a mixture or use of each compound separately—must be analyzed.
5 Downstream Processing of Bioactive Compounds From Agro-industrial Wastes
The recovery and purification of bioactive compounds are strongly related to the particularities of the target biomolecule and its future application. Some bioactive compounds’ characteristics, such as the nature of the compound, cell location (intracellular or extracellular), size, structure, charge, and solubility, among others, will determine the steps to be applied for its recovery and purification. Furthermore, the purity required of the target compound as well as the preservation of their bioactivity must also be considered when establishing the purification steps.
Using agro-industrial wastes to obtain bioactive compounds using biotechnological approaches also influences the subsequent recovery and purification steps. Waste features such as particle size, solubility, viscosity, and recalcitrance can interfere with the extraction, cell disruption, and purification of the target compound, in addition to determining the number of downstream steps. Another critical point is related to the separation of bioactive compounds produced simultaneously in the same medium. All these aspects determine the complexity and costs of purification designs (Lemes et al., 2014; Lemes et al., 2020a).
Bioactive compounds produced by enzymatic hydrolysis or SmF procedures can be separated from the medium using solid–liquid techniques (e.g., filtration and centrifugation). Target compounds produced by SSF need to be recovered by extraction. The extraction approaches applied to bioactive compounds include solvent extraction (e.g., organic, eutectic, and ionic liquid solvents), ultrasound-assisted extraction, microwave-assisted extraction, enzymatic-assisted extraction, pulsed electric field, subcritical and supercritical fluid extraction, aqueous two-phase system, and three-phase partitioning (Zainal-Abidin et al., 2017; Sagar et al., 2018; Yan et al., 2018). Some examples include the extraction of β-carotene (Kaur et al., 2019) and phenolic compounds (Gulsunoglu et al., 2020) using organic solvents after SSF of fruit and vegetable peels; the extraction of lycopene using organic solvent (ethanol) after enzymatic-assisted treatment of tomato by-products (Azabou et al., 2016); the extraction of phenolic compounds using ionic liquids (Magro and Castro, 2020) and ultrasound-assisted procedure (Ajila et al., 2011) after SSF of lentil grains and apple pomace, respectively; and the extraction of phenolic compounds from fermented orange pomace using supercritical CO2 and cosolvents (Espinosa-Pardo et al., 2017).
Regarding intracellular target compounds, a step of cell disruption or cell permeabilization and subsequent extraction procedure is required, and both can occur separately or simultaneously (Kalil et al., 2017). The cell disruption/destabilization techniques applied to releasing compounds include bead milling, ultrasonication, high-pressure homogenization, osmotic shock, freeze–thawing, and lysis procedures with enzymes, chemicals, and heating (Gomes et al., 2020).
Purification processes applied to bioactive compounds are diverse and range from low- to high-resolution techniques. Some of the mentioned procedures in the literature are aqueous-phase separation (Wang et al., 2019), membrane technology (Pezeshk et al., 2019; Singh et al., 2020), and chromatography (Moayedi et al., 2018; Fontoura et al., 2019). Each technique can be applied alone or combined in purification designs, considering the aspects of the target compounds mentioned above. The challenge is to establish a purification process that results in desirable compound purity in the highest yield possible (Lemes et al., 2014).
6 Potential Applications of Bioactive Compounds Recovered From Waste and By-products
The growing demand for foods with beneficial effects on health, while contributing to the sustainable use of natural resources, stimulates the use of by-products to obtain bioactive compounds (Vilas-Boas et al., 2021), which have multiple applications in food, acting as antimicrobials, antioxidants, natural dyes, fortifying ingredients, texture modifiers, and others (Veneziani et al., 2017).
Figure 3 shows an overview of the potential application of bioactive compounds recovered from food by-products in meat, dairy, bakery, chocolate, and juice products, where the compounds are used as food ingredients with a defined role in the protection and technological properties of food.
A large number of studies report the addition of bioactive compounds from by-products in various food systems (Table 2), being applied as antioxidant and antimicrobial agents and also as agents to improve the nutritional and functional value of food products such as frozen fish (Özen et al., 2011), yogurt (Fidelis et al., 2020), dry cured sausage “chorizo” (Lorenzo et al., 2013), beef patties (Zamuz et al., 2018), bread (Rizzello et al., 2009; Peng et al., 2010), and petit Suisse cheese (Deolindo et al., 2019).
The antioxidant potential of extracts from by-products is frequently used in foods and has been mainly associated with their content of total phenolic compounds determined through different methods (Lorenzo et al., 2013; Zamuz et al., 2018; Fidelis et al., 2020) since the compounds have chemical properties and structural diversity that influences the mechanism of action associated with this bioactivity (Vuolo et al., 2019).
Phenolic compounds such as aliphatic alcohols, terpenes, acids, aldehydes, ketones, anthocyanins, and isoflavonoids are the main bioactive compounds in by-products with antimicrobial properties (Arshad and Batool, 2017) and, therefore, with potential for incorporation into food matrices. In addition to these compounds, water-soluble extracts rich in antimicrobial peptides can be obtained from agro-industrial by-products such as amaranth seed extract to inhibit fungal species isolated from bakery products, in which when applied to the bread matrix (gluten free and with bread flour wheat), the inhibitory activity is verified during the entire shelf life of the products (Rizzello et al., 2009).
In addition to the antioxidant and antimicrobial potentials, bioactive compounds may have other biological properties such as antiproliferative, antidiabetic, and antihypertensive activities (Ben-Othman et al., 2020). For example, Fidelis et al. (2020) observed that camu-camu seed extract has a high content of total phenolics (46.3% w/w), contain mainly vescalagin, castalagin, gallic acid, procyanidin A2, and (−)-epicatechin. This extract demonstrated high antioxidant and antiproliferative activities against HepG2 cells and Caco-2 cells, inhibiting α-amylase (40.7%), α-glucosidase (16.6%), and angiotensin-converting enzymes (34.4%). The application of the extract in yogurts increased the antioxidant capacity without affecting sensory acceptance (84%), an important factor for the application of any new ingredient in formulations.
Other components, such as betalain anthocyanins, curcumins, tannins, and carotenoids, commonly applied in foods as natural colorings (Luzardo-Ocampo et al., 2021), have also been used for the development of active and smart biodegradable food packaging (Figure 4) (Alizadeh-Sani et al., 2020). Anthocyanins extracted from the residue of processing blueberry juice, for example, have already been used in the production of smart films using cassava starch capable of monitoring the quality of orange juice, corn oil, and chicken pieces. Anthocyanin acts as an indicator of pH change during storage, as its color is altered due to structural changes when there is pH variation (Luchese et al., 2018).
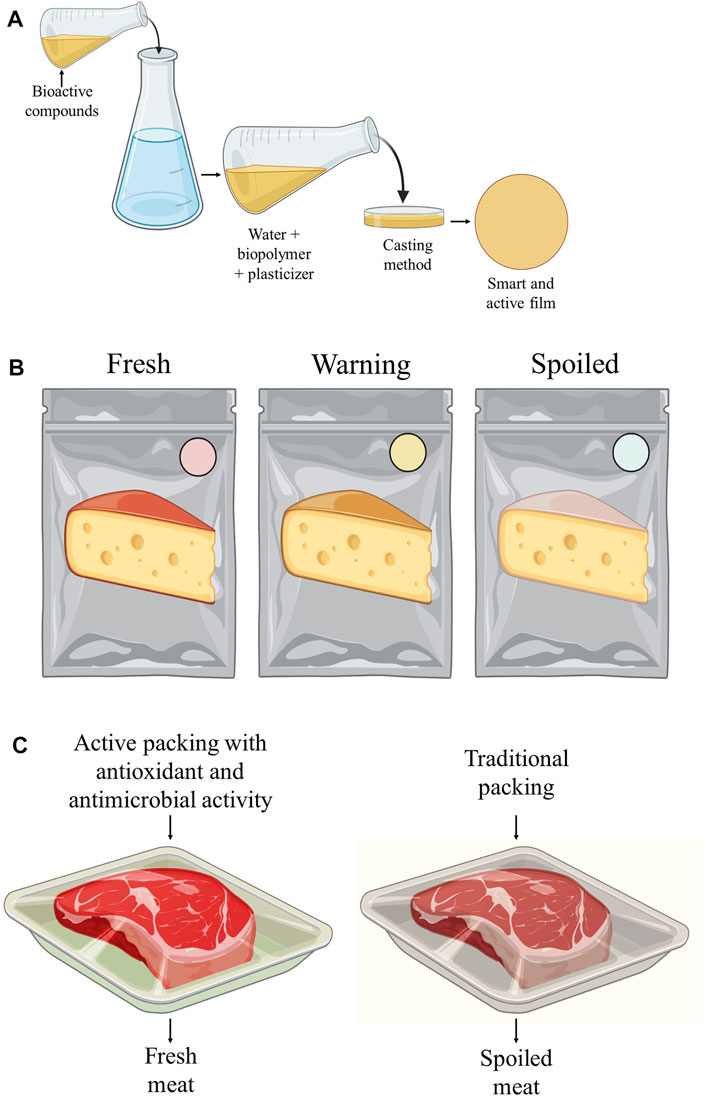
FIGURE 4. (A) production of films by casting. (B) application as smart packaging, and (C) application as active food packaging.
Anthocyanin extracted from black plum bark is also efficiently applied in films based on chitosan and TiO2, where incorporation results in high barrier properties against water vapor and UV-vis light and better mechanical strength (Zhang et al., 2019). In addition, it results in a higher free radical scavenging capacity and antimicrobial activity (Escherichia coli, Staphylococcus aureus, Salmonella, and Listeria monocytogenes), besides promoting the production of films capable of eliminating ethylene with potential application in pH-sensitive foods by detecting their changes and causing a color change.
Betacyanins extracted from the shell of dragon fruits can also be used to monitor the quality of fish freshness through their incorporation into intelligent packaging based on glucomannan–polyvinyl alcohol (Ardiyansyah et al., 2018). The presence of betacyanins also promotes a noticeable change from purple to yellow coloration due to the deterioration process of the product, which is, consequently, accompanied by increased levels of total volatile basic nitrogen (TVBN).
Protein hydrolysates, obtained through the enzymatic hydrolysis of proteins from agro-industrial by-products, have also been used as active agents in food films. Active food films based on alginate and protein hydrolysates obtained from the by-product of cottonseed oil extraction promote the formation of films with excellent visible light barrier properties, antioxidant activity, and antimicrobial potential against S. aureus, Colletotrichum gloeosporioides, and Rhizopus oligosporus (Oliveira-Filho et al., 2019).
Another prominent use of by-products is in the production of nutraceuticals, which are bioactive compounds used to meet the body’s needs and usually consumed in pharmaceutical preparations, such as pills, tablets, capsules, powders, and bottles (Kumar et al., 2017). Among the most commonly marketed and used nutraceuticals are amino acids, carotenoids (β-carotene, lutein, zeaxanthin, and lycopene), fatty acids (omega-3 and omega-6), minerals (copper, selenium, and zinc), polyphenols, vitamins (C and E), and several others (Souyoul et al., 2018), which can be extracted from agro-industrial by-products.
The possibility of applying bioactive components in food products and in new technologies to promote food quality and safety is huge. Due to the diversity of compounds and their possible interactions and diverse activities, each component must be properly evaluated to produce food, beverages, and active and smart packaging applied to food to guarantee maximum potential in the applications.
7 Conclusion and Future Directions
Large amounts of agro-industrial residues are generated during the processing of animal and vegetable materials, making it extremely necessary to adopt strategies for the integral use of residues or, even, for conversion into higher-value-added products. Biological approaches have several advantages compared to nonbiological processes, including the provision of extracts with high quality and high bioactivity, as well as with low toxicity.
Bioactive compounds obtained from by-products using the biological approach can be applied to develop foods and active or smart agents for biodegradable materials and packaging while contributing to consumer health, food safety, and sustainable use of natural resources.
That is why the biological approach is an important tool that must be continually improved and encouraged, especially due to the diversity of components that can be produced and the possible interactions and varied activities; each component must be properly evaluated to guarantee maximum potential in the applications.
Author Contributions
All authors listed have made a substantial, direct, and intellectual contribution to the work and approved it for publication.
Conflict of Interest
The authors declare that the research was conducted in the absence of any commercial or financial relationships that could be construed as a potential conflict of interest.
Publisher’s Note
All claims expressed in this article are solely those of the authors and do not necessarily represent those of their affiliated organizations, or those of the publisher, the editors, and the reviewers. Any product that may be evaluated in this article, or claim that may be made by its manufacturer, is not guaranteed or endorsed by the publisher.
Acknowledgments
The authors acknowledge the Coordenação de Aperfeiçoamento de Pessoal de Nível Superior—Brasil (CAPES—Finance Code 001), Conselho Nacional de Desenvolvimento Científico (CNPq), Fundação Carlos Chagas Filho de Amparo à Pesquisa do Estado do Rio de Janeiro (FAPERJ), and Instituto Federal Goiano (IF Goiano).
References
Abdeshahian, P., Ascencio, J. J., Philippini, R. R., Antunes, F. A. F., dos Santos, J. C., and da Silva, S. S. (2020). Utilization of Sugarcane Straw for Production of β-glucan Biopolymer by Lasiodiplodia Theobromae CCT 3966 in Batch Fermentation Process. Bioresour. Techn. 314, 123716. doi:10.1016/j.biortech.2020.123716
Acosta, S. B. P., Marchioro, M. L. K., Santos, V. A. Q., Calegari, G. C., Lafay, C. B. B., Barbosa-Dekker, A. M., et al. (2020). Valorization of Soybean Molasses as Fermentation Substrate for the Production of Microbial Exocellular β-Glucan. J. Polym. Environ. 28 (8), 2149–2160. doi:10.1007/s10924-020-01758-z
Adetunji, C. O., Akram, M., Mtewa, A. G., Jeevanandam, J., Egbuna, C., Ogodo, A. C., et al. (2021). “Biochemical and Pharmacotherapeutic Potentials of Lycopene in Drug Discovery,” in Preparation of Phytopharmaceuticals for the Management of Disorders (Elsevier), 307–360. doi:10.1016/b978-0-12-820284-5.00015-0
Aguiar, L. M., Geraldi, M. V., Betim Cazarin, C. B., and Maróstica Junior, M. R. (2019). “Chapter 11 - Functional Food Consumption and its Physiological Effects,” in Bioactive Compounds. Editor M.R.S. Campos (Woodhead Publishing), 205–225. doi:10.1016/b978-0-12-814774-0.00011-6
Ahmad, T., Aadil, R. M., Ahmed, H., Rahman, U. u., Soares, B. C. V., Souza, S. L. Q., et al. (2019). Treatment and Utilization of Dairy Industrial Waste: A Review. Trends Food Sci. Techn. 88, 361–372. doi:10.1016/j.tifs.2019.04.003
Ahmad, W., Tayyab, M., Aftab, M. N., Hashmi, A. S., Ahmad, M. D., Firyal, S., et al. (2020). Optimization of Conditions for the Higher Level Production of Protease: Characterization of Protease from Geobacillus SBS-4S. Waste Biomass Valor. 11 (12), 6613–6623. doi:10.1007/s12649-020-00935-4
Ajila, C. M., Brar, S. K., Verma, M., Tyagi, R. D., and Valéro, J. R. (2011). Solid-state Fermentation of Apple Pomace Using Phanerocheate Chrysosporium - Liberation and Extraction of Phenolic Antioxidants. Food Chem. 126 (3), 1071–1080. doi:10.1016/j.foodchem.2010.11.129
Alizadeh-Sani, M., Mohammadian, E., Rhim, J.-W., and Jafari, S. M. (2020). pH-Sensitive (Halochromic) Smart Packaging Films Based on Natural Food Colorants for the Monitoring of Food Quality and Safety. Trends Food Sci. Technol. 105, 93–144. doi:10.1016/j.tifs.2020.08.014
Alongi, M., and Anese, M. (2021). Re-thinking Functional Food Development through a Holistic Approach. J. Funct. Foods 81, 104466. doi:10.1016/j.jff.2021.104466
Alves Magro, A. E., and de Castro, R. J. S. (2020). Effects of Solid-State Fermentation and Extraction Solvents on the Antioxidant Properties of Lentils. Biocatal. Agric. Biotechnol. 28, 101753. doi:10.1016/j.bcab.2020.101753
Amorim, C., Silvério, S. C., and Rodrigues, L. R. (2019). One-step Process for Producing Prebiotic Arabino-Xylooligosaccharides from brewer's Spent Grain Employing Trichoderma Species. Food Chem. 270, 86–94. doi:10.1016/j.foodchem.2018.07.080
Amorim, C., Silvério, S. C., Silva, S. P., Coelho, E., Coimbra, M. A., Prather, K. L. J., et al. (2018). Single-step Production of Arabino-Xylooligosaccharides by Recombinant Bacillus Subtilis 3610 Cultivated in Brewers' Spent Grain. Carbohydr. Polym. 199, 546–554. doi:10.1016/j.carbpol.2018.07.017
Anarjan, N., and Jouyban, A. (2017). Preparation of Lycopene Nanodispersions from Tomato Processing Waste: Effects of Organic Phase Composition. Food Bioproducts Process. 103, 104–113. doi:10.1016/j.fbp.2017.03.003
Antunes-Ricardo, M., Mendiola, J. A., García-Cayuela, T., Ibañez, E., Gutiérrez-Uribe, J. A., Pilar Cano, M., et al. (2020). Enzyme-assisted Supercritical Fluid Extraction of Antioxidant Isorhamnetin Conjugates from Opuntia Ficus-Indica (L.) Mill. J. Supercrit. Fluids 158, 104713. doi:10.1016/j.supflu.2019.104713
Arah, I. K., Ahorbo, G. K., Anku, E. K., Kumah, E. K., and Amaglo, H. (2016). Postharvest Handling Practices and Treatment Methods for Tomato Handlers in Developing Countries: A Mini Review. Adv. Agric. 2016, 1–8. doi:10.1155/2016/6436945
Ardiyansyah, M. W., Apriliyanti, M. W., Wahyono, A., Fatoni, M., Poerwanto, B., and Suryaningsih, W. (2018). The Potency of Betacyanins Extract from a Peel of Dragon Fruits as a Source of Colourimetric Indicator to Develop Intelligent Packaging for Fish Freshness Monitoring. IOP Conf. Ser. Earth Environ. Sci. 207, 012038. doi:10.1088/1755-1315/207/1/012038
Arshad, M. S., and Batool, S. (2017). “Natural Antimicrobials, Their Sources and Food Safety,” in Food Additives. Editors D. N. Karunaratne, and G. Pamunuwa (London, UK: IntechOpen), 87–102.
Azabou, S., Abid, Y., Sebii, H., Felfoul, I., Gargouri, A., and Attia, H. (2016). Potential of the Solid-State Fermentation of Tomato by Products by Fusarium Solani Pisi for Enzymatic Extraction of Lycopene. LWT - Food Sci. Techn. 68, 280–287. doi:10.1016/j.lwt.2015.11.064
Bagewadi, Z. K., Mulla, S. I., and Ninnekar, H. Z. (2018). Optimization of Endoglucanase Production from Trichoderma harzianum Strain HZN11 by central Composite Design under Response Surface Methodology. Biomass Conv. Bioref. 8 (2), 305–316. doi:10.1007/s13399-017-0285-3
Barbosa, P. d. P. M., Ruviaro, A. R., and Macedo, G. A. (2021). Conditions of Enzyme-Assisted Extraction to Increase the Recovery of Flavanone Aglycones from Pectin Waste. J. Food Sci. Technol. 58 (11), 4303–4312. doi:10.1007/s13197-020-04906-4
Baruah, J., Nath, B. K., Sharma, R., Kumar, S., Deka, R. C., Baruah, D. C., et al. (2018). Recent Trends in the Pretreatment of Lignocellulosic Biomass for Value-Added Products. Front. Energ. Res. 6, 141. doi:10.3389/fenrg.2018.00141
Basso, A., and Serban, S. (2019). Industrial Applications of Immobilized Enzymes-A Review. Mol. Catal. 479, 110607. doi:10.1016/j.mcat.2019.110607
Bedoić, R., Ćosić, B., and Duić, N. (2019). Technical Potential and Geographic Distribution of Agricultural Residues, Co-products and By-Products in the European Union. Sci. Total Environ. 686, 568–579. doi:10.1016/j.scitotenv.2019.05.219
Ben Hamad Bouhamed, S., Krichen, F., and Kechaou, N. (2020). Feather Protein Hydrolysates: a Study of Physicochemical, Functional Properties and Antioxidant Activity. Waste Biomass Valor. 11 (1), 51–62. doi:10.1007/s12649-018-0451-2
Ben-Othman, S., Jõudu, I., and Bhat, R. (2020). Bioactives from Agri-Food Wastes: Present Insights and Future Challenges. Molecules 25 (3), 510. doi:10.3390/molecules25030510
Bernardo, B. d. S., Ramos, R. F., Callegaro, K., and Daroit, D. J. (2019). Co-production of Proteases and Bioactive Protein Hydrolysates from Bioprocessing of Feather Meal. Braz. Arch. Biol. Technol. 62, 1–9. doi:10.1590/1678-4324-2019180621
Bertolini, D., Jiménez, M. E. P., Dos Santos, C., Corrêa, A. P. F., and Brandelli, A. (2021). Microbial Bioconversion of Feathers into Antioxidant Peptides and Pigments and Their Liposome Encapsulation. Biotechnol. Lett. 43 (4), 835–844. doi:10.1007/s10529-020-03067-w
Dey, T. B., Chakraborty, S., Jain, K. K., Sharma, A., and Kuhad, R. C. (2016). Antioxidant Phenolics and Their Microbial Production by Submerged and Solid State Fermentation Process: A Review. Trends Food Sci. Techn. 53, 60–74. doi:10.1016/j.tifs.2016.04.007
Birch, C. S., and Bonwick, G. A. (2018). Ensuring the Future of Functional Foods. Int. J. Food Sci. Technol. 54 (5), 1467–1485. doi:10.1111/ijfs.14060
Bonilla-Méndez, J. R., and Hoyos-Concha, J. L. (2018). Methods of Extraction Refining and Concentration of Fish Oil as a Source of omega-3 Fatty Acids. Ciencia y Tecnología Agropecuaria 19, 645–668. doi:10.21930/rcta.vol19_num2_art:684
Braga, A. R. C., Silva, M. F., Oliveira, J. V., Treichel, H., and Kalil, S. J. (2014). a New Approach to Evaluate Immobilization of β-Galactosidase on Eupergitc: Structural, Kinetic, and Thermal Characterization. Química Nova 37 (5), 796–803. doi:10.5935/0100-4042.20140128
Brandelli, A., Daroit, D. J., and Corrêa, A. P. F. (2015). Whey as a Source of Peptides with Remarkable Biological Activities. Food Res. Int. 73, 149–161. doi:10.1016/j.foodres.2015.01.016
Buenrostro-Figueroa, J. J., Velázquez, M., Flores-Ortega, O., Ascacio-Valdés, J. A., Huerta-Ochoa, S., Aguilar, C. N., et al. (2017). Solid State Fermentation of Fig ( Ficus Carica L.) By-Products Using Fungi to Obtain Phenolic Compounds with Antioxidant Activity and Qualitative Evaluation of Phenolics Obtained. Process Biochem. 62, 16–23. doi:10.1016/j.procbio.2017.07.016
Bzducha-Wróbel, A., Koczoń, P., Błażejak, S., Kozera, J., and Kieliszek, M. (2020). Valorization of Deproteinated Potato Juice Water into β-Glucan Preparation of C. Utilis Origin: Comparative Study of Preparations Obtained by Two Isolation Methods. Waste Biomass Valor. 11 (7), 3257–3271. doi:10.1007/s12649-019-00641-w
Caseiro, M., Ascenso, A., Costa, A., Creagh-Flynn, J., Johnson, M., and Simões, S. (2020). Lycopene in Human Health. LWT 127, 109323. doi:10.1016/j.lwt.2020.109323
Chauhan, C., Dhir, A., Akram, M. U., and Salo, J. (2021). Food Loss and Waste in Food Supply Chains. A Systematic Literature Review and Framework Development Approach. J. Clean. Prod. 295, 126438. doi:10.1016/j.jclepro.2021.126438
Chen, H. (2015). “Lignocellulose Biorefinery Feedstock Engineering,” in Lignocellulose Biorefinery Engineering. Editor H. Chen (Woodhead Publishing), 37–86. doi:10.1016/b978-0-08-100135-6.00003-x
Cipolatti, E. P., Remedi, R. D., Sá, C. d. S., Rodrigues, A. B., Gonçalves Ramos, J. M., Veiga Burkert, C. A., et al. (2019). Use of Agroindustrial Byproducts as Substrate for Production of Carotenoids with Antioxidant Potential by Wild Yeasts. Biocatal. Agric. Biotechnol. 20, 101208. doi:10.1016/j.bcab.2019.101208
Coelho, M., Pereira, R., Rodrigues, A. S., Teixeira, J. A., and Pintado, M. E. (2019). Extraction of Tomato By-Products' Bioactive Compounds Using Ohmic Technology. Food Bioproducts Process. 117, 329–339. doi:10.1016/j.fbp.2019.08.005
Coman, V., Teleky, B.-E., Mitrea, L., Martău, G. A., Szabo, K., Călinoiu, L.-F., et al. (2020). “Bioactive Potential of Fruit and Vegetable Wastes,” in Advances in Food and Nutrition Research. Editor F. Toldrá (Academic Press), 157–225. doi:10.1016/bs.afnr.2019.07.001
Costa, W. A. d., Padilha, C. E. d. A., Oliveira Júnior, S. D. d., Silva, F. L. H. d., Silva, J., Ancântara, M. A., et al. (2020). Oil-lipids, Carotenoids and Fatty Acids Simultaneous Production by Rhodotorula Mucilaginosa CCT3892 Using Sugarcane Molasses as Carbon Source. Braz. J. Food Technol. 23, 1–11. doi:10.1590/1981-6723.06419
Pereira, A. S., Fontes-Sant’Ana, G. C., and Amaral, P. F. F. (2019). Mango Agro-Industrial Wastes for Lipase Production from Yarrowia Lipolytica and the Potential of the Fermented Solid as a Biocatalyst. Food Bioproducts Process. 115, 68–77. doi:10.1016/j.fbp.2019.02.002
Daliu, P., Santini, A., and Novellino, E. (2018). A Decade of Nutraceutical Patents: where Are We Now in 2018. Expert Opin. Ther. Patents 28 (12), 875–882. doi:10.1080/13543776.2018.1552260
Dave, D., and Routray, W. (2018). Current Scenario of Canadian Fishery and Corresponding Underutilized Species and Fishery Byproducts: A Potential Source of omega-3 Fatty Acids. J. Clean. Prod. 180, 617–641. doi:10.1016/j.jclepro.2018.01.091
Di-Medeiros-Leal, M. C. B., Ribeiro, G. O., Ribeiro, M. L. R., Ferreira, A. G., Braga, A. R. C., Egea, M. B., et al. (2021). “Analysis and Characterization of Starches from Alternative Sources,” in Biodegradable Polymers, Blends and Composites. Editors Sanjay Mavinkere. Rangappa, Jyotishkumar. Parameswaranpillai, Suchart. Siengchin, and M. Ramesh (United Kingdom: Elsevier), 465–488.
Domínguez-Rodríguez, G., Marina, M. L., and Plaza, M. (2021). Enzyme-assisted Extraction of Bioactive Non-extractable Polyphenols from Sweet Cherry (Prunus Avium L.) Pomace. Food Chem. 339, 128086. doi:10.1016/j.foodchem.2020.128086
Dora, M., Wesana, J., Gellynck, X., Seth, N., Dey, B., and De Steur, H. (2020). Importance of Sustainable Operations in Food Loss: Evidence from the Belgian Food Processing Industry. Ann. Oper. Res. 290 (1), 47–72. doi:10.1007/s10479-019-03134-0
Du, B., Zhu, F., and Xu, B. (2014). β-Glucan Extraction from Bran of hull-less Barley by Accelerated Solvent Extraction Combined with Response Surface Methodology. J. Cereal Sci. 59 (1), 95–100. doi:10.1016/j.jcs.2013.11.004
Dulf, F. V., Vodnar, D. C., Dulf, E.-H., and Pintea, A. (2017). Phenolic Compounds, Flavonoids, Lipids and Antioxidant Potential of Apricot (Prunus Armeniaca L.) Pomace Fermented by Two Filamentous Fungal Strains in Solid State System. Chem. Cent. J. 11 (1), 92. doi:10.1186/s13065-017-0323-z
Egea, M. B., Bolanho, B. C., Lemes, A. C., Bragatto, M. M., Silva, M. R., Carvalho, J. C. M., et al. (2018). Low Cost Cassava, Peach palm and Soy By-Products for the Nutritional Enrichment of Cookies: Physical, Chemical and Sensorial Characteristics. Int. Food Res. J. 25 (3), 1204–1212.
EPA (2012). “Industrial Food Processing Waste Analyses,” in Office of Resource Conservation and Recovery (U.S. Environmental Protection Agency). https://www.epa.gov.
Espinosa-Pardo, F. A., Nakajima, V. M., Macedo, G. A., Macedo, J. A., and Martínez, J. (2017). Extraction of Phenolic Compounds from Dry and Fermented orange Pomace Using Supercritical CO2 and Cosolvents. Food Bioproducts Process. 101, 1–10. doi:10.1016/j.fbp.2016.10.002
Ezejiofor, T., Enebaku, U. E., and Ogueke, C. (2014). Waste to Wealth- Value Recovery from Agro-Food Processing Wastes Using Biotechnology: A Review. Bbj 4, 418–481. doi:10.9734/bbj/2014/7017
FAOFood and Agriculture Organization of the United Nations (2017). The Future of Food Andagriculture - Trends and Challenges. Roma: FAO.
Fidelis, M., de Oliveira, S. M., Sousa Santos, J., Bragueto Escher, G., Silva Rocha, R., Gomes Cruz, A., et al. (2020). From Byproduct to a Functional Ingredient: Camu-Camu (Myrciaria Dubia) Seed Extract as an Antioxidant Agent in a Yogurt Model. J. Dairy Sci. 103 (2), 1131–1140. doi:10.3168/jds.2019-17173
Fontoura, R., Daroit, D. J., Corrêa, A. P. F., Moresco, K. S., Santi, L., Beys-da-Silva, W. O., et al. (2019). Characterization of a Novel Antioxidant Peptide from Feather Keratin Hydrolysates. New Biotechnol. 49, 71–76. doi:10.1016/j.nbt.2018.09.003
Fortunati, E., Luzi, F., Puglia, D., and Torre, L. (2016). “Extraction of Lignocellulosic Materials from Waste Products,” in Multifunctional Polymeric Nanocomposites Based on Cellulosic Reinforcements. Editors D. Puglia, E. Fortunati, and J. M. Kenny (William Andrew Publishing), 1–38. doi:10.1016/b978-0-323-44248-0.00001-8
Gaur, S., and Agnihotri, R. (2014). Green tea: A Novel Functional Food for the Oral Health of Older Adults. Geriatr. Gerontol. Int. 14 (2), 238–250. doi:10.1111/ggi.12194
Gautério, G. V., da Silva, L. G. G., Hübner, T., da Rosa Ribeiro, T., and Kalil, S. J. (2020). Maximization of Xylanase Production by Aureobasidium Pullulans Using a By-Product of rice Grain Milling as Xylan Source. Biocatal. Agric. Biotechnol. 23, 101511. doi:10.1016/j.bcab.2020.101511
Gautério, G. V., da Silva, L. G. G., Hübner, T., Ribeiro, T. d. R., and Kalil, S. J. (2021a). Xylooligosaccharides Production by Crude and Partially Purified Xylanase from Aureobasidium Pullulans: Biochemical and Thermodynamic Properties of the Enzymes and Their Application in Xylan Hydrolysis. Process Biochem. 104, 161–170. doi:10.1016/j.procbio.2021.03.009
Gautério, G. V., Hübner, T., Ribeiro, T. d. R., Ziotti, A. P. M., and Kalil, S. J. (2021b). Xylooligosaccharide Production with Low Xylose Release Using Crude Xylanase from Aureobasidium Pullulans: Effect of the Enzymatic Hydrolysis Parameters. Appl. Biochem. Biotechnol., 1–20. doi:10.1007/s12010-021-03658-x
Ghilardi, C., Sanmartin Negrete, P., Carelli, A. A., and Borroni, V. (2020). Evaluation of Olive Mill Waste as Substrate for Carotenoid Production by Rhodotorula Mucilaginosa. Bioresour. Bioproc. 7 (1), 52. doi:10.1186/s40643-020-00341-7
Giahi, E., Jahadi, M., and Khosravi-Darani, K. (2021). Enzyme-assisted Extraction of Glycyrrhizic Acid from Licorice Roots Using Heat Reflux and Ultrasound Methods. Biocatal. Agric. Biotechnol. 33, 101953. doi:10.1016/j.bcab.2021.101953
Giuseppe Rizzello, C., Coda, R., De Angelis, M., Di Cagno, R., Carnevali, P., and Gobbetti, M. (2009). Long-term Fungal Inhibitory Activity of Water-Soluble Extract from Amaranthus Spp. Seeds during Storage of Gluten-free and Wheat Flour Breads. Int. J. Food Microbiol. 131 (2-3), 189–196. doi:10.1016/j.ijfoodmicro.2009.02.025
Gligor, O., Mocan, A., Moldovan, C., Locatelli, M., Crișan, G., and Ferreira, I. C. F. R. (2019). Enzyme-assisted Extractions of Polyphenols - A Comprehensive Review. Trends Food Sci. Techn. 88, 302–315. doi:10.1016/j.tifs.2019.03.029
Gomes, T. A., Zanette, C. M., and Spier, M. R. (2020). An Overview of Cell Disruption Methods for Intracellular Biomolecules Recovery. Prep. Biochem. Biotechnol. 50 (7), 635–654. doi:10.1080/10826068.2020.1728696
Guimarães, R. M., Pimentel, T. C., de Rezende, T. A. M., Silva, J. d. S., Falcão, H. G., Ida, E. I., et al. (2019). Gluten-free Bread: Effect of Soy and Corn Co-products on the Quality Parameters. Eur. Food Res. Technol. 245 (7), 1365–1376. doi:10.1007/s00217-019-03261-9
Gulsunoglu, Z., Purves, R., Karbancioglu-Guler, F., and Kilic-Akyilmaz, M. (2020). Enhancement of Phenolic Antioxidants in Industrial Apple Waste by Fermentation with Aspergillus Spp. Biocatal. Agric. Biotechnol. 25, 101562. doi:10.1016/j.bcab.2020.101562
Guedes, J. S., Pimentel, T. C., Diniz-Silva, H. T., Tayse da Cruz Almeida, E., Tavares, J. F., Leite de Souza, E., et al. (2019). Protective Effects of β-glucan Extracted from Spent brewer Yeast during Freeze-Drying, Storage and Exposure to Simulated Gastrointestinal Conditions of Probiotic Lactobacilli. Lebensmittel-Wissenschaft und-Technologie 116, 108496. doi:10.1016/j.lwt.2019.108496
Hamdi, M., Nasri, R., Dridi, N., Li, S., and Nasri, M. (2020). Development of Novel High-Selective Extraction Approach of Carotenoproteins from Blue Crab (Portunus Segnis) Shells, Contribution to the Qualitative Analysis of Bioactive Compounds by HR-ESI-MS. Food Chem. 302, 125334. doi:10.1016/j.foodchem.2019.125334
Hassan, G., Shabbir, M. A., Ahmad, F., Pasha, I., Aslam, N., Ahmad, T., et al. (2021). Cereal Processing Waste, an Environmental Impact and Value Addition Perspectives: A Comprehensive Treatise. Food Chem. 363, 130352. doi:10.1016/j.foodchem.2021.130352
Hatami, T., Meireles, M. A. A., and Ciftci, O. N. (2019). Supercritical Carbon Dioxide Extraction of Lycopene from Tomato Processing By-Products: Mathematical Modeling and Optimization. J. Food Eng. 241, 18–25. doi:10.1016/j.jfoodeng.2018.07.036
Heemann, A. C. W., Heemann, R., Spier, M. R., and Santin, E. (2019). Enzyme-assisted Extraction of Polyphenols from green Yerba Mate. Braz. J. Food Techn. 22, 1–10. doi:10.1590/1981-6723.22217
Hemker, A. K., Nguyen, L. T., Karwe, M., and Salvi, D. (2020). Effects of Pressure-Assisted Enzymatic Hydrolysis on Functional and Bioactive Properties of tilapia (Oreochromis niloticus) By-Product Protein Hydrolysates. Lebensmittel-Wissenschaft und-Technologie 122, 109003. doi:10.1016/j.lwt.2019.109003
Hernández Becerra, E., De Jesús Pérez López, E., and Zartha Sossa, J. W. (2021). Recovery of Biomolecules from Agroindustry by Solid-Liquid Enzyme-Assisted Extraction: a Review. Food Anal. Methods 14 (8), 1744–1777. doi:10.1007/s12161-021-01974-w
Hu, B., Li, Y., Song, J., Li, H., Zhou, Q., Li, C., et al. (2020). Oil Extraction from Tiger Nut (Cyperus Esculentus L.) Using the Combination of Microwave-Ultrasonic Assisted Aqueous Enzymatic Method - Design, Optimization and Quality Evaluation. J. Chromatogr. A 1627, 461380. doi:10.1016/j.chroma.2020.461380
Huang, M., Wang, H., Xu, X., Lu, X., Song, X., and Zhou, G. (2020). Effects of Nanoemulsion-Based Edible Coatings with Composite Mixture of Rosemary Extract and ε-poly-l-lysine on the Shelf Life of Ready-To-Eat carbonado Chicken. Food Hydrocolloids 102, 105576. doi:10.1016/j.foodhyd.2019.105576
Irmak, S. (2017). “Biomass as Raw Material for Production of High‐Value Products,” in Biomass Volume Estimation and Valorization for Energy. Editor J. S. Tumuluru (London, UK: IntechOpen). doi:10.5772/65507
Ishangulyyev, R., Kim, S., and Lee, S. (2019). Understanding Food Loss and Waste-Why Are We Losing and Wasting Food. Foods 8 (8), 297. doi:10.3390/foods8080297
Jain, S., and Anal, A. K. (2016). Optimization of Extraction of Functional Protein Hydrolysates from Chicken Egg Shell Membrane (ESM) by Ultrasonic Assisted Extraction (UAE) and Enzymatic Hydrolysis. Food Sci. Techn. (Campinas) 69, 295–302. doi:10.1016/j.lwt.2016.01.057
Jayathilakan, K., Sultana, K., Radhakrishna, K., and Bawa, A. S. (2012). Utilization of Byproducts and Waste Materials from Meat, Poultry and Fish Processing Industries: a Review. J. Food Sci. Technol. 49 (3), 278–293. doi:10.1007/s13197-011-0290-7
Jegatheesan, V., Shu, L., Lens, P., and Chiemchaisri, C. (2020). Valorisation of Agro-Industrial Residues – Volume I: Biological Approaches. Springer.
Jiang, H., Qin, X., Wang, Q., Xu, Q., Wang, J., Wu, Y., et al. (2021). Application of Carbohydrates in Approved Small Molecule Drugs: A Review. Eur. J. Med. Chem. 223, 113633. doi:10.1016/j.ejmech.2021.113633
Jiang, X., Cui, Z., Wang, L., Xu, H., and Zhang, Y. (2020). Production of Bioactive Peptides from Corn Gluten Meal by Solid-State Fermentation with Bacillus Subtilis MTCC5480 and Evaluation of its Antioxidant Capacity In Vivo. Lebensmittel-Wissenschaft und-Technologie 131, 109767. doi:10.1016/j.lwt.2020.109767
Kalil, S. J., Moraes, C. C., Sala, L., and Burkert, C. A. V. (2017). “Bioproduct Extraction from Microbial Cells by Conventional and Nonconventional Techniques,” in Food Bioconversion (Elsevier), 179–206. doi:10.1016/b978-0-12-811413-1.00005-x
Kalogeropoulos, N., Chiou, A., Pyriochou, V., Peristeraki, A., and Karathanos, V. T. (2012). Bioactive Phytochemicals in Industrial Tomatoes and Their Processing Byproducts. LWT - Food Sci. Techn. 49 (2), 213–216. doi:10.1016/j.lwt.2011.12.036
Karimi, R., Azizi, M. H., and Xu, Q. (2019). Effect of Different Enzymatic Extractions on Molecular Weight Distribution, Rheological and Microstructural Properties of Barley Bran β-glucan. Int. J. Biol. Macromolecules 126, 298–309. doi:10.1016/j.ijbiomac.2018.12.165
Karwowska, M., Łaba, S., and Szczepański, K. (2021). Food Loss and Waste in Meat Sector-Why the Consumption Stage Generates the Most Losses. Sustainability 13 (11), 6227. doi:10.3390/su13116227
Kaur, P., Ghoshal, G., and Jain, A. (2019). Bio-utilization of Fruits and Vegetables Waste to Produce β-carotene in Solid-State Fermentation: Characterization and Antioxidant Activity. Process Biochem. 76, 155–164. doi:10.1016/j.procbio.2018.10.007
K. Habeebullah, S. F., Sattari, Z., Al-Haddad, S., Fakhraldeen, S., Al-Ghunaim, A., Al-Yamani, F., et al. (2020). Enzyme-assisted Extraction of Bioactive Compounds from Brown Seaweeds and Characterization. J. Appl. Phycol 32 (1), 615–629. doi:10.1007/s10811-019-01906-6
Khairil Anuar, M., Mohd Zin, Z., Juhari, N. H., Hasmadi, M., Smedley, K. L., and Zainol, M. K. (2020). Influence of Pectinase–Assisted Extraction Time on the Antioxidant Capacity of Spent Coffee Ground (SCG). Food Res. 4, 2054–2061.
Knoblich, M., Anderson, B., and Latshaw, D. (2005). Analyses of Tomato Peel and Seed Byproducts and Their Use as a Source of Carotenoids. J. Sci. Food Agric. 85 (7), 1166–1170. doi:10.1002/jsfa.2091
Kot, A. M., Błażejak, S., Kieliszek, M., Gientka, I., Bryś, J., Reczek, L., et al. (2019). Effect of Exogenous Stress Factors on the Biosynthesis of Carotenoids and Lipids by Rhodotorula Yeast Strains in media Containing Agro-Industrial Waste. World J. Microbiol. Biotechnol. 35 (10), 157. doi:10.1007/s11274-019-2732-8
Krishania, M., Sindhu, R., Binod, P., Ahluwalia, V., Kumar, V., Sangwan, R. S., et al. (2018). “Design of Bioreactors in Solid-State Fermentation,” in Current Developments in Biotechnology and Bioengineering. Editors A. Pandey, C. Larroche, and C. R. Soccol (Elsevier), 83–96. doi:10.1016/b978-0-444-63990-5.00005-0
Kumar, K., Yadav, A. N., Kumar, V., Vyas, P., and Dhaliwal, H. S. (2017). Food Waste: a Potential Bioresource for Extraction of Nutraceuticals and Bioactive Compounds. Bioresour. Bioproc. 4 (1), 1–14. doi:10.1186/s40643-017-0148-6
Kumar, S., Kushwaha, R., and Verma, M. L. (2020). “Recovery and Utilization of Bioactives from Food Processing Waste,” in Biotechnological Production of Bioactive Compounds. Editors M. L. Verma, and A. K. Chandel (Elsevier), 37–68. doi:10.1016/b978-0-444-64323-0.00002-3
Kupski, L., Telles, A. C., Gonçalves, L. M., Nora, N. S., and Furlong, E. B. (2018). Recovery of Functional Compounds from Lignocellulosic Material: An Innovative Enzymatic Approach. Food Biosci. 22, 26–31. doi:10.1016/j.fbio.2018.01.001
Leite, P., Silva, C., Salgado, J. M., and Belo, I. (2019). Simultaneous Production of Lignocellulolytic Enzymes and Extraction of Antioxidant Compounds by Solid-State Fermentation of Agro-Industrial Wastes. Ind. Crops Prod. 137, 315–322. doi:10.1016/j.indcrop.2019.04.044
Lemes, A. C., Álvares, G. T., Egea, M. B., Brandelli, A., and Kalil, S. J. (2016a). Simultaneous Production of Proteases and Antioxidant Compounds from Agro-Industrial By-Products. Bioresour. Techn. 222, 210–216. doi:10.1016/j.biortech.2016.10.001
Lemes, A. C., Gautério, G. V., Folador, G. O., Sora, G. T. S., and Pala, L. C. (2020a). “Reintrodução de resíduos agroindustriais na produção de alimentos,” in Realidades e perspectivas em Ciência dos Alimentos. Editor W. V. Nogueira (Nova Xavantina: MT: Editora Pantanal).
Lemes, A. C., Machado, J. R., Brites, M. L., Luccio, M. D., and Kalil, S. J. (2014). Design Strategies for Integratedβ-Galactosidase Purification Processes. Chem. Eng. Technol. 37 (10), 1805–1812. doi:10.1002/ceat.201300433
Lemes, A. C., Paula, L. C., Oliveira Filho, J. G., Prado, D. M. F., Medronha, G. A., and Egea, M. B. (2020b). Bioactive Peptides with Antihypertensive Property Obtained from Agro-Industrial Byproducts - Mini Review. Austin J. Nutr. Metab. 7 (3), 1–5.
Lemes, A. C., Silvério, S. C., Rodrigues, S., and Rodrigues, L. R. (2019). Integrated Strategy for Purification of Esterase from Aureobasidium Pullulans. Sep. Purif. Techn. 209, 409–418. doi:10.1016/j.seppur.2018.07.062
Lemes, A., Sala, L., Ores, J., Braga, A., Egea, M., and Fernandes, K. (2016b). A Review of the Latest Advances in Encrypted Bioactive Peptides from Protein-Rich Waste. International Journal of Molecular Sciences 17 (6), 1–24. doi:10.3390/ijms17060950
Liang, Z., Pai, A., Liu, D., Luo, J., Wu, J., Fang, Z., et al. (2020). Optimizing Extraction Method of Aroma Compounds from Grape Pomace. J. Food Sci. 85 (12), 4225–4240. doi:10.1111/1750-3841.15533
Liu, Y., Wu, Q., Wu, X., Algharib, S. A., Gong, F., Hu, J., et al. (2021). Structure, Preparation, Modification, and Bioactivities of β-glucan and Mannan from Yeast Cell wall: A Review. Int. J. Biol. Macromolecules 173, 445–456. doi:10.1016/j.ijbiomac.2021.01.125
Lombardelli, C., Benucci, I., Mazzocchi, C., and Esti, M. (2021). A Novel Process for the Recovery of Betalains from Unsold Red Beets by Low-Temperature Enzyme-Assisted Extraction. Foods 10 (2), 236. doi:10.3390/foods10020236
Lombardelli, C., Liburdi, K., Benucci, I., and Esti, M. (2020). Tailored and Synergistic Enzyme-Assisted Extraction of Carotenoid-Containing Chromoplasts from Tomatoes. Food Bioproducts Process. 121, 43–53. doi:10.1016/j.fbp.2020.01.014
Lopes, F. C., and Ligabue-Braun, R. (2021). Agro-Industrial Residues: Eco-Friendly and Inexpensive Substrates for Microbial Pigments Production. Front. Sustain. Food Syst. 5, 65. doi:10.3389/fsufs.2021.589414
Lorenzo, J. M., González-Rodríguez, R. M., Sánchez, M., Amado, I. R., and Franco, D. (2013). Effects of Natural (Grape Seed and Chestnut Extract) and Synthetic Antioxidants (Buthylatedhydroxytoluene, BHT) on the Physical, Chemical, Microbiological and Sensory Characteristics of Dry Cured Sausage "chorizo". Food Res. Int. 54 (1), 611–620. doi:10.1016/j.foodres.2013.07.064
Lovegrove, A., Edwards, C. H., De Noni, I., Patel, H., El, S. N., Grassby, T., et al. (2017). Role of Polysaccharides in Food, Digestion, and Health. Crit. Rev. Food Sci. Nutr. 57 (2), 237–253. doi:10.1080/10408398.2014.939263
Luchese, C. L., Abdalla, V. F., Spada, J. C., and Tessaro, I. C. (2018). Evaluation of Blueberry Residue Incorporated Cassava Starch Film as pH Indicator in Different Simulants and Foodstuffs. Food Hydrocolloids 82, 209–218. doi:10.1016/j.foodhyd.2018.04.010
Luzardo-Ocampo, I., Ramírez-Jiménez, A. K., Yañez, J., Mojica, L., and Luna-Vital, D. A. (2021). Technological Applications of Natural Colorants in Food Systems: A Review. Foods 10 (3), 634. doi:10.3390/foods10030634
Macedo, G. A., Santana, Á. L., Crawford, L. M., Wang, S. C., Dias, F. F. G., and de Moura Bell, J. M. L. N. (2021). Integrated Microwave- and Enzyme-Assisted Extraction of Phenolic Compounds from Olive Pomace. Lebensmittel-wissenschaft + [i.e. Technologie 138, 110621. doi:10.1016/j.lwt.2020.110621
Machmudah, S., Zakaria, S., Winardi, S., Sasaki, M., Goto, M., Kusumoto, N., et al. (2012). Lycopene Extraction from Tomato Peel By-Product Containing Tomato Seed Using Supercritical Carbon Dioxide. J. Food Eng. 108 (2), 290–296. doi:10.1016/j.jfoodeng.2011.08.012
Maciel, J. L., Werlang, P. O., Daroit, D. J., and Brandelli, A. (2017). Characterization of Protein-Rich Hydrolysates Produced through Microbial Conversion of Waste Feathers. Waste Biomass Valor. 8 (4), 1177–1186. doi:10.1007/s12649-016-9694-y
Mankar, A. R., Pandey, A., Modak, A., and Pant, K. K. (2021). Pretreatment of Lignocellulosic Biomass: A Review on Recent Advances. Bioresour. Techn. 334, 125235. doi:10.1016/j.biortech.2021.125235
Marathe, S. J., Jadhav, S. B., Bankar, S. B., Kumari Dubey, K., and Singhal, R. S. (2019). Improvements in the Extraction of Bioactive Compounds by Enzymes. Curr. Opin. Food Sci. 25, 62–72. doi:10.1016/j.cofs.2019.02.009
Martínez-Espinosa, R. (2020). “Introductory Chapter: A Brief Overview on Fermentation and Challenges for the Next Future,” in New Advances on Fermentation Processes. Editor R. M. Martínez-Espinosa (London, UK: IntechOpen).
Maysonnave, G. S., Mello, R. d. O., Vaz, F. N., Ávila, M. M. d., Pascoal, L. L., and Rodrigues, A. C. T. (2020). Physicochemical Characterization of By-Products from Beef Cattle slaughter and Economic Feasibility of Commercialization. Acta Sci. Anim. Sci. 42, e46545–7. doi:10.4025/actascianimsci.v42i1.46545
Mechmeche, M., Kachouri, F., Ksontini, H., and Hamdi, M. (2017). Production of Bioactive Peptides from Tomato Seed Isolate by Lactobacillus Plantarum Fermentation and Enhancement of Antioxidant Activity. Food Biotechnol. 31 (2), 94–113. doi:10.1080/08905436.2017.1302888
Menezes, B. S., Rossi, D. M., and Ayub, M. A. Z. (2017). Screening of Filamentous Fungi to Produce Xylanase and Xylooligosaccharides in Submerged and Solid-State Cultivations on rice Husk, Soybean hull, and Spent Malt as Substrates. World J. Microbiol. Biotechnol. 33 (3), 58. doi:10.1007/s11274-017-2226-5
Moayedi, A., Hashemi, M., and Safari, M. (2016). Valorization of Tomato Waste Proteins through Production of Antioxidant and Antibacterial Hydrolysates by Proteolytic Bacillus Subtilis: Optimization of Fermentation Conditions. J. Food Sci. Technol. 53 (1), 391–400. doi:10.1007/s13197-015-1965-2
Moayedi, A., Mora, L., Aristoy, M. C., Safari, M., Hashemi, M., and Toldrá, F. (2018). Peptidomic Analysis of Antioxidant and ACE-Inhibitory Peptides Obtained from Tomato Waste Proteins Fermented Using Bacillus Subtilis. Food Chem. 250, 180–187. doi:10.1016/j.foodchem.2018.01.033
Mohd Sairazi, N. S., and Sirajudeen, K. N. S. (2020). Natural Products and Their Bioactive Compounds: Neuroprotective Potentials against Neurodegenerative Diseases. Evidence-Based Complement. Altern. Med. 2020, 1–30. doi:10.1155/2020/6565396
Moreira, M. D., Melo, M. M., Coimbra, J. M., Reis, K. C. d., Schwan, R. F., and Silva, C. F. (2018). Solid Coffee Waste as Alternative to Produce Carotenoids with Antioxidant and Antimicrobial Activities. Waste Manag. 82, 93–99. doi:10.1016/j.wasman.2018.10.017
Moritz, B., and Tramonte, V. L. C. (2006). Biodisponibilidade Do Licopeno. Rev. Nutr. 19, 265–273. doi:10.1590/s1415-52732006000200013
Nguyen Tram Anh, M., Van Hung, P., and Thi Lan Phi, N. (2021). Optimized Conditions for Flavonoid Extraction from Pomelo Peel Byproducts under Enzyme- and Ultrasound-Assisted Extraction Using Response Surface Methodology. J. Food Qual. 2021, 1–10. doi:10.1155/2021/6666381
Nogales‐Bueno, J., Baca‐Bocanegra, B., Heredia, F. J., and Hernández‐Hierro, J. M. (2020). Phenolic Compounds Extraction in Enzymatic Macerations of Grape Skins Identified as Low‐level Extractable Total Anthocyanin Content. J. Food Sci. 85 (2), 324–331. doi:10.1111/1750-3841.15006
Nogueira, G. F., Oliveira, R. A. d., Velasco, J. I., and Fakhouri, F. M. (2020). Methods of Incorporating Plant-Derived Bioactive Compounds into Films Made with Agro-Based Polymers for Application as Food Packaging: A Brief Review. Polymers 12 (11), 2518. doi:10.3390/polym12112518
Nour, V., Panaite, T., Ropota, M., Turcu, R., Trandafir, I., and Corbu, A. (2018). Nutritional and Bioactive Compounds in Dried Tomato Processing Waste. CyTA - J. Food 16 (1), 222–229. doi:10.1080/19476337.2017.1383514
Ockerman, H. W., and Hansen, C. L. (2000). “Poultry By-Products,” in Animal by Product Processing and Utilization. Editors H. W. Ockerman, and C. L. Hansen (New York: CRC Press), 439–455.
Oliveira Filho, J. G. d., Braga, A. R. C., Oliveira, B. R. d., Gomes, F. P., Moreira, V. L., Pereira, V. A. C., et al. (2021). The Potential of Anthocyanins in Smart, Active, and Bioactive Eco-Friendly Polymer-Based Films: A Review. Food Res. Int. 142, 110202. doi:10.1016/j.foodres.2021.110202
Oliveira, C. F., Corrêa, A. P. F., Coletto, D., Daroit, D. J., Cladera-Olivera, F., and Brandelli, A. (2015). Soy Protein Hydrolysis with Microbial Protease to Improve Antioxidant and Functional Properties. J. Food Sci. Technol. 52 (5), 2668–2678. doi:10.1007/s13197-014-1317-7
Oliveira Filho, J. G. d., Rodrigues, J. M., Valadares, A. C. F., Almeida, A. B. d., Lima, T. M. d., Takeuchi, K. P., et al. (2019). Active Food Packaging: Alginate Films with Cottonseed Protein Hydrolysates. Food Hydrocolloids 92, 267–275. doi:10.1016/j.foodhyd.2019.01.052
Oliveira Filho, J. G., Rodrigues, J. M., Valadares, A. C. F., de Almeida, A. B., Valencia-Mejia, E., Fernandes, K. F., et al. (2020). Bioactive Properties of Protein Hydrolysate of Cottonseed Byproduct: Antioxidant, Antimicrobial, and Angiotensin-Converting Enzyme (ACE) Inhibitory Activities. Waste Biomass Valor. 12, 1395–1404. doi:10.1007/s12649-020-01066-6
Otero, D. M., Bulsing, B. A., Huerta, K. d. M., Rosa, C. A., Zambiazi, R. C., Burkert, C. A. V., et al. (2019). Carotenoid-producing Yeasts in the Brazilian Biodiversity: Isolation, Identification and Cultivation in Agroindustrial Waste. Braz. J. Chem. Eng. 36, 117–129. doi:10.1590/0104-6632.20190361s20170433
Özalp Özen, B., Eren, M., Pala, A., Özmen, İ., and Soyer, A. (2011). Effect of Plant Extracts on Lipid Oxidation during Frozen Storage of Minced Fish Muscle. Int. J. Food Sci. Techn. 46 (4), 724–731. doi:10.1111/j.1365-2621.2010.02541.x
Palhares, J. C. P., Oliveira, V. B. V., Freire-Junior, M., Cerdeira, A. L., and Prado, H. A. (2020). Responsible Consumption and Production: Contributions of Embrapa. Brasília, DF: Embrapa.
Pasini Deolindo, C. T., Monteiro, P. I., Santos, J. S., Cruz, A. G., Cristina da Silva, M., and Granato, D. (2019). Phenolic-rich Petit Suisse Cheese Manufactured with Organic Bordeaux Grape Juice, Skin, and Seed Extract: Technological, Sensory, and Functional Properties. LWT - Food Sci. Techn. 115, 108493. doi:10.1016/j.lwt.2019.108493
Pataro, G., Carullo, D., Falcone, M., and Ferrari, G. (2020). Recovery of Lycopene from Industrially Derived Tomato Processing By-Products by Pulsed Electric fields-assisted Extraction. Innovative Food Sci. Emerging Tech. 63, 102369. doi:10.1016/j.ifset.2020.102369
Paula, L. C. d., Lemes, A. C., Silva Neri, H. F. d., Ghedini, P. C., Batista, K. d. A., and Fernandes, K. F. (2020). Antioxidant and Anitoperoxidative Effect of Polypeptides from Common Beans (Phaseolus vulgaris, Cv BRS Pontal). Braz. J. Develop. 6 (7), 50569–50580. doi:10.34117/bjdv6n7-635
Pauline, M., Roger, P., Sophie Natacha Nina, N. E., Arielle, T., Eugene, E. E., and Robert, N. (2020). Physico-chemical and Nutritional Characterization of Cereals Brans Enriched Breads. Scientific Afr. 7, e00251. doi:10.1016/j.sciaf.2019.e00251
Peng, X., Ma, J., Cheng, K.-W., Jiang, Y., Chen, F., and Wang, M. (2010). The Effects of Grape Seed Extract Fortification on the Antioxidant Activity and Quality Attributes of Bread. Food Chem. 119 (1), 49–53. doi:10.1016/j.foodchem.2009.05.083
Pereira, G. F., de Bastiani, D., Gabardo, S., Squina, F., and Ayub, M. A. Z. (2018). Solid-state Cultivation of Recombinant Aspergillus nidulans to Co-produce Xylanase, Arabinofuranosidase, and Xylooligosaccharides from Soybean Fibre. Biocatal. Agric. Biotechnol. 15, 78–85. doi:10.1016/j.bcab.2018.05.012
Pérez, J., Muñoz-Dorado, J., De la Rubia, T., and Martínez, J. (2002). Biodegradation and Biological Treatments of Cellulose, Hemicellulose and Lignin: an Overview. Int. Microbiol. 5 (2), 53–63. doi:10.1007/s10123-002-0062-3
Petti, S., and Scully, C. (2009). Polyphenols, Oral Health and Disease: A Review. J. dentistry 37 (6), 413–423. doi:10.1016/j.jdent.2009.02.003
Pezeshk, S., Ojagh, S. M., Rezaei, M., and Shabanpour, B. (2019). Fractionation of Protein Hydrolysates of Fish Waste Using Membrane Ultrafiltration: Investigation of Antibacterial and Antioxidant Activities. Probiotics Antimicro. Prot. 11 (3), 1015–1022. doi:10.1007/s12602-018-9483-y
Picot-Allain, C., Mahomoodally, M. F., Ak, G., and Zengin, G. (2021). Conventional versus green Extraction Techniques - a Comparative Perspective. Curr. Opin. Food Sci. 40, 144–156. doi:10.1016/j.cofs.2021.02.009
Pinto, M., Coelho, E., Nunes, A., Brandão, T., and Coimbra, M. A. (2015). Valuation of Brewers Spent Yeast Polysaccharides: A Structural Characterization Approach. Carbohydr. Polym. 116, 215–222. doi:10.1016/j.carbpol.2014.03.010
Pires, A. F., Marnotes, N. G., Rubio, O. D., Garcia, A. C., and Pereira, C. D. (2021). Dairy By-Products: A Review on the Valorization of Whey and Second Cheese Whey. Foods 10 (5), 1067. doi:10.3390/foods10051067
Prado, D. M. F., Almeida, A. B., Oliveira-Filho, J. G., Alves, C. C. F., Egea, M. B., and Lemes, A. C. (2020). Extraction of Bioactive Proteins from Seeds (Corn, Sorghum, and sunflower) and sunflower Byproduct: Enzymatic Hydrolysis and Antioxidant Properties. Curr. Nutr. Food Sci. 17 (3), 310–320. doi:10.2174/1573401316999200731005803
Rangel-Huerta, O., Pastor-Villaescusa, B., Aguilera, C., and Gil, A. (2015). A Systematic Review of the Efficacy of Bioactive Compounds in Cardiovascular Disease: Phenolic Compounds. Nutrients 7 (7), 5177–5216. doi:10.3390/nu7075177
Reque, P., and Brandelli, A. (2021). Encapsulation of Probiotics and Nutraceuticals: Applications in Functional Food Industry. Trends Food Sci. Techn. 114, 1–10. doi:10.1016/j.tifs.2021.05.022
Reque, P. M., Orlandini Werner, J. A., Barreto Pinilla, C. M., Folmer Corrêa, A. P., Rodrigues, E., and Brandelli, A. (2017). Biological Activities of Wheat Middlings Bioprocessed with Bacillus Spp. LWT 77, 525–531. doi:10.1016/j.lwt.2016.12.010
Reque, P. M., Pinilla, C. M. B., Gautério, G. V., Kalil, S. J., and Brandelli, A. (2019). Xylooligosaccharides Production from Wheat Middlings Bioprocessed with Bacillus Subtilis. Food Res. Int. 126, 108673. doi:10.1016/j.foodres.2019.108673
Ribeiro, B. D., Barreto, D. W., and Coelho, M. A. Z. (2013). Enzyme-Enhanced Extraction of Phenolic Compounds and Proteins from Flaxseed Meal. ISRN Biotechnol. 2013, 1–6. doi:10.5402/2013/521067
Ribeiro, B. D., Coelho, M. A. Z., and Barreto, D. W. (2012). Obtenção de extratos de guaraná ricos em cafeína por processo enzimático e adsorção de taninos. Braz. J. Food Technol. 15, 261–270. doi:10.1590/s1981-67232012005000020
Rodrigues, T. V. D., Amore, T. D., Teixeira, E. C., and Burkert, J. F. d. M. (2019). Carotenoid Production by Rhodotorula Mucilaginosa in Batch and Fed-Batch Fermentation Using Agroindustrial Byproducts. Food Technol. Biotechnol. (Online) 57 (3), 388–398. doi:10.17113/ftb.57.03.19.6068
Sadh, P. K., Duhan, S., and Duhan, J. S. (2018a). Agro-industrial Wastes and Their Utilization Using Solid State Fermentation: a Review. Bioresour. Bioproc. 5 (1), 1. doi:10.1186/s40643-017-0187-z
Sadh, P., Kumar, S., Chawla, P., and Duhan, J. (2018b). Fermentation: A Boon for Production of Bioactive Compounds by Processing of Food Industries Wastes (By-Products). Molecules 23 (10), 2560. doi:10.3390/molecules23102560
Sagar, N. A., Pareek, S., Sharma, S., Yahia, E. M., and Lobo, M. G. (2018). Fruit and Vegetable Waste: Bioactive Compounds, Their Extraction, and Possible Utilization. Compr. Rev. Food Sci. Food Saf. 17 (3), 512–531. doi:10.1111/1541-4337.12330
Sánchez, Ó. J., Montoya, S., and Vargas, L. M. (2021). “Polysaccharide Production by Submerged Fermentation,” in Polysaccharides: Bioactivity and Biotechnology. Editors K. G. Ramawat, and J. -M. Mérillon (Cham: Springer International Publishing), 1–19.
Saraf, S., and Kaur, C. (2010). Phytoconstituents as Photoprotective Novel Cosmetic Formulations. Phcog Rev. 4 (7), 1. doi:10.4103/0973-7847.65319
Sharif, T., Bhatti, H. N., Bull, I. D., and Bilal, M. (2021). Recovery of High-Value Bioactive Phytochemicals from Agro-Waste of Mango (Mangifera Indica L.) Using Enzyme-Assisted Ultrasound Pretreated Extraction. Biomass Conv. Bioref. doi:10.1007/s13399-021-01589-5
Sharma, P., Gaur, V. K., Sirohi, R., Varjani, S., Hyoun Kim, S., and Wong, J. W. C. (2021). Sustainable Processing of Food Waste for Production of Bio-Based Products for Circular Bioeconomy. Bioresour. Techn. 325, 124684. doi:10.1016/j.biortech.2021.124684
Sharma, R., and Ghoshal, G. (2020). Optimization of Carotenoids Production by Rhodotorula Mucilaginosa (MTCC-1403) Using Agro-Industrial Waste in Bioreactor: A Statistical Approach. Biotechnol. Rep. 25, e00407. doi:10.1016/j.btre.2019.e00407
Shimotori, Y., Watanabe, T., Kohari, Y., Chiou, T.-Y., Ohtsu, N., Nagata, Y., et al. (2020). Enzyme-assisted Extraction of Bioactive Phytochemicals from Japanese Peppermint (Mentha Arvensis L. Cv. 'Hokuto'). J. Oleo Sci. 69 (6), 635–642. doi:10.5650/jos.ess19181
Shin, H.-Y., Kim, S.-M., Lee, J. H., and Lim, S.-T. (2019). Solid-state Fermentation of Black rice Bran with Aspergillus Awamori and Aspergillus Oryzae: Effects on Phenolic Acid Composition and Antioxidant Activity of Bran Extracts. Food Chem. 272, 235–241. doi:10.1016/j.foodchem.2018.07.174
Silva, J., Honorato da Silva, F. L., Santos Ribeiro, J. E., Nóbrega de Melo, D. J., Santos, F. A., and Lucena de Medeiros, L. (2020). Effect of Supplementation, Temperature and pH on Carotenoids and Lipids Production by Rhodotorula Mucilaginosa on Sisal Bagasse Hydrolyzate. Biocatal. Agric. Biotechnol. 30, 101847. doi:10.1016/j.bcab.2020.101847
Silva, L. B. A. R., Pinheiro-Castro, N., Novaes, G. M., Pascoal, G. d. F. L., and Ong, T. P. (2019). Bioactive Food Compounds, Epigenetics and Chronic Disease Prevention: Focus on Early-Life Interventions with Polyphenols. Food Res. Int. 125, 108646. doi:10.1016/j.foodres.2019.108646
Silveira, R. L., Stoyanov, S. R., Gusarov, S., Skaf, M. S., and Kovalenko, A. (2013). Plant Biomass Recalcitrance: Effect of Hemicellulose Composition on Nanoscale Forces that Control Cell Wall Strength. J. Am. Chem. Soc. 135 (51), 19048–19051. doi:10.1021/ja405634k
Singh, R. D., Muir, J., and Arora, A. (2020). Concentration of Xylooligosaccharides with a Low Degree of Polymerization Using Membranes and Their Effect on Bacterial Fermentation. Biofuels, Bioproducts and Biorefining 15, 61–73. doi:10.1002/bbb.2145
Singh, R., Kumar, M., Mittal, A., and Mehta, P. K. (2016). Microbial Enzymes: Industrial Progress in 21st century. 3 Biotech. 6 (2), 174. doi:10.1007/s13205-016-0485-8
Sinha, S., Singh, G., Arora, A., and Paul, D. (2021). Carotenoid Production by Red Yeast Isolates Grown in Agricultural and "Mandi" Waste. Waste Biomass Valor. 12 (7), 3939–3949. doi:10.1007/s12649-020-01288-8
Soccol, C. R., Costa, E. S. F. d., Letti, L. A. J., Karp, S. G., Woiciechowski, A. L., and Vandenberghe, L. P. d. S. (2017). Recent Developments and Innovations in Solid State Fermentation. Biotechnol. Res. Innovation 1 (1), 52–71. doi:10.1016/j.biori.2017.01.002
Souyoul, S. A., Saussy, K. P., and Lupo, M. P. (2018). Nutraceuticals: A Review. Dermatol. Ther. (Heidelb) 8 (1), 5–16. doi:10.1007/s13555-018-0221-x
Su, C. H., Pham, T. T. T., and Cheng, H. H. (2020). Aqueous Enzymatic Extraction of Rosmarinic Acid from Salvia Officinalis : Optimisation Using Response Surface Methodology. Phytochem. Anal. 31 (5), 575–582. doi:10.1002/pca.2922
Tanase, C., Boz, I., Stingu, A., Volf, I., and Popa, V. I. (2014). Physiological and Biochemical Responses Induced by spruce Bark Aqueous Extract and Deuterium Depleted Water with Synergistic Action in sunflower (Helianthus Annuus L.) Plants. Ind. Crops Prod. 60, 160–167. doi:10.1016/j.indcrop.2014.05.039
Tanase, C., Coșarcă, S., and Muntean, D.-L. (2019). A Critical Review of Phenolic Compounds Extracted from the Bark of Woody Vascular Plants and Their Potential Biological Activity. Molecules 24 (6), 1182. doi:10.3390/molecules24061182
Tavanandi, H. A., and Raghavarao, K. S. M. S. (2020). Ultrasound-assisted Enzymatic Extraction of Natural Food Colorant C-Phycocyanin from Dry Biomass of Arthrospira Platensis. Lebensmittel-Wissenschaft und-Technologie 118, 108802. doi:10.1016/j.lwt.2019.108802
Thomas, L., Larroche, C., and Pandey, A. (2013). Current Developments in Solid-State Fermentation. Biochem. Eng. J. 81, 146–161. doi:10.1016/j.bej.2013.10.013
Tosh, S. M., Brummer, Y., Miller, S. S., Regand, A., Defelice, C., Duss, R., et al. (2010). Processing Affects the Physicochemical Properties of β-Glucan in Oat Bran Cereal. J. Agric. Food Chem. 58 (13), 7723–7730. doi:10.1021/jf904553u
Trombino, S., Cassano, R., Procopio, D., Di Gioia, M. L., and Barone, E. (2021). Valorization of Tomato Waste as a Source of Carotenoids. Molecules 26 (16), 5062. doi:10.3390/molecules26165062
Urbonaviciene, D., and Viskelis, P. (2017). The Cis-Lycopene Isomers Composition in Supercritical CO2 Extracted Tomato By-Products. LWT - Food Sci. Techn. 85, 517–523. doi:10.1016/j.lwt.2017.03.034
Valencia-Mejía, E., Batista, K. A., Fernández, J. J. A., and Fernandes, K. F. (2019). Antihyperglycemic and Hypoglycemic Activity of Naturally Occurring Peptides and Protein Hydrolysates from Easy-To-cook and Hard-To-cook Beans (Phaseolus vulgaris L.). Food Res. Int. 121, 238–246. doi:10.1016/j.foodres.2019.03.043
Varelas, V., Tataridis, P., Liouni, M., and Nerantzis, E. T. (2016). Valorization of Winery Spent Yeast Waste Biomass as a New Source for the Production of β-Glucan. Waste Biomass Valor. 7, 807–817. doi:10.1007/s12649-016-9530-4
Varzakas, T., Zakynthinos, G., and Verpoort, F. (2016). Plant Food Residues as a Source of Nutraceuticals and Functional Foods. Foods 5 (4), 88. doi:10.3390/foods5040088
Velliquette, R. A., Fast, D. J., Maly, E. R., Alashi, A. M., and Aluko, R. E. (2020). Enzymatically Derived sunflower Protein Hydrolysate and Peptides Inhibit NFκB and Promote Monocyte Differentiation to a Dendritic Cell Phenotype. Food Chem. 319, 126563. doi:10.1016/j.foodchem.2020.126563
Veneziani, G., Novelli, E., Esposto, S., Taticchi, A., and Servili, M. (2017). “Chapter 11 - Applications of Recovered Bioactive Compounds in Food Products,” in Olive Mill Waste. Editor C. M. Galanakis (Academic Press), 231–253. doi:10.1016/b978-0-12-805314-0.00011-x
Verni, M., Pontonio, E., Krona, A., Jacob, S., Pinto, D., Rinaldi, F., et al. (2020). Bioprocessing of Brewers' Spent Grain Enhances its Antioxidant Activity: Characterization of Phenolic Compounds and Bioactive Peptides. Front. Microbiol. 11, 1831. doi:10.3389/fmicb.2020.01831
Vieira, E. F., Melo, A., and Ferreira, I. M. P. L. V. O. (2017). Autolysis of Intracellular Content of Brewer's Spent Yeast to Maximize ACE-Inhibitory and Antioxidant Activities. LWT - Food Sci. Techn. 82, 255–259. doi:10.1016/j.lwt.2017.04.046
Vilas-Boas, A. A., Pintado, M., and Oliveira, A. L. S. (2021). Natural Bioactive Compounds from Food Waste: Toxicity and Safety Concerns. Foods 10 (7), 1564. doi:10.3390/foods10071564
Vuolo, M. M., Lima, V. S., and Maróstica Junior, M. R. (2019). “Chapter 2 - Phenolic Compounds: Structure, Classification, and Antioxidant Power,” in Bioactive Compounds. Editor M. R. S. Campos (Woodhead Publishing), 33–50. doi:10.1016/b978-0-12-814774-0.00002-5
Waldron, K. (2007). “Waste Minimization, Management and Co-product Recovery in Food Processing: an Introduction,” in Handbook of Waste Management and Co-product Recovery in Food Processing. Editor K. Waldron (Cambridge, England: Woodhead Publishing), 3–20. doi:10.1533/9781845692520.1.3
Wang, T., and Lü, X. (2021). “Overcome Saccharification Barrier,” in Advances in 2nd Generation of Bioethanol Production. Editor X. Lü (Woodhead Publishing), 137–159. doi:10.1016/b978-0-12-818862-0.00005-4
Wang, W., Gao, Y.-T., Wei, J.-W., Chen, Y.-F., Liu, Q.-L., and Liu, H.-M. (2021). Optimization of Ultrasonic Cellulase-Assisted Extraction and Antioxidant Activity of Natural Polyphenols from Passion Fruit. Molecules 26 (9), 2494. doi:10.3390/molecules26092494
Wang, Y.-Y., Ma, H., Ding, Z.-C., Yang, Y., Wang, W.-H., Zhang, H.-N., et al. (2019). Three-phase Partitioning for the Direct Extraction and Separation of Bioactive Exopolysaccharides from the Cultured Broth of Phellinus Baumii. Int. J. Biol. Macromolecules 123, 201–209. doi:10.1016/j.ijbiomac.2018.11.065
Webb, C., and Webb, C. (2017). Design Aspects of Solid State Fermentation as Applied to Microbial Bioprocessing. Jabb 4, 1–25. doi:10.15406/jabb.2017.04.00094
Wen, L., Zhang, Z., Sun, D.-W., Sivagnanam, S. P., and Tiwari, B. K. (2020). Combination of Emerging Technologies for the Extraction of Bioactive Compounds. Crit. Rev. Food Sci. Nutr. 60 (11), 1826–1841. doi:10.1080/10408398.2019.1602823
Yan, J.-K., Wang, Y.-Y., Qiu, W.-Y., Ma, H., Wang, Z.-B., and Wu, J.-Y. (2018). Three-phase Partitioning as an Elegant and Versatile Platform Applied to Nonchromatographic Bioseparation Processes. Crit. Rev. Food Sci. Nutr. 58 (14), 2416–2431. doi:10.1080/10408398.2017.1327418
Yang, G., Tan, H., Li, S., Zhang, M., Che, J., Li, K., et al. (2020). Application of Engineered Yeast Strain Fermentation for Oligogalacturonides Production from Pectin-Rich Waste Biomass. Bioresour. Techn. 300, 122645. doi:10.1016/j.biortech.2019.122645
Yang, J., Wu, X.-B., Chen, H.-L., Sun-Waterhouse, D., Zhong, H.-B., and Cui, C. (2019). A Value-Added Approach to Improve the Nutritional Quality of Soybean Meal Byproduct: Enhancing its Antioxidant Activity through Fermentation by Bacillus Amyloliquefaciens SWJS22. Food Chem. 272, 396–403. doi:10.1016/j.foodchem.2018.08.037
Yu, J., Mikiashvili, N., Bonku, R., and Smith, I. N. (2021). Allergenicity, Antioxidant Activity and ACE-Inhibitory Activity of Protease Hydrolyzed Peanut Flour. Food Chem. 360, 129992. doi:10.1016/j.foodchem.2021.129992
Zainal-Abidin, M. H., Hayyan, M., Hayyan, A., and Jayakumar, N. S. (2017). New Horizons in the Extraction of Bioactive Compounds Using Deep Eutectic Solvents: A Review. Analytica Chim. Acta 979, 1–23. doi:10.1016/j.aca.2017.05.012
Zamuz, S., López-Pedrouso, M., Barba, F. J., Lorenzo, J. M., Domínguez, H., and Franco, D. (2018). Application of hull, Bur and Leaf Chestnut Extracts on the Shelf-Life of Beef Patties Stored under MAP: Evaluation of Their Impact on Physicochemical Properties, Lipid Oxidation, Antioxidant, and Antimicrobial Potential. Food Res. Int. 112, 263–273. doi:10.1016/j.foodres.2018.06.053
Zanutto-Elgui, M. R., Vieira, J. C. S., Prado, D. Z. d., Buzalaf, M. A. R., Padilha, P. d. M., Elgui de Oliveira, D., et al. (2019). Production of Milk Peptides with Antimicrobial and Antioxidant Properties through Fungal Proteases. Food Chem. 278, 823–831. doi:10.1016/j.foodchem.2018.11.119
Zeng, Y., Himmel, M. E., and Ding, S.-Y. (2017). Visualizing Chemical Functionality in Plant Cell walls. Biotechnol. Biofuels 10 (1), 263. doi:10.1186/s13068-017-0953-3
Zhang, D., Bi, W., Kai, K., Ye, Y., and Liu, J. (2020). Effect of Chlorogenic Acid on Controlling Kiwifruit Postharvest Decay Caused by Diaporthe Sp. Lebensmittel-wissenschaft + [i.e. Technologie 132, 109805. doi:10.1016/j.lwt.2020.109805
Zhang, S., Zhang, M., Yang, R., Zhang, S., and Lin, S. (2019a). Preparation, Identification, and Activity Evaluation of Antioxidant Peptides from Protein Hydrolysate of Corn Germ Meal. J. Food Process. Preservation 43 (10), e14160. doi:10.1111/jfpp.14160
Keywords: enzyme extraction, fermentation, health benefits, bioactivities, agroindustrial
Citation: Lemes AC, Egea MB, Oliveira Filho JGd, Gautério GV, Ribeiro BD and Coelho MAZ (2022) Biological Approaches for Extraction of Bioactive Compounds From Agro-industrial By-products: A Review. Front. Bioeng. Biotechnol. 9:802543. doi: 10.3389/fbioe.2021.802543
Received: 26 October 2021; Accepted: 14 December 2021;
Published: 27 January 2022.
Edited by:
Jun Xia, Huaiyin Normal University, ChinaReviewed by:
Haixia Chen, Tianjin University, ChinaAmir Mahboubi Soufiani, University of Boras, Sweden
Copyright © 2022 Lemes, Egea, Oliveira Filho, Gautério, Ribeiro and Coelho. This is an open-access article distributed under the terms of the Creative Commons Attribution License (CC BY). The use, distribution or reproduction in other forums is permitted, provided the original author(s) and the copyright owner(s) are credited and that the original publication in this journal is cited, in accordance with accepted academic practice. No use, distribution or reproduction is permitted which does not comply with these terms.
*Correspondence: Ailton Cesar Lemes, YWlsdG9ubGVtZXNAZXEudWZyai5icg==; Maria Alice Zarur Coelho, YWxpY2VAZXEudWZyai5icg==